- 1D'Or Institute for Research and Education, Rio de Janeiro, Brazil
- 2Evandro Chagas National Institute of Infectious Disease, Oswaldo Cruz Foundation, Rio de Janeiro, Brazil
- 3Hospital São Luiz Jabaquara, São Paulo, Brazil
- 4Santa Casa de São Paulo, São Paulo, Brazil
- 5Duke Clinical Research Institute, Duke University Medical Center, Durham, NC, United States
- 6Brazilian Clinical Research Institute, São Paulo, Brazil
- 7Institute of Biophysics Carlos Chagas Filho, Federal University of Rio de Janeiro, Rio de Janeiro, Brazil
- 8Hospital São Luiz Anália Franco, São Paulo, Brazil
- 9Hospital São Luiz São Caetano, São Caetano do Sul, Brazil
- 10Instituto de Microbiologia Paulo de Góes, Federal University of Rio de Janeiro, Rio de Janeiro, Brazil
- 11Cardiology Department, Rio de Janeiro State University, Rio de Janeiro, Brazil
- 12Hospital Sino Brasileiro, Osasco, Brazil
- 13Hospital Villa Lobos, São Paulo, Brazil
- 14Hospital São Luiz Morumbi, São Paulo, Brazil
- 15Hospital Copa Star, Rio de Janeiro, Brazil
- 16Institute for Studies in Public Health—IESC, Federal University of Rio de Janeiro, Rio de Janeiro, Brazil
- 17National Center for Structural Biology and Bioimaging, Federal University of Rio de Janeiro, Rio de Janeiro, Brazil
Background: Cardiovascular comorbidities such as hypertension and inflammatory response dysregulation are associated with worse COVID-19 prognoses. Different cytokines have been proposed to play vital pathophysiological roles in COVID-19 progression, but appropriate prognostic biomarkers remain lacking. We hypothesized that the combination of immunological and clinical variables at admission could predict the clinical progression of COVID-19 in hypertensive patients.
Methods: The levels of biomarkers, including C-reactive protein, lymphocytes, monocytes, and a panel of 29 cytokines, were measured in blood samples from 167 hypertensive patients included in the BRACE-CORONA trial. The primary outcome was the highest score during hospitalization on the modified WHO Ordinal Scale for Clinical Improvement. The probability of progression to severe disease was estimated using a logistic regression model that included clinical variables and biomarkers associated significantly with the primary outcome.
Results: During hospitalization, 13 (7.8%) patients showed progression to more severe forms of COVID-19, including three deaths. Obesity, diabetes, oxygen saturation, lung involvement on computed tomography examination, the C-reactive protein level, levels of 15 cytokines, and lymphopenia on admission were associated with progression to severe COVID-19. Elevated levels of interleukin-10 and interleukin-12 (p70) combined with two or three of the abovementioned clinical comorbidities were associated strongly with progression to severe COVID-19. The risk of progression to severe disease reached 97.5% in the presence of the five variables included in our model.
Conclusions: This study demonstrated that interleukin-10 and interleukin-12 (p70) levels, in combination with clinical variables, at hospital admission are key biomarkers associated with an increased risk of disease progression in hypertensive patients with COVID-19.
Introduction
COVID-19 may evolve to severe viral pneumonia and acute respiratory distress syndrome with a high mortality rate. Importantly, patients with cardiac comorbidities have been found in various studies to be at greater risk of severe disease (1–6). In addition, patients with cardiovascular disease are more prone to myocardial injury development after SARS-CoV-2 infection (7–9).
The pathophysiological mechanisms related to these increased risks in patients with cardiac comorbidities are not completely understood. Concern has been raised about the use of angiotensin-converting enzyme inhibitors (ACEIs) and angiotensin receptor blockers (ARBs) in hypertensive patients, as preclinical studies have suggested that renin-angiotensin-aldosterone system inhibitors increase the expression of angiotensin-converting enzyme 2, the functional SARS-CoV-2 receptor (10–12). A recent randomized trial from our group (the BRACE-CORONA trial), in which 659 hypertensive patients were included, demonstrated that the discontinuation of ACEIs and ARBs for 30 days does not impact the number of days over a 30-day follow-up period that patients hospitalized with mild to moderate COVID-19 remain alive and out of the hospital (13, 14).
In addition to cardiac risk factors, several studies have suggested the occurrence of a dysregulated inflammatory response, characterized by the simultaneous release of pro- and anti-inflammatory mediators, known as a cytokine storm and established as a key factor in the physiopathology and clinical progression of COVID-19 in a subset of patients (15, 16). An exacerbated immune response is well-accepted to potentially strongly impair cardiac function (17–20). Several cytokines have been proposed to be potential biomarkers of COVID-19 severity (21–24); interferon gamma–induced protein 10 (IP-10), interleukin (IL)-6, and IL-10 have been associated consistently with greater severity of this disease (25–28).
The uncertainty and variability of the innate immune response, associated with an unpredictable disease course ranging from mild to fatal, highlights the need to identify prognostic factors related to a greater risk of progression to severe disease, particularly in more susceptible patients with comorbidities such as hypertension. To our knowledge, however, no data have been provided about biomarkers that could allow clinicians to identify, in the first 48 h after hospital admission, hypertensive patients at increased risk of disease progression, thereby helping them to choose the best therapeutic option.
