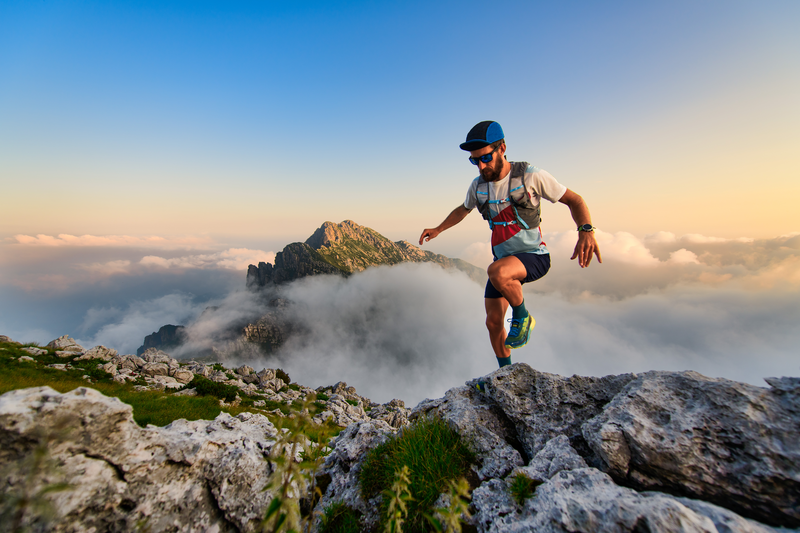
95% of researchers rate our articles as excellent or good
Learn more about the work of our research integrity team to safeguard the quality of each article we publish.
Find out more
REVIEW article
Front. Cardiovasc. Med. , 08 April 2021
Sec. Atherosclerosis and Vascular Medicine
Volume 8 - 2021 | https://doi.org/10.3389/fcvm.2021.647071
The occurrence and development of atherosclerosis could be influenced by intermittent hypoxia. Obstructive sleep apnea (OSA), characterized by intermittent hypoxia, is world-wide prevalence with increasing morbidity and mortality rates. Researches remain focused on the study of its mechanism and improvement of diagnosis and treatment. However, the underlying mechanism is complex, and the best practice for OSA diagnosis and treatment considering atherosclerosis and related cardiovascular diseases is still debatable. In this review, we provided an update on research in OSA in the last 5 years with regard to atherosclerosis. The processes of inflammation, oxidative stress, autonomic nervous system activation, vascular dysfunction, platelet activation, metabolite dysfunction, small molecule RNA regulation, and the cardioprotective occurrence was discussed. Additionally, improved diagnosis such as, the utilized of portable device, and treatment especially with inconsistent results in continuous positive airway pressure and mandibular advancement devices were illustrated in detail. Therefore, further fundamental and clinical research should be carried out for a better understanding the deep interaction between OSA and atherosclerosis, as well as the suggestion of newer diagnostic and treatment options.
Obstructive sleep apnea (OSA) is known to commonly occur worldwide, with a recent dramatic increase in prevalence. Globally, an estimated 936 million and 425 million adults aged between 30 and 69 years experience mild-to-severe and moderate-to-severe OSA respectively (1). OSA is characterized by recurrent pauses in breathing during the sleep and results in upper airway collapse and intermittent hypoxemia (IH) (2). OSA is a systemic disorder and recognized as an independent factor for cardiovascular disease (3).
Atherosclerosis (AS) is a chronic disease of medium- and large-sized arteries leading to ischemic heart disease and cardiovascular disease (4). Over the past few years, many studies have shown a link between OSA and atherosclerosis, and sex-based differences were surprisingly observed. Female patients with OSA but not males were significantly associated with incident heart failure or death (5). Further, in a larger scale, female patients were more likely to have cardiovascular diseases compared with males (6). OSA causes endothelial dysfunction (7), unstable plaque characteristics (8) and further atherosclerosis (9). Similarly, IH also causes endothelial dysfunction (10) and accelerated atherosclerosis (11, 12). However, some reports argue that OSA may play a protective role in ischemic insult (13) and coronary occlusion (14) recently. Thus, the relationship between OSA and AS and related diseases needs to be further explored.
This review includes the clinical and fundamental research undertaken in the last 5 years describing the mechanism of OSA and its role in AS, as well as the latest research advances in the diagnosis and treatment of OSA and its influence on AS-related diseases.
The process of AS development in patients with OSA is complex. We discuss the underlying mechanism focusing on inflammation, oxidative stress, autonomic nervous system, vascular dysfunction, platelet activation, metabolite dysfunction, small molecule RNA, and the cardioprotective function (Figure 1).
Figure 1. The mechanism and latest discoveries of the link between obstructive sleep apnea and atherosclerosis. NO, nitric oxide; HIF-1, hypoxia inducible factor-1; IH, intermittent hypoxemia.
Since AS is a chronic inflammatory process, IH can produce a variety of inflammatory cytokines; therefore IH-mediated AS may be primarily due to the activation of inflammatory pathways (15). NF-κB is a key inflammation pathway that has been extensively studied.
Some reports illustrated the development of AS when NF-κB pathway was blocked. Mice with apolipoprotein E deficiency and knocked down NF-κB p50 showed higher serum levels of TNF-α, IL-6, and cholesterol and more pronounced atherosclerotic lesions than mice with apolipoprotein E deficiency alone (16). When IKKβ, a regulator of NF-κB, was deleted in mice, IH-mediated pulmonary artery atherosclerosis was abolished (17). Another study utilized the overexpression of IkBα, an inhibitor of NF-κB, only in mice endothelial cells and showed a decline in developing atherosclerotic lesions. IkBα overexpression resulted in the suppression of E-selectin and vascular cell adhesion molecule-1 (VCAM-1) and inhibited the NF-κB pathway. Hence, AS was weakened under IH exposure (18). Collectively, these studies supported IH-induced AS via the NF-κB pathway.
Upstream of NF-κB has also been discussed in the IH-mediated AS. Besides common molecules such as IL6, TNF-α, and p38 MAPK, TLR4/ NF-κB was discovered as a new therapeutic target. Increased TLR4 expression was observed both in IH-treated mice (19) and OSA patients (20). When TLR4 was inhibited in mice, enhanced NF-κB pathway, and augmented atherosclerotic plaque loads were diminished (19). When TLR4 was depleted in mice, smaller intima-media aorta thickness was observed than in normal mice (21). Thus, TLR4/NF-κB plays an important role in inflammation, indicating alternative therapeutic option.
Oxidative stress is another fundamental mechanism contributing to the cardiovascular diseases in OSA. It has been shown that IH triggers the activation of NADPH oxidase and increases leukocytes oxidation, resulting in lipid peroxidation and isoprostane formation. Meanwhile, the function of nitric oxide in endothelial cells is inhibited because of reduced endothelial nitric oxide (NO) synthase and regulation of asymmetric dimethylarginine (22). All these processes are involved in the formation of AS and related cardiovascular diseases. Further, other proteins were found to participate in AS formation in IH, such as, non-muscle myosin light chain kinase which was discovered in the mouse model (23). Interestingly, recent studies have also shown that IH and oxidative stress can activate the protective mechanism of endothelial cell-colony forming units in a cell model extracted from healthy volunteers, which is closely linked with vascular function and maintains vascular health (24).
