- 1Section of Vascular Surgery, Department of Surgery, Washington University School of Medicine, St. Louis, MO, United States
- 2Division of Cardiothoracic Surgery, Department of Surgery, Washington University School of Medicine, St. Louis, MO, United States
- 3Division of Molecular Cell Biology, Washington University School of Medicine, St. Louis, MO, United States
- 4Department of Biomedical Engineering, McKelvey School of Engineering, Washington University, St. Louis, MO, United States
- 5Veterans Affairs St. Louis Health Care System, St. Louis, MO, United States
The coronavirus disease-2019 (COVID-19) pandemic has rapidly spread across the world. The disease is caused by severe acute respiratory syndrome coronavirus 2 (SARS-CoV-2), which first appeared in Wuhan, China in December, 2019. Ever increasing data is continuing to emerge about the impact of COVID-19 on cardiovascular tissue and other organ system. Clinical features associated with COVID-19 suggest that endothelial cell dysfunction and microvascular thrombosis are to a large extent contributing to resultant multi-organ complications. This review is aimed at highlighting the critical aspects associated with COVID-19 and its presumed microvascular angiopathic consequences on the cardiovascular system leading to multi-organ dysfunction.
Introduction
Coronaviruses (CoVs) are enveloped, non-segmented, single strand positive sense RNA viruses that are widely distributed among humans and other mammals. This group of viruses belongs to the coronaviridae family of viruses, and are harbored in animals such as civets, dogs, cats, bats, and camels (1–3). To-date there are six coronaviruses (CoVs) known to infect humans and these include; 229E, OC43, NL63, HKU1, Middle East Respiratory Syndrome Coronavirus (MERS-CoV), and severe acute respiratory syndrome coronavirus (SARS-CoV) (1, 3, 4). The 2002–2003 pandemic in Guangdong, Southern China was caused by SARS-CoV-1 (5), and resulted in >8,000 human infections, and 774 deaths in 37 countries (6). More recently in 2012 and 2015, MERS-CoV was responsible for the outbreaks that caused nearly 2,500 infections, and >850 deaths (7, 8). Although another CoV outbreak was predicted by various organizations, the scale of a resultant global pandemic was not completely anticipated.
The coronavirus disease-2019 (COVID-19) is a novel emerging infectious disease that is caused by SARS-CoV-2. COVID-19 was declared a global pandemic by the World Health Organization on March 11, 2020 (9). This viral disease first appeared in Wuhan, Hubei province, China in December 2019. According to the Johns Hopkins University Coronavirus resource center dashboard, as of September 2020 the disease was been confirmed in 188 countries, infected >26 million individuals, and caused >800,000 deaths world-wide (10). In the United States (US) alone there was >6 million confirmed cases, and >180,000 deaths (10).
Like other SARS-CoV, COVID-19 can cause a severe pulmonary viral pneumonia leading to severe hypoxia and subsequent respiratory failure. However, emerging evidence demonstrates that unlike the majority of upper respiratory viral illness, COVID-19 can also lead to cardiovascular and multi-organ complications (11–13). Although the basic biological understanding of these complications is still evolving and yet to be fully elucidated, there is emerging evidence that demonstrates that COVID-19 can cause microvascular angiopathy in various organ systems and tissue beds—including cardiovascular tissue (14, 15).
Basic Biology
The SARS-CoV2 has been reported to bind to angiotensin converting enzyme 2 (ACE2) protein (16). Specifically, it was determined that the S1 domain of the SARS-CoV S protein can efficiently bind to the ACE protein on living cells. In these experiments, ACE2 antibodies were found to effectively block viral replication, but ACE1 antibodies did not have an impact on viral replication (16). Therefore, this data convincingly demonstrated that the ACE2 protein served as a critical attachment and entry vehicle for SARS-CoV2 viral particles into mammalian cells (Figure 1).
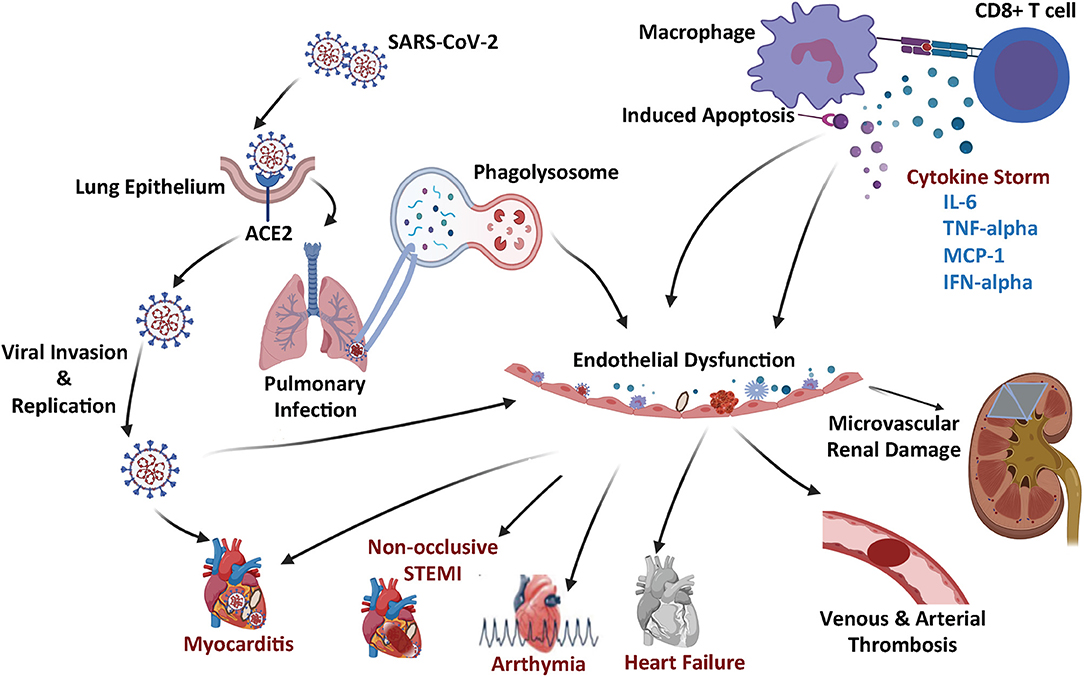
Figure 1. Schematic summary of the COVID-19 microangiopathic consequences leading to myocarditis, non-occlusive STEMI, arrhythmia, heart failure, vascular thrombosis, and microvascular renal dysfunction.
Upon binding onto the cell membrane ACE2 protein, the SARS-CoV2 virus enters the cell via a receptor mediated endocytosis and uses the host cell nuclear machinery to replicate (17), which produces multiples of virion replicates triggering an inflammatory response leading to the production of various cytokines, including but not limited to Interleukin-1beta (L-1β), interferon gamma (IFN-γ), and Monocyte Chemoattractant Protein-1 (MCP-1) (Figure 1). The chemokine (C-C mofit) ligand 2 (CCL2) also known as MCP-1 binds to its receptor on the surface of the microvascular ECs cells and pro-inflammatory macrophages (18, 19), further triggering more cytokine release and recruitment of additional inflammatory cells [such as monocytes, CD4 T helper cells, CD8 T cells, and Natural killer (NK) cells]. The resultant release of additional cytokines leads to a severe cytokine storm, which causes significant cellular and tissue damage (18).
The ACE2 mRNA is detectable in virtually all mammalian tissue, however, the protein is most remarkably expressed on the cell surface of lung alveolar epithelial cells and intestinal enterocytes (17). The protein is also highly expressed on the arterial and venous endothelium (17). Even prior to the COVID-19 pandemic, it was speculated that SARS-CoV2 viruses could potentially lead to vascular dysfunction, endothelial cell damage, and microvascular thrombosis given the relative abundance of ACE2 on the cell surface of endothelial cells (15, 20).
