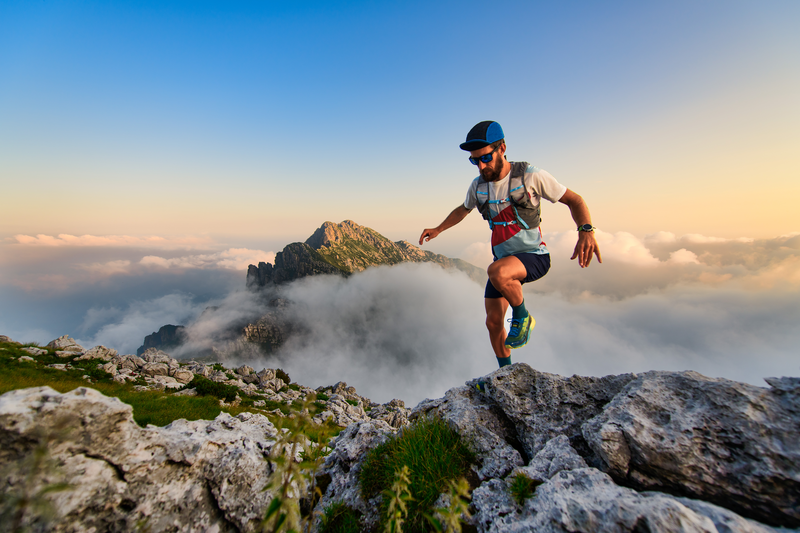
94% of researchers rate our articles as excellent or good
Learn more about the work of our research integrity team to safeguard the quality of each article we publish.
Find out more
REVIEW article
Front. Cardiovasc. Med. , 15 February 2021
Sec. Cardiovascular Therapeutics
Volume 8 - 2021 | https://doi.org/10.3389/fcvm.2021.642843
This article is part of the Research Topic New Strategies to Inhibit Cell Death in Myocardial Ischemia-Reperfusion Injury: How to succeed? View all 17 articles
Therapeutic hypothermia has been used for treating brain injury after out-of-hospital cardiac arrest. Its potential benefit on minimizing myocardial ischemic injury has been explored, but clinical evidence has yet to confirm positive results in preclinical studies. Importantly, therapeutic hypothermia for myocardial infarction is unique in that it can be initiated prior to reperfusion, in contrast to its application for brain injury in resuscitated cardiac arrest patients. Recent advance in cooling technology allows more rapid cooling of the heart than ever and new clinical trials are designed to examine the efficacy of rapid therapeutic hypothermia for myocardial infarction. In this review, we summarize current knowledge regarding the effect of hypothermia on normal and ischemic hearts and discuss issues to be solved in order to realize its clinical application for treating acute myocardial infarction.
The impact of temperature on human biology has been researched extensively and a number of experimental studies have shown that lowering body temperature is capable of protecting tissues from injury (1–7). To take advantage of this protective effect, the concept of therapeutic hypothermia (TH) has been developed and tested in patients with various diseases. Different levels of hypothermia including mild (32–35°C), moderate (28–32°C), severe (20–28°C), and profound (<20°C) were proposed (8). Clinical application of TH for acute diseases is mainly limited to mild and moderate hypothermia due to technical challenges and increased risks of arrhythmias at lower temperature range. Meanwhile, deeper TH has been applied to protect organs during circulatory arrest for cardiac and aortic surgeries (9). Successful demonstration of injury limitation in experimental studies and early clinical trials (10, 11) prompted researchers to use TH also for ST-elevation myocardial infarction (STEMI). However, much of the current clinical evidence of TH in organ protection was derived from studies that focused on neurological injury, while those focusing on the ischemic heart remain limited. Whereas reperfusion has already taken place in the brains of patients after resuscitation for out-of-hospital cardiac arrest (OHCA), the circumstances of STEMI are unique in that hypothermia can be applied prior to the reperfusion of ischemic myocardium. It therefore offers targeting of reperfusion injury in addition to post-reperfusion injury. The emergence of new devices and techniques that allow rapid cooling of the heart opens the door for discussion on whether priority should be placed on the attainment of a target temperature or on more rapid reperfusion. In this review, we summarize our current knowledge related to the impact of TH on the heart and discuss its potential benefit for treating STEMI.
A decrease in heart rate is the most consistently reported electrophysiological change associated with TH in both normal and ischemic hearts (12–15). In addition to a reduced sinus rate, atrial and ventricular conduction velocities seem to decrease under hypothermia as represented by prolongation of PR, QRS, and QT intervals (16–18). Whether profound bradycardia during TH for MI would require intervention remains unclear, but bradycardia was apparently a favorable marker for patients undergoing TH after resuscitation following OHCA (14). Although it is unknown if lower heart rate in this study actually contributed to the good outcome or it was just reflecting less myocardial injury, bradycardia may be treated conservatively unless there is an evidence of organ hypo-perfusion.
The J wave is a characteristic ECG change found in some hypothermic patients. Up to 30% of patients after OHCA presented with J waves during TH and its prevalence was found to be higher in patients with STEMI (19). In cases of accidental hypothermia, the J wave was observed more frequently in patients having lower body temperatures (20, 21), suggesting a temperature dependent increase in its appearance. Interestingly, there was also an inverse correlation between temperature and the size of the J wave (21). These ECG changes sometimes mimic those of STEMI. In fact, Rolfast et al. (19) reported ST changes during TH in some OHCA patients who lacked actual coronary occlusion, which was confirmed by coronary angiograms.
Potential increase in incidence of atrial and ventricular arrhythmias has been a concern for applying TH in STEMI patients. In the COOL AMI EU pilot trial, which used an endovascular cooling method, the incidence of atrial fibrillation was more common in the TH group (32%) compared to the control group (8%, P = 0.07) (22). Using naïve pigs, Manninger et al. (18) found that TH prolongs the effective atrial refractory period at 33°C, which was accompanied by an increase in pacing-induced atrial fibrillation. However, the serum potassium level was decreased during hypothermia in this study, suggesting a potential influence of the dysregulated electrolyte. In contrast, in the post hoc analysis of a Targeted Temperature Management (TTM) trial, TH was not associated with the incidence of atrial fibrillation in patients with new-onset STEMI (23). Combined analysis of the RAPID MI-ICE and the CHILL-MI trials also exhibited no difference in atrial fibrillation incidence (24). Within the temperature range used in STEMI cooling studies, no significant increase in ventricular arrhythmias has been reported (22, 24), a finding consistent with animal studies (25). Taken together, mild TH seems not to significantly increase the occurrence of arrhythmia in general STEMI patients, but there could be a subpopulation of patients more prone to the development of arrhythmias, such as those with electrolyte dysregulation. Because hypothermia can dysregulate electrolyte balance through volume shift and by influencing kidney excretion (26), careful electrolyte monitoring is likely important.
In non-diseased hearts, several ex vivo studies reproducibly showed that hypothermia increased cardiac contractility (27–30). Despite increasing contractility, however, myocardial oxygen consumption remained similar and hypothermia was thus believed to improve myocardial energy efficiency (27, 31). The contractility increase was accompanied by prolonged systolic time (27, 32), resulting in a delay in achieving end-systole during ventricular ejection. Because of the prolonged systole, systolic functional parameters that include time component (e.g., maximum dP/dt, tissue Doppler velocity) did not necessary indicate improvement, whereas time-independent contractility parameters such as Emax or slope of end-systolic pressure-volume relationship generally showed an increase (12, 27–30). Some conflicting results exist for in vivo studies showing decreases in stroke volume or cardiac output (33, 34), but this was likely associated with complex biological interactions such as neuromodulation (35) and altered vascular resistance (36, 37).