This study was conducted to test the hypothesis that the immunological profiles of hypertensive patients upon admission to hospital with COVID-19 provide additional information about disease severity and progression. The analysis of cellular components, such as lymphocytes and monocytes, and the quantification of cytokine concentrations were performed to identify potential biomarkers.
Materials and Methods
Population and Design
Patients included in this study were from the BRACE-CORONA trial (14), an academically led, investigator-initiated phase IV multicenter open-label registry-based randomized trial involving 659 patients on ACEIs/ARBs with confirmed COVID-19 diagnoses at 29 centers in Brazil. The present study was conducted with blood samples from 167 hospitalized hypertensive patients enrolled consecutively in the trial at six centers in the state of São Paulo, Brazil. The samples were collected within 24 h of COVID-19 diagnosis confirmation between 21 May and 27 June 2020. The trial protocol (13) was approved by the Brazilian Ministry of Health National Commission for Research Ethics and by institutional review boards or ethics committees at participating sites. All patients provided informed consent before enrollment.
Patients eligible for the BRACE-CORONA trial were aged ≥18 years and chronic ACEI/ARB users. Patients with clinical indications for ACEI/ARB treatment termination, such as hypotension, acute kidney injury, and/or shock, were excluded. Patients on mechanical ventilation and those with hemodynamic instability, acute renal failure, or shock also were excluded (14). The inclusion and exclusion criteria are provided in full in the Supplementary Data.
Outcomes
The primary outcome was defined as the highest score during hospitalization on the modified WHO Ordinal Scale for Clinical Improvement [range, 0 (no evidence of infection) to 8 (death)]. COVID-19 was classified as non-severe (mild to moderate, scores of 3–5), ranging from the lack of need for oxygen therapy to conditions requiring noninvasive ventilation, and severe (scores of 6–8), including disease necessitating the use of mechanical ventilation, inotropic support, and/or renal replacement therapy, and that causing death (Supplementary Table 1) (29). Secondary outcomes were the lengths of stay (LOSs) in the hospital and intensive care unit (ICU), acute myocardial infarction, new or worsening heart failure, hypertensive crisis, transient ischemic attack, stroke, myocarditis, pericarditis, arrhythmias requiring treatment, and thromboembolic events.
Biomarker Quantification
Blood samples were collected in tubes containing ethylenediaminetetraacetic acid as an anticoagulant and centrifuged immediately for plasma separation. Plasma samples were then frozen and stored at −20°C until analysis. Levels of epidermal growth factor, eotaxin, granulocyte colony-stimulating factor (G-CSF), granulocyte-macrophage colony-stimulating factor (GM-CSF), interferon (IFN)-α2, IFN-γ, IL-1α, IL-1β, IL-1ra, IL-2–8, IL-10, IL-12 (p40), IL-12 (p70), IL-13, IL-15, IL-17A, IP-10, monocyte chemoattractant protein-1 (MCP-1), macrophage inflammatory protein (MIP)-1α, MIP-1β, tumor necrosis factor (TNF)-α, TNF-β, and vascular endothelial growth factor in undiluted samples were measured using the MILLIPLEX MAP human cytokine/chemokine magnetic bead panel (HCYTMAG-60K-PX29; Merck Millipore, Billerica, MA, USA) according to the manufacturer's instructions. The assay plates were read immediately and analyzed in a MAGPIX® system (Merck Millipore). All samples and standards were measured in duplicate. Lymphocyte and monocyte quantification was performed in an automized Horiba ABX Micros 60 system (Horiba Medical, Montpellier, France) using photometry. C-reactive protein (CRP) was measured by latex-enhanced immunoturbidimetric assay. Cytokines not detected in >50% of the patient samples were excluded from further analyses.
Statistical Analysis
Continuous variables were described as medians, means, and standard deviations; categorical variables were characterized by proportions. For the primary outcome, 95% confidence intervals (CIs) were calculated. Fisher's exact test was used to detect statistical associations between the outcome and categorical clinical variables. For continuous variables, receiver operating characteristic (ROC) curves were used to discriminate between severe and non-severe cases, and those associated statistically with the primary outcome were dichotomized using cutoff points of 90% sensitivity. P ≤ 0.05 was used to define significance and for automatic forward stepwise selection of clinical variables for inclusion in a binary logistic regression model. The significance levels for entry and removal of variables selected by the automatic regression model were defined at 5 and 10%, respectively. The beta coefficients and odd ratios were calculated for all variables in each step of the model to quantify the association with the outcome. The goodness of fit for the final model was evaluated by the Hosmer–Lemeshow test and by ROC curve. Predicted probabilities for the primary outcome were estimated using variables showing significant associations in the final model. All analysis were performed using SPSS software (version 24.0; IBM Corporation, Armonk, NY, USA).
Results
Of the 167 hypertensive patients, 13.8% were using ACEIs and 86.2% were using ARBs. The mean patient age was 54.1 ± 12.3 years; 57 (34.1%) patients were female, 88 (52.7%) were obese, 41 (24.6%) had diabetes, and 29 (17.4%) had dyslipidemia. Coronary artery disease and chronic pulmonary disease were present in 2.4% of the cases each, and 2.4% of the patients were smokers. Data on all comorbidities are provided in Supplementary Figure 1.