Microvascular Angiopathy
Microvascular angiopathy is a pathological sequelae of a multitude of conditions that results from over-activation of host immune defense mechanisms. Patients impacted by sepsis or septic shock from a bacterial or viral infection, or autoimmune process, may fall victim to hyperimmune responses that can lead to significant host consequences (21–25). For example, dengue viral infections can lead an infected host to mount a hyperimmune response that results in activation of M1 macrophages and the release of pro-inflammatory cytokines such as TNFα, IL-1β, and IL-6 (23, 24). These pro-inflammatory mediators can directly act on the endothelium leading to acute cellular dysfunction, loss of endothelial barrier function and increased vascular leakage, and higher risk of microvascular thrombosis. As a consequence patients afflicted by dengue fever can sometimes present with clinical manifestations of multi-organ dysfunction, including acute respiratory distress syndrome (ARDS), myocarditis, acute kidney injury (AKI), and skin changes such as petechiae, bruising, and purpura (23).
Bacterial infections such as Lyme disease (Borrelia burgdorferi) and Rocky Mountain spotted fever (RMSF; caused by Rickettsia rickettsii) also can lead to severe cytokine storm, overexpression of CCL2, and resultant microvascular injury. In presence of such infections, patients can often develop microvascular dysfunction and thrombosis, as well as over-activation of the host fibrinolytic system (21, 22, 25, 26).
Prior literature establishes a clear interplay between inflammation, hypercoagulation, and thrombosis. Presumably part of this interplay is mediated by cytokine storm that leads to a higher propensity to development disseminated intravascular coagulopathy (DIC) (27). The systemic activation of the coagulation pathways by mediators generated during cytokine storm, can lead to a prothrombotic state characterized by the deposition of microthrombi, diffuse capillary obstruction, and the resultant tissue ischemia and organ damage. The tissue damage leads to further inflammation, and hence a vicious cycle ensues with additional inflammation leading to further coagulopathy, tissue damage, and increased risk of morbidity and mortality (27, 28). Current evidence estimates that >71% of patients who died from COVID-19 met the International Society on Thrombosis and Hemostasis (ISTH) criteria for DIC (29). Coagulation profiles for these individuals were typically notable for higher serum D-dimers, lower antithrombin III levels, and higher fibrin degradation products compared to healthy controls (30).
Clinical Features
Since the emergence of the pandemic, there has been a multitude of clinical series published regarding the clinical manifestations and outcomes of COVID-19 (12, 31–34). The majority of the initial symptoms were related to the upper respiratory tract and gastrointestinal system, and varied significantly in severity between affected subjects. The common initial symptoms included; cough, dyspnea, fatigue, and myalgia (35). Additional minor symptoms included; headaches, and diarrhea (36).
The median incubation period has been reported to be 5 days and individuals who develop symptoms do so within approximately 11.5 days of infection (37). The median time from onset of initial symptoms to dyspnea is 8 days (38, 39). In some cases, the disease rapidly progressed to ARDS and septic shock, while in others, the disease took a milder course (38). Critically ill patients who required mechanical ventilation (15/21; 71%) typically presented with major multi-organ complications (31, 32, 40). These included non-atherosclerotic ST-elevation myocardial infarction, interstitial pulmonary tissue edema, AKI, brainstem infarcts, liver dysfunction, skin petechial rashes, and gastrointestinal mucosal bleeding—which were often associated with worse clinical outcomes (31).
Organ System Complication
Cardiac
In addition to the known initial pulmonary consequences of COVID-19, various reports have demonstrated that patients infected with SARS-CoV2 can develop cardiac dysfunction and myocardial injury (41, 42). The mechanism of myocardial injury is unclear, but several have been proposed that severe respiratory dysfunction and consequential hypoxemia leads to increased myocardial demand, mixed severe respiratory and metabolic acidosis, which then results in myocardial injury and cellular apoptosis (12). Others propose that severe cytokine storm resulting from the host hyper-immune response to the primary SARS-CoV2 infection leads to secondary myocarditis, acute heart failure, malignant arrhythmias, and demand ischemia (Figures 2, 3) (43, 44). In addition, emerging reports suggest that SARS-CoV2 may act directly on myocardial tissue since ACE2 is presumed to be expressed on the myocardium with adequate quantities (41, 45). Primary viral myocarditis can occur with viruses such as cytomegalovirus (CMV) and Rubella but has not yet been confirmed with SARS-CoV infections (46–48). The occurrence of primary COVID-19 induced myocarditis would be a novel finding and may be consistent with recently reported echocardiographic findings that resemble other primary infectious myocardial complications such as Kawasaki syndrome (49, 50).
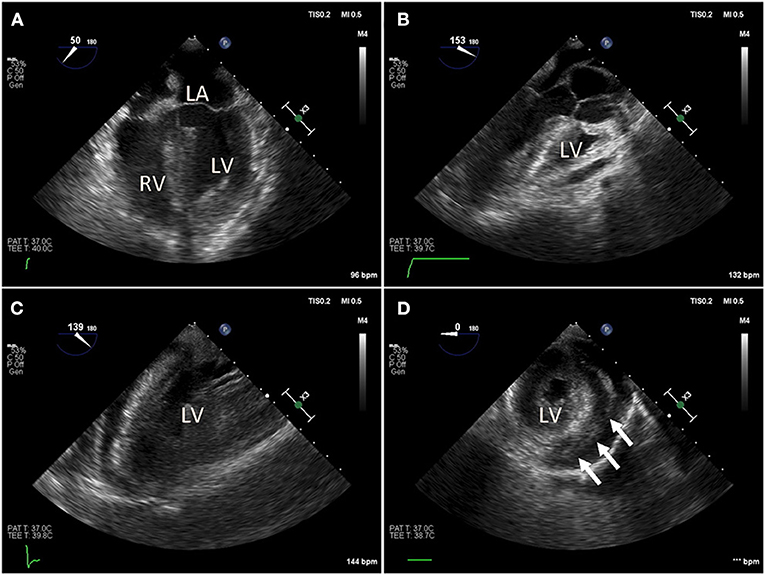
Figure 2. Intraoperative trans-esophageal echocardiography (TEE) in a COVID-19 positive patient with myocarditis, acute pericardial effusion and cardiac tamponade. After bedside venous-arterial extracorporeal membrane oxygenation (VA-ECMO) cannulation was performed, the patient was taken to the operating room emergently for ventral cardiac window exposure and decompression of the pericardial effusion. (A) Preoperative mid-esophageal four chamber view demonstrated severely reduced global left ventricle (LV) function. (B,C) Similarly, mid-esophageal long axis view demonstrated severely reduced LV contraction. (D) Transgastric short axis view demonstrated under-filling of the LV. Patient was taken emergently to the operating room for cardiac window decompression of the pericardial effusion and a large pericardial effusion. An Impella device is seen in the LV.
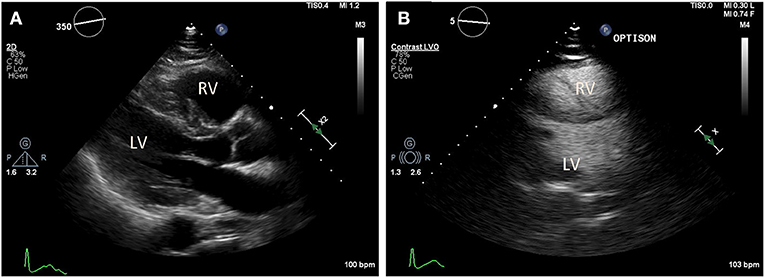
Figure 3. Transthoracic echocardiography (TTE) in a COVID-19 positive patient who presented with acute bilateral pulmonary emboli and acute right sided heart failure requiring emergent veno-venous extracorporeal membrane oxygenation (VV-ECMO) cannulation. (A) Parasternal long axis view demonstrate a McConnell's sign: Right ventricle (RV) enlargement and hypokinesis with preserved apical contractility. (B) Optison enhanced images demonstrate RV enlargement, and paradoxical septal motion consistent with RV dysfunction.
