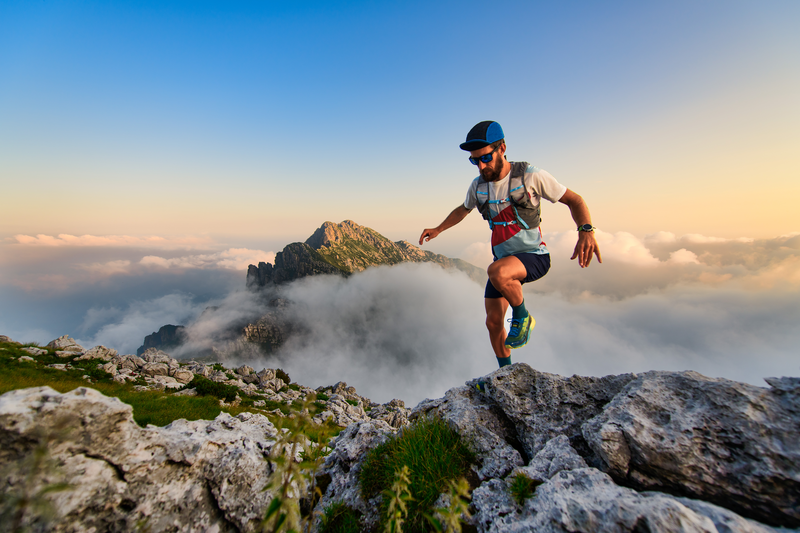
94% of researchers rate our articles as excellent or good
Learn more about the work of our research integrity team to safeguard the quality of each article we publish.
Find out more
REVIEW article
Front. Cardiovasc. Med. , 11 June 2021
Sec. Structural Interventional Cardiology
Volume 8 - 2021 | https://doi.org/10.3389/fcvm.2021.639058
This article is part of the Research Topic Current Devices in Mitral Valve Replacement and their Potential Complications View all 6 articles
Mitral regurgitation is the most common valvular lesion in the developed world, with increasing prevalence, morbidity, and mortality. The experience with surgical mitral valve repair or replacement is very well-validated. However, more than 45% of these patients get denied surgery due to an elevated risk profile and advanced disease of the left ventricle at the time of presentation, promoting the need for less invasive transcatheter options such as transcatheter repair and transcatheter mitral valve replacement (TMVR). Early available TMVR studies have shown promising results, and several dedicated devices are under clinical evaluation. However, TMVR is still in the early developmental stages and is associated with a non-negligible risk of periprocedural and post-procedural complications. In this review, we discuss the current challenges facing TMVR and the potential TMVR-related complications, offering an overview on the measures implemented to mitigate these complications, and future implications.
Mitral regurgitation (MR) is the most common valvular disease in the developed world and is associated with high morbidity and mortality. The prevalence of MR increases with age, reaching up to 10% in individuals over the age of 75 (1, 2). Surgical mitral valve repair (MVr) or replacement has been a very well-established therapeutic option (3, 4). However, due to the high risk associated with surgical interventions, and the level of advanced disease in this patient population, more than 49% of MR patients get rejected from surgical mitral valve replacement (5, 6). This creates an unmet clinical need and a push for novel less invasive percutaneous mitral valve treatment alternatives—whether repair or replacement—with less periprocedural mortality and good clinical outcomes (7, 8).
Transcatheter MVr is a well-established treatment strategy for MR (primary and secondary MR), with more than 100,000 transcatheter MVr cases performed to date worldwide (9). However, transcatheter MVr has technical challenges and limitations: MR reduction is not always guaranteed, suboptimal anatomies limit patient suitability, limited percutaneous options if MR recurs, a single repair device runs the risk of being inadequate, and many cases may require more than one device to achieve surgical-like repair results (10, 11). Therefore, to accommodate the unmet needs of this patient's population, transcatheter mitral valve replacement (TMVR) has emerged as a promising intervention that can help reduce MR in non-surgical patients and in those with unsuitable anatomy for transcatheter edge-to-edge repair. Moreover, TMVR represents a new treatment option for inoperable or high-risk patients with degenerated or failed bioprostheses or failed repairs [valve-in-valve (ViV) or valve-in-ring (ViR), or in patients with severe annular calcifications, valve-in-mitral annular calcification (ViMAC)] (12). Despite the technological advancements in the field of structural heart disease, TMVR is still being performed in very high-risk surgical patients, restricted to high-volume experienced centers, and with a relevant risk of periprocedural and post-procedural complications. In one study examining outcomes of 203 patients with severe MR who have been excluded from the available TMVR protocols, more than 88% of patients were rejected from the early feasibility studies of TMVR due to advanced frailty, with mortality reaching up to 12% in those who were ineligible. That same study found that frailty (15%), severe tricuspid regurgitation (TR) (14%), prior aortic valve therapy (13), mitral anatomical exclusion (16%), severe MAC (7%), and risk of left ventricular outflow tract (LVOT) obstruction (LVOTO; 4%) are the most common causes of TMVR exclusion (6). This review offers a brief insight on the current challenges, potential complications of TMVR, and the measures available to mitigate these complications.
The mitral valve apparatus is a complex structure, consisting of highly dynamic annulus, the two leaflets (anterior and posterior), chordae tendinae, and papillary muscles (14). Moreover, the advanced disease of the left ventricle (LV) at the time of presentation due to different etiologies can limit the available transcatheter options and patient's eligibility. One advantage of surgical MV intervention is that it can be tailored to target the specific pathology of the valvular apparatus. However, today with the detailed pre-procedural planning and with the availability of several transcatheter devices, a targeted pathology approach could be achieved with transcatheter interventions (13). Moreover, the saddle asymmetrical shape of the non-stiff mitral valve annulus and its anatomical relation to the insertion of the papillary muscles make the task to design a transcatheter heart valve (THV) for the treatment of MR incredibly challenging. The ideal THV should match the native annulus without running the risk of delayed device detachment, paravalvular leak (PVL), or MR recurrence (15).
TMVR complications can be divided based on either the time of occurrence (procedural vs. post-procedural) or complications related to the device or to its pathway (16, 17). For the purpose of simplicity and due to the different risk profiles, we will divide the complications into procedural and post-procedural (Table 1), stratified by TMVR in native valve, ViV, ViR, and ViMAC. The first TMVR case series was performed in a failed prosthesis as ViV; all patients received inverted Edwards Life Sciences Sapien XT (Edwards Lifesciences, Irvine, CA) valve through the transapical (TA) approach (18). This was followed by TMVR in native valve (19). Early feasibility TMVR in native valve studies with relatively small sample sizes tested the safety and efficacy of these new THVs are summarized in Table 2 (20–28). Whereas Table 3 reports data on ViV and ViR and Table 4 reports data on VinMac procedures (16, 21, 22, 29–31, 33–43, 45–47). The early experience from early feasibility studies and registries showed that the anatomical nature and complexity of the mitral valve impose unique complications that are summarized in Table 1.
Prosthetic valve embolization has not been reported in the surgical literature, and it has been described as a unique complication of transcatheter valves (48). The friction between the frame of the transcatheter prosthetic mitral valve and the surrounding tissue generates the anchoring force of the THV. Therefore, deployment in suboptimal position could decrease this force, leading to malposition or migration. In the TMVR case series of 23 consecutive patients undergoing mitral ViV by Cheung et al. (18), one patient was readmitted with heart failure, and echocardiography showed 5-mm valve migration to the left atrium with severe intervalvular regurgitation that required a second uneventful TA TMVR. In the ViMAC study by Guerrero et al. (16, 49), six patients had migration of the implanted device and five patients with TVE required a second THV. Bapat et al. (50) reported two cases of device migration after successful THV implantation and delayed presentation of recurrent severe MR on echocardiography within 1 week and 3 months. Both cases were treated with open surgical mitral valve replacement. Upon further study of the explanted bioprosthesis, the authors hypothesized that delayed migration occurred due to the elevated closing pressure of the LV that the device must cope with, THV undersizing, and the lack of extensive calcification of the mitral leaflets (50).
