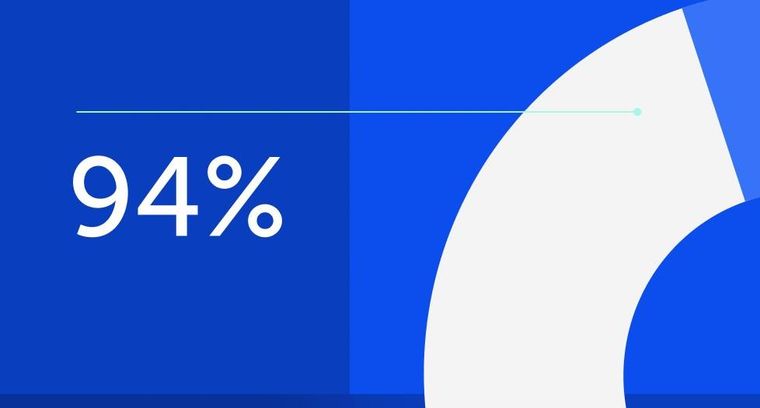
94% of researchers rate our articles as excellent or good
Learn more about the work of our research integrity team to safeguard the quality of each article we publish.
Find out more
ORIGINAL RESEARCH article
Front. Cardiovasc. Med., 20 July 2021
Sec. Pediatric Cardiology
Volume 8 - 2021 | https://doi.org/10.3389/fcvm.2021.687150
Objective: Adverse factors of postoperative hemodynamic and myocardial performance remain largely unexplored in children with congenital heart disease following cardiopulmonary bypass due to technical limitations. Pressure recording analytical method (PRAM) is a continuous hemodynamic and myocardial performance monitoring technique based on beat-to-beat arterial pressure waveform. Using PRAM, we examined the temporal trends and adverse factors, in clinical management, of these performances.
Methods: We monitored blood pressure, cardiac index, cardiac cycle efficiency (CCE), dP/dTmax, and systematic vascular resistance index in 91 children (aged 186 ± 256 days) during their first 48 h after cardiopulmonary bypass. Above parameters, inotropic and vasoactive drug dosages, and serum lactate were recorded 3-hourly. NT-proBNP was measured daily.
Results: CCE and dP/dTmax gradually increased (Ps < 0.0001), while systematic vascular resistance index, diastolic blood pressure and inotrope dosages decreased (Ps < 0.0001) over time. Cardiac index, systolic blood pressure, and heart rate did not change significantly (Ps ≥ 0.231). Patients undergoing deep hypothermic circulatory arrest had significantly higher heart rate and lower CCE (Ps ≤ 0.006) over time. Multivariate analyses indicated that epinephrine dose significantly correlated with systolic blood pressure, cardiac index, CCE, and dP/dTmax after polynomial transformation, with the peak ranging from 0.075 to 0.097.
Conclusions: Systemic hemodynamic and myocardial performance gradually improved in the first 48 h after cardiopulmonary bypass without the “classic” nadir at 9–12 h. Deep hypothermic circulatory arrest and higher epinephrine doses were adversely associated with these performances. CCE, rather than cardiac index or other common-used parameters, was the most sensitive and consistent indicator.
Hemodynamic monitoring is the definite cornerstone of the postoperative management in children with congenital heart disease (CHD) undergoing cardiopulmonary bypass surgery (CPB) (1). The early post-CPB period is characterized by myocardial injury, reduced cardiac output, and hemodynamic instability (1), requiring prompt and adequate adjustment of treatments, e.g., inotropic and vasoactive drugs. Cardiac output-guided hemodynamic therapy algorithm has been shown to improve outcomes in adults following cardiac surgery (2). No such study has been conducted in post-CPB children due largely to the technical limitations to directly monitor hemodynamics in young children and infants. The risk factors of postoperative hemodynamic and myocardial performance remain largely to be explored.
Since the invention of the Fick principle in 1870 (3), there have been intermittent technological developments to measure cardiac output, namely, Swan-Ganz catheter and thermodilution method in the 1970's (4), respiratory mass spectrometer in the 1990's (5–7). Thermodilution has well-known limitations when applied to post-CPB children (8). The presence of intracardiac shunt and tricuspid and pulmonary regurgitation can significantly affect its accuracy. Additionally, repeated cold saline injections are also undesirable in the fragile condition of cardiac function after CPB. It has been rarely used in children after CPB (1). Respiratory mass spectrometry is a desirable technique to measure oxygen consumption. Combined with arterial and venous blood gases, it is able to derive almost all elements of hemodynamics in varied circulations (5–7, 9). However, it is technically and timely highly demanding and hardly used outside of clinical research settings (8).