Hypoxia inducible factor-1 (HIF-1) is a transcription factor that promotes genes involved in adaptation to insufficient oxygen and hypoxia environment. ROS induced by desaturation-reoxygenation under IH could up-regulate HIF-1 activity through complex processes (25). In brief, ROS activates phospholipase C, followed by calcium-calmodulin kinase and protein kinase C activation. Protein kinase C stimulates the mTOR-dependent production of HIF-1α and inhibited PHD-dependent degradation of HIF-1α (26). HIF-1 promotes numerous adaptive genes such as, endothelin-1, which could destroy the cardiovascular system (25). It is reported that the profiling of gene expression including HIF-1 in skin biopsies from OSA patients could possibly be utilized to predict cardiovascular risk (27).
The autonomic nervous system including the sympathetic and vagal system is altered in patients with OSA. In brief, IH stimulates the peripheral chemoreceptor and activates sympathetic nervous system, followed by an increase in renin, angiotensin II, and aldosterone, and the enhancement of vasoconstrictor activity. At the same time, impaired baroreflex and reduction of NO resulted in the increase of endothelin and receptor as well as intracellular calcium sensitivity (28). In these ways, IH induces abnormal vasoconstriction and elevates systemic blood pressure.
Disruption of vascular endothelium homeostasis in OSA is triggered by inflammation and oxidative stress of endothelial cells (29) and is modestly linked with subclinical atherosclerotic coronary artery disease (CAD) (30). Also, individual patient data meta-analysis showed that severe OSA is independently linked with an increased endothelial dysfunction (31). Non-muscular myosin light chain kinase was discovered to have been involved in IH-induced endothelial dysfunction through the secretion of IL-6, NO production, and acetylcholine in human aortic endothelial cells (32). Besides, the endothelial barrier function was also destroyed by phosphorylated ERK and JNK in human lung microvascular endothelial cells (33). Some protective measures have recently been investigated to improve endothelial dysfunction. Infliximab and glutathione, served as anti-inflammatory and antioxidant treatment, were shown to inhibit the vascular injury process from mouse models (34). Rhodiola Crenulata extract might protect the damage of human umbilical vein endothelial cells through the AMPK and ERK pathway (35).
IH triggers excessive proliferation of vascular smooth muscle cells, which play important roles in AS progression (36). It is reported that the production of IL-6 induced the upregulation of epiregulin, which contributed to the proliferation of smooth muscle cells (37).
Patients with OSA suffer persistent platelet activation, as a consequence of increased sympathetic activity, inflammation, and endothelial dysfunction, which leads to AS lesions (38). Platelet activation leads to alteration in their shape and the phospholipid bilayer, resulting in the stimulation of coagulation factors and upregulation of surface receptors and adhesion molecules, enabling them to interact with other cells. The platelet-lymphocyte ratio is observed to be associated with OSA severity regardless of OSA progression, indicating that it may serve as an independent marker (39). In addition, platelets were reported to serve as immune cells participating in the pathophysiology of autoimmune disorders (40). Persistent platelet activation induces constant production of pro-inflammatory and proatherogenic substances, infiltration of immune cells in the endothelium and further the progression of AS plaques.
Patients with OSA have abnormal glucose and lipid metabolism, contributing to the generation of AS (41, 42). The functional proteins involved in glycolipid metabolism in IH-mediated AS has garnered considerable research interest. A recent study described SREBP-1 signaling in the aorta, skeletal muscle, and liver, considering the synergistic effect of both IH and abnormal glucose metabolism. It was found that SREBP-1c and FAS increased, while IRS-1 and its phosphorylation decreased, thereby promoting AS in vitro and in vivo (43). In addition, augmented angiopoietin-like 4 in OSA via HIF-1 also played an important role in abnormal IH-induced lipid metabolism contributed to AS formation (11, 44).
Small molecules RNAs such as miRNA, mRNA, and lncRNA provide detailed information about AS development in patients with OSA miRNAs from plasma exosomes were identified via arrays, and differentially expressed miRNAs would describe the altered endothelial function accounting for the mechanism of cardiovascular morbidities in OSA (45). Another report depicted the profiling of mRNAs and lncRNAs in the aorta when treated with IH. The description of this system may provide potential candidates for future research on IH-induced AS (46). In addition, its unique RNA was also explored to understand its function. It was reported that miR-193a-3p impaired human umbilical vein endothelial cells under IH exposure via Fas apoptotic inhibitory molecule 2 (47). Similarly, miR-146a-5p aggravated IH-induced heart myoblast injury through an X-linked inhibitor of apoptosis protein (48). However, both studies were only conducted in vitro only. Taken together, RNA showed its potentiality in inspecting the mechanism and treatment therapy of cardiovascular diseases in OSA.
More recently, increasing evidence has shown that intermittent hypoxia can trigger an adaptation in cardiovascular system (49), such as, the resistance to an ischemic insult (50) and attenuation of ischemic brain injury (51). In acute coronary syndrome, myocardial infarct size significantly decreased in patients with OSA compared to in patients without, as determined by measuring peak, and area of serial cardiac troponin I levels (52). These results were consistent with the study that patients with OSA and acute myocardial infarction showed lower peak troponin-T levels (53). However, these results should interpreted with cautions because of some limitations (13). First, the measurement of infarct size can hardly be accurate and would be affected by multiple factors. Second, the number of cases recruited was small, and the results need to be verified in a larger study. Third, the patient group had a large dynamic range of troponin levels with approximately a 100-fold difference. Thus, although the clinical protective effect of IH cannot yet be clearly established, these results indicated that OSA might upregulate the cardioprotective pathway and promote adaptive process.
The apnea-hypopnea index (AHI) is widely used in the identification and classification of clinical OSA. The gold standard of AHI detection is measured by overnight polysomnography in the sleep laboratory, and values of 5–15, 15–30, and >30 are defined as mild, moderate, and severe OSA, respectively (54). Further, polysomnography could give mechanistic indices such as loop gain, arousal threshold, and pharyngeal collapsibility with validated methods, and it was able to distinguish sex differences in patients with OSA (55). However, cost and accessibility limit the application of polysomnography in all settings. Recently, other methods such as, home sleep apnea testing and questionnaires have been further investigated to diagnose patients with OSA and those with AS. Moreover, new algorithms have also emerged to ensure a much more convenient diagnosis (Table 1).
Home sleep apnea testing (HSAT), also known as out-of-center sleep testing, portable sleeping monitoring, and portable monitoring, is an alternative for the diagnosis of moderate-to-severe OSA (56). However, according to the statement of the American Academy of Sleep Medicine, the decision should not be based solely on the automatically scored HSAT data (57). More recently, HSAT has been used to assess the relationship between sleep apnea and cardiovascular diseases. The results showed that a portable ApneaLink device could be used to identify patients with heart failure with sleep apnea (AHI≥15 and <5 events/h), which contained both obstructive and central apneas (58). In another study, researchers distinguished the central and obstructive AHI values in another study, and also found a strong correlation coefficient between HSAT and polysomnography, which indicated a possible diagnosis (59). However, HSAT does not appear to have reduced the cost, as it is only 10% lower than the cost of polysomnography for the provider (60).
To screen patients with OSA, researchers developed questionnaires such as the Berlin questionnaire (BQ), Epworth Sleepiness Scale (ESS), STOP questionnaire (STOP), and STOP-Bang questionnaire (SBQ). It was found that SBQ showed the most sensitivity and diagnostic odds ratio among these four questionnaires and was used to estimate OSA severity (61, 62). However, it remained obscure whether a sleep disorder with cardiovascular diseases could be diagnosed by questionnaires, as typical sleep-disordered symptoms may not be observed in these patients. According to the results of 89 patients with cardiovascular diseases, ESS showed no association with sleep disorder while BQ showed the sensitivity of 73% with a specificity of 42%. SBQ showed the most sensitivity (97%) with low specificity (13%) (63). Besides, insufficient specificity was observed in OSA with atrial fibrillation (64), and poor correlation was discussed in OSA with stroke (65). Taken together, to better reflect the phenotypes in patients with co-morbid conditions, the questionnaires need to be improved.