The treatment of valve migration or embolization can be performed by transcatheter snaring, re-do transcatheter ViV, or open-heart surgery. Choosing the right option of treatment depends on the severity of MR, the urgency of treatment, the migrated valve position, and the patient's surgical risk profile (51).
Severe LVOTO is a life-threatening complication of TMVR. The native LVOT is confined by the most basal septum, intervalvular fibrosa (aortomitral tissue), and the basal portion of the anterior mitral leaflet (AML). AML sequestration by the newly implanted THV can lead to elongation of the LVOT, determining what is known today as the neo-LVOT (25, 52). The risk of LVOTO can be predicted on pre-procedural multidetector cardiac tomography (MDCT), which can help inform the optimal depth of device implantation and the need for further intervention by predicting the neo-LVOT area. A decrease in neo-LVOT area is a risk factor for LVOTO, which can manifest as immediate hemodynamic instability after THV deployment, with intra-procedural echocardiographic evidence of valve displacement or AML sequestration leading to LVOTO, and LVOT gradient >10 mmHg than baseline (53). Factors that are taken into consideration when analyzing pre-procedural imaging are the AML length, neo-LVOT area <200 mm2, device-related dimensions, aortomitral angle, and basal septal bulge. Three-dimensional (3D) prototyping of available cardiac CT images can be used to predict the risk of LVOTO and enhance procedural outcome (54). In one study evaluating eight patients who underwent TMVR, when compared to post-procedural imaging, 3D printed models were able to predict LVOTO in two out of the five printed models (55, 56). In an analysis of approximately 200 cases of the TMVR international multicenter registry, the prevalence of LVOTO was 13%, with the highest rate in ViMAC, then in ViR and ViV (54, 8, and 2%, respectively). Moreover, the authors showed that the estimated neo-LVOT area (measured during mid-end systole on MDCT) was inversely related to LVOT gradient and significantly correlated with the actual neo-LVOT area after THV deployment. Other predictors of LVOTO were distance of the mitral annulus to the interventricular septum and left ventricular end diastolic diameter. Patients with LVOTO had higher rates of procedural adverse events and related deaths (35 vs. 2%, P < 0.001) (57). In patients with LVOTO (N = 26), 11% were managed medically, 19% underwent emergent open-heart surgery, 8% underwent emergent TAVR, while alcohol septal ablation (ASA) was performed in 31% of patients.
In the ViMAC registry by Guerrero et al. (16), LVOTO was associated with all-cause mortality. LVOTO happens in mid-late systole, and it is at the end of systole when the LVOT is at its smallest diameter (45% of the cardiac cycle on MDCT) (57). However, in a retrospective analysis using a novel approach of physiologic early systolic assessment of the dynamic LVOT, the authors found that measuring LVOT in end systole may overestimate the risk of LVOTO and could increase the rate of screen failure due to non-anatomical conditions. Moreover, they proposed a novel multiphase physiological evaluation of the LVOT, which leads to an increase in their TMVR patients' eligibility by more than 50%, and no cases of LVOTO at 30-days follow-up (58). These observations are indicators of the learning curve and improvement of the groundwork for the TMVR procedure and its complications. The high prevalence of this complication and its association with mortality lead to a reassessment of the steps followed in the evaluation and pre-procedural planning of TMVR. Moreover, implementation of multiple imaging modalities to help predict LVOTO and intra-procedural bailout strategies have been described (59–62). Pre-TMVR screening remains an evolving field with data from ongoing registries continuing to contribute to our understanding and learning.
Intra-procedural transesophageal echocardiogram (TEE) with 3D imaging is key to identify the MV relationship to adjacent structures, which can help improve procedural outcomes and lower the risk of LVOTO (56).
AML laceration is a well-established treatment of systolic anterior motion (SAM) and LVOTO in surgical MV replacement. However, it has been implemented in TMVR to a lesser extent, with few reported cases of LVOTO that lead to conversion to open-heart surgery with controlled cardiac arrest and successful resection of the AML on bypass (63, 64). In these cases, AML resection was a successful bailout option for LVOTO.
LVOTO happens mainly due to AML deflection toward the septum, and it can be predicted by measuring the neo-LVOT area or by other anatomical predictors such as acute aortomitral angulation, prominent septal bulge, long AML, and redundant mitral chordae (52, 57, 61, 65). Greenbaum et al. (66) presented case vignettes of LVOTO in support of the removal or reduction of the AML, a technique that has been described in the surgical literature. This led to the development of Laceration of the Anterior Mitral Leaflet to Prevent Outflow ObstructioN (LAMPOON). LAMPOON, the intentional electrosurgical laceration of the AML to prevent LVOTO (67, 68), a challenging procedure, which modified the available surgical approach and has been used successfully before TMVR with the Sapien THV or with dedicated devices designed for the mitral valve, in which successful outcome of patent LVOT was confirmed by measuring LVOT gradient (by means of both echocardiography and catheterization). A National Institutes of Health (NIH)-sponsored trial is ongoing to test the safety and efficacy of LAMPOON in TMVR.
Exaggerated basal septal bulge is a risk factor for LVOTO and has been the target of ASA to lower the associated risk. The early cases of ASA in TMVR were performed as a bailout intervention after LVOTO; in these reported cases, patients survived and were hemodynamically stable after the procedure (59, 69, 70). In another study, ASA was performed as a precautionary measure in patients who were identified to be at high risk of LVOTO. Thereafter, ASA has emerged as an intervention to lower the risk of LVOTO by increasing the neo-LVOT surface area by at least 111.2 mm2 (interquartile range: 71.4–193.1 mm2) and eliminating the exaggerated septal bulge (59). Other techniques such as kissing balloon inflation, medical therapy with aggressive intravenous hydration, and transatrial resection of the AML have been implemented as bailout strategies in LVOTO. However, when outcomes of all the available techniques were compared in the multicenter TMVR registry, survival was achieved only in those who were treated with ASA (33). In the ViMAC study by Guerrero et al. (16), the prevalence of LVOTO was 12% (N = 13), with five of the 13 patients treated with medical treatment, one treated with kissing balloon inflation, one treated with surgical intervention, and six patients treated with ASA; among all 13 patients, only two of those who received ASA were alive at 1 year.
Transatrial TMVR approach with AML resection on cardiac bypass has emerged as an alternative option, especially in patients who are identified at high risk of LVOTO. Moreover, transatrial resection has been employed as a bailout procedure in some of the cases that needed conversion to open-heart surgery and immediate hemodynamic stabilization (16). Praz et al. (22) and Kassar et al. (71) described their experience with 26 consecutive patients with an average STS risk score of 9.4% and 30-days mortality of 27%. In this series, the rate of new-onset atrial fibrillation after the procedure was at 27%. In another single-center case series of six patients who underwent transatrial TMVR for mitral stenosis, 30-days mortality was more than 50% due to severe PVL or device migration (32). In a multicenter study of 21 patients who underwent transatrial TMVR, the authors proposed new techniques of mitral annulus analysis that showed promising transatrial procedural success in patients with severe MAC (72).