More recently, there have been several systems developed to directly monitor cardiac output and other hemodynamic parameters based on the arterial pulse contour analysis methods such as PiCCO2 and MostCare, etc (10). They are minimally invasive and in a real-time fashion, which is fundamental in post-CPB children. PiCCO2 combines pulse contour method with thermodilution for calibration, thereby inheriting the limitations (3). MostCare (Vygon Vytech, Padova, Italy) uses the pressure recording analytical method (PRAM) without the need for calibration (11, 12). It has been validated against the Fick method in pediatric patients (13) and used in children during CPB (14, 15), not yet in post-CPB children.
By using PRAM, we aimed to examine the temporal trends of systemic hemodynamic and myocardial performance parameters of children with CHD during the first 48 post-CPB hours, and more importantly to identify adverse factors of these parameters in routine clinical management.
After ethics approval (No. 46201), 91 children with CHD undergoing CPB were enrolled from July 2019 to June 2020. The informed consent was waived since PRAM monitoring by MostCare is our clinical routine. More complex CHD patients were selected daily when MostCare devices were available. Patients with other congenital anomalies or previous CPB were excluded.
Standard procedures were performed (16). DHCA (time ranged 15–26 min, median 18 min) was used in 17 patients to repair the coarctation of the aorta with ventricular septal defect or interruption of the aortic arch.
Patients receive time-cycled pressure control/pressure support ventilation on arrival in the CICU, followed by non-invasive ventilation when appropriate. Ventilation settings are adjusted to maintain a PaCO2 at 35–45 mmHg and a SaO2 between 70 and 100% according to palliative or complete repair. The rectal temperature is maintained at 36–37°C. Sedation consists of continuous intravenous infusion of sufentanil (0.2 μg/kg/min) and dexmedetomidine (2 μg/kg/min) and intermittent injections of midazolam (0.1 mg/kg). Hematocrit is maintained at 34–45%. Inotropic and vasoactive drugs include dopamine, milrinone, and epinephrine to maintain arterial blood pressure (systolic pressure 60–90 and 90–105 mmHg and diastolic pressure of 40–60 and 55–66 mmHg in neonates and children, respectively). Despite the protocol, management varied among clinicians.
A MostCare device based on PRAM was used to monitor patient during their first 48 postoperative hours, and data were collected 3-hourly. PRAM assesses systemic hemodynamics by analyzing frequently sampled (1,000 Hz) arterial pressure waveform. In any artery, volume changes with the radial expansion in response to pressure variations, which is mutually determined by left ventricle contraction, arterial elasticity, and backward pressure reflections (17). To estimate stroke volume, the whole pulsatile area under the systolic portion of the pressure curve is measured. Arterial impedance is determined from both systolic and diastolic phases of the pressure waveform, hence no calibration or preloaded data is necessary (17). PRAM measures heart rate, systolic blood pressure (SBP), diastolic blood pressure (DBP), cardiac index (CI), systemic vascular resistance index (SVRI), the maximal slope of systolic upstroke (dP/dTmax), and cardiac cycle efficiency (CCE) (11). dP/dTmax indicates the maximal rate of the rise of arterial pressure manifesting left ventricular pressure and is used as an index of myocardial contractility (11). CCE is uniquely derived by PRAM and calculated according to the ratio of systolic energetic performance to the total energetic expenditure of the heartbeat using the following formula (18):
W(t)sys is the power of the systolic stage of the heartbeat, W(t)beat is the power of the whole cycle of the heartbeat, and K(t) is the ratio of mean pressure expected and mean pressure measured.
CCE evaluates the compensating interplay of different cardiovascular system compartments, i.e., left and right ventricular contractility, pre- and after-load, heart rate, reflected waves, as well as the elasticity of great arteries and the ventricular-arterial coupling (18). It ranges from negative values to +1. The negative values indicate the activation of in vivo compensation mechanisms supporting compartments of the cardiovascular system that do not work properly, while a higher CCE indicates a better overall cardiovascular functioning (18).
