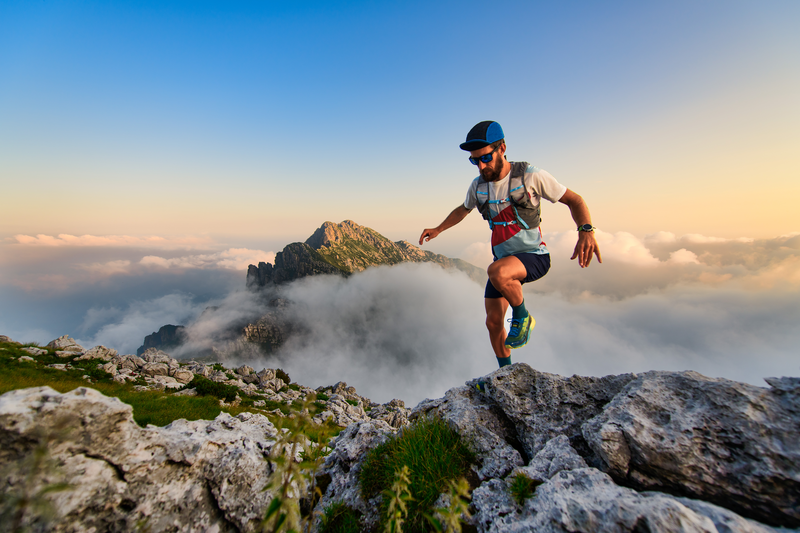
94% of researchers rate our articles as excellent or good
Learn more about the work of our research integrity team to safeguard the quality of each article we publish.
Find out more
SPECIALTY GRAND CHALLENGE article
Front. Cardiovasc. Med. , 04 May 2021
Sec. Thrombosis and Haemostasis
Volume 8 - 2021 | https://doi.org/10.3389/fcvm.2021.637005
This article is part of the Research Topic Highlights in Thrombosis: 2021 View all 9 articles
Thrombosis is the formation of a blood clot that partially or completely hinders blood flow in one or more blood vessels, either in the venous or arterial circulation. Thrombosis is the immediate trigger of a number of acute cardiovascular diseases (CVD), including myocardial infarction, ischemic stroke, and venous thromboembolism (VTE), which are all leading causes of mortality and long-term morbidity, affecting men and women of all ethnicities all over the world. In 2016, an estimated 17.9 million people died from CVD, representing 31% of all global deaths; of these deaths, 85% were due to myocardial infarction and stroke, half of which due to thromboembolic brain injury1. The number of VTE events is less accurately monitored, but estimates included 684,000 deep vein thrombosis (DVT) events, 435,000 pulmonary embolism (PE) events, and a total of about 543,000 VTE-related deaths among the population of the European Union, of ~450 million total subjects in 2004 (1).
In all these acute conditions, thrombosis is a localized problem, causing ischemia in the downstream vascular bed in case of arterial thrombosis, and impaired return of blood flow to the heart, with a risk of thromboembolic organ injury in the case of VTE, respectively. Thrombosis can also be a more systemic or combined local-systemic phenomenon and the current Covid-19 pandemic introduces a dramatic example of systemic hypercoagulability and localized thrombosis, associated with SARS-CoV-2 virus pneumonia (Covid-19). The latter type of systemic coagulopathy, with both microvascular thrombo-inflammation and large vessel thrombosis (and/or VTE), was initially regarded as disseminated intravascular coagulation (DIC), later thrombotic microangiopathy, both attempts to describe and define the specific type of thrombotic tendency (2, 3). Thrombotic thrombocytopenic purpura (4) and heparin induced thrombocytopenia (5) are yet other systemic thrombotic phenomena.
Essentially, thrombosis is an excess response-to-injury, meant to arrest bleeding from a damaged vascular bed, in case of trauma; or, to respond to an infectious attack, involving perturbation of the vascular endothelium by inflammatory cells and platelets (6), secreted inflammatory mediators and generation of extracellular vesicles, challenging the normal anticoagulant endothelial function to turn into a more prothrombotic phenotype (7–10). The latter type of immune mediated thrombosis is also referred to as immunothrombosis (11), but DIC and other systemic coagulopathies also qualify as immune mediated manifestations of thrombo-inflammatory reactions. Whereas, “thrombosis” as a result of an exaggerated response-to-injury is still targeted against exogenous elements (traumatic, pathogens, particulate matter) in case of recognizing “self” as “foreign” thrombosis can also be part of autoimmune pathologies like antiphospholipid syndrome (APS) (12). Whatever the cause, thrombosis is either directly harmful, due to vascular occlusion, or indicative of an increased likelihood of dying, in conjunction with severe underlying diseases like cancer (13), or sepsis (DIC also referred to as “death is coming”) (14).