Cough (62.3%), fever (57.5%), myalgia (46.7%), shortness of breath (44.9%), and fatigue (44.9%) were the most common symptoms at presentation (Supplementary Figure 2). The mean interval from symptom onset to hospital presentation was 5 ± 3.1 days, and 20.4% of patients had ≤ 93% baseline oxygen saturation. All patients included in this study had non-severe COVID-19 (WHO scores of 3–5) on admission. On chest computed tomography (CT) examinations, 59.9% of patients showed ≤ 25% lung involvement, 35.3% showed 26–50% involvement, and 4.8% showed >50% lung involvement. Thirty-six (21.6%) cases presented criteria for significant pulmonary involvement (oxygen saturation ≤ 93% and/or >50% lung involvement on CT) at admission (Supplementary Table 2).
Primary Outcome
Worst WHO clinical improvement scores during hospitalization were 3 (mild disease) in 81 (48.5%; 95% CI, 41.0–56.1%) cases, 4 or 5 (moderate disease) in 73 (43.7%; 95% CI, 36.3–51.3%) cases, and 6–8 (severe disease) in 13 (7.8%; 95% CI, 4.4–12.6) cases (Supplementary Table 3). Progression to severe disease was associated with obesity (p = 0.003), diabetes (p < 0.001), and oxygen saturation (p = 0.001) and lung involvement (p = 0.001) on admission, but not with age or sex (Table 1).
Secondary Outcomes
The mean hospital LOS was 9.1 ± 6.9 days. In total, 119 patients were admitted to the ICU; the mean ICU LOS was 7.6 ± 6.8 days (Supplementary Table 4). According to the report on the BRACE-CORONA trial (13), the mean numbers of days alive and out of hospital did not differ among patients hospitalized with mild to moderate COVID-19 according to ACEI/ARB discontinuation or continuation.
At least one complication occurred during hospitalization in 29 (17.4%) patients. The number of complications per patient ranged from one to nine. The most common complication was acute renal injury [n = 13 (7.8%); Figure 1]. The criteria used for the identification of these complications have been provided in the BRACE-CORONA trial (13). The most commonly administered treatments were antibiotics (98.2%), anticoagulants (68.3%), and corticosteroids (59.9%; Supplementary Figure 3).
Biomarkers
Blood samples were collected a mean of 2.8 days after hospitalization. Levels of IL-10, IP-10, G-CSF, IFN- α2, IL-1ra, IL-15, IL-1α, IL-12 (p70), IL-2, IL-17A, GM-CSF, IL-8, IL-6, MCP-1, and CRP were higher in patients with severe than in those with non-severe disease. In contrast, levels of lymphocytes and MIP-1β were lower in patients with severe than in those with non-severe disease (Table 2). IL-3 and IL-4 were not detected in >50% of patients and were excluded from further analyses.
Fifteen cytokines were found to be useful for the prediction of progression to severe COVID-19 [areas under the ROC curve (AUCs), 0.667–0.836]. Increased levels of 14 cytokines and decreased levels of MIP-1β were associated with COVID-19 severity. AUCs for IL-10, IP-10, G-CSF, IFN- α2, IL-1ra, and IL-15 were ≥0.75 (Table 3). Increased CRP levels and reduced lymphocyte counts were also associated with disease severity (AUCs, 0.825 and 0.742, respectively; Supplementary Figure 4).
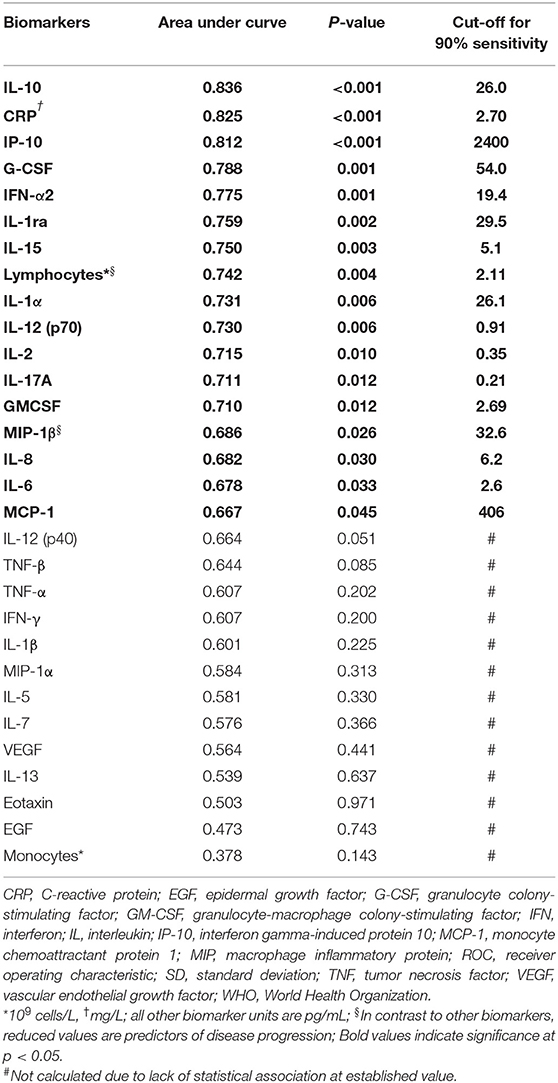
Table 3. Distinction of severe (modified WHO score 6–8) and non-severe (modified WHO score 3–5) cases by areas under ROC curves.
