- 1Division of Cardiovascular Surgery, Department of Surgery, Wan Fang Hospital, Taipei Medical University, Taipei, Taiwan
- 2Institute of Clinical Medicine, National Yang Ming Chiao Tung University, Taipei, Taiwan
- 3Taipei Heart Institute, Taipei Medical University, Taipei, Taiwan
- 4Division of Cardiology, Department of Internal Medicine, Taipei Medical University Hospital, Taipei, Taiwan
- 5Department of Internal Medicine, School of Medicine, College of Medicine, Taipei Medical University, Taipei, Taiwan
- 6Division of Cardiology, Department of Medicine, Taipei Veterans General Hospital, Taipei, Taiwan
- 7Department of Medicine, School of Medicine, National Yang Ming Chiao Tung University, Taipei, Taiwan
- 8The Ph.D. Program for Translational Medicine, College of Medical Science and Technology, Taipei Medical University, Taipei, Taiwan
- 9Division of Nephrology, Department of Internal Medicine, Taipei Medical University Hospital, Taipei, Taiwan
- 10Department of Surgery, School of Medicine, College of Medicine, Taipei Medical University, Taipei, Taiwan
Abdominal aortic aneurysm (AAA) ruptures are unpredictable and lethal. A biomarker predicting AAA rupture risk could help identify patients with small, screen-detected AAAs. Galectin-3 (Gal-3), a β-galactosidase–binding lectin, is involved in inflammatory processes and may be associated with AAA incidence. We investigated whether Gal-3 can be used as a biomarker of AAA size. Plasma Gal-3 protein concentrations were examined in patients with AAA (n = 151) and control patients (n = 195) using Human ProcartaPlex multiplex and simplex kits. Circulating Gal-3 levels were significantly higher in patients with AAA than in control patients. The area under the receiver operating characteristic curve for Gal-3 was 0.91. Multivariate logistic regression analysis revealed a significant association between Gal-3 level and the presence of AAA. Circulating Gal-3 levels were significantly correlated with aortic diameter in a concentration-dependent manner. In conclusion, higher plasma Gal-3 concentrations may be a useful biomarker of AAA progression.
Introduction
Abdominal aortic aneurysm (AAA) can cause segmental expansion and rupturing of the aorta, which are common among older adults and potentially life threatening (1). Common risk factors for AAA are male sex, older age, smoking, and hypertension (2). AAAs are characterized by the destruction of elastin and collagen in the media and adventitia, loss of smooth muscle cells (SMCs), thinning of the medial wall, infiltration of lymphocytes and macrophages, and neovascularization (3). Unclear pathological mechanisms have hindered the development of effective therapeutic strategies related to AAA. At present, surgical intervention is the only treatment option. A biomarker of AAA growth and subsequent rupture risk would help in patient selection for aneurysm repair. However, no reliable markers have been identified.
AAA pathogenesis plays a major role in tissue-destructive inflammation, which involves the accumulation of inflammatory cells in the adventitia through the recruitment of circulating monocytes or the proliferation of resident macrophages (4). Inflammatory biomarkers, such as C-reactive protein, tumor necrosis factor-α, interleukin (IL)-6, IL-β, and interferon-γ, are mediators that are reportedly involved in AAA development (5, 6). A meta-analysis of case–control studies suggested that AAAs are associated with high circulating IL-6 levels (7). Furthermore, numerous members of the cysteinyl cathepsin and matrix metalloproteinase (MMP) subfamilies are potent elastases and collagenases that mediate the degradation of these extracellular matrix proteins, causing AAA expansion and rupture (8, 9). Changes in systemic cytokine levels may activate cells in the periphery and initiate complex machinery, causing the recruitment of immune cells to the aneurysmal lesion.
Galectin-3 (Gal-3), a β-galactoside–binding lectin, is characterized by a conserved sequence within the carbohydrate recognition domain and amino-terminal tandem repeats (10). It regulates chemotaxis and inflammation (11, 12). Gal-3 has been reported as a prognostic marker for cardiovascular disease as it is linked to myocardial fibrosis, tissue remodeling, and heart failure development (13, 14) as well as heart failure severity (15, 16). Plasma Gal-3 levels were reported to be markedly higher in patients with coronary artery disease (17). Fernandez-García et al. suggested that increased Gal-3 levels are associated with AAA because of the CCL5 expression caused by STAT3 activation (18).