Demographics, STAT categories (19), operative data, and clinical outcomes were recorded (Table 1). Doses of inotropic and vasoactive drugs and serum lactate were recorded 3-hourly. NT-proBNP was measured daily.
Data were described as mean ± SD, median (range), or frequency (%) when appropriate. Mixed linear regression for repeated measures was used to (1) analyze the temporal trend of variables; (2) compare the difference between DHCA group and non-DHCA group in levels and trends with the analysis of the group effect and interaction between time and group. The parameter estimate and P-value of the group effect (Pgroup) indicate the difference in the level of each measure between groups. Those of the time and group interaction (Pgroup × time) indicate the difference in the trend of each measure between groups; (3) analyze correlations between measures. The fixed predictors were clinical variables, and outcomes were hemodynamic and myocardial variables. Polynomial transformation of the drug dose was tested regarding the best fit for the drug dose, in which the parameter estimate and P-value of dose (parameter estimatedose and Pdose) indicated lower doses, those for time2 or dose2 indicated higher doses. The peak value in the polynomial trend was determined by the following equation: Xpeak = parameter estimatedose/(-2*). All analyses were performed with SAS 9.4.
Table 1 presents the demographic and clinical data of the cohort.
During the first 48 post-CPB hours, CCE and dP/dTmax gradually and significantly increased (Ps < 0.0001), while SVRI and DBP decreased (Ps ≤ 0.0003). The doses of epinephrine, dopamine, and milrinone decreased significantly (Ps < 0.0001). CI, SBP, and heart rate did not change significantly (Ps ≥ 0.231) (Figure 1 and Supplementary Table 1).
Figure 1. Temporal trends of systemic hemodynamic and myocardial status and doses of inotropic and vasoactive drugs during the first 48 h after CPB (n = 91). The mean value is presented, and the error bar represents the standard deviation. CCE, cardiac cycle efficiency; CI, cardiac index; DBP, diastolic blood pressure; dP/dTmax, the maximal slope of systolic upstroke; SBP, systolic blood pressure; SVRI, systematic vascular resistance.
Patients undergoing DHCA (n = 17), compared with those without (n = 74), had or trended to have significantly higher heart rate (Pgroup = 0.006) and SVRI (Pgroup = 0.064), and lower CCE (Pgroup = 0.001) and dP/dTmax (Pgroup = 0.099) over time. They received significantly higher epinephrine (Pgroup = 0.020) and lower dopamine (Pgroup = 0.002), but were not different in milrinone (Pgroup = 0.479) (Figure 2 and Supplementary Table 2). After adjusted for the doses of inotropic drugs, the differences remained significant in heart rate (Pgroup = 0.031) and CCE (Pgroup = 0.006), but not in dP/dTmax (Pgroup = 0.395) and SVRI (Pgroup = 0.208) (Supplementary Table 1). CI did not differ between groups either including inotropic and vasoactive drug doses or not (P > 0.280).
Figure 2. Comparison of the temporal trends of the systemic hemodynamic and myocardial status between DHCA (n = 17) and non-DHCA groups (n = 74) during the first 48 h after CPB. The mean value is presented, and the error bar represents the standard deviation. CCE, cardiac cycle efficiency; CI, cardiac index; DBP, diastolic blood pressure; dP/dTmax, the maximal slope of systolic upstroke; SBP, systolic blood pressure; SVRI, systematic vascular resistance.
As presented in Table 2, after adjusted for time, epinephrine dose significantly and positively correlated with heart rate, SBP, DBP, SVRI, dP/dTmax, lactate, and NT-proBNP (Ps ≤ 0.002). It significantly correlated with CCE after polynomial transformation, being positive at lower doses <0.083 mcg/kg/min (parameter estimate dose = 2.63 Pdose <0.0001), and negative at higher doses ( = −15.88, = 0.001). Dopamine dose significantly and positively correlated with CCE (P < 0.0001), negatively correlated with SVRI and NT-proBNP (Ps ≤ 0.020). Milrinone dose significantly and positively correlated with CI (P = 0.033), negatively correlated with SVRI and lactate (Ps ≤ 0.031). When tested as the dependent variable, epinephrine dose significantly and positively correlated STAT category and CPB time (parameter estimate = 0.01 and P = 0.016 for STAT; parameter estimate = 0.0002 and P = 0.016 for CPB time).
Table 2. Univariate and multivariate analyses of the correlations between inotropic and vasoactive drug doses and hemodynamic parameters in 91 children during the first 48 h after cardiopulmonary bypass.
