- Department of Cardiac, Thoracic, Vascular Sciences and Public Health, University of Padua Medical School, Padua, Italy
Anatomy of subepicardial coronary arteries became a topic of investigation at autopsy in Florence (Italy) by Banchi in the early twentieth century, with the discovery of dominant and balanced patterns. Thereafter, in the 60's of the same century Baroldi in Milan did post-mortem injection with spectacular three-dimensional casts. Later Sones at the Cleveland Clinic introduced selective coronary arteriography for in vivo visualization of coronary arteries. In the present chapter we show these patterns, as well as normal variants of origin and course with questionable risk of ischemia, like myocardial bridge as well as origin of the left circumflex coronary artery from the right sinus with retroaortic course. As far as embryology, the coronary arteries and veins are epicardial in origin and finally connect the former with the aorta, and the latter with the sinus venosus. At the time of spongy myocardium, intramural blood supply derives directly by the ventricular cavities, whereas later, at the time of myocardial compaction, vascularization originates from the subepicardial network. The connection of the subepicardial plexus with the aorta occurs with prongs of the peritruncal ring, which penetrate the facing aortic sinuses. Septation of truncus arteriosus is not responsible for the final position of the coronary orifices. Infact in transposition of the great arteries coronary ostia are regularly located within facing sinuses of the anterior aorta.
Introduction
This chapter on anatomy and embryology of coronary arteries (CAs) has been written having in mind that the target readers are clinicians. Explaining embryology is a difficult task, and we did our best to simplify the message and facilitate the comprehension. Coronary arteries anomalies and their clinical implications are the argument of another chapter from our group for this e-book.
Moreover, it has been our deliberate intention to cover some history of CA anatomy, with relevant illustrations, to enhance the interest of the readers.
Historical Notes
The pathology of CAs started to be a topic of interest and investigation at the beginning of the twentieth century, when myocardial infarction was found to be related to coronary obstruction. Even Morgagni in his book, dated 1761, skipped the issue (1).
Antonio Banchi, an anatomist in Florence, in 1903 first published a milestone paper, written in Italian, on subepicardial CAs in normal hearts and introduced the concept of right and left dominance as well as balanced coronary circulation (2), according to the CA supply of the posterior wall of the left ventricle (Figure 1).
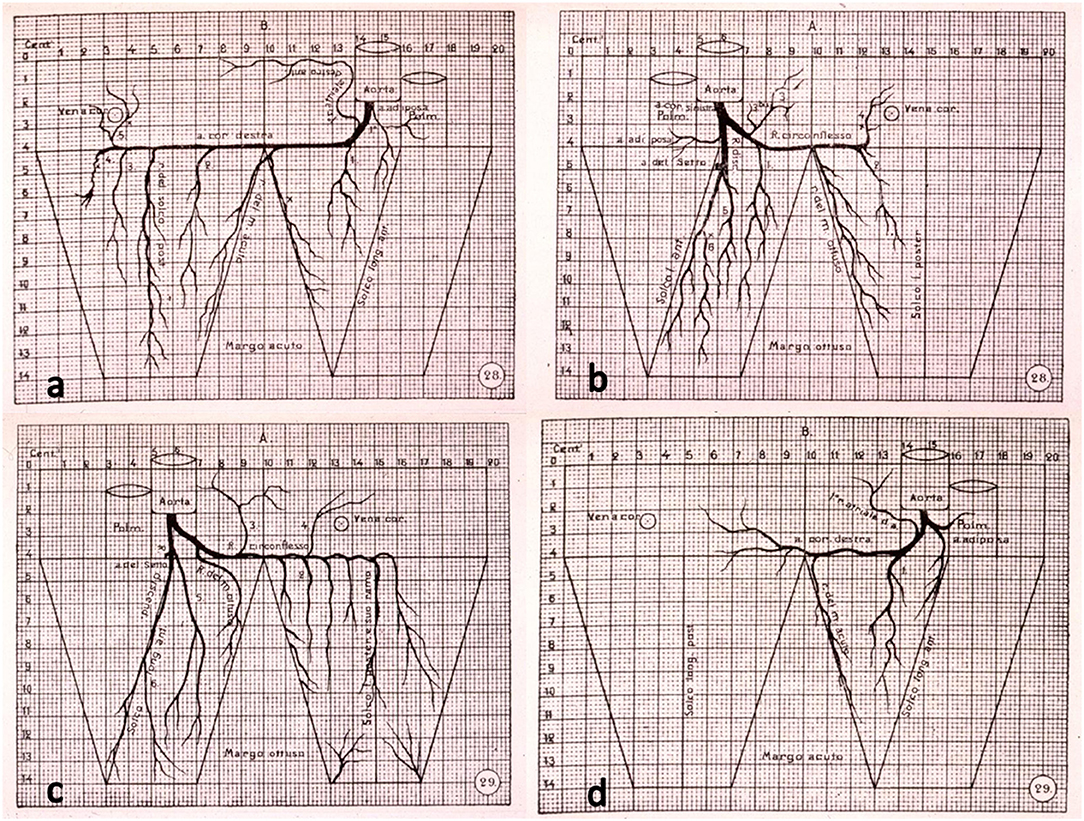
Figure 1. The original drawings of coronary artery dominant patterns, from Banchi (2). (a,b) Right dominance and (c,d) left dominance.
These anatomical patterns of the coronary arterial system were confirmed through postmortem injection and casts by Giorgio Baroldi in 1963 (Figure 2) (3). The plastic substances were “Geon Latex 576” and “Neoprene 842A.” These substances, which are fluid at room temperature, solidify at 40–50°C. They were injected into the aorta under pressure, ranging from 130 to 200 mmHg, maintained for a 5- to 10-min period.
