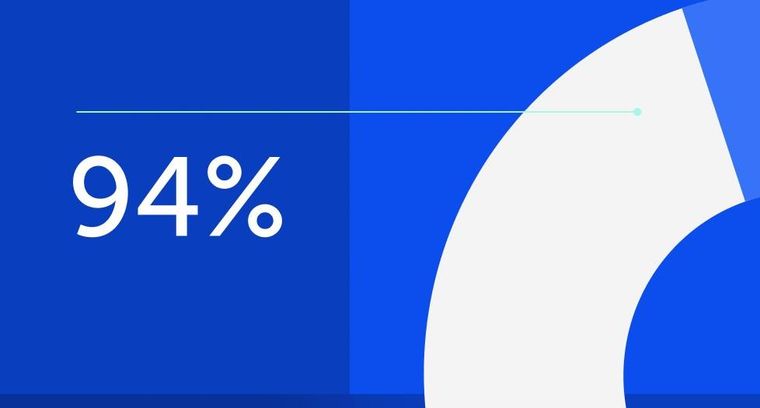
94% of researchers rate our articles as excellent or good
Learn more about the work of our research integrity team to safeguard the quality of each article we publish.
Find out more
ORIGINAL RESEARCH article
Front. Cardiovasc. Med., 20 August 2021
Sec. Cardiovascular Metabolism
Volume 8 - 2021 | https://doi.org/10.3389/fcvm.2021.685748
This article is part of the Research TopicInsulin Resistance, Metabolic Syndrome and Cardiovascular DiseaseView all 36 articles
Background: Vascular calcification (VC) is a landmark of aging, while β-hydroxybutyric acid (BHB) induced by calorie restriction has been identified as a promising factor to extend the lifespan. However, the effect of BHB on VC and the potential mechanism remain unknown.
Methods: A total of 160 subjects with or without metabolic abnormalities (MAs) were assigned to four groups according to different calcification severities. The association between BHB, MAs, and VC was investigated via mediation analysis. Then, with high phosphate-induced calcification models, the effect of BHB on arterial ring calcification and osteogenic phenotypic differentiation of vascular smooth muscle cells (VSMCs) was investigated. Hereafter the expressions of autophagy biomarkers, autophagy flux, and effects of autophagy inhibitors on VC were detected.
Results: Severe VC was observed in the elderly, accompanied with a higher proportion of hypertension, chronic kidney disease, and lower estimated glomerular filtration rate. The serum BHB level was an independent influencing factor of VC severities. With mediation analysis, BHB was determined as a significant mediator in the effects of MAs on VC, and the indirect effect of BHB accounted for 23% of the total effect. Furthermore, BHB directly inhibited arterial ring calcification and osteogenic phenotypic differentiation in VSMCs, accompanied with autophagy enhancement in VSMCs. In accordance, the inhibition of autophagy counteracted the protective effect of BHB on VC.
Conclusion: The present study demonstrated that BHB mediated the effects of MAs on VC; then, it further elucidated that BHB could inhibit arterial and VSMC calcification via autophagy enhancement.
Vascular calcification (VC), characterized by the accumulation of calcium phosphate crystals and the phenotypical transition of vascular smooth muscle cells (VSMCs) inside the vessels, is related to a variety of diseases, such as diabetes, chronic kidney disease (CKD), atherosclerosis, and other cardiovascular diseases (1–3). Although the incidence of VC remains high in clinics, there are still no approved or substantially effective therapies for the treatment of VC. Therefore, exploring the novel therapy for VC has been an important tough problem waiting to be solved.
It has become increasingly clear that metabolic syndrome (METs) is a major risk factor of VC (4, 5). Emerging evidence has demonstrated that calorie restriction (CR), a nutritional intervention of reduced energy intake but with adequate nutrition, effectively regulates the metabolic balance, ameliorates metabolic abnormalities (MAs), and reduces VC (6–9). Meanwhile, β-hydroxybutyric acid (BHB), which could be generated by CR, has been proven to promote autophagy and prevent VSMC senescence (10). However, whether BHB can prevent arterial calcification in populations with MAs or VC and the potential mechanism remain unclear.
Among the numerous etiologies, autophagy, an important biological process that contributes to cellular homeostasis, has been recognized as an important influencing factor of survival and function in VSMCs in the process of VC (11, 12). Autophagy exists in three separate forms: microautophagy, chaperone-mediated autophagy, and macroautophagy (13). The term autophagy usually refers to macroautophagy, which is the most prevalent and the only form of autophagy mentioned here. Alterations in autophagy have been documented in VSMCs in response to various stimuli, resulting in the modulation of VSMC functions, including VC (14, 15). On the contrary, the activation of autophagy stimulates VSMC survival, maintains normal vascular cell function, and protects against VC (16, 17). Notably, the accumulated evidence has confirmed that BHB plays a broad role in regulating longevity and affects the development of aging (18). Hence, it may discreetly propose that BHB intervention may protect VC and the underlying mechanism is related to autophagy.
