- 1Department of Anesthesiology, Xi'an Children's Hospital, Xi'an Jiaotong University, Xi'an, China
- 2Department of Cardiovascular Surgery, Xijing Hospital, Air Force Medical University, Xi'an, China
- 3Department of Anaesthesiology, Beijing Hospital, National Center of Gerontology, Institute of Geriatric Medicine, Chinese Academy of Medical Science, Beijing, China
- 4Center for Translational Medicine, Lewis Katz School of Medicine at Temple University, Philadelphia, PA, United States
Myocardial ischemia/reperfusion injury is a common clinical problem and can result in severe cardiac dysfunction. Previous studies have demonstrated the protection of electroacupuncture against myocardial ischemia/reperfusion injury. However, the role of X-box binding protein I (XBP1) signaling pathway in the protection of electroacupuncture was still elusive. Thus, we designed this study and demonstrated that electroacupuncture significantly improved cardiac function during myocardial ischemia/reperfusion injury and reduced cardiac infarct size. Electroacupuncture treatment further inhibited cardiac injury manifested by the decrease of the activities of serum lactate dehydrogenase and creatine kinase-MB. The results also revealed that electroacupuncture elevated the expressions of XBP1, glucose-regulated protein 78 (GRP78), Akt, and Bcl-2 and decreased the Bax and cleaved Caspase 3 expressions. By using the inhibitor of XBP1 in vitro, the results revealed that suppression of XBP1 expression could markedly increase the activities of lactate dehydrogenase and creatine kinase-MB and cell apoptosis, thus exacerbating stimulated ischemia/reperfusion-induced H9c2 cell injury. Compared with stimulated ischemia/reperfusion group, inhibition of XBP1 inhibited the downstream GRP78 and Akt expressions during stimulated ischemia/reperfusion injury. Collectively, our data demonstrated that electroacupuncture treatment activated XBP1/GRP78/Akt signaling to protect hearts from myocardial ischemia/reperfusion injury. These findings revealed the underlying mechanisms of electroacupuncture protection against myocardial ischemia/reperfusion injury and may provide novel therapeutic targets for the clinical treatment of myocardial ischemia/reperfusion injury.
Introduction
Ischemic heart disease is a common cardiovascular problem with high morbidity and mortality (1). Although it is important to timely restore the blood flow of an ischemic myocardium, cardiovascular outcomes can be further aggravated by the so-called myocardial ischemia/reperfusion (MI/R) injury. Therefore, exploring a safe and effective treatment is urgently needed to mitigate MI/R-induced injury.
The endoplasmic reticulum (ER) is an organelle where the target proteins are processed so as to prompt its post-translational modifications, proper folding, and protein transport (2). However, under cellular stress, the imbalance of ER protein processing and accumulation of the unfolded proteins and/or misfolded proteins results in ER stress, which further induces the unfolded protein response (UPR) as an adaptive response to restore ER homeostasis (3, 4). The UPR is initiated by three classical ER transmembrane sensors: protein kinase R-like ER kinase (PERK), inositol-requiring kinase 1 (IRE1), and activating transcription factor 6 (ATF6) (3). As a key player of ER stress, X-box binding protein I (XBP1) expression was induced by ATF6 and then was spliced by IRE1 (5). The resulting spliced form of XBP1 can further activate UPR to cope with ER stress (5). Previous studies further revealed that ER stress participated in the pathogenesis of numerous cardiac diseases (6). In transverse aortic constriction (TAC)-induced hypertrophic and falling hearts, sustained ER stress resulted in cardiomyocyte apoptosis and contributed to the progression from cardiac hypertrophy to heart failure through the canonical and non-canonical pathways (7, 8). Surprisingly, hypoxia induced ATF6 and glucose-regulated protein 78 (GRP78) expressions and protected cardiomyocytes from ischemic injury (9). ATF6 transgenic mice alleviated MI/R damage via decreasing oxidative stress, enhancing catalase expression, and reducing cell necrosis and apoptosis (10, 11). Notably, GRP78 as an XBP1 target was shown to stimulate Akt pathway to protect hearts from I/R injury (12). Furthermore, XBP1 can protect β-cells from lipotoxicity via activation of Akt pathway (13). However, the role of XBP1–Akt pathway in the MI/R injury was not fully investigated.
Based on the Chinese traditional medical theory, acupuncture is used to treat diseases for more than 2000 years (14, 15). Especially, electroacupuncture (EA) pretreatment at specific acupoints has been demonstrated as an effective approach to improve cardiac function in diverse pathological conditions. In spontaneously hypertensive rats, long-term EA reduced the wall thickness of left ventricle via regulating the NOS pathway (16). It was revealed that EA at PC6 (Neiguan) and PC5 (Jianshi) acupoints reduced myocardial malondialdehyde (MDA) level, norepinephrine concentration, and cell apoptosis to attenuate MI/R injury in animal models (17, 18). Furthermore, in our previous clinical study, EA pretreatment significantly attenuated MI/R injury in patients with heart valve replacement surgery (19). However, whether EA pretreatment regulates ER stress signaling pathways to protect hearts against MI/R injury has not been explored previously.