The incidence of acute cardiac injury in patients hospitalized with COVID-19 ranges between 7 and 17% (33, 35, 39). In one single-center series of 138 patients, individuals who were critically ill with COVID-19, were found to be more likely to have elevated myocardial injury serum biomarkers such as Troponin I and CK-MB (35). In another series of 120 patient hospital admissions due to COVID-19, 27.5 and 10% of the patients were found to have elevated levels of N-terminal pro B-type natriuretic peptide (NT-proBNP) and Troponin I, respectively (11). And, in another review of 187 patients with COVID-19, it was observed that 27.8% of patients who had myocardial injury and elevated cardiac serum biomarkers, had developed organic evidence of cardiac dysfunction and arrhythmias (51). Notably, in this cohort, patients with elevated serum Troponin I also had a 6.7 times higher odds of mortality. Non-occlusive STEMI is presumed to be the cause of cardiac enzyme release, suggesting that the primary cardiac pathology in the setting of COVID-19 may not be due to coronary artery thromboembolic obstruction (52, 53). The increased risk of mortality from cardiac factors also appears to be notably higher among individuals with baseline cardiovascular risk factors such as coronary heart disease, hypertension, and chronic cardiomyopathy (41, 51). Whether these baseline cardiovascular risk factors in some way are contributing to the primary COVID-19-induced pathology, rather than just serving as pre-disposing factors, is the subject of ongoing investigations.
Cardiac arrhythmias have also been reported as common complication in patients afflicted by COVID-19. One study of 121 patients, it was reported that >71% of patients experienced periods of sinus tachycardia, >14% of patients experienced bradycardia, and 1 patient developed new-onset transient atrial fibrillation (49). Other reports have cited development of malignant tachyarrhythmias is a strong predictor of mortality in patients infected with COVID-19 (11, 12, 35, 54). In one study the incidence of malignant ventricular arrhythmias was 75% more likely to be observed in patients with elevated serum Troponin (51). It is currently unclear whether these diverse types of arrhythmias are primarily a complication of COVID-19 infection itself, adverse effect of medication administered for treatment of COVID-19, or whether they are a result of exacerbation of the previously underlying heart disease.
Similarly, heart failure and fulminant myocarditis have been reported in a subset of patients infected with COVID-19. An analysis of 191 patients, reported that heart failure was observed in 23% of patients who were hospitalized due to COVID-19 infection. Patients with heart failure were noted to have an elevated (up to 28%) risk of mortality (33). Several reports also demonstrate clinical evidence of fulminant myocarditis with associated cardiogenic shock (42, 51). Preliminary evidence suggested that these conditions responded with hemodynamic improvement and stability following therapy with methylprednisolone, immunoglobulins, milrinone, and diuresis (42). It is not entirely clear whether resultant heart failure in these acute settings is an exacerbation of pre-existing heart disease or if it is due to the COVID-19 related myocarditis and stress-induced cardiomyopathy (55).
Various treatment modalities have been employed in the treatment of patients with COVID-19 and cardiac manifestations. Antivirals such as ribavirin, remdesivir, and oseltamivir, and antibiotics such as ceftriaxone, azithromycin, and immunoglobulins to modulate the immune status have also been reported with variable success (11, 49, 54). In addition, there have been reports on the utilization of invasive and non-invasive ventilation, extracorporeal membrane oxygenation, and intra-aortic balloon pump as rescue therapy maneuvers (11, 12, 35, 42).
Brain and Neurologic
Various reports have cited evidence to suggest microvascular dysfunction in the central nervous system of patients with severe COVID-19 infections (56–60). The signs and symptoms associated with COVID-19 related brain dysfunction include; headache, seizures, anosmia and hypogeusia, impaired consciousness, ataxia, delirium, paralysis, and strokes (36, 56, 61, 62). A review of 214 patients from Wuhan, China, who were admitted to the hospital due to COVID-19 infections, demonstrated that 36.4% had neurologic manifestations. Patients with severe infection were more likely to have neurologic manifestations such as acute strokes (5.7%), and altered consciousness 14.8% (61). Brain computed tomographic (CT) scans confirmed the presence of large ischemic strokes in five patients, and one patient with intracranial hemorrhage (61). In another review of 219 Chinese patients, the authors also reported findings of acute ischemic stroke on head CT in 4.6% patients (63). Similar large ischemic stroke complications were also reported among patients treated in the New York Health System (64).
The ACE2 receptor is expressed on brain endothelial and smooth muscle cells (17, 56). Therefore, it is presumed that COVID-19 viral particles may be able to directly infect microvascular structures in the central nervous system, and lead to the observed neurological complications. This was confirmed by a report by Paniz-Mondolfi et al. (65) that demonstrated using transmission electron microscopy the presence of viral particles in small vesicles of brain endothelial cells. In another study involving 50 postmortem cases, a varied range of histopathological findings in the brain including focal and/or diffuse cortical, brainstem, and leptomeningeal inflammation (36%), encephalitis with localized perivascular and interstitial infiltrates with axonal degeneration and neuronal cell loss (12%), and inflammatory T-cell infiltrates with clusters of macrophages and axonal injury (66). These findings suggest that COVID-19-induced microvascular angiopathy can directly lead to brain and nerve tissue injury. It remains unclear which what patient populations are most prone to these complications, and what pre-disposing factors may lead to the more serious brain ischemic stroke manifestations.
Liver
The ACE2 receptor is highly expressed in the gastrointestinal epithelium, and in liver cholangiocytes and arterial endothelium. However, it is not as highly expressed in the liver sinusoidal endothelium (67–69). A large subsets of patients infected with COVID-19 are noted to have liver pathology and transaminitis during the course of their hospitalizations (68–72). Reverse Transcriptase-Polymerase Chain Reaction (RT-PCR) has confirmed the presence of SARS-CoV genome in hepatic tissue, suggesting that COVID-19 may be directly binding to the liver microvasculature and causing hepatic complications (73, 74). Post-mortem studies of patients who died following COVID-19 infections demonstrated platelet-fibrin micro-thrombi within the hepatic microvasculature, various degrees of steatosis and portal histiocytic hyperplasia, and hepatic tissue ischemic necrosis (75). Another study reported patchy necrosis of the liver as well as wide infiltration by small lymphocytes (76). In a study of 10 postmortem examinations, the authors reported minimal periportal lymphoplasmacellular infiltration and significant fibrosis within the liver body (77). Although these studies have collectively examined a small subset of patients, they provide interesting insights into additional microangiopathic complications that may occur in the liver due to COVID-19 infection. Further studies may surely focus on potential disposing factors, or the contribution of underlying pathologies, in the resulting range of observed liver complications in patients who are acutely infected or chronically recovered from COVID-19.
Renal
Initial reports from Wuhan, China informed us that AKI occurred in 7% of individuals who are COVID-19 positive (39). In patients who developed severe infection and required hospitalization, kidney abnormalities were observed in 25–50% of patients, and manifestations included acute proteinuria and hematuria (78). In a prospective cohort of 701 patients, from three different hospital centers in China, the authors observed that 43.9% of patients had proteinuria and 26.7% had hematuria, and were reported as adjusted risk factors for in-hospital death (79).
The ACE2 receptor is known to be expressed in the renal capillary endotheilum, and is thought to lead to localized tissue inflammation due to COVID-19 infections. Electron microscopy of renal capillary endothelium demonstrated COVID-19 viral inclusion structures, and on tissue histology inflammatory cells were visualized to be associated with endothelium—consistent with endothelitis (17). In a study reviewing renal histopathology from 26 patients who died following COVID-19 infections, there was histological evidence of diffuse proximal tubule injury, red blood cell aggregates obstructing capillary lumens, and clusters of viral particles in the tubular epithelium (80).
Several mechanisms of COVID-19 induced renal injury have been proposed. One potential mechanism may simply be due to hypo-perfusion from dehydration and diarrhea related to the systemic inflammatory (81–83). However, this mechanism would not necessarily explain findings of proteinuria and hematuria, which are not typically observed with other pre-renal conditions (83). An alternative hypothesis is that COVID-19 induced endothelial dysfunction may be disruptive to the vascular hemostatic equilibrium that shifts it toward a more vasoconstrictive state. This microangiopathic effect can lead to acute tissue malperfusion, ischemia, and a pro-coagulation state (84, 85).
The care of patients impacted by COVID-19 related AKI remains largely supportive. Depending on the severity of the presentation, patients may be managed with renal replacement therapy (RRT) in the form of intermittent hemodialysis or continuous renal replacement therapy (CRRT), or slow low efficiency dialysis (SLED). In the current absence of data clearly demonstrating superiority of one modality over another, the choice of dialysis modality is mostly informed by the availability of resources and local clinical expertise (82).
