- 1Guang'an Men Hospital, China Academy of Chinese Medical Sciences, Beijing, China
- 2National Clinical Research Center for Chinese Medicine Cardiology, Xiyuan Hospital, China Academy of Chinese Medical Sciences, Beijing, China
- 3Graduate School, Beijing University of Chinese Medicine, Beijing, China
With improvement in living standards and average life expectancy, atherosclerotic cardiovascular disease incidences and mortality have been increasing annually. Paeonia suffruticosa, a natural herb, has been used for the treatment of atherosclerotic cardiovascular disease for thousands of years in Eastern countries. Paeonol is an active ingredient extracted from Paeonia suffruticosa. Previous studies have extensively explored the clinical benefits of paeonol. However, comprehensive reviews on the cardiovascular protective effects of paeonol have not been conducted. The current review summarizes studies reporting on the protective effects of paeonol on the cardiovascular system. This study includes studies published in the last 10 years. The biological characteristics of Paeonia suffruticosa, pharmacological mechanisms of paeonol, and its toxicological and pharmacokinetic characteristics were explored. The findings of this study show that paeonol confers protection against atherosclerotic cardiovascular disease through various mechanisms, including inflammation, platelet aggregation, lipid metabolism, mitochondria damage, endoplasmic reticulum stress, autophagy, and non-coding RNA. Further studies should be conducted to elucidate the cardiovascular benefits of paeonol.
Introduction
Atherosclerotic cardiovascular disease poses a significant health threat in many countries. Advances in medical technology have led to the discovery of drugs and interventional therapies for atherosclerotic cardiovascular disease (1). However, available drugs are associated with various adverse effects, including high tolerance (2, 3), rhabdomyolysis (4), and restenosis (5). It has been shown that natural compounds may confer protection against atherosclerotic cardiovascular disease through various mechanisms (6, 7).
Cortex Moutan, the root bark of Paeonia suffruticosa, has been widely used in traditional Chinese medicine to prevent diabetes (8, 9), arthritis (10), and cancer (11). Paeonol is a biologically active ingredient that is extracted from Cortex Moutan. In Asian countries, paeonol is commonly used in combination therapy for the management of the atherosclerotic cardiovascular disease (12). This review aims at elucidating the cardiovascular protective effects and pharmacological mechanisms of paeonol.
Biological Characteristics of Paeonia suffruticosa
Nomenclature of Paeonia suffruticosa
Paeonia suffruticosa, belonging to the Paeoniaceae family, is an ornamental plant that is widely distributed around the globe. Cortex Moutan, the root bark of Paeonia suffruticosa, has been used for nearly 2,000 years as a natural medicine for alleviating pathogenic heat from the blood, activation of blood circulation, and elimination of stasis (13, 14). Details on characteristics of Paeonia suffruticosa and Cortex Moutan are presented in Figure 1.
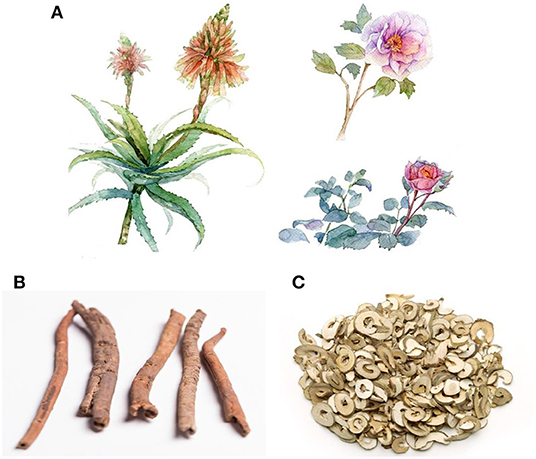
Figure 1. Whole plant and root bark of Paeonia suffruticosa. (A) Paeonia suffruticosa is a kind of plant with ornamental and medicinal values. (B) Cortex Moutan, the root bark of Paeonia suffruticosa, is a natural medicine with a variety of biological effects. (C) prepared slices of Cortex Moutan, containing various bioactive pharmacological compounds.
Botanical Characteristics
Peonies predominantly grow in temperate Eurasia, northwest Africa, and western North America. However, wild peony is only found in China, where it was first domesticated (15). Perennial shrubs grow to 1.5 m in height with brown-gray stems. Its leaflets are long ovate or ovoids. Its flowers are solitary, single or double, with an irregular apex. The root system is well-developed, the root bark is yellow to brown, while the fleshy center is lignified (13, 16, 17). The bark is tubular or semi-tubular, with cracks along the longitudinal section. In addition, the bark is slightly curled inward, 3–8 cm long, 0.5–1.2 cm in diameter, and 0.1–0.4 cm thick. The outer skin is grayish-brown or reddish-brown. The cork drop is pink with protruding lenticels while the inner surface is light brown or gray-yellow with a fine longitudinal texture and shiny crystals. It has a special aroma and a slightly bitter taste (18).
Paeonol: The Main Therapeutic Ingredient of Paeonia suffruticosa
Paeonia suffruticosa contains various bioactive constituents, including monoterpene glycosides, flavonoids, gallic derivatives, and triterpenoids, especially phenols (19–22). The main active ingredients include paeonol, paeoniflorin, gallic acid, and 1,2,3,4,6-pentakis-O-galloyl-β-D-glucose, and the chemical structures are presented in Figure 2. The extraction methods of active ingredients include organic solvent extraction, ultrasonic-assisted extraction, steam distillation, and CO2 supercritical fluid extraction (23–25).
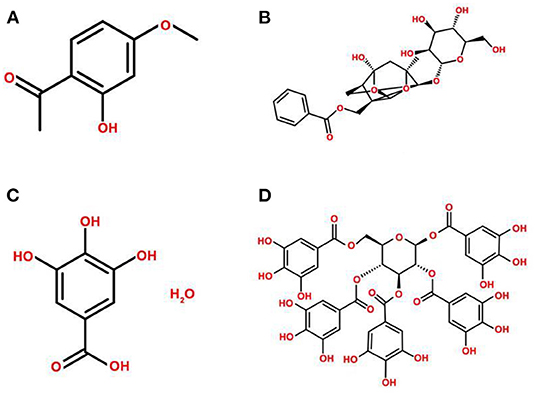
Figure 2. Chemical structures of the main active ingredients of Paeonia suffruticosa. (A) paeonol, (B) paeoniflorin, (C) gallic acid, (D) 1,2,3,4,6-pentakis-O-galloyl-β-D-glucose.
In recent years, paeonol has been widely used in medical health care and as a food supplement (26). Although it has several pharmacological benefits against various diseases, paeonol is mainly used for the treatment of atherosclerotic cardiovascular disease (27), such as myocardial ischemia, myocardial infarction, atherosclerotic stroke, and myocardial ischemia-reperfusion injury (28).
Extraction Methods and Quality Control
The main extraction methods for paeonol include organic solvent extraction, steam distillation, and CO2 supercritical fluid extraction (23, 25). The steam distillation method is relatively simple and has a high transfer rate, while the CO2 supercritical fluid extraction method has the advantages of low temperatures and high efficiency, and is widely used for paeonol extraction. Artificial synthesis can also yield large amounts of paeonol at low costs for industrial production. Methods such as microwave-assisted extraction and ionic liquid extraction have also been widely used in recent years (29, 30).
Based on the provisions of the 2015 edition of Chinese pharmacopeia, the total ash content of the cortex of medicinal materials should not exceed 5.0% (General Rule 2302). Paeonol (C9H10O3) content, as determined by high-performance liquid chromatography (HPLC, General Rule 0512), should not be <1.2%. By determining alcohol-soluble extract (General Rule 2201) under the hot immersion method, using ethanol as a solvent, the extract shall not be <15.0% (31).
Pharmacological Mechanisms of Paeonol on Atherosclerotic Cardiovascular Disease
Inflammation
Inflammatory processes are involved in the pathogenesis of various cardiovascular diseases (32–34). Paeonol exhibits significant anti-inflammatory properties in colitis (35), arthritis (36), neuroinflammation (37), and other conditions. Similarly, paeonol can exert anti-inflammatory effects to inhibit the progression of atherosclerotic cardiovascular disease.
Cytokines are crucial mediators of inflammation. Studies have revealed that paeonol inhibits the formation and development of atherosclerosis by suppressing the release of inflammatory cytokines. In atherosclerosis rabbit models, paeonol intervention decreased atherosclerotic plaques and normalized serum levels of tumor necrosis factor (TNF)-α, interleukin (IL)-1β, and C-reaction protein (CRP) (38). Another study reported that paeonol inhibited lipopolysaccharide (LPS)-induced expression of nitrous oxide (NO), prostaglandin (PG) E2, and IL-6 (39). These findings confirm that paeonol inhibits the release of various inflammatory factors.
Inhibitory effects of paeonol on inflammatory factors are mainly associated with the regulation of various inflammatory pathways (40). Kim et al. reported that paeonol inhibited the migration of human umbilical vein endothelial cells (HUVECs) and the ability to form new blood vessels under the induction of basic fibroblast growth factor (bFGF). The study proved that paeonol inhibited Akt signaling pathways and the activity of matrix metalloproteinases (MMPs), hence suppressing angiogenesis and metastasis (41). Moreover, paeonol suppressed oxidized low-density lipoprotein (ox-LDL)-induced endothelial cell (EC) apoptosis by inhibiting the p38 mitogen-activated protein kinase (MAPK)-nuclear factor-kappa B (NF-κB) signaling pathway (42, 43). Choy et al. evaluated the effects of paeonol on LPS-induced inflammatory damage in HUVECs and C57BL/6J mice. It was found that paeonol suppressed LPS-induced EC dysfunction by independently inhibiting Toll-like receptor (TLR) 4 and bone morphogenetic protein (BMP) 4 signaling pathways (44). Other studies reported that the inhibitory effects of paeonol on inflammation might be related to the regulation of microRNA (miRNA) expression (45, 46). In dog models, paeonol significantly reduced the area of myocardial infarction and the release of myocardial enzymes (47). Similarly, paeonol conferred protection against myocardial infarction in a rat model (48, 49). Zhou et al. used a coronary artery left ventricular branch ligation model to explore the effect of paeonol on ventricular remodeling in rabbits with myocardial infarction. Paeonol treatment improved ventricular function and significantly suppressed TLR4 and TNF-α mRNA expression levels. Paeonol down-regulated the expression of downstream inflammatory factor, TNF-α, by inhibiting the TLR4 signaling pathway to improve ventricular remodeling after myocardial infarction (50). Paeonol was also found to reverse ventricular remodeling after myocardial infarction by inhibiting NF-κB-p65 and down-regulating MMP-9 (51). Studies also suggested that paeonol regulated ventricular remodeling through the transforming growth factor (TGF) -β signaling pathway (52–54). Therefore, inhibitory effects of paeonol on inflammatory factors are mainly associated with the regulation of MAPK, TLR, MMP, and TGF pathways, and may be correlated with the regulation of apoptosis, autophagy, and the transcription of miRNAs.