A new algorithm was developed for identifying OSA. Electrocardiograph-based algorithm, such as, support vector machines (66), Kernel density classifier (67), and convolutional neural network (68), provide an alternative approach to screen suspected patients with OSA. It was observed that the convolutional neural network strategy exhibited the highest accuracy, sensitivity, and specificity among all existing algorithms. An alternative algorithm comprising optimized cardiovascular signals showed good representation of AHI and could be utilized to screen for OSA severity (69). Another study used six objective parameters including age, sex, body mass index, blood pressure, neck circumference, and the ESS and demonstrated the possibility for OSA screening and risk prediction (70).
OSA patients with cardiovascular conditions such as the heart failure and hypertension has been discussed before (71, 72), but their link to peripheral arterial disease (PAD) has been underestimated. The mechanism such as inflammation, oxidative stress, and endothelial dysfunction also contributed to the development of PAD in patients with OSA, and clinical evidences have been paid attention recently (73). Underdiagnosis of OSA in PAD were observed. A sleep apnea prevalence accounted for 78.0% in patients with lower extremity artery disease, and AHI increased with the severity of lower extremity artery disease (74). On the other hand, PAD prevalence of 98% in patients with confirmed OSA was observed (75). The association of OSA and PAD was further verified by a larger extent (76).
Different approaches to treat OSA have been illustrated, and their influence on the AS and related diseases have gained considerable attention. The update treatment is discussed in detail in Table 2.
Continuous positive airway pressure (CPAP) is widely used in the treatment of OSA to reduce excessive daytime sleepiness (77) and improve sleep quality (78). However, the cardiovascular effects of CPAP remain controversial. Some reports have supported that CPAP treatment attenuates AS (79). A further well-designed randomized controlled trial showed the modest ability of CPAP treatment to decrease blood pressure (80), indicating the potential to reduce cardiovascular morbidity and mortality. Patients with CAD also showed the attenuation with CPAP treatment. The risk of coronary heart disease in patients with OSA treated with CPAP was similar to those without OSA (81), and the risk of repeat revascularization under percutaneous coronary intervention was lowered when utilizing CPAP treatment (82). On the other hand, some evidence showed that CPAP therapy in preventing cardiovascular events lacked clinical benefit. A RCT showed that CPAP treatment did not significantly prevent hypertension or cardiovascular events (83). According to a large trial of sleep apnea cardiovascular endpoints in patients with OSA, additional CPAP treatment under usual care did not demonstrate the prevention of cardiovascular events (84). Most importantly, the average duration of CPAP should be paid attention to. In patients with CAD, CPAP had no significant effects on cardiovascular outcomes, but significant improvement was observed in patients with CPAP treatment for over 4 h (85). Therefore, improving CPAP compliance seems essential for treatment.
Many more studies have looked into CPAP adherence. Educational videos were utilized in patients with poor CPAP adherence and did not show superiority compared with usual care (86). In addition, remote telemonitoring for CPAP seems to be an effective alternative to improve adherence (87, 88). Text messages showed great improvement in medication compliance in chronic disease (89), and CPAP telemonitoring with automated feedback messages improved adherence in patients with OSA (88). The utilization of mobile applications associated with telemonitoring increase CPAP adherence with an average of 1 h (90). Notably, cardiovascular consequences in patients with OSA has been discussed under the CPAP treatment of remote patient telemonitoring. Given the large sample size of 306 patients, home self-measured blood pressure in patients with high cardiovascular risk did not show a significant difference between telemonitoring and usual care in CPAP treatment after 6 months (91). It was argued that telemonitoring focuses on CPAP without encouraging physical activity and thus, may not contribute to the reduction of blood pressure (91). However, the increase in CPAP adherence and the improvement of life quality was observed in favor of telemonitoring (91). Taken together, CPAP telemonitoring might allow better scrutiny of individual patient risks by connecting with devices measuring physical activity and blood pressure and further provide personalized care to patients with OSA and high cardiovascular risk.
Oral appliances have become an alternative way to treat OSA, especially mandibular advancement devices (MAD) (92). Some evidence has shown that MAD had a beneficial outcome in cardiovascular consequences. Compared with inactive control from the meta-analysis, MAD was associated with significant lower blood pressure in both systolic and diastolic blood pressure with a reduction of 2.1 and 1.9 mm Hg, respectively (80). Interestingly, it was reported that sex might affect the treatment with MAD. For women with MAD, the nighttime systolic and diastolic blood pressure was 10.8 and 6.6 mm Hg, respectively, lower than those in the sham group, while no significant differences were observed in men (93). However, the results comprised of 27 women and 58 men and might not present an unbiased conclusion. On the other hand, some reported that MAD had no effect on blood pressure and endothelial function although MAD improved the AHI, micro-arousal index and symptoms of fatigue, sleepiness and snoring (94). It was worth noting that patients included in this study had severe OSA without overt cardiovascular disease, which could not provide an objective result. Another study also failed to show significant beneficial effect on the endothelial function and sleep-time blood pressure under MAD treatment in patients with 20–40/h of AHI (95). That is, the cardiovascular outcome of MAD treatment remained unclear and needs further investigation.
In contrast to CPAP, the clinical effectiveness of MAD treatment was not good enough in terms of AHI reduction, but the cost effectiveness was better considering quality-adjusted life-years based on the questionnaire in moderate OSA (96). As for cardiovascular outcome, systolic and diastolic blood pressure showed no significant differences in both treatments according to the meta-analysis (80). Additionally, endothelial function, and sleep-time blood pressure showed similar performances (95). In conclusion, MAD may be a good alternative approach for patients refusing CPAP therapy or for those who prefer MAD due to the less-invasive nature of the device.
Reduced exercise capacity was observed in patients with OSA, and comorbidities such as, daytime hypoxemia and severe mean nocturnal desaturation made the exercise capacity lower (97). Further, exercise training contributed to either decrease of AHI (98) or reduction of body weight (99) in patients with OSA. Recent studies emphasized the importance of exercise treatment, and evidence showed exercise would bring a great improvement in patients with OSA and cardiovascular diseases. In all, 35% (100) and 33% (101) reduction of AHI was observed in OSA patients with heart failure and CAD, respectively, after exercise treatment. In addition, peak oxygen consumption, muscle strength, and endurance greatly improved with exercise treatment instead of CPAP (100) in patients with OSA and heart failure, which have important clinical implications. The performance of increasing peak oxygen consumption was also observed in patients with OSA and CAD (102). Therefore, exercise is an important therapy for patients with OSA, especially with cardiovascular comorbidity.
Hypoglossal nerve stimulation devices are used to dilate/reinforce the airway by neuromodulation. A case report showed that in a patient with unsuccessful surgery, HNS treatment drastically improved their condition and outcome (103). Then, long-time HNS treatment with 48 months were investigated, and stable improvement was observed among 91 patients with moderate-to-severe OSA (104). Alternation of the nocturnal sleep architecture and improvement of the objective level of alertness were also reported after HNS therapy (105). However, the side effect of pain, tongue abrasion, and device malfunction should be noted for further use (106).
Some medications have recently been investigated for OSA. As oxidative stress is an aspect of OSA, antioxidants was designed for treatment (107–109). Losartan, an antioxidant, and anti-inflammatory drug (108), showed a significant effect in the treatment of cardiovascular complications in OSA (109). Another therapeutic candidate is melatonin, a regulating hormone with antioxidant properties, which showed its cardioprotective effects on myocardial injuries (110). Taken together, antioxidants might be considered as a prospective drug in patients with OSA and cardiovascular diseases.