Primary thrombosis prevention would be theoretically attractive, but the options are limited and not without risk, because like with the immune system, it can harm and inhibit the coagulation system, and impaired coagulation results in a bleeding tendency. Interestingly, clinical evidence suggests that an increased risk for thrombosis, such as that associated with the carriership of the factor V Leiden mutation, might alter susceptibility for specific infections, which may illustrate a role for coagulation in host defense (15). Experimental studies support a protective effect for factor V Leiden in pneumonia (16). On the other hand, protein C deficiency aggravated polymicrobial sepsis in mice (17), showing the complexity of such risk associations linked to specific coagulation factors, i.e., factor V and protein C (linked via the proteolytic effect of activated protein C on factor Va). A related issue is whether the use of anticoagulant agents would alter susceptibility to infection and this issue has become highly relevant in the current Covid-19 pandemic. Although we are awaiting stronger evidence, recent data suggest that anticoagulation use may protect against mortality related to Covid-19 (18) but in very high-risk patients this protective effect is not yet evident (19). These issues are exciting from an etiological perspective but practical management does not yet take into account thrombophilia traits to initiate thrombosis prophylaxis. The primary prevention of arterial thrombosis is limited to patients with symptomatic atherosclerosis, whereas the use of aspirin in subjects without vascular disease is only considered in those at fairly high risk of CV events, e.g., diabetics. For VTE, primary prevention is limited to high-risk situations, e.g., major surgery and major medical illnesses like Covid-19, or in specific malignancies (multiple myeloma) where chemotherapy brings additional thrombosis risk.
Unfortunately, in many cases, a first thrombosis cannot be properly prevented, and management focuses on optimal secondary prevention. In the case of atherothrombosis (thrombosis associated with atherosclerosis, like in myocardial infarction), this means lifelong antiplatelet therapy (APT) with aspirin or a P2Y12 inhibitor or both. In other settings with high risk of arterial thrombosis, like in most patients with atrial fibrillation (AF), but also in all patients with VTE, oral anticoagulation for a limited time, or lifelong (AF) administration, is indicated. These indications concern millions of subjects worldwide. In VTE, secondary prevention also tends to become lifelong in patients with unprovoked VTE.
Based on the above sketched intensive interactions between blood coagulation and cardiovascular and infectious diseases, emerging in a substantial fraction of the global population, it is both timely and important to address a number of specific challenges in future research.
A first grand challenge is the substantial risk of major bleeding including potentially fatal bleeding (e.g., intracranial) imposed on all subjects (millions worldwide) that require long-term oral anticoagulation for prevention of (recurrent) thrombosis. A feasible strategy may be to select the optimal oral anticoagulant based on clinical characteristics (e.g., age, renal function, presence of cancer or not) in conjunction with individual biomarker profiles, supporting such clinical decision making (20–22). A second strategy is to develop antithrombotic agents with a better efficacy safety profile and this approach is beginning to take shape in this era. It will be exciting to see whether it is possible to design drugs that target specific points in the hemostatic mechanism that protect against thrombosis, without causing major bleeding (23). Drugs aimed at factor XIa (24) or XIIa may, by acting just outside core enzymes in the coagulation cascade, theoretically provide safer agents, but clinical studies need to corroborate this. “New” targets including kallikrein (25) or PAR-4 on platelets (26) are just a few examples of epitopes that may be blocked to prevent thrombosis, at a lower risk of bleeding.
A second challenge is to determine whether dampening the coagulation cascade by the above strategies, could pave the way for primary prevention in more subjects, also those at risk of atherothrombosis. This should preferably be done in a tailored manner, i.e., taking into account the patient's ambient level of coagulation activity including knowledge of the relevant determinants of this coagulation profile (27). For instance, ambient coagulation may be characterized by hyperreactivity of platelets in one person or by changes in the plasmatic system in the other. In addition, variation in fibrinolytic activity may have consequences for the individual's risk of thrombosis. The net hypercoagulability may require antiplatelet therapy (APT) in one and anticoagulants, or both, in the other person. The combination of low dose rivaroxaban and aspirin is the first and most promising example of combined treatment to further reduce the risk of atherothrombosis (28). Potentially, comparable regimens would work in patients with other thrombotic disorders including those with combined arterial and venous risk factors.