Monocyte adhesion, induced by the adhesion factors, is an essential step in the pathogenesis of atherosclerosis. Studies have confirmed the negative role of adhesion factors, especially vascular cell adhesion molecule (VCAM)-1 and intercellular adhesion molecule (ICAM)-1 on the development of cardiovascular diseases (55, 56). Levels of these factors may serve as markers of cardiovascular events (57). Nizamutdinova et al. reported that paeonol inhibited the production of ICAM-1. The binding of monocytes to ECs was inhibited by paeonol, probably due to the inhibition of p38, extracellular signal-regulated kinase (ERK), and NF-κB signaling pathways. Inhibition of these signaling pathways eventually altered the regulatory effects of TNF-α on ICAM-1 (58). Similarly, paeonol was shown to inhibit VCAM-1 expression through the p38 and ERK1/2 signal transduction pathways in rat aortic ECs (RAECs) (59, 60). In vascular ECs (VECs) extracted from the thoracic aorta of ox-LDL-induced injury rats, paeonol conferred endothelium protective effects by inhibiting monocyte adhesion to VECs and blocked the activation of phosphoinositide 3-kinase (PI3K)/Akt/NF-κB signaling pathway (61). In summary, paeonol regulates the expression of VCAM-1, ICAM-1 and inhibits the adhesion of monocytes by regulating NF-κB, ERK, and other inflammatory pathways.
Paeonol prevents the development of atherosclerosis by inhibiting the proliferation of vascular smooth muscle cells (VSMCs) (62, 63). A study reported that paeonol significantly inhibited the expression of proliferating cell nuclear antigen and the proliferation of VSMCs (64). Wu et al. found that paeonol down-regulated the expression of potent vasoconstrictor peptide endothelin (ET)-1, and inhibited the proliferation of VSMCs by suppressing the diacylglycerol-protein kinase C signaling pathway (65). Paeonol suppressed the release of vascular endothelial growth factor (VEGF) and platelet-derived growth factor B in a co-culture system of VSMCs and VECs. In addition, paeonol inhibited the proliferation of VSMCs through the Ras-Raf-ERK1/2 signaling pathway (66). Recent studies revealed that paeonol inhibited the invasion and proliferation of VSMCs. This process was accompanied by increased expression of microtubule-associated protein light chain 3 (LC3), degradation of p62, and the appearance of autophagosomes in the arterial middle layer. It was postulated that paeonol up-regulated autophagy and activated adenosine monophosphate-activated protein kinase (AMPK)/mechanistic target of rapamycin (mTOR) signaling pathway to inhibit the proliferation of VSMCs (67). Therefore, paeonol inhibits the proliferation of VSMCs by regulating diacylglycerol-protein kinase C, ERK signaling pathways, and autophagy. Details of experiments for the anti-inflammatory mechanisms of paeonol are presented in Table 1.
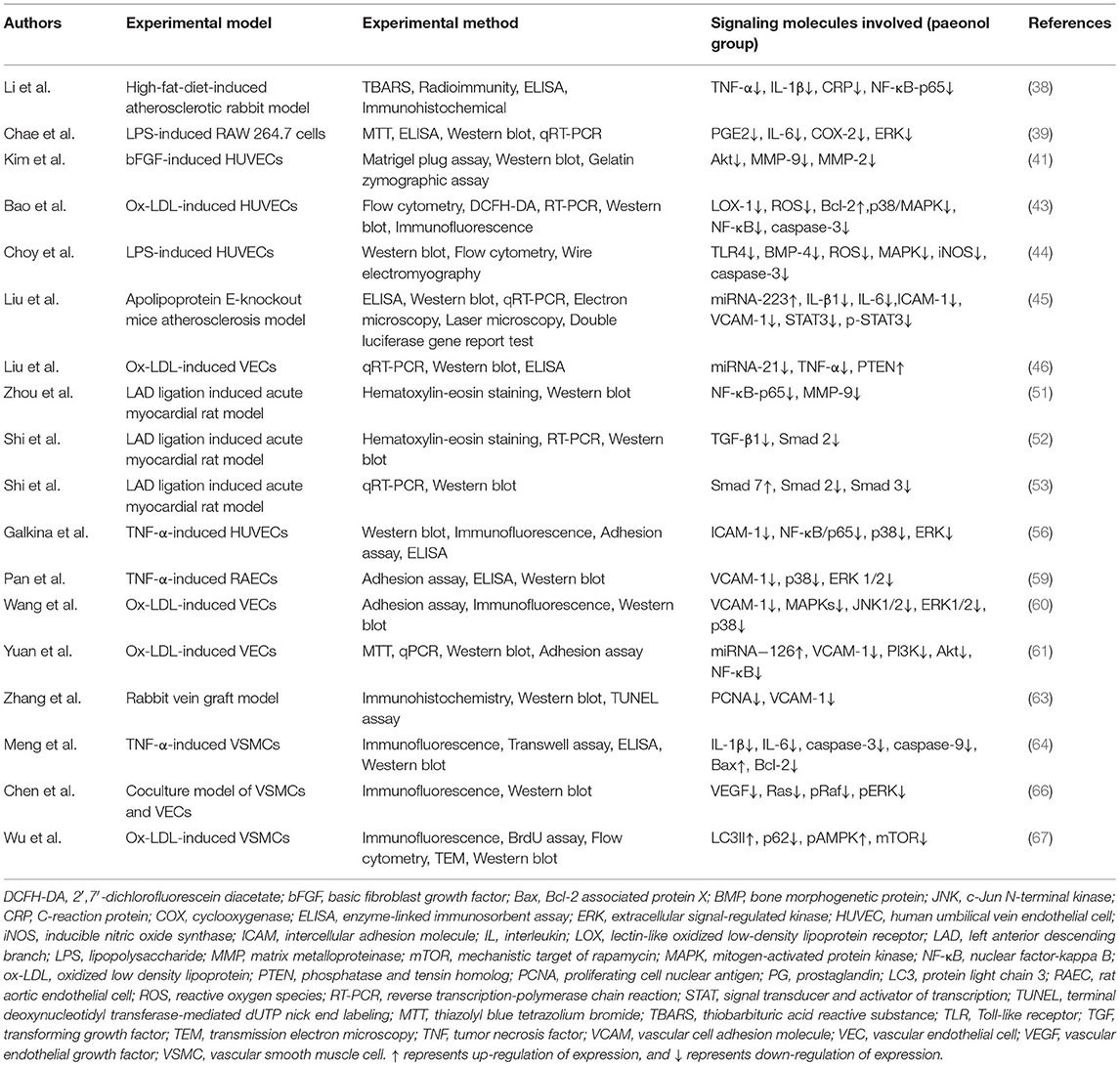
Table 1. Experiments on the anti-inflammatory mechanism of paeonol in atherosclerotic cardiovascular disease.
Abnormal Lipid Metabolism
Abnormal lipid metabolism is one of the independent risk factors for atherosclerosis (68). Paeonol suppressed serum levels of total cholesterol, triglyceride, low-density lipoprotein, very low-density lipoprotein, apolipoprotein B100, as well as total cholesterol levels in the aorta and liver (69). Moreover, paeonol increased high-density lipoprotein levels, improved blood and plasma viscosity in rabbit models (70). A study reported that paeonol could enhance the activity of hepatic lipase, lipoprotein lipase and inhibit the activity of 3-hydroxy-3-methyl glutaryl coenzyme A reductase (HMGCR) (71).
Foam cells, typical pathologic cells in atherosclerotic plaques, are formed by macrophage phagocytosis of ox-LDL and intracellular lipids (72–74). Reverse cholesterol transport is an important mechanism for inhibiting the formation of foam cells (75, 76). A study found that paeonol inhibited cholesterol accumulation in macrophages following treatment with ox-LDL. Paeonol significantly elevated mRNA and protein expression of adenosine triphosphate (ATP)-binding cassette transporter A1 (ABCA1). Notably, ABCA1 inhibitors abrogated the effects of paeonol on cholesterol efflux and accumulation. This result suggested that paeonol conferred protection to macrophages by promoting cholesterol efflux (77). In RAW264.7 macrophages and apolipoprotein E-knockout mice, paeonol reduced lipid accumulation in macrophages by suppressing ox-LDL uptake and by enhancing cholesterol outflow. A small hairpin RNA targeting heme oxygenase (HO)-1 inhibited paeonol-induced benefits on the expression of c-Jun, CD36, ABCA1, calpain activity in macrophages (78). Collectively, these results show that paeonol regulates lipid metabolism by inhibiting lipid synthesis, enhancing the activity of lipase, and regulating the reverse transport process in macrophages. Pharmacological mechanisms of paeonol on anti-inflammatory and regulating lipid metabolism in atherosclerotic cardiovascular disease are presented in Figure 3.
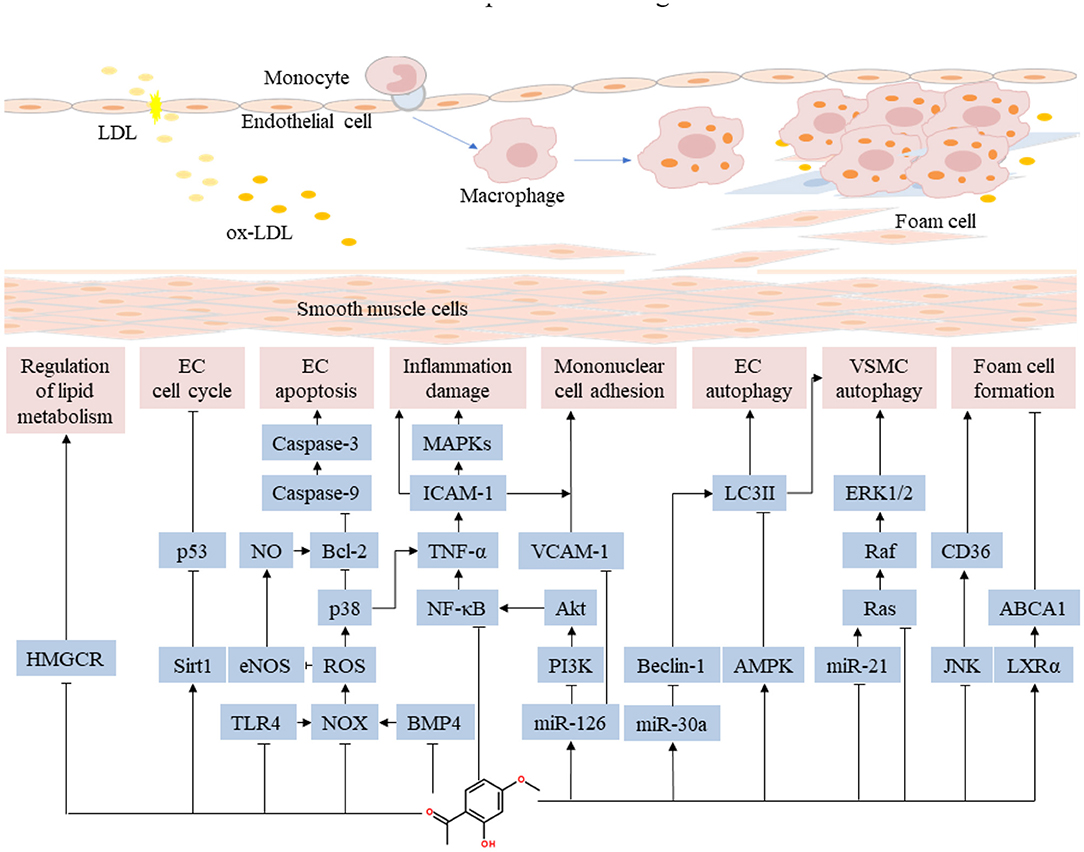
Figure 3. Pharmacological mechanisms of paeonol on anti-inflammatory and regulating lipid metabolism in atherosclerotic cardiovascular disease. Paeonol confers anti-inflammatory effect by suppressing the release of inflammatory cytokines through a variety of pathways, inhibiting the monocyte adhesion and the proliferation of VSMCs. Paeonol regulates lipid metabolism by inhibiting lipid synthesis, enhancing the activity of lipase, and regulating the reverse transport process in macrophages. ABCA1, adenosine triphosphate-binding cassette transporter A1; JNK, c-Jun N-terminal kinase; EC, endothelial cell; eNOS, endothelial nitric oxide synthase; ERK, extracellular signal-regulated kinase; ICAM, intercellular adhesion molecule; LXRα, liver X receptor-alpha; MAPK, mitogen-activated protein kinase; NOX, nitrogen oxide; NO, nitrous oxide; NF-κB, nuclear factor-kappa B; PI3K, phosphoinositide 3-kinase; ROS, reactive oxygen species; HMGCR, recombinant 3-hydroxy-3-methylglutaryl coenzyme A reductase; TLR, Toll-like receptor; TNF, tumor necrosis factor; VCAM, vascular cell adhesion molecule; VSMC, vascular smooth muscle cell.