As discussed above, heart rate slows by TH and helps to compensate for reduced diastolic time associated with prolonged systole. But even with lower heart rate, diastolic functional parameters are usually impaired under hypothermic conditions (37). Both active relaxation, as assessed by cardiac relaxation time constant, tau, or minimum dP/dt, and left ventricular stiffness, as assessed by left ventricular end-diastolic pressure or end-diastolic pressure-volume relationships, can be impaired by cooling (12, 38, 39). Myocardial stiffening associated with lower myocardial temperature might be responsible for increase in end-systolic and end-diastolic elastance. Meanwhile, increasing the heart rate by pacing has been shown to impair systolic function and also to worsen diastolic dysfunction (40). These results suggest mechanistic importance of prolonged systole for maintaining systolic function.
There are also limited data on the impact of hypothermia on cardiac function during myocardial ischemia, but available data suggest that functional changes in response to hypothermia are generally similar to those of the normal heart (41, 42). Interestingly, some previous studies before the reperfusion era showed that myocardial function (cardiac output and left ventricular stroke work) was better in hypothermia-treated animals after rewarming compared to the normothermic animals, despite the absence of reperfusion (33, 43). Whether hypothermia and rewarming also improves cardiac function without coronary reperfusion as these authors suggested or it was associated with rewarming induced vasodilation remains unclear. In either case, more data on rewarming after reperfusion is necessary to devise appropriate exit strategies for cardiac TH.
In summary, TH seems to have positive or at least neutral effects on contractility, but negative effects on diastolic function. Yet, studies that examined the impact of TH on in vivo heart function are limited and data are not always consistent with ex vivo findings. These are likely related to the difference in experimental settings including method and speed of cooling, animal species, anesthesia, and means of functional assessment.
A large body of data on protective mechanisms associated with hypothermia derives from studies in neurons or in the arrested human heart at much lower temperatures. Limited studies have investigated mild hypothermia mediated protection in the myocardial ischemia setting (44–50). Nevertheless, available studies report similar mechanisms in the ischemic myocardium to those found in neuron studies in preventing post-reperfusion injury (8). However, TH can be applied prior to reperfusion in STEMI and it may offer an additive benefit by alleviating ischemia before reperfusion and also by reducing reperfusion injury at the early phase of reperfusion. Potential mechanisms of infarct size reduction by TH are discussed here.
As discussed above, cardiac contractility is expected to be preserved during mild TH whereas myocardial energy efficiency is improved. TH also reduces heart rate. These are expected to reduce myocardial oxygen consumption related to the pump function (mechanical work) and alleviate ischemia progression. TH can also affect cardiac metabolism. Whole body metabolism and oxygen consumption decrease substantially as the temperature decreases (51). The relationship between temperature and oxygen consumption is likely non-linear, with a greater reduction of oxygen consumption in the first few degrees from normothermia (52, 53). It is expected that the ischemic myocardium also follows this relationship, and thus oxygen demand as well as tissue metabolism are likely suppressed early after TH induction. In a rabbit heart, initiation of epicardial cooling before MI preserved tissue adenosine triphosphate (ATP) and glycogen in the ischemic myocardium 20 min after MI (44). Reduced metabolism would also alleviate cellular acidosis, which can trigger cell death (54). However, it remains uncertain if and to what extent reductions in metabolism and oxygen consumption would offer benefit in the already ischemic myocardium, since energy stores are likely depleted by the time TH is initiated, unless started immediately after the onset of ischemia. Using a dog isolated heart, Jones et al. (55) reported that despite a 50% reduction in both ATP utilization and anaerobic glycolytic ATP production, energy deprivation could not be prevented and all hearts resulted in contracture-rigor, although with some delay in the hypothermia treated hearts. Thus, it is convincing that mechanisms other than mere reduction in energy consumption play important roles in myocardial protection during ischemia and reperfusion processes. That being said, slowing of energy utilization might offer large benefits to patients who have rich collateral supply to the ischemic myocardium, those with partially recanalized coronary, or those who arrived hospital early after the ischemia onset.
Reperfusion injury is estimated to cause around 50% of total myocardial injury in MI (56). Various mechanisms contribute to reperfusion injury and hypothermia seems to inhibit many of these pathological processes at the cellular level (26, 57). For example, TH has been shown to reduce cellular calcium load after reperfusion (58), which causes cell necrosis via the opening of mitochondrial permeability transition pores. Hypothermia-mediated apoptosis inhibition has been shown in many studies of neuron injury (59, 60), but data in myocardial ischemia-reperfusion injury remain scarce. In vitro studies using cardiomyocyte cell lines indicate that apoptosis of these cells at reperfusion following oxygen/energy deprivation is suppressed by TH (61, 62). Using an isolated rabbit heart, Ning et al. (63) reported reduced apoptosis in hearts maintained at 30°C during ischemia compared to those maintained at 34°C, but unfortunately, this study did not include settings at higher temperatures. Upon ischemia-reperfusion, rapid increases in oxygen radicals induce tissue oxidative stress, which has been shown to be inhibited by TH in both the heart (64) and neurons (65). Additionally, maintenance of cellular membrane integrity by hypothermia might prevent cellular edema. This is supported by a study that reported reduced myocardial edema after TH which was detected by magnetic resonance imaging in a pig model of ischemia-reperfusion (66). Hypothermia has also been reported to suppress post-ischemic inflammation via reduction of pro-inflammatory cytokine release (67) and local immune cell activation (66).
At the molecular level, Yang et al. (68) showed that hypothermia (35°C) increases extracellular signal-regulated kinase (ERK) activity in isolated rabbit hearts, the inhibition of which abolished the beneficial effects on infarct size. Using rat isolated hearts, Mochizuki et al. (46) reported that nitric oxide (NO) and phosphatidylinositol 3'-kinase (PI3K) are the key molecules in hypothermia (34°C) mediated infarct size reduction. Other studies also reported that increased AKT phosphorylation (47), reduced p53 expression, and increased heme-oxygenase 1 (50) play major roles in hypothermia-mediated reduction of reperfusion injury.
Interestingly, several reports indicate that hypothermia initiated after reperfusion does not reduce infarct size, whereas its initiation before reperfusion does so even if it is delayed from onset of the ischemia (69, 70). These results suggest that hypothermia may precondition the heart to alleviate injury associated with the very acute phase of reperfusion, which is expected to be the major portion of total reperfusion injury as shown in Figure 1. This might be the unique feature of hypothermia that allows alteration of ischemic myocardial wall property i.e., temperature, through endocardial transmission in the absence of coronary recanalization, which is not possible by pharmacological approaches. It remains uncertain, however, what mechanisms underlie in this protective preconditioning effect, since most of the previous reports studied myocardial molecular changes after the reperfusion.
Figure 1. Expected impact of therapeutic hypothermia on myocardial ischemia and reperfusion injury. The pink line shows the time course of myocardial injury associated with ischemia and reperfusion. X axis indicates time after ischemia onset and Y axis indicates infarcted myocardium relative to ischemia area-at-risk. Largest injury is expected just after the reperfusion. Blue line shows the expected time course of myocardial injury with TH when applied at the same time as the ischemia. Depending on when TH is started, the line may diverge at that time point. Preclinical data suggest that TH started at the time of reperfusion does not reduce myocardial injury. Therefore, TH during ischemia likely has preconditioning effect that prepares the myocardium for reperfusion. Expected strength of this preconditioning effect, ischemia alleviation, and attenuation of reperfusion injury are shown in the top of the Figure.