Dampening ambient coagulation activity has similarities with attempts to dampen chronic inflammation. In a recent webinar on the occasion of the American Heart Association's annual meeting, Dr. Paul Ridker discussed the use of tailored anti-inflammatory treatment to dampen the inflammasome in the future, in particular, interleukin (Il)-1 beta and Il-6, as key cytokines (29). He outlined a near future of patient and biomarker tailored modification of the inflammasome to reverse residual cardiovascular (CV) risk (after having implemented management of all conventional CV risk factors like cholesterol and hypertension). For inflammation, this approach finds strong support in the data from Jupiter (stratifying on CRP), Cantos (blocking Il-1beta), and most recent studies with colchicine (30). The idea of similarly using (combined) antithrombotic drugs to also diminish the “thrombo” element of thrombo-inflammatory vascular disease by improving vascular “health” is a tempting and major challenge for the years ahead (27).
A third challenge concerns the optimization of the use of laboratory methods to improve the efficacy to safety ratio of antithrombotic medication in the cardiovascular arena. Some examples include recent studies that yielded quite impressive data regarding the genotype based, selective use of the platelet inhibitor clopidogrel in place of more potent drugs of the same class of P2Y12 inhibitors, in patients with sufficient metabolizing capacity for the prodrug clopidogrel that required a percutaneous coronary intervention (PCI) and stenting: use of the generic, hence cheaper, clopidogrel was non-inferior as compared to the standard arm, while there was a reduction in bleeding in those treated with clopidogrel (all on top of aspirin) (31). While two other studies either did not or partially supported the advantages of genotyped guided P2Y12 inhibition (32, 33). Data suggest that laboratory supported approaches including genotyping, may potentially finetune antithrombotic management. The use of platelet function testing may find a place in the safer management of patients at very high bleeding risk, i.e., frail elderly people (34).
Another example is the antithrombotic management of patients with thrombosis in conjunction with cancer and bleeding enhancing risk factors like thrombocytopenia, limiting adequate anticoagulant treatment due to bleeding risk (35). In the future, the use of tests like thrombin generation in plasma, with or without platelets, may be useful to better estimate the true clotting potential and the impact of a relative shortage of platelets (36). Moreover, the clinical application of assays that probe the function of platelets in a flow-based vessel-on-a-chip model system, could provide a valuable diagnostic addition to determine the individual's hemostatic capacity, rather than just the platelet number, an unreliable biomarker for bleeding risk (37).
A third example of where laboratory guided therapeutic management could become relevant is in the use of integral laboratory assays like thrombin generation (38), viscoelastic assays like Rotem, TEG, or other methods for studying fibrin clot formation and lysis, preferably in a point of care (POC) application (39). Although viscoelastic assays are increasingly applied in peri-operative and other high risk bleeding settings to guide transfusion management (40), such assays in conjunction with platelet function assays may help to guide prohemostatic dosing [e.g., the use of antidotes in patients bleeding on oral anticoagulants (41)] but also to optimize anticoagulant therapy. An example of the latter is the management of unfractionated heparin in extracorporeal circuits, in which the occurrence of “heparin resistance” can pose substantial management problems as now seen in severe cases of Covid-19 (42).
A fourth challenge is to elucidate the different mechanisms that underlie specific types of thrombosis. One of the current areas of interest concerns pulmonary thromboembolism. Traditionally, PE is regarded to be a consequence of venous thrombosis at distant sites, most often in the leg/pelvic veins. However, PE also occurs in the absence of overt thrombosis (isolated PE) and may occur in situ, as documented in Covid-19 patients. In Covid-19 two pathways may be operational. The first is in situ thrombosis resulting from progressive pulmonary infection and thrombo-inflammation due to a microvascular coagulopathy, in a “dose dependent” manner (the worse the disease, the more thrombosis). The second is due to “conventional” embolism from a distant site of venous thrombosis (43). These are clinically relevant for at least two reasons. First, pulmonary thrombosis forms in pulmonary arteries, and risk factors may be distinctly different from those that affect VTE. Second, isolated PE (or perhaps better pulmonary thrombosis) appears to be associated more strongly with arterial cardiovascular disease risk factors than isolated venous thrombosis (44). Thus, vascular bed and risk factor specific conditions may determine the type of thrombosis and should perhaps influence treatment and secondary prevention in different ways than currently employed. This perspective also fuels an older discussion on overlapping mechanisms between arterial and venous thrombosis (45).