Thus, the present study was designed to investigate the protection of EA pretreatment against MI/R injury and the potential role of XBP1/GRP78 signaling in this process. These findings may provide the theoretical basis for the clinical use of EA pretreatment against MI/R injury.
Materials and Methods
Animal Model of Myocardial Ischemia/Reperfusion Injury
The animal experimental protocol in this study was approved by the Animal Care Committee of Air Force Medical University. All animal procedures were performed in accordance with the Guidelines for the Care and Use of Laboratory Animals by the Institute of Laboratory Animal Research from US National Institutes of Health (National Institutes of Health Publication No. 8523, revised 1996). Male C57BL/6 mice aged 10~12 weeks and weighing 22~26 g were obtained from the Laboratory Animal Center of Air Force Medical University. All mice were housed at 20–25°C under a 12-h light/dark cycle and received a standard diet and water ad libitum.
MI/R injury mouse model was established according to the previous study (20). Briefly, mice were anesthetized with 1–2% isoflurane via an isoflurane vaporizer (Matrx, Orchard Park, NY, USA). A skin scar was cut in the left chest, and a tiny hole was made at the fourth intercostal space. Afterwards, the heart was smoothly squeezed out of the thoracic cavity. The left anterior descending (LAD) coronary artery was ligated by a 6-0 silk suture for 30 min, and then the slipknot was released. The reperfusion phase lasted for 2–4 h, and the heart samples were collected for protein expressions analysis. The cardiac function, myocardial infarct size, cell apoptosis, and lactate dehydrogenase (LDH) as well as creatine kinase-MB (CK-MB) were assessed following a 24-h reperfusion. The same procedure except ligation of LAD was performed in the mice of sham group.
Electroacupuncture Pretreatment
EA pretreatment was conducted by using the Hwato Electronic Acupuncture Treatment Instrument (Suzhou Medical Appliances, Suzhou, China). Briefly, mice were anesthetized and maintained by inhalation of 1–2% isoflurane via an isoflurane vaporizer. The needles connected to the electrodes were inserted into 2- to 3-mm depth of muscle layers at the Neiguan acupoint (PC6) of both forelimbs, which are located between the palmar tendon and flexor carpi ulnaris (21). Mice were stimulated at the density of 1 mA with a frequency of 2/15 Hz for 30 min once a day for 3 days. The MI/R surgery or sham surgery was performed within the 30 min after the last EA treatment. Mice from Sham and Sham+EA groups were anesthetized for 30 min to avoid the effects of isoflurane between different groups.
The mice were divided into the following four groups with 12–15 mice for each: sham group (Sham), sham group with EA pretreatment (Sham+EA), MI/R injury group (MI/R), and MI/R group with EA pretreatment (MI/R+EA). Mice in the Sham+EA or MI/R+EA groups received EA preconditioning for 3 consecutive days followed by sham or MI/R surgery, while mice in the Sham or MI/R group underwent the sham or MI/R surgery, respectively.
Echocardiography
Mice were anesthetized and maintained by inhalation of 1–2% isoflurane after 24-h reperfusion. Cardiac function was evaluated by Doppler echocardiography with a 15-MHz linear transducer (Visual Sonic Vevo 2100, Toronto, ON, Canada). Mice were placed on a heating pad to maintain the body temperature during the whole procedure. M-mode echocardiography was recorded and used to assess cardiac function. Left ventricular ejection fraction (LVEF) and left ventricular fractional shortening (LVFS) were obtained by using Vevo LAB 3.1.0 software.
Activities Measurements of Lactate Dehydrogenase and Creatine Kinase-MB
The serum was obtained by centrifugation of mouse blood at 3,000 rpm for 10 min after 24-h reperfusion and used for LDH and CK-MB determination. The assay was conducted according to the manufacturer's instruction (Jiancheng Bioengineering, Nanjing, China). The activities of LDH and CK-MB were calculated based on the methods described in the manufacturer's instruction.
Triphenyltetrazolium Chloride/Evans Blue Double Staining
The mice were anesthetized with 1–2% isoflurane, and the LAD was occluded at the same position following 24-h reperfusion. The 3% Evans blue solution was injected into the hearts via the aorta to stain the non-ischemic area of the heart. The whole hearts were then collected and frozen on dry ice for 10 min and cut into four slices transversally from the bottom of the hearts. The slices were stained in 1.5% triphenyltetrazolium chloride (TTC) in phosphate solution (pH 7.4) and incubated at 37°C for 20 min. Then the slices were fixed in 4% paraformaldehyde for 12 h, and the images were obtained by using a digital camera. The infarct size was calculated by the ratio of white area to white and red areas. The size was determined by using Image-Pro Plus software (Media Cybernetics, Inc., Rockville, MD, USA).