Platelet Aggregation
Adhesive aggregation of platelets and the release of EC growth factors can stimulate smooth muscle cells (SMCs) to migrate into the intima (79–81), thereby accelerating the formation of foam cells. Moreover, in the unstable plaque rupture process, high platelet aggregation causes fatal thrombosis (82), which leads to myocardial infarction. Previous studies reported that paeonol reduced whole blood viscosity, plasma viscosity, and platelet adhesion, which exerted a positive effect on hemodynamics (83, 84). Doble et al. performed a platelet aggregation test using paeonol analogs and built a back-propagation neural network model for testing. It was found that paeonol analogs could not interact with the cyclooxygenase (COX)-1 enzyme to inhibit platelet activity, which suggested that the effect of paeonol on platelet aggregation may involve other mechanisms (85). Koo et al. reported that paeonol could promote blood circulation by inhibiting platelet aggregation and coagulation (86). Another study reported that paeonol analogs effectively and selectively inhibited the arachidonic acid-induced aggregation of rabbit platelets. Furthermore, these analogs inhibited the arachidonic acid-induced formation of thromboxane (TX) A2 and promoted the production of PGD2 (87). Ye et al. elucidated that D-dimer and TXB2 levels were suppressed and the expression levels of ERK 1/2 and VEGF were significantly increased after paeonol treatment in a rat model of thrombus recanalization (88). In summary, paeonol inhibits platelet aggregation through the regulation of TX, PG, and enhances thrombus recanalization via the ERK1/2 signaling pathway (Figure 4).
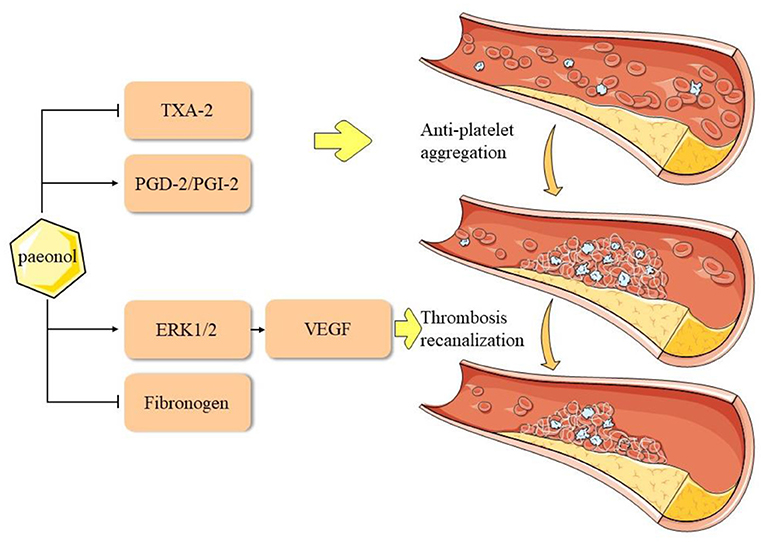
Figure 4. Pharmacological mechanisms of paeonol in inhibition of platelet aggregation in atherosclerotic cardiovascular disease. ERK, extracellular signal-regulated kinase; PG, prostaglandin; PGI2, prostacyclin; TX, thromboxane; VEGF, vascular endothelial growth factor.
Mitochondria Damage and Apoptosis
Oxidative stress plays a significant role in the development of atherosclerosis (89–91). Studies have shown that paeonol confers antioxidant properties in various cardiovascular diseases (92, 93). Paeonol was shown to enhance NO production while reducing reactive oxygen species (ROS) production (94), thereby preventing EC damage caused by ROS.
Mitochondria are the main sources of intracellular ROS and are closely associated with oxidative stress. Atherosclerosis, inflammation (95), hyperglycemia (96), hyperlipidemia (97), and other risk factors can lead to mitochondrial damage and stimulate the overproduction of ROS. This process promotes the apoptosis of ECs and SMCs, resulting in atherosclerosis (98). A previous study reported that paeonol inhibited hypoxia-induced damage of primary human pulmonary artery SMCs (PASMCs) mitochondrial damage in vitro. Moreover, paeonol improved adverse effects of decreased ATP production, morphological changes, mitochondrial polarization, and increased ROS generation. In addition, paeonol caused significant changes in mitochondrial-dependent apoptosis through peroxisome proliferator-activated receptor (PPAR) γ coactivator (PGC)-1α (99). These results show the protective effects of paeonol on mitochondrial under hypoxic conditions and confirm the crucial role of PGC-1α in PASMCs apoptosis.
Studies have shown that paeonol plays a protective role in the myocardium by regulating cell apoptosis. Li et al. reported the protective effect of paeonol on myocardium in myocardial infarction in rats. The effect was correlated with the antioxidant defense system through the activation of the nuclear factor erythroid 2-related factor (Nrf2) signaling pathway and the regulation of Bcl-2 associated protein X (Bax), Bcl-2, and caspase-3 (51). Other findings suggested that the protective effect of paeonol on myocardium in ischemia-reperfusion injury was associated with inhibition of cell apoptosis (100). The hypoxic/reoxygenated rat cardiomyocyte model showed that paeonol could down-regulate the expression of apoptosis-related proteins to restore the viability of H9c2 cells. The mechanism was correlated with the activation of the PI3K/Akt pathway (101). In conclusion, paeonol exerts cardiovascular protective effect by protecting mitochondria and inhibiting apoptosis, and the mechanism is related to the activation of PGC-1α, Nrf2, and PI3K pathways.
Endoplasmic Reticulum Stress
Pathological stimuli including hypoxia, ischemia, inflammation, and oxidative stress interrupt the homeostatic function of the endoplasmic reticulum, leading to the accumulation of unfolded proteins, a condition referred to as ERS (102). Endoplasmic reticulum stress determines cell fate and function (103, 104). A large number of studies show that ERS aggravates cardiovascular diseases (105, 106), especially atherosclerosis (107, 108).
Studies have shown that paeonol plays a cardiovascular protective role by inhibiting ERS. Choy et al. investigated the protective mechanism of paeonol against tunicamycin-induced ERS in isolated mouse aortas ECs and HUVECs. The results confirmed that paeonol treatment increased ERS markers, oxidative stress, and reduction of NO bioavailability induced by tunicamycin were reversed. Paeonol increased the expression of AMPK, PPARδ while restoring the decreased phosphorylation of endothelial nitric oxide synthase. The study showed that paeonol alleviated tunicamycin-induced vascular endothelial dysfunction by inhibition of ERS and oxidative stress, thus elevating NO bioavailability via the AMPK/PPARδ signaling pathway (109). Similar studies explored the vascular protective effects of paeonol on ER stress-induced endothelial dysfunction in mice (110). The findings indicated that paeonol preserved endothelial function in mice induced by tunicamycin via inhibiting ERS.
Autophagy
Autophagy is a highly conserved mechanism of lysosome-mediated protein and organelle degradation that plays a crucial role in maintaining cellular homeostasis (111). In the cardiovascular system, autophagy appears to be essential to heart and vessel homeostasis and function. However, defective or excessive autophagy activity is associated with atherosclerosis (112, 113).
Wu et al. reported that paeonol restricted the development of atherosclerosis in apolipoprotein E-knockout mice and decreased the amount of VSMCs in the media layer. Paeonol enhanced the formation of autophagosomes and up-regulated the expression of LC3II. In addition, Paeonol induced phosphorylation of AMPK and reduced phosphorylation of mTOR. These results showed that paeonol inhibited the proliferation of VSMCs by up-regulating autophagy and activating the AMPK/mTOR signaling pathway (69). Similarly, recent research found that paeonol prevented lipid metabolism dysfunction in palmitic acid-induced HepG2 injury by promoting Sirtuin 1-FoxO1 autophagy-related pathway (114).
Li et al. used ox-LDL-induced rat VECs as a model system to elucidate the protective effect of paeonol on VECs injury. The study showed that paeonol significantly reduced ox-LDL-induced formation of autophagy vacuoles and the expression of LC3II in VECs. Moreover, the study reported that ox-LDL decreased miRNA-30a and increased Beclin-1 expression, whereas pretreatment with paeonol reversed the process of regulation (115). Another research investigated the molecular mechanisms of the crosstalk between apoptosis and autophagy subjected to myocardial ischemia/reperfusion (I/R) injury. The result showed that paeonol significantly improved cardiac function after I/R. Compared with vehicle treatment, paeonol significantly downregulated the cleaved forms of caspase-8, caspase-9, caspase-3 protein expression, and myocardial I/R-induced autophagy was significantly reversed by paeonol treatment (116). Similar research showed that paeonol could induce VSMCs autophagy by activating the class III PI3K/Beclin-1 signaling pathway, thus ultimately inhibiting VSMCs apoptosis (117).
In conclusion, paeonol exerts cardiovascular protective effects by regulating autophagy, and the mechanism may be related to AMPK and Sirtuin 1 pathways. Recent studies have found that the regulatory effect of paeonol on autophagy may be related to miRNA and the crosstalk between autophagy and apoptosis, which may be the focus of further research on the mechanism of the cardiovascular protective effect of paeonol.