Fifth, we are on the verge of better understanding the ways that selective proteases like thrombin and factor Xa direct processes of thrombo-inflammation and the impact that this crosstalk may have in several complex CVD (such as atherosclerosis, atrial fibrillation, ischemic stroke, etc.) (27, 46). The fact that millions of patients are on long term oral anticoagulants with, like with vitamin K antagonists (VKA) potential impact on the vasculature (calcification in case of VKA), cannot be overestimated (47). The challenge here is to translate the robust animal data that show that anticoagulant agents reduce atherosclerosis burden (48), to clinically relevant applications, considering human (patient) heterogeneity, including genetic background, sex, comorbidity, and medication use. Individual risk profiles and related biomarker phenotyping will again help to better stratify subsets of patients to better assess the effects of anticoagulant agents in specific patients (49). This may help to begin to understand the impact of anticoagulant medication on atherosclerosis and the potential for curing vascular disease with antithrombotic therapy, making optimal use of the “off target” (or pleiotropic) effects of such agents.
It is pivotal to understand how single proteases act on cells and which receptors are involved. Protease activated receptors (PARs) have been studied for several decades, yet, many questions remain to be answered, for example how one enzyme-like thrombin acts both in protective and offensive directions on cells (50). The intelligent use of specific mutant mice that lack target epitopes in PARs, or the use of modified proteases, will help to unravel the ways that proteases like factor Xa, VIIa, thrombin, or activated protein C signal in cells, not only in the CV system but also in other complex diseases like cancer and neuroinflammatory disorders (51, 52). These mechanistic approaches make intelligent use of expensive, but also increasingly controversial animal models, optimally employing state of the art technology like single cell RNA sequencing and high sensitivity imaging to gain detailed and fundamental new knowledge on complex biologic processes that are still hard to mimic in full in vitro.
A related, more translational sixth challenge is to make optimal use of the expanding spectrum of “omics” technology. Genomics has been around for a while now, widely applied in CVD areas like hypertension, heart failure, and atherosclerosis. Gradually, new information is emerging related to VTE (53–55). The miRNA's linked to venous thrombosis are also being identified, their properties are being explored and the clinical impact needs to be studied (56, 57). Expectations are high, but as we observed in the genomics era, one must be cautious with high expectations from the “omics” field, since GWAS studies did not produce large numbers of new candidate modifiers of venous thrombosis risk. At the same time, the new knowledge from these large and unbiased searches will reveal the candidate modifiers of thrombosis, but also uncover the networks between coagulation and other modifying systems that will also allow better characterization of the individual at risk of thrombosis. Large-scale consortium-based efforts to unravel human biology and thrombotic pathophysiology in mankind are finally taking off (58).
A final challenge is to generate the funding required to address these rather comprehensive goals. Over the past decades, clinical trials in thrombosis were substantially financed by pharmaceutical companies. However, since the registration of a generation of direct oral anticoagulants for the largest indications (AF and VTE), pharma's interest in (large trials with) antithrombotic agents has sharply diminished. This may depend on the fact that the margins for gain in benefit/risk ratio have become smaller and also be because efforts to individually tailor antithrombotic therapies have no immediate financial profit for pharma, while this is of greater interest to diagnostic companies that traditionally do not invest substantially in biomedical research. Unfortunately, governments have become more and more economical in the budgets they spend on biomedical research, without any societal justification. For now, much will rely on the creativity of researchers to work in consortia or other models of multidisciplinary, international research to assemble the best investigators for the complex issues at stake. Research has become a team sport, more than ever before. It is essential to tackle the major challenges in thrombosis with swift and concerted actions.
The author confirms being the sole contributor of this work and has approved it for publication.