Some drug therapies have focused on the phenotype in OSA, such as, high loop gain, pharyngeal hypotonia, and low arousal threshold (111). Oxygen could be utilized for downregulation of the loop gain. Thirty-six patients with an average AHI of 57.9 events/h accomplished two nights of polysomnography in favor of supplemental oxygen (40%) and sham (air). Nine of the patients exhibited a reduction of 70% in AHI and 7 mm Hg overnight change in blood pressure (112). The decrease of pharyngeal dilator muscle activity during sleep is one of the key factors resulting in upper airway collapse. It was reported that desipramine, a noradrenergic agonist, improved pharyngeal collapsibility, and might be a new pharmacologic therapy for patients with OSA (113, 114). In addition, it was observed that the respiratory arousal threshold could be increased by drugs such as, trazodone, but it was insufficient to improve the compromised upper airway anatomy (115). However, drug-induced prolonged arousal trait might contribute to the undesirable high loop gain trait, which could be hazardous in patients with cardiovascular diseases. The precision-medicine approach targeting the phenotypic traits of OSA showed a fair degree of uncertainty and thus, more research should be undertaken to comprehensively investigate its safety and efficacy.
Many studies have provided descriptions of patients with OSA and AS-associated cardiovascular diseases. Intermittent hypoxia, an important characteristic of OSA, facilitates the occurrence, and development of AS. The mechanism discussed in this review have not develop into diagnosis and therapy. Although, some proteins and molecules showed its distinct characteristics in the progress, they almost remained in a cell or mouse model without the support of human experiments. In addition, the relationship between IH and AS is extremely complex and the results from in vitro and in vivo studies are sometimes inconsistent. Thus, signaling processes need further exploration, and the comprehensive theory is also required for better diagnosis and treatment.
Updated diagnosis and treatment methods are summarized in Tables 1, 2, except for the gold standard polysomnography and CPAP. Since the relationship of PAD and OSA was underestimated in the diagnosis and treatment, much more attention should be paid in the further research. The development of portable device for diagnosis gave the possibility to cover more and more patients with OSA especially for those without hospitalization, and it is much more convenient and cost-efficient. Additionally, treatments are well-discussed, and their effect on AS and related cardiovascular diseases are not clear. For example, the clinical effect of CPAP and MAD on cardiovascular diseases is controversial. Therefore, large-scale and long-term clinical studies with a robust scientific design are crucial for the comparison of different methods that contribute to diagnosis and treatment of acute apnea syndrome with AS and related diseases.
JC drafted the manuscript. YZ and SL supervised and revised it. All authors contributed to the article and approved the submitted version.
We are grateful to the National Key R&D Program of China (2016YFC1304003), National Natural Science Foundation of China (81900034 and 81670402), and the Second Affiliated Hospital of Fujian Medical University (BS201807) for financial aid.
The authors declare that the research was conducted in the absence of any commercial or financial relationships that could be construed as a potential conflict of interest.
1. Benjafield AV, Ayas NT, Eastwood PR, Heinzer R, Ip MSM, Morrell MJ, et al. Estimation of the global prevalence and burden of obstructive sleep apnoea: a literature-based analysis. Lancet Respir Med. (2019) 7:687–98. doi: 10.1016/S2213-2600(19)30198-5
2. Malhotra A, White DP. Obstructive sleep apnoea. Lancet. (2002) 360:237–45. doi: 10.1016/S0140-6736(02)09464-3
3. Bradley TD, Floras JS. Obstructive sleep apnoea and its cardiovascular consequences. Lancet. (2009) 373:82–93. doi: 10.1016/S0140-6736(08)61622-0
4. Kobiyama K, Ley K. Atherosclerosis. Circ Res. (2018) 123:1118–20. doi: 10.1161/CIRCRESAHA.118.313816
5. Roca GQ, Redline S, Claggett B, Bello N, Ballantyne CM, Solomon SD, et al. Sex-specific association of sleep apnea severity with subclinical myocardial injury, ventricular hypertrophy, and heart failure risk in a community-dwelling cohort. Circulation. (2015) 132:1329–37. doi: 10.1161/CIRCULATIONAHA.115.016985
6. Bouloukaki I, Mermigkis C, Markakis M, Pataka A, Alexaki I, Ermidou C, et al. Cardiovascular effect and symptom profile of obstructive sleep apnea: does sex matter? J Clin Sleep Med. (2019) 15:1737–45. doi: 10.5664/jcsm.8074
7. Hoyos CM, Melehan KL, Liu PY, Grunstein RR, Phillips CL. Does obstructive sleep apnea cause endothelial dysfunction? A critical review of the literature. Sleep Med Rev. (2015) 20:15–26. doi: 10.1016/j.smrv.2014.06.003
8. Konishi T, Kashiwagi Y, Funayama N, Yamamoto T, Murakami H, Hotta D, et al. Obstructive sleep apnea is associated with increased coronary plaque instability: an optical frequency domain imaging study. Heart Vessels. (2019) 34:1266–79. doi: 10.1007/s00380-019-01363-8
9. Trzepizur W, Martinez MC, Priou P, Andriantsitohaina R, Gagnadoux F. Microparticles and vascular dysfunction in obstructive sleep apnoea. Eur Respir J. (2014) 44:207–16. doi: 10.1183/09031936.00197413
10. Krause BJ, Del Rio R, Moya EA, Marquez-Gutierrez M, Casanello P, Iturriaga R. Arginase-endothelial nitric oxide synthase imbalance contributes to endothelial dysfunction during chronic intermittent hypoxia. J Hypertens. (2015) 33:515–24; discussion 24. doi: 10.1097/HJH.0000000000000453
11. Drager LF, Yao Q, Hernandez KL, Shin MK, Bevans-Fonti S, Gay J, et al. Chronic intermittent hypoxia induces atherosclerosis via activation of adipose angiopoietin-like 4. Am J Respir Crit Care Med. (2013) 188:240–8. doi: 10.1164/rccm.201209-1688OC
12. Lin H, Zeng Y, Wang Z. Maternal chronic intermittent hypoxia in rats causes early atherosclerosis with increased expression of Caveolin-1 in offspring. Sleep Breath. (2019) 23:1071–7. doi: 10.1007/s11325-019-01781-y
13. Aronson D, Lavie L, Lavie P. Does OSA upregulate cardioprotective pathways to an ischemic insult? Chest. (2018) 153:295–7. doi: 10.1016/j.chest.2017.07.036