Based on the uncertainties mentioned above, we hypothesized that insufficient autophagy is associated with VC, and autophagy enhancement induced by BHB maintains the VSMC function and protects against VC. To address these assumptions, the role of BHB in the correlation of MAs and VC was investigated, and then the effect of BHB administration on high phosphate-induced VC models and the potential mechanism related to autophagy were further explored. This study may provide herein novel insights into the mechanism of autophagy in VC and shed novel light on the promising therapeutic target of the early prevention of VC.
This study was designed to investigate the relations between BHB and calcification under different metabolic status. The study recruited 160 patients from the Affiliated Hospitals of Sun Yat-sen University from January 2015 to July 2020. The participants, ages between 42 and 91, all underwent chest computerized tomographic (CT) scan and serum β-hydroxybutyric acid measurement and had no missing data on any components of the METs. Patients with severe diseases that might affect nutrient absorption and energy metabolism were excluded, including inflammatory bowel disease, cachexia, cirrhotic hepatic disease, malignant tumor, etc. Demographic data and medical history were acquired using standard questionnaires. The trial was conducted according to the International Conference on Harmonization—Good Clinical Practice guidelines and the Declaration of Helsinki. All patients were enrolled after informed consents were obtained.
All the patients underwent a non-enhanced chest CT scan to evaluate thoracic aorta calcification (TAC). As described by Agatston et al. (19), the calcium score of each lesion was calculated by multiplying the area of the calcified speck by the density factor derived from the maximal Hounsfield units within this area (20). The grouping criteria of TAC severity are as follows: TAC score = 0: no TAC; TAC score = 1–100: mild TAC; TAC score = 101–500: moderate TAC; and TAC score >500: severe TAC.
The chest CT scans were performed using the same equipment (SOMATOM Sensation 64 CT). The details of the scanning acquisition were described previously (21). Two experienced investigators, blinded to all clinical information, randomly analyzed all the CT images.
The definition of METs was diverse from each other, and developing a standard one has been challenging. One of the best accepted definitions was provided by the US National Heart, Lung, and Blood Institute and the American Heart Association Consensus Statement (22). In this study, we referred to the METs definition and determined MAs with one and more of the following parameters: large waist circumference (women >80 cm and men >90 cm, according to the standard of abdominal obesity in the Chinese population), elevated triglycerides [≥1.7 mmol/L (150 mg/dl)], low high-density lipoprotein cholesterol (HDL-C) [men <1.03 mmol/L (40 mg/dl) and women <1.29 mmol/L (50 mg/dl)], impaired fasting glucose [≥6.1 mmol/L (110 mg/dl) or on antidiabetic medication), and elevated blood pressure (≥130/85 mmHg or self-reported use of medications for hypertension).
Blood samples were collected from the patients in the morning after more than 10 h of fasting. The following parameters were tested or determined in the Institutional Central Laboratory of the Affiliated Hospital of Sun Yat-sen University: fasting glucose, total cholesterol, triglyceride (TG), high-density lipoprotein cholesterol (HDL-C), low-density lipoprotein cholesterol (LDLC), serum calcium (Ca), serum phosphate (Pi), and estimated glomerular filtration rate (eGFR). All the biochemical parameters were analyzed by using a standardized and certified TBA-120 autoanalyzer (Toshiba Medical Systems, Japan).
The arterial rings and VSMCs were isolated or extracted as previously described (23). Calcification was induced by adding inorganic phosphate (Na2HPO4, Sigma, USA) to normal medium at a concentration of 2.6 mM (calcification medium). To evaluate the impact of BHB on calcification, BHB (Sigma, USA) was administrated into a calcification medium at a concentration of 4.0 mM (BHB intervention medium) (24). The arterial rings were cultured for 14 days, while VSMCs were cultured for 7 days, with the medium for both refreshed every 2 to 3 days (23).
To evaluate whether autophagy inhibition could reverse the protective effects of BHB on VC, the autophagosome inhibitor 3-methyladenine (Selleck, USA), at a concentration of 20 μM, and the autophagic flux inhibitor chloroquine (Selleck, USA), at a concentration of 10 μM, were administrated to the calcification models with BHB intervention (25).
Von Kossa staining was used to detect the calcified depositions of arterial rings. The staining experiments were performed as previously described (25). The calcium deposits displayed black or dark brown colors under the microscope.
For Alizarin Red S staining, VSMCs in six-well plates were washed three times with phosphate-buffered saline (PBS) after the indicated treatments and then fixed with 4% paraformaldehyde for 30 min. After that, the fixed VSMCs were washed and then stained with 2% Alizarin Red S solution (Servicebio, China) for 10 min. The stained calcium deposits were observed and photographed under a microscope. The area positive for Alizarin Red S staining displayed a red color.
VSMCs were washed three times with PBS and then decalcified with 0.6 M hydrochloric acid for 12 h at 4°C. The supernatant was collected, of which the calcium concentration was determined as previously described (25).