Predictive Model
The initial model for the prediction of the risk of progression of COVID-19 included diabetes, obesity, hypoxemia, lung involvement on CT, the CRP level, the lymphocyte count, and levels of 15 cytokines. Five variables were selected automatically in a forward stepwise manner: the IL-10 level (>26.0 pg/mL), diabetes, the IL-12 (p70) level (>0.91 pg/mL), obesity, and significant lung involvement on admission (oxygen saturation ≤ 93% or >50% lung involvement on CT). The IL-10 level was associated strongly with disease severity [odds ratio (OR) = 32]. The ORs for the other four variables also showed associations with progression to severe disease (Table 4), and the predictive value of the model increased strongly with the addition of these variables (OR = 78.3). The ROC curve for the predictive model showed a very high discriminatory power between the two groups with an AUC of 0.981 (Supplementary Figure 5).
In the presence of two or three clinical comorbidities, the predictive capability of these biomarkers increased markedly (Figure 2). In patients with diabetes and obesity, for example, the likelihood of disease progression increased from 0.1% with low IL-10 and IL-12 (p70) levels to >80% with levels of these cytokines exceeding the 90% sensitivity thresholds. Similarly, the risk of progression to severe disease in the presence of three clinical comorbidities was 1.0% with IL-10 and IL-12 (p70) levels below the thresholds and 97.5% with levels exceeding the thresholds (Table 5).
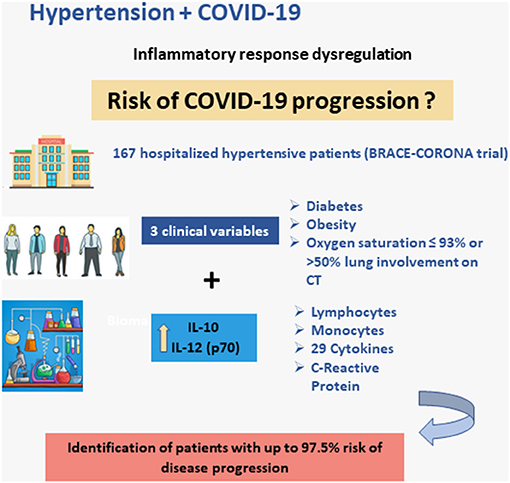
Figure 2. Schematic illustration of the main findings: model for the prediction of the risk of progression of COVID-19 including three clinical variables and two biomarkers: IL-10 and IL-12 (p70).
Discussion
In this study, we analyzed immune response patterns, including levels of 29 cytokines, CRP, monocytes, and lymphocytes, in a large sample (n = 167) of hospitalized hypertensive patients from the BRACE-CORONA trial (13). In univariate analysis, progression to severe COVID-19 was associated with clinical factors (diabetes, obesity, and lung involvement on admission) and levels of biomarkers, including 15 cytokines, CRP, and lymphocytes. We propose a logistic regression model that includes clinical variables (diabetes, obesity, and significant lung involvement) and critical biomarkers [IL-10 and IL-12 (p70)]. This combined use of clinical risk factors and biomarkers for the prediction of COVID-19 severity at admission is novel.
Clinical comorbidities, particularly diabetes, hypertension, and other cardiovascular diseases, have been associated with COVID-19 severity, as they are more prevalent in non-survivors and patients requiring ICU care (1, 30–32). However, the mechanisms involved in the increased risk of COVID-19 in these patients are not understood completely. Infections are more prevalent and have more complicated courses in patients with diabetes, possibly due to disturbances in humoral and cellular immunity and exaggerated pro-inflammatory cytokine responses (33, 34). In addition, obesity has been associated with ICU admission and mortality in patients with COVID-19, which may be related to the presence of angiotensin-converting enzyme 2 receptors in adipose tissue, elevated pro-inflammatory cytokine levels, increased susceptibility to infection by various pathogens (35), and pro-coagulant profiles (36). Moreover, the extent of CT lung involvement has been correlated with COVID-19 severity, and severity scores for chest CT findings have been proposed to enable the differentiation of clinical forms and prediction of clinical outcomes (37, 38). The lack of association between age and the outcome in the present study may be related to the relative young mean age of our sample, due to the exclusion of patients with severe disease in the first 24 h after admission.
In this study, admission levels of 17 biomarkers (increased levels of CRP and 14 cytokines and reduced levels of MIP-1β and lymphocytes) were associated significantly with progression to severe disease. Our biomarker findings are similar to previously reported associations of the levels of several cytokines (e.g., IL-1ra, IL-2, IL-6, IL-8, IL-10, and IP-10) with COVID-19 severity and mortality (25, 27, 39–41). The association of the IL-10 level with COVID-19 progression to severity has been reported in a considerable number of publications (40–42). IL-10 is an immunoregulatory cytokine with the main functions of limiting inflammatory responses and regulating immune cell differentiation and proliferation (43). Information about the role of IL-12 (p70) in COVID-19 is more limited. Consistent with our findings, higher levels of IL-12 (p70) have been associated with severe COVID-19 (44, 45). IL-12 is a heterodimeric cytokine composed of p35 and p40 subunits that enhances connections between the innate and adaptive immune responses; its expression is induced via a pathogen-associated molecular response when a virus enters a cell (46).