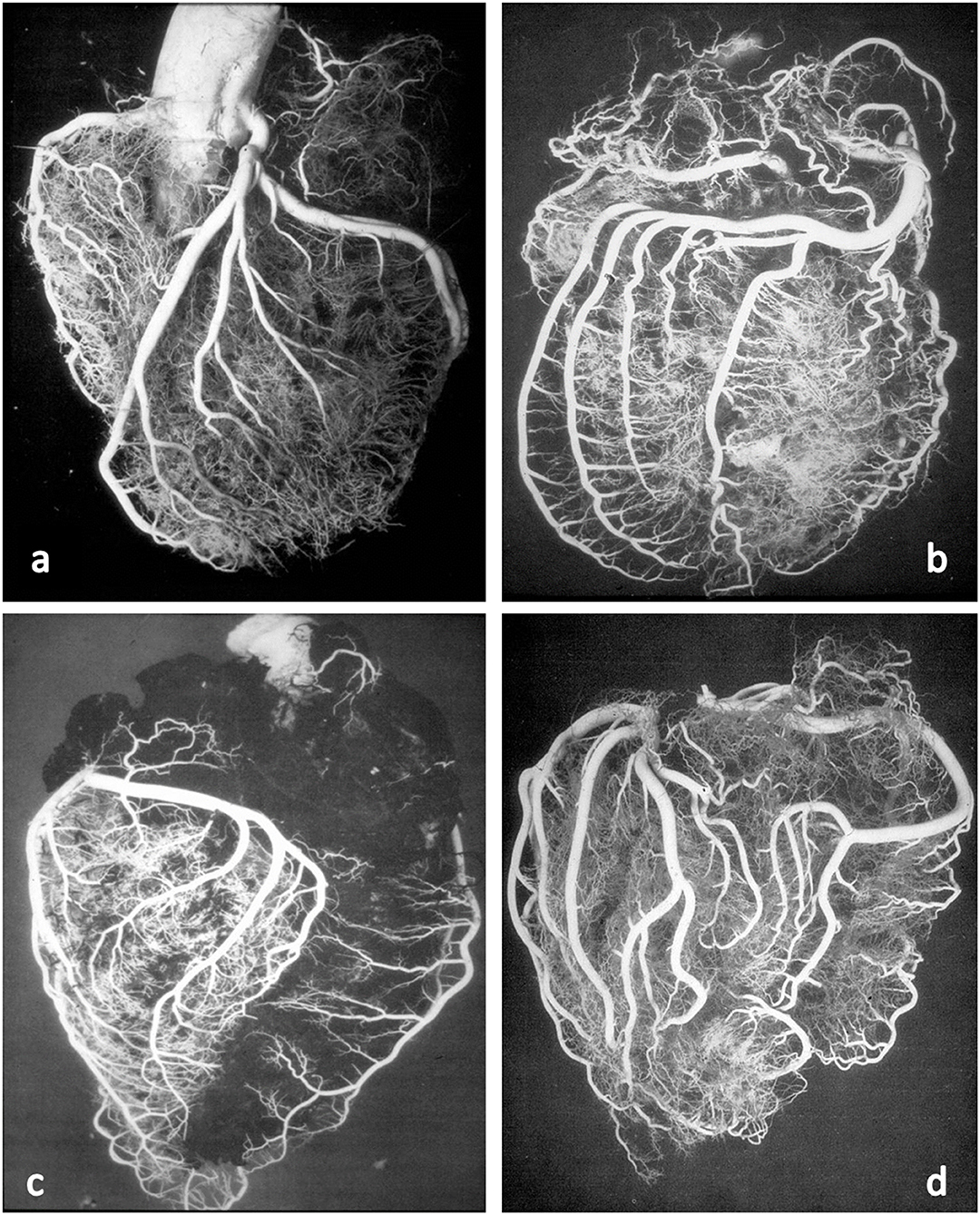
Figure 2. Postmortem casts of coronary arteries. From Baroldi and Scomazzoni (3). (a) Left coronary artery anatomy, (b) dominant right pattern, (c) dominant left pattern, and (d) balanced pattern.
With the latex maintained under pressure, it was solidified by placing the heart in 10% formalin at 40–50°C from 48 to 72 h. Following solidification of the latex, the heart was allowed to further fix in a new 10% formalin solution at room temperature for a period of 12–48 h. Finally, the injected and fixed heart was digested in a concentrated hydrochloric acid solution.
The invention and clinical diagnostic application of selective coronary arteriography by Sones at the Cleveland Clinic (4) permitted to identify these arterial patterns in vivo, as a requisite for bypass surgery.
Anatomy of Coronary Arteries
The CAs take origin from the sinus portion of the aortic root, with the orifices located usually at the sino-tubular junction, with a variability of up to 2.5 mm (5) (Figures 3, 4). The left coronary ostium is located in the left anterior aortic sinus and the right coronary ostium in the right anterior aortic sinus, namely, the aortic sinuses facing the pulmonary artery (6) (Figure 5). Right and left CAs originate perpendicularly from the aorta, and their proximal course is not hindered by the pulmonary trunk (Figure 5). Usually, a single orifice is located in the left aortic sinus, giving origin to the left main trunk, which divides into anterior descending and left circumflex coronary branches (Figures 2a, 5). The course of the former occurs over the interventricular septum while the latter in the left atrioventricular (av) groove, with different lengths according to the dominant pattern. An intermediate artery may originate in between the two, so that the left main stem trifurcates. From the left anterior descending CA, diagonal lateral arteries take origin, for the blood supply of antero-lateral free wall of the left ventricle (Figure 2a), and perforating arteries, for the blood supply of two-thirds of the anterior ventricular septum (Figure 6).
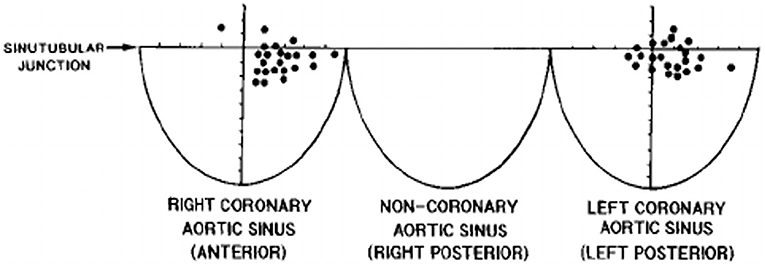
Figure 3. The topographical variability of coronary artery orifices in normal hearts. From Muriago et al. (5).
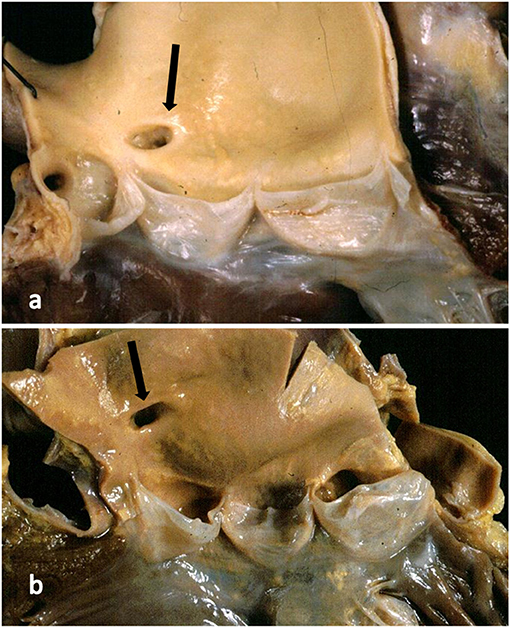
Figure 4. (a) The right coronary artery orifice (arrow) is just above (2 mm) the sinotubular junction, within normal limits. (b) The right coronary orifice (arrow) is well above the sinotubular junction (10 mm), over the threshold of normal limits.