Total RNA was extracted, reverse-transcribed, and amplified to detect some specific mRNA transcription as previously described (26). The primers were designed on Primerbank and verified with primer-BLAST on PubMed. The PCR primers were as follows:
GAPDH, forward 5′-AGGTCGGTGTGAACGGATTTG-3′ and reverse 5′-GGGGTCGTTGATGGCAACA-3′; Runx2, forward 5′-GACTGTGGTTACCGTCATGGC-3′ and reverse 5′-ACTTGGTTTTTCATAACAGCGGA-3′; BMP-2, forward 5′-GGGACCCGCTGTCTTCTAGT-3′ and reverse 5′-TCAACTCAAATTCGCTGAGGAC-3′; SM22a, forward 5′-CCAACAAGGGTCCATCCTACG-3′ and reverse 5′-ATCTGGGCGGCCTACATCA-3′; α-SMA, forward 5′-CCCAGACATCAGGGAGTAATGG-3′ and reverse 5′-TCTATCGGATACTTCAGCGTCA-3′.
The qPCR experiments were independently repeated three times, and the quantification was performed using the ΔΔCt method. The results were normalized to GAPDH transcription.
VSMCs were washed three times with PBS and collected after treatment with RIPA lysis buffer as described previously (26). The proteins were boiled, and the same amount of proteins was subjected to SDS-PAGE and transferred onto polyvinylidene difluoride membranes (Merck-Millipore, the USA). The membranes were incubated successively with 5% bovine serum albumin for 1 h and then stained with primary antibodies for 12 h at 4°C, including anti-smoothelin (1:1,000 dilution, Abcam, USA), anti-Runx2 (1:500 dilution, CST, USA), anti-BMP2 (1:1,000 dilution, Novus, USA), anti-GAPDH (1:1,000 dilution, CST, USA), and anti-SM22α (1:1,000 dilution, Abcam, USA). The membranes were then incubated with secondary goat anti-rabbit IgG antibody (1:5,000 dilution, Santa Cruz, USA) for 1 h. Finally, the reaction was visualized by a chemiluminescence image system (Proteinsimple, USA), and the density of the bands was semi-quantified via the image software Image J (National Institutes of Health, V 1.53, USA).
mRFP-GFP-LC3 double-labeled adenovirus was purchased from HanBio Technology Co., Ltd., and adenoviral infection was performed according to the instructions of the manufacturer (25). Isolated mouse VSMCs were plated in six orifice plates, and the cells were transduced with adenovirus after reaching 50% confluence by Dulbecco's modified Eagle's medium supplemented with 5% fetal bovine serum (FBS) for 24 h at 37°C. After infection, the VSMCs were then grown in a medium with 2.5% FBS for 24 h before changing to a complete culture medium administrated with 2.6 mM Pi and 4.0 mM BHB. Autophagy was observed through a fluorescence microscope (Nikon Eclipse Tis). Autophagic flux was determined by evaluating the number of GFP and RFP puncta (puncta/cell was counted).
Data of the continuous variables are presented as mean and standard deviation (SD), skewed data as median (25th and 75th percentiles), and categorical variables as absolute numbers and percentages. Comparisons among groups were attained by one-way ANOVA (for distributed variables) or chi-square test (for categorical variables). For skewed data, the cases were ranked as new variables, and then an analysis of variance was performed.
Regression analysis was performed to examine whether BHB concentration was an independent influencing variable for TAC severities. A univariate linear regression model (model 1) was initially analyzed. Then, model 1 was adjusted with significant and potential confounding variables, which formed multivariate linear regression models (models 2 and 3), to further investigate the association between BHB and TAC severities. Specifically, model 2 was adjusted with age, sex, BMI, waist circumference, hypertension (HTN), and type 2 diabetes mellitus (T2DM), while model 3 was adjusted with age, sex, BMI, waist circumference, HTN, T2DM, TG, HDL, eGFR, Ca, and Pi.
Mediation analysis was conducted on SPSS 22.0 with model 4 in PROCESS plugin, which was introduced by (27). Before the analysis, we hypothesized that MAs (the independent variable) influenced TAC severities (dependent variable) directly as well as indirectly via BHB (mediator variable). Mediation analysis was used to validate the hypothesis, which was composed of three steps. Firstly, the direct effect of MAs on TAC severities (c′) was evaluated. Secondly, the effect of MAs on serum BHB level (a) was analyzed. Thirdly, the indirect effect of MAs on TAC severities via serum BHB level (a * b) was evaluated, while b referred to the effect of BHB on TAC severities controlling for MAs. The sum of the direct effect and the indirect effect was considered as the total effect of MAs on TAC severities (c), meaning that c = c′ + a * b. In this research, age was included as a control variable. The effects were significant if their 95% confidence interval (CI) did not cross zero (28). BHB would be determined as a mediating factor if the results showed that both the total effect and the indirect effect were evident.
We used IBM SPSS Statistics 22.0 for all data analyses, and a two-tail P < 0.05 has been considered significant after Bonferroni correction.