Additionally, CCE significantly correlated with NT-proBNP (parameter estimate = −9072.59 and P < 0.0001) but not with lactate (P = 0.627). No significant correlations were found between CI and NT-proBNP (P = 0.764) or lactate (P = 0.721).
As presented in Table 2, epinephrine dose remained significantly correlated with CCE after polynomial transformation ( =2.86 and −14.70, respectively, Ps ≤ 0.015 for both). Its correlations with other variables were different from the linear ones shown in the univariate analyses. After polynomial transformation, epinephrine dose significantly correlated with SBP ( = 125.19 and −806.87, respectively, Ps ≤ 0.001 for both), CI ( = 3.46 and −22.95, respectively, Ps ≤ 0.041 for both), and dP/dTmax ( = 3.17 and −17.03, respectively, Ps ≤ 0.001 for both). SBP, CI, CCE, and dP/dTmax peaked at an epinephrine dose of 0.078, 0.075, 0.097, and 0.093 mcg/kg/min, respectively. Epinephrine dose linearly and positively correlated with heart rate, DBP, SVRI, lactate, and NT-proBNP (Ps ≤ 0.003). Dopamine dose positively correlated with CCE (P = 0.010). Age significantly and negatively correlated with heart rate, and positively with SBP and CCE (Ps ≤ 0.014). CPB time significantly and negatively correlated with CCE (P = 0.036). No significant correlation was found between other clinical and hemodynamic variables (Ps ≥ 0.074).
By monitoring children with CHD during the first 48 post-CPB hours using PRAM, we found the followings: (1) systemic hemodynamics and myocardial status gradually improved without the “classic” nadir at 9–12 h; (2) patients undergoing DHCA had significantly higher heart rate and lower CCE; (3) higher epinephrine doses were adversely associated with overall systemic hemodynamics and myocardial performance, indicated by lower SBP, CI, dP/dTmax and CCE, and higher heart rate, DBP, SVRI, lactate, and NT-proBNP; (4) CCE was the most sensitive and consistent indicator among all PRAM parameters.
Wernovsky et al. in 1995 compared the postoperative hemodynamic profile in infants undergoing the arterial switch operation using low-flow CPB or DHCA (1). No significant between-group difference was found in blood pressure, CI, and SVRI measured by the thermodilution technique. They reported that, in both groups, CI reached a nadir, while SVRI reached a peak, at 9–12 h postoperatively and returned to baselines 24 h after surgery. They attributed the nadir to ischemic/reperfusion and systemic inflammatory response. This temporal trend has been widely used to guide clinical management. However, data from the present study showed a gradual improvement in overall systemic hemodynamics and myocardial status during the 48-h postoperative period, as indicated by the increase in CCE and dP/dTmax and the decrease in SVRI and inotropic and vasoactive drug doses; CI did not change significantly and no other parameters showed the nadir. This profile is consistent with our other studies that used more advanced monitoring techniques, such as respiratory mass spectrometry to oxygen consumption combined with the Fick principle in the past two decades, in which CI gradually increased over 72 h in post-CPB children including neonates following the Norwood procedure and infants with less complex CHDs following complete repair (5, 6). Other than these studies, few have addressed the temporal trend of CI in post-CPB children (20). The difference between the previous study (1) and ours may be due to a couple of reasons as follows. First, our cohort included a heterogeneous group of less complex CHDs with a lower proportion of neonatal surgery (18.7%) compared to the previous study in a homogeneous group of neonates with transposition of the great arteries undergoing arterial switch operation (1). Second, intraoperative management has improved significantly in the later era, e.g., the application of modified ultrafiltration (21), which reduces the systemic inflammatory response and improves ventricular function and oxygen transport (5, 22). The gradual hemodynamic improvement coincides with the reciprocal profiles of circulating cytokines and C-reactive protein (5). Such hemodynamic profiles should be kept in mind when managing post-CPB children in the current era.
Another interesting finding was the inferior myocardial status, indicated by lower CCE and higher heart rate and epinephrine doses, among DHCA patients compared to those undergoing CPB (Figure 2). Neither perfusion technique perfused the heart (with lungs). Thus, this difference may be attributed to the difference between systemic and multi-organ ischemia/reperfusion caused by DHCA and that of a single organ by CPB (23). Correspondingly, rats undergoing DHCA had greater multi-organ vascular endothelial dysfunction and myocardial apoptosis as compared to CPB (24). Intestinal ischemia/reperfusion may lead to local and systemic inflammatory responses mediated by intestinal mast cells (25). Besides, deeper hypothermia used in DHCA may also contribute to the difference (26), as a result of greater myocardial excitation-contraction coupling disruption (27).