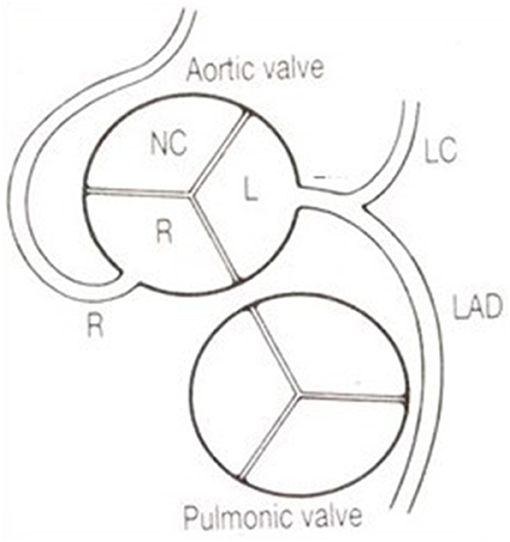
Figure 5. The coronary arteries arise from the facing aortic sinuses, perpendicular to the aortic wall, and the pulmonary root does not interfere with their proximal course. Note the left stem dividing into the left anterior descending (LAD) and the left circumflex (LC) branches. From Roberts (6).
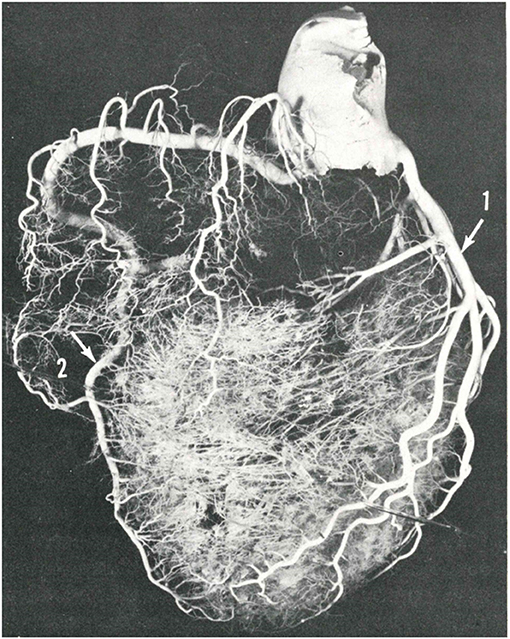
Figure 6. The blood flow of the ventricular septum is supported by perforating branches, originating from the anterior and posterior descending coronary arteries. From Baroldi and Scomazzoni (3).
A normal variant is the double descending CA (Figure 7), the interventricular one originating septal arteries and the other one diagonal branches.
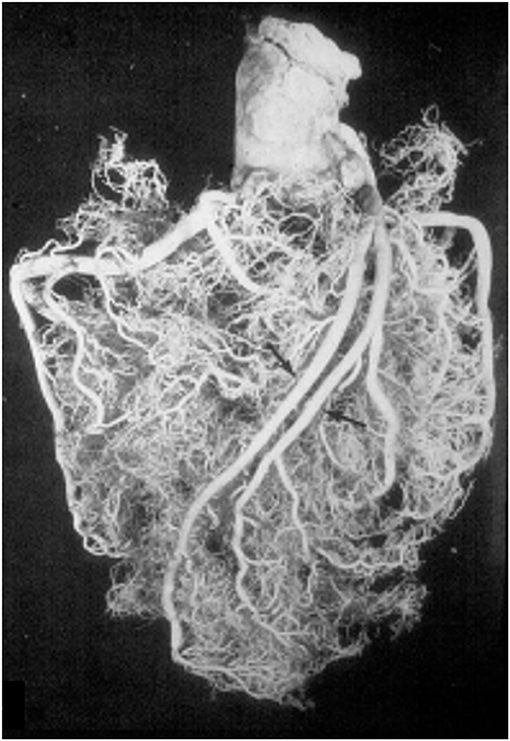
Figure 7. A double left anterior descending coronary artery (arrows). From Baroldi and Scomazzoni (3).
From the left circumflex artery, the obtuse angle branch takes origin to supply the left lateral wall. It may represent the end of the left circumflex artery, in case of extremely dominant right CA.
According to Baroldi (3), a right dominant pattern is present in 79% of cases, left dominant in 7% and balanced in 14%. In two-thirds of the cases, the left anterior descending CA turns at the apex and runs in the posterior interventricular groove, even up to the crux cordis.
The left circumflex artery may originate from a separate orifice of the left aortic sinus, a condition considered a normal variant since it does not imply myocardial ischemia.
The same occurs to the right CA, since a conus artery almost regularly arises from a separate, anteriorly located small orifice. The right CA runs in the right av groove and reaches the crux cordis in a right dominant pattern, giving origin to the descending CA (Figures 1a, 2b) to take over the blood supply of the posterior third of the ventricular septum. It continues in the posterior left av sulcus, originating branches for the blood supply of the posterior left ventricular free wall.
Branches for the conduction system take origin from the dominant right CA, proximally the sinoatrial node artery and distally the av node artery from the crux cordis. In the setting of left dominant CA, both of these two small arteries originate from the left circumflex artery.
Another quite intriguing condition is the origin of the left circumflex artery from the right CA or from the right anterior aortic sinus itself, with a retroaortic course before reaching the left av sulcus (Figure 8). It has been observed in cases of sudden death as infarct-related artery in a postero-lateral myocardial infarction in the absence of any other explanation (7).
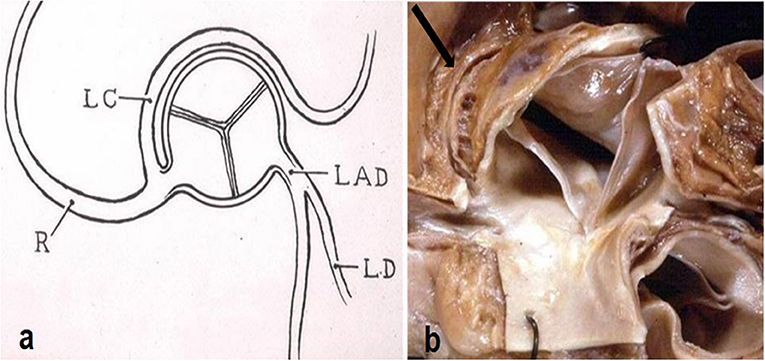
Figure 8. (a) Diagram with origin of the left circumflex artery from the right coronary artery and retroaortic course. From Roberts (6). (b) Gross view of the aortic root. Arrow indicates the retroaortic course of the anomalous left circumflex artery.