Non-coding RNA
Non-coding RNA is a class of transcripts that do not encode proteins and include miRNA, long non-coding RNA, and circular RNA (118). Studies have found that miRNAs are closely associated with cardiovascular disease. The potential of miRNAs as therapeutic targets for heart and vascular disease, and the use of miRNAs as novel biomarkers (119, 120).
Shi et al. used high-fat-diet-induced hyperlipidemic rats as a model to investigate if paeonol could inhibit nucleotide-binding oligomerization domain (NOD)-like receptor family pyrin domain containing 3 (NLRP3) inflammasome by elevating plasma-derived exosomal miRNA-223. In vivo experiments confirmed that paeonol increased the survival rate of RAECs and the expression of exosomal miRNA-223. Moreover, paeonol decreased the expression of NLRP3, caspase-1, and ICAM-1. These results showed that paeonol inhibited the downstream NLRP3 inflammasome pathway by increasing the level of miRNA-223 in plasma-derived exosomes of hyperlipidemic rats (121). Yuan et al. explored the effects of paeonol on miRNA-126 expression and its ability to inhibit monocyte adhesion to ox-LDL-injured VECs. Results showed that paeonol promoted miRNA-126 expression and suppressed VCAM-1 expression at the mRNA and protein level. In addition, it inhibited monocyte adhesion to ox-LDL-injured VECs by upregulating miRNA-126 expression. Furthermore, it was demonstrated that paeonol blocked the activation of the NF-κB signaling pathway by promoting miRNA-126 expression (63). Another study reported that paeonol alleviated ox-LDL-induced VECs injury by targeting miRNA-30a thus inhibiting excessive autophagy (115).
In summary, miRNAs such as miRNA-223, miRNA-126, and miRNA-126 play important roles in the protective activity of paeonol on cardiovascular disease. Notably, miRNAs in exosomes can be targeted for the treatment of atherosclerotic cardiovascular disease.
Intestinal Flora
Alterations in the composition and function of intestinal flora, known as gut microflora dysbiosis, can accelerate the progression of a variety of diseases (122). The interaction between intestinal flora and natural medicine is crucial for host health (123).
Previous studies have confirmed that natural medicines can be used for the treatment of a variety of intestinal and digestive system diseases by regulating intestinal flora (124, 125). Intestinal flora plays an important role in the treatment of cardiovascular diseases using natural compounds (126). Studies have explored the regulatory effect of paeonol on the intestinal flora. Paeonol exerts anti-inflammatory effects by regulating intestinal flora (127). In addition, paeonol regulates the brain-gut axis mediated NF-κB signaling pathway and exerts a therapeutic effect in cerebral infarction (128). The cross-talk between gut microbiota and the heart presents a new therapeutic target for paeonol in the treatment of cardiovascular diseases. Potential targets and mechanisms of paeonol in the treatment of atherosclerotic cardiovascular disease are presented in Figure 5.
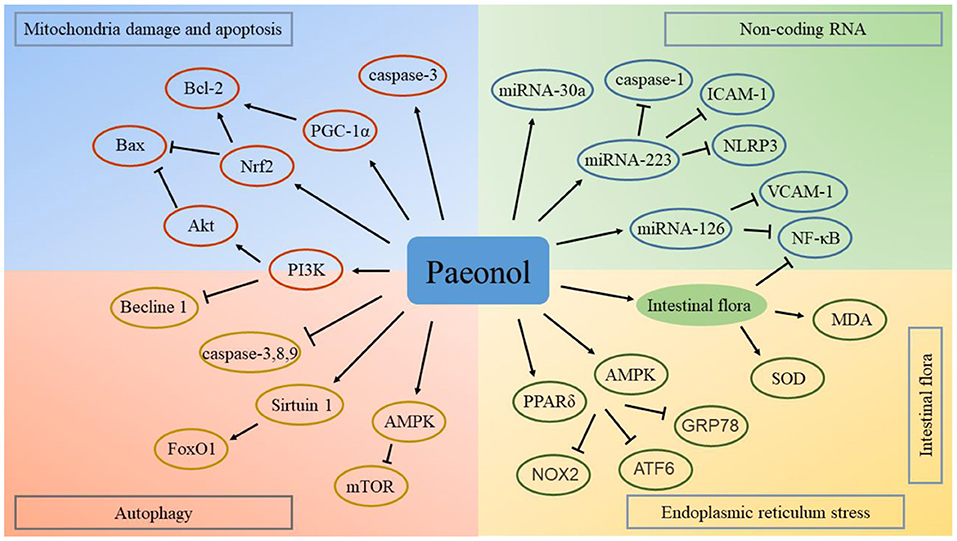
Figure 5. Potential targets and mechanisms of paeonol in the treatment of atherosclerotic cardiovascular disease. ATF6, activating transcription factor 6; AMPK, adenosine monophosphate-activated protein kinase; Bax, Bcl-2 associated protein X; GRP78, glucose-regulated protein 78; ICAM, intercellular adhesion molecule; MDA, malonaldehyde; mTOR, mechanistic target of rapamycin; NOX2, nicotinamide adenine dinucleotide phosphate oxidase; Nrf2, nuclear factor erythroid 2-related factor; NF-κB, nuclear factor-kappa B; NLRP3, nucleotide binding oligomerization domain-like receptor family pyrin domain containing 3; PPAR, peroxisome proliferator-activated receptor; PGC, peroxisome proliferator-activated receptor γ coactivator; PI3K, phosphoinositide 3-kinase; SOD, superoxide dismutase; VCAM, vascular cell adhesion molecule.
Toxicology and Pharmacokinetics of Paeonol
Studies have not evaluated the toxicity and safety of paeonol, however, its pharmacokinetics have been reported. Oral paeonol is well-absorbed in the entire intestines, except for the colon. Paeonol is absorbed through passive diffusion (129), which conforms to the first-order elimination kinetics (130), and the drug-time curve has double peaks (131).
The ultra-HPLC-tandem mass spectrometry method was used to assess the absorption of orally administered paeonol in rats. The findings showed that paeonol was distributed in various tissues without long-term accumulation. Paeonol and its metabolites were mainly distributed in the kidney, liver, and heart. Paeonol could pass through the blood-brain barrier, however, its level in the brain dropped rapidly after 10 min (132). This conclusion was inconsistent with previous reports using gas chromatography-mass spectrometry showing that paeonol could not easily penetrate the blood-brain barrier but accumulated in the brain for long periods (133). Reverse-phase HPLC revealed that paeonol was mainly excreted in urine (134, 135). Paeonol was affected by the interactions of coexisting ingredients during preparation (136). For example, co-administration could alter pharmacokinetics and tissue distribution of paeonol in rats, mainly in the heart and brain (137).
Wu et al. used HPLC to measure paeonol concentration in human plasma after oral medication and found that the linear relationship was acceptable within the concentration range of 10–500 ng/ml. Analysis showed no statistical differences in the main pharmacokinetic parameters of the tablet and capsule dosage forms of the drug (138). Other studies reported that the pharmacokinetics of paeonol varied with administration routes (139). The absolute bioavailability of paeonol after intramuscular injection was 68.68% (140), while the absolute bioavailability of orally administered paeonol was 28.92%, which was relatively low (141). Poor water solubility and stability as well as high volatility at room temperatures limited clinical applications of paeonol (28).
Several studies improved the absorption efficiency and bioavailability of paeonol by changing the administrative route (142, 143). Paeonol is administered in conventional formulations such as tablets, topical gels, hydrogels, and polymer delivery systems such as nanocapsules and polymeric nanoparticles, microemulsions, liposomes, and lipid-based nanoparticles (144–146). Studies on dosage forms focused on transdermal delivery. Isopropyl myristate, cremophor, and polyethylene-glycol were used to prepare microemulsion gels, and glycerol monooleate to prepare cubic gels. The two formulations had higher skin permeabilities compared with paeonol solution, and their relative bioavailability was higher by 1.28 times and 1.51 times, respectively, compared with paeonol solution (147). Using skin-blood synchronous microdialysis coupled with the liquid chromatograph-mass spectrometer, Liu et al. reported that stable concentrations of paeonol microemulsion gel in blood were higher than that of general dosage forms (148, 149). Shi et al. used the Franz diffusion cell and reported that the positive ion liposome gel had superior stabilities and permeabilities compared with conventional gels (150). Ethanol injection was used to prepare paeonol-loaded nanovesicles, while HPLC was used to analyze the concentrations of paeonol in rat plasma after transdermal administration. The nanovesicle formulation showed an enhanced transdermal flux, and paeonol bioavailability was significantly improved in line with the one-compartment absorption model. Similarly, nanocapsules improved the bioavailability and pharmacological properties of paeonol (151, 152). These new drug delivery systems have improved the stability, bioavailability, and pharmacological properties of paeonol.
Concluding Remarks
The cardiovascular effects of paeonol have been extensively studied. Paeonol suppresses inflammatory factor production by modulating signaling pathways such as MAPK, TLR, and ERK. In addition, paeonol improves lipid metabolism by inducing lipase activity and inhibiting lipid synthesis. Paeonol plays an important role in reverse cholesterol transport thus preventing the formation of foam cells. Furthermore, it protects mitochondria and reduces ROS accumulation. This is achieved by up-regulating cell autophagy and induction of cell apoptosis. Paeonol protects ischemic tissues and prevents platelet aggregation. Moreover, paeonol regulates ERS, non-coding RNA, and intestinal flora. Although the pharmacokinetics of paeonol has been extensively studied, its toxicological effects and safety have not been evaluated. High-quality clinical controlled trials should be conducted to explore the clinical benefits of paeonol in atherosclerotic cardiovascular diseases.
Author Contributions
MW and LL: the conception and design of the study. ZY: the conception and design of the study and drafting the article. XL: drafting the article. XZ, SW and LL: revising the article critically. SY and LH: revising the article. All authors contributed to the article and approved the submitted version.
Funding
This study was financially supported by the National Natural Science Foundation of China (Grant No. 81973689, 82074254) and the Beijing Natural Science Foundation (7202176).
Conflict of Interest
The authors declare that the research was conducted in the absence of any commercial or financial relationships that could be construed as a potential conflict of interest.
Acknowledgments
Thanks to Servier and Visual China Group for the figures materials.