Inflammation caused by macrophage activation is a pathological marker of AAA progression. Although Gal-3 is reportedly associated with AAA, little is known about its potential application as a biomarker for AAA. While IL-6, as well as Gal-3, is an inflammatory mediator. IL-6 has a critical role in elevating the circulating concentrations of several plasma proteins, including fibrinogen and C-reactive protein (CRP). Therefore, IL-6 has been identified as an essential pro-inflammatory cytokine in the pathogenesis of AAA (7, 19, 20). However, the prognostic value of IL-6 is controversial. The present study aimed to characterize the potential prognostic value of Gal-3. Our results indicated that Gal-3 was an independent variable for identifying subaneurysmal aortic dilatation and large aneurysms compared with IL-6.
Materials and Methods
Patient Recruitment
Plasma samples were collected from 151 patients diagnosed as having an AAA by computed tomography who underwent open surgical repair or endovascular aneurysm repair (EVAR) or those diagnosed as having subaneurysmal aortic dilatation at the Taipei Veterans General Hospital between 2016 and 2018. AAA and subaneurysmal aortic dilatation were diagnosed through ultrasound examination. Other inclusion criteria for patients with AAA were aortic diameter of ≥30 mm and age of 20–80 years. Patients with dissecting, infectious, traumatic, or inflammatory AAA and those with coexisting malignant tumors were excluded. The control group comprised 195 patients who regularly visited the cardiology clinic and had a normal aortic diameter (infrarenal aortic diameter of <30 mm) without a history of ischemic heart disease or renal insufficiency. The study patients were divided into three groups based on aortic diameter: control (infrarenal aortic diameter, <30 mm), large AAA (LAA, infrarenal aortic diameter, ≥55 mm), and small AAA (SAA, infrarenal aortic diameter, 30–55 mm) groups. We used G*power to determine the sample size with 0.99. Information on smoking status, hypertension, dyslipidemia, diabetes mellitus, chronic obstructive pulmonary disease, and use of β-blockers, angiotensin-converting enzyme inhibitors, angiotensin receptor blockers, statins, or calcium channel blockers was recorded. All procedures followed were in accordance with the Declaration of Helsinki and the ethical standards of the responsible committee on human experimentation (Taipei Veterans General Hospital). All patients signed consent forms before participation.
Sample Collection and Biomarker Measurement
Peripheral blood was collected from each participant in ethylenediaminetetraacetic acid tubes. All samples were processed within 3 h of collection. The blood samples were centrifuged (3,000 × g, 10 min, 4°C) to remove cells and debris. The supernatants were transferred to ribonuclease-free tubes and stored at −80°C until analysis. Gal-3 and IL-6 levels were measured using Human ProcartaPlex Multiplex and Simplex kits (Thermo Fisher Scientific, Waltham, MA, USA).
Statistical Analysis
Dichotomous variables were expressed as proportions; cases and controls were compared using the chi-squared test, whereas continuous variables were examined using the Mann–Whitney U-test to identify potential confounders. Associations with a probability of <0.10 were considered potential confounders and used in the multivariate analyses. The association between Gal-3 and IL-6 was studied using Spearman's correlation analysis. A multivariate logistic regression analysis was performed to assess the association between Gal-3 and the risk of AAA after adjustment for the identified potential confounders. These data analyses were performed using PRISM software (version 5.0, GraphPad, San Diego, CA, USA). A one-way analysis of variance with Tukey's post-hoc test was used to evaluate between-group differences. Statistical significance was defined as p < 0.05.
Results
We performed a cross-sectional study to analyze plasma Gal-3 and IL-6 levels in both control patients (n = 195) and patients with AAA (n = 151). The demographic characteristics are presented in Table 1. Age, sex, hypertension, smoking, hypercholesterolemia, diabetes mellitus, peripheral vascular disease, and chronic obstructive pulmonary disease as well as the use of angiotensin-converting enzyme inhibitors, angiotensin receptor blockers, statins, β-blockers, and calcium channel blockers differed significantly between the two groups.
Plasma Gal-3 levels were significantly higher in patients with AAA than in control patients (96.9 ± 4.5L vs. 76.5 ± 1.9 ng/mL, Figure 1A). In this sample set, we analyzed the plasma concentrations of IL-6, which is reportedly associated with AAA (7, 19, 20). The levels of IL-6 were higher in AAA samples than in healthy control samples (92.8 ± 5.2 pg/mL vs. 72.5 ± 3.0 pg/mL; Figure 1B).