Nearly 30% of the population shows an intramural course of the proximal left descending CA (8–11) (Figure 9). It is considered a variant of normal when just covered by a myocardial bridge. It may be a cause of myocardial ischemia if completely surrounded by a sleeve of the myocardium with disarray and an intramyocardial course at least 2.5 cm long and 0.5 cm deep (9–11) (Figure 10). The condition is particularly at risk when the intramyocardial course is just over the first septal perforating artery (Figure 6).
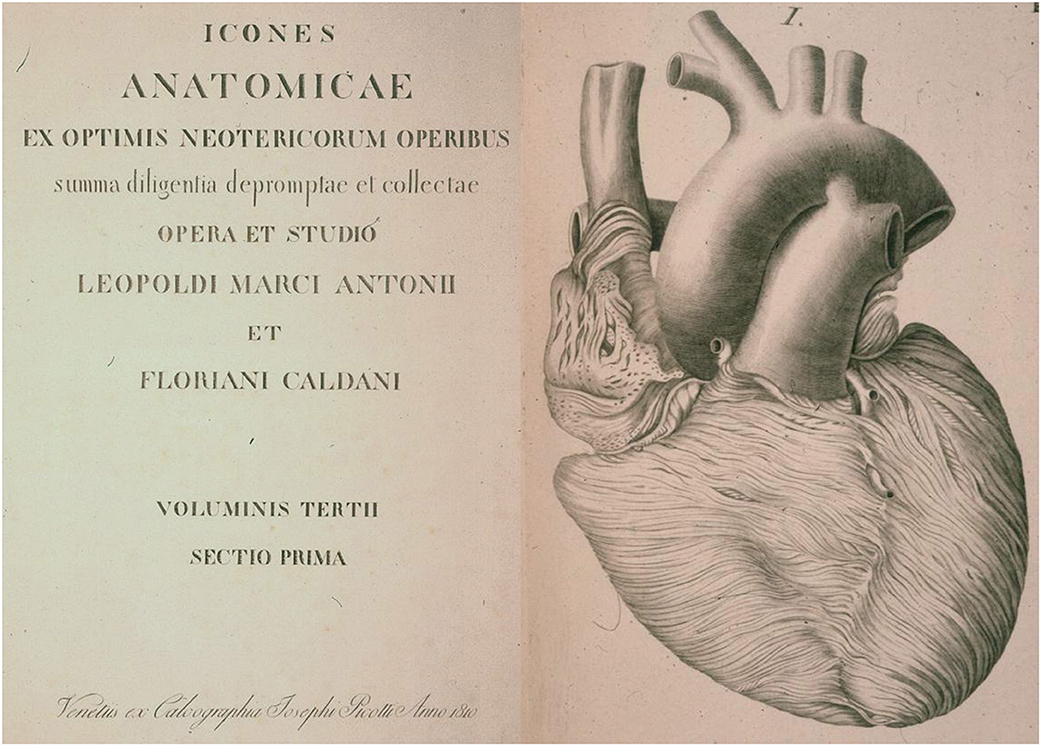
Figure 9. The first historical description of myocardial bridge made in 1834 by Leopoldo and Floriano Caldani, Professors of Anatomy at the University of Padua. From Caldani et al. (8).
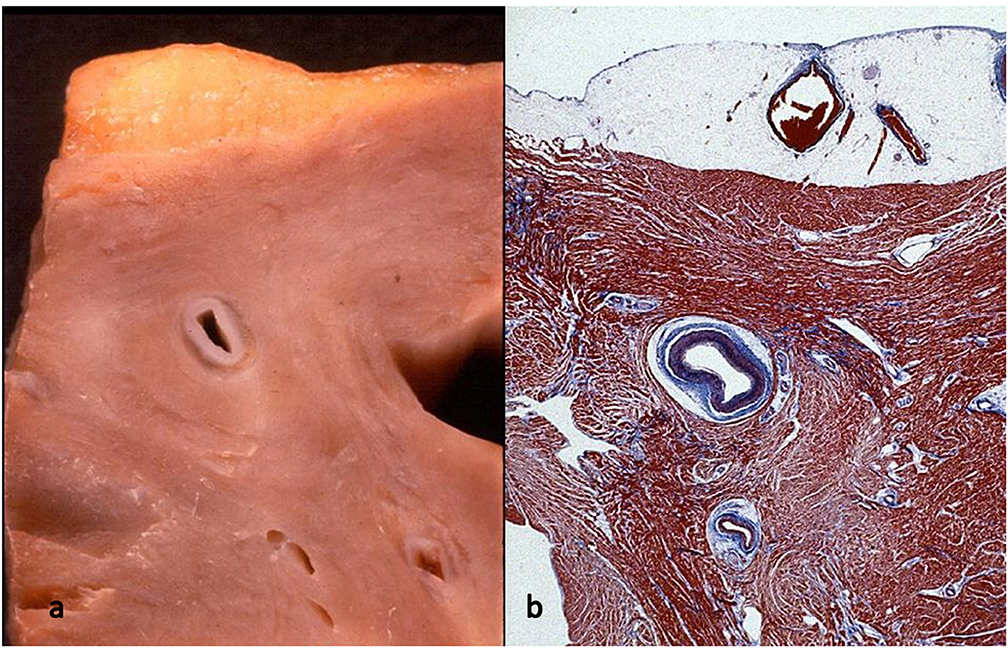
Figure 10. Myocardial bridge with deep intramural course: the coronary segment is completely surrounded by a myocardial sleeve. Gross (a) and histological views (b). Azan Mallory stain.
The origin of the left anterior descending CA from the proximal right CA is also another normal variant, since it does not entail myocardial ischemia (Figure 11). However, the surgeon should be well aware of this unpredictable course while repairing conotruncal congenital heart diseases with a transannular patch or conduit implant.
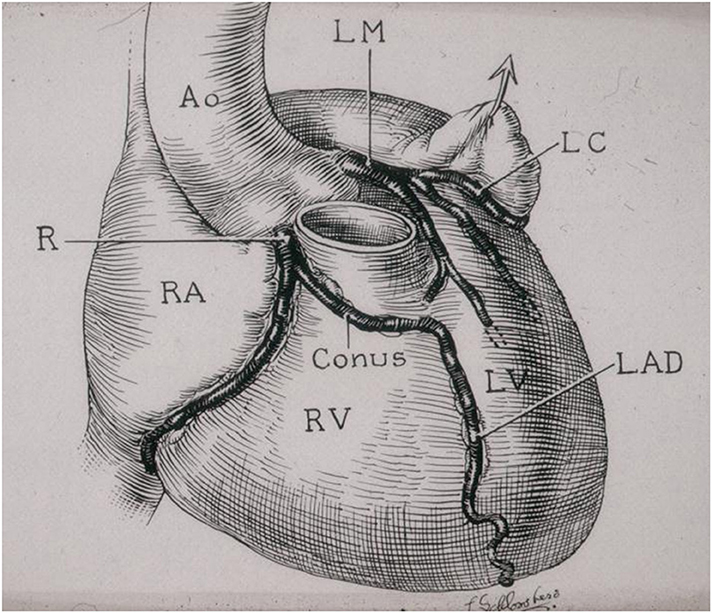
Figure 11. Origin of the left anterior descending coronary artery from the right coronary artery. Note the anomalous proximal course in front of the pulmonary infundibular. From Roberts (6).