In addition to the reduction of infarct size, TH has been shown to offer beneficial effects on post-reperfusion coronary flow. In a series of experiments, Hale et al. (71, 72) reported that direct ice bag cooling of the rabbit heart initiated at the peri-reperfusion period reduced the no-reflow phenomenon without a change in acute infarct size. This result is supported by a study in pigs by Gotberg et al. (73), which demonstrated significant reduction of microvascular obstruction in the ischemic area as assessed by single photon emission computed tomography. However, clinical trials have yet to confirm these findings in humans and one randomized trial failed to find differences in the size of microvascular obstruction areas between patients treated with hypothermia and controls (74). More recently, Testori et al. (75) also reported that microvascular obstruction area assessed by cardiac magnetic resonance imaging was not different between the patients treated with and without TH 4 days after the onset of STEMI.
As discussed above, mild TH increases cardiac contractility. Vasoconstriction of the peripheral vasculature increases systemic vascular resistance (36, 37) and raises arterial pressure. In addition, systemic hypothermia reduces metabolic demand of the whole body (76), which improves supply/demand of the non-cardiac organs. Therefore, theoretically, hypothermia would be an appropriate therapy for cardiogenic shock. Clinical studies in cardiogenic shock patients after cardiac surgery reported an increase in venous oxygen saturation upon hypothermia, indicating improved whole body oxygen supply/demand (12, 77, 78). Meanwhile, post-hoc analysis of TTM trial found that patients that required high dose of vasopressors were more common in the 33°C group than 36°C group (79). Whether this would be the same for patients in cardiogenic shock remains unclear. In a recent randomized trial involving 40 patients with cardiogenic shock, hypothermia failed to improve cardiac power as well as clinical outcome (80). Because only around half of the patients were STEMI-associated cardiogenic shock, more studies are needed to determine if hypothermia is safe and efficacious in treating STEMI-associated cardiogenic shock.
Infarct size reduction by TH has been studied in various animal species with variety of cooling methods, ischemia duration, cooling duration, and timing of cooling initiation. While small animal studies are useful in studying the mechanisms, large differences in body size, morphology, and heart size that allow much faster cooling may not fully represent cooling conditions in a clinical setting. Large animal models offer a simulation of hypothermia in clinically relevant conditions and also allow endovascular or intracoronary cooling approaches, which are not feasible in small animals. A summary of representative preclinical experiments in large animals that examined the impact of hypothermia on infarct size is provided in Supplementary Table 1 (33, 42, 64, 66, 69–73, 81–97). In general, studies that initiated cooling prior to reperfusion have shown reduction of infarct size, whereas studies that initiated cooling just prior or after reperfusion tended to show no benefit, regardless of the cooling method. The majority of these studies only looked at the acute impact of TH and there are very few data on the impact of rewarming on infarct size. Accordingly, whether acute benefits on the infarct size can be maintained throughout the chronic phase remain unclear.
Similar to preclinical studies, various approaches and devices have been employed for the controlled and efficient cooling of patients in clinical trials targeting STEMI (Table 1) (22, 74, 75, 98–109). The ideal cooling method for STEMI application would be one that offers rapid cooling with the ability to control body temperature throughout the temperature management period, from initiation through the rewarming phase. The ideal method would also be minimally invasive and implemented easily, in an ambulance if necessary, and without significant side effects. Shivering in response to cooling in awake patients is another factor that needs attention because it can significantly increase body oxygen demand and slow cooling speed. Counter-heating of the skin during TH seems to be effective in reducing the shivering (110, 111), which is obviously not available for surface cooling methods and necessitate anti-shivering drug administrations. There is currently no single method that meets all above ideal features, leaving each with its own advantages and disadvantages (Table 2 and Supplementary Table 2).
Ly et al. (101) used surface cooling and reported that target temperature of 34.5°C could be achieved in an average of 79 min. Although the trial did not find safety issues, no follow-up studies have been conducted, likely due to advances in cooling technology that offered much faster cooling. Peritoneal cooling was tested in 54 STEMI patients who were randomized to hypothermia (n = 28) and control (n = 26) (103). The study demonstrated that peritoneal cooling offers rapid cool-down of patients. However, there was no reduction of infarct size, whereas some concerns regarding increased safety issues were noted. Intracoronary hypothermia is another invasive approach that has been shown to be effective in rapidly and locally lowering the myocardial temperature (112). In a recent trial conducted in Europe, 10 patients were treated with intracoronary hypothermia by injecting room temperature saline through the coronary balloon catheter wire lumen, which was followed by 4°C saline injection after reperfusion (99). The authors reported arrhythmic events in patients with inferior MI, but not in the anterior MI, concluding that it was safe and feasible in patients with anterior MI. Follow up randomized studies are currently recruiting patients in Europe with an expected enrollment of 200 patients [Clinicaltrials.gov identifier NCT03447834 (113) accessed on Jan 15th, 2021]. Endovascular cooling has been the most popular method in the past STEMI trials likely owing to its relatively fast cooling, feasible application, and without requiring substantial amount of fluid loading. Some of these trials examined feasibility and safety (100, 102, 104), which were successfully confirmed, but none of the subsequent efficacy trials were able to meet the primary efficacy endpoints (22, 74, 75, 98, 106). More recently, Dae et al. (90) combined the data of 6 previous randomized clinical trials that used endovascular cooling method and analyzed the infarct size at 1 month after MI on a patient basis. Overall, 629 patients were included in the analysis and the study identified that anterior MI patients who were cooled to below 35°C at the time or reperfusion did show reduced infarct size over the control group, whereas other patient populations failed to show infarct size reduction. These results strongly suggest that rapid cooling to below 35°C is necessary, and patients with larger MI benefit most from TH. As we illustrated in Figure 1, we expect that the inhibition of reperfusion injury offers major benefit in TH. If 35°C is the threshold temperature to inhibit reperfusion injury, some patients may require additional time to reach this point before reperfusion. Existing data is insufficient to determine if delaying reperfusion for a short period to achieve target temperature below 35°C offers more benefit than immediate reperfusion. Currently, a clinical trial that aims to test the safety of new powerful endovascular cooling device is planned in the US [Clinicaltrials.gov identifier NCT03361995 (114) accessed on Jan 15th, 2021] and might provide more information on the temperature threshold at the time of reperfusion.
Although blood temperature is expected to correlate with cardiac temperature, direct monitoring of ischemic myocardial temperature in STEMI setting is challenging. Moreover, cooling speed of the heart and other organs varies depending on the employed method (115). Therefore, inconclusive results in above discussed studies might be associated with insufficient lowering of the ischemic myocardium in contrast to the reported measured temperature. Unlike animal experiments, infarct size measurement in humans relies on imaging modalities and these indirect measures of infarct size assessment could be the source of measurement errors that can obscure the results (75). Additionally, some of the patients presenting STEMI might already have reperfusion at the time of the first coronary angiogram (116). Based on preclinical studies and the recent report by Dae et al. (90), these patients may not benefit from TH since reperfusion has already taken place. The efficacy of some drugs is known to be impaired at low temperature and these drug interactions need careful attention (26). There is a possibility that TH might have synergistic effects when combined with other therapies directed at reducing myocardial infarction (117), and this area remains largely unexplored yet.