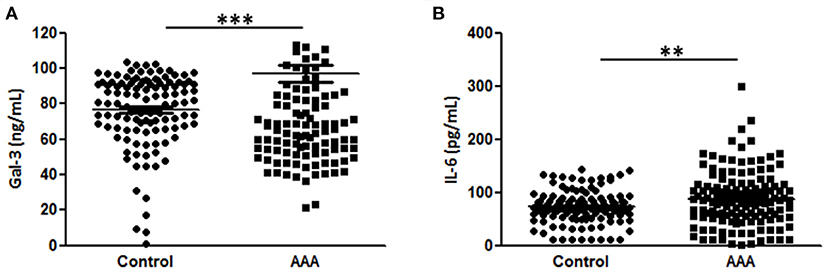
Figure 1. Diagnostic value of Gal-3 and IL-6. (A) Gal-3 and (B) IL-6 concentrations in plasma samples from patients with AAA (n = 151) and control patients (n = 195). Data are expressed as mean ± standard error of the mean. **p < 0.001, ***p < 0.001.
We performed a classification and regression tree (CART) analysis and the diagnostic values of Gal-3 and IL-6 were evaluated using receiver operating characteristic curve analysis (Figure 2A). The results revealed that Gal-3 levels predicted AAA presence (area under the curve [95% confidence interval], 0.91 [0.83–0.92]) significantly more accurately than did IL-6 levels (0.72 [0.62–0.74]). A poor correlation was observed between plasma Gal-3 and IL-6 levels in patients with AAA (Pearson's r2 = 0.05, Figure 2B). Using AAA as the dependent variable by using CART analysis, we divided patients into two categories based on plasma Gal-3 levels: low (<68.95 ng/mL) and high (≥68.95 ng/mL; Figure 2C). The low Gal-3 group included 27.9% of patients with AAA, whereas the high Gal-3 group included 55.1% of patients with AAA. Furthermore, CART analysis divided the high Gal-3 group into two categories-those with Gal-3 concentrations of <99.55 ng/mL and ≥99.55 ng/mL. It is noteworthy that the 98.8% of patients with Gal-3 ≥99.55 ng/mL had AAA (p <2.2e-16).
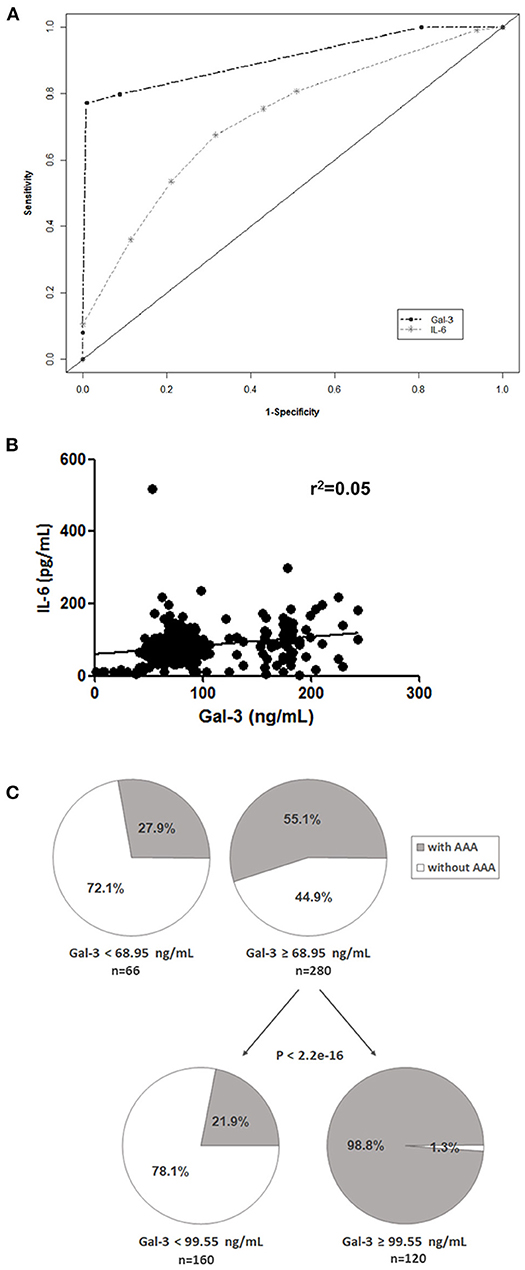
Figure 2. Potential prognostic value of Gal-3. (A) Receiver operating characteristic curves of Gal-3 and IL-6 were used to differentiate patients with AAA from control patients. (B) Correlation between Gal-3 and IL-6 levels in patients with AAA and control patients. (C) CART analysis with plasma Gal-3 levels as the independent variable and AAA as the dependent variable (all patients included).