Abbreviations
HMGCR, 3-hydroxy-3-methyl glutaryl coenzyme A reductase; AMPK, adenosine monophosphate-activated protein kinase; ATP, adenosine triphosphate; ABCA1, adenosine triphosphate-binding cassette transporter A1; bFGF, basic fibroblast growth factor; Bax, Bcl-2 associated protein X; BMP, bone morphogenetic protein; CRP, C-reaction protein; COX, cyclooxygenase; ERS, endoplasmic reticulum stress; EC, endothelial cell; ET, endothelin; ERK, extracellular signal-regulated kinase; HO, heme oxygenase; HPLC, high-performance liquid chromatography; HUVEC, human umbilical vein endothelial cell; ICAM, intercellular adhesion molecule; IL, interleukin; I/R, ischemia/reperfusion; LPS, lipopolysaccharide; MMP, matrix metalloproteinase; mTOR, mechanistic target of rapamycin; miRNA, microRNA; MAPK, mitogen-activated protein kinase; NO, nitrous oxide; NLRP3, NOD-like receptor family pyrin domain containing 3; Nrf2, nuclear factor erythroid 2-related factor; NF-κB, nuclear factor-kappa B; NOD, nucleotide binding oligomerization domain; ox-LDL, oxidized low-density lipoprotein; PPAR, peroxisome proliferator-activated receptor; PGC, peroxisome proliferator-activated receptor γ coactivator; PI3K, phosphoinositide 3-kinase; PG, prostaglandin; LC3, protein light chain 3; PASMC, pulmonary artery smooth muscle cell; RAEC, rat aortic endothelial cell; ROS, reactive oxygen species; SMC, smooth muscle cell; TX, thromboxane; TLR, Toll-like receptor; TGF, transforming growth factor; TNF, tumor necrosis factor; VCAM, vascular cell adhesion molecule; VEC, vascular endothelial cell; VEGF, vascular endothelial growth factor; VSMC, vascular smooth muscle cell.
References
1. Ferraro R, Latina JM, Alfaddagh A, Michos ED, Blaha MJ, Jones SR, et al. Evaluation and management of patients with stable angina: beyond the ischemia paradigm: JACC state of the art review. J Am Coll Cardiol. (2020) 76:2252–66. doi: 10.1016/j.jacc.2020.08.078
2. Bij de Weg JM, Abheiden CNH, Fuijkschot WW, Harmsze AM, de Boer MA, Thijs A, et al. Resistance of aspirin during and after pregnancy: a longitudinal cohort study. Pregnancy Hypertens. (2020) 19:25–30. doi: 10.1016/j.preghy.2019.11.008
3. Nicola H, Ho KM. Aspirin resistance incidence and associations between aspirin effect and outcomes in cardiac surgery. Ann Thorac Surg. (2019) 108:1815–21. doi: 10.1016/j.athoracsur.2019.04.114
4. Ben Salem C, Sahnoun D, Slim R, Ben Fradj F, Aouani C. Atorvastatin and sildenafil interaction-induced rhabdomyolysis. Ann Pharmacother. (2020) 54:1047–8. doi: 10.1177/1060028020919933
5. Waksman R, Iantorno M. Refractory instent restenosis: improving outcomes by standardizing our approach. Curr Cardiol Rep. (2018) 20:140. doi: 10.1007/s11886-018-1076-6
6. Penson PE, Banach M. Natural compounds as anti-atherogenic agents: clinical evidence for improved cardiovascular outcomes. Atherosclerosis. (2021) 316:58–65. doi: 10.1016/j.atherosclerosis.2020.11.015
7. Arauna D, Furrianca M, Espinosa-Parrilla Y, Fuentes E, Alarcón M, Palomo I. Natural bioactive compounds as protectors of mitochondrial dysfunction in cardiovascular diseases and aging. Molecules. (2019) 24:4259. doi: 10.3390/molecules24234259
8. Wang Z, He C, Peng Y, Chen F, Xiao P. Origins, phytochemistry, pharmacology, analytical methods and safety of Cortex Moutan (Paeonia suffruticosa Andrew): a systematic review. Molecules. (2017) 22:946. doi: 10.3390/molecules22060946
9. Zhang L, Chen Z, Gong W, Zou Y, Xu F, Chen L, et al. Paeonol ameliorates diabetic renal fibrosis through promoting the activation of the Nrf2/ARE pathway via up-regulating Sirt 1. Front Pharmacol. (2018) 9:501–12. doi: 10.3389/fphar.2018.00512
10. Chen G, Jia P, Yin ZY, Kong SZ, Xiang ZB, Zheng XX. Paeonol ameliorates monosodium urate-induced arthritis in rats through inhibiting nuclear factor-κB-mediated proinflammatory cytokine production. Phytother Res. (2019) 33:2971–8. doi: 10.1002/ptr.6472
11. Lin MY, Lee YR, Chiang SY, Li YZ, Chen YS, Hsu CD, et al. Cortex Moutan induces bladder cancer cell death via apoptosis and retards tumor growth in mouse bladders. Evid Based Complement Alternat Med. (2013) 2013:207279. doi: 10.1155/2013/207279
12. Li P, Shen J, Wang Z, Liu S, Liu Q, Li Y, et al. Genus Paeonia: a comprehensive review on traditional uses, phytochemistry, pharmacological activities, clinical application, and toxicology. J Ethnopharmacol. (2021) 269:113708. doi: 10.1016/j.jep.2020.113708
13. Zhao M, Wu SPA. review of the ethnobotany, phytochemistry and pharmacology of tree peony. South Afr J Bot. (2019) 124:556–63. doi: 10.1016/j.sajb.2019.05.018
14. Zhang X, Zhai Y, Yuan J. Hu Y. New insights into Paeoniaceae used as medicinal plants in China. Sci Rep. (2019) 9:18469. doi: 10.1038/s41598-019-54863-y
15. Zhou ZQ. Taxonomy, geographic distribution and ecological habitats of tree peonies. Genet Resour Crop Evol. (2006) 53:11–22. doi: 10.1007/s10722-005-0778-y
16. Wang X, Fan H, Li Y, Sun X, Sun X, Wang W, et al. Analysis of genetic relationships in tree peony of different colors using conserved DNA-derived polymorphism markers. Sci Hortic. (2014) 175:68–73. doi: 10.1016/j.scienta.2014.05.026
17. He L, Suo Z, Zhang C, Jin X, Zhao D, Zhao X, et al. Classification of Chinese medicinal tree peony cultivars based on Chloroplast DNA sequences. AASRI Procedia. (2012) 1:344–52. doi: 10.1016/j.aasri.2012.06.053
18. Guo X, Cheng FY, Zhong Y. Genetic diversity of Paeonia rockii (Flare Tree Peony) germplasm accessions revealed by phenotypic traits, EST-SSR markers and chloroplast DNA sequences. Forests. (2020) 11:672. doi: 10.3390/f11060672
19. Pan Y, Gao Z, Huang XY, Chen JJ, Geng CA. Chemical and biological comparison of different parts of Paeonia suffruticosa (Mudan) based on LCMS-IT-TOF and multi-evaluation in vitro. Ind Crops Prod. (2020) 144:112028. doi: 10.1016/j.indcrop.2019.112028
20. Wang X, Cheng C, Sun Q, Li F, Liu J, Zheng C. Isolation and purification of four flavonoid constituents from the flowers of Paeonia suffruticosa by high-speed counter-current chromatography. J Chromatogr A. (2005) 1075:127–31. doi: 10.1016/j.chroma.2005.04.017
21. Ogawa K, Nakamura S, Sugimoto S, Tsukioka J, Hinomaru F, Nakashima S, et al. Constituents of flowers of Paeoniaceae plants, Paeonia suffruticosa and Paeonia lactiflora. Phytochem Lett. (2015) 12:98–104. doi: 10.1016/j.phytol.2015.03.002
22. Wu SH, Wu DG, Chen YW. Chemical constituents and bioactivities of plants from the genus Paeonia. Chem Biodivers. (2010) 7:90–104. doi: 10.1002/cbdv.200800148
23. Feng Y, Yin L, Liu Y, Cao L, Zheng N, Li M, et al. Quantitative determination and optimun extraction technique of nine compounds of Paeoniae Radix Alba. Zhejiang Da Xue Xue Bao Yi Xue Ban. (2020) 49:356–63. doi: 10.3785/j.issn.1008-9292.2020.05.01
24. Jiao MJ, Deng Z, Zhang J, Wang SH, Cui WJ, Zhang GY, et al. Preparation and quality standard of standard decoction of Chinese herbal medicine containing volatile components-case study of Moutan Cortex. Zhongguo Zhong Yao Za Zhi. (2018) 43:891–6. doi: 10.19540/j.cnki.cjcmm.20180105.017
25. Sun M, Huang L, Zhu J, Bu W, Sun J, Fang Z. Screening nephroprotective compounds from cortex Moutan by mesangial cell extraction and UPLC. Arch Pharm Res. (2015) 38:1044–53. doi: 10.1007/s12272-014-0469-3
26. Zhang L, Li DC, Liu LF. Paeonol: pharmacological effects and mechanisms of action. Int Immunopharmacol. (2019) 72:413–21. doi: 10.1016/j.intimp.2019.04.033
27. Ji X, Shi S, Liu B, Shan M, Tang D, Zhang W, et al. Bioactive compounds from herbal medicines to manage dyslipidemia. Biomed Pharmacother. (2019) 118:109338. doi: 10.1016/j.biopha.2019.109338
28. Adki KM, Kulkarni YA. Chemistry, pharmacokinetics, pharmacology and recent novel drug delivery systems of paeonol. Life Sci. (2020) 250:117544. doi: 10.1016/j.lfs.2020.117544
29. Chen FL, Mo KL, Zhang Q, Fei SM, Zu YG, Yang L, et al. novel approach for distillation of paeonol and simultaneous extraction of paeoniflorin by microwave irradiation using an ionic liquid solution as the reaction medium. Sep Purif Technol. (2017) 183:73–82. doi: 10.1016/j.seppur.2017.03.069
30. Sui Y, Zhao LS, Wang ZZ, Zhao YT, Xiao W, Xiong ZL. Simultaneous determination of seven bioactive components in Guizhi Fuling capsule by microwave-assisted extraction combined with ultra-performance liquid chromatography tandem mass spectrometry. Nat Prod Res. (2016) 30:354–7. doi: 10.1080/14786419.2015.1052068
31. Wang ZQ, Shen J, Li P, Liu SS, Yi F, Liu HB, et al. Research on quality markers of Moutan Cortex: quality evaluation and quality standards of Mountan Cortex. Chin Herbal Med. (2017) 9:307–20. doi: 10.1016/S1674-6384(17)60110-2