Available evidence suggests that TH has the potential to reduce myocardial ischemic injury in humans. However, randomized clinical trials have yet to prove promising results in preclinical studies. Compared to the large number of studies focusing on TH for post-resuscitation brain injury or myocardial protection for surgery, that of alleviating myocardial reperfusion injury remains much less. Accordingly, there remain many questions that are only vaguely answered. These include: (1) Optimal target temperature for STEMI application; (2) Optimal TH method; (3) Whether target temperature needs to be achieved prior to reperfusion; (4) Optimal duration of hypothermia; (5) Mechanisms of myocardial protection; (6) Optimal target patient population; and (7) Optimal protocol for rewarming. Emergence of new devices that allow faster cooling may help to better define some of these questions and lead to positive results in forthcoming clinical trials.
KI drafted the manuscript. All other authors critically edited the manuscript and provided key information on this review article. All authors contributed to the article and approved the submitted version.
KI was supported by NIH R01 HL139963 and AHA-SDG 17SDG33410873. TA was supported by fellowships from the Uehara memorial Foundation, the Kanzawa Medical Research Foundation, the Suginome Memorial Foundation, and the Nakayama Foundation for Human Science.
The authors declare that the research was conducted in the absence of any commercial or financial relationships that could be construed as a potential conflict of interest.
The Supplementary Material for this article can be found online at: https://www.frontiersin.org/articles/10.3389/fcvm.2021.642843/full#supplementary-material
1. Delbridge MS, Shrestha BM, Raftery AT, El Nahas AM, Haylor JL. The effect of body temperature in a rat model of renal ischemia-reperfusion injury. Transplant Proc. (2007) 39:2983–5. doi: 10.1016/j.transproceed.2007.04.028
2. Zager RA, Gmur DJ, Bredl CR, Eng MJ. Degree and time sequence of hypothermic protection against experimental ischemic acute renal failure. Circ Res. (1989) 65:1263–9. doi: 10.1161/01.RES.65.5.1263
3. Kuboyama K, Safar P, Radovsky A, Tisherman SA, Stezoski SW, Alexander H. Delay in cooling negates the beneficial effect of mild resuscitative cerebral hypothermia after cardiac arrest in dogs: a prospective, randomized study. Crit Care Med. (1993) 21:1348–58. doi: 10.1097/00003246-199309000-00019
4. Frohlich M, Hildebrand F, Weuster M, Mommsen P, Mohr J, Witte I, et al. Induced hypothermia reduces the hepatic inflammatory response in a swine multiple trauma model. J Trauma Acute Care Surg. (2014) 76:1425–32. doi: 10.1097/TA.0000000000000224
5. Garnacho-Castano MV, Alva N, Sanchez-Nuno S, Bardallo RG, Palomeque J, Carbonell T. Hypothermia can reverse hepatic oxidative stress damage induced by hypoxia in rats. J Physiol Biochem. (2016) 72:615–23. doi: 10.1007/s13105-016-0500-x
6. Kim K, Kim W, Rhee JE, Jo YH, Lee JH, Kim KS, et al. Induced hypothermia attenuates the acute lung injury in hemorrhagic shock. J Trauma. (2010) 68:373–81. doi: 10.1097/TA.0b013e3181a73eea
7. Lo TP Jr, Cho KS, Garg MS, Lynch MP, Marcillo AE, et al. Systemic hypothermia improves histological and functional outcome after cervical spinal cord contusion in rats. J Comp Neurol. (2009) 514:433–48. doi: 10.1002/cne.22014
8. Tissier R, Chenoune M, Ghaleh B, Cohen MV, Downey JM, Berdeaux A. The small chill: mild hypothermia for cardioprotection? Cardiovasc Res. (2010) 88:406–14. doi: 10.1093/cvr/cvq227
9. Engelman R, Baker RA, Likosky DS, Grigore A, Dickinson TA, Shore-Lesserson L, et al. The Society of Thoracic Surgeons, The Society of Cardiovascular Anesthesiologists, and The American Society of ExtraCorporeal Technology: clinical practice guidelines for cardiopulmonary bypass–temperature management during cardiopulmonary bypass. J Cardiothorac Vasc Anesth. (2015) 29:1104–13. doi: 10.1053/j.jvca.2015.07.011
10. Hypothermia after Cardiac Arrest Study Group. Mild therapeutic hypothermia to improve the neurologic outcome after cardiac arrest. N Engl J Med. (2002) 346:549–56. doi: 10.1056/NEJMoa012689
11. Bernard SA, Gray TW, Buist MD, Jones BM, Silvester W, Gutteridge G, et al. Treatment of comatose survivors of out-of-hospital cardiac arrest with induced hypothermia. N Engl J Med. (2002) 346:557–63. doi: 10.1056/NEJMoa003289
12. Post H, Schmitto JD, Steendijk P, Christoph J, Holland R, Wachter R, et al. Cardiac function during mild hypothermia in pigs: increased inotropy at the expense of diastolic dysfunction. Acta Physiol. (2010) 199:43–52. doi: 10.1111/j.1748-1716.2010.02083.x
13. Bergman R, Braber A, Adriaanse MA, van Vugt R, Tjan DH, van Zanten AR. Haemodynamic consequences of mild therapeutic hypothermia after cardiac arrest. Eur J Anaesthesiol. (2010) 27:383–7. doi: 10.1097/EJA.0b013e3283333a7d
14. Staer-Jensen H, Sunde K, Olasveengen TM, Jacobsen D, Draegni T, Nakstad ER, et al. Bradycardia during therapeutic hypothermia is associated with good neurologic outcome in comatose survivors of out-of-hospital cardiac arrest. Crit Care Med. (2014) 42:2401–8. doi: 10.1097/CCM.0000000000000515
15. Villablanca PA, Rao G, Briceno DF, Lombardo M, Ramakrishna H, Bortnick A, et al. Therapeutic hypothermia in ST elevation myocardial infarction: a systematic review and meta-analysis of randomised control trials. Heart. (2016) 102:712–9. doi: 10.1136/heartjnl-2015-308559
16. Ujhelyi MR, Sims JJ, Dubin SA, Vender J, Miller AW. Defibrillation energy requirements and electrical heterogeneity during total body hypothermia. Crit Care Med. (2001) 29:1006–11. doi: 10.1097/00003246-200105000-00025
17. Mattu A, Brady WJ, Perron AD. Electrocardiographic manifestations of hypothermia. Am J Emerg Med. (2002) 20:314–26. doi: 10.1053/ajem.2002.32633
18. Manninger M, Alogna A, Zweiker D, Zirngast B, Reiter S, Herbst V, et al. Mild hypothermia (33 degrees C) increases the inducibility of atrial fibrillation: An in vivo large animal model study. Pacing Clin Electrophysiol. (2018) 41:720–6. doi: 10.1111/pace.13351
19. Rolfast CL, Lust EJ, de Cock CC. Electrocardiographic changes in therapeutic hypothermia. Crit Care. (2012) 16:R100. doi: 10.1186/cc11369
20. Rankin AC, Rae AP. Cardiac arrhythmias during rewarming of patients with accidental hypothermia. Br Med J. (1984) 289:874–7. doi: 10.1136/bmj.289.6449.874
21. Okada M, Nishimura F, Yoshino H, Kimura M, Ogino T. The J wave in accidental hypothermia. J Electrocardiol. (1983) 16:23–8. doi: 10.1016/S0022-0736(83)80155-1
22. Noc M, Erlinge D, Neskovic AN, Kafedzic S, Merkely B, Zima E, et al. COOL AMI EU pilot trial: a multicentre, prospective, randomised controlled trial to assess cooling as an adjunctive therapy to percutaneous intervention in patients with acute myocardial infarction. EuroIntervention. (2017) 13:e531–9. doi: 10.4244/EIJ-D-17-00279