14. Steiner S, Schueller PO, Schulze V, Strauer BE. Occurrence of coronary collateral vessels in patients with sleep apnea and total coronary occlusion. Chest. (2010) 137:516–20. doi: 10.1378/chest.09-1136
15. Ma L, Zhang J, Liu Y. Roles and mechanisms of obstructive sleep apnea-hypopnea syndrome and chronic intermittent hypoxia in atherosclerosis: evidence and prospective. Oxid Med Cell Longev. (2016) 2016:8215082. doi: 10.1155/2016/8215082
16. Fang G, Song D, Ye X, Mao SZ, Liu G, Liu SF. Chronic intermittent hypoxia exposure induces atherosclerosis in ApoE knockout mice: role of NF-kappaB p50. Am J Pathol. (2012) 181:1530–9. doi: 10.1016/j.ajpath.2012.07.024
17. Imamura T, Xue T, Poulsen O, Zhou D, Karin M, Haddad GG. Intermittent hypoxia and hypercapnia induces inhibitor of nuclear factor-kappa B kinase subunit beta-dependent atherosclerosis in pulmonary arteries. Am J Physiol Regul Integr Comp Physiol. (2019) 317:R763–9. doi: 10.1152/ajpregu.00056.2019
18. Song D, Fang G, Mao SZ, Ye X, Liu G, Miller EJ, et al. Selective inhibition of endothelial NF-kappaB signaling attenuates chronic intermittent hypoxia-induced atherosclerosis in mice. Atherosclerosis. (2018) 270:68–75. doi: 10.1016/j.atherosclerosis.2018.01.027
19. Zeng X, Guo R, Dong M, Zheng J, Lin H, Lu H. Contribution of TLR4 signaling in intermittent hypoxia-mediated atherosclerosis progression. J Transl Med. (2018) 16:106. doi: 10.1186/s12967-018-1479-6
20. Olejarz W, Gluszko A, Cyran A, Bednarek-Rajewska K, Proczka R, Smith DF, et al. TLRs and RAGE are elevated in carotid plaques from patients with moderate-to-severe obstructive sleep apnea syndrome. Sleep Breath. (2020) 24:1573–80. doi: 10.1007/s11325-020-02029-w
21. Poulain L, Richard V, Levy P, Dematteis M, Arnaud C. Toll-like receptor-4 mediated inflammation is involved in the cardiometabolic alterations induced by intermittent hypoxia. Mediators Inflamm. (2015) 2015:620258. doi: 10.1155/2015/620258
22. Eisele HJ, Markart P, Schulz R. Obstructive sleep apnea, oxidative stress, and cardiovascular disease: evidence from human studies. Oxid Med Cell Longev. (2015) 2015:608438. doi: 10.1155/2015/608438
23. Arnaud C, Bouyon S, Recoquillon S, Brasseur S, Lemarié E, Briançon-Marjollet A, et al. Non-muscle myosin light chain kinase: a key player in intermittent hypoxia-induced vascular alterations. J Am Heart Assoc. (2018) 7:e007893. doi: 10.1161/JAHA.117.007893
24. Avezov K, Aizenbud D, Lavie L. Intermittent hypoxia induced formation of “Endothelial Cell-Colony Forming Units (EC-CFUs)” is affected by ros and oxidative stress. Front Neurol. (2018) 9:447. doi: 10.3389/fneur.2018.00447
25. Belaidi E, Morand J, Gras E, Pepin JL, Godin-Ribuot D. Targeting the ROS-HIF-1-endothelin axis as a therapeutic approach for the treatment of obstructive sleep apnea-related cardiovascular complications. Pharmacol Ther. (2016) 168:1–11. doi: 10.1016/j.pharmthera.2016.07.010
26. Prabhakar NR, Semenza GL. Adaptive and maladaptive cardiorespiratory responses to continuous and intermittent hypoxia mediated by hypoxia-inducible factors 1 and 2. Physiol Rev. (2012) 92:967–1003. doi: 10.1152/physrev.00030.2011
27. Kaczmarek E, Bakker JP, Clarke DN, Csizmadia E, Kocher O, Veves A, et al. Molecular biomarkers of vascular dysfunction in obstructive sleep apnea. PLoS ONE. (2013) 8:e70559. doi: 10.1371/journal.pone.0070559
28. Levy P, Kohler M, McNicholas WT, Barbe F, McEvoy RD, Somers VK, et al. Obstructive sleep apnoea syndrome. Nat Rev Dis Primers. (2015) 1:15015. doi: 10.1038/nrdp.2015.24
29. Jelic S, Le Jemtel TH. Inflammation, oxidative stress, and the vascular endothelium in obstructive sleep apnea. Trends Cardiovas Med. (2008) 18:253–60. doi: 10.1016/j.tcm.2008.11.008
30. Shpilsky D, Erqou S, Patel SR, Kip KE, Ajala O, Aiyer A, et al. Association of obstructive sleep apnea with microvascular endothelial dysfunction and subclinical coronary artery disease in a community-based population. Vasc Med. (2018) 23:331–9. doi: 10.1177/1358863X18755003
31. Bironneau V, Tamisier R, Trzepizur W, Andriantsitohaina R, Berger M, Goupil F, et al. Sleep apnoea and endothelial dysfunction: an individual patient data meta-analysis. Sleep Med Rev. (2020) 52:101309. doi: 10.1016/j.smrv.2020.101309
32. Recoquillon S, Gomez-Guzman M, Rodier M, Koffi C, Nitiema M, Gagnadoux F, et al. Non-muscular myosin light chain kinase triggers intermittent hypoxia-induced interleukin-6 release, endothelial dysfunction and permeability. Sci Rep. (2017) 7:13664. doi: 10.1038/s41598-017-13268-5
33. Makarenko VV, Usatyuk PV, Yuan G, Lee MM, Nanduri J, Natarajan V, et al. Intermittent hypoxia-induced endothelial barrier dysfunction requires ROS-dependent MAP kinase activation. Am J Physiol Cell Physiol. (2014) 306:C745–52. doi: 10.1152/ajpcell.00313.2013
34. Tuleta I, Franca CN, Wenzel D, Fleischmann B, Nickenig G, Werner N, et al. Hypoxia-induced endothelial dysfunction in apolipoprotein E-deficient mice; effects of infliximab and L-glutathione. Atherosclerosis. (2014) 236:400–10. doi: 10.1016/j.atherosclerosis.2014.08.021
35. Chang PK, Yen IC, Tsai WC, Chang TC, Lee SY. Protective effects of rhodiola crenulata extract on hypoxia-induced endothelial damage via regulation of AMPK and ERK pathways. Int J Mol Sci. (2018) 19:2286. doi: 10.3390/ijms19082286
36. Kyotani Y, Takasawa S, Yoshizumi M. Proliferative pathways of vascular smooth muscle cells in response to intermittent hypoxia. Int J Mol Sci. (2019) 20:2706. doi: 10.3390/ijms20112706
37. Kyotani Y, Itaya-Hironaka A, Yamauchi A, Sakuramoto-Tsuchida S, Makino M, Takasawa S, et al. Intermittent hypoxia-induced epiregulin expression by IL-6 production in human coronary artery smooth muscle cells. FEBS Open Bio. (2018) 8:868–76. doi: 10.1002/2211-5463.12430
38. Gabryelska A, Lukasik ZM, Makowska JS, Bialasiewicz P. Obstructive sleep apnea: from intermittent hypoxia to cardiovascular complications via blood platelets. Front Neurol. (2018) 9:635. doi: 10.3389/fneur.2018.00635
39. Koseoglu S, Ozcan KM, Ikinciogullari A, Cetin MA, Yildirim E, Dere H. Relationship between neutrophil to lymphocyte ratio, platelet to lymphocyte ratio, and obstructive sleep apnea syndrome. Adv Clin Exp Med. (2015) 24:623–7. doi: 10.17219/acem/58969
40. Lukasik ZM, Makowski M, Makowska JS. From blood coagulation to innate and adaptive immunity: the role of platelets in the physiology and pathology of autoimmune disorders. Rheumatol Int. (2018) 38:959–74. doi: 10.1007/s00296-018-4001-9