The major subepicardial CAs are of medium size and, as such, with a “muscular” tunica media. After birth, a myointimal layer develops with time, due to migration of smooth muscle cells from the tunica media (12).
Embryology of Coronary Stems
Both subepicardial CAs and veins are extracardiac in origin, deriving from epicardial cells (13, 14).
Their development begins with the formation of a plexus-like vasculature located in the subepicardium, which invades the myocardium and develops small vessels and capillaries.
Earlier, the myocardial blood supply derives directly from the ventricular cavities through the intertrabecular spaces lined by the endocardium (Figure 12a). This source of blood to the primitive spongy myocardium disappears with the myocardial compaction (Figure 12b). At this point, the whole intramyocardial vascularization consists of vessels with the endothelium derived from the subepicardium (14–16).
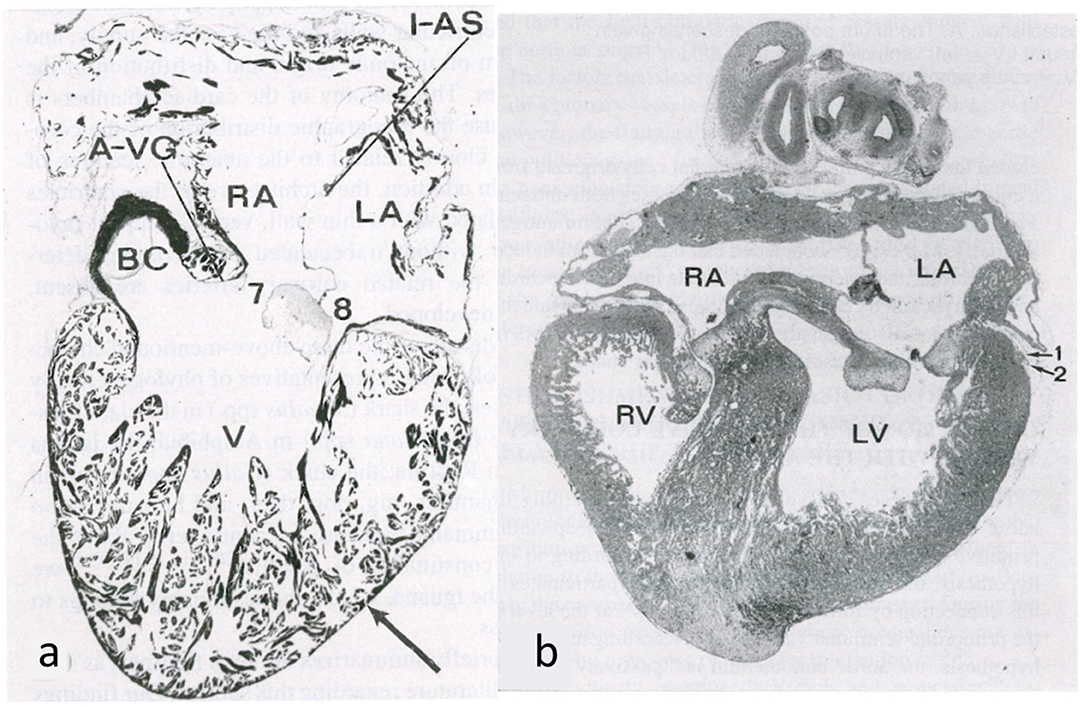
Figure 12. Embryology of the myocardium. (a) Spongy myocardium from a mature fog with blood supply deriving directly from the endocardium of the ventricular cavities. (b) The myocardium of a fetal chick heart becomes compact, with blood supply deriving from the subepicardial vasculature. From de la Cruz et al. (13).
The origin of CAs and veins, whether intra- or extramural, is similar. Their definitive identity and function depend upon the connection, arteries with the aorta, and veins with the sinus venosus.
A subepicardial network of cells (“bioepicardial organ”) surrounds the orifices of the great arteries (peritruncal ring) (Figure 13) and eventually connects with the facing aortic sinuses.
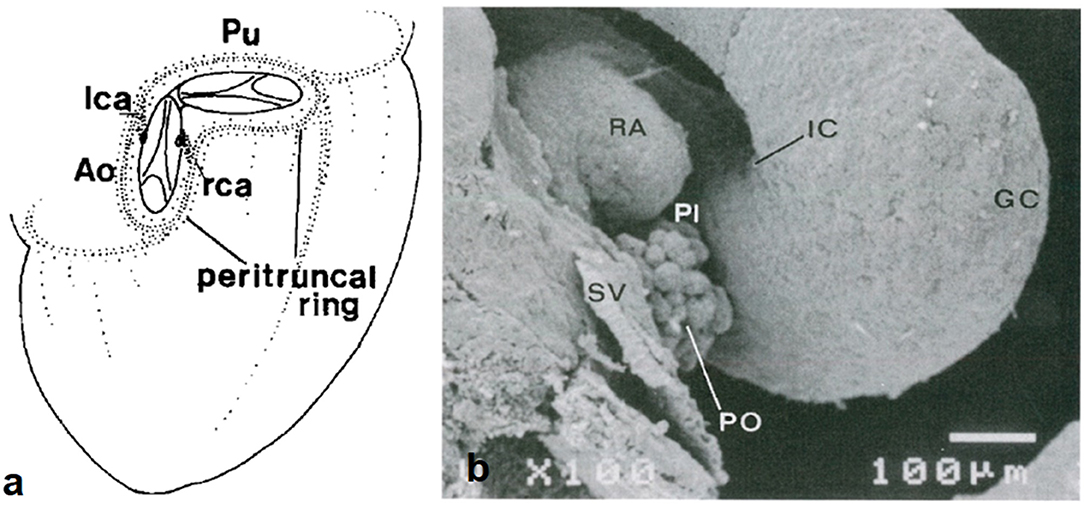
Figure 13. Origin of coronary arterial stems from the peritruncal epicardial ring. (a) From Bogers et al. (17). (b) From de la Cruz et al. (13).