A total of 160 participants (69.4% are female; ages between 42 and 91) were finally enrolled within the specified time, and the baseline characteristics of the subjects by different degrees of TAC are described in Table 1. The severity of TAC increased with aging; in addition, a higher occurrence of hypertension and CKD and a lower eGFR were observed in the severe TAC group. Meanwhile, no significant differences were found in gender, glucolipid, and Ca/Pi metabolism between groups.
In this study, the patients with MAs had higher TAC scores than those without MAs [205.3 (4.7, 1,031.5) vs. 26.1 (0.0, 310.3), P < 0.01]. Furthermore, we investigated the relationship between serum BHB levels and TAC severities with linear regression analysis (Table 2). The results showed that, in the univariate linear regression (model 1), BHB was a protective factor for TAC. After adjusting for some potential influencing factors and metabolic parameters, including age, sex, WC, HTN, T2DM, TG, HDL-C, etc., the results still showed that BHB was an independent protective factor for TAC (P < 0.05).
It has been well-accepted that MAs contribute to the occurrence of VC, while BHB affected the metabolic status (4, 29). Here we performed a mediation analysis to further analyze whether BHB mediated the effects of MAs on TAC severities. The results showed that, in this study (Figure 1), the presence of MAs had a negative impact on serum BHB level (β = −0.0169, P < 0.05), and the BHB level also had a negative impact on TAC (β = −4.984, P < 0.01). Moreover, the presence of MAs had a significant positive effect on TAC severities (β = 0.365, P < 0.001), and after the mediator variable BHB was placed, the effect of MAs on TAC was still significant (β = 0.281, P < 0.05). In other words, MAs negatively affect BHB, while BHB partly mediated the effects of MAs on TAC. Specifically, BHB accounted for 23% of the association between MAs and TAC (direct effect: β = 0.281, 95%CI: 0.023–0.548; indirect effect: β = 0.084, 95%CI: 0.015–0.170).
Figure 1. BHB partly mediated the relations between MAs and TAC severities. BHB, β-hydroxybutyric acid; MAs, metabolic abnormalities; TAC, thoracic aorta calcification.
To further evaluate the effects of BHB on VC, we established calcification models with arterial rings and VSMCs and found that BHB co-incubation delayed the progression of the arterial calcification (Figure 2), and BHB intervention (2.6 mM Pi + 4.0 mM BHB) ameliorated VSMC calcification and decreased the calcium content in VSMCs (Figures 3A,B). Furthermore, with the analysis of RT-PCR and WB, the expressions of osteogenic markers, Runx2 and BMP2, were decreased in VSMCs treated with BHB intervention medium compared to cells treated with phosphate (2.6 mM Pi) alone, whereas BHB maintains the expressions of contractile proteins, smoothelin, and SM22α (Figures 3C,D). These results demonstrated that BHB may serve as an effective intervention for VC via regulating VSMC phenotypical transition.
Figure 2. β-Hydroxybutyric acid ameliorated the Pi-induced arterial calcification. (A) Von Kossa staining of arterial rings. Pieces of mice aorta were cultured in calcification medium (Pi: 2.6 mM) for 14 days. Scale bar: 50 μm. (B) Proportion of calcium areas in the total cross-sectional aorta area. The analysis was performed by Image J. Pi, phosphate; **P < 0.01; ***P < 0.001.
Figure 3. β-Hydroxybutyric acid (BHB) inhibited the osteogenic phenotypical transition of vascular smooth muscle cells (VSMCs). (A) Alizarin Red S staining of VSMCs. Primary VSMCs were treated with Pi (2.6 mM), BHB (4.0 mM), or Pi (2.6 mM) + BHB (4.0 mM) for 7 days. Scale bar: 500 μm. (B) Calcium density analysis examined the calcium concentration in supernatant collected from VSMCs. (C) qPCR detected the differences in the transcription levels of Runx2, BMP2, SM22α, and α-SMA. (D) Representative Western blot bands and semi-quantitative analysis of the protein expression of smoothelin, Runx2, BMP2, and SM22a. *P < 0.05; **P < 0.01; ***P < 0.001.
To further investigate whether autophagy is the potential mechanism in BHB-mediated VC inhibition, this study performed several ex vivo and in vitro experiments. The results showed that BHB introduction postponed the progression of arterial calcification, enhanced the expression of autophagy-related protein LC3B, and upregulated the ratio of LC3II/LC3I (Figures 4A,B). To investigate the effects of BHB on autophagy flux, VSMCs were infected with mRFP-GFP-LC3 adenovirus (multiplicity of infection = 100). As a result, BHB administration induced a considerable increase in RFP-positive autolysosomes (red dots) in cells compared with those treated with high Pi alone (Figures 4C,D), while no differences were shown in GFP/RFP double-positive autophagosomes (yellow dots) between groups. Taken together, these results indicated that phosphate inhibits autophagy mainly by suppressing autophagic flux at late stage, while BHB offset this effect and promoted the fusion of autophagosomes with lysosomes to form autolysosomes. The inhibition of autophagy in VSMCs counteracted the protective effect of BHB on arterial calcification and increased the calcium content in VSMCs (Figure 5). In conclusion, the findings demonstrated that the BHB-induced autophagy enhancement may be a potential mechanism that inhibited the development of VC.