HC received funding for research from Bayer and Pfizer, is a consultant to Alveron and stockholder in Coagulation Profile, and is a Principal Investigator on the following consortia sponsored by the Dutch Heart Foundation (CVON RACE-5 and CONTRAST), ZON-MW (Dutch Coalition on Thrombosis in COVID-19), and REG MED XB.
I am grateful to my research team for their continuous support and to the patients that continue to challenge my knowledge and fuel my curiosity. The Dutch Heart Foundation, Thrombosis Foundation and Gutenberg University are acknowledged for sponsoring large parts of our research.
1. ^www.WHO.int
1. Cohen AT, Agnelli G, Anderson FA, Arcelus JI, Bergqvist D, Brecht JG, et al. Venous thromboembolism (VTE) in Europe. The number of VTE events and associated morbidity and mortality. Thromb Haemost. (2007) 98:756–64. doi: 10.1160/TH07-03-0212
2. Jin S, Jin Y, Xu B, Hong J, Yang X. Prevalence and impact of coagulation dysfunction in COVID-19 in China: a meta-analysis. Thromb Haemost. (2020) 120:1524–35. doi: 10.1055/s-0040-1714369
3. Connors JM, Levy JH. COVID-19 and its implications for thrombosis and anticoagulation. Blood. (2020) 135:2033–40. doi: 10.1182/blood.2020006000
4. Kremer Hovinga JA, Coppo P, Lämmle B, Moake JL, Miyata T, Vanhoorelbeke K. Thrombotic thrombocytopenic purpura. Nat Rev Dis Primers. (2017) 3:17020. doi: 10.1038/nrdp.2017.20
5. Greinacher A. Clinical practice. Heparin-induced thrombocytopenia. N Engl J Med. (2015) 373:252–61. doi: 10.1056/NEJMcp1411910
6. Mezger M, Nording H, Sauter R, Graf T, Heim C, von Bubnoff N, et al. Platelets and immune responses during thromboinflammation. Front Immunol. (2019) 10:1731. doi: 10.3389/fimmu.2019.01731
7. Nawroth PP, Stern DM. Endothelial cell procoagulant properties and the host response. Semin Thromb Hemost. (1987) 13:391–7. doi: 10.1055/s-2007-1003516
8. Mantovani A, Dejana E. Cytokines as communication signals between leukocytes and endothelial cells. Immunol Today. (1989) 10:370–5. doi: 10.1016/0167-5699(89)90270-3
9. Cotran RS, Pober JS. Cytokine-endothelial interactions in inflammation, immunity, and vascular injury. J Am Soc Nephrol. (1990) 1:225–35.
10. van der Poll T, Levi M. Crosstalk between inflammation and coagulation: the lessons of sepsis. Curr Vasc Pharmacol. (2012) 10:632–8. doi: 10.2174/157016112801784549
11. Engelmann B, Massberg S. Thrombosis as an intravascular effector of innate immunity. Nat Rev Immunol. (2013) 13:34–45. doi: 10.1038/nri3345
12. de Groot PG, Urbanus RT. Antiphospholipid syndrome–not a noninflammatory disease. Semin Thromb Hemost. (2015) 41:607–14. doi: 10.1055/s-0035-1556725
13. Levitan N, Dowlati A, Remick SC, Tahsildar HI, Sivinski LD, Beyth R, et al. Rates of initial and recurrent thromboembolic disease among patients with malignancy versus those without malignancy. Risk analysis using Medicare claims data. Medicine. (1999) 78:285–91. doi: 10.1097/00005792-199909000-00001
14. Levi M, Ten Cate H. Disseminated intravascular coagulation. N Engl J Med. (1999) 341:586–92. doi: 10.1056/NEJM199908193410807
15. Benfield TL, Dahl M, Nordestgaard BG, Tybjaerg-Hansen A. Influence of the factor V Leiden mutation on infectious disease susceptibility and outcome: a population-based study. J Infect Dis. (2005) 192:1851–7. doi: 10.1086/497167
16. Schouten M, van't Veer C, Roelofs JJ, Levi M, van der Poll T. Impact of the factor V Leiden mutation on the outcome of pneumococcal pneumonia: a controlled laboratory study. Crit Care. (2010) 14:R145. doi: 10.1186/cc9213