23. Thomsen JH, Hassager C, Erlinge D, Nielsen N, Horn J, Hovdenes J, et al. Atrial fibrillation following out-of-hospital cardiac arrest and targeted temperature management-are we giving it the attention it deserves? Crit Care Med. (2016) 44:2215–22. doi: 10.1097/CCM.0000000000001958
24. Erlinge D, Gotberg M, Noc M, Lang I, Holzer M, Clemmensen P, et al. Therapeutic hypothermia for the treatment of acute myocardial infarction-combined analysis of the RAPID MI-ICE and the CHILL-MI trials. Ther Hypothermia Temp Manag. (2015) 5:77–84. doi: 10.1089/ther.2015.0009
25. Kudlicka J, Mlcek M, Belohlavek J, Hala P, Lacko S, Janak D, et al. Inducibility of ventricular fibrillation during mild therapeutic hypothermia: electrophysiological study in a swine model. J Transl Med. (2015) 13:72. doi: 10.1186/s12967-015-0429-9
26. Polderman KH. Mechanisms of action, physiological effects, and complications of hypothermia. Crit Care Med. (2009) 37:S186–202. doi: 10.1097/CCM.0b013e3181aa5241
27. Suga H, Goto Y, Igarashi Y, Yasumura Y, Nozawa T, Futaki S, et al. Cardiac cooling increases Emax without affecting relation between O2 consumption and systolic pressure-volume area in dog left ventricle. Circ Res. (1988) 63:61–71. doi: 10.1161/01.RES.63.1.61
28. Fukunami M, Hearse DJ. The inotropic consequences of cooling: studies in the isolated rat heart. Heart Vessels. (1989) 5:1–9. doi: 10.1007/BF02058352
29. Monroe RG, Strang RH, Lafarge CG, Levy J. Ventricular performance, pressure-volume relationships, and o2 consumption during hypothermia. Am J Physiol. (1964) 206:67–73. doi: 10.1152/ajplegacy.1964.206.1.67
30. Nishimura Y, Naito Y, Nishioka T, Okamura Y. The effects of cardiac cooling under surface-induced hypothermia on the cardiac function in the in situ heart. Interact Cardiovasc Thorac Surg. (2005) 4:101–5. doi: 10.1510/icvts.2004.097188
31. Mikane T, Araki J, Suzuki S, Mizuno J, Shimizu J, Mohri S, et al. O(2) cost of contractility but not of mechanical energy increases with temperature in canine left ventricle. Am J Physiol. (1999) 277:H65–73. doi: 10.1152/ajpheart.1999.277.1.H65
32. Kerans V, Espinoza A, Skulstad H, Halvorsen PS, Edvardsen T, Bugge JF. Systolic left ventricular function is preserved during therapeutic hypothermia, also during increases in heart rate with impaired diastolic filling. Intensive Care Med Exp. (2015) 3:41. doi: 10.1186/s40635-015-0041-6
33. Bergmann SR, Angelakos ET, Torres JC. Salutary effects of moderate hypothermia on the circulatory and myocardial consequences of acute coronary occlusion in dogs. Cryobiology. (1985) 22:555–68. doi: 10.1016/0011-2240(85)90032-X
34. He X, Su F, Taccone FS, Maciel LK, Vincent JL. Cardiovascular and microvascular responses to mild hypothermia in an ovine model. Resuscitation. (2012) 83:760–6. doi: 10.1016/j.resuscitation.2011.11.031
35. Hodges GJ, Ferguson SAH, Cheung SS. Cardiac autonomic function during hypothermia and its measurement repeatability. Appl Physiol Nutr Metab. (2019) 44:31–6. doi: 10.1139/apnm-2018-0248
36. Rose JC, McDermott TF, Lilienfield LS, Porfido FA, Kelley RT. Cardiovascular function in hypothermic anesthetized man. Circulation. (1957) 15:512–7. doi: 10.1161/01.CIR.15.4.512
37. Fischer UM, Cox CS Jr, Laine GA, Mehlhorn U, Allen SJ. Mild hypothermia impairs left ventricular diastolic but not systolic function. J Invest Surg. (2005) 18:291–6. doi: 10.1080/08941930500327047
38. Schwarzl M, Steendijk P, Huber S, Truschnig-Wilders M, Obermayer-Pietsch B, Maechler H, et al. The induction of mild hypothermia improves systolic function of the resuscitated porcine heart at no further sympathetic activation. Acta Physiol. (2011) 203:409–18. doi: 10.1111/j.1748-1716.2011.02332.x
39. Filseth OM, How OJ, Kondratiev T, Gamst TM, Tveita T. Post-hypothermic cardiac left ventricular systolic dysfunction after rewarming in an intact pig model. Crit Care. (2010) 14:R211. doi: 10.1186/cc9334
40. Lewis ME, Al-Khalidi AH, Townend JN, Coote J, Bonser RS. The effects of hypothermia on human left ventricular contractile function during cardiac surgery. J Am Coll Cardiol. (2002) 39:102–8. doi: 10.1016/S0735-1097(01)01694-1
41. Schwarzl M, Huber S, Maechler H, Steendijk P, Seiler S, Truschnig-Wilders M, et al. Left ventricular diastolic dysfunction during acute myocardial infarction: effect of mild hypothermia. Resuscitation. (2012) 83:1503–10. doi: 10.1016/j.resuscitation.2012.05.011
42. Hale SL, Kloner RA. Myocardial temperature in acute myocardial infarction: protection with mild regional hypothermia. Am J Physiol. (1997) 273:H220–7. doi: 10.1152/ajpheart.1997.273.1.H220
43. Kuhn LA, Apter R, Weinrauch H, Jacobson TE, Meltzer L. Hemodynamic and Metabolic Effects of Skin and Blood-Stream Cooling in Experimental Myocardial Infarction with Shock. Am J Cardiol. (1963) 12:795–801. doi: 10.1016/0002-9149(63)90283-2
44. Simkhovich BZ, Hale SL, Kloner RA. Metabolic mechanism by which mild regional hypothermia preserves ischemic tissue. J Cardiovasc Pharmacol Ther. (2004) 9:83–90. doi: 10.1177/107424840400900203
45. Ning XH, Xu CS, Song YC, Xiao Y, Hu YJ, Lupinetti FM, et al. Temperature threshold and preservation of signaling for mitochondrial membrane proteins during ischemia in rabbit heart. Cryobiology. (1998) 36:321–9. doi: 10.1006/cryo.1998.2091
46. Mochizuki T, Yu S, Katoh T, Aoki K, Sato S. Cardioprotective effect of therapeutic hypothermia at 34 degrees C against ischaemia/reperfusion injury mediated by PI3K and nitric oxide in a rat isolated heart model. Resuscitation. (2012) 83:238–42. doi: 10.1016/j.resuscitation.2011.08.013
47. Shao ZH, Sharp WW, Wojcik KR, Li CQ, Han M, Chang WT, et al. Therapeutic hypothermia cardioprotection via Akt- and nitric oxide-mediated attenuation of mitochondrial oxidants. Am J Physiol Heart Circ Physiol. (2010) 298:H2164–73. doi: 10.1152/ajpheart.00994.2009
48. Olivecrona GK, Gotberg M, Harnek J, Van der Pals J, Erlinge D. Mild hypothermia reduces cardiac post-ischemic reactive hyperemia. BMC Cardiovasc Disord. (2007) 7:5. doi: 10.1186/1471-2261-7-5
49. Jacobshagen C, Pelster T, Pax A, Horn W, Schmidt-Schweda S, Unsold BW, et al. Effects of mild hypothermia on hemodynamics in cardiac arrest survivors and isolated failing human myocardium. Clin Res Cardiol. (2010) 99:267–76. doi: 10.1007/s00392-010-0113-2
50. Ning XH, Chi EY, Buroker NE, Chen SH, Xu CS, Tien YT, et al. Moderate hypothermia (30 degrees C) maintains myocardial integrity and modifies response of cell survival proteins after reperfusion. Am J Physiol Heart Circ Physiol. (2007) 293:H2119–28. doi: 10.1152/ajpheart.00123.2007
51. Fairley HB. Metabolism in hypothermia. Br Med Bull. (1961) 17:52–5. doi: 10.1093/oxfordjournals.bmb.a069865
52. Ziganshin BA, Elefteriades JA. Deep hypothermic circulatory arrest. Ann Cardiothorac Surg. (2013) 2:303–15. doi: 10.3978/j.issn.2225-319X.2013.01.05
53. Kirklin JW. Barratt-Boyes BG, editors. Hypothermia, circulatory arrest and cardiopulmonary bypass. In: Cardiac Surgery. New York, NY: Churchill Livingstone (1993). p. 61–127.