Venous Thromboembolism (VTE)
Coagulopathy is a common phenomenon that can occur with sepsis and may predict outcomes in patients with severe pulmonary infections and multisystem organ dysfunction (86). Several reports indicate that patients with COVID-19 infections who are critically ill are at increased risk of developing VTE, including deep venous thrombosis (DVT) and pulmonary emboli (PE) (87–89). In a single center cohort study of 198 patients, 20% of patients were diagnosed with VTE and with extensive symptomatic thrombophlebitis for which anticoagulation treatment was initiated (90). The cumulative incidences of VTE at 7, 14, and 21 days was found to be 16, 33, and 42%, respectively (90). The authors also reported that VTE was significantly associated with death, with a hazard ratio of 2.7. In a separate single center study of 147 patients admitted to hospital for COVID-19, 17% were found to have VTE (91). Of these patients, 64% had acute PE, and 56% had acute DVT, and the all-cause mortality in these patients was significantly greater when compared to patients with no VTE (48% vs. 22%) (Figure 4) (91).
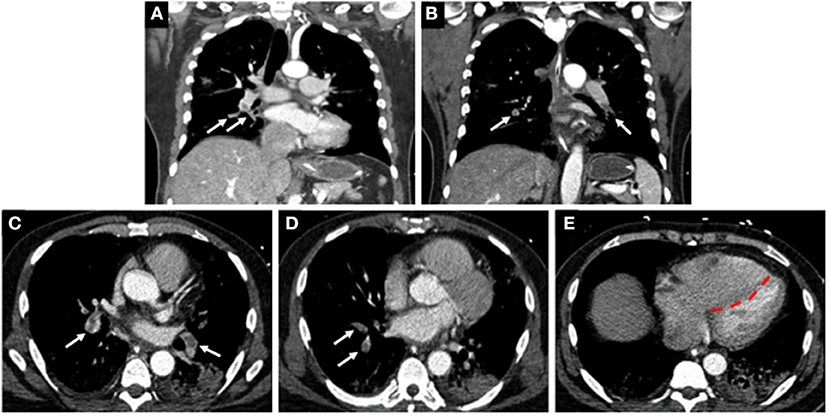
Figure 4. Computed tomographic (CT) images of a COVID-19 positive patient who presented with bilateral lobar pulmonary embolism (white arrows; A–D), and right ventricular enlargement and bowing of the interventricular septum to the left, consistent with right heart strain (red line; E).
Some screening serum biomarkers have been proposed to monitor severity and incidence of VTE. In one study an admission D-dimer level >1,500 ng/mL was found to be an independent marker associated with the incidence of VTE (91). In another retrospective single center study with a total of 81 patients diagnosed with severe COVID-19 pneumonia admitted to the intensive care unit (ICU), D-dimer level >1,500 ng/mL again was a good index for identifying high risk groups (92). Similarly, in another study of 150 patients who were admitted to ICU due to COVID-19 infections, serum Von Willebrand (vWF) activity, vWF antigen, and FVIII were considerably increased, and 87.7% had positive lupus anticoagulant (93).
Multiple studies have demonstrated an overall higher index of morbidity and mortality in patients affected by VTE (94–96). For example, in a Dutch cohort of 184 patients who were admitted to ICU with COVID-19, the authors observed an adjusted cumulative incidence of symptomatic acute PE, DVT, ischemic stroke, myocardial infarction and/or systemic arterial embolism of 49% (96). Notably, the majority of the thrombotic events were PE (87%) in nature (96). Patients with thrombotic complications were at a higher risk of all-cause mortality (HR 5.4) and the use of therapeutic anticoagulation was associated with a 21% increased likelihood of survival (all-cause mortality HR 0.79) (96).
Treatment Strategies
The treatment of COVID-19 associated microvascular angiopathies and their effects on the cardiovascular system is yet to be defined, but various strategies can potentially be derived from treatments used for management of other microvascular angiopathies. Conditions such as Kawasaki's disease, Henoch-Schonlein purpura, Rheumatoid Arthritis and Buerger's disease, are typically managed by targeting the underlying disorder and palliating symptoms (97–101). Some are vasculitidies responsive to treatment with immunoglobulins, calcium channel blockers, thrombolytics, prostaglandin analogs, glucocorticoids, and other immune-modulators such as methotrexate (97, 99, 101). Various such agents have been used in the battle against COVID-19 with variable success (12, 29, 89, 102–107).
Anti-virals
On May 1st, 2020 the US Food and drug administration (FDA) issued an emergency use authorization of Remdesivir for adults and children with suspected or laboratory confirmed severe COVID-19 (102). Remdesivir binds and inhibits the RNA-dependent RNA polymerase that is essential for SARS-CoV2 viral replication (104). In a study of 61 patients with confirmed severe COVID-19 and evidence of hypoxia (56% receiving mechanical ventilation and 8% receiving veno-venous or veno-arterial extracorporeal bypass), at least one dose of remdesivir on a compassionate use basis, demonstrated clinical improvement and improved heart function in 68% of patients (108). Similarly, in a randomized double-blind placebo-controlled multicenter trial, comprising of 237 patients, the authors reported that remdesivir was not associated with statistically significant clinical benefits, however, the numerically faster time to clinical improvement requires confirmation in larger studies (109). Other antivirals like lopinavir and ritonavir (used for treatment of HIV), ribavirin (used for treatment of Hepatitis C), are under clinical investigation for the treatment of COVID-19 (102, 105, 110).
Anti-coagulation
Anticoagulants have recently emerged as an important treatment modality for patients infected with COVID-19, and in particular patients who are considered higher risk individuals (111). Various institutional guidelines have recommended initiation of prophylactic or therapeutic anticoagulation in patients impacted by COVID-19 (112–115). In one retrospective study, it was observed that patients who received either prophylactic or therapeutic anticoagulation had lower incidence of myocardial dysfunction, mechanical ventilation, and in-hospital mortality (114). In another study, of 2,773 COVID-19 positive patients who were receiving mechanical ventilation, the hospital mortality rate was significantly reduced in patients that received therapeutic anticoagulation (62.7 vs. 29.1%) (116). Despite these clear advantages in the application of therapeutic anticoagulation in critically ill patients afflicted with COVID-19, there remains important unanswered questions about the role, duration of therapy, and potential consequences of anticoagulation therapy. It is also unclear whether anticoagulation therapy may be used to help in prophylaxis against COVID-19-induced multi-organ dysfunction.
Steroids
Steroid therapy has been used for the treatment of severe ARDS due to COVID-19 infection (12). In a multi-center study of 213 patients with moderate to severe COVID-19 related symptoms, it was found that patient who were treated with methylprednisolone had a reduced incidence of escalation of care and improved clinical and cardiac outcomes (117). Similarly, in a multicenter partially randomized, preference open label trial, patients with a COVID-19 pneumonia, impaired gas exchange, and biochemical evidence of hyper-inflammation, demonstrated reduced incidence of death, cardiac dysfunction, ICU admission, and non-invasive ventilation following treatment with methylprednisolone (118). In another multicenter observational study, methylprednisolone administration in patients with severe COVID-19 pneumonia significantly lowered rates of ventilator dependence, myocardial infarction, and death (119).
Conclusion
The body of evidence demonstrating the role of microvascular angiopathy in COVID-19 is evolving rapidly. It is evident from the current body of knowledge that COVID-19-induced microvascular angiopathy can lead to a wide range of tissue pathology and clinical complications. Understanding the molecular, biochemical and pathophysiological mechanisms underlying this process is paramount to our ongoing management efforts of individuals afflicted by COVID-19. Additional research is necessary to help further our understanding of microvascular angiopathic complications related to COVID-19, help develop novel therapeutics, and provide an effective cure to this new global pandemic.
Author Contributions
All authors listed have made a substantial, direct and intellectual contribution to the work, and approved it for publication.