Epinephrine is widely used in children after CPB (20), with a wide dose range from 0.01 to 0.3 mcg/kg/min, and usually <0.1 mcg/kg/min (28). However, few studies evaluated its hemodynamic effects in children following CPB. Our data revealed that epinephrine was associated with a favorable hemodynamic and myocardial performance at lower doses (<0.08–0.1 mcg/kg/min), indicated by higher SBP, CCE, dP/dTmax, and CI; at higher doses, these effects became adverse and counterintuitive.
The causal relationship between epinephrine and hemodynamics remains to be clarified, and it is further confounded by the fact that clinicians tend to use higher epinephrine doses in sicker patients, which is also the case in our patients. The favorable effects of low doses shown in our data are consistent with the finding in post-CPB adults. Stepwise increases of epinephrine from 0.02 to 0.08 mcg/kg/min linearly increased CI and decreased SVRI (29). When a wider dose range was tested in anesthetized piglets, the curvilinear effect was observed (30). Several mechanisms may underlie these observations. β-adrenergic overstimulation may cause tachycardia and cardiac dysfunction impairing cardiac filling and contractility (31). At the same time, α-adrenergic overstimulation may cause peripheral (32) and coronary arterial vasoconstriction (33), leading to increased SVRI and cardiac afterload, and reduced myocardial oxygen delivery (34). The latter may be further compounded by tachycardia result from increased myocardial oxygen consumption.
Both dopamine and milrinone doses positively correlated with hemodynamic and myocardial status. We previously reported that dopamine at 5 μg/kg/min in neonates after the Norwood procedure (STAT category 5) increased systemic and myocardial oxygen consumption but failed to increase CI and oxygen delivery, therefore exerting an adverse effect on systemic and myocardial oxygen transport (7). The patient population in this study is distinct from the previous study, with much less complex CHD (STAT category 1–4) and older age ranging 47–208 days (median 104), and they may have more cardiac function reserve and less stimulated increase in oxygen consumption due to less brown fat tissue (35). The beneficial effects of milrinone have been well-documented (36).
Finally, CCE significantly and positively correlated with NT-proBNP, while CI did not, consistent with previous findings in adults (12, 37). Taken together, CCE, rather than CI or other parameters, was most consistent and sensitive to reflect the hemodynamic effects of the clinical factors examined in this study. While CI is the final cardiac outcome from the complex interactions among the endogenous cardiac and neurohumoral responses and the exogenous inotropic and vasoactive drugs (38), CCE reflects the cost of cardiovascular work to maintain such a CI.
The observational nature of this study has several limitations. First, the causal relationship between epinephrine dose and hemodynamic outcomes showed by regression analysis remains speculative. Second, the epinephrine doses were relatively high and often combined with dopamine in our center. The situation is suboptimal but allowed us to reveal the curvilinear effect of epinephrine. The cut-off value might be different if not combined with dopamine or other inotropic and vasoactive drugs. This might also be applicable to the effect of dopamine if used along. Third, our cohort included a heterogeneous group of less complex CHDs with a lower proportion of neonatal surgery compared to contemporary patient populations from the advanced centers. A larger multi-center cohort study, including neonatal surgeries and complex cases and using more advanced techniques, is warranted to confirm our findings, in particular to the temporal trends of hemodynamics.
In CHD children early after CPB, systemic hemodynamic and myocardial performance improved gradually during the first 48 h without the “classic” nadir at 9–12 h. These performances were worse in patients undergoing DHCA and adversely associated with higher doses of epinephrine >0.08–0.10 mcg/kg/min. CCE, rather than CI or other parameters, was most consistent and sensitive to reflect patient's hemodynamic status.
The raw data supporting the conclusions of this article will be made available by the authors, without undue reservation.
The studies involving human participants were reviewed and approved by Guangzhou Women and Children's Hospital. Written informed consent from the participants' legal guardian/next of kin was not required to participate in this study in accordance with the national legislation and the institutional requirements.