32. Soysal P, Arik F, Smith L, Jackson SE, Isik AT. Inflammation, frailty and cardiovascular disease. Adv Exp Med Biol. (2020) 1216:55–64. doi: 10.1007/978-3-030-33330-0_7
33. Hedin U, Matic LP. Recent advances in therapeutic targeting of inflammation in atherosclerosis. J Vasc Surg. (2019) 69:944–51. doi: 10.1016/j.jvs.2018.10.051
34. Chan YH, Ramji DP. A perspective on targeting inflammation and cytokine actions in atherosclerosis, Future Med Chem. (2020) 12:613–26. doi: 10.4155/fmc-2019-0301
35. Zong SY, Pu YQ, Xu BL, Zhang T, Wang B. Study on the physicochemical properties and anti-inflammatory effects of paeonol in rats with TNBS-induced ulcerative colitis. Int Immunopharmacol. (2017) 42:32–8. doi: 10.1016/j.intimp.2016.11.010
36. Huang L, Zhang B, Yang Y, Gong X, Chen Z, Wang Z, et al. Synthesis and anti-inflammatory activity of paeonol analogues in the murine model of complete Freund's adjuvant induced arthritis. Bioorg Med Chem Lett. (2016) 26:5218–21. doi: 10.1016/j.bmcl.2016.09.060
37. Himaya SW, Ryu B, Qian ZJ, Kim SK. Paeonol from Hippocampus kuda Bleeler suppressed the neuro-inflammatory responses in vitro via NF-kappaB and MAPK signaling pathways. Toxicol In Vitro. (2012) 26:878–87. doi: 10.1016/j.tiv.2012.04.022
38. Li HK, Dai M, Jia W. Paeonol attenuates high-fat-diet-induced atherosclerosis in rabbits by anti-inflammatory activity. Planta Med. (2009) 75:7–11. doi: 10.1055/s-0028-1088332
39. Chae HS, Kang OH, Lee YS, Choi JG, Oh YC, Jang HJ, et al. Inhibition of LPS-induced iNOS, COX-2 and inflammatory mediator expression by paeonol through the MAPKs inactivation in RAW 2647 cells. Am J Chin Med. (2009) 37:181–94. doi: 10.1142/S0192415X0900676X
40. Huang H, Chang EJ, Lee Y, Kim JS, Kang SS, Kim HH, et al. genome-wide microarray analysis reveals anti-inflammatory target genes of paeonol in macrophages. Inflamm Res. (2008) 57:189–98. doi: 10.1007/s00011-007-7190-3
41. Kim SA, Lee HJ, Ahn KS, Lee HJ, Lee EO, Ahn KS, et al. Paeonol exerts anti-angiogenic and anti-metastatic activities through downmodulation of Akt activation and inactivation of matrix metalloproteinases. Biol Pharm Bull. (2009) 32:1142–7. doi: 10.1248/bpb.32.1142
42. Bao MH, Zhang YW, Zhou HH. Paeonol suppresses oxidized low-density lipoprotein induced endothelial cell apoptosis via activation of LOX-1/p38MAPK/NF-kappa B pathway. J Ethnopharmacol. (2013) 146:543–51. doi: 10.1016/j.jep.2013.01.019
43. Fiordelisi A, Iaccarino G, Morisco C, Coscioni E, Sorriento D. NF kappa B is a key player in the crosstalk between inflammation and cardiovascular diseases. Int J Mol Sci. (2019) 20:1599. doi: 10.3390/ijms20071599
44. Choy KW, Lau YS, Murugan D, Vanhoutte PM, Mustafa MR. Paeonol attenuates LPS-induced endothelial dysfunction and apoptosis by inhibiting BMP4 and TLR4 signaling simultaneously but independently. J Pharmacol Exp Therap. (2018) 364:420–32. doi: 10.1124/jpet.117.245217
45. Liu YR, Chen JJ, Dai M. Paeonol protects rat vascular endothelial cells from ox-LDL-induced injury in vitro via downregulating microRNA-21 expression and TNF-alpha release. Acta Pharmacol Sin. (2014) 35:483–8. doi: 10.1038/aps.2013.190
46. Liu YR, Li C, Wu HF, Xie XM, Sun Y, Dai M. Paeonol attenuated inflammatory response of endothelial cells via stimulating monocytes-derived exosomal microRNA-223. Front Pharmacol. (2018) 9:1105. doi: 10.3389/fphar.2018.01105
47. Feng QQ, Zhou Y, Zhang XM. Protective effect of paeonol on coronary ligation induced myocardial ischemia in anesthetized dogs. Chin J Biochem Pharm. (2008) 29:320–3.
48. Li Y, Hong X, Zhang D. Protective effect of paeonol, paeoniflorin and their different-proportion combinations on acute myocardial infarction in rats. Tradit Chi Drug Res Clin Plarmacol. (2010) 21:254–6.
49. Li H, Xie YH, Yang Q, Wang SW, Zhang BL, Wang JB, et al. Cardioprotective effect of paeonol and danshensu combination on isoproterenol-induced myocardial injury in rats. PLoS ONE. (2012) 7:e48872. doi: 10.1371/journal.pone.0048872
50. Zhou X, Ren L, Liu W. Paeonol inhibiting TLR4 signal pathway and improving ventricular remodeling in rabbit with myocardia infarction. Chin J Gerontol. (2012) 32:4690–2. doi: 10.3969/j.issn.1005-9202.2012.21.038
51. Zhou XH, Zhao JY, Zhang SF, Qian X. Paeonol improved ventricular remodeling in rats after myocardial infarction by inhibiting nf-kappab signaling pathway and down-regulating mmp-9 expression. Chin Tradit Patent Med. (2014) 36:2599–602. doi: 10.3969/j.issn.1001-1528.2014.12.035
52. Shi Z, Zhou X, Xu Q, Cao K. Effect of paeonol on Smad2, Smad3, Smad7 mRNA expression in acute myocardial infarction rat heart. Chin J Exp Tradit Med Formul. (2015) 21:146–50. doi: 10.13422/j.cnki.syfjx.2015020146
53. Shi Z, Zhou X, Xu Q, Zhao J, Cao K. Effect of paeonol on myocardial pathological changes and TGF-beta-1, collagenIII expression in rat models with acute myocardial infarction. Chin J Exp Tradit Med Formul. (2015) 21:98–103. doi: 10.13422/j.cnki.syfjx.2015240098
54. Shi Z, Zhou X, Xu Q, Cao K. Effect and eechanism of paeonol on myocardial fibrosis induced by myocardial infarction in rats. Chin J Exp Tradit Med Formul. (2015) 21:150–5. doi: 10.13422/j.cnki.syfjx.2015090150
55. Peter K, Weirich U, Nordt TK, Ruef J, Bode C. Soluble vascular cell adhesion molecule-1 (VCAM-1) as potential marker of atherosclerosis. Thromb Haemost. (1999) 82:38–43. doi: 10.1055/s-0037-1615551
56. Galkina E, Ley K. Vascular adhesion molecules in atherosclerosis. Arterioscler Thromb Vasc Biol. (2007) 27:2292–301. doi: 10.1161/ATVBAHA.107.149179
57. Mizia Stec K, Zahorska Markiewicz B, Goliszek L. Adhesion molecules: atherosclerosis and coronary artery disease. Przegl Lek. (2003) 60:147–50.
58. Nizamutdinova IT, Oh HM, Min YN, Park SH, Lee MJ, Kim JS. Paeonol suppresses intercellular adhesion molecule-1 expression in tumor necrosis factor-alpha-stimulated human umbilical vein endothelial cells by blocking p38, ERK and nuclear factor-kappa B signaling pathways. Int Immunopharmacol. (2007) 7:343–50. doi: 10.1016/j.intimp.2006.11.004
59. Pan LL, Dai M. Paeonol from Paeonia suffruticosa prevents TNF-alpha-induced monocytic cell adhesion to rat aortic endothelial cells by suppression of VCAM-1 expression. Phytomedicine. (2009) 16:1027–32. doi: 10.1016/j.phymed.2009.04.003
60. Wang YQ, Dai M, Zhong JC, Yin DK. Paeonol inhibits oxidized low-density lipoprotein-induced monocyte adhesion to vascular endothelial cells by inhibiting the mitogen activated protein kinase pathway. Biol Pharm Bull. (2012) 35:767–72. doi: 10.1248/bpb.35.767
61. Yuan XS, Chen JJ, Dai M. Paeonol promotes microRNA-126 expression to inhibit monocyte adhesion to ox-LDL-injured vascular endothelial cells and block the activation of the PI3K/Akt/NF-kappa B pathway. Int J Mol Med. (2016) 38:1871–8. doi: 10.3892/ijmm.2016.2778
62. Dai M, Li HK. Effect of paeonol on proliferation of vascular smooth muscle cell in atherosclerosis rabbits. Zhongguo Yaolixue Tongbao. (2006) 22:587–91. doi: 10.3321/j.issn:1001-1978.2006.05.019
63. Zhang JY, Lei L, Shang J, Huo TM, Zhang B, Chen G, et al. Local application of paeonol prevents early restenosis: a study with a rabbit vein graft model. J Surg Res. (2017) 212:278–87. doi: 10.1016/j.jss.2016.11.020
64. Meng L, Xu W, Guo L, Ning W, Zeng X. Paeonol inhibits the proliferation, invasion, and inflammatory reaction induced by TNF-alpha in vascular smooth muscle cells. Cell Biochem Biophys. (2015) 73:495–503. doi: 10.1007/s12013-015-0686-5
65. Wu HF, Su XF, Dai M. Paeonol inhibits overproliferation of VSMCs in ox-LDL-injured co-cultured system by suppression of ET-1 expression. Latin Am J Pharm. (2015) 34:1858–65.
66. Chen JJ, Dai M, Wang YQ. Paeonol inhibits proliferation of vascular smooth muscle cells stimulated by high glucose via Ras-Raf-ERK1/2 signaling pathway in coculture model. Evid Based Complement Altern Med. (2014) 2014:484269. doi: 10.1155/2014/484269
67. Wu HF, Song AW, Hu WJ, Dai M. The anti-atherosclerotic effect of paeonol against vascular smooth muscle cell proliferation by up-regulation of autophagy via the AMPK/mTOR signaling pathway. Front Pharmacol. (2017) 8:948. doi: 10.3389/fphar.2017.00948
68. Helen WH, Gabriella G, Min L, Piero P, David QH. Cholesterol and lipoprotein metabolism and atherosclerosis: recent advances in reverse cholesterol transport. Ann Hepatol. (2017) 16(Suppl. 1):s27–42. doi: 10.5604/01.3001.0010.5495
69. Dai M, Zhi X, Peng D, Liu Q. Inhibitory effect of paeonol on experimental atherosclerosis in quails. China J Chin Mater Medica. (1999) 24:488–512.