To further examine the efficacy of Gal-3 for AAA diagnosis, we performed a logistic regression analysis after adjustment for age, sex, hypertension, smoking, hypercholesterolemia, diabetes mellitus, chronic obstructive pulmonary disease, and medication usage. The significant association between circulating Gal-3 levels and AAA persisted after adjustment for those factors (Table 2).
Moreover, we analyzed the association of Gal-3 with aortic diameter as a surrogate marker of AAA evolution. Plasma Gal-3 levels were significantly correlated with aortic diameter after adjustment for potential confounding factors (Table 3). A subgroup analysis indicated that IL-6 levels were markedly higher in the LAA group compared with in the control group, but no significant difference was observed between the SAA and LAA groups (Figure 3A). However, Gal-3 was an independent variable for identifying subaneurysmal aortic dilatation and large aneurysms (Figure 3B).
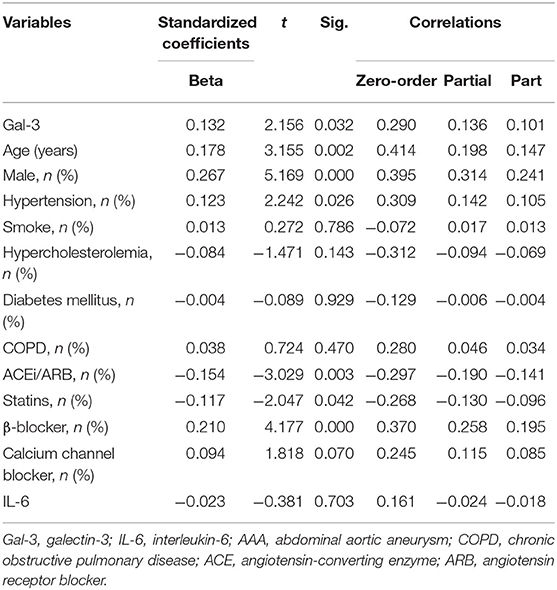
Table 3. Multivariate linear analysis of Gal-3 for aortic diameter, including partial correlation coefficients.
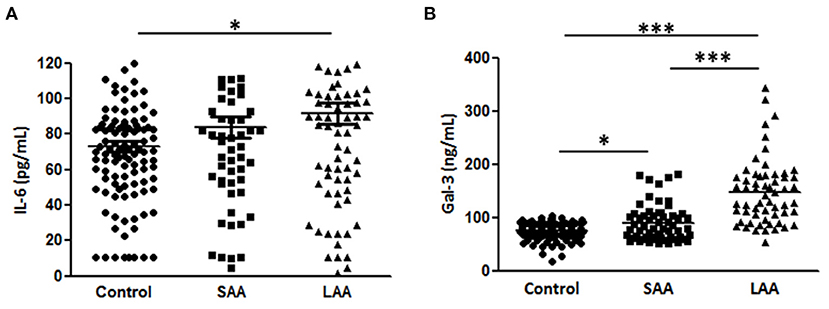
Figure 3. Gal-3 and IL-6 levels according to aneurysm size. (A) Gal-3 and (B) IL-6 concentrations in plasma samples from patients with a normal aortic diameter (control, n = 195), small aortic aneurysm (SAA, n = 58), and large aortic aneurysm (LAA, n = 93). Data are expressed as mean ± standard error of the mean. *p < 0.05, ***p < 0.0001.
Discussion
The inflammatory process appears critical in the formation of AAAs, as demonstrated by extensive medial and adventitial inflammatory cell infiltration (21). Increased expression of proinflammatory cytokines is observed in aneurysmal tissue, and patients with AAA have elevated levels of circulating inflammatory cytokines (22, 23). Cytokines regulate the expression of MMPs, serine proteases, and cathepsin proteases, and local cytokine environments can drive aneurysm formation (24). Thus, circulation markers representing AAA pathology can be valuable for numerous reasons, such as in AAA diagnosis and prognosis. Although ultrasound is the gold standard for the diagnosis and surveillance of AAAs, with high sensitivity and specificity (25, 26), the frequency of ultrasound surveillance varies with aneurysm diameter. Furthermore, ultrasound is not recommended for patients with subaneurysmal aortic dilatation (27). Therefore, circulating biomarkers of inflammation, which reflect the aneurysmal size, can assist in the detection and prognosis of AAA (28). This study investigated the associations of two inflammatory markers (Gal-3 and IL-6) with aneurysmal size in patients with AAA and controls.