For a successful implantation of THV in TMVR, it is mandatory to achieve robust anchoring of the prosthesis and to overcome the loading force of the left ventricle (14), which is easier through the TA approach compared to the TF approach because of shorter path and coaxiality to the MV (73). Data from the mitral VIVID study showed that most devices were delivered through TA access (79%) (47). In fact, most available dedicated TMVR devices are delivered through TA approach, except for CardiaQ (now Evoque), Cardiovalve, Cephea, and Caisson valves, which are delivered transseptally through TF access. As has already been demonstrated in several TAVR studies, TF is favored over TA, since it is less invasive, associated with less complications, and can be performed under moderate sedation (74–77). Vascular access-related complications can still be seen with TF access. Another complication of the transseptal approach is iatrogenic laceration of the left atrial septum during balloon septostomy or post-procedural expansion of the iatrogenic atrial septal defect (iASD). Therefore, extra caution is mandated when performing balloon sizing of the atrial septum. Data are scarce regarding the outcome of iASD post-procedure and whether it needs to be occluded post-procedure. However, most of the studies point to the fact that most iASDs close at 1 year of follow-up and no correlation with symptoms at 12 months (78, 79). Whereas, TA can be associated with major bleeding, LV apex pseudoaneurysm, and subsequent fibrosis due to sheath positioning. In studies comparing echocardiographic outcomes between TA and TF during TAVR, LVEF recovery and longitudinal strain at follow-up were reduced in the TA group (77). A recent study evaluating mitral ViV TMVR outcomes in a large cohort of 1,529 patients found that the transseptal approach associated with lower 1-year all-cause mortality at 1-year follow-up (16 vs. 22%, P = 0.03) (80). In a case series of TMVR comparing TF vs. TA access, there was no difference in procedural duration between the two accesses. However, only TF was associated with an increase in cardiac output and improved survival when compared to TA access (73). Although the Tendyne valve is delivered through TA, data from the first 100 patients showed a procedural success rate of 96% with no intra-procedural mortality (25). Moreover, in a subanalysis of 36 patients who received Tendyne, cardiac CT analysis performed at 1 month post-intervention showed left ventricular end diastolic volumes reverse remodeling. In fact, the authors of this study found that the closer the position of the Tendyne apical pad to the true apex, the more left ventricular remodeling (81). Eleid et al. (44) stratified their TMVR group by early cases of TMVR vs. subsequent cases of TMVR performed after certain modifications applied to TA access led to lower rates of LV perforation and bleeding. However, currently available TMVR devices are mostly delivered through TA access; a significant improvement in the TMVR field will be the implementation and clinical validation of new dedicated TF devices, aiming to minimize access-related complications and to simplify the procedure.
LV perforation is a rare and fatal complication of MV interventions. In the TMVR studies, LV perforation has been observed especially in the early cases; it is usually related to TA access or directly due to stiff instrumentation when trying to cross the valve, but its rate did not exceed 1% in most studies (49). Moreover, patients at higher risk of LV perforation in transcatheter valvular interventions can be identified on pre-procedural MDCT. For example, in one retrospective study of LV perforations in TAVR, anatomical factors such as small left ventricular cavity, hyperdynamic LV, thick interventricular septum, and narrow mitral angle were predictors of LV perforation (82).
Coronary artery injury is a rare complication of MV surgery with prevalence ranging from 0.5 to 2%. The left circumflex coronary artery (LCx) lies close to the mitral annulus, with the distance ranging from 1 to 9 mm, hence it is at high risk of perioperative injury during MV replacement, and this risk is even higher in left dominant coronary circulation. It can present as an abrupt occlusion intraoperatively or less common as late angina months after the procedure (83). In one study of MDCT analysis of the LCx relation to the mitral annulus, the proximal LCx can be remarkably close to mitral annulus in mid systole. Another study suggests that LCx place can be used as a marker to locate the mitral annulus plane during the procedure, with distance of <5 mm between the two planes (83–87). In TMVR studies, there was no LCx injury or occlusion reported, and rather it seems a complication of transcatheter annuloplasty.
Conversion to open-heart surgery is not uncommon; it is usually due to the occurrence of other complications that require immediate surgical intervention to relieve hemodynamic compromise. The decision to convert to open-heart surgery in TMVR is usually made because of LVOTO, valve embolization, severe MR post-deployment, and LV perforation. Conversion to open-heart surgery or LV perforation occurred in 16 patients of the international TMVR registry (3%), with the highest rate in ViMAC, whereas in the ViMAC study by Guerrero et al. (16), Yoon et al. (33), and Kvitting et al. (63), the incidence was 5%, and it was found to be a predictor of all-cause mortality at 1 year.
The prevalence of cerebrovascular events in the early studies investigating new dedicated TMVR devices seems to be variable, with rates ranging from 0% to as high as 7% (88). However, definitive recommendations on stroke prevention and antithrombotic therapy in TMVR are not well-established. In this context, the well-established clinical experience with surgical mitral valve replacement provides the reference for antithrombotic management in TMVR. Indeed, it has been shown that the risk of embolic cerebrovascular events is higher in the early postoperative period, with overall annual risk of 2.3%, with the highest rates occurring in the first 90 days after surgery (89). This risk is mitigated by the number and duration of the antithrombotic agents prescribed (90, 91). Moreover, patient's related risk factors could increase the risk of cerebral embolic events, such as history of atrial fibrillation, prior embolic events, LV dysfunction, and hypercoagulable states (92, 93). Therefore, the current guidelines recommend the use of oral anticoagulation with vitamin K antagonist (VKA) for 3–6 months (3, 4) at the expense of an increased risk of bleeding.
Valve thrombosis can manifest as functional or clinical status deterioration, heart failure symptoms, increased transmitral gradient, or rarely as a visible thrombus or leaflet thickening on echocardiography or MDCT (94, 95). Despite the risk of early valve thrombosis, late valve thrombosis has been recognized as a relevant clinical entity. In a recent study, the median time to explantation for bioprosthetic thrombosis was 2 years, with more than 15% of cases occurring at least 5 years after surgery (96). Hence, long-term clinical and imaging surveillance is indicated to detect delayed valve thrombosis during follow-ups, which can improve with antithrombotic therapy. In the early feasibility studies testing novel THVs in the mitral position, relatively high rates of device thrombosis (6–8%) were reported after Tendyne (Abbott Vascular, Abbot Park, Illinois), Highlife (HighLife Medical, Irvine, California), and Fortis (Edwards Lifesciences, Irvine, California) THV implantation (97). Interestingly, no cases of THV thrombosis were reported after Intrepid THV (Medtronic Inc., Redwood City, California) implantation and the prescription of an aggressive antithrombotic therapy VKA with target international normalized ratio (INR) of 2.5–3.5 plus single antiplatelet therapy for at least 3 months, which came at the expense of higher major bleeding rates (98). Considering the available early evidence, an anticoagulation-based antithrombotic strategy seems to be necessary to prevent the risk of valve thrombosis and thromboembolic events after TMVR, tailoring the intensity and duration of the prescribed antithrombotic regimen on the individual bleeding and thrombotic risk profile of the single treated patient.
PVL is a common complication after prosthetic valve implantation, with a significant increase in morbidity and mortality, especially in patients with severe PVL. The prevalence of PVL in the early studies of surgically implanted bioprosthetic valves was 2.5% (99). After TMVR, the prevalence of PVL that required closure was ~3.5% (33). Moreover, around 3% of patients with PVL after TMVR will develop hemolysis. PVL often results from malposition of the valve or less commonly from valve endocarditis. Mild-to-moderate PVL can frequently be subclinical, with a minimal impact on clinical outcomes, and can be followed up with serial echocardiography studies. Significant (moderate-to-severe) PVL can manifest as heart failure, hemolysis, or a combination of the two (100, 101). Significant PVL is relatively rare in cases of TMVR in non-calcified mitral annuli, while in cases of ViMAC, the rate of moderate-to-severe PVL at 30 days can reach up to 14% (57). Due to the high mortality associated with surgical PVL closure, transcatheter PVL closure emerged as a safe and effective procedure. Today, the operator has the opportunity of using multiple devices concurrently, achieving outstanding results, and eliminating this common and serious complication (102–106).