17. Ganopolsky JG, Castellino FJ. A protein C deficiency exacerbates inflammatory and hypotensive responses in mice during polymicrobial sepsis in a cecal ligation and puncture model. Am J Pathol. (2004) 165:1433–46. doi: 10.1016/S0002-9440(10)63401-3
18. Nadkarni GN, Lala A, Bagiella E, Chang HL, Moreno PR, Pujadas E, et al. Anticoagulation, bleeding, mortality, and pathology in hospitalized patients with COVID-19. J Am Coll Cardiol. (2020) 76:1815–26. doi: 10.1016/j.jacc.2020.08.041
19. Brouns SH, Brüggemann R, Linkens AEMJH, Magdelijns FJ, Joosten H, Heijnen R, et al. Mortality and the use of antithrombotic therapies among nursing home residents with COVID-19. J Am Geriatr Soc. (2020) 68:1647–52. doi: 10.1111/jgs.16664
20. Overvad TF, Larsen TB, Albertsen IE, Rasmussen LH, Lip GY. Balancing bleeding and thrombotic risk with new oral anticoagulants in patients with atrial fibrillation. Expert Rev Cardiovasc Ther. (2013) 11:1619–29. doi: 10.1586/14779072.2013.839214
21. Menapace LA, McCrae KR, Khorana AA. Predictors of recurrent venous thromboembolism and bleeding on anticoagulation. Thromb Res. (2016) 140 (Suppl. 1):S93–8. doi: 10.1016/S0049-3848(16)30106-2
22. Sideris S, Archontakis S, Latsios G, Lazaros G, Toutouzas K, Tsiamis E, et al. Biomarkers associated with bleeding risk in the setting of atrial fibrillation. Curr Med Chem. (2019) 26:824–36. doi: 10.2174/0929867324666170718124742
23. Fredenburgh JC, Weitz JI. New anticoagulants: moving beyond the direct oral anticoagulants. J Thromb Haemost. (2020) 19:20–29. doi: 10.1111/jth.15126
24. Büller HR, Bethune C, Bhanot S, Gailani D, Monia BP, Raskob GE, et al. Factor XI antisense oligonucleotide for prevention of venous thrombosis. N Engl J Med. (2015) 372:232–40. doi: 10.1056/NEJMoa1405760
25. Visser M, Heitmeier S, Ten Cate H, Spronk HMH. Role of factor XIa and plasma kallikrein in arterial and venous thrombosis. Thromb Haemost. (2020) 120:883–993. doi: 10.1055/s-0040-1710013
26. Meah MN, Raftis J, Wilson SJ, Perera V, Garonzik SM, Murthy B, et al. Antithrombotic effects of combined PAR (protease-activated receptor)-4 antagonism and factor Xa inhibition. Arterioscler Thromb Vasc Biol. (2020) 40:2678–85. doi: 10.1161/ATVBAHA.120.314960
27. Ten Cate H, Guzik TJ, Eikelboom J, Spronk HMH. Pleiotropic actions of factor Xa inhibition in cardiovascular prevention - mechanistic insights and implications for anti-thrombotic treatment. Cardiovasc Res. (2020) cvaa263. doi: 10.1093/cvr/cvaa263
28. Eikelboom JW, Connolly SJ, Bosch J, Dagenais GR, Hart RG, Shestakovska O, et al. Rivaroxaban with or without aspirin in stable cardiovascular disease. N Engl J Med. (2017) 377:1319–30. doi: 10.1056/NEJMoa1709118
29. Aday AW, Ridker PM. Targeting residual inflammatory risk: a shifting paradigm for atherosclerotic disease. Front Cardiovasc Med. (2019) 6:16. doi: 10.3389/fcvm.2019.00016
30. Ridker PM. From CANTOS to CIRT to COLCOT to clinic: will all atherosclerosis patients soon be treated with combination lipid-lowering and inflammation-inhibiting agents? Circulation. (2020) 141:787–9. doi: 10.1161/CIRCULATIONAHA.119.045256
31. Claassens DMF, Vos GJA, Bergmeijer TO, Hermanides RS, van't Hof AWJ, van der Harst P, et al. A genotype-guided strategy for oral P2Y12 inhibitors in primary PCI. N Engl J Med. (2019) 381:1621–31. doi: 10.1056/NEJMoa1907096
32. Pereira NL, Farkouh ME, So D, Lennon R, Geller N, Mathew V, et al. Effect of genotype-guided oral P2Y12 inhibitor selection vs conventional clopidogrel therapy on ischemic outcomes after percutaneous coronary intervention: the TAILOR-PCI randomized clinical trial. JAMA. (2020) 324:761–71. doi: 10.1001/jama.2020.12443