70. Yang QA, Wang SW, Xie YH, Wang JB, Li H, Zhou XX, et al. Effect of Salvianolic acid b and paeonol on blood lipid metabolism and hemorrheology in myocardial ischemia rabbits induced by Pituitruin. Int J Mol Sci. (2010) 11:3696–704. doi: 10.3390/ijms11103696
71. Chen Y, Kang L. Protection effect of paeonol on regulation of lipid metabolism in hyperlipidemia mice and its mechanisms. Chin J Clin Pharmacol. (2017) 33:2273–7. doi: 10.13699/j.cnki.1001-6821.2017.22.017
72. Aviram M, Rosenblat M. Paraoxonases 1, 2, and 3, oxidative stress, and macrophage foam cell formation during atherosclerosis development. Free Rad Biol Med. (2004) 37:1304–16. doi: 10.1016/j.freeradbiomed.2004.06.030
73. Poznyak AV, Wu WK, Melnichenko AA, Wetzker R, Sukhorukov V, Markin AM, et al. Signaling pathways and key genes involved in regulation of foam cell formation in atherosclerosis. Cells. (2020) 9:584. doi: 10.3390/cells9030584
74. Yu XH, Fu YC, Zhang DW, Yin K, Tang CK. Foam cells in atherosclerosis. Clinica Chimica Acta. (2013) 424:245–52. doi: 10.1016/j.cca.2013.06.006
75. Yu XH, Zhang DW, Zheng XL, Tang CK. Cholesterol transport system: an integrated cholesterol transport model involved in atherosclerosis. Prog Lipid Res. (2019) 73:65–91. doi: 10.1016/j.plipres.2018.12.002
76. Chistiakov DA, Bobryshev YV, Orekhov AN. Macrophage-mediated cholesterol handling in atherosclerosis. J Cell Mol Med. (2016) 20:17–28. doi: 10.1111/jcmm.12689
77. Zhao JF, Leu SJJ, Shyue SK, Su KH, Wei J, Lee TS. Novel effect of paeonol on the formation of foam cells: promotion of LXR alpha-ABCA1-dependent cholesterol efflux in macrophages. Am J Chin Med. (2013) 41:1079–96. doi: 10.1142/S0192415X13500730
78. Li X, Zhou Y, Yu C, Yang H, Zhang C, Ye Y, et al. Paeonol suppresses lipid accumulation in macrophages via upregulation of the ATP-binding cassette transporter A1 and downregulation of the cluster of differentiation 36. Int J Oncol. (2015) 46:764–74. doi: 10.3892/ijo.2014.2757
79. Christian W, Heidi N. Atherosclerosis: current pathogenesis and therapeutic options. Nat Med. (2011) 17:1410–22. doi: 10.1038/nm.2538
80. Lu Y, Hu Q, Jiang C, Gu Z. Platelet for drug delivery. Curr Opin Biotechnol. (2019) 58:81–91. doi: 10.1016/j.copbio.2018.11.010
81. Grover SP, Bergmeie W, Mackman N. Platelet signaling pathways and new inhibitors. Arterioscler Thromb Vasc Biol. (2018) 38:e28–35. doi: 10.1161/ATVBAHA.118.310224
82. Michael H. Normal platelet function. Cancer Metastasis Rev. (2017) 36:195–8. doi: 10.1007/s10555-017-9677-x
83. Meng QW, Liu HJ. Paeonol attenuates atherosclerosis in rats through regulating blood lipid profile and resisting inflammatory reaction. Latin Am J Pharm. (2020) 39:475–81.
84. Terawaki K, Noguchi M, Yuzurihara M, Omiya Y, Ikarashi Y, Kase Y. Keishibukuryogan, a traditional Japanese medicine, inhibits platelet aggregation in Guinea pig whole blood. Evid Based Complement Altern Med. (2015) 2015:295706. doi: 10.1155/2015/295706
85. Doble M, Karthikeyan S, Padmawar PA, Akamanchi KG QSAR studies of paeonol analogues for inhibition of platelet aggregation. Bioorg Med Chem. (2005) 13:5996–6001. doi: 10.1016/j.bmc.2005.07.027
86. Koo YK, Kim JM, Koo JY, Kang SS, Bae K, Kim YS, et al. Platelet anti-aggregatory and blood anti-coagulant effects of compounds isolated from Paeonia lactiflora and Paeonia suffruticosa. Pharmazie. (2010) 65:624–8. doi: 10.1691/ph.2010.9870
87. Lin HC, Ding HY, Ko FN, Teng CM, Wu YC. Aggregation inhibitory activity of minor acetophenones from Paeonia species. Planta Med. (1999) 65:595–9. doi: 10.1055/s-1999-14030
88. Ye SS, Liu XJ, Mao BY, Yang L, Liu N. Paeonol enhances thrombus recanalization by inducing vascular endothelial growth factor 165 via ERK1/2 signaling pathway. Mol Med Rep. (2016) 13:4853–8. doi: 10.3892/mmr.2016.5135
89. Senoner T, Dichtl W. Oxidative stress in cardiovascular diseases: still a therapeutic target? Nutrients. (2019) 11:2090. doi: 10.3390/nu11092090
90. Kattoor AJ, Pothineni NVK, Palagiri D, Mehta JL. Oxidative stress in atherosclerosis. Curr Atheroscler Rep. (2017) 19:42. doi: 10.1007/s11883-017-0678-6
91. Ulrich F, Ning X, Huige L. Roles of vascular oxidative stress and nitric oxide in the pathogenesis of atherosclerosis. Circ Res. (2017) 120:713–35. doi: 10.1161/CIRCRESAHA.116.309326
92. Liu MH, Lin AH, Lee HF, Ko HK, Lee TS, Kou YR, et al. Paeonol attenuates cigarette smoke-induced lung inflammation by inhibiting ROS-sensitive inflammatory signaling. Mediators Inflamm. (2014) 2014:654890. doi: 10.1155/2014/651890
93. Hafez H, Morsy M, Mohamed M, Zenhom N. Mechanisms underlying gastroprotective effect of paeonol against indomethacin-induced ulcer in rats. Hum Exp Toxicol. (2019) 38:510–8. doi: 10.1177/0960327118818254
94. Ping M, Xiao W, Mo L, Xiao X, Song S, Tang W, et al. Paeonol attenuates advanced oxidation protein product-induced oxidative stress injury in THP-1 macrophages. Pharmacology. (2014) 93:286–95. doi: 10.1159/000363577
95. Alain M, Gilles L, Livio B, Laure CA, Ghada A, Julien P, et al. Mitochondria: an organelle of bacterial origin controlling inflammation. Front Immunol. (2018) 9:536. doi: 10.3389/fimmu.2018.00536
96. Susana RL, Nadezda A, Celia B, Jordi M, Milagros R. Victor VM. Mitochondria, the NLRP3 inflammasome, and Sirtuins in type 2 diabetes: new therapeutic targets. Antioxid Redox Signal. (2018) 29:749–91. doi: 10.1089/ars.2017.7313
97. Safiedeen Z, Andriantsitohaina R, Martinez MC. Dialogue between endoplasmic reticulum and mitochondria as a key actor of vascular dysfunction associated to metabolic disorders. Int J Biochem Cell Biol. (2016) 77:10–4. doi: 10.1016/j.biocel.2016.05.011
98. Zhang Y, Wang C, Jin Y, Yang Q, Meng Q, Liu Q, et al. Activating the PGC-1 α /TERT pathway by catalpol ameliorates atherosclerosis via modulating ROS production, DNA damage, and telomere function: implications on mitochondria and telomere link. Oxid Med Cell Longev. (2018) 2018:2876350. doi: 10.1155/2018/2876350
99. Wang D, Du Y, Xu H, Pan H, Wang R. Paeonol protects mitochondrial injury and prevents pulmonary vascular remodeling in hypoxia. Respir Physiol Neurobiol. (2019) 268:103252. doi: 10.1016/j.resp.2019.103252
100. Nizamutdinova IT, Jin YC, Kim JS, Yean MH, Kang SS, Kim YS. Paeonol and paeoniflorin, the main active principles of Paeonia albiflora, protect the heart from myocardial ischemia/reperfusion injury in rats. Planta Med. (2008) 74:14–8. doi: 10.1055/s-2007-993775
101. Li X, Huang X, Tang Y, Zhao F, Cao Y, Yin L, et al. Assessing the pharmacological and therapeutic efficacy of traditional Chinese medicine Liangxue Tongyu prescription for intracerebral hemorrhagic stroke in neurological disease models. Front Pharmacol. (2018) 9:1169. doi: 10.3389/fphar.2018.01169
102. Wang M, Kaufman RJ. Protein misfolding in the endoplasmic reticulum as a conduit to human disease. Nature. (2016) 529:326–35. doi: 10.1038/nature17041
103. Yang X, Srivastava R, Howell SH, Bassham DC. Activation of autophagy by unfolded proteins during endoplasmic reticulum stress. Plant J. (2016) 85:83–95. doi: 10.1111/tpj.13091
104. Iurlaro R, Muñoz-Pinedo C. Cell death induced by endoplasmic reticulum stress. FEBS J. (2016) 283:2640–52. doi: 10.1111/febs.13598
105. Yang Y, Zhou Q, Gao A, Chen L, Li L. Endoplasmic reticulum stress and focused drug discovery in cardiovascular disease. Clin Chim Acta. (2020) 504:125–37. doi: 10.1016/j.cca.2020.01.031
106. Zhang C, Syed TW, Liu R, Yu J. Role of endoplasmic reticulum stress, Autophagy, and inflammation in cardiovascular disease. Front Cardiovasc Med. (2017) 4:29. doi: 10.3389/fcvm.2017.00029
107. Yang S, Wu M, Li X, Zhao R, Zhao Y, Liu L, et al. Role of endoplasmic reticulum stress in atherosclerosis and its potential as a therapeutic target. Oxid Med Cell Longev. (2020) 2020:9270107. doi: 10.1155/2020/9270107
108. Di Pasquale E, Condorelli G. Endoplasmic reticulum stress at the crossroads of progeria and atherosclerosis. EMBO Mol Med. (2019) 11:e10360. doi: 10.15252/emmm.201910360
109. Ker Woon C, Rais MM, Siang LY, Jian L, Dharmani M, Wai LC, et al. Paeonol protects against endoplasmic reticulum stress-induced endothelial dysfunction via AMPK/PPARδ signaling pathway. Biochem Pharmacol. (2016) 116:51–62. doi: 10.1016/j.bcp.2016.07.013
110. Woon CK, Siang LY, Dharmani M, Rais MM. Chronic treatment with paeonol improves endothelial function in mice through inhibition of endoplasmic reticulum stress-mediated oxidative stress. PLoS ONE. (2017) 12:e0178365. doi: 10.1371/journal.pone.0178365
111. Mialet-Perez J, Vindis C. Autophagy in health and disease: focus on the cardiovascular system. Essays Biochem. (2017) 61:721–32. doi: 10.1042/EBC20170022
112. Ren J, Zhang Y. Targeting autophagy in aging and aging-related cardiovascular diseases. Trends Pharmacol Sci. (2018) 39:1064–76. doi: 10.1016/j.tips.2018.10.005
113. Bravo-San Pedro JM, Kroemer G, Galluzzi L. Autophagy and mitophagy in cardiovascular disease. Circ Res. (2017) 120:1812–24. doi: 10.1161/CIRCRESAHA.117.311082
114. Dong Z, Xie X, Sun Y, Wu H, Dai M. Paeonol prevents lipid metabolism dysfunction in palmitic acid-induced HepG2 injury through promoting SIRT1-FoxO1-ATG14-dependent autophagy. Eur J Pharmacol. (2020) 880:173145. doi: 10.1016/j.ejphar.2020.173145