Other less common fatal structural complications have been reported in TMVR studies with typically early presentation, and the urgent need for conversion to open heart surgery, these include ventricular septal defect, LV pseudoaneurysm, mitral annular disruption, MV leaflet/chordal disruption, and pulmonary vein perforation (34, 36, 49, 107).
As the population ages and the number of patients with MR expand, the therapeutic options available must grow as well. The advent and early success seen with TMVR has yielded much promise. These early outcomes may appear similar to the early success of TAVR, with the majority of patients now receiving transcatheter therapy instead of surgical replacement (108). The TMVR procedure complexity is much higher than that of TAVR and will limit the pace at which operators become comfortable with and regularly incorporate TMVR into their practice. Moreover, patient selection itself is also less defined for TMVR than it is for TAVR. Therefore, one must be cautious in comparing the two therapies due to distinct differences in patient selection, indications, and procedural considerations. Unlike aortic stenosis, for which clear guidelines exist regarding when to intervene, there are currently no clear guidelines as to when a patient should be considered for a mitral intervention for functional MR (4). Hence, we propose a workflow algorithm for the structural heart team when evaluating these patients, given the high morbidity and mortality associated with TMVR; we recommend a heart team comprehensive approach, including interventionalist, echocardiographers, cardiac surgeons, and heart failure specialists, to identify those who would benefit the most from this high-risk intervention. Figure 1 is a brief step-by-step algorithm describing the workflow from identifying those eligible TMVR candidates to post-procedural follow-up (109).
Finally, anatomical considerations such as geometrically dynamic non-planar annular characteristics and complex subvalvular structure make the creation and proper positioning of a valve vastly more difficult at the mitral than the aortic position. The potential for unique complications (i.e., LVOTO), as well as challenging procedural considerations (i.e., transseptal puncture and left atrial maneuvering to achieve coaxial orientation) will require the involvement of not only experienced operators but also a dedicated heart team, surgical and anesthesia staff with every case. These undefined guidelines, unanswered questions, and procedural considerations appear daunting in aggregate (110, 111). Two pivotal ongoing studies will help answer some of these challenging questions regarding different inoperable patient subpopulations with promising devices. The Summit clinical trial has three study cohorts evaluating the Tendyne valve in patients with moderate to severe MR: first cohort is a randomized comparison of the Tendyne heart valve to the MitraClip (Abbott Vascular, Santa Clara, CA), second cohort tests a nonrandomized comparison, and a third cohort evaluates Tendyne device in patients with severe MAC. Another study is the Apollo trial, a prospective non-randomized clinical trial evaluating the safety and efficacy of the Intrepid system (Medtronic, Minneapolis, MN, USA) vs. conventional surgery in patients with severe MR, with another single arm for inoperable patients.
TMVR represents an evolving therapeutic option to address the unmet clinical need of severe MR. However, several interventional challenges and procedure-related complications need to be addressed. The implementation of multimodality imaging is essential in procedural planning and to identify patients at high risk of complications. Careful pre-procedural planning to help in early identification of those who are at risk for complications, prompt detection and acute management of serious complications, and access refinement are key issues for TMVR advancement.
FK performed the literature review, the study outline, and was responsible for manuscript writing. MP and KR participated in the literature review and manuscript writing. EH, MC, YG, and AC helped with writing the manuscript. AL had the overall responsibility for the study and as the corresponding author confirms full access to all aspects of the research and writing process and takes final responsibility for the paper. All authors have participated in the work and have reviewed and agree with the content of the article.
AL has served on the advisory boards of Medtronic, Abbott Vascular, and Edwards Lifesciences.
The remaining authors declare that the research was conducted in the absence of any commercial or financial relationships that could be construed as a potential conflict of interest.
MR, mitral regurgitation; MV, mitral valve; MVr, mitral valve repair; TMVR, transcatheter mitral valve replacement; TAVR, transcatheter aortic valve replacement; TA, transapical; TF, transfemoral; LV, left ventricle; MAC, mitral annulus calcification; ViV, valve-in-valve; ViR, valve-in-ring; ViMAC, valve-in-MAC; MDCT, multidetector cardiac tomography; STS, Society of Thoracic Surgeons; THV, transcatheter heart valve; PVL, paravalvular leak; ASA, alcohol septal ablation; LCx, left circumflex coronary artery; AML, anterior mitral leaflet; LVOT, left ventricular outflow tract; LVOTO, left ventricular outflow tract obstruction; iASD, iatrogenic atrial septal defect.
1. Coffey S, Cairns BJ, Iung B. The modern epidemiology of heart valve disease. Heart. (2016) 102:75–85. doi: 10.1136/heartjnl-2014-307020
2. Nkomo VT, Gardin JM, Skelton TN, Gottdiener JS, Scott CG, Enriquez-Sarano M. Burden of valvular heart diseases: a population-based study. Lancet. (2006) 368:1005–11. doi: 10.1016/S0140-6736(06)69208-8
3. Baumgartner H, Falk V, Bax JJ, De Bonis M, Hamm C, Holm PJ, et al. 2017 ESC/EACTS Guidelines for the management of valvular heart disease. Eur Heart J. (2017) 38:2739–86. doi: 10.1093/eurheartj/ehx391
4. Nishimura RA, Otto CM, Bonow RO, Carabello BA, Erwin JP, Guyton RA, et al. 2014 AHA/ACC guideline for the management of patients with valvular heart disease: a report of the American college of cardiology/American heart association task force on practice guidelines. J Am Coll Cardiol. (2014) 63: doi: 10.1016/j.jacc.2014.02.536
5. Mirabel M, Iung B, Baron G, Messika-Zeitoun D, Détaint D, Vanoverschelde JL, et al. What are the characteristics of patients with severe, symptomatic, mitral regurgitation who are denied surgery? Eur Heart J. (2007) 28:1358–65. doi: 10.1093/eurheartj/ehm001
6. Niikura H, Gössl M, Kshettry V, Olson S, Sun B, Askew J, et al. Causes and clinical outcomes of patients who are ineligible for transcatheter mitral valve replacement. JACC Cardiovasc Interv. (2019) 12:196–204. doi: 10.1016/j.jcin.2018.10.042