Patients with AAA have high levels of systemic inflammatory acute-phase reactants, such as hs-C-reactive protein, IL-1β, IL-6, interferon-γ, tumor necrosis factor-α, α-1-antitrypsin, orosomucoid, haptoglobin, and fibrinogen (5, 29–31). Furthermore, the cytokines IL-β, IL-6, IL-8, tumor necrosis factor-α, PGE2, and CCL2 were upregulated in AAAs compared with normal aortic samples (23, 31–35). Taken together, these findings have emphasized that inflammation is a crucial aspect of AAA pathogenesis. The aneurysmal growth rate is closely correlated with aortic size, with larger aneurysms having a higher growth rate (36, 37). A large population-based cohort study suggested that plasma Gal-3 concentration has a moderate positive association with AAA incidence (38). Our findings confirmed that Gal-3 is a specific marker of AAA. Gal-3 is likely a chemotactic molecule for macrophages (12). Thus, its expression could be associated with various cardiovascular diseases. The increased risk of AAA observed in patients with higher Gal-3 levels may reflect the recruitment of inflammatory cells, including activated macrophages, in the arterial system and the subsequent secretion of Gal-3. Moreover, Gal-3 expression is enhanced when macrophages or aortic vascular SMCs are loaded with lipids and transformed into foam cells. Therefore, Gal-3 can be a marker of vascular SMC phenotype switching (39).
A study revealed a positive correlation between aneurysm surface area and mean IL-6 level (Spearman's rank correlation r = 0.48; p = 0.003) (40). Furthermore, Flondell-Site et al. reported a significant correlation between AAA size and IL-6 levels (Spearman's r = 0.237, p < 0.0001) (41). However, Juvonen et al. observed no correlation between IL-6 levels and aneurysm diameter or expansion (22), and Lindqvist et al. identified no significant correlation between IL-6 levels and the maximum diameter of unruptured AAAs (42). In the present study, multivariate logistic regression analysis also failed to demonstrate a significant association between IL-6 levels and AAA. Therefore, the role of IL-6 in predicting AAA progression remains controversial.
This study has several limitations. Our sample size was not high enough to yield sufficient power for determining the clinical significance of these biomarkers. Information on the time between biomarker identification and disease outcome was not available in this cross-sectional study without prospective follow-up. Therefore, we could not reach conclusions regarding aneurysm progression. Furthermore, performing multiple comparisons is associated with a risk of false significance. However, we attempted to minimize this risk by using univariate and multivariate analyses.
Conclusions
Circulating Gal-3 may be an independent variable for distinguishing between subaneurysmal aortic dilatation and large aneurysms. Further investigation is required to determine whether Gal-3 predicts aneurysm growth.
Data Availability Statement
The original contributions presented in the study are included in the article/supplementary material, further inquiries can be directed to the corresponding author/s.
Ethics Statement
The studies involving human participants were reviewed and approved by Taipei Veterans General Hospital. Reference number: 2016-07-013AC. The patients/participants provided their written informed consent to participate in this study.
Author Contributions
H-YL performed the experiments. H-YL and C-MS analyzed the data. H-YL and S-HS interpreted the results of the experiments. H-YL and AW prepared the figures. H-YL and T-MC drafted the manuscript. Y-CL and C-CS edited and revised the manuscript. Y-CL conceived and designed the research. All authors approved the final version of the manuscript.
Funding
This work was supported by Taipei Medical University (TMU108-F-005) and Wan Fang Hospital, Taipei Medical University (109-wf-eva-02, 109-wf-eva-07, and 109TMU-WFH-19) in Taiwan.
Conflict of Interest
The authors declare that the research was conducted in the absence of any commercial or financial relationships that could be construed as a potential conflict of interest.
Acknowledgments
We thank Chih-Han Li for her technical assistance.