41. Drager LF, Polotsky VY, Lorenzi-Filho G. Obstructive sleep apnea: an emerging risk factor for atherosclerosis. Chest. (2011) 140:534–42. doi: 10.1378/chest.10-2223
42. Levy P, Bonsignore MR, Eckel J. Sleep, sleep-disordered breathing, and metabolic consequences. Eur Respir J. (2009) 34:243–60. doi: 10.1183/09031936.00166808
43. Ma L, Zhang J, Qiao Y, Sun X, Mao T, Lei S, et al. Intermittent hypoxia composite abnormal glucose metabolism-mediated atherosclerosis in vitro and in vivo: the role of SREBP-1. Oxid Med Cell Longev. (2019) 2019:4862760. doi: 10.1155/2019/4862760
44. Al-Terki A, Abu-Farha M, AlKhairi I, Cherian PT, Sriraman D, Shyamsundar A, et al. Increased level of angiopoietin like proteins 4 and 8 in people with sleep apnea. Front Endocrinol. (2018) 9:651. doi: 10.3389/fendo.2018.00651
45. Khalyfa A, Zhang C, Khalyfa AA, Foster GE, Beaudin AE, Andrade J, et al. Effect on intermittent hypoxia on plasma exosomal micro RNA signature and endothelial function in healthy adults. Sleep. (2016) 39:2077–90. doi: 10.5665/sleep.6302
46. Zhang J, Hu C, Jiao X, Yang Y, Li J, Yu H, et al. Potential role of mRNAs and LncRNAs in chronic intermittent hypoxia exposure-aggravated atherosclerosis. Front Genet. (2020) 11:290. doi: 10.3389/fgene.2020.00290
47. Chen Q, Lin G, Huang J, Chen L, Liu Y, Huang J, et al. Inhibition of miR-193a-3p protects human umbilical vein endothelial cells against intermittent hypoxia-induced endothelial injury by targeting FAIM2. Aging. (2020) 12:1899–909. doi: 10.18632/aging.102729
48. Lin G, Huang J, Chen Q, Chen L, Feng D, Zhang S, et al. miR-146a-5p mediates intermittent hypoxia-induced injury in H9c2 cells by targeting XIAP. Oxid Med Cell Longev. (2019) 2019:6581217. doi: 10.1155/2019/6581217
49. Almendros I, Wang Y, Gozal D. The polymorphic and contradictory aspects of intermittent hypoxia. Am J Physiol Lung Cell Mol Physiol. (2014) 307:L129–40. doi: 10.1152/ajplung.00089.2014
50. Xu WQ, Yu Z, Xie Y, Huang GQ, Shu XH, Zhu Y, et al. Therapeutic effect of intermittent hypobaric hypoxia on myocardial infarction in rats. Basic Res Cardiol. (2011) 106:329–42. doi: 10.1007/s00395-011-0159-y
51. Jackman KA, Zhou P, Faraco G, Peixoto PM, Coleman C, Voss HU, et al. Dichotomous effects of chronic intermittent hypoxia on focal cerebral ischemic injury. Stroke. (2014) 45:1460–7. doi: 10.1161/STROKEAHA.114.004816
52. Sanchez-de-la-Torre A, Soler X, Barbe F, Flores M, Maisel A, Malhotra A, et al. Cardiac troponin values in patients with acute coronary syndrome and sleep apnea: a pilot study. Chest. (2018) 153:329–38. doi: 10.1016/j.chest.2017.06.046
53. Shah N, Redline S, Yaggi HK, Wu R, Zhao CG, Ostfeld R, et al. Obstructive sleep apnea and acute myocardial infarction severity: ischemic preconditioning? Sleep Breath. (2013) 17:819–26. doi: 10.1007/s11325-012-0770-7
55. Won CHJ, Reid M, Sofer T, Azarbarzin A, Purcell S, White D, et al. Sex differences in obstructive sleep apnea phenotypes, the multi-ethnic study of atherosclerosis. Sleep. (2020) 43:zsz274. doi: 10.1093/sleep/zsz274
56. Miller JN, Schulz P, Pozehl B, Fiedler D, Fial A, Berger AM. Methodological strategies in using home sleep apnea testing in research and practice. Sleep Breath. (2017) 22:569–77. doi: 10.1007/s11325-017-1593-3
57. Rosen IM, Kirsch DB, Carden KA, Malhotra RK, Ramar K, Aurora RN, et al. Clinical use of a home sleep apnea test: an updated American Academy of Sleep Medicine Position Statement. J Clin Sleep Med. (2018) 14:2075–7. doi: 10.5664/jcsm.7540
58. Araujo I, Marques F, Andre S, Araujo M, Marques S, Ferreira R, et al. Diagnosis of sleep apnea in patients with stable chronic heart failure using a portable sleep test diagnostic device. Sleep Breath. (2018) 22:749–55. doi: 10.1007/s11325-017-1607-1
59. Aurora RN, Patil SP, Punjabi NM. Portable sleep monitoring for diagnosing sleep apnea in hospitalized patients with heart failure. Chest. (2018) 154:91–8. doi: 10.1016/j.chest.2018.04.008
60. Kim RD, Kapur VK, Redline-Bruch J, Rueschman M, Auckley DH, Benca RM, et al. An economic evaluation of home versus laboratory-based diagnosis of obstructive sleep apnea. Sleep. (2015) 38:1027–37. doi: 10.5665/sleep.4804
61. Nagappa M, Liao P, Wong J, Auckley D, Ramachandran SK, Memtsoudis S, et al. Validation of the STOP-bang questionnaire as a screening tool for obstructive sleep apnea among different populations: a systematic review and meta-analysis. PLoS ONE. (2015) 10:e0143697. doi: 10.1371/journal.pone.0143697
62. Chiu HY, Chen PY, Chuang LP, Chen NH, Tu YK, Hsieh YJ, et al. Diagnostic accuracy of the Berlin questionnaire, STOP-BANG, STOP, and Epworth sleepiness scale in detecting obstructive sleep apnea: a bivariate meta-analysis. Sleep Med Rev. (2017) 36:57–70. doi: 10.1016/j.smrv.2016.10.004
63. Reuter H, Herkenrath S, Treml M, Halbach M, Steven D, Frank K, et al. Sleep-disordered breathing in patients with cardiovascular diseases cannot be detected by ESS, STOP-BANG, and Berlin questionnaires. Clin Res Cardiol. (2018) 107:1071–8. doi: 10.1007/s00392-018-1282-7
64. Abumuamar AM, Dorian P, Newman D, Shapiro CM. The STOP-BANG questionnaire shows an insufficient specificity for detecting obstructive sleep apnea in patients with atrial fibrillation. J Sleep Res. (2018) 27:e12702. doi: 10.1111/jsr.12702
65. Srijithesh PR, Shukla G, Srivastav A, Goyal V, Singh S, Behari M. Validity of the Berlin questionnaire in identifying obstructive sleep apnea syndrome when administered to the informants of stroke patients. J Clin Neurosci. (2011) 18:340–3. doi: 10.1016/j.jocn.2010.04.048
66. Jafari A. Sleep apnoea detection from ECG using features extracted from reconstructed phase space and frequency domain. Biomed Signal Proces. (2013) 8:551–8. doi: 10.1016/j.bspc.2013.05.007
67. Chen L, Zhang X, Song C. An automatic screening approach for obstructive sleep apnea diagnosis based on single-lead electrocardiogram. IEEE T Autom Sci Eng. (2015) 12:106–15. doi: 10.1109/TASE.2014.2345667
68. Urtnasan E, Park J-U, Joo E-Y, Lee K-J. Automated detection of obstructive sleep apnea events from a single-lead electrocardiogram using a convolutional neural network. J Med Syst. (2018) 42:104. doi: 10.1007/s10916-018-0963-0
69. Papini GB, Fonseca P, van Gilst MM, van Dijk JP, Pevernagie DAA, Bergmans JWM, et al. Estimation of the apnea-hypopnea index in a heterogeneous sleep-disordered population using optimised cardiovascular features. Sci Rep. (2019) 9:17448. doi: 10.1038/s41598-019-53403-y