The question is whether the development of CA origin is a matter of ingrowth or outgrowth. There are two hypotheses to explain the connection.
The first is the outgrowth hypothesis, namely, the development of sprouts or buds from the aortic wall of facing sinuses, capturing the peritruncal ring of coronary subepicardial arterial vasculature (13, 18) (Figure 14).
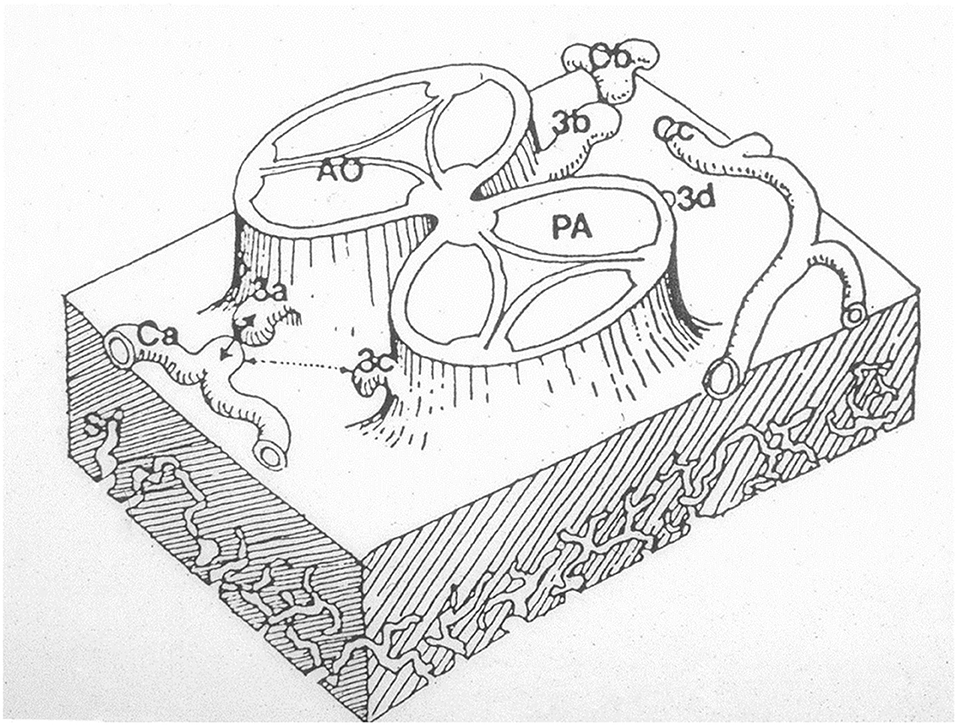
Figure 14. The hypothesis according to which sprouts or buds arise from the facing aortic sinuses and make contact with the subepicardial coronary vasculature. From Angelini (18).
The second developmental hypothesis, proven by serial sectioning of both human and rat embryos, is supported by the observation that the prongs of the peritruncal ring penetrate the aortic wall and make contact with the endothelial lining of the aorta (17, 19).
Recent investigations (20) confirmed that the proximal CAs do not grow from the aorta; on the contrary, they grow into the aorta from the peritruncal ring of the subepicardial vascular plexus (Figure 15).
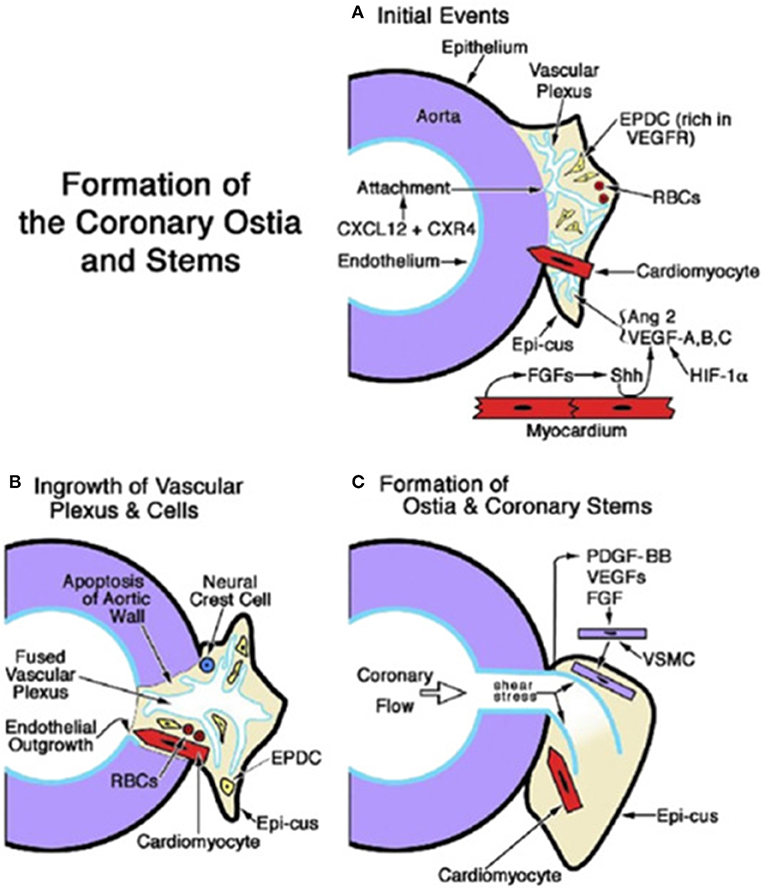
Figure 15. Embryology of coronary artery orifices and main stems. (A) Formation of the coronary ostia and stems is initiated when the capillary ring that encircles the aortic root expands and attaches as a vascular plexus (in response to CXCL12 + CXR4). The sites of ostial formation are adjacent to an epicardial cusp (Epi-cus), a thickened portion of the subepicardium that contains epicardium-derived cells (EPDCs) that are rich in Vascular endothelial growth factor (VEGF) receptors and erythroblasts [red blood cells (RBCs)]. Cardiomyocytes guide the attachment of the vascular plexus. Fibroblast growth factors (FGFs) from the myocardium promote Sonic hedgehog (Shh) signaling, which, together with hypoxia inducible factor-1 (Hif-1), stimulates VEGFs and angiopoietin (Ang) 2, thus facilitating angiogenesis of the vascular plexus. (B) Myocardium-derived cardiomyocytes and neural crest cells facilitate the entry of the vascular plexus into an opening in the aortic wall, created by apoptosis. An endothelial ingrowth demarcates the pathway of the ostium formation. (C) The onset of coronary flow and shear stress is key to the remodeling of the vascular plexus. Differentiation, migration, and attachment of vascular smooth muscle cells (VSMCs) are influenced by (1) platelet-derived growth factor (PDGF)-BB activation in endothelial cells and the ligand's interaction with PDGFR-β in VSMC progenitors; and (2) the influence of VEGFs and fibroblast growth factors (FGFs). From Tomanek and Angelini (20).