33. Hulot JS, Chevalier B, Belle L, Cayla G, Khalife K, Funck F, et al. Routine CYP2C19 genotyping to adjust thienopyridine treatment after primary PCI for STEMI: results of the GIANT study. JACC Cardiovasc Interv. (2020) 13:621–30. doi: 10.1016/j.jcin.2020.01.219
34. Vries MJ, Bouman HJ, Olie RH, Veenstra LF, Zwaveling S, Verhezen PW, et al. Determinants of agreement between proposed therapeutic windows of platelet function tests in vulnerable patients. Eur Heart J Cardiovasc Pharmacother. (2017) 3:11–7. doi: 10.1093/ehjcvp/pvw026
35. Leader A, Ten Cate V, Ten Cate-Hoek AJ, Beckers EAM, Spectre G, Giaccherini C, et al. Anticoagulation in thrombocytopenic patients with hematological malignancy: a multinational clinical vignette-based experiment. Eur J Intern Med. (2020) 77:86–96. doi: 10.1016/j.ejim.2020.03.005
36. Makhoul S, Panova-Noeva M, Regnault V, Ruf W, Wenzel P, Lagrange J. Rivaroxaban effects illustrate the underestimated importance of activated platelets in thrombin generation assessed by calibrated automated thrombography. J Clin Med. (2019) 8:1990. doi: 10.3390/jcm8111990
37. Brouns SLN, Provenzale I, van Geffen JP, van der Meijden PEJ, Heemskerk JWM. Localized endothelial-based control of platelet aggregation and coagulation under flow: a proof-of-principle vessel-on-a-chip study. J Thromb Haemost. (2020) 18:931–41. doi: 10.1111/jth.14719
38. Kintigh J, Monagle P, Ignjatovic V. A review of commercially available thrombin generation assays. Res Pract Thromb Haemost. (2017) 2:42–8. doi: 10.1002/rth2.12048
39. Carll T, Wool GD. Basic principles of viscoelastic testing. Transfusion. (2020) 60 (Suppl. 6):S1–9. doi: 10.1111/trf.16071
40. Santos AS, Oliveira AJF, Barbosa MCL, Nogueira JLDS. Viscoelastic haemostatic assays in the perioperative period of surgical procedures: systematic review and meta-analysis. J Clin Anesth. (2020) 64:109809. doi: 10.1016/j.jclinane.2020.109809
41. Taune V, Skeppholm M, Ågren A, Gryfelt G, Malmström RE, Wikman A, et al. Rapid determination of anticoagulating effects of dabigatran in whole blood with rotational thromboelastometry and a thrombin-based trigger. J Thromb Haemost. (2018) 16:2462–70. doi: 10.1111/jth.14308
42. White D, MacDonald S, Bull T, Hayman M, de Monteverde-Robb R, Sapsford D, et al. Heparin resistance in COVID-19 patients in the intensive care unit. J Thromb Thrombolysis. (2020) 50:287–91. doi: 10.1007/s11239-020-02145-0
43. Maiese A, Manetti AC, La Russa R, Di Paolo M, Turillazzi E, Frati P, et al. Autopsy findings in COVID-19-related deaths: a literature review. Forensic Sci Med Pathol. (2020) 1–18. doi: 10.1007/s12024-020-00310-8
44. Ten Cate V, Eggebrecht L, Schulz A, Panova-Noeva M, Lenz M, Koeck T, et al. Isolated pulmonary embolism is associated with a high risk of arterial thrombotic disease: results from the VTEval study. Chest. (2020) 158:341–9. doi: 10.1016/j.chest.2020.01.055
45. Prandoni P. Is there a link between venous and arterial thrombosis? A reappraisal. Intern Emerg Med. (2020) 15:33–6. doi: 10.1007/s11739-019-02238-6
46. Ten Cate H, Hackeng TM, García de Frutos P. Coagulation factor and protease pathways in thrombosis and cardiovascular disease. Thromb Haemost. (2017) 117:1265–71. doi: 10.1160/TH17-02-0079
47. Olie RH, van der Meijden PEJ, Spronk HMH, Ten Cate H. Antithrombotic therapy: prevention and treatment of atherosclerosis and atherothrombosis. Handb Exp Pharmacol. (2020). doi: 10.1007/164_2020_357