Funding
This work was supported by the Veterans Affairs Clinical Science Research and Development Award COVID-19-8900-13 (MZ), and NIH/National Heart, Lung, and Blood Institute (NHLBI) Grant No. K08 HL132060 (MZ), and NIH/NHLBI Grant No. R01 HL150891-01 (MZ).
Conflict of Interest
The authors declare that the research was conducted in the absence of any commercial or financial relationships that could be construed as a potential conflict of interest.
References
1. Su S, Wong G, Shi W, Liu J, Lai ACK, Zhou J, et al. Epidemiology, genetic recombination, and pathogenesis of coronaviruses. Trends Microbiol. (2016) 24:490–502. doi: 10.1016/j.tim.2016.03.003
2. Gorbalenya AE, Enjuanes L, Ziebuhr J, Snijder EJ. Nidovirales: evolving the largest RNA virus genome. Virus Res. (2006) 117:17–37. doi: 10.1016/j.virusres.2006.01.017
4. Kin N, Miszczak F, Lin W, Gouilh MA, Vabret A, Consortium E. Genomic analysis of 15 human coronaviruses OC43 (HCoV-OC43s) circulating in France from 2001 to 2013 reveals a high intra-specific diversity with new recombinant genotypes. Viruses. (2015) 7:2358–77. doi: 10.3390/v7052358
5. Ge XY, Li JL, Yang XL, Chmura AA, Zhu G, Epstein JH, et al. Isolation and characterization of a bat SARS-like coronavirus that uses the ACE2 receptor. Nature. (2013) 503:535–8. doi: 10.1038/nature12711
6. Cherry JD. The chronology of the 2002-2003 SARS mini pandemic. Paediatr Respir Rev. (2004) 5:262–9. doi: 10.1016/j.prrv.2004.07.009
7. Zaki AM, van Boheemen S, Bestebroer TM, Osterhaus AD, Fouchier RA. Isolation of a novel coronavirus from a man with pneumonia in Saudi Arabia. N Engl J Med. (2012) 367:1814–20. doi: 10.1056/NEJMoa1211721
8. Lee J, Chowell G, Jung E. A dynamic compartmental model for the Middle East respiratory syndrome outbreak in the Republic of Korea: a retrospective analysis on control interventions and superspreading events. J Theor Biol. (2016) 408:118–26. doi: 10.1016/j.jtbi.2016.08.009
9. World Health Organization. WHO Director-General's Opening Remarks at the Media Briefing on COVID-19. (2020). [updated March 11, 2020; cited 2020 April 1]. Available online at: https://www.who.int/dg/speeches/detail/who-director-general-s-opening-remarks-at-the-media-briefing-on-covid-19-−11-march-2020 (accessed November 15, 2020).
10. Johns Hopkins University & Medicine. COVID-19 Dashboard by the Center for Systems Science and Engineering (CSSE) at Johns Hopkins University (JHU). (2020) [updated April 17, 2020; cited 2020 April 1]. Available online at: https://coronavirus.jhu.edu/map.html (accessed November 15, 2020).
11. Chen C, Zhou Y, Wang DW. SARS-CoV-2: a potential novel etiology of fulminant myocarditis. Herz. (2020) 45:230–2. doi: 10.1007/s00059-020-04909-z
12. Driggin E, Madhavan MV, Bikdeli B, Chuich T, Laracy J, Bondi-Zoccai G, et al. Cardiovascular considerations for patients, health care workers, and health systems during the coronavirus disease 2019 (COVID-19) pandemic. J Am Coll Cardiol. (2020) 75:2352–71. doi: 10.1016/j.jacc.2020.03.031
13. Guzik TJ, Mohiddin SA, Dimarco A, Patel V, Savvatis K, Marelli-Berg FM, et al. COVID-19 and the cardiovascular system: implications for risk assessment, diagnosis, and treatment options. Cardiovasc Res. (2020) 116:1666–87. doi: 10.1093/cvr/cvaa106
14. Klok FA, Kruip M, van der Meer NJM, Arbous MS, Gommers D, Kant KM, et al. Incidence of thrombotic complications in critically ill ICU patients with COVID-19. Thromb Res. (2020) 191:145–7. doi: 10.1016/j.thromres.2020.04.013
15. Escher R, Breakey N, Lammle B. Severe COVID-19 infection associated with endothelial activation. Thromb Res. (2020) 190:62. doi: 10.1016/j.thromres.2020.04.014
16. Li W, Moore MJ, Vasilieva N, Sui J, Wong SK, Berne MA, et al. Angiotensin-converting enzyme 2 is a functional receptor for the SARS coronavirus. Nature. (2003) 426:450–4. doi: 10.1038/nature02145
17. Hamming I, Timens W, Bulthuis ML, Lely AT, Navis G, van Goor H. Tissue distribution of ACE2 protein, the functional receptor for SARS coronavirus. A first step in understanding SARS pathogenesis. J Pathol. (2004) 203:631–7. doi: 10.1002/path.1570
18. Zhou G, Chen S, Chen Z. Advances in COVID-19: the virus, the pathogenesis, and evidence-based control and therapeutic strategies. Front Med. (2020) 14:117–25. doi: 10.1007/s11684-020-0773-x
19. Chen J, Subbarao K. The Immunobiology of SARS*. Annu Rev Immunol. (2007) 25:443–72. doi: 10.1146/annurev.immunol.25.022106.141706
20. Ding Y, Wang H, Shen H, Li Z, Geng J, Han H, et al. The clinical pathology of severe acute respiratory syndrome (SARS): a report from China. J Pathol. (2003) 200:282–9. doi: 10.1002/path.1440
21. Brissette CA, Kees ED, Burke MM, Gaultney RA, Floden AM, Watt JA. The multifaceted responses of primary human astrocytes and brain microvascular endothelial cells to the Lyme disease spirochete, Borrelia burgdorferi. ASN Neuro. (2013) 5:221–9. doi: 10.1042/AN20130010
22. Grab DJ, Nyarko E, Nikolskaia OV, Kim YV, Dumler JS. Human brain microvascular endothelial cell traversal by Borrelia burgdorferi requires calcium signaling. Clin Microbiol Infect. (2009) 15:422–6. doi: 10.1111/j.1469-0691.2009.02869.x
23. Rathore AP, Mantri CK, Aman SA, Syenina A, Ooi J, Jagaraj CJ, et al. Dengue virus-elicited tryptase induces endothelial permeability and shock. J Clin Invest. (2019) 130:4180–93. doi: 10.1172/JCI128426
24. Soe HJ, Khan AM, Manikam R, Samudi Raju C, Vanhoutte P, Sekaran SD. High dengue virus load differentially modulates human microvascular endothelial barrier function during early infection. J Gen Virol. (2017) 98:2993–3007. doi: 10.1099/jgv.0.000981
25. Woods ME, Olano JP. Host defenses to Rickettsia rickettsii infection contribute to increased microvascular permeability in human cerebral endothelial cells. J Clin Immunol. (2008) 28:174–85. doi: 10.1007/s10875-007-9140-9
26. Rydkina E, Turpin LC, Sahni SK. Rickettsia rickettsii infection of human macrovascular and microvascular endothelial cells reveals activation of both common and cell type-specific host response mechanisms. Infect Immun. (2010) 78:2599–606. doi: 10.1128/IAI.01335-09
27. Levi M. Pathogenesis and diagnosis of disseminated intravascular coagulation. Int J Lab Hematol. (2018) 40(Suppl 1):15–20. doi: 10.1111/ijlh.12830