XL and YL has contributed to the conducting and reporting of the work described in the article. YC, JianL, LL, LM, and MZ has contributed to the conducting of the work. XC has contributed to the planning and conducting of the research. JiaL has contributed to the planning, conducting, and reporting of the research. All authors contributed to the article and approved the submitted version.
The Key-Area Research and Development Program of Guangdong Province (No. 2019B020227001). JL's start-up fund provided by Guangzhou Women and Children's Medical Center.
The authors declare that the research was conducted in the absence of any commercial or financial relationships that could be construed as a potential conflict of interest.
The Supplementary Material for this article can be found online at: https://www.frontiersin.org/articles/10.3389/fcvm.2021.687150/full#supplementary-material
1. Wernovsky G, Wypij D, Richard J, John M, Frank H, Paul H, et al. Postoperative course and hemodynamic profile after the arterial switch operation in neonates and infants. Circulation. (1995) 92:2226–35. doi: 10.1161/01.CIR.92.8.2226
2. Osawa EA, Rhodes A, Landoni G, Galas FRBG, Fukushima JT, Park CHL, et al. Effect of perioperative goal-directed hemodynamic resuscitation therapy on outcomes following cardiac surgery: a randomized clinical trial and systematic review. Crit Care Med. (2016) 44:724–33. doi: 10.1097/CCM.0000000000001479
3. Fick A. Über die Messung des Blutquantums in den Herzventrikeln. Sitzungsberichte der Physiol Gesellschaft zu Würzbg. (1870) 2:16–17.
4. Frone A, Ganz V. Measurement of flow in single blood vessels including cardiac output by local thermodilution. Circ Res. (1960) 8:175–82. doi: 10.1161/01.RES.8.1.175
5. Li J, Hoschtitzky A, Allen ML, Elliott MJ, Redington AN. An analysis of oxygen consumption and oxygen delivery in euthermic infants after cardiopulmonary bypass with modified ultrafiltration. Ann Thorac Surg. (2004) 78:1389–96. doi: 10.1016/j.athoracsur.2004.02.032
6. Li J, Zhang G, McCrindle BW, Holtby H, Humpl T, Cai S, et al. Profiles of hemodynamics and oxygen transport derived by using continuous measured oxygen consumption after the Norwood procedure. J Thorac Cardiovasc Surg. (2007) 133:441–8. doi: 10.1016/j.jtcvs.2006.09.033
7. Li J, Zhang G, Holtby H, Humpl T, Caldarone CA, Van Arsdell GS, et al. Adverse effects of dopamine on systemic hemodynamic status and oxygen transport in neonates after the Norwood procedure. J Am Coll Cardiol. (2006) 48:1859–64. doi: 10.1016/j.jacc.2006.07.038
8. Li J. Evolution of the concept of oxygen transport in the critically ill, with a focus on children after cardiopulmonary bypass. Cardiol Young. (2018) 28:186–91. doi: 10.1017/S1047951117001706
9. Hoskote A, Li J, Hickey C, Erickson S, Van Arsdell G, Stephens D, et al. The effects of carbon dioxide on oxygenation and systemic, cerebral, and pulmonary vascular hemodynamics after the bidirectional superior cavopulmonary anastomosis. J Am Coll Cardiol. (2004) 44:1501–9. doi: 10.1016/j.jacc.2004.06.061
10. Grensemann J. Cardiac output monitoring by pulse contour analysis, the technical basics of less-invasive techniques. Front Med. (2018) 5:64–72. doi: 10.3389/fmed.2018.00064
11. Romagnoli S, Bevilacqua S, Lazzeri C, Ciappi F, Dini D, Pratesi C, et al. Most Care: a minimally invasive system for hemodynamic monitoring powered by the Pressure Recording Analytical Method (PRAM). HSR Proc Intensive Care Cardiovasc Anesth. (2009) 1:20–7.