References
1. Sakalihasan N, Limet R, Defawe DO. Abdominal aortic aneurysm. Lancet. (2005) 365:1577–89. doi: 10.1016/S0140-6736(05)66459-8
2. Michel JB, Martin-Ventura JL, Egido J, Sakalihasan N, Treska V, Lindholt J, et al. Novel aspects of the pathogenesis of aneurysms of the abdominal aorta in humans. Cardiovasc Res. (2011) 90:18–27. doi: 10.1093/cvr/cvq337
3. Lopez-Candales A, Holmes DR, Liao S, Scott MJ, Wickline SA, Thompson RW. Decreased vascular smooth muscle cell density in medial degeneration of human abdominal aortic aneurysms. Am J Pathol. (1997) 150:993–1007.
4. McCormick ML, Gavrila D, Weintraub NL. Role of oxidative stress in the pathogenesis of abdominal aortic aneurysms. Arterioscler Thromb Vasc Biol. (2007) 27:461–9. doi: 10.1161/01.ATV.0000257552.94483.14
5. Choke E, Cockerill G, Wilson WR, Sayed S, Dawson J, Loftus I, et al. A review of biological factors implicated in abdominal aortic aneurysm rupture. Eur J Vasc Endovasc Surg. (2005) 30:227–44. doi: 10.1016/j.ejvs.2005.03.009
6. Rizas KD, Ippagunta N, Tilson MD 3rd. Immune cells and molecular mediators in the pathogenesis of the abdominal aortic aneurysm. Cardiol Rev. (2009) 17:201–10. doi: 10.1097/CRD.0b013e3181b04698
7. Takagi H, Watanabe T, Mizuno Y, Kawai N, Umemoto TG. All-Literature investigation of cardiovascular evidence: circulating interleukin-6 levels are associated with abdominal aortic aneurysm presence: a meta-analysis and meta-regression of case-control studies. Ann Vasc Surg. (2014) 28:1913–22. doi: 10.1016/j.avsg.2014.06.058
8. Cheng XW, Huang Z, Kuzuya M, Okumura K, Murohara T. Cysteine protease cathepsins in atherosclerosis-based vascular disease and its complications. Hypertension. (2011) 58:978–86. doi: 10.1161/HYPERTENSIONAHA.111.180935
9. Qin Y, Shi G-P. Cysteinyl cathepsins and mast cell proteases in the pathogenesis and therapeutics of cardiovascular diseases. Pharmacol Ther. (2011) 131:338–50. doi: 10.1016/j.pharmthera.2011.04.010
10. Barondes SH, Castronovo V, Cooper DN, Cummings RD, Drickamer K, Feizi T, et al. Galectins: a family of animal beta-galactoside-binding lectins. Cell. (1994) 76:597–8. doi: 10.1016/0092-8674(94)90498-7
11. Papaspyridonos M, McNeill E, de bono JP, Smith A, Burnand KG, Channon KM, et al. Galectin-3 is an amplifier of inflammation in atherosclerotic plaque progression through macrophage activation and monocyte chemoattraction. Arterioscler Thromb Vasc Biol. (2008) 28:433–40. doi: 10.1161/ATVBAHA.107.159160
12. Sano H, Hsu DK, Yu L, Apgar JR, Kuwabara I, Yamanaka T, et al. Human galectin-3 is a novel chemoattractant for monocytes and macrophages. J Immunol. (2000) 165:2156–64. doi: 10.4049/jimmunol.165.4.2156
13. Besler C, Lang D, Urban D, Rommel KP, von Roeder M, Fengler K, et al. Plasma and cardiac galectin-3 in patients with heart failure reflects both inflammation and fibrosis: implications for its use as a biomarker. Circ Heart Fail. (2017) 10:e003804. doi: 10.1161/CIRCHEARTFAILURE.116.003804
14. Martinez-Martinez E, Calvier L, Fernandez-Celis A, Rousseau E, Jurado-Lopez R, Rossoni LV, et al. Galectin-3 blockade inhibits cardiac inflammation and fibrosis in experimental hyperaldosteronism and hypertension. Hypertension. (2015) 66:767–75. doi: 10.1161/HYPERTENSIONAHA.115.05876
15. Sharma UC, Pokharel S, van Brakel TJ, van Berlo JH, Cleutjens JP, Schroen B, et al. Galectin-3 marks activated macrophages in failure-prone hypertrophied hearts and contributes to cardiac dysfunction. Circulation. (2004) 110:3121–8. doi: 10.1161/01.CIR.0000147181.65298.4D
16. de Boer RA, Daniels LB, Maisel AS, Januzzi JL Jr. State of the art: newer biomarkers in heart failure. Eur J Heart Fail. (2015) 17:559–69. doi: 10.1002/ejhf.273
17. Kusaka H, Yamamoto E, Hirata Y, Fujisue K, Tokitsu T, Sugamura K, et al. Clinical significance of plasma galectin-3 in patients with coronary artery disease. Int J Cardiol. (2015) 201:532–4. doi: 10.1016/j.ijcard.2015.08.099