Terminal Deoxynucleotidyl Transferase-Mediated Dutp Nick-End Labeling Staining
Myocardial apoptosis and cell apoptosis were determined by an in situ cell death detection kit (Roche Molecular Biochemicals, Mannheim, Germany) as previously described (22). In brief, at the end of the experiment, the myocardial tissues and H9c2 cells were fixed in 4% paraformaldehyde for at least 24 h. After the paraffin-imbedded sections were prepared, the manufacturer's instruction for TUNEL staining was followed. The apoptotic myocardial cells and H9c2 cells were stained with TUNEL staining solution, and nuclei were visualized by DAPI staining. Then the images were obtained with an Olympus FV10i microscope (Olympus, Tokyo, Japan); and an apoptotic rate was presented as the count of TUNEL-positive cardiomyocytes to the total number of cells.
Cell Culture and Stimulated Ischemia/Reperfusion Model
H9c2 cells were cultured in Dulbecco's Modified Eagle Medium (DMEM; HyClone, Logan, UT, USA) with 10% fetal bovine serum (Gibco, Grand Island, NY, USA) at 37°C in a 5% CO2 air incubator. The experiments included four groups: (1) the cells in the CON group were cultured in the serum-free DMEM. (2) H9c2 cells in stimulated I/R (SI/R) group were cultured in serum-free DMEM for 12 h and then subjected to the ischemic buffer (10 mM of deoxyglucose, 137 mM of NaCl, 12 mM of KCl, 0.49 mM of MgCl2, 0.9 mM of CaCl2·2H2O, 0.75 mM of sodium dithionate, 20 mM of lactate, and 4 mM of Hepes, pH 6.5) for 2 h. The cells were then cultured in normal DMEM at 37°C in an incubator (5% CO2, 95% air) for 4 h to establish in vitro SI/R model. (3) The cells in XI group (XBP1 inhibitor, 4μ8C) were cultured in the serum-free DMEM and then incubated with 5 μM of 4μ8C for 2 h. (4) The cells in SI/R+XI group were incubated 5 μM of 4μ8C for 2 h and then subjected to ischemic medium for 2 h. Then the cells were cultured in normal DMEM at 37°C in an incubator (5% CO2, 95% air) for 4 h. The supernatant of cell culture was collected for LDH and CK-MB activities measurements following the protocol described above.
Western Blotting
The proteins were isolated from the heart left ventricles including the infarct zone and broader zone and H9c2 cells for western blotting detection. The proteins were separated with sodium dodecyl sulfate–polyacrylamide gel electrophoresis (SDS-PAGE) and then transferred on to a polyvinylidene difluoride (PVDF) membrane (Millipore, Billerica, MA, USA); subsequently, the membrane is incubated with 5% non-fat milk in TBST. Western blotting was then performed with antibodies against XBP1 (Cat. 83418, 1:1,000; Cell Signaling Technology, Danvers, MA, USA), GRP78 (Cat. 3183, 1:1,000; Cell Signaling Technology), p-Akt (Cat. 4060, 1:1,000; Cell Signaling Technology), Akt (Cat. 4691, 1:1,000; Cell Signaling Technology), Bcl-2 (Cat. ab196495, 1:1,000; Abcam, Cambridge, UK), Bax (Cat. 2772, 1:1,000; Cell Signaling Technology), cleaved Caspase 3 (Cat. 9664, 1:1,000; Cell Signaling Technology), and GAPDH (Cat. AT0002, CMC TAG, 1:5,000). After that, the proteins were probed with horseradish peroxidase (HRP)-conjugated secondary antibodies and visualized by a ChemiDoc Imaging System (Bio-Rad Laboratories, Hercules, CA, USA). Then the relative quantification of proteins was presented as the ratio of target proteins to GAPDH.
Statistical Analysis
All data were presented as mean ± SD. Data were analyzed with the GraphPad Prism Software version 7.0 (GraphPad Software, San Diego, CA, USA). Normality analysis of data was performed by the Shapiro–Wilk test. Statistical significance (P < 0.05) was estimated by one-way ANOVA followed by Bonferroni correction for post-hoc t-test.
Results
Electroacupuncture Pretreatment Protected Cardiac Function Against Myocardial Ischemia/Reperfusion Injury
To evaluate the effects of EA on cardiac function following MI/R injury and EA treatment, echocardiography was performed. M-mode images were obtained to measure LVEF and LVFS so as to evaluate cardiac contractile function. As shown in Figure 1, MI/R significantly reduced LVEF and LVFS compared with sham group, while EA pretreatment for 3 consecutive days greatly increased LVEF and LVFS compared with MI/R group. These data demonstrated that MI/R resulted in compromised cardiac function, while EA pretreatment improved cardiac function, which was impaired by MI/R injury. However, cardiac function in EA+sham group was not significantly altered compared with sham group, implying no obvious effects on cardiac function by EA pretreatment in sham-operated mice. These results data together revealed that EA pretreatment elevated MI/R-induced reduction of LVEF and LVFS, thus protecting hearts from MI/R injury.