A consensus document from the Development, Anatomy, and Pathology Working Group of the European Society of Cardiology (21) was in favor of the ingrowth hypothesis with a statement that deserves full quotation “…CAs were originally thought to form by angiogenesis from the aortic root endothelium” (22). “Angiogenesis implies the formations of new vessels from pre-existing ones, mostly by means of controlled endothelial sprouting” (23). “Indeed, until the late 1980s, it was thought that CAs entirely derived from an aortic endothelial outgrowth that would expand to form the complete coronary system, including coronary veins. Further research in avian models (19, 24–26) partially argued against, demonstrating that: (i) prospective CA endothelial cells do not bud from the aortic root, but instead grow into the aortic wall from an aortic peritruncal plexus to connect to the systemic circulation, most likely under the guidance of vascular endothelial growth factor (VEGF-C) and periaortic cardiomyocytes (27) and (ii) at least part of the early arterial coronary vascular system forms through a process of vasculogenesis…and subsequent fusion of endothelial cell clusters to form new blood vessels” (28).
As CA stems connect the aorta to the ventricular coronary tree, Thevenieu-Ruissy et al. did an investigation in both wild-type and Tbx1 null mice embryos (29). They demonstrated that a periarterial plexus bridges limited outgrowth of the aortic endothelium with the ventricular plexus during CA stem development, supporting the hypothesis that outgrowth of aortic endothelium contributes little to proximal CA stems.
Septation of the arterial pole of the heart (42 days in the human embryo) precedes the appearance of coronary ostia when cells from the peritruncal ring migrate into the aortic root. Septation therefore cannot be responsible for the final position of coronary orifices.
Formation of the left CA precedes the right CA. Moreover, different from the tunica media of the ascending aorta, the tunica media of the CAs does not derive from the neural crest.
Cellular cross talks and signaling pathways (notch and hippo signals, transcription factors, angiogenic molecules, and apoptosis) take place (30–34). VEGF plays a crucial role in the development of coronary ostia and main stem formation (20) (Figure 15). Absence of VEGF was shown to inhibit ostia formation. Epicardial inhibition, reducing apoptotic remodeling at the ventricular–arterial junction, alters vascular connection with the aorta and may produce CA anomalies equal to those observed in humans (35).
Why the primitive subepicardial coronary arterial vasculature tends to connect with the facing aortic sinuses, instead with facing pulmonary sinuses, is still unknown. The explanation cannot be the posterior position of the aorta since in transposition of the great arteries (TGA), where the aorta is anterior, the CA regularly connects with the facing sinuses of the aorta. An anomalous origin of a coronary artery from the posterior pulmonary artery in TGA is indeed extremely rare (36, 37).
Author Contributions
GT designed and drafted the work. CF, MP, CB, and SR revised the work. All authors contributed to the article and approved the submitted version.
Funding
This paper was supported by Registry for Cardio-cerebro-vascular Pathology, Veneto Region, Venice, Italy.
Conflict of Interest
The authors declare that the research was conducted in the absence of any commercial or financial relationships that could be construed as a potential conflict of interest.
References
2. Banchi A. Le variazioni delle “arteriae coronariae cordis” e la morfologia di questi vasi. Sperimentale. (1903) 57:367–69.
3. Baroldi G, Scomazzoni G. Coronary Circulation in the Normal and Pathologic Heart. Washington DC: Office of the Surgeon General, Department of the Army (1967) p. 1–37.
5. Muriago M, Sheppard MN, Ho SY, Anderson RH. Location of the coronary arterial orifices in the normal heart. Clin Anat. (1997) 10:297–302. doi: 10.1002/(SICI)1098-2353(1997)10:5<297::AID-CA1>3.0.CO;2-O
6. Roberts WC. Major anomalies of coronary arterial origin seen in adulthood. Am Heart J. (1986) 111:941–63. doi: 10.1016/0002-8703(86)90646-0
7. Corrado D, Pennelli T, Piovesana P, Thiene G. Anomalous origin of the left circumflex coronary artery from the right aortic sinus of Valsalva and sudden death. Cardiovasc Pathol. (1994) 3:269–71. doi: 10.1016/1054-8807(94)90013-2
8. Caldani L, Caldani F. Icones anatomicae ex optimis neotericum operibus summa diligentia depromptae et collected. In: Picotti J, editor. Venetiis ex Calcographia (Venice) (1810).
9. Frescura C, Basso C, Thiene G, Corrado D, Pennelli T, Angelini A, et al. Anomalous origin of coronary arteries and risk of sudden death: a study based on an autopsy population of congenital heart disease. Hum Pathol. (1998) 29:689–95. doi: 10.1016/S0046-8177(98)90277-5
10. Thiene G, Rizzo S, Frescura C, Basso C. Chapter 21: Specific cardiovascular diseases and competitive sports participation: coronary anomalies and myocardial bridging at risk of sudden death. In: Pressler A, Niebauer J, editors. Textbook of Sports and Exercise Cardiology. Cham: Switzerland Springer (2020). p. 403–21. doi: 10.1007/978-3-030-35374-2_21
11. Angelini P, Trivellato M, Donis J, Leachman RD. Myocardial bridge: a review. Prog Cardiovasc Dis. (1983) 26:75–88. doi: 10.1016/0033-0620(83)90019-1
12. Angelini A, Thiene G, Frescura C, Baroldi G. Coronary arterial wall and atherosclerosis in youth (1-20 years): a histologic study in a northern Italian population. Int J Cardiol. (1990) 28:361–70. doi: 10.1016/0167-5273(90)90320-5
13. de la Cruz MV, Moreno-Rodriguez R, Angelini P. Chapter 2: Ontogeny of the coronary vessels. In: Angelini P, Fairchild VD, editors. Coronary Artery Anomalies: A Comprehensive Approach. Philadelphia, PA: Lippincott Williams & Wilkins (1999). p. 11–16.