Although the ability of clinical and laboratory variables to independently predict COVID-19 severity has been assessed extensively and predictive models have been proposed, no definitive prognostic biomarker or effective predictive model for the identification, at the time of hospital admission, of patients who will require ICU care, mechanical ventilation, or inotropic support has emerged (47, 48). According to the model we propose, the probability of progression to severe disease in hypertensive patients with obesity and diabetes is 0.1% in the absence of increased IL-10 and IL-12 (p70) levels, but 81.5% with levels of these two cytokines exceeding the 90% sensitivity thresholds. Similarly, in the presence of the three clinical comorbidities (obesity, diabetes, and oxygen saturation ≤ 93% or >50% lung involvement on CT), the probability of progression is 1% with lower IL-10 and IL-12 (p70) levels, but 97.5% with elevated levels of these biomarkers. A practical approach to model application for the estimation of the risk of progression to severe COVID-19 would be to measure IL-10 and IL-12 (p70) levels on admission in hypertensive patients with two or three of the relevant clinical comorbidities.
Limitations
This study has some limitations. Blood samples were collected a mean of 2.8 days after hospitalization (usually within 24 h after confirmation of SARS-CoV-2 infection); with a median 6-day interval between symptom onset and hospital admission, and our population included only hypertensive patients who were taking ACEi or ARBs, which might limit the generalizability of our results. Nevertheless, we believe that the widespread use of these drugs in the hypertensive population associated with the multicentric nature of the study might help to ensure a good external validity. Besides, we were not able to validate our model with a different patient sample. Additional studies are needed to validate the results obtained here in more heterogeneous populations of hypertensive patients and also to evaluate the applicability of the proposed model in non-hypertensive COVID-19 populations.
Conclusion
The measurement of IL-10 and IL-12 (p70) levels on admission may be useful for the identification of hypertensive patients at greater risk of COVID-19 progression, particularly in the presence of classical clinical comorbidities (obesity, diabetes, and extensive lung involvement). We propose a new biomarker-based approach to improve the prediction of COVID-19 progression in hypertensive patients, which may help physicians identify patients at high risk who would benefit from more intensive surveillance and treatment.
Data Availability Statement
The raw data supporting the conclusions of this article will be made available by the authors, without undue reservation.
Ethics Statement
The studies involving human participants were reviewed and approved by Brazilian Ministry of Health's National Commission for Research Ethics (CAAE # 30432020.2.0000.5249). The patients/participants provided their written informed consent to participate in this study.
Author Contributions
RM-B, EM, AS, FB, and RRL: study design. OS, AM, RDL, AF, GA, DA, and MS: patient recruitment, data, and sample collection organization. RM-B and EM: application for the funding and writing-original draft preparation. AM, AF, GA, TP, TF, VL, KG, NO, FD, MK, and RD: patient recruitment and sample collection. NV, LM, MC, and PP-C: biomarker processing and analyses. RDL, RM-B, AM, and RRL: data curation. RRL: statistical analyses. AS, FB, and RDL writing-review and editing. All authors contributed to the article and approved the submitted version.
Funding
This work was supported by intramural grants from the D'Or Institute of Research and Education, the Brazilian Research Institute, the Fundação Carlos Chagas Filho de Amparo à Pesquisa do Estado do Rio de Janeiro (nos. E-26/210.155/2020, E-26/203.169/2017, E-26/210.191/2020, and E-26/210.253/2020), the CNPq (no. 310681/2018-9), CAPES, FINEP, and the Serrapilheira Institute.
Conflict of Interest
RDL reports receiving grant support from Bristol-Myers Squibb, GlaxoSmithKline, Medtronic, Sanofi, and Pfizer, and consulting fees from Bayer, Boehringer Ingelheim, Bristol-Myers Squibb, Daiichi-Sankyo, GlaxoSmithKline, Medtronic, Merck, Pfizer, Sanofi, and Portola. AM reports receiving consulting fees from Pfizer, Bayer, AstraZeneca, Novartis, Daiichi-Sankyo, Zodiac, Roche, and Janssen. AF reports receiving consulting fees from Pfizer, Bayer, Daiichi-Sankyo, Boehringer, and Servier. GA reports receiving consulting fees from Bayer, Pfizer, Servier, AstraZeneca, and Daichii Sankyo. DA reports receiving consulting fees from Boehringer Ingelheim, AstraZeneca, Bayer, and Servier. OS reports receiving grant support from Boehringer Ingelheim and consulting fees from Pfizer, Bayer, Daiichi-Sankyo, and Boehringer Ingelheim.
The remaining authors declare that the research was conducted in the absence of any commercial or financial relationships that could be construed as a potential conflict of interest.
Publisher's Note
All claims expressed in this article are solely those of the authors and do not necessarily represent those of their affiliated organizations, or those of the publisher, the editors and the reviewers. Any product that may be evaluated in this article, or claim that may be made by its manufacturer, is not guaranteed or endorsed by the publisher.
Acknowledgments
We thank all staff and research assistants from: D'Or Institute of Research and Education (IDOR), Brazilian Clinical Research Institute (BCRI) and Rede D'Or Hospitals which took part in this study. In addition, the authors thank all patients for accepting participating in this project.
Supplementary Material
The Supplementary Material for this article can be found online at: https://www.frontiersin.org/articles/10.3389/fcvm.2021.702507/full#supplementary-material
Abbreviations
ACEI, angiotensin-converting enzyme inhibitor; ARB, angiotensin receptor blocker; AUC, area under the receiver operating characteristic curve; CI, confidence interval; CRP, C-reactive protein; CT, computed tomography; G-CSF, granulocyte colony-stimulating factor; GM-CSF, granulocyte-macrophage colony-stimulating factor; ICU, intensive care unit; IFN, interferon; IL, interleukin; IP-10, interferon gamma–induced protein 10; LOS, length of stay; MCP-1, monocyte chemoattractant protein-1; MIP, macrophage inflammatory protein; OR, odds ratio; TNF, tumor necrosis factor; WHO, World Health Organization.