Figure 4. β-Hydroxybutyric acid-enhanced autophagy. (A) Immunohistochemical analysis detected the expression of LC3B and Runx2. Scale bar: 100 μm. (B) Representative Western blot bands and semi-quantitative analysis of LC3II/LC3-I. (C,D) Representative confocal images (scale bar, 20 μm) and quantitative analysis of mRFP-GFP-LC3 puncta in vascular smooth muscle cells. The bar graph shows the mean number of autophagosomes (yellow dots) and autolysosomes (red dots) per cell. N = 5 per group. *P < 0.05; **P < 0.01.
Figure 5. Autophagy inhibitors counteracted the protective effect of β-hydroxybutyric acid (BHB) on vascular calcification. (A) Von Kossa staining of arterial rings. (B,C) Alizarin Red S staining and calcium deposition analysis of vascular smooth muscle cells (VSMCs). Scale bar: 50 μm. *P < 0.05, **P < 0.01 vs. VSMCs treated with Pi alone; #P < 0.05 vs. VSMCs treated with Pi+BHB.
The aim of this study was to determine whether BHB ameliorated arterial calcification and to investigate the potential mechanism related to autophagy. The major findings of our present study are as follows: (1) BHB was negatively correlated with VC and mediated the effects of MAs on VC, (2) BHB postponed arterial calcification and inhibited the osteogenic phenotype transdifferentiation of VSMCs induced by high phosphate, and (3) autophagy enhancement promoted by BHB in VSMCs was found to contribute to the inhibition of arterial calcification. For the first time, this study demonstrated the role and effect of BHB in VC and MAs. Furthermore, the results may provide a potential interventional target for the prevention of VC.
MAs were common in the aging population. Data from the National Health and Nutrition Examination Survey showed that the prevalence of MAs was about 46.7% among subjects of age >60 years (30). It has been well-accepted that aging is a powerful risk factor for VC and METs (31). In accordance, interventions toward aging or MAs may possess potency in preventing VC (32, 33). We confirmed, with a univariate linear regression analysis, that serum BHB levels were negatively related with TAC severities. Moreover, after including various potential variables into the regression model, we showed that BHB was still an independent protective factor for severe TAC. To figure out whether BHB transmitted the effects of MAs to VC, we performed a mediation analysis, and the result confirmed that BHB was the mediator (intervening variable), indicating that BHB is a potential target to regulate metabolic conditions and therefore affects the pathogenesis of VC. However, the underlying effects and mechanisms of BHB on VC remain unclear.
Accumulating data from observational and randomized clinical trials indicated that CR might improve cardiovascular health and metabolic status (34, 35). CR upregulates the production of ketone bodies, in which BHB accounted for more than 70%, a multifunctional metabolic substance which could be physiologically elevated under glucose deprivation. Recent studies suggested that BHB played an important role in delaying aging and improving metabolic conditions (18, 24, 29, 36).
Exercise and calorie restriction diets are believed to improve metabolic status. The metabolic status amelioration may be linked to the upregulation of BHB. Interestingly, sodium–glucose cotransporter 2 inhibitors, such as dapagliflozin, an effective drug for T2DM, could induce mild ketonemia and downregulate serum glucose, reduce body weight, and blood pressure (37). BHB is the most promising effector during the process, for researchers have confirmed that the administration of BHB precursor could attenuate hypertension and protect against heart diseases (29).
Moreover, BHB takes a part in maintaining the functions of the cardiovascular system—for example, BHB could delay vascular aging, improve cardiac ischemia/reperfusion injury, and inhibit inflammation (38, 39). However, it remains unclear whether BHB affects VC, and the underlying mechanism needs to be elucidated. In this study, we established vascular calcification models and evaluate the effects of BHB on VC. The Von Kossa staining of the aorta rings showed that BHB significantly alleviated arterial calcification, while the Alizarin Red S staining demonstrated that BHB reduced the calcium content of VSMCs. Moreover, we further demonstrated that BHB inhibited the osteogenic phenotype transdifferentiation of VSMCs, which was characterized by the upregulation of osteogenic phenotype markers (RUNX2 and BMP2) and downregulation of contractile phenotype markers (smoothelin and SM22a).
However, the underlying mechanisms in the regulation of BHB on VSMC calcification need further studies. It has been proven that autophagy, an evolutionarily conserved mechanism linking to several cellular pathways, impacts VSMC survival and functions. Dai et al. demonstrated that the inhibition of autophagy enhanced the phosphate-induced matrix vesicle release, thereby exacerbating VSMC calcification (40). Otherwise, BHB was found to enhance autophagy regulated by the histone acetylation of specific genes and improve proteostasis through increased chaperone expression (41). Thus, it is meaningful to further explore whether BHB can promote autophagy in VSMCs to protect against osteogenic phenotype transdifferentiation, resulting in inhibiting VC. In the present study, BHB enhanced the expression of autophagy-related protein LC3B and promoted the formation of autolysosomes. The results may raise the perspective attention for the intervention of BHB on inhibiting VC.