28. Arlati S. Pathophysiology of acute illness and injury. In: Aseni P, De Carlis L, Mazzola A, Grande AM, editors. Operative Techniques and Recent Advances in Acute Care and Emergency Surgery. Cham: Springer (2018). p. 11–42. doi: 10.1007/978-3-319-95114-0_2
29. Tang N, Li D, Wang X, Sun Z. Abnormal coagulation parameters are associated with poor prognosis in patients with novel coronavirus pneumonia. J Thromb Haemost. (2020) 18:844–7. doi: 10.1111/jth.14768
30. Han H, Yang L, Liu R, Liu F, Wu KL, Li J, et al. Prominent changes in blood coagulation of patients with SARS-CoV-2 infection. Clin Chem Lab Med. 2020. doi: 10.1515/cclm-2020-0188
31. Shi S, Qin M, Shen B, Cai Y, Liu T, Yang F, et al. Association of cardiac injury with mortality in hospitalized patients with COVID-19 in Wuhan, China. JAMA Cardiol. (2020) 5:802-10. doi: 10.1001/jamacardio.2020.0950
32. Arentz M, Yim E, Klaff L, Lokhandwala S, Riedo FX, Chong M, et al. Characteristics and outcomes of 21 critically Ill patients with COVID-19 in Washington State. JAMA. (2020). 323:1612-4. doi: 10.1001/jama.2020.4326
33. Zhou F, Yu T, Du R, Fan G, Liu Y, Liu Z, et al. Clinical course and risk factors for mortality of adult inpatients with COVID-19 in Wuhan, China: a retrospective cohort study. Lancet. (2020). 395:1054–62. doi: 10.1016/S0140-6736(20)30566-3
34. Zhang Y, Xiao M, Zhang S, Xia P, Cao W, Jiang W, et al. Coagulopathy and antiphospholipid antibodies in patients with COVID-19. N Engl J Med. (2020) 382:e38. doi: 10.1056/NEJMc2007575
35. Wang D, Hu B, Hu C, Zhu F, Liu X, Zhang J, et al. Clinical characteristics of 138 hospitalized patients with 2019 novel coronavirus-infected pneumonia in Wuhan, China. JAMA. (2020). 323:1061–9 doi: 10.1001/jama.2020.1585
36. Li LQ, Huang T, Wang YQ, Wang ZP, Liang Y, Huang TB, et al. 2019 novel coronavirus patients' clinical characteristics, discharge rate, and fatality rate of meta-analysis. J Med Virol. (2020) 92:577–83. doi: 10.1002/jmv.25757
37. Lauer SA, Grantz KH, Bi Q, Jones FK, Zheng Q, Meredith HR, et al. The incubation period of coronavirus disease 2019 (COVID-19) from publicly reported confirmed cases: estimation and application. Ann Intern Med. (2020) 172:577–82. doi: 10.7326/M20-0504
38. Liu K, Fang YY, Deng Y, Liu W, Wang MF, Ma JP, et al. Clinical characteristics of novel coronavirus cases in tertiary hospitals in Hubei Province. Chin Med J (Engl). (2020). 133:1025–31 doi: 10.1097/CM9.0000000000000744
39. Huang C, Wang Y, Li X, Ren L, Zhao J, Hu Y, et al. Clinical features of patients infected with 2019 novel coronavirus in Wuhan, China. Lancet. (2020) 395:497–506. doi: 10.1016/S0140-6736(20)30183-5
40. Team CC-R. Severe outcomes among patients with coronavirus disease 2019 (COVID-19) - United States, February 12-March 16, 2020. MMWR Morb Mortal Wkly Rep. (2020) 69:343–6. doi: 10.15585/mmwr.mm6912e2
41. Zheng YY, Ma YT, Zhang JY, Xie X. COVID-19 and the cardiovascular system. Nat Rev Cardiol. (2020) 17:259–60. doi: 10.1038/s41569-020-0360-5
42. Hu H, Ma F, Wei X, Fang Y. Coronavirus fulminant myocarditis saved with glucocorticoid and human immunoglobulin. Eur Heart J. (2020) 16:ehaa190. doi: 10.1093/eurheartj/ehaa190
43. Wong CK, Lam CW, Wu AK, Ip WK, Lee NL, Chan IH, et al. Plasma inflammatory cytokines and chemokines in severe acute respiratory syndrome. Clin Exp Immunol. (2004) 136:95–103. doi: 10.1111/j.1365-2249.2004.02415.x
44. Ruan Q, Yang K, Wang W, Jiang L, Song J. Clinical predictors of mortality due to COVID-19 based on an analysis of data of 150 patients from Wuhan, China. Intensive Care Med. (2020) 46:846–8. doi: 10.1007/s00134-020-06028-z
45. Xiong TY, Redwood S, Prendergast B, Chen M. Coronaviruses and the cardiovascular system: acute and long-term implications. Eur Heart J. (2020) 41:1798–800. doi: 10.1093/eurheartj/ehaa231
46. Saraca LM, Lazzari L, di Giuli C, Lavagna A, Mezzetti P, Bovelli D, et al. Cytomegalovirus myocarditis in a patient with systemic lupus erythematosus (SLE) successfully treated with ganciclovir. IDCases. (2018) 12:4–6. doi: 10.1016/j.idcr.2018.02.008
47. Harada T, Ohtaki E, Tobaru T, Kitahara K, Sumiyoshi T, Hosoda S. Rubella-associated perimyocarditis–a case report. Angiology. (2002) 53:727–32. doi: 10.1177/000331970205300615
48. Magno Palmeira M, Umemura Ribeiro HY, Garcia Lira Y, Machado Juca Neto FO, da Silva Rodrigues IA, Fernandes da Paz LN, et al. Heart failure due to cytomegalovirus myocarditis in immunocompetent young adults: a case report. BMC Res Notes. (2016) 9:391. doi: 10.1186/s13104-016-2181-5
49. Yu CM, Wong RS, Wu EB, Kong SL, Wong J, Yip GW, et al. Cardiovascular complications of severe acute respiratory syndrome. Postgrad Med J. (2006) 82:140–4. doi: 10.1136/pgmj.2005.037515
50. Kochi AN, Tagliari AP, Forleo GB, Fassini GM, Tondo C. Cardiac and arrhythmic complications in patients with COVID-19. J Cardiovasc Electrophysiol. (2020) 31:1003–8. doi: 10.1111/jce.14479
51. Guo T, Fan Y, Chen M, Wu X, Zhang L, He T, et al. Cardiovascular implications of fatal outcomes of patients with coronavirus disease 2019 (COVID-19). JAMA Cardiol. (2020) 5:811–8. doi: 10.1001/jamacardio.2020.1017
52. Bansal M. Cardiovascular disease and COVID-19. Diabetes Metab Syndr. (2020) 14:247–50. doi: 10.1016/j.dsx.2020.03.013
53. Wu CI, Postema PG, Arbelo E, Behr ER, Bezzina CR, Napolitano C, et al. SARS-CoV-2, COVID-19, and inherited arrhythmia syndromes. Heart Rhythm. (2020) 17:1456–62. doi: 10.1016/j.hrthm.2020.03.024
54. Du Y, Tu L, Zhu P, Mu M, Wang R, Yang P, et al. Clinical features of 85 fatal cases of COVID-19 from Wuhan. A retrospective observational study. Am J Respir Crit Care Med. (2020) 201:1372–9. doi: 10.1164/rccm.202003-0543OC
55. Buzon J, Roignot O, Lemoine S, Perez P, Kimmoun A, Levy B, et al. Takotsubo cardiomyopathy triggered by influenza A virus. Intern Med. (2015) 54:2017–9. doi: 10.2169/internalmedicine.54.3606
56. Hess DC, Eldahshan W, Rutkowski E. COVID-19-related stroke. Transl Stroke Res. (2020) 11:322–5. doi: 10.1007/s12975-020-00818-9
57. Aghagoli G, Gallo Marin B, Katchur NJ, Chaves-Sell F, Asaad WF, Murphy SA. Neurological involvement in COVID-19 and potential mechanisms: a review. Neurocrit Care. (2020). doi: 10.1007/s12028-020-01049-4. [Epub ahead of print].
58. Qi X, Keith KA, Huang JH. COVID-19 and stroke: a review. Brain Hemorrhages. (2020). doi: 10.1016/j.hest.2020.11.001. [Epub ahead of print].