48. Posma JJ, Grover SP, Hisada Y, Owens AP 3rd, Antoniak S, Spronk HM, et al. Roles of coagulation proteases and PARs (protease-activated receptors) in mouse models of inflammatory diseases. Arterioscler Thromb Vasc Biol. (2019) 39:13–24. doi: 10.1161/ATVBAHA.118.311655
49. d'Alessandro E, Becker C, Bergmeier W, Bode C, Bourne JH, Brown H, et al. Thrombo-inflammation in cardiovascular disease: an expert consensus document from the third maastricht consensus conference on thrombosis. Thromb Haemost. (2020) 120:538–64. doi: 10.1055/s-0040-1708035
50. Coughlin SR. Protease-activated receptors in hemostasis, thrombosis and vascular biology. J Thromb Haemost. (2005) 3:1800–14. doi: 10.1111/j.1538-7836.2005.01377.x
51. Ruf W, Graf C. Coagulation signaling and cancer immunotherapy. Thromb Res. (2020) 191 (Suppl. 1):S106–11. doi: 10.1016/S0049-3848(20)30406-0
52. Lazic D, Sagare AP, Nikolakopoulou AM, Griffin JH, Vassar R, Zlokovic BV. 3K3A-activated protein C blocks amyloidogenic BACE1 pathway and improves functional outcome in mice. J Exp Med. (2019) 216:279–93. doi: 10.1084/jem.20181035
53. Zöller B, Svensson PJ, Dahlbäck B, Lind-Hallden C, Hallden C, Elf J. Genetic risk factors for venous thromboembolism. Expert Rev Hematol. (2020) 13:971–81. doi: 10.1080/17474086.2020.1804354
54. Hansen ES, Hindberg K, Latysheva N, Aukrust P, Ueland T, Hansen JB, et al. Plasma levels of growth differentiation factor 15 are associated with future risk of venous thromboembolism. Blood. (2020) 136:1863–70. doi: 10.1182/blood.2019004572
55. Desch KC, Ozel AB, Halvorsen M, Jacobi PM, Golden K, Underwood M, et al. Whole-exome sequencing identifies rare variants in STAB2 associated with venous thromboembolic disease. Blood. (2020) 136:533–41. doi: 10.1182/blood.2019004161
56. Thibord F, Munsch G, Perret C, Suchon P, Roux M, Ibrahim-Kosta M, et al. Bayesian network analysis of plasma microRNA sequencing data in patients with venous thrombosis. Eur Heart J Suppl. (2020) 22 (Suppl. C):C34–45. doi: 10.1093/eurheartj/suaa008
57. Hembrom AA, Srivastava S, Garg I, Kumar B. MicroRNAs in venous thrombo-embolism. Clin Chim Acta. (2020) 504:66–72. doi: 10.1016/j.cca.2020.01.034
Keywords: thrombosis, anticoagulant (MeSH), coagulation factor, protease activated receptor, bleeding
Citation: ten Cate H (2021) Thrombosis: Grand Challenges Ahead! Front. Cardiovasc. Med. 8:637005. doi: 10.3389/fcvm.2021.637005
Received: 02 December 2020; Accepted: 29 March 2021;
Published: 04 May 2021.
Edited and reviewed by: Hendrik Tevaearai Stahel, Bern University Hospital, Switzerland
Copyright © 2021 ten Cate. This is an open-access article distributed under the terms of the Creative Commons Attribution License (CC BY). The use, distribution or reproduction in other forums is permitted, provided the original author(s) and the copyright owner(s) are credited and that the original publication in this journal is cited, in accordance with accepted academic practice. No use, distribution or reproduction is permitted which does not comply with these terms.
*Correspondence: Hugo ten Cate, aC50ZW5jYXRlQG1hYXN0cmljaHR1bml2ZXJzaXR5Lm5s
Disclaimer: All claims expressed in this article are solely those of the authors and do not necessarily represent those of their affiliated organizations, or those of the publisher, the editors and the reviewers. Any product that may be evaluated in this article or claim that may be made by its manufacturer is not guaranteed or endorsed by the publisher.
Research integrity at Frontiers
Learn more about the work of our research integrity team to safeguard the quality of each article we publish.