Our study has some limitations which must be appreciated. Firstly, the clinical study was retrospective; confounding factors or selection bias may have affected our findings. A prospective study for the effect of BHB on VC should be considered in the future. Secondly, the current project should be further validated in BHB-knock-out animal models; however, the model is not available because BHB is important for animal survival.
In conclusion, we identified for the first time that BHB mediated the correlation between MAs and VC; then, we further demonstrated BHB could promote autophagy to inhibit VC. Our present study provides a novel insight into the potential of BHB as an effective strategy for the treatment of arterial calcification.
This is the first study to demonstrate that BHB mediated the correlation between MAs and arterial calcification. In addition, the enhancement of autophagy in VSMCs induced by BHB treatment may be an effective intervention target to ameliorate arterial calcification and delay aging.
The original contributions presented in the study are included in the article/supplementary material, further inquiries can be directed to the corresponding author/s.
The studies involving human participants were reviewed and approved by Ethics Committee of the Eighth Affiliated Hospital, Sun Yat-sen University. The patients/participants provided their written informed consent to participate in this study. The animal study was reviewed and approved by Ethics Committee of the Eighth Affiliated Hospital, Sun Yat-sen University.
JL: funding acquisition, writing—original draft, and writing—review and editing. JH: conceptualization, data curation, formal analysis, and investigation. WH: data curation, formal analysis, and investigation. GS: computerized tomographic analysis. JC: supervision. HH: funding acquisition and supervision. All authors contributed to the article and approved the submitted version.
This work is supported by the by National Natural Science Foundation of China [8201101103, 82073408, 81870506, 81670676, and 81422011], project of traditional Chinese medicine in Guangdong province [20201062], Basic Research Project of Shenzhen Science and Technology Innovation Committee [JCYJ20180306180229307, JCYJ20180306174648342, and JCYJ20190808102005602], Guangdong Medical Scientific Research Foundation [A2021045], Shenzhen futian district public health research project [FTWS2019003, FTWS2020009], Sun Yatsen University Clinical Medicine Research 5010 Cultivation Project' (No: 2018027), Shenzhen Key Medical Discipline Construction Fund (No: SZXK002).
The authors declare that the research was conducted in the absence of any commercial or financial relationships that could be construed as a potential conflict of interest.
All claims expressed in this article are solely those of the authors and do not necessarily represent those of their affiliated organizations, or those of the publisher, the editors and the reviewers. Any product that may be evaluated in this article, or claim that may be made by its manufacturer, is not guaranteed or endorsed by the publisher.
2. Durham AL, Speer MY, Scatena M, Giachelli CM, Shanahan CM. Role of smooth muscle cells in vascular calcification: implications in atherosclerosis and arterial stiffness. Cardiovasc Res. (2018) 114:590–600. doi: 10.1093/cvr/cvy010
3. McClelland RL, Chung H, Detrano R, Post W, Kronmal RA. Distribution of coronary artery calcium by race, gender, and age: results from the Multi-Ethnic Study of Atherosclerosis (MESA). Circulation. (2006) 113:30–7. doi: 10.1161/CIRCULATIONAHA.105.580696
4. Hayden MR, Tyagi SC, Kolb L, Sowers JR, Khanna R. Vascular ossification-calcification in metabolic syndrome, type 2 diabetes mellitus, chronic kidney disease, and calciphylaxis-calcific uremic arteriolopathy: the emerging role of sodium thiosulfate. Cardiovasc Diabetol. (2005) 4:4. doi: 10.1186/1475-2840-4-4
5. Cheung CL, Tan KC, Lam KS, Cheung BM. The relationship between glucose metabolism, metabolic syndrome, and bone-specific alkaline phosphatase: a structural equation modeling approach. J Clin Endocrinol Metab. (2013) 98:3856–63. doi: 10.1210/jc.2013-2024
6. Most J, Tosti V, Redman LM, Fontana L. Calorie restriction in humans: an update. Ageing Res Rev. (2017) 39:36–45. doi: 10.1016/j.arr.2016.08.005