12. Romano S, Olivotto I, Chiostri M, Giglioli C, Margheri M, Gensini G. Minimally invasive and noninvasive hemodynamic monitoring of the cardiovascular system: available options and future perspectives. Curr Cardiol Rev. (2006) 2:37–9. doi: 10.2174/157340306775515308
13. Alonso-Iñigo JM, Escribá FJ, Carrasco JI, Fas MJ, Argente P, Galvis JM, et al. Measuring cardiac output in children undergoing cardiac catheterization: comparison between the Fick method and PRAM (pressure recording analytical method). Pediatr Anesth. (2016) 26:1097–105. doi: 10.1111/pan.12997
14. Garisto C, Favia I, Ricci Z, Romagnoli S, Haiberger R, Polito A, et al. Pressure recording analytical method and bioreactance for stroke volume index monitoring during pediatric cardiac surgery. Pediatr Anesth. (2015) 25:143–9. doi: 10.1111/pan.12360
15. Han D, Liu Y-G, Luo Y, Li J, Ou-Yang C. Prediction of fluid responsiveness using pulse pressure variation in infants undergoing ventricular septal defect repair with median sternotomy or minimally invasive right thoracotomy. Pediatr Cardiol. (2017) 38:184–90. doi: 10.1007/s00246-016-1500-x
16. Bercovitz RS, Shewmake AC, Newman DK, Niebler RA, Scott JP, Stuth E, et al. Validation of a definition of excessive postoperative bleeding in infants undergoing cardiac surgery with cardiopulmonary bypass. J Thorac Cardiovasc Surg. (2018) 155:2112–24. doi: 10.1016/j.jtcvs.2017.12.038
17. Romano SM, Pistolesi M. Assessment of cardiac output from systemic arterial pressure in humans. Crit Care Med. (2002) 30:1834–41. doi: 10.1097/00003246-200208000-00027
18. Romano SM. Cardiac cycle efficiency: a new parameter able to fully evaluate the dynamic interplay of the cardiovascular system. Int J Cardiol. (2011) 155:326–7. doi: 10.1016/j.ijcard.2011.12.008
19. Jacobs ML, O'Brien SM, Jacobs JP, Mavroudis C, Lacour-Gayet F, Pasquali SK, et al. An empirically based tool for analyzing morbidity associated with operations for congenital heart disease. J Thorac Cardiovasc Surg. (2013) 145:1046–57. doi: 10.1016/j.jtcvs.2012.06.029
20. Roeleveld PP, de Klerk JCA. The perspective of the intensivist on inotropes and postoperative care following pediatric heart surgery: an international survey and systematic review of the literature. World J Pediatr Congenit Heart Surg. (2018) 9:10–21. doi: 10.1177/2150135117731725
21. Naik SK, Knight A, Elliott M. A prospective randomized study of a modified technique of ultrafiltration during pediatric open-heart surgery. Circulation. (1991) 84:422–31.
22. Davies MJ, Nguyen K, Gaynor JW, Elliott MJ. Modified ultrafiltration improves left ventricular systolic function in infants after cardiopulmonary bypass. J Thorac Cardiovasc Surg. (1998) 115:361–70. doi: 10.1016/S0022-5223(98)70280-6
23. Eltzschig HK, Eckle T. Ischemia and reperfusion—from mechanism to translation. Nat Med. (2011) 17:1391–401. doi: 10.1038/nm.2507
24. Cooper WA, Duarte IG, Thourani VH, Nakamura M, Wang NP, Brown WM, et al. Hypothermic circulatory arrest causes multisystem vascular endothelial dysfunction and apoptosis. Ann Thorac Surg. (2000) 69:696–702. doi: 10.1016/S0003-4975(99)01524-6
25. Karhausen J, Qing M, Gibson A, Moeser AJ, Griefingholt H, Hale LP, et al. Intestinal mast cells mediate gut injury and systemic inflammation in a rat model of deep hypothermic circulatory arrest. Crit Care Med. (2013) 41:200–10. doi: 10.1097/CCM.0b013e31827cac7a
26. Kellermann S, Janssen C, Münch F, Koch A, Schneider-Stock R, Cesnjevar RA, et al. Deep hypothermic circulatory arrest or tepid regional cerebral perfusion: impact on haemodynamics and myocardial integrity in a randomized experimental trial. Interact Cardiovasc Thorac Surg. (2018) 26:667–72. doi: 10.1093/icvts/ivx393
27. Groban L, Zapata-Sudo G, Lin M, Nelson T. Effects of moderate and deep hypothermia on Ca2+ signaling in rat ventricular myocytes. Cell Physiol Biochem. (2002) 12:101–10. doi: 10.1159/000063786
28. Jentzer JC, Hollenberg SM. Vasopressor and inotrope therapy in cardiac critical care. J Intensive Care Med. (2020) 1:1–14. doi: 10.1177/0885066620917630