18. Fernandez-Garcia CE, Tarin C, Roldan-Montero R, Martinez-Lopez D, Torres-Fonseca M, Lindhot JS, et al. Increased galectin-3 levels are associated with abdominal aortic aneurysm progression and inhibition of galectin-3 decreases elastase-induced AAA development. Clin Sci. (2017) 131:2707–19. doi: 10.1042/CS20171142
19. Harrison SC, Smith AJ, Jones GT, Swerdlow DI, Rampuri R, Bown MJ, et al. Interleukin-6 receptor pathways in abdominal aortic aneurysm. Eur Heart J. (2013) 34:3707–16. doi: 10.1093/eurheartj/ehs354
20. Paige E, Clement M, Lareyre F, Sweeting M, Raffort J, Grenier C, et al. Interleukin-6 receptor signaling and abdominal aortic aneurysm growth rates. Circ Genom Precis Med. (2019) 12:e002413. doi: 10.1101/428516
21. Hellenthal FA, Geenen IL, Teijink JA, Heeneman S, Schurink GW. Histological features of human abdominal aortic aneurysm are not related to clinical characteristics. Cardiovasc Pathol. (2009) 18:286–93. doi: 10.1016/j.carpath.2008.06.014
22. Juvonen J, Surcel HM, Satta J, Teppo AM, Bloigu A, Syrjala H, et al. Elevated circulating levels of inflammatory cytokines in patients with abdominal aortic aneurysm. Arterioscler Thromb Vasc Biol. (1997) 17:2843–7. doi: 10.1161/01.ATV.17.11.2843
23. Newman KM, Jean-Claude J, Li H, Ramey WG, Tilson MD. Cytokines that activate proteolysis are increased in abdominal aortic aneurysms. Circulation. (1994) 90(5 Pt 2):II224–7.
24. Shimizu K, Libby P, Mitchell RN. Local cytokine environments drive aneurysm formation in allografted aortas. Trends Cardiovasc Med. (2005) 15:142–8. doi: 10.1016/j.tcm.2005.05.003
25. Guirguis-Blake JM, Beil TL, Senger CA, Whitlock EP. Ultrasonography screening for abdominal aortic aneurysms: a systematic evidence review for the U.S. preventive services task force. Ann Intern Med. (2014) 160:321–9. doi: 10.7326/M13-1844
26. Collaborators R, Bown MJ, Sweeting MJ, Brown LC, Powell JT, Thompson SG. Surveillance intervals for small abdominal aortic aneurysms: a meta-analysis. JAMA. (2013) 309:806–13. doi: 10.1001/jama.2013.950
27. Chun KC, Teng KY, Van Spyk EN, Carson JG, Lee ES. Outcomes of an abdominal aortic aneurysm screening program. J Vasc Surg. (2013) 57:376–81. doi: 10.1016/j.jvs.2012.08.038
28. Golledge J, Tsao PS, Dalman RL, Norman PE. Circulating markers of abdominal aortic aneurysm presence and progression. Circulation. (2008) 118:2382–92. doi: 10.1161/CIRCULATIONAHA.108.802074
29. Golledge J, Muller J, Daugherty A, Norman P. Abdominal aortic aneurysm: pathogenesis and implications for management. Arterioscler Thromb Vasc Biol. (2006) 26:2605–13. doi: 10.1161/01.ATV.0000245819.32762.cb
30. Lindholt JS, Shi GP. Chronic inflammation, immune response, and infection in abdominal aortic aneurysms. Eur J Vasc Endovasc Surg. (2006) 31:453–63. doi: 10.1016/j.ejvs.2005.10.030
31. Middleton RK, Lloyd GM, Bown MJ, Cooper NJ, London NJ, Sayers RD. The pro-inflammatory and chemotactic cytokine microenvironment of the abdominal aortic aneurysm wall: a protein array study. J Vasc Surg. (2007) 45:574–80. doi: 10.1016/j.jvs.2006.11.020
32. Lenk GM, Tromp G, Weinsheimer S, Gatalica Z, Berguer R, Kuivaniemi H. Whole genome expression profiling reveals a significant role for immune function in human abdominal aortic aneurysms. BMC Genomics. (2007) 8:237. doi: 10.1186/1471-2164-8-237
33. Treska V, Kocova J, Boudova L, Neprasova P, Topolcan O, Pecen L, et al. Inflammation in the wall of abdominal aortic aneurysm and its role in the symptomatology of aneurysm. Cytokines Cell Mol Ther. (2002) 7:91–7. doi: 10.1080/13684730310001652
34. Tung WS, Lee JK, Thompson RW. Simultaneous analysis of 1176 gene products in normal human aorta and abdominal aortic aneurysms using a membrane-based complementary DNA expression array. J Vasc Surg. (2001) 34:143–50. doi: 10.1067/mva.2001.113310
35. Koch AE, Kunkel SL, Pearce WH, Shah MR, Parikh D, Evanoff HL, et al. Enhanced production of the chemotactic cytokines interleukin-8 and monocyte chemoattractant protein-1 in human abdominal aortic aneurysms. Am J Pathol. (1993) 142:1423–31.