7. Jawed S, Zubair U, Zubair Z. Transcatheter mitral valve replacement: an alternate treatment methodology for patients at high surgical risk. Cureus. (2018) 10:e2504. doi: 10.7759/cureus.2504
8. Sorajja P, Cavalcante JL, Gössl M. The need for transcatheter mitral valve replacement. J Am Coll Cardiol. (2019) 73:1247–9. doi: 10.1016/j.jacc.2018.11.062
9. Khan F, Winkel M, Ong G, Brugger N, Pilgrim T, Windecker S, et al. Percutaneous mitral edge-to-edge repair: state of the art and a glimpse to the future. Front Cardiovasc Med. (2019) 6:122. doi: 10.3389/fcvm.2019.00122
10. Latib A, Ancona MB, Ferri L, Montorfano M, Mangieri A, Regazzoli D, et al. Percutaneous direct annuloplasty with cardioband to treat recurrent mitral regurgitation after mitraclip implantation. JACC Cardiovasc Interv. (2016) 9:e191–2. doi: 10.1016/j.jcin.2016.06.028
11. Messika-Zeitoun D, Nickenig G, Latib A, Kuck KH, Baldus S, Schueler R, et al. Transcatheter mitral valve repair for functional mitral regurgitation using the Cardioband system: 1 year outcomes. Eur Heart J. (2019) 40:466–72. doi: 10.1093/eurheartj/ehy424
12. Paradis J-M, Del Trigo M, Puri R, Rodés-Cabau J. Transcatheter valve-in-valve and valve-in-ring for treating aortic and mitral surgical prosthetic dysfunction. In: Paradis J-M, Del Trigo M, Puri R, Rodés-Cabau J, editors. Transcatheter valve-in-valve and valve-in-ring for treating aortic and mitral. J Am Coll Cardiol. (2015) 66:2019–37. doi: 10.1016/j.jacc.2015.09.015
13. Mangieri A, Laricchia A, Giannini F, Gallo F, Kargoli F, Ladanyi A, et al. Emerging technologies for percutaneous mitral valve repair. Front Cardiovasc Med. (2019) 6:161. doi: 10.3389/fcvm.2019.00161
14. Sorajja P, Leon MB, Adams DH, Webb JG, Farivar RS. Transcatheter therapy for mitral regurgitation clinical challenges and potential solutions. Circulation. (2017) 136:404–17. doi: 10.1161/CIRCULATIONAHA.117.025264
15. Iyer R, Chalekian A, Lane R, Evans M, Yi S, Morris J. Transcatheter mitral valve replacement: functional requirements for device design, bench-top, and pre-clinical evaluation. Cardiovasc Eng Technol. (2018) 9:301–38. doi: 10.1007/s13239-018-0364-z
16. Guerrero M, Urena M, Himbert D, Wang DD, Eleid M, Kodali S, et al. 1-year outcomes of transcatheter mitral valve replacement in patients with severe mitral annular calcification. J Am Coll Cardiol. (2018) 71:1841–53. doi: 10.1016/j.jacc.2018.02.054
17. Overtchouk P, Piazza N, Granada JF, Modine T. Predictors of adverse outcomes after transcatheter mitral valve replacement. Expert Rev Cardiovasc Ther. (2019) 17:625–32. doi: 10.1080/14779072.2019.1653186
18. Cheung A, Webb JG, Barbanti M, Freeman M, Binder RK, Thompson C, Wood DA, Ye J. 5-year experience with transcatheter transapical mitral valve-in-valve implantation for bioprosthetic valve dysfunction. J Am Coll Cardiol. (2013) 61:1759–66. doi: 10.1016/j.jacc.2013.01.058
19. Mahadevan VS, Manoly I, Hasan R. 2-year follow-up of the first in human transapical implantation of transcatheter inverted aortic valve to treat native mitral valve stenosis. JACC Cardiovasc Interv. (2015) 8:e247–9. doi: 10.1016/j.jcin.2015.07.037
20. Modine T, Vahl TP, Khalique OK, Coisne A, Vincent F, Montaigne D, et al. First-in-human implant of the cephea transseptal mitral valve replacement system. Circ Cardiovasc Interv. (2019) 12:3. doi: 10.1161/CIRCINTERVENTIONS.119.008003
21. Russell HM, Guerrero ME, Salinger MH, Manzuk MA, Pursnani AK, Wang D, et al. Open atrial transcatheter mitral valve replacement in patients with mitral annular calcification. J Am Coll Cardiol. (2018) 72:1437–48. doi: 10.1016/j.jacc.2018.07.033
22. Praz F, Khalique OK, Lee R, Veeragandham R, Russell H, Guerrero M, et al. Transatrial implantation of a transcatheter heart valve for severe mitral annular calcification. J Thorac Cardiovasc Surg. (2018) 156:132–42. doi: 10.1016/j.jtcvs.2018.03.016
25. Sorajja P, Moat N, Badhwar V, Walters D, Paone G, Bethea B, et al. Initial feasibility study of a new transcatheter mitral prosthesis: the first 100 patients. J Am Coll Cardiol. (2019) 73:1250–60. doi: 10.1016/j.jacc.2018.12.066
26. Makkar RR. Sapien M3: Device Attributes, Implant Procedure and Early Results. San Diego, CA: Transcatheter Cardiovascular Therapeutics (TCT)
27. Regueiro A, Ye J, Fam N, Bapat VN, Dagenais F, Peterson MD, et al. 2-Year outcomes after transcatheter mitral valve replacement. JACC Cardiovasc Interv. (2017) 10:1671–678. doi: 10.1016/j.jcin.2017.05.032
28. Williams MR. Transfemoral TMVR: Caisson Transcatheter Mitral Valve Replacement. San Diego, CA: Transcatheter Cardiovascular Therapeutics.
29. Eng MH, Greenbaum A, Wang DD, Wyman J, Dnp, Arjomand H, et al. Thrombotic valvular dysfunction with transcatheter mitral interventions for postsurgical failures. Catheter Cardiovasc Interv. (2017) 90:321–8. doi: 10.1002/ccd.26909
30. Bouleti C, Fassa A-A, Himbert D, Brochet E, Ducrocq G, Nejjari M, et al. Transfemoral implantation of transcatheter heart valves after deterioration of mitral bioprosthesis or previous ring annuloplasty. JACC Cardiovasc Interv. (2015) 8:83–91. doi: 10.1016/j.jcin.2014.07.026
31. Kamioka N, Babaliaros V, Morse MA, Frisoli T, Lerakis S, Iturbe JM, et al. Comparison of clinical and echocardiographic outcomes after surgical redo mitral valve replacement and transcatheter mitral valve-in-valve therapy. JACC Cardiovasc Interv. (2018) 11:1131–8. doi: 10.1016/j.jcin.2018.03.011
32. El Sabbagh A, Eleid MF, Foley TA, Al-Hijji MA, Daly RC, Rihal CS, et al. Direct transatrial implantation of balloon-expandable valve for mitral stenosis with severe annular calcifications: early experience and lessons learned. Eur J Cardiothorac Surg. (2018) 53:162–9. doi: 10.1093/ejcts/ezx262
33. Yoon SH, Whisenant BK, Bleiziffer S, Delgado V, Dhoble A, Schofer N, et al. Outcomes of transcatheter mitral valve replacement for degenerated bioprostheses, failed annuloplasty rings, and mitral annular calcification. Eur Heart J. (2019) 40:441–51. doi: 10.1093/eurheartj/ehy590
34. Guerrero M. MITRAL (Mitral Implantation of TRAnscatheter Valves) - 30-Day Outcomes of Transcatheter MV Replacement in Patients With Severe Mitral Valve Disease Secondary to Mitral Annular Calcifcation or Failed Annuloplasty Rings. Denver, CO: Transcatheter Cardiovascular Therapeutics (TCT).
35. Cullen MW, Cabalka AK, Alli OO, Pislaru S V, Sorajja P, Nkomo VT, et al. Transvenous, antegrade Melody valve-in-valve implantation for bioprosthetic mitral and tricuspid valve dysfunction: a case series in children and adults. JACC Cardiovasc Interv. (2013) 6:598–605. doi: 10.1016/j.jcin.2013.02.010
36. Guerrero M. 30-Day Outcomes of Transcatheter Mitral Valve Replacement in Native Mitral Valve Disease With Severe Mitral Annular Calcificaiton in the United States: Data From STS/ACC/TVT Registry. Paris: EuroPCR.