54. Ding D, Moskowitz SI, Li R, Lee SB, Esteban M, Tomaselli K, et al. Acidosis induces necrosis and apoptosis of cultured hippocampal neurons. Exp Neurol. (2000) 162:1–12. doi: 10.1006/exnr.2000.7226
55. Jones RN, Reimer KA, Hill ML, Jennings RB. Effect of hypothermia on changes in high-energy phosphate production and utilization in total ischemia. J Mol Cell Cardiol. (1982) 3:123–30. doi: 10.1016/0022-2828(82)90140-7
56. Hausenloy DJ, Yellon DM. Myocardial ischemia-reperfusion injury: a neglected therapeutic target. J Clin Invest. (2013) 123:92–100. doi: 10.1172/JCI62874
57. Ferrari R, Raddino R, Di Lisa F, Ceconi C, Curello S, Albertini A, et al. Effects of temperature on myocardial calcium homeostasis and mitochondrial function during ischemia and reperfusion. J Thorac Cardiovasc Surg. (1990) 99:919–28. doi: 10.1016/S0022-5223(19)36910-7
58. Inoue K, Ando S, Gyuan F, Takaba T. A study of the myocardial protective effect of rapid cooling based on intracellular Ca, intracellular pH, and HSP70. Ann Thorac Cardiovasc Surg. (2003) 9:301–6.
59. Xu L, Yenari MA, Steinberg GK, Giffard RG. Mild hypothermia reduces apoptosis of mouse neurons in vitro early in the cascade. J Cereb Blood Flow Metab. (2002) 22:21–8. doi: 10.1097/00004647-200201000-00003
60. Adachi M, Sohma O, Tsuneishi S, Takada S, Nakamura H. Combination effect of systemic hypothermia and caspase inhibitor administration against hypoxic-ischemic brain damage in neonatal rats. Pediatr Res. (2001) 50:590–5. doi: 10.1203/00006450-200111000-00010
61. Krech J, Tong G, Wowro S, Walker C, Rosenthal LM, Berger F, et al. Moderate therapeutic hypothermia induces multimodal protective effects in oxygen-glucose deprivation/reperfusion injured cardiomyocytes. Mitochondrion. (2017) 35:1–10. doi: 10.1016/j.mito.2017.04.001
62. Shao ZH, Chang WT, Chan KC, Wojcik KR, Hsu CW, Li CQ, et al. Hypothermia-induced cardioprotection using extended ischemia and early reperfusion cooling. Am J Physiol Heart Circ Physiol. (2007) 292:H1995–2003. doi: 10.1152/ajpheart.01312.2005
63. Ning XH, Chen SH, Xu CS, Li L, Yao LY, Qian K, et al. Hypothermic protection of the ischemic heart via alterations in apoptotic pathways as assessed by gene array analysis. J Appl Physiol. (2002) 92:2200–7. doi: 10.1152/japplphysiol.01035.2001
64. Otake H, Shite J, Paredes OL, Shinke T, Yoshikawa R, Tanino Y, et al. Catheter-based transcoronary myocardial hypothermia attenuates arrhythmia and myocardial necrosis in pigs with acute myocardial infarction. J Am Coll Cardiol. (2007) 49:250–60. doi: 10.1016/j.jacc.2006.06.080
65. Ostadal P, Mlcek M, Kruger A, Horakova S, Skabradova M, Holy F, et al. Mild therapeutic hypothermia is superior to controlled normothermia for the maintenance of blood pressure and cerebral oxygenation, prevention of organ damage and suppression of oxidative stress after cardiac arrest in a porcine model. J Transl Med. (2013) 11:124. doi: 10.1186/1479-5876-11-124
66. Dash R, Mitsutake Y, Pyun WB, Dawoud F, Lyons J, Tachibana A, et al. Dose-dependent cardioprotection of moderate (32 degrees C) versus mild (35 degrees C) therapeutic hypothermia in porcine acute myocardial infarction. JACC Cardiovasc Interv. (2018) 11:195–205. doi: 10.1016/j.jcin.2017.08.056
67. Kimura A, Sakurada S, Ohkuni H, Todome Y, Kurata K. Moderate hypothermia delays proinflammatory cytokine production of human peripheral blood mononuclear cells. Crit Care Med. (2002) 30:1499–502. doi: 10.1097/00003246-200207000-00017
68. Yang X, Liu Y, Yang XM, Hu F, Cui L, Swingle MR, et al. Cardioprotection by mild hypothermia during ischemia involves preservation of ERK activity. Basic Res Cardiol. (2011) 106:421–30. doi: 10.1007/s00395-011-0165-0
69. Hale SL, Dave RH, Kloner RA. Regional hypothermia reduces myocardial necrosis even when instituted after the onset of ischemia. Basic Res Cardiol. (1997) 92:351–7. doi: 10.1007/BF00788947
70. Miki T, Liu GS, Cohen MV, Downey JM. Mild hypothermia reduces infarct size in the beating rabbit heart: a practical intervention for acute myocardial infarction? Basic Res Cardiol. (1998) 93:372–83. doi: 10.1007/s003950050105
71. Hale SL, Herring MJ, Kloner RA. Delayed treatment with hypothermia protects against the no-reflow phenomenon despite failure to reduce infarct size. J Am Heart Assoc. (2013) 2:e004234. doi: 10.1161/JAHA.112.004234
72. Hale SL, Dae MW, Kloner RA. Hypothermia during reperfusion limits 'no-reflow' injury in a rabbit model of acute myocardial infarction. Cardiovasc Res. (2003) 59:715–22. doi: 10.1016/S0008-6363(03)00456-5
73. Gotberg M, Olivecrona GK, Engblom H, Ugander M, van der Pals J, Heiberg E, et al. Rapid short-duration hypothermia with cold saline and endovascular cooling before reperfusion reduces microvascular obstruction and myocardial infarct size. BMC Cardiovasc Disord. (2008) 8:7. doi: 10.1186/1471-2261-8-7
74. Erlinge D, Gotberg M, Lang I, Holzer M, Noc M, Clemmensen P, et al. Rapid endovascular catheter core cooling combined with cold saline as an adjunct to percutaneous coronary intervention for the treatment of acute myocardial infarction the CHILL-MI trial: a randomized controlled study of the use of central venous catheter core cooling combined with cold saline as an adjunct to percutaneous coronary intervention for the treatment of acute myocardial infarction. J Am Coll Cardiol. (2014) 63:1857–65. doi: 10.1016/j.jacc.2013.12.027
75. Testori C, Beitzke D, Mangold A, Sterz F, Loewe C, Weiser C, et al. Out-of-hospital initiation of hypothermia in ST-segment elevation myocardial infarction: a randomised trial. Heart. (2019) 105:531–7. doi: 10.1136/heartjnl-2018-313705
76. Baraka A. Influence of surface cooling and rewarming on whole-body oxygen supply-demand balance. Br J Anaesth. (1994) 73:418–20. doi: 10.1093/bja/73.3.418