References
1. Clerkin KJ, Fried JA, Raikhelkar J, Sayer G, Griffin JM, Masoumi A, et al. COVID-19 and cardiovascular disease. Circulation. (2020) 141:1648–55. doi: 10.1161/CIRCULATIONAHA.120.046941
2. Mehra MR, Desai SS, Kuy S, Henry TD, Patel AN. Cardiovascular disease, drug therapy, and mortality in Covid-19. N Engl J Med. (2020) 382:e102. doi: 10.1056/NEJMoa2007621
3. Wang D, Hu B, Hu C, Zhu F, Liu X, Zhang J, et al. Clinical characteristics of 138 hospitalized patients with 2019 novel coronavirus-infected pneumonia in Wuhan, China. JAMA. (2020) 323:1061–69. doi: 10.1001/jama.2020.1585
4. Zheng YY, Ma YT, Zhang JY, Xie X. COVID-19 and the cardiovascular system. Nat Rev Cardiol. (2020) 17:259–60. doi: 10.1038/s41569-020-0360-5
5. Zhou F, Yu T, Du R, Fan G, Liu Y, Liu Z, et al. Clinical course and risk factors for mortality of adult inpatients with COVID-19 in Wuhan, China: a retrospective cohort study. Lancet. (2020) 395:1054–62. doi: 10.1016/S0140-6736(20)30566-3
6. Wu C, Chen X, Cai Y, Xia J, Zhou X, Xu S, et al. Risk factors associated with acute respiratory distress syndrome and death in patients with coronavirus disease 2019 pneumonia in Wuhan, China. JAMA Intern Med. (2020) 180:934–43. doi: 10.1001/jamainternmed.2020.0994
7. Guo T, Fan Y, Chen M, Wu X, Zhang L, He T, et al. Cardiovascular implications of fatal outcomes of patients with coronavirus disease 2019 (COVID-19). JAMA Cardiol. (2020) 5:811–8. doi: 10.1001/jamacardio.2020.1017
8. Liu PP, Blet A, Smyth D, Li H. The science underlying COVID-19: implications for the cardiovascular system. Circulation. (2020) 142:68–78. doi: 10.1161/CIRCULATIONAHA.120.047549
9. Nguyen LS, Dolladille C, Drici M-D, Fenioux C, Alexandre J, Mira J-P, et al. Cardiovascular toxicities associated with hydroxychloroquine and azithromycin: an analysis of the World Health Organization Pharmacovigilance Database. Circulation. (2020) 142:303–5. doi: 10.1161/CIRCULATIONAHA.120.048238
10. Vaduganathan M, Vardeny O, Michel T, McMurray JJ, Pfeffer MA, Solomon SD. Renin-angiotensin-aldosterone system inhibitors in patients with Covid-19. N Engl J Med. (2020) 382:1653–9. doi: 10.1056/NEJMsr2005760
11. Gheblawi M, Wang K, Viveiros A, Nguyen Q, Zhong J-C, Turner AJ, et al. Angiotensin-converting enzyme 2: SARS-CoV-2 receptor and regulator of the renin-angiotensin system: celebrating the 20th anniversary of the discovery of ACE2. Circ Res. (2020) 126:1456–74. doi: 10.1161/CIRCRESAHA.120.317015
12. Reynolds HR, Adhikari S, Pulgarin C, Troxel AB, Iturrate E, Johnson SB, et al. Renin-angiotensin-aldosterone system inhibitors and risk of Covid-19. N Engl J Med. (2020) 382:2441–8. doi: 10.1056/NEJMoa2008975
13. Lopes RD, Macedo AVS, de Barros ESPGM, Moll-Bernardes RJ, Dos Santos TM, Mazza L, et al. Effect of discontinuing vs continuing angiotensin-converting enzyme inhibitors and angiotensin II receptor blockers on days alive and out of the hospital in patients admitted with COVID-19: a randomized clinical trial. JAMA. (2021) 325:254–64. doi: 10.1001/jama.2020.25864
14. Lopes RD, Macedo AVS, de Barros ESPGM, Moll-Bernardes RJ, Feldman A, D'Andrea Saba Arruda G, et al. Continuing versus suspending angiotensin-converting enzyme inhibitors and angiotensin receptor blockers: Impact on adverse outcomes in hospitalized patients with severe acute respiratory syndrome coronavirus 2 (SARS-CoV-2)–The BRACE CORONA Trial. Am Heart J. (2020) 226:49–59. doi: 10.1016/j.ahj.2020.05.002