37. Seiffert M, Conradi L, Baldus S, Schirmer J, Knap M, Blankenberg S, et al. Transcatheter mitral valve-in-valve implantation in patients with degenerated bioprostheses. JACC Cardiovasc Interv. (2012) 5:341–9. doi: 10.1016/j.jcin.2011.12.008
38. Werner N, Kilkowski C, Sutor D, Weisse U, Schneider S, Zahn R. Transcatheter mitral valve implantation (TMVI) Using Edwards SAPIEN 3 prostheses in patients at very high or prohibitive surgical risk: A single-center experience. J Interv Cardiol. (2020) 2020:247. doi: 10.1155/2020/9485247
39. Kliger C, Angulo R, Maranan L, Kumar R, Jelnin V, Kronzon I, et al. Percutaneous complete repair of failed mitral valve prosthesis: simultaneous closure of mitral paravalvular leaks and transcatheter mitral valve implantation - single-centre experience. EuroIntervention. (2015) 10:1336–45. doi: 10.4244/EIJY14M05_01
40. Cerillo AG, Chiaramonti F, Murzi M, Bevilacqua S, Cerone E, Palmieri C, et al. Transcatheter valve in valve implantation for failed mitral and tricuspid bioprosthesis. Catheter Cardiovasc Interv. (2011) 78:987–95. doi: 10.1002/ccd.23112
41. Descoutures F, Himbert D, Maisano F, Casselman F, de Weger A, Bodea O, et al. Transcatheter valve-in-ring implantation after failure of surgical mitral repair. Eur J Cardio-thoracic Surg. (2013) 44:155. doi: 10.1093/ejcts/ezt155
42. Wilbring M, Alexiou K, Tugtekin SM, Sill B, Hammer P, Schmidt T, et al. Transapical transcatheter valve-in-valve implantation for deteriorated mitral valve bioprostheses. Ann Thorac Surg. (2013) 95:111–7. doi: 10.1016/j.athoracsur.2012.08.004
43. Urena M, Brochet E, Lecomte M, Kerneis C, Carrasco JL, Ghodbane W, et al. Clinical and haemodynamic outcomes of balloon-expandable transcatheter mitral valve implantation: a 7-year experience. Eur Heart J. (2018) 39:2679–89. doi: 10.1093/eurheartj/ehy271
44. Eleid MF, Whisenant BK, Cabalka AK, Williams MR, Nejjari M, Attias D, et al. Early outcomes of percutaneous transvenous transseptal transcatheter valve implantation in failed bioprosthetic mitral valves, ring annuloplasty, and severe mitral annular calcification. JACC Cardiovasc Interv. (2017) 10:1932–42. doi: 10.1016/j.jcin.2017.08.014
45. Whisenant B, Jones K, Miller D, Horton S, Miner E. Thrombosis following mitral and tricuspid valve-in-valve replacement. J Thorac Cardiovasc Surg. (2015) 149:e26–e9. doi: 10.1016/j.jtcvs.2014.10.075
46. Latib A, Ruparelia N, Bijuklic K, De Marco F, Gatto F, Hansen L, et al. First-in-man transcatheter mitral valve-in-ring implantation with a repositionable and retrievable aortic valve prosthesis. EuroIntervention. (2016) 11:1148–52. doi: 10.4244/EIJY15M11_02
48. Mylotte D, Andalib A, Thériault-Lauzier P, Dorfmeister M, Girgis M, Alharbi W, et al. Transcatheter heart valve failure: a systematic review. Eur Heart J. (2015) 36:1306–27. doi: 10.1093/eurheartj/ehu388
49. Yoon SH, Whisenant BK, Bleiziffer S, Delgado V, Schofer N, Eschenbach L, et al. Transcatheter mitral valve replacement for degenerated bioprosthetic valves and failed annuloplasty rings. J Am Coll Cardiol. (2017) 70:1121–31. doi: 10.1016/j.jacc.2017.07.714
50. Bapat VVN, Khaliel F, Ihleberg L. Delayed migration of Sapien valve following a transcatheter mitral valve-in-valve implantation. Catheter Cardiovasc Interv. (2014) 83:E150–4. doi: 10.1002/ccd.25076
51. Binder RK, Webb JG. Transcatheter heart valve migration and embolization: rare and preventable? Eur Heart J. (2019) 40:3166–8. doi: 10.1093/eurheartj/ehz562
52. Blanke P, Naoum C, Dvir D, Bapat V, Ong K, Muller D, et al. Predicting LVOT obstruction in transcatheter mitral valve implantation: Concept of the Neo-LVOT. JACC Cardiovasc Imaging. (2017) 10:482–85. doi: 10.1016/j.jcmg.2016.01.005
53. Stone GW, Adams DH, Abraham WT, Kappetein AP, Généreux P, Vranckx P, et al. Clinical trial design principles and endpoint definitions for transcatheter mitral valve repair and replacement: part 2: endpoint definitions: a consensus document from the mitral valve academic research consortium. J Am Coll Cardiol. (2015) 66:308–21. doi: 10.1016/j.jacc.2015.05.049
54. Vaquerizo B, Theriault-Lauzier P, Piazza N. Percutaneous transcatheter mitral valve replacement: patient-specific three-dimensional computer-based heart model and prototyping. Rev Esp Cardiol. (2015) 68:1165–73. doi: 10.1016/j.rec.2015.08.005
55. El Sabbagh A, Eleid MF, Matsumoto JM, Anavekar NS, Al-Hijji MA, Said SM, et al. Three-dimensional prototyping for procedural simulation of transcatheter mitral valve replacement in patients with mitral annular calcification. Catheter Cardiovasc Interv. (2018) 92:E537–49. doi: 10.1002/ccd.27488
56. Gheorghe LL, Mobasseri S, Agricola E, Wang DD, Milla F, Swaans M, et al. Imaging for native mitral valve surgical and transcatheter interventions. Imaging Best Outcomes Struct Hear Interv Spec Issue. (2021) 14:112–27. doi: 10.1016/j.jcmg.2020.11.021
57. Yoon S-H, Bleiziffer S, Latib A, Eschenbach L, Ancona M, Vincent F, et al. Predictors of left ventricular outflow tract obstruction after transcatheter mitral valve replacement. JACC Cardiovasc Interv. (2019) 12:182–93. doi: 10.1016/j.jcin.2018.12.001
58. Meduri CU, Reardon MJ, Lim DS, Howard E, Dunnington G, Lee DP, et al. Novel multiphase assessment for predicting left ventricular outflow tract obstruction before transcatheter mitral valve replacement. JACC Cardiovasc Interv. (2019) 12:2402–12. doi: 10.1016/j.jcin.2019.06.015
59. Wang DD, Guerrero M, Eng MH, Eleid MF, Meduri CU, Rajagopal V, et al. Alcohol septal ablation to prevent left ventricular outflow tract obstruction during transcatheter mitral valve replacement: first-in-man study. JACC Cardiovasc Interv. (2019) 12:1268–79. doi: 10.1016/j.jcin.2019.02.034
60. Leipsic J, Nørgaard BL, Khalique O, Cavalcante JL, Wang DD, Jilaihawi H, et al. Core competencies in cardiac ct for imaging structural heart disease interventions: an expert consensus statement. JACC Cardiovasc Imaging. (2019) 12:2555–9. doi: 10.1016/j.jcmg.2019.09.023