29. Sato Y, Matsuzawa H, Eguchi S. Comparative study of effects of adrenaline, dobutamine and dopamine on systemic hemodynamics and renal blood flow in patients following open heart surgery. Jpn Circ J. (1982) 46:1059–72. doi: 10.1253/jcj.46.1059
30. Nachar RA, Booth EA, Friedlich P, Borzage M, Soleymani S, Wider MD, et al. Dose-dependent hemodynamic and metabolic effects of vasoactive medications in normotensive, anesthetized neonatal piglets. Pediatr Res. (2011) 70:473–9. doi: 10.1203/PDR.0b013e31822e178e
31. Dünser MW, Hasibeder WR. Sympathetic overstimulation during critical illness: adverse effects of adrenergic stress. J Intensive Care Med. (2009) 24:293–316. doi: 10.1177/0885066609340519
32. Tanoue A, Nasa Y, Koshimizu T, Shinoura H, Oshikawa S, Kawai T, et al. The α1D-adrenergic receptor directly regulates arterial blood pressure via vasoconstriction. J Clin Invest. (2002) 109:765–75. doi: 10.1172/JCI200214001
33. Nari K, Joonyong C, Euiyong K, Jin H. Changes in the Ca2+ activated K+ channels of the coronary artery during left ventricular hypertrophy. Circ Res. (2003) 93:541–7. doi: 10.1161/01.RES.0000090087.66390.F2
34. Dilli D, Soylu H, Tekin N. Turkish neonatal society guideline on the neonatal hemodynamics and management of hypotension in newborns. Turk Pediatr Ars. (2018) 53:65–75. doi: 10.5152/TurkPediatriArs.2018.01801
35. Sell H, Deshaies Y, Richard D. The brown adipocyte: update on its metabolic role. Int J Biochem Cell Biol. (2004) 36:2098–104. doi: 10.1016/j.biocel.2004.04.003
36. Hoffman TM, Wernovsky G, Atz AM, Kulik TJ, Nelson DP, Chang AC, et al. Efficacy and safety of milrinone in preventing low cardiac output syndrome in infants and children after corrective surgery for congenital heart disease. Circulation. (2003) 107:996–1002. doi: 10.1161/01.CIR.0000051365.81920.28
37. Scolletta S, Ranaldi G, Carlucci F, Franchi F, Romano SM, Biagioli B. Relationship between N-terminal pro-B-type natriuretic peptide (Nt-proBNP) and cardiac cycle efficiency in cardiac surgery. Biomed Pharmacother. (2010) 64:511–5. doi: 10.1016/j.biopha.2010.01.001
Keywords: pressure recording analytical method, systemic hemodynamics, cardiac cycle efficiency, epinephrine, pediatric cardiopulmonary bypass, deep hypothermic circulatory arrest
Citation: Lou X, Liu Y, Cui Y, Li J, Li L, Ma L, Zou M, Chen X and Li J (2021) Contemporary Trends and Risk Factors of Hemodynamic and Myocardial Mechanics Derived by the Pressure Recording Analytical Method After Pediatric Cardiopulmonary Bypass. Front. Cardiovasc. Med. 8:687150. doi: 10.3389/fcvm.2021.687150
Received: 29 March 2021; Accepted: 25 June 2021;
Published: 20 July 2021.
Edited by:
Umberto Morbiducci, Politecnico di Torino, ItalyReviewed by:
Giuseppe De Nisco, Polytechnic University of Turin, ItalyCopyright © 2021 Lou, Liu, Cui, Li, Li, Ma, Zou, Chen and Li. This is an open-access article distributed under the terms of the Creative Commons Attribution License (CC BY). The use, distribution or reproduction in other forums is permitted, provided the original author(s) and the copyright owner(s) are credited and that the original publication in this journal is cited, in accordance with accepted academic practice. No use, distribution or reproduction is permitted which does not comply with these terms.
*Correspondence: Xinxin Chen, emluZ2VyY2hlbkAxNjMuY29t; Jia Li, amlhbGlfYmVpamluZ0AxMjYuY29t
†These authors have contributed equally to this work
Disclaimer: All claims expressed in this article are solely those of the authors and do not necessarily represent those of their affiliated organizations, or those of the publisher, the editors and the reviewers. Any product that may be evaluated in this article or claim that may be made by its manufacturer is not guaranteed or endorsed by the publisher.
Research integrity at Frontiers
Learn more about the work of our research integrity team to safeguard the quality of each article we publish.