70. Mihaicuta S, Udrescu M, Topirceanu A, Udrescu L. Network science meets respiratory medicine for OSAS phenotyping and severity prediction. PeerJ. (2017) 5:e3289. doi: 10.7717/peerj.3289
71. Torres G, Sánchez-de-la-Torre M, Barbé F. Relationship between OSA and hypertension. Chest. (2015) 148:824–32. doi: 10.1378/chest.15-0136
72. Parati G, Lombardi C, Castagna F, Mattaliano P, Filardi PP, Agostoni P. Heart failure and sleep disorders. Nat Rev Cardiol. (2016) 13:389–403. doi: 10.1038/nrcardio.2016.71
73. Ahsan MJ, Latif A, Fazeel HM, Lateef N, Zoraiz Ahsan M, Kapoor V, et al. Obstructive sleep apnea and peripheral vascular disease: a systematic review based on current literature. J Community Hosp Intern Med Perspect. (2020) 10:188–93. doi: 10.1080/20009666.2020.1764276
74. Pizarro C, Schaefer C, Kimeu I, Pingel S, Horlbeck F, Tuleta I, et al. Underdiagnosis of obstructive sleep apnoea in peripheral arterial disease. Respiration. (2015) 89:214–20. doi: 10.1159/000371355
75. Schaefer CA, Adam L, Weisser-Thomas J, Pingel S, Vogel G, Klarmann-Schulz U, et al. High prevalence of peripheral arterial disease in patients with obstructive sleep apnoea. Clin Res Cardiol. (2015) 104:719–26. doi: 10.1007/s00392-015-0834-3
76. Nagayoshi M, Lutsey PL, Benkeser D, Wassel CL, Folsom AR, Shahar E, et al. Association of sleep apnea and sleep duration with peripheral artery disease: the Multi-Ethnic Study of Atherosclerosis (MESA). Atherosclerosis. (2016) 251:467–75. doi: 10.1016/j.atherosclerosis.2016.06.040
77. Marshall NS, Barnes M, Travier N, Campbell AJ, Pierce RJ, McEvoy RD, et al. Continuous positive airway pressure reduces daytime sleepiness in mild to moderate obstructive sleep apnoea: a meta-analysis. Thorax. (2006) 61:430–4. doi: 10.1136/thx.2005.050583
78. Antic NA, Catcheside P, Buchan C, Hensley M, Naughton MT, Rowland S, et al. The effect of CPAP in normalizing daytime sleepiness, quality of life, and neurocognitive function in patients with moderate to severe OSA. Sleep. (2011) 34:111–9. doi: 10.1093/sleep/34.1.111
79. Drager LF, Bortolotto LA, Figueiredo AC, Krieger EM, Lorenzi GF. Effects of continuous positive airway pressure on early signs of atherosclerosis in obstructive sleep apnea. Am J Respir Crit Care Med. (2007) 176:706–12. doi: 10.1164/rccm.200703-500OC
80. Bratton DJ, Gaisl T, Wons AM, Kohler M. CPAP vs. mandibular advancement devices and blood pressure in patients with obstructive sleep apnea: a systematic review and meta-analysis. JAMA. (2015) 314:2280–93. doi: 10.1001/jama.2015.16303
81. Peker Y, Thunstrom E, Glantz H, Wegscheider K, Eulenburg C. Outcomes in coronary artery disease patients with sleepy obstructive sleep apnoea on CPAP. Eur Respir J. (2017) 50:1700749. doi: 10.1183/13993003.00749-2017
82. Wu X, Lv S, Yu X, Yao L, Mokhlesi B, Wei Y. Treatment of OSA reduces the risk of repeat revascularization after percutaneous coronary intervention. Chest. (2015) 147:708–18. doi: 10.1378/chest.14-1634
83. Barbe F, Duran-Cantolla J, Sanchez-de-la-Torre M, Martinez-Alonso M, Carmona C, Barcelo A, et al. Effect of continuous positive airway pressure on the incidence of hypertension and cardiovascular events in non-sleepy patients with obstructive sleep apnea: a randomized controlled trial. JAMA. (2012) 307:2161–8. doi: 10.1001/jama.2012.4366
84. McEvoy RD, Antic NA, Heeley E, Luo Y, Ou Q, Zhang X, et al. CPAP for prevention of cardiovascular events in obstructive sleep apnea. N Engl J Med. (2016) 375:919–31. doi: 10.1056/NEJMoa1606599
85. Peker Y, Glantz H, Eulenburg C, Wegscheider K, Herlitz J, Thunstrom E. Effect of positive airway pressure on cardiovascular outcomes in coronary artery disease patients with non-sleepy obstructive sleep apnea. The RICCADSA randomized controlled trial. Am J Respir Crit Care Med. (2016) 194:613–20. doi: 10.1164/rccm.201601-0088OC
86. Guralnick AS, Balachandran JS, Szutenbach S, Adley K, Emami L, Mohammadi M, et al. Educational video to improve CPAP use in patients with obstructive sleep apnoea at risk for poor adherence: a randomised controlled trial. Thorax. (2017) 72:1132–9. doi: 10.1136/thoraxjnl-2017-210106
87. Pépin JL, Tamisier R, Hwang D, Mereddy S, Parthasarathy S. Does remote monitoring change OSA management and CPAP adherence? Respirology. (2017) 22:1508–17. doi: 10.1111/resp.13183
88. Hwang D, Chang JW, Benjafield AV, Crocker ME, Kelly C, Becker KA, et al. Effect of telemedicine education and telemonitoring on continuous positive airway pressure adherence. The Tele-OSA randomized trial. Am J Respir Crit Care Med. (2018) 197:117–26. doi: 10.1164/rccm.201703-0582OC
89. Thakkar J, Kurup R, Laba TL, Santo K, Thiagalingam A, Rodgers A, et al. Mobile telephone text messaging for medication adherence in chronic disease: a meta-analysis. JAMA Intern Med. (2016) 176:340–9. doi: 10.1001/jamainternmed.2015.7667
90. Malhotra A, Crocker ME, Willes L, Kelly C, Lynch S, Benjafield AV. Patient engagement using new technology to improve adherence to positive airway pressure therapy. Chest. (2018) 153:843–50. doi: 10.1016/j.chest.2017.11.005
91. Pépin J-L, Jullian-Desayes I, Sapène M, Treptow E, Joyeux-Faure M, Benmerad M, et al. Multimodal remote monitoring of high cardiovascular risk patients with OSA initiating CPAP. Chest. (2019) 155:730–9. doi: 10.1016/j.chest.2018.11.007
92. Basyuni S, Barabas M, Quinnell T. An update on mandibular advancement devices for the treatment of obstructive sleep apnoea hypopnoea syndrome. J Thorac Dis. (2018) 10(Suppl. 1):S48–56. doi: 10.21037/jtd.2017.12.18
93. Rietz H, Franklin KA, Carlberg B, Sahlin C, Marklund M. Nocturnal blood pressure is reduced by a mandibular advancement device for sleep apnea in women: findings from secondary analyses of a randomized trial. J Am Heart Assoc. (2018) 7:e008642. doi: 10.1161/JAHA.118.008642
94. Gagnadoux F, Pepin JL, Vielle B, Bironneau V, Chouet-Girard F, Launois S, et al. Impact of mandibular advancement therapy on endothelial function in severe obstructive sleep apnea. Am J Respir Crit Care Med. (2017) 195:1244–52. doi: 10.1164/rccm.201609-1817OC
95. Yamamoto U, Nishizaka M, Tsuda H, Tsutsui H, Ando SI. Crossover comparison between CPAP and mandibular advancement device with adherence monitor about the effects on endothelial function, blood pressure and symptoms in patients with obstructive sleep apnea. Heart Vessels. (2019) 34:1692–702. doi: 10.1007/s00380-019-01392-3