61. Leipsic J, Blanke P. The neo LVOT: from concept to clinical practice. JACC Cardiovasc Interv. (2019) 12:2413–5. doi: 10.1016/j.jcin.2019.07.009
62. Bax JJ, Debonnaire P, Lancellotti P, Ajmone Marsan N, Tops LF, Min JK, et al. Transcatheter interventions for mitral regurgitation: multimodality imaging for patient selection and procedural guidance. JACC Cardiovasc Imaging. (2019) 12:2029–48. doi: 10.1016/j.jcmg.2019.03.036
63. Kvitting J-P, Nielsen NE, Vanhanen I, Baranowski J. Surgical management of outflow tract obstruction after transapical mitral valve implantation. J Card Surg. (2018) 33:545–7. doi: 10.1111/jocs.13771
64. Bapat V, Pirone F, Kapetanakis S, Rajani R, Niederer S. Factors influencing left ventricular outflow tract obstruction following a mitral valve-in-valve or valve-in-ring procedure, part 1. Catheter Cardiovasc Interv. (2015) 86:747–60. doi: 10.1002/ccd.25928
65. Wang DD, Eng MH, Greenbaum AB, Myers E, Forbes M, Karabon P, et al. Validating a prediction modeling tool for left ventricular outflow tract (LVOT) obstruction after transcatheter mitral valve replacement (TMVR). Catheter Cardiovasc Interv. (2018) 92:379–87. doi: 10.1002/ccd.27447
66. Greenbaum AB, Condado JF, Eng M, Lerakis S, Wang DD, Kim DW, et al. Long or redundant leaflet complicating transcatheter mitral valve replacement: Case vignettes that advocate for removal or reduction of the anterior mitral leaflet. Catheter Cardiovasc Interv. (2018) 92:627–632. doi: 10.1002/ccd.27054
67. Khan JM, Babaliaros VC, Greenbaum AB, Foerst JR, Yazdani S, McCabe JM, et al. Anterior leaflet laceration to prevent ventricular outflow tract obstruction during transcatheter mitral valve replacement. J Am Coll Cardiol. (2019) 73:2521–34. doi: 10.1016/j.jacc.2019.02.076
68. Babaliaros VC, Greenbaum AB, Khan JM, Rogers T, Wang DD, Eng MH, et al. Intentional percutaneous laceration of the anterior mitral leaflet to prevent outflow obstruction during transcatheter mitral valve replacement: first-in-human experience. JACC Cardiovasc Interv. (2017) 10:798–809. doi: 10.1016/j.jcin.2017.01.035
69. Guerrero M, Wang DD, Himbert D, Urena M, Pursnani A, Kaddissi G, et al. Short-term results of alcohol septal ablation as a bail-out strategy to treat severe left ventricular outflow tract obstruction after transcatheter mitral valve replacement in patients with severe mitral annular calcification. Catheter Cardiovasc Interv. (2017) 90:1220–26. doi: 10.1002/ccd.26975
70. Deharo P, Urena M, Himbert D, Brochet E, Rouleau F, Pinaud F, et al. Bail-out alcohol septal ablation for left ventricular outflow tract obstruction after transcatheter mitral valve replacement. JACC Cardiovasc Interv. (2016) 9:e73–e6. doi: 10.1016/j.jcin.2016.01.010
71. Kassar M, Khalique OK, Pilgrim T, Reineke D, Carrel T, Windecker S, et al. Surgical transatrial implantation of transcatheter heart valves in severe mitral annular calcification. Interv ardiol Clin. (2019) 8:313–9. doi: 10.1016/j.iccl.2019.02.006
72. Praz F, Khalique OK, Lee R, Wu IY, Russell H, Guerrero M, et al. Imaging in patients with severe mitral annular calcification: insights from a multicentre experience using transatrial balloon-expandable valve replacement. Eur Heart J Cardiovasc Imaging. (2019) 20:1395–406. doi: 10.1093/ehjci/jez050
73. Frerker C, Schmidt T, Schlüter M, Bader R, Schewel J, Schewel D, et al. Transcatheter implantation of aortic valve prostheses into degenerated mitral valve bioprostheses and failed annuloplasty rings: outcomes according to access route and Mitral Valve Academic Research Consortium (MVARC) criteria. EuroIntervention. (2016) 12:1520–6. doi: 10.4244/EIJ-D-16-00209
74. Doshi R, Shah P, Meraj PM. In-hospital outcomes comparison of transfemoral vs transapical transcatheter aortic valve replacement in propensity-matched cohorts with severe aortic stenosis. Clin Cardiol. (2018) 41:326–32. doi: 10.1002/clc.22866
75. Ferrari E, Eeckhout E, Keller S, Muller O, Tozzi P, Berdajs D, et al. Transfemoral versus transapical approach for transcatheter aortic valve implantation: hospital outcome and risk factor analysis. J Cardiothorac Surg. (2017) 12:78. doi: 10.1186/s13019-017-0638-9
76. Furukawa N, Kuss O, Emmel E, Scholtz S, Scholtz W, Fujita B, et al. Minimally invasive versus transapical versus transfemoral aortic valve implantation: a one-to-one-to-one propensity score–matched analysis. J Thorac Cardiovasc Surg. (2018) 156:1825–34. doi: 10.1016/j.jtcvs.2018.04.104
77. Biancari F, Rosato S, D'Errigo P, Ranucci M, Onorati F, Barbanti M, et al. Immediate and intermediate outcome after transapical versus transfemoral transcatheter aortic valve replacement. Am J Cardiol. (2016) 117:245–51. doi: 10.1016/j.amjcard.2015.10.036
78. Singh SM, Douglas PS, Reddy VY. The incidence and long-term clinical outcome of iatrogenic atrial septal defects secondary to transseptal catheterization with a 12F transseptal sheath. Circ Arrhythmia Electrophysiol. (2011) 4:166–71. doi: 10.1161/CIRCEP.110.959015
79. Cruz-Gonzalez I, Rama-Merchan JC, Calvert PA, Rodríguez-Collado J, Barreiro-Pérez M, Martín-Moreiras J, et al. Percutaneous closure of paravalvular leaks: a systematic review. J Interv Cardiol. (2016) 29:382–92. doi: 10.1111/joic.12295
80. Whisenant B, Kapadia SR, Eleid MF, Kodali SK, McCabe JM, Krishnaswamy A, et al. One-year outcomes of mitral valve-in-valve using the SAPIEN 3 transcatheter heart valve. JAMA Cardiol. (2020) 5:1–8. doi: 10.1001/jamacardio.2020.2974
81. Fukui M, Sorajja P, Gössl M, Bae R, Lesser JR, Sun B, et al. Left ventricular remodeling after transcatheter mitral valve replacement with tendyne: new insights from computed tomography. JACC Cardiovasc Interv. (2020) 13:2038–48. doi: 10.1016/j.jcin.2020.06.009
82. Owais T, El Garhy M, Fuchs J, Disha K, ElKaffas S, Breuer M, et al. Pathophysiological factors associated with left ventricular perforation in transcatheter aortic valve implantation by transfemoral approach - pubmed. J Heart Valve Dis. (2017) 26:430–6.