77. Moriyama Y, Iguro Y, Shimokawa S, Saigenji H, Toyohira H, Taira A. Successful application of hypothermia combined with intra-aortic balloon pump support to low-cardiac-output state after open heart surgery. Angiology. (1996) 47:595–9. doi: 10.1177/000331979604700608
78. Yahagi N, Kumon K, Watanabe Y, Tanigami H, Haruna M, Hayashi H, et al. Value of mild hypothermia in patients who have severe circulatory insufficiency even after intra-aortic balloon pump. J Clin Anesth. (1998) 10:120–5. doi: 10.1016/S0952-8180(97)00255-9
79. Bro-Jeppesen J, Annborn M, Hassager C, Wise MP, Pelosi P, Nielsen N, et al. Hemodynamics and vasopressor support during targeted temperature management at 33 degrees C Versus 36 degrees C after out-of-hospital cardiac arrest: a post hoc study of the target temperature management trial*. Crit Care Med. (2015) 43:318–27. doi: 10.1097/CCM.0000000000000691
80. Furnau G, Beck J, Desch S, Eitel I, Jung C, Erbs S, et al. Mild hypothermia in cardiogenic shock complicating myocardial infarction - the randomized SHOCK-COOL pilot trial. Wien Klin Wochenschr. (2017) 129:S94. doi: 10.1016/S0735-1097(17)34572-2
81. Maeng M, Mortensen UM, Kristensen J, Kristiansen SB, Andersen HR. Hypothermia during reperfusion does not reduce myocardial infarct size in pigs. Basic Res Cardiol. (2006) 101:61–8. doi: 10.1007/s00395-005-0550-7
82. Hale SL, Kloner RA. Ischemic preconditioning and myocardial hypothermia in rabbits with prolonged coronary artery occlusion. Am J Physiol. (1999) 276:H2029–34. doi: 10.1152/ajpheart.1999.276.6.H2029
83. Dave RH, Hale SL, Kloner RA. Hypothermic, closed circuit pericardioperfusion: a potential cardioprotective technique in acute regional ischemia. J Am Coll Cardiol. (1998) 31:1667–71. doi: 10.1016/S0735-1097(98)00129-6
84. Kanemoto S, Matsubara M, Noma M, Leshnower BG, Parish LM, Jackson BM, et al. Mild hypothermia to limit myocardial ischemia-reperfusion injury: importance of timing. Ann Thorac Surg. (2009) 87:157–63. doi: 10.1016/j.athoracsur.2008.08.012
85. Tissier R, Hamanaka K, Kuno A, Parker JC, Cohen MV, Downey JM. Total liquid ventilation provides ultra-fast cardioprotective cooling. J Am Coll Cardiol. (2007) 49:601–5. doi: 10.1016/j.jacc.2006.09.041
86. Tissier R, Couvreur N, Ghaleh B, Bruneval P, Lidouren F, Morin D, et al. Rapid cooling preserves the ischaemic myocardium against mitochondrial damage and left ventricular dysfunction. Cardiovasc Res. (2009) 83:345–53. doi: 10.1093/cvr/cvp046
87. Duncker DJ, Klassen CL, Ishibashi Y, Herrlinger SH, Pavek TJ, Bache RJ. Effect of temperature on myocardial infarction in swine. Am J Physiol. (1996) 270:H1189–99. doi: 10.1152/ajpheart.1996.270.4.H1189
88. Yannopoulos D, Zviman M, Castro V, Kolandaivelu A, Ranjan R, Wilson RF, et al. Intra-cardiopulmonary resuscitation hypothermia with and without volume loading in an ischemic model of cardiac arrest. Circulation. (2009) 120:1426–35. doi: 10.1161/CIRCULATIONAHA.109.848424
89. Gotberg M, van der Pals J, Gotberg M, Olivecrona GK, Kanski M, Koul S, et al. Optimal timing of hypothermia in relation to myocardial reperfusion. Basic Res Cardiol. (2011) 106:697–708. doi: 10.1007/s00395-011-0195-7
90. Dae M, O'Neill W, Grines C, Dixon S, Erlinge D, Noc M, et al. Effects of endovascular cooling on infarct size in ST-segment elevation myocardial infarction: A patient-level pooled analysis from randomized trials. J Interv Cardiol. (2018) 31:269–76. doi: 10.1111/joic.12485
91. Abendschein DR, Tacker WA Jr, Babbs CF. Protection of ischemic myocardium by whole-body hypothermia after coronary artery occlusion in dogs. Am Heart J. (1978) 96:772–80. doi: 10.1016/0002-8703(78)90010-8
92. Schwartz DS, Bremner RM, Baker CJ, Uppal KM, Barr ML, Cohen RG, et al. Regional topical hypothermia of the beating heart: preservation of function and tissue. Ann Thorac Surg. (2001) 72:804–9. doi: 10.1016/S0003-4975(01)02822-3
93. Haendchen RV, Corday E, Meerbaum S, Povzhitkov M, Rit J, Fishbein MC. Prevention of ischemic injury and early reperfusion derangements by hypothermic retroperfusion. J Am Coll Cardiol. (1983) 1:1067–80. doi: 10.1016/S0735-1097(83)80109-0
94. Wakida Y, Haendchen RV, Kobayashi S, Nordlander R, Corday E. Percutaneous cooling of ischemic myocardium by hypothermic retroperfusion of autologous arterial blood: effects on regional myocardial temperature distribution and infarct size. J Am Coll Cardiol. (1991) 18:293–300. doi: 10.1016/S0735-1097(10)80251-7
95. Meerbaum S, Haendchen RV, Corday E, Povzhitkov M, Fishbein MC, J YR, et al. Hypothermic coronary venous phased retroperfusion: a closed-chest treatment of acute regional myocardial ischemia. Circulation. (1982) 65:1435–45. doi: 10.1161/01.CIR.65.7.1435
96. Hamamoto H, Sakamoto H, Leshnower BG, Parish LM, Kanemoto S, Hinmon R, et al. Very mild hypothermia during ischemia and reperfusion improves postinfarction ventricular remodeling. Ann Thorac Surg. (2009) 87:172–7. doi: 10.1016/j.athoracsur.2008.08.015
97. Dae MW, Gao DW, Sessler DI, Chair K, Stillson CA. Effect of endovascular cooling on myocardial temperature, infarct size, and cardiac output in human-sized pigs. Am J Physiol Heart Circ Physiol. (2002) 282:H1584–91. doi: 10.1152/ajpheart.00980.2001
98. Dixon SR, Whitbourn RJ, Dae MW, Grube E, Sherman W, Schaer GL, et al. Induction of mild systemic hypothermia with endovascular cooling during primary percutaneous coronary intervention for acute myocardial infarction. J Am Coll Cardiol. (2002) 40:1928–34. doi: 10.1016/S0735-1097(02)02567-6
99. Otterspoor LC, Van 't Veer M, van Nunen LX, Brueren GRG, Tonino PAL, Wijnbergen IF, et al. Safety and feasibility of selective intracoronary hypothermia in acute myocardial infarction. Eurointervention. (2017) 13:E1475–82. doi: 10.4244/EIJ-D-17-00240