36. Norman P, Spencer CA, Lawrence-Brown MM, Jamrozik K. C-reactive protein levels and the expansion of screen-detected abdominal aortic aneurysms in men. Circulation. (2004) 110:862–6. doi: 10.1161/01.CIR.0000138746.14425.00
37. Rohde LE, Arroyo LH, Rifai N, Creager MA, Libby P, Ridker PM, et al. Plasma concentrations of interleukin-6 and abdominal aortic diameter among subjects without aortic dilatation. Arterioscler Thromb Vasc Biol. (1999) 19:1695–9. doi: 10.1161/01.ATV.19.7.1695
38. Fashanu OE, Folsom AR, Oyenuga A, Ballantyne CM, Lutsey PL, Tang W. Galectin-3 and the incidence of abdominal aortic aneurysm: the atherosclerosis risk in communities (ARIC) study. Am J Cardiovasc Dis. (2017) 7:114–21.
39. Rong JX, Shapiro M, Trogan E, Fisher EA. Transdifferentiation of mouse aortic smooth muscle cells to a macrophage-like state after cholesterol loading. Proc Natl Acad Sci USA. (2003) 100:13531–6. doi: 10.1073/pnas.1735526100
40. Dawson J, Cockerill GW, Choke E, Belli AM, Loftus I, Thompson MM. Aortic aneurysms secrete interleukin-6 into the circulation. J Vasc Surg. (2007) 45:350–6. doi: 10.1016/j.jvs.2006.09.049
41. Flondell-Site D, Lindblad B, Kolbel T, Gottsater A. Cytokines and systemic biomarkers are related to the size of abdominal aortic aneurysms. Cytokine. (2009) 46:211–5. doi: 10.1016/j.cyto.2009.01.007
Keywords: galectin-3, interlukin-6, abdominal aortic aneurysm, inflammation, biomarker
Citation: Lu H-Y, Shih C-M, Sung S-H, Wu ATH, Cheng T-M, Lin Y-C and Shih C-C (2021) Galectin-3 as a Biomarker for Stratifying Abdominal Aortic Aneurysm Size in a Taiwanese Population. Front. Cardiovasc. Med. 8:663152. doi: 10.3389/fcvm.2021.663152
Received: 02 February 2021; Accepted: 05 May 2021;
Published: 31 May 2021.
Edited by:
Masuko Ushio-Fukai, Augusta University, United StatesReviewed by:
Bhupesh Singla, Augusta University, United StatesShiyou Chen, University of Missouri, United States
Copyright © 2021 Lu, Shih, Sung, Wu, Cheng, Lin and Shih. This is an open-access article distributed under the terms of the Creative Commons Attribution License (CC BY). The use, distribution or reproduction in other forums is permitted, provided the original author(s) and the copyright owner(s) are credited and that the original publication in this journal is cited, in accordance with accepted academic practice. No use, distribution or reproduction is permitted which does not comply with these terms.
*Correspondence: Yen-Chung Lin, yclin0229@tmu.edu.tw; Chun-Che Shih, profccshih@gmail.com
†These authors have contributed equally to this work and share last authorship