15. Mehta P, McAuley DF, Brown M, Sanchez E, Tattersall RS, Manson JJ. COVID-19: consider cytokine storm syndromes and immunosuppression. Lancet. (2020) 395:1033–4. doi: 10.1016/S0140-6736(20)30628-0
16. Lowenstein CJ, Solomon SD. Severe COVID-19 is a microvascular disease. Circulation. (2020) 142:1609–11. doi: 10.1161/CIRCULATIONAHA.120.050354
17. Monnerat G, Alarcón ML, Vasconcellos LR, Hochman-Mendez C, Brasil G, Bassani RA, et al. Macrophage-dependent IL-1β production induces cardiac arrhythmias in diabetic mice. Nat Commun. (2016) 7:1–15. doi: 10.1038/ncomms13344
18. Alarcon MML, Trentin-Sonoda M, Panico K, Schleier Y, Duque T, Moreno-Loaiza O, et al. Cardiac arrhythmias after renal I/R depend on IL-1β. J Mol Cell Cardiol. (2019) 131:101–11. doi: 10.1016/j.yjmcc.2019.04.025
19. Belhadjer Z, Méot M, Bajolle F, Khraiche D, Legendre A, Abakka S, et al. Acute heart failure in multisystem inflammatory syndrome in children in the context of global SARS-CoV-2 pandemic. Circulation. (2020) 142:429–36. doi: 10.1161/CIRCULATIONAHA.120.048360
20. Lazzerini PE, Boutjdir M, Capecchi PL. COVID-19, arrhythmic risk, and inflammation: mind the gap! Circulation. (2020) 142:7–9. doi: 10.1161/CIRCULATIONAHA.120.047293
21. Wan S, Yi Q, Fan S, Lv J, Zhang X, Guo L, et al. Relationships among lymphocyte subsets, cytokines, and the pulmonary inflammation index in coronavirus (COVID-19) infected patients. Br J Haematol. (2020) 189:428–37. doi: 10.1111/bjh.16659
22. Costela-Ruiz VJ, Illescas-Montes R, Puerta-Puerta JM, Ruiz C, Melguizo-Rodriguez L. SARS-CoV-2 infection: the role of cytokines in COVID-19 disease. Cytokine Growth Factor Rev. (2020) 54:62–75. doi: 10.1016/j.cytogfr.2020.06.001
23. Conti P, Ronconi G, Caraffa A, Gallenga CE, Ross R, Frydas I, Kritas SK. Induction of pro-inflammatory cytokines (IL-1 and IL-6) and lung inflammation by Coronavirus-19 (COVI-19 or SARS-CoV-2): anti-inflammatory strategies. J Biol Regul Homeost Agents. (2020) 34:327–31. doi: 10.23812/CONTI-E
24. Han H, Ma Q, Li C, Liu R, Zhao L, Wang W, et al. Profiling serum cytokines in COVID-19 patients reveals IL-6 and IL-10 are disease severity predictors. Emerg Microbes Infect. (2020) 9:1123–30. doi: 10.1080/22221751.2020.1770129
25. Yang Y, Shen C, Li J, Yuan J, Wei J, Huang F, et al. Plasma IP-10 and MCP-3 levels are highly associated with disease severity and predict the progression of COVID-19. J Allergy Clin Immunol. (2020) 146:119–27.e114. doi: 10.1016/j.jaci.2020.04.027
26. Chen G, Wu D, Guo W, Cao Y, Huang D, Wang H, et al. Clinical and immunological features of severe and moderate coronavirus disease 2019. J Clin Invest. (2020) 130:2620–9. doi: 10.1172/JCI137244
27. Sun HB, Zhang YM, Huang LG, Lai QN, Mo Q, Ye XZ, et al. The changes of the peripheral CD4+ lymphocytes and inflammatory cytokines in Patients with COVID-19. PLoS One. (2020) 15:e0239532. doi: 10.1371/journal.pone.0239532
28. Dhar SK, K V, Damodar S, Gujar S, Das M. IL-6 and IL-10 as predictors of disease severity in COVID-19 patients: results from meta-analysis and regression. Heliyon. (2021) 7:e06155. doi: 10.1016/j.heliyon.2021.e06155
29. World Health Organization. WHO R&D Blueprint: COVID-19 Therapeutic Trial Synopsis. (2020). Available online at: https://www.who.int/publications/i/item/covid-19-therapeutic-trial-synopsis (accessed October 20, 2020).