115. Li C, Yang L, Wu H, Dai M. Paeonol inhibits oxidized low-density lipoprotein-induced vascular endothelial cells autophagy by upregulating the expression of miRNA-30a. Front Pharmacol. (2018) 9:95. doi: 10.3389/fphar.2018.00095
116. Tsai CF, Su HH, Chen KM, Liao JM, Yao YT, Chen YH, et al. Paeonol protects against myocardial ischemia/reperfusion-induced injury by mediating apoptosis and autophagy crosstalk. Front Pharmacol. (2020) 11:586498. doi: 10.3389/fphar.2020.586498
117. Liu Y, Song A, Wu H, Sun Y, Dai M. Paeonol inhibits apoptosis of vascular smooth muscle cells via up-regulation of autophagy by activating class III PI3K/Beclin-1 signaling pathway. Life Sci. (2021) 264:118714. doi: 10.1016/j.lfs.2020.118714
118. Zhu L, Li N, Sun L, Zheng D, Shao G. Non-coding RNAs: the key detectors and regulators in cardiovascular disease. Genomics. (2021) 113:1233–46. doi: 10.1016/j.ygeno.2020.10.024
119. Barwari T, Joshi A, Mayr M. MicroRNAs in cardiovascular disease. J Am Coll Cardiol. (2016) 68:2577–84. doi: 10.1016/j.jacc.2016.09.945
120. Huang CK, Kafert-Kasting S, Thum T. Preclinical and clinical development of noncoding RNA therapeutics for cardiovascular disease. Circ Res. (2020) 126:663–78. doi: 10.1161/CIRCRESAHA.119.315856
121. Shi X, Xie X, Sun Y, He H, Huang H, Liu Y, et al. Paeonol inhibits NLRP3 mediated inflammation in rat endothelial cells by elevating hyperlipidemic rats plasma exosomal miRNA-223. Eur J Pharmacol. (2020) 885:173473. doi: 10.1016/j.ejphar.2020.173473
122. Jin M, Qian Z, Yin J, Xu W, Zhou X. The role of intestinal microbiota in cardiovascular disease. J Cell Mol Med. (2019) 23:2343–50. doi: 10.1111/jcmm.14195
123. Li X, Wu D, Niu J, Sun Y, Wang Q, Yang B, et al. Intestinal flora: a pivotal role in investigation of traditional Chinese medicine. Am J Chin Med. (2021) 49:237–68. doi: 10.1142/S0192415X21500130
124. You Y, Luo L, You Y, Lin Y, Hu H, Chen Y, et al. Shengmai Yin formula modulates the gut microbiota of spleen-deficiency rats. Chin Med. (2020) 15:114. doi: 10.1186/s13020-020-00394-y
125. Li L, Cui H, Li T, Qi J, Chen H, Gao F, et al. Synergistic effect of berberine-based Chinese medicine assembled nanostructures on diarrhea-predominant irritable bowel syndrome in vivo. Front Pharmacol. (2020) 11:1210. doi: 10.3389/fphar.2020.01210
126. Ou Y, Zhang C, Yao M, Wang L. Gut flora: novel therapeutic target of Chinese medicine for the treatment of cardiovascular diseases. Evid Based Complement Alternat Med. (2019) 2019:3719596. doi: 10.1155/2019/3719596
127. Guo Y, Du Y, Xie L, Pu Y, Yuan J, Wang Z, et al. Effects of paeonol and gastroretention tablets of paeonol on experimental gastric ulcers and intestinal flora in rats. Inflammation. (2020) 43:2178–90. doi: 10.1007/s10753-020-01285-y
128. He X, Cai Q, Li J, Guo W. Involvement of brain-gut axis in treatment of cerebral infarction by β-asaron and paeonol. Neurosci Lett. (2018) 666:78–84. doi: 10.1016/j.neulet.2017.12.036
129. Hu RF, Fang CW, Zou AF, Mei KK, Tang JH, Han LL. Studies on absorption kinetics of paeonol and paeonol-beta-CD in rat's intestines. China J Chin Mater Medica. (2008) 33:35–7. doi: 10.3321/j.issn:1001-5302.2008.01.009
130. Li WL, Wang XD, Ji YB, Hu ZT, Wang J, Ren XL. Pharmacokinetics of effective constituent in Jinkui Shenqi Pill. Chin Tradit Patent Med. (2008) 30:1432–5. doi: 10.3969/j.issn.1001-1528.2008.10.009
131. Liao ZG, Liang XL, Jiang QY, Ping QN. Determination of paeonol in Beagle dog plasma administered with Guizhi Fuling capsule by HPLC and pharmacokinetic studies. West China J Pharmaceut Sci. (2007) 22:621–3. doi: 10.13375/j.cnki.wcjps.2007.06.021
132. Hu X, Ding L, Cao S, Cheng L, Wang K, Guang C, et al. Pharmacokinetics, tissue distribution and excretion of paeonol and its major metabolites in rats provide a further insight into paeonol effectiveness. Front Pharmacol. (2020) 11:190–190. doi: 10.3389/fphar.2020.00190
133. Qun C, Shimin LI. Distribution of paeonol in plasma and brain tissue of mice. China Pharmacy. (2009) 20:1620–1.
134. Wu XJ, Li GX, Zhao LM. Determination of paeonol in plasma and urine by RP-HPLC. Chin Pharmaceut J. (2007) 42:863–5. doi: 10.3321/j.issn:1001-2494.2007.11.018
135. Hu X, Ding L, Li W, Qiu F. Pharmacokinetics of paeonol and its metabolites after oral administration of Moutan Cortex in rats. Chin Tradit Herbal Drugs. (2019) 50:6017–23. doi: 10.7501/j.issn.0253-2670.2019.24.015
136. Xie Y, Zhou H, Wong YF, Xu HX, Jiang ZH, Liu L. Study on the pharmacokinetics and metabolism of paeonol in rats treated with pure paeonol and an herbal preparation containing paeonol by using HPLC-DAD-MS method. J Pharm Biomed Anal. (2008) 46:748–56. doi: 10.1016/j.jpba.2007.11.046
137. Li H, Wang S, Zhang B, Xie Y, Wang J, Yang Q, et al. Influence of co-administered Danshensu on pharmacokinetic fate and tissue distribution of paeonol in rats. Planta Med. (2012) 78:135–40. doi: 10.1055/s-0031-1280269
138. Wu J, Wang BJ, Wei CM, Kong XL, Guo RC. Pharmacokinetics and bioequivalence of paeonol capsules and tablets by the HPLC method. J Shandong Univ Health Sci. (2007) 45:726–9. doi: 10.3969/j.issn.1671-7554.2007.07.021
139. Chen X, Lu Y, Du S, Xu B, Wang S, Zhai Y, et al. In situ and in vivo study of nasal absorption of paeonol in rats. Int J Mol Sci. (2010) 11:4882–90. doi: 10.3390/ijms11124882
140. Ma LY, Xu XD, Zhang Q, Miao JH, Tang BL. Paeonol pharmacokinetics in the rat following im administration. Eur J Drug Metab Pharmacokinet. (2008) 33:133–6. doi: 10.1007/BF03191109
141. Ma LY, Miu JH, Xu XD, Tang BL, Zhang Q. Pharmacokinetic features and absolute bioavailability of paeonol in conscious rat. Lishizhen Med Mater Medica Res. (2009) 20:413–4. doi: 10.3969/j.issn.1008-0805.2009.02.084
142. Chen ZX, Li B, Liu T, Wang X, Zhu Y, Wang L, et al. Evaluation of paeonol-loaded transethosomes as transdermal delivery carriers. Eur J Pharmaceut Sci. (2017) 99:240–5. doi: 10.1016/j.ejps.2016.12.026
143. He RX, Ye X, Li R, Chen W, Ge T, Huang TQ, et al. PEGylated niosomes-mediated drug delivery systems for Paeonol: preparation, pharmacokinetics studies and synergistic anti-tumor effects with 5-FU. J Liposome Res. (2017) 27:161–70. doi: 10.1080/08982104.2016.1191021
144. Li SS, Li GF, Liu L, Li H, Jiang X, Li XL, et al. Optimization of paeonol-loaded microparticle formulation by response surface methodology. J Microencapsul. (2015) 32:677–86. doi: 10.3109/02652048.2014.918664
145. Chu KD, Chen LD, Xu W, Li H, Zhang YQ, Xie WR, et al. Preparation of a paeonol-containing temperature-sensitive in situ gel and Its preliminary efficacy on allergic rhinitis. Int J Mol Sci. (2013) 14:6499–515. doi: 10.3390/ijms14036499
146. Wu RG, Dai JD, Wu FG, Zhang XH, Li WF, Wang YR. Competitive molecular interaction among paeonol-loaded liposomes: differential scanning calorimetry and synchrotron X-ray diffraction studies. Int J Pharm. (2012) 438:91–7. doi: 10.1016/j.ijpharm.2012.08.052
147. Luo M, Shen Q, Chen J. Transdermal delivery of paeonol using cubic gel and microemulsion gel. Int J Nanomedicine. (2011) 6:1603–10. doi: 10.2147/IJN.S22667
148. Liu JY, Han Y, Hu JH, Wang ZT, Chen KX. The preparation of paeonol transdermal delivery systems based on the microemulsion-based gels and its pharmacokinetics characters. Yaoxue Xuebao. (2012) 47:244–9. doi: 10.16438/j.0513-4870.2012.02.007
149. Jiang X, Liu L, Li SS, Zhang B, Li XL, Liu ZG, et al. Preparation of paeonol transdermal delivery systems based on proniosomes-based ointment and its pharmacokinetics characters. China J Chin Mater Medica. (2014) 39:2131–5. doi: 10.4268/cjcmm20141137
150. Shi J, Wang F, Teng XF, Huang SH. Study on stability and cutaneous permeation kinetics in vitro of positive-ionized liposome gel containing paeonol. J Chin Med Mater. (2012) 35:803–7. doi: 10.13863/j.issn1001-4454.2012.05.022
151. Yao JJ, Zhang YX, Hu QM, Zeng DC, Hua F, Meng W, et al. Optimization of paeonol-loaded poly(butyl-2-cyanoacrylate) nanocapsules by central composite design with response surface methodology together with the antibacterial properties. Eur J Pharmaceut Sci. (2017) 101:189–99. doi: 10.1016/j.ejps.2017.01.028
Keywords: Paeonia suffruticosa, paeonol, traditional Chinese medicine, atherosclerotic cardiovascular disease, review, pharmacology, mechanism
Citation: Wu M, Yu Z, Li X, Zhang X, Wang S, Yang S, Hu L and Liu L (2021) Paeonol for the Treatment of Atherosclerotic Cardiovascular Disease: A Pharmacological and Mechanistic Overview. Front. Cardiovasc. Med. 8:690116. doi: 10.3389/fcvm.2021.690116
Received: 02 April 2021; Accepted: 25 June 2021;
Published: 21 July 2021.
Edited by:
Tzong-Shyuan Lee, National Taiwan University, TaiwanReviewed by:
Vicente Andres, Spanish National Centre for Cardiovascular Research, SpainPatricia B. Maguire, University College Dublin, Ireland
Copyright © 2021 Wu, Yu, Li, Zhang, Wang, Yang, Hu and Liu. This is an open-access article distributed under the terms of the Creative Commons Attribution License (CC BY). The use, distribution or reproduction in other forums is permitted, provided the original author(s) and the copyright owner(s) are credited and that the original publication in this journal is cited, in accordance with accepted academic practice. No use, distribution or reproduction is permitted which does not comply with these terms.
*Correspondence: Longtao Liu, liulongtao1976@126.com
†These authors have contributed equally to this work and share first authorship