100. Gotberg M, Olivecrona GK, Koul S, Carlsson M, Engblom H, Ugander M, et al. A pilot study of rapid cooling by cold saline and endovascular cooling before reperfusion in patients with ST-elevation myocardial infarction. Circ-Cardiovasc Inte. (2010) 3:400–7. doi: 10.1161/CIRCINTERVENTIONS.110.957902
101. Ly HQ, Denault A, Dupuis J, Vadeboncoeur A, Harel F, Arsenault A, et al. A pilot study: the noninvasive surface cooling thermoregulatory system for mild hypothermia induction in acute myocardial infarction (The NICAMI study). Am Heart J. (2005) 150:933. doi: 10.1016/j.ahj.2005.02.049
102. Kandzari DE, Chu A, Brodie BR, Stuckey TA, Hermiller JB, Vetrovec GW, et al. Feasibility of endovascular cooling as an adjunct to primary percutaneous coronary intervention (results of the LOWTEMP pilot study). Am J Cardiol. (2004) 93:636–9. doi: 10.1016/j.amjcard.2003.11.038
103. Nichol G, Strickland W, Shavelle D, Maehara A, Ben-Yehuda O, Genereux P, et al. Multicenter, randomized, controlled pilot trial of peritoneal hypothermia in patients with st-segment-elevation myocardial infarction. Circ Cardiovasc Inter. (2015) 8:e001965. doi: 10.1161/CIRCINTERVENTIONS.114.001965
104. Testori C, Sterz F, Delle-Karth G, Malzer R, Holzer M, Stratil P, et al. Strategic target temperature management in myocardial infarction-a feasibility trial. Heart. (2013) 99:1663–7. doi: 10.1136/heartjnl-2013-304624
105. Koreny M, Sterz F, Uray T, Schreiber W, Holzer M, Laggner A, et al. Effect of cooling after human cardiac arrest on myocardial infarct size. Resuscitation. (2009) 80:56–60. doi: 10.1016/j.resuscitation.2008.08.019
106. O'Neill WW, Dixon SR. The year in interventional cardiology. J Am Coll Cardiol. (2004) 43:875–90. doi: 10.1016/j.jacc.2003.12.024
107. Wolfrum S, Pierau C, Radke PW, Schunkert H, Kurowski V. Mild therapeutic hypothermia in patients after out-of-hospital cardiac arrest due to acute ST-segment elevation myocardial infarction undergoing immediate percutaneous coronary intervention. Crit Care Med. (2008) 36:1780–6. doi: 10.1097/CCM.0b013e31817437ca
108. Schefold JC, Storm C, Joerres A, Hasper D. Mild therapeutic hypothermia after cardiac arrest and the risk of bleeding in patients with acute myocardial infarction. Int J Cardiol. (2009) 132:387–91. doi: 10.1016/j.ijcard.2007.12.008
109. Knafelj R, Radsel P, Ploj T, Noc M. Primary percutaneous coronary intervention and mild induced hypothermia in comatose survivors of ventricular fibrillation with ST-elevation acute myocardial infarction. Resuscitation. (2007) 74:227–34. doi: 10.1016/j.resuscitation.2007.01.016
110. Cheng C, Matsukawa T, Sessler DI, Ozaki M, Kurz A, Merrifield B, et al. Increasing mean skin temperature linearly reduces the core-temperature thresholds for vasoconstriction and shivering in humans. Anesthesiology. (1995) 82:1160–8. doi: 10.1097/00000542-199505000-00011
111. Lopez M, Sessler DI, Walter K, Emerick T, Ozaki M. Rate and gender dependence of the sweating, vasoconstriction, and shivering thresholds in humans. Anesthesiology. (1994) 80:780–8. doi: 10.1097/00000542-199404000-00009
112. Otterspoor LC, van Nunen LX, Rosalina TT, Veer MV, Tuijl SV, Stijnen M, et al. Intracoronary hypothermia for acute myocardial infarction in the isolated beating pig heart. Am J Transl Res. (2017) 9:558–68.
113. European Intracoronary Cooling Evaluation in Patients With ST-Elevation Myocardial Infarction. Available online at: https://ClinicalTrials.gov/show/NCT03447834 (accessed January 15, 2021).
114. STEMI Cool Pilot Trial to Assess Cooling as an Adjunctive Therapy to PCI in Patients With Acute MI (Phase A). Available online at: https://ClinicalTrials.gov/show/NCT03361995 (accessed January 15, 2021).
115. Hoedemaekers CW, Ezzahti M, Gerritsen A, van der Hoeven JG. Comparison of cooling methods to induce and maintain normo- and hypothermia in intensive care unit patients: a prospective intervention study. Crit Care. (2007) 11:R91. doi: 10.1186/cc6104
116. Kapur NK, Alkhouli MA, DeMartini TJ, Faraz H, George ZH, Goodwin MJ, et al. Unloading the left ventricle before reperfusion in patients with anterior st-segment-elevation myocardial infarction. Circulation. (2019) 139:337–46. doi: 10.1161/CIRCULATIONAHA.118.038269
Keywords: hypothermia, myocardial infarction, infarct size, endovascular, physiology, cardiac function, clinical trial, preclinical
Citation: Yamada KP, Kariya T, Aikawa T and Ishikawa K (2021) Effects of Therapeutic Hypothermia on Normal and Ischemic Heart. Front. Cardiovasc. Med. 8:642843. doi: 10.3389/fcvm.2021.642843
Received: 16 December 2020; Accepted: 21 January 2021;
Published: 15 February 2021.
Edited by:
Sarawut Kumphune, Chiang Mai University, ThailandReviewed by:
Christoph Testori, Medical University of Vienna, AustriaCopyright © 2021 Yamada, Kariya, Aikawa and Ishikawa. This is an open-access article distributed under the terms of the Creative Commons Attribution License (CC BY). The use, distribution or reproduction in other forums is permitted, provided the original author(s) and the copyright owner(s) are credited and that the original publication in this journal is cited, in accordance with accepted academic practice. No use, distribution or reproduction is permitted which does not comply with these terms.
*Correspondence: Kiyotake Ishikawa, a2l5b3Rha2UuaXNoaWthd2FAbXNzbS5lZHU=
Disclaimer: All claims expressed in this article are solely those of the authors and do not necessarily represent those of their affiliated organizations, or those of the publisher, the editors and the reviewers. Any product that may be evaluated in this article or claim that may be made by its manufacturer is not guaranteed or endorsed by the publisher.
Research integrity at Frontiers
Learn more about the work of our research integrity team to safeguard the quality of each article we publish.