30. Iaccarino G, Grassi G, Borghi C, Ferri C, Salvetti M, Volpe M. Age and multimorbidity predict death among COVID-19 patients: results of the SARS-RAS study of the Italian Society of Hypertension. Hypertension. (2020) 76:366–72. doi: 10.1161/HYPERTENSIONAHA.120.15324
31. Semenzato L, Botton J, Drouin J, Baricault B, Vabre C, Cuenot F, et al. Antihypertensive drugs and COVID-19 risk: a cohort study of 2 million hypertensive patients. Hypertension. (2021) 77:833–42. doi: 10.1161/HYPERTENSIONAHA.120.16314
32. Silverio A, Di Maio M, Citro R, Esposito L, Iuliano G, Bellino M, et al. Cardiovascular risk factors and mortality in hospitalized patients with COVID-19: systematic review and meta-analysis of 45 studies and 18,300 patients. BMC Cardiovasc Disord. (2021) 21:23. doi: 10.1186/s12872-020-01816-3
33. Geerlings SE, Hoepelman AI. Immune dysfunction in patients with diabetes mellitus (DM). FEMS Immunol Med Microbiol. (1999) 26:259–65. doi: 10.1111/j.1574-695X.1999.tb01397.x
34. Pal R, Bhansali A. COVID-19, diabetes mellitus and ACE2: the conundrum. Diabetes Res Clin Pract. (2020) 162:108132. doi: 10.1016/j.diabres.2020.108132
35. Dicker D, Bettini S, Farpour-Lambert N, Frühbeck G, Golan R, Goossens G, et al. Obesity and COVID-19: the two sides of the coin. Obes Facts. (2020) 13:430–8. doi: 10.1159/000510005
36. Sattar N, McInnes IB, McMurray JJ. Obesity is a risk factor for severe COVID-19 infection: multiple potential mechanisms. Circulation. (2020) 142:4–6. doi: 10.1161/CIRCULATIONAHA.120.047659
37. Yang R, Li X, Liu H, Zhen Y, Zhang X, Xiong Q, et al. Chest CT severity score: an imaging tool for assessing severe COVID-19. Radiology. (2020) 2:e200047. doi: 10.1148/ryct.2020200047
38. Francone M, Iafrate F, Masci GM, Coco S, Cilia F, Manganaro L, et al. Chest CT score in COVID-19 patients: correlation with disease severity and short-term prognosis. Eur Radiol. (2020) 30:6808–17. doi: 10.1007/s00330-020-07033-y
39. Jin M, Shi N, Wang M, Shi C, Lu S, Chang Q, et al. CD45: a critical regulator in immune cells to predict severe and non-severe COVID-19 patients. Aging (Albany NY). (2020) 12:19867. doi: 10.18632/aging.103941
40. Varchetta S, Mele D, Oliviero B, Mantovani S, Ludovisi S, Cerino A, et al. Unique immunological profile in patients with COVID-19. Cell Mol Immunol. (2020) 18:1–11. doi: 10.21203/rs.3.rs-23953/v1
41. Li Q, Xu W, Li W, Huang C, Chen L. Dynamics of cytokines and lymphocyte subsets associated with the poor prognosis of severe COVID-19. Eur Rev Med Pharmacol Sci. (2020) 24:12536–44. doi: 10.26355/eurrev_202012_24051
42. Liu QQ, Cheng A, Wang Y, Li H, Hu L, Zhao X, et al. Cytokines and their relationship with the severity and prognosis of coronavirus disease 2019 (COVID-19): a retrospective cohort study. BMJ Open. (2020) 10:e041471. doi: 10.1136/bmjopen-2020-041471
43. Asadullah K, Sterry W, Volk H. Interleukin-10 therapy-review of a new approach. Pharmacol Rev. (2003) 55:241–69. doi: 10.1124/pr.55.2.4
44. Young BE, Ong SWX, Ng LFP, Anderson DE, Chia WN, Chia PY, et al. Viral dynamics and immune correlates of COVID-19 disease severity. Clin Infect Dis. (2020). doi: 10.1093/cid/ciaa1280. [Epub ahead of print].
45. Liu Y, Chen D, Hou J, Li H, Cao D, Guo M, et al. An inter-correlated cytokine network identified at the center of cytokine storm predicted COVID-19 prognosis. Cytokine. (2021) 138:155365. doi: 10.1016/j.cyto.2020.155365
46. Guo Y, Cao W, Zhu Y. Immunoregulatory functions of the IL-12 family of cytokines in antiviral systems. Viruses. (2019) 11:772. doi: 10.3390/v11090772
47. Wynants L, Van Calster B, Collins GS, Riley RD, Heinze G, Schuit E, et al. Prediction models for diagnosis and prognosis of covid-19: systematic review and critical appraisal. BMJ. (2020) 369:m1328. doi: 10.1136/bmj.m1328
Keywords: hypertension, cytokine, COVID-19, biomarker, inflammation, prognosis
Citation: Moll-Bernardes R, Sousa AS, Macedo AVS, Lopes RD, Vera N, Maia LCR, Feldman A, Arruda GDAS, Castro MJC, Pimentel-Coelho PM, Albuquerque DC, de Paula TC, Furquim TAB, Loures VA, Giusti KGD, Oliveira NM, De Luca FA, Kotsugai MDM, Domiciano RAM, Santos MF, Souza OF, Bozza FA, Luiz RR and Medei E (2021) IL-10 and IL-12 (P70) Levels Predict the Risk of Covid-19 Progression in Hypertensive Patients: Insights From the BRACE-CORONA Trial. Front. Cardiovasc. Med. 8:702507. doi: 10.3389/fcvm.2021.702507
Received: 29 April 2021; Accepted: 02 July 2021;
Published: 27 July 2021.
Edited by:
Masanori Aikawa, Brigham and Women's Hospital and Harvard Medical School, United StatesReviewed by:
Angelo Silverio, University of Salerno, ItalyValeria Visco, University of Salerno, Italy
Copyright © 2021 Moll-Bernardes, de Sousa, Macedo, Lopes, Vera, Maia, Feldman, Arruda, Castro, Pimentel-Coelho, de Albuquerque, de Paula, Furquim, Loures, Giusti, de Oliveira, De Luca, Kotsugai, Domiciano, Santos, de Souza, Bozza, Luiz and Medei. This is an open-access article distributed under the terms of the Creative Commons Attribution License (CC BY). The use, distribution or reproduction in other forums is permitted, provided the original author(s) and the copyright owner(s) are credited and that the original publication in this journal is cited, in accordance with accepted academic practice. No use, distribution or reproduction is permitted which does not comply with these terms.
*Correspondence: Emiliano Medei, ZW1lZGVpNzBAYmlvZi51ZnJqLmJy
†These authors have contributed equally to this work and share last authorship