59. Achar A, Ghosh C. COVID-19-associated neurological disorders: the potential route of CNS invasion and blood-brain relevance. Cells. (2020) 9:2360. doi: 10.3390/cells9112360
60. Alharthy A, Faqihi F, Memish ZA, Karakitsos D. Fragile endothelium and brain dysregulated neurochemical activity in COVID-19. ACS Chem Neurosci. (2020) 11:2159–62. doi: 10.1021/acschemneuro.0c00437
61. Mao L, Jin H, Wang M, Hu Y, Chen S, He Q, et al. Neurologic manifestations of hospitalized patients with coronavirus disease 2019 in Wuhan, China. JAMA Neurol. (2020) 77:683–90. doi: 10.1001/jamaneurol.2020.1127
62. Fotuhi M, Mian A, Meysami S, Raji CA. Neurobiology of COVID-19. J Alzheimers Dis. (2020) 76:3–19. doi: 10.3233/JAD-200581
63. Li Y, Li M, Wang M, Zhou Y, Chang J, Xian Y, et al. Acute cerebrovascular disease following COVID-19: a single center, retrospective, observational study. Stroke Vasc Neurol. (2020) 5:279–84. doi: 10.1136/svn-2020-000431
64. Oxley TJ, Mocco J, Majidi S, Kellner CP, Shoirah H, Singh IP, et al. Large-vessel stroke as a presenting feature of Covid-19 in the young. N Engl J Med. (2020) 382:e60. doi: 10.1056/NEJMc2009787
65. Paniz-Mondolfi A, Bryce C, Grimes Z, Gordon RE, Reidy J, Lednicky J, et al. Central nervous system involvement by severe acute respiratory syndrome coronavirus-2 (SARS-CoV-2). J Med Virol. (2020) 92:699–702. doi: 10.1002/jmv.25915
66. Younger DS. Postmortem neuropathology in Covid-19. Brain Pathol. (2020) 23:e12915. doi: 10.1111/bpa.12915
67. Xiao F, Tang M, Zheng X, Liu Y, Li X, Shan H. Evidence for gastrointestinal infection of SARS-CoV-2. Gastroenterology. (2020) 158:1831–3.e3. doi: 10.1053/j.gastro.2020.02.055
68. Galanopoulos M, Gkeros F, Doukatas A, Karianakis G, Pontas C, Tsoukalas N, et al. COVID-19 pandemic: pathophysiology and manifestations from the gastrointestinal tract. World J Gastroenterol. (2020) 26:4579–88. doi: 10.3748/wjg.v26.i31.4579
69. Jothimani D, Venugopal R, Abedin MF, Kaliamoorthy I, Rela M. COVID-19 and the liver. J Hepatol. (2020) 73:1231–40. doi: 10.1016/j.jhep.2020.06.006
70. Alqahtani SA, Schattenberg JM. Liver injury in COVID-19: the current evidence. United European Gastroenterol J. (2020) 8:509–19. doi: 10.1177/2050640620924157
71. Sun J, Aghemo A, Forner A, Valenti L. COVID-19 and liver disease. Liver Int. (2020) 40:1278–81. doi: 10.1111/liv.14470
72. Ridruejo E, Soza A. The liver in times of COVID-19: what hepatologists should know. Ann Hepatol. (2020) 19:353–8. doi: 10.1016/j.aohep.2020.05.001
73. Farcas GA, Poutanen SM, Mazzulli T, Willey BM, Butany J, Asa SL, et al. Fatal severe acute respiratory syndrome is associated with multiorgan involvement by coronavirus. J Infect Dis. (2005) 191:193–7. doi: 10.1086/426870
74. Ding Y, He L, Zhang Q, Huang Z, Che X, Hou J, et al. Organ distribution of severe acute respiratory syndrome (SARS) associated coronavirus (SARS-CoV) in SARS patients: implications for pathogenesis and virus transmission pathways. J Pathol. (2004) 203:622–30. doi: 10.1002/path.1560
75. Zhao CL, Rapkiewicz A, Maghsoodi-Deerwester M, Gupta M, Cao W, Palaia T, et al. Pathological findings in the postmortem liver of COVID-19 patients. Hum Pathol. (2020) 109:59–68. doi: 10.1016/j.humpath.2020.11.015
76. Tian S, Xiong Y, Liu H, Niu L, Guo J, Liao M, et al. Pathological study of the 2019 novel coronavirus disease (COVID-19) through postmortem core biopsies. Mod Pathol. (2020) 33:1007–14. doi: 10.1038/s41379-020-0536-x
77. Schaller T, Hirschbuhl K, Burkhardt K, Braun G, Trepel M, Markl B, et al. Postmortem examination of patients with COVID-19. JAMA. (2020) 323:2518–20. doi: 10.1001/jama.2020.8907
78. Nephrology ISo. Recommendations for the Novel Coronavirus 2019 Epidemic. (2020). [cited 2020 April 3]. Available online at: https://www.theisn.org/covid19/recommendations (accessed November 15, 2020).
79. Cheng Y, Luo R, Wang K, Zhang M, Wang Z, Dong L, et al. Kidney disease is associated with in-hospital death of patients with COVID-19. Kidney Int. (2020) 97:829–38. doi: 10.1101/2020.02.18.20023242
80. Su H, Yang M, Wan C, Yi LX, Tang F, Zhu HY, et al. Renal histopathological analysis of 26 postmortem findings of patients with COVID-19 in China. Kidney Int. (2020) 98:219–27. doi: 10.1016/j.kint.2020.04.003
81. Naicker S, Yang CW, Hwang SJ, Liu BC, Chen JH, Jha V. The novel coronavirus 2019 epidemic and kidneys. Kidney Int. (2020) 97:824–8. doi: 10.1016/j.kint.2020.03.001
82. Durvasula R, Wellington T, McNamara E, Watnick S. COVID-19 and kidney failure in the acute care setting: our experience from seattle. Am J Kidney Dis. (2020) 76:4–6. doi: 10.1053/j.ajkd.2020.04.001
83. Valizadeh RBA, Mirzazadeh A, Bhaskar LVKS. Coronavirus-nephropathy; renal involvement in COVID-19. J Renal Inj Prevent. (2020) 2:1–2. doi: 10.34172/jrip.2020.18
84. Bonetti PO, Lerman LO, Lerman A. Endothelial dysfunction: a marker of atherosclerotic risk. Arterioscler Thromb Vasc Biol. (2003) 23:168–75. doi: 10.1161/01.ATV.0000051384.43104.FC
85. Varga Z, Flammer AJ, Steiger P, Haberecker M, Andermatt R, Zinkernagel AS, et al. Endothelial cell infection and endotheliitis in COVID-19. Lancet. (2020) 395:1417–8. doi: 10.1016/S0140-6736(20)30937-5
86. Simmons J, Pittet JF. The coagulopathy of acute sepsis. Curr Opin Anaesthesiol. (2015) 28:227–36. doi: 10.1097/ACO.0000000000000163
87. Guan WJ, Ni ZY, Hu Y, Liang WH, Ou CQ, He JX, et al. Clinical characteristics of coronavirus disease 2019 in China. N Engl J Med. (2020) 382:1708–20. doi: 10.1101/2020.02.06.20020974
88. Danzi GB, Loffi M, Galeazzi G, Gherbesi E. Acute pulmonary embolism and COVID-19 pneumonia: a random association? Eur Heart J. (2020) 41:1858. doi: 10.1093/eurheartj/ehaa254
89. Rotzinger DC, Beigelman-Aubry C, von Garnier C, Qanadli SD. Pulmonary embolism in patients with COVID-19: time to change the paradigm of computed tomography. Thromb Res. (2020) 190:58–9. doi: 10.1016/j.thromres.2020.04.011
90. Middeldorp S, Coppens M, van Haaps TF, Foppen M, Vlaar AP, Muller MCA, et al. Incidence of venous thromboembolism in hospitalized patients with COVID-19. J Thromb Haemost. (2020) 18:1995–2002. doi: 10.20944/preprints202004.0345.v1
91. Rali P, O'Corragain O, Oresanya L, Yu D, Sheriff O, Weiss R, et al. Incidence of venous thromboembolism in coronavirus disease 2019: an experience from a single large academic center. J Vasc Surg Venous Lymphat Disord. (2020). doi: 10.1016/j.jvsv.2020.09.006. [Epub ahead of print].