7. Yang J, Zeng P, Liu LP, Yu M, Su JM, Yan YL, et al. Food with calorie restriction reduces the development of atherosclerosis in apoE-deficient mice. Biochem Biophys Res Commun. (2020) 524:439–45. doi: 10.1016/j.bbrc.2020.01.109
8. Flanagan EW, Most J, Mey JT, Redman LM. Calorie restriction and aging in humans. Annu Rev Nutr. (2020) 40:105–33. doi: 10.1146/annurev-nutr-122319-034601
9. Redman LM, Smith SR, Burton JH, Martin CK, Il'yasova D, Ravussin E. Metabolic slowing and reduced oxidative damage with sustained caloric restriction support the rate of living and oxidative damage theories of aging. Cell Metab. (2018) 27:805–15. doi: 10.1016/j.cmet.2018.02.019
10. Newman JC, Verdin E. β-hydroxybutyrate: a signaling metabolite. Annu Rev Nutr. (2017) 37:51–76. doi: 10.1146/annurev-nutr-071816-064916
11. Kurabayashi M. Molecular mechanism of vascular calcification. Clin Calcium. (2019) 29:157–63. doi: 10.20837/4201902157
12. Demer LL, Tintut Y. Inflammatory, metabolic, and genetic mechanisms of vascular calcification. Arterioscler Thromb Vasc Biol. (2014) 34:715–23. doi: 10.1161/ATVBAHA.113.302070
13. Nussenzweig SC, Verma S, Finkel T. The role of autophagy in vascular biology. Cir Res. (2015) 116:480–8. doi: 10.1161/CIRCRESAHA.116.303805
14. Salabei JK, Hill BG. Implications of autophagy for vascular smooth muscle cell function and plasticity. Free Radic Biol Med. (2013) 65:693–703. doi: 10.1016/j.freeradbiomed.2013.08.003
15. Salabei JK, Hill BG. Autophagic regulation of smooth muscle cell biology. Redox Biol. (2015) 4:97–103. doi: 10.1016/j.redox.2014.12.007
16. Jia G, Cheng G, Gangahar DM, Agrawal DK. Insulin-like growth factor-1 and TNF-alpha regulate autophagy through c-jun N-terminal kinase and Akt pathways in human atherosclerotic vascular smooth cells. Immunol Cell Biol. (2006) 84:448–54. doi: 10.1111/j.1440-1711.2006.01454.x
17. Frauscher B, Kirsch AH, Schabhüttl C, Schweighofer K, Kétszeri M, Pollheimer M, et al. Autophagy protects from uremic vascular media calcification. Front Immunol. (2018) 9:1866. doi: 10.3389/fimmu.2018.01866
18. Newman JC, Verdin E. Ketone bodies as signaling metabolites. Trends Endocrinol Metab. (2014) 25:42–52. doi: 10.1016/j.tem.2013.09.002
19. Agatston AS, Janowitz WR, Hildner FJ, Zusmer NR, Viamonte MJ, Detrano R. Quantification of coronary artery calcium using ultrafast computed tomography. J Am Coll Cardiol. (1990) 15:827–32. doi: 10.1016/0735-1097(90)90282-T
20. Gao JW, Hao QY, Zhang HF, Li XZ, Yuan ZM, Guo Y, et al. Low-carbohydrate diet score and coronary artery calcium progression: results from the CARDIA study. Arterioscler Thromb Vasc Biol. (2021) 41:491–500. doi: 10.1161/ATVBAHA.120.314838
21. Nakanishi R, Delaney JA, Post WS, Dailing C, Blaha MJ, Palella F, et al. A novel density-volume calcium score by non-contrast CT predicts coronary plaque burden on coronary CT angiography: results from the macs (multicenter aids cohort study). J Cardiovasc Comput Tomogr. (2020) 14:266–71. doi: 10.1016/j.jcct.2019.09.016
22. Dabke K, Hendrick G, Devkota S. The gut microbiome and metabolic syndrome. J Clin Invest. (2019) 129: 4050–7. doi: 10.1172/JCI129194
23. Wei N, Lu L, Zhang H, Gao M, Ghosh S, Liu Z, et al. Warfarin accelerates aortic calcification by upregulating senescence-associated secretory phenotype maker expression. Oxid Med Cell Longev. (2020) 2020:2043762. doi: 10.1155/2020/2043762
24. Han YM, Bedarida T, Ding Y, Somba BK, Lu QL, Wang QL, et al. β-hydroxybutyrate prevents vascular senescence through hnRNP A1-mediated upregulation of Oct4. Mol Cell. (2018) 71:1064–78.e5doi: 10.1016/j.molcel.2018.07.036
25. Gao JW, He WB, Xie CM, Gao M, Feng LY, Liu ZY, et al. Aldosterone enhances high phosphate-induced vascular calcification through inhibition of AMPK-mediated autophagy. J Cell Mol Med. (2020) 24:13648–59. doi: 10.1111/jcmm.15813
26. Zhang K, Zhang Y, Feng W, Chen RH, Chen J, Touyz RM, et al. Interleukin-18 enhances vascular calcification and osteogenic differentiation of vascular smooth muscle cells through TRPM7 activation. Arterioscler Thromb Vasc Biol. (2017) 37:1933–43. doi: 10.1161/ATVBAHA.117.309161
27. Hayes AF. PROCESS: A Versatile Computational Tool for Observed Variable Mediation, Moderation, and Conditional Process Modeling [White paper]. (2012). Available online at: http://www.afhayes.com/public/process2012.pdf