14. Winter EM, Gittenberger-de Groot AC. Epicardium-derived cells in cardiogenesis and cardiac regeneration. Cell Mol Life Sci. (2007) 64:692–703. doi: 10.1007/s00018-007-6522-3
15. Waldo KL, Willner W, Kirby ML. Origin of the proximal coronary artery stems and a review of ventricular vascularization in the chick embryo. Am J Anat. (1990) 188:109–20. doi: 10.1002/aja.1001880202
16. Poelmann RE, Gittenberger-de Groot AC, Mentink MM, Bökenkamp R, Hogers B. Development of the cardiac coronary vascular endothelium, studied with antiendothelial antibodies, in chicken-quail chimeras. Circ Res. (1993) 73:559–68. doi: 10.1161/01.RES.73.3.559
17. Bogers AJ, Gittenberger-de Groot AC, Dubbeldam JA, Huysmans HA. The inadequacy of existing theories on development of the proximal coronary arteries and their connexions with the arterial trunks. Int J Cardiol. (1988) 20:117–23. doi: 10.1016/0167-5273(88)90321-X
18. Angelini P. Normal and anomalous coronary arteries: definitions and classification. Am Heart J. (1989) 117:418–34. doi: 10.1016/0002-8703(89)90789-8
19. Bogers AJ, Gittenberger-de Groot AC, Poelmann RE, Péault BM, Huysmans HA. Development of the origin of the coronary arteries, a matter of ingrowth or outgrowth? Anat Embryol (Berl). (1989) 180:437–41. doi: 10.1007/BF00305118
20. Tomanek R, Angelini P. Embryology of coronary arteries and anatomy/pathophysiology of coronary anomalies. A comprehensive update. Int J Cardiol. (2019) 281:28–34. doi: 10.1016/j.ijcard.2018.11.135
21. Pérez-Pomares JM, de la Pompa JL, Franco D, Henderson D, Ho SY, Houyel L, et al. Congenital coronary artery anomalies: a bridge from embryology to anatomy and pathophysiology–a position statement of the development, anatomy, and pathology ESC Working Group. Cardiovasc Res. (2016) 109:204–16. doi: 10.1093/cvr/cvv251
23. Folkman J, Haudenschild C. Angiogenesis in vitro. Nature. (1980) 288:551–6. doi: 10.1038/288551a0
24. Mikawa T, Fischman DA. Retroviral analysis of cardiac morphogenesis: discontinuous formation of coronary vessels. Proc Natl Acad Sci USA. (1992) 89:9504–8. doi: 10.1073/pnas.89.20.9504
25. Pérez-Pomares JM, Macías D, García-Garrido L, Muñoz-Chápuli R. The origin of the subepicardial mesenchyme in the avian embryo: an immunohistochemical and quail-chick chimera study. Dev Biol. (1998) 200:57–68. doi: 10.1006/dbio.1998.8949
26. Männer J. Does the subepicardial mesenchyme contribute myocardioblasts to the myocardium of the chick embryo heart? A quail-chick chimera study tracing the fate of the epicardial primordium. Anat Rec. (1999) 255:212–6. doi: 10.1002/(SICI)1097-0185(19990601)255:2<212::AID-AR11>3.0.CO;2-X
27. Chen HI, Poduri A, Numi H, Kivela R, Saharinen P, McKay AS, et al. VEGF-C and aortic cardiomyocytes guide coronary artery stem development. J Clin Invest. (2014) 124:4899–914. doi: 10.1172/JCI77483
28. Risau W, Flamme I. Vasculogenesis. Annu Rev Cell Dev Biol. (1995) 11:73–91. doi: 10.1146/annurev.cb.11.110195.000445
29. Théveniau-Ruissy M, Pérez-Pomares JM, Parisot P, Baldini A, Miquerol L, Kelly RG. Coronary stem development in wild-type and Tbx1 null mouse hearts. Dev Dyn. (2016) 245:445–59. doi: 10.1002/dvdy.24380
30. Eralp I, Lie-Venema H, DeRuiter MC, van den Akker NMS, Bogers AJJC, Mentink MMT, et al. Coronary artery and orifice development is associated with proper timing of epicardial outgrowth and correlated Fas-ligand-associated apoptosis patterns. Circ Res. (2005) 96:526–34. doi: 10.1161/01.RES.0000158965.34647.4e
31. Mu H, Ohashi R, Lin P, Yao Q, Chen C. Cellular and molecular mechanisms of coronary vessel development. Vasc Med. (2005) 10:37–44. doi: 10.1191/1358863x05vm584ra
32. Lie-Venema H, Gittenberger-de Groot AC, van Empel LJ, Boot MJ, Kerkdijk H, de Kant E, et al. Ets-1 and Ets-2 transcription factors are essential for normal coronary and myocardial development in chicken embryos. Circ Res. (2003) 92:749–56. doi: 10.1161/01.RES.0000066662.70010.DB
33. van den Akker NM, Caolo V, Wisse LJ, Peters PP, Poelmann RE, Carmeliet P, et al. Developmental coronary maturation is disturbed by aberrant cardiac vascular endothelial growth factor expression and Notch signalling. Cardiovasc Res. (2008) 78:366–75. doi: 10.1093/cvr/cvm108
34. Lavine KJ, Ornitz DM. Shared circuitry: developmental signaling cascades regulate both embryonic and adult coronary vasculature. Circ Res. (2009) 104:159–69. doi: 10.1161/CIRCRESAHA.108.191239
35. Gittenberger-de Groot AC, Vrancken Peeters MP, Bergwerff M, Mentink MM, Poelmann RE. Epicardial outgrowth inhibition leads to compensatory mesothelial outflow tract collar and abnormal cardiac septation and coronary formation. Circ Res. (2000) 87:969–71. doi: 10.1161/01.RES.87.11.969
36. Chandrasekhar S, Caldwell RL, Brown JW. Anomalous origin of the left coronary artery from the pulmonary artery in D-transposition of great vessels. Am Heart J. (1994) 127:722–3. doi: 10.1016/0002-8703(94)90694-7
Keywords: embriology, anatomy, coronary arteries, history, normal variants
Citation: Thiene G, Frescura C, Padalino M, Basso C and Rizzo S (2021) Coronary Arteries: Normal Anatomy With Historical Notes and Embryology of Main Stems. Front. Cardiovasc. Med. 8:649855. doi: 10.3389/fcvm.2021.649855
Received: 05 January 2021; Accepted: 27 April 2021;
Published: 31 May 2021.
Edited by:
Peter Martin Wenaweser, Heart Clinic Zurich, SwitzerlandReviewed by:
Cristina Aurigemma, Catholic University of the Sacred Heart, ItalyPablo Codner, Rabin Medical Center, Israel
Copyright © 2021 Thiene, Frescura, Padalino, Basso and Rizzo. This is an open-access article distributed under the terms of the Creative Commons Attribution License (CC BY). The use, distribution or reproduction in other forums is permitted, provided the original author(s) and the copyright owner(s) are credited and that the original publication in this journal is cited, in accordance with accepted academic practice. No use, distribution or reproduction is permitted which does not comply with these terms.
*Correspondence: Gaetano Thiene, Z2FldGFuby50aGllbmUmI3gwMDA0MDt1bmlwZC5pdA==