83. Hiltrop N, Bennett J, Desmet W. Circumflex coronary artery injury after mitral valve surgery: A report of four cases and comprehensive review of the literature. Catheter Cardiovasc Interv. (2017) 89:78–92. doi: 10.1002/ccd.26449
84. Kaklikkaya I, Yeginoglu G. Damage to coronary arteries during mitral valve surgery. Heart Surg Forum. (2003) 6:E138–42. doi: 10.1532/hsf.974
85. Kapadia SR, Mentias A, Barakat AF, Raza MQ, Lal Poddar K, Baeza C, et al. Relationship of mitral valve annulus plane and circumflex-right coronary artery plane: Implications for Transcatheter Mitral Valve Implantation. Catheter Cardiovasc Interv. (2017) 89:932–43. doi: 10.1002/ccd.26575
86. Hinzpeter R, Eberhard M, Pozzoli A, von Spiczak J, Manka R, Tanner FC, et al. Dynamic anatomic relationship of the coronary arteries to the valves. Part 1: mitral annulus and circumflex artery. EuroIntervention. (2019) 15:919–22. doi: 10.4244/EIJ-D-19-00669
87. Ziadi J, Mleyhi S, Denguir R, Khayati A. Iatrogenic occlusion of the circumflex artery and left ventricle pseudoaneurysm after mitral annuloplasty. J Cardiol cases. (2014) 9:104–5. doi: 10.1016/j.jccase.2013.10.013
88. Pagnesi M, Moroni F, Beneduce A, Giannini F, Colombo A, Weisz G, et al. Thrombotic Risk and Antithrombotic Strategies After Transcatheter Mitral Valve Replacement. JACC Cardiovasc Interv. (2019) 12:2388–401. doi: 10.1016/j.jcin.2019.07.055
89. Heras M, Chesebro JH, Fuster V, Penny WJ, Grill DE, Bailey KR, et al. High risk of thromboemboli early after bioprosthetic cardiac valve replacement. J Am Coll Cardiol. (1995) 25:1111–9. doi: 10.1016/0735-1097(94)00563-6
90. Brennan JM, Edwards FH, Zhao Y, O'Brien S, Booth ME, Dokholyan RS, et al. Early anticoagulation of bioprosthetic aortic valves in older patients: results from the Society of Thoracic Surgeons Adult Cardiac Surgery National Database. J Am Coll Cardiol. (2012) 60:971–7. doi: 10.1016/j.jacc.2012.05.029
91. Mérie C, Køber L, Skov Olsen P, Andersson C, Gislason G, Skov Jensen J, et al. Association of warfarin therapy duration after bioprosthetic aortic valve replacement with risk of mortality, thromboembolic complications, and bleeding. JAMA - J Am Med Assoc. (2012) 308:2118–125. doi: 10.1001/jama.2012.54506
92. Dangas GD, Weitz JI, Giustino G, Makkar R, Mehran R. Prosthetic heart valve thrombosis. J Am Coll Cardiol. (2016) 68:2670–89. doi: 10.1016/j.jacc.2016.09.958
93. Puri R, Auffret V, Rodés-Cabau J. Bioprosthetic Valve Thrombosis. J Am Coll Cardiol. (2017) 69:2193–211. doi: 10.1016/j.jacc.2017.02.051
94. Jilaihawi H, Asch FM, Manasse E, Ruiz CE, Jelnin V, Kashif M, et al. Systematic CT methodology for the evaluation of subclinical leaflet thrombosis. JACC Cardiovasc Imaging. (2017) 10:461–70. doi: 10.1016/j.jcmg.2017.02.005
95. Makkar RR, Fontana G, Jilaihawi H, Chakravarty T, Kofoed KF, De Backer O, et al. Possible subclinical leaflet thrombosis in bioprosthetic aortic valves. N Engl J Med. (2015) 373:2015–24. doi: 10.1056/NEJMoa1509233
96. Egbe AC, Pislaru S V, Pellikka PA, Poterucha JT, Schaff H V, Maleszewski JJ, et al. Bioprosthetic valve thrombosis versus structural failure: clinical and echocardiographic predictors. J Am Coll Cardiol. (2015) 66:2285–94. doi: 10.1016/j.jacc.2015.09.022
97. Edwards Life Sciences C. Edwards Pauses Enrollment in Early Stage Mitral Program. Edwards Lifescience. (2015). Available online at: https://www.edwards.com/ns20150519A
98. Bapat V, Rajagopal V, Meduri C, Farivar RS, Walton A, Duffy SJ, et al. Early experience with new transcatheter mitral valve replacement. J Am Coll Cardiol. (2018) 71:12–21. doi: 10.1016/j.jacc.2017.10.061
99. Bloch G, Vouhe PR, Menu P, Poulain H, Cachera JP, Aubry P, et al. Long-term evaluation of bioprosthetic valves: 615 consecutive cases. Eur Heart J. (1984) 5 (Suppl. D):73–80. doi: 10.1093/eurheartj/5.suppl_d.73
100. Jindani A, Neville EM, Venn G, Williams BT. Paraprosthetic leak: a complication of cardiac valve replacement. J Cardiovasc Surg (Torino). (1991) 32:503–8.
101. Taramasso M, Maisano F, Denti P, Guidotti A, Sticchi A, Pozzoli A, et al. Surgical treatment of paravalvular leak: Long-term results in a single-center experience (up to 14 years). J Thorac Cardiovasc Surg. (2015) 149:1270–5. doi: 10.1016/j.jtcvs.2014.12.041
102. Asami M, Pilgrim T, Windecker S, Praz F. Case report of simultaneous transcatheter mitral valve-in-valve implantation and percutaneous closure of two paravalvular leaks. Eur Hear J Case Rep. (2019) 3:ytz123. doi: 10.1093/ehjcr/ytz123
103. Cruz-Gonzalez I, Rama-Merchan JC, Rodríguez-Collado J, Martín-Moreiras J, Diego-Nieto A, Barreiro-Pérez M, et al. Transcatheter closure of paravalvular leaks: state of the art. Neth Heart J. (2017) 25:116–4. doi: 10.1007/s12471-016-0918-3
104. Dziubek M, Pierrakos C, Chebli L, Demanet H, Sanoussi A, Wauthy P. Para-prosthetic leaks following mitral valve replacement: case analysis on a 20-year period. Curr Cardiol Rev. (2018) 14:15–24. doi: 10.2174/1573403x13666171110110344
105. Greenbaum AB, Frisoli TM. Surgical paravalvular leak closure: no more second chances. JACC Cardiovasc Interv. (2017) 10:508–9. doi: 10.1016/j.jcin.2017.01.031
106. Kumar R, Jelnin V, Kliger C, Ruiz CE. Percutaneous paravalvular leak closure. Cardiol Clin. (2013) 31:431–40. doi: 10.1016/j.ccl.2013.05.004
107. Guerrero M, Dvir D, Himbert D, Urena M, Eleid M, Wang DD, et al. Transcatheter mitral valve replacement in native mitral valve disease with severe mitral annular calcification: results from the first multicenter global registry. JACC Cardiovasc Interv. (2016) 9:1361–71. doi: 10.1016/j.jcin.2016.04.022
108. Mack MJ, Leon MB, Thourani VH, Makkar R, Kodali SK, Russo M, et al. Transcatheter aortic-valve replacement with a balloon-expandable valve in low-risk patients. N Engl J Med. (2019) 380:1695–705. doi: 10.1056/NEJMoa1814052
109. Urena M, Himbert D, Brochet E, Carrasco JL, Iung B, Nataf P, et al. Transseptal transcatheter mitral valve replacement using balloon-expandable transcatheter heart valves: a step-by-step approach. JACC Cardiovasc Interv. (2017) 10:1905–19. doi: 10.1016/j.jcin.2017.06.069
110. Meng Z, Zhang E-L, Wu Y-J. Current status and future direction of transcatheter mitral valve replacement. Chin Med J (Engl). (2018) 131:505–7. doi: 10.4103/0366-6999.226080
Keywords: TMVR, TMVR complications, lvot obstruction, valve thrombosis, valve embolization
Citation: Kargoli F, Pagnesi M, Rahgozar K, Goldberg Y, Ho E, Chau M, Colombo A and Latib A (2021) Current Devices and Complications Related to Transcatheter Mitral Valve Replacement: The Bumpy Road to the Top. Front. Cardiovasc. Med. 8:639058. doi: 10.3389/fcvm.2021.639058
Received: 08 December 2020; Accepted: 13 April 2021;
Published: 11 June 2021.
Edited by:
Jan Van Der Heyden, St. Jan Hospital, BelgiumReviewed by:
Neil P. Fam, St. Michael's Hospital, CanadaCopyright © 2021 Kargoli, Pagnesi, Rahgozar, Goldberg, Ho, Chau, Colombo and Latib. This is an open-access article distributed under the terms of the Creative Commons Attribution License (CC BY). The use, distribution or reproduction in other forums is permitted, provided the original author(s) and the copyright owner(s) are credited and that the original publication in this journal is cited, in accordance with accepted academic practice. No use, distribution or reproduction is permitted which does not comply with these terms.
*Correspondence: Azeem Latib, YWxhdGliQGdtYWlsLmNvbQ==
Disclaimer: All claims expressed in this article are solely those of the authors and do not necessarily represent those of their affiliated organizations, or those of the publisher, the editors and the reviewers. Any product that may be evaluated in this article or claim that may be made by its manufacturer is not guaranteed or endorsed by the publisher.
Research integrity at Frontiers
Learn more about the work of our research integrity team to safeguard the quality of each article we publish.