28. Ghosh S, He W, Gao J, Luo D, Wang J, Chen J, et al. Whole milk consumption is associated with lower risk of coronary artery calcification progression: evidences from the Multi-Ethnic Study of Atherosclerosis. Eur J Nutr. (2021) 60:1049–58. doi: 10.1007/s00394-020-02301-5
29. Chakraborty S, Galla S, Cheng X, Yeo JY, Mell B, Singh V, et al. Salt-responsive metabolite, β-hydroxybutyrate, attenuates hypertension. Cell Rep. (2018) 3:677–89.e4. doi: 10.1016/j.celrep.2018.09.058
30. Dominguez LJ, Barbagallo M. The biology of the metabolic syndrome and aging. Curr Opin Clin Nutr Metab Care. (2016) 19:5–11. doi: 10.1097/MCO.0000000000000243
31. Lindman BR, Clavel MA, Mathieu P, Lung B, Lancellotti P, Otto CM, et al. Calcific aortic stenosis. Nat Rev Dis Primers. (2016) 2:16006. doi: 10.1038/nrdp.2016.6
32. Jura M, Kozak LP. Obesity and related consequences to ageing. Age. (2016) 38:23. doi: 10.1007/s11357-016-9884-3
33. Kane AE, Sinclair DA. Sirtuins and NAD (+) in the development and treatment of metabolic and cardiovascular diseases. Circ Res. (2018) 123:868–85. doi: 10.1161/CIRCRESAHA.118.312498
34. López-Lluch G, Navas P. Calorie restriction as an intervention in ageing. J Physiol. (2016) 594:2043–60. doi: 10.1113/JP270543
35. Veech RL, Bradshaw PC, Clarke K, Curtis W, Pawlosky R, King MT. Ketone bodies mimic the life span extending properties of caloric restriction. IUBMB Life. (2017) 69:305–14. doi: 10.1002/iub.1627
36. Goldberg EL, Asher JL, Molony RD, Shaw AC, Zeiss CJ, Wang C, et al. β-hydroxybutyrate deactivates neutrophil NLRP3 inflammasome to relieve gout flares. Cell Rep. (2017) 18:2077–87. doi: 10.1016/j.celrep.2017.02.004
37. Min SH, Oh TJ, Baek SI, Lee DH, Kim KM, Moon JH, et al. Degree of ketonaemia and its association with insulin resistance after dapagliflozin treatment in type 2 diabetes. Diabetes Metab. (2018) 44:73–6. doi: 10.1016/j.diabet.2017.09.006
38. Yu YS, Yu YH, Zhang YF, Zhang ZG, An WS, et al. Treatment with D-β-hydroxybutyrate protects heart from ischemia/reperfusion injury in mice. Eur J Pharmacol. (2018) 829:121–8. doi: 10.1016/j.ejphar.2018.04.019
39. Youm YH, Nguyen KY, Grant RW, Goldberg EL, Bodogai M, Kim D, et al. The ketone metabolite β-hydroxybutyrate blocks NLRP3 inflammasome-mediated inflammatory disease. Nat Med. (2015) 21:263–9. doi: 10.1038/nm.3804
40. Dai XY, Zhao MM, Cai Y, Guan QC, Zhao Y, Guan Y, et al. Phosphate-induced autophagy counteracts vascular calcification by reducing matrix vesicle release. Kidney Int. (2013) 83:1042–51. doi: 10.1038/ki.2012.482
Keywords: vascular calcification, β-hydroxybutyric acid, metabolic abnormalities, osteogenic phenotypic differentiation, vascular smooth muscle cells
Citation: Liang J, Huang J, He W, Shi G, Chen J and Huang H (2021) β-Hydroxybutyric Inhibits Vascular Calcification via Autophagy Enhancement in Models Induced by High Phosphate. Front. Cardiovasc. Med. 8:685748. doi: 10.3389/fcvm.2021.685748
Received: 25 March 2021; Accepted: 26 July 2021;
Published: 20 August 2021.
Edited by:
Yuli Huang, Southern Medical University, ChinaCopyright © 2021 Liang, Huang, He, Shi, Chen and Huang. This is an open-access article distributed under the terms of the Creative Commons Attribution License (CC BY). The use, distribution or reproduction in other forums is permitted, provided the original author(s) and the copyright owner(s) are credited and that the original publication in this journal is cited, in accordance with accepted academic practice. No use, distribution or reproduction is permitted which does not comply with these terms.
*Correspondence: Hui Huang, aHVhbmdoOEBtYWlsLnN5c3UuZWR1LmNu
†These authors have contributed equally to this work
Disclaimer: All claims expressed in this article are solely those of the authors and do not necessarily represent those of their affiliated organizations, or those of the publisher, the editors and the reviewers. Any product that may be evaluated in this article or claim that may be made by its manufacturer is not guaranteed or endorsed by the publisher.
Research integrity at Frontiers
Learn more about the work of our research integrity team to safeguard the quality of each article we publish.