96. de Vries GE, Hoekema A, Vermeulen KM, Claessen JQPJ, Jacobs W, van der Maten J, et al. Clinical- and cost-effectiveness of a mandibular advancement device versus continuous positive airway pressure in moderate obstructive sleep apnea. J Clin Sleep Med. (2019) 15:1477–85. doi: 10.5664/jcsm.7980
97. Vitacca M, Paneroni M, Braghiroli A, Balbi B, Aliani M, Guido P, et al. Exercise capacity and comorbidities in patients with obstructive sleep apnea. J Clin Sleep Med. (2020) 16:531–8. doi: 10.5664/jcsm.8258
98. Mendelson M, Bailly S, Marillier M, Flore P, Borel JC, Vivodtzev I, et al. Obstructive sleep apnea syndrome, objectively measured physical activity and exercise training interventions: a systematic review and meta-analysis. Front Neurol. (2018) 9:73. doi: 10.3389/fneur.2018.00073
99. Torres-Castro R, Vilaro J, Marti JD, Garmendia O, Gimeno-Santos E, Romano-Andrioni B, et al. Effects of a combined community exercise program in obstructive sleep apnea syndrome: a randomized clinical trial. J Clin Med. (2019) 8:361. doi: 10.3390/jcm8030361
100. Servantes DM, Javaheri S, Kravchychyn ACP, Storti LJ, Almeida DR, de Mello MT, et al. Effects of exercise training and CPAP in patients with heart failure and OSA: a preliminary study. Chest. (2018) 154:808–17. doi: 10.1016/j.chest.2018.05.011
101. Mendelson M, Lyons OD, Yadollahi A, Inami T, Oh P, Bradley TD. Effects of exercise training on sleep apnoea in patients with coronary artery disease: a randomised trial. Eur Respir J. (2016) 48:142–50. doi: 10.1183/13993003.01897-2015
102. Mendelson M, Inami T, Lyons O, Alshaer H, Marzolini S, Oh P, et al. Long-term effects of cardiac rehabilitation on sleep apnea severity in patients with coronary artery disease. J Clin Sleep Med. (2020) 16:65–71. doi: 10.5664/jcsm.8124
103. Strohl M, Strohl K, Palomo JM, Ponsky D. Hypoglossal nerve stimulation rescue surgery after multiple multilevel procedures for obstructive sleep apnea. Am J Otolaryngol. (2016) 37:51–3. doi: 10.1016/j.amjoto.2015.08.008
104. Gillespie MB, Soose RJ, Woodson BT, Strohl KP, Maurer JT, de Vries N, et al. Upper airway stimulation for obstructive sleep apnea: patient-reported outcomes after 48 months of follow-up. Otolaryngol Head Neck Surg. (2017) 156:765–71. doi: 10.1177/0194599817691491
105. Philip P, Heiser C, Bioulac S, Altena E, Penchet G, Cuny E, et al. Hypoglossal nerve stimulation on sleep and level of alertness in OSA: A preliminary study. Neurology. (2018) 91:e615–9. doi: 10.1212/WNL.0000000000006001
106. Kompelli AR, Ni JS, Nguyen SA, Lentsch EJ, Neskey DM, Meyer TA. The outcomes of hypoglossal nerve stimulation in the management of OSA: A systematic review and meta-analysis. World J Otorhinolaryngol Head Neck Surg. (2019) 5:41–8. doi: 10.1016/j.wjorl.2018.04.006
107. Wang W, Song A, Zeng Y, Chen X, Zhang Y, Shi Y, et al. Telmisartan protects chronic intermittent hypoxic mice via modulating cardiac renin-angiotensin system activity. BMC Cardiovasc Disord. (2018) 18:133. doi: 10.1186/s12872-018-0875-4
108. Siti HN, Kamisah Y, Kamsiah J. The role of oxidative stress, antioxidants and vascular inflammation in cardiovascular disease (a review). Vascul Pharmacol. (2015) 71:40–56. doi: 10.1016/j.vph.2015.03.005
109. Thunstrom E, Manhem K, Rosengren A, Peker Y. Blood pressure response to losartan and continuous positive airway pressure in hypertension and obstructive sleep apnea. Am J Respir Crit Care Med. (2016) 193:310–20. doi: 10.1164/rccm.201505-0998OC
110. Yeung HM, Hung MW, Lau CF, Fung ML. Cardioprotective effects of melatonin against myocardial injuries induced by chronic intermittent hypoxia in rats. J Pineal Res. (2015) 58:12–25. doi: 10.1111/jpi.12190
111. Javaheri S, Brown LK, Abraham WT, Khayat R. Apneas of heart failure and phenotype-guided treatments: part one: OSA. Chest. (2020) 157:394–402. doi: 10.1016/j.chest.2019.02.407
112. Sands SA, Edwards BA, Terrill PI, Butler JP, Owens RL, Taranto-Montemurro L, et al. Identifying obstructive sleep apnoea patients responsive to supplemental oxygen therapy. Eur Respir J. (2018) 52:1800674. doi: 10.1183/13993003.00674-2018
113. Taranto-Montemurro L, Sands SA, Edwards BA, Azarbarzin A, Marques M, de Melo C, et al. Desipramine improves upper airway collapsibility and reduces OSA severity in patients with minimal muscle compensation. Eur Respir J. (2016) 48:1340–50. doi: 10.1183/13993003.00823-2016
114. Taranto-Montemurro L, Edwards BA, Sands SA, Marques M, Eckert DJ, White DP, et al. Desipramine increases genioglossus activity and reduces upper airway collapsibility during non-REM sleep in healthy subjects. Am J Respir Crit Care Med. (2016) 194:878–85. doi: 10.1164/rccm.201511-2172OC
Keywords: obstructive sleep apnea, atherosclerosis, hypoxia, mechanism, treatment
Citation: Chen J, Lin S and Zeng Y (2021) An Update on Obstructive Sleep Apnea for Atherosclerosis: Mechanism, Diagnosis, and Treatment. Front. Cardiovasc. Med. 8:647071. doi: 10.3389/fcvm.2021.647071
Received: 29 December 2020; Accepted: 16 March 2021;
Published: 08 April 2021.
Edited by:
Tetsuro Miyazaki, Juntendo University Urayasu Hospital, JapanReviewed by:
Jian Xu, University of Oklahoma Health Sciences Center, United StatesCopyright © 2021 Chen, Lin and Zeng. This is an open-access article distributed under the terms of the Creative Commons Attribution License (CC BY). The use, distribution or reproduction in other forums is permitted, provided the original author(s) and the copyright owner(s) are credited and that the original publication in this journal is cited, in accordance with accepted academic practice. No use, distribution or reproduction is permitted which does not comply with these terms.
*Correspondence: Yiming Zeng, emVuZ195aV9taW5nQDEyNi5jb20=; Shu Lin, c2h1bGluMTk1NkAxMjYuY29t
Disclaimer: All claims expressed in this article are solely those of the authors and do not necessarily represent those of their affiliated organizations, or those of the publisher, the editors and the reviewers. Any product that may be evaluated in this article or claim that may be made by its manufacturer is not guaranteed or endorsed by the publisher.
Research integrity at Frontiers
Learn more about the work of our research integrity team to safeguard the quality of each article we publish.