92. Cui S, Chen S, Li X, Liu S, Wang F. Prevalence of venous thromboembolism in patients with severe novel coronavirus pneumonia. J Thromb Haemost. (2020) 18:1421–4. doi: 10.1111/jth.14830
93. Helms J, Tacquard C, Severac F, Leonard-Lorant I, Ohana M, Delabranche X, et al. High risk of thrombosis in patients with severe SARS-CoV-2 infection: a multicenter prospective cohort study. Intensive Care Med. (2020) 46:1089–98. doi: 10.1007/s00134-020-06062-x
94. Lodigiani C, Iapichino G, Carenzo L, Cecconi M, Ferrazzi P, Sebastian T, et al. Venous and arterial thromboembolic complications in COVID-19 patients admitted to an academic hospital in Milan, Italy. Thromb Res. (2020) 191:9–14. doi: 10.1016/j.thromres.2020.04.024
95. Artifoni M, Danic G, Gautier G, Gicquel P, Boutoille D, Raffi F, et al. Systematic assessment of venous thromboembolism in COVID-19 patients receiving thromboprophylaxis: incidence and role of D-dimer as predictive factors. J Thromb Thrombolysis. (2020) 50:211–6. doi: 10.1007/s11239-020-02146-z
96. Klok FA, Kruip M, van der Meer NJM, Arbous MS, Gommers D, Kant KM, et al. Confirmation of the high cumulative incidence of thrombotic complications in critically ill ICU patients with COVID-19: an updated analysis. Thromb Res. (2020) 191:148–50. doi: 10.1016/j.thromres.2020.04.041
97. Dargon PT, Landry GJ. Buerger's disease. Ann Vasc Surg. (2012) 26:871–80. doi: 10.1016/j.avsg.2011.11.005
99. Sundel RP. Kawasaki disease. Rheum Dis Clin North Am. (2015) 41:63–73, viii. doi: 10.1016/j.rdc.2014.09.010
100. Agarwal S, Agrawal DK. Kawasaki disease: etiopathogenesis and novel treatment strategies. Expert Rev Clin Immunol. (2017) 13:247–58. doi: 10.1080/1744666X.2017.1232165
101. Smolen JS, Aletaha D, McInnes IB. Rheumatoid arthritis. Lancet. (2016) 388:2023–38. doi: 10.1016/S0140-6736(16)30173-8
102. Administration USFaD. Coronavirus (COVID-19) Update: FDA Issues Emergency Use Authorization for Potential COVID-19 Treatment. (2020). [updated May 1, 2020; cited 2020 May 3, ]. Available online at: https://www.fda.gov/news-events/press-announcements/coronavirus-covid-19-update-fda-issues-emergency-use-authorization-potential-covid-19-treatment (accessed November 15, 2020).
103. Chu CM, Cheng VC, Hung IF, Wong MM, Chan KH, Chan KS, et al. Role of lopinavir/ritonavir in the treatment of SARS: initial virological and clinical findings. Thorax. (2004) 59:252–6. doi: 10.1136/thorax.2003.012658
104. Elfiky AA. Anti-HCV, nucleotide inhibitors, repurposing against COVID-19. Life Sci. (2020) 248:117477. doi: 10.1016/j.lfs.2020.117477
105. Medicine NUSNLo. Lopinavir/Ritonavir, Ribavirin and IFN-beta Combination for nCoV Treatment. (2020). [updated April 15, 2020; cited 2020 April 1]. Available online at: https://clinicaltrials.gov/ct2/show/NCT04276688 (accessed November 15, 2020).
106. Wang M, Cao R, Zhang L, Yang X, Liu J, Xu M, et al. Remdesivir and chloroquine effectively inhibit the recently emerged novel coronavirus (2019-nCoV) in vitro. Cell Res. (2020) 30:269–71. doi: 10.1038/s41422-020-0282-0
107. Fan BE, Chong VCL, Chan SSW, Lim GH, Lim KGE, Tan GB, et al. Hematologic parameters in patients with COVID-19 infection. Am J Hematol. (2020) 95:E131–4. doi: 10.1002/ajh.25774
108. Grein J, Ohmagari N, Shin D, Diaz G, Asperges E, Castagna A, et al. Compassionate use of remdesivir for patients with severe Covid-19. N Engl J Med. (2020) 382:2327–36. doi: 10.1056/NEJMoa2007016
109. Wang Y, Zhang D, Du G, Du R, Zhao J, Jin Y, et al. Remdesivir in adults with severe COVID-19: a randomised, double-blind, placebo-controlled, multicentre trial. Lancet. (2020) 395:1569–78. doi: 10.1016/S0140-6736(20)31022-9
110. Mulangu S, Dodd LE, Davey RT Jr., Tshiani Mbaya O, Proschan M, Mukadi D, et al. A randomized, controlled trial of ebola virus disease therapeutics. N Engl J Med. (2019) 381:2293–303. doi: 10.1056/NEJMoa1910993
111. Rico-Mesa JS, Rosas D, Ahmadian-Tehrani A, White A, Anderson AS, Chilton R. The role of anticoagulation in COVID-19-induced hypercoagulability. Curr Cardiol Rep. (2020) 22:53. doi: 10.1007/s11886-020-01328-8
112. Marietta M, Ageno W, Artoni A, De Candia E, Gresele P, Marchetti M, et al. COVID-19 and haemostasis: a position paper from Italian Society on Thrombosis and Haemostasis (SISET). Blood Transfus. (2020) 18:167–9. doi: 10.2450/2020.0083-20
113. Konstantinides SV. Thrombosis and thromboembolism related to COVID-19: increase the level of awareness, lower the threshold of suspicion, and keep following the guidelines. JACC Case Rep. (2020) 2:1388–90. doi: 10.1016/j.jaccas.2020.05.016
114. Nadkarni GN, Lala A, Bagiella E, Chang HL, Moreno P, Pujadas E, et al. Anticoagulation, mortality, bleeding and pathology among patients hospitalized with COVID-19: a single health system study. J Am Coll Cardiol. (2020) 16:1815–26. doi: 10.1016/j.jacc.2020.08.041
115. Tang N, Bai H, Chen X, Gong J, Li D, Sun Z. Anticoagulant treatment is associated with decreased mortality in severe coronavirus disease 2019 patients with coagulopathy. J Thromb Haemost. (2020) 18:1094–9. doi: 10.1111/jth.14817
116. Paranjpe I, Fuster V, Lala A, Russak AJ, Glicksberg BS, Levin MA, et al. Association of treatment dose anticoagulation with in-hospital survival among hospitalized patients with COVID-19. J Am Coll Cardiol. (2020) 76:122–4. doi: 10.1016/j.jacc.2020.05.001
117. Fadel R, Morrison AR, Vahia A, Smith ZR, Chaudhry Z, Bhargava P, et al. Early short course corticosteroids in hospitalized patients with COVID-19. Clin Infect Dis. (2020) 71:2114–20. doi: 10.1101/2020.05.04.20074609
118. Corral L BA, Arnaiz delas Revillas F, Gomez-Barquero J, Abadia-Otero J, Garcia-Ibarbia C, et al. GLUCOCOVID: a controlled trial of methylprednisolone in adults hospitalized with COVID-19 pneumonia. medRxiv. (2020). doi: 10.1101/2020.06.17.20133579
Keywords: COVID-19, micovascular disease, angiopathy, cardiac dysfunction, vascular thrombosis
Citation: Nalugo M, Schulte LJ, Masood MF and Zayed MA (2021) Microvascular Angiopathic Consequences of COVID-19. Front. Cardiovasc. Med. 8:636843. doi: 10.3389/fcvm.2021.636843
Received: 02 December 2020; Accepted: 11 January 2021;
Published: 02 February 2021.
Edited by:
Masuko Ushio-Fukai, Augusta University, United StatesReviewed by:
Paolo Severino, Sapienza University of Rome, ItalyHirinori Nakagami, Osaka University, Japan
Copyright © 2021 Nalugo, Schulte, Masood and Zayed. This is an open-access article distributed under the terms of the Creative Commons Attribution License (CC BY). The use, distribution or reproduction in other forums is permitted, provided the original author(s) and the copyright owner(s) are credited and that the original publication in this journal is cited, in accordance with accepted academic practice. No use, distribution or reproduction is permitted which does not comply with these terms.
*Correspondence: Mohamed A. Zayed, emF5ZWRtQHd1c3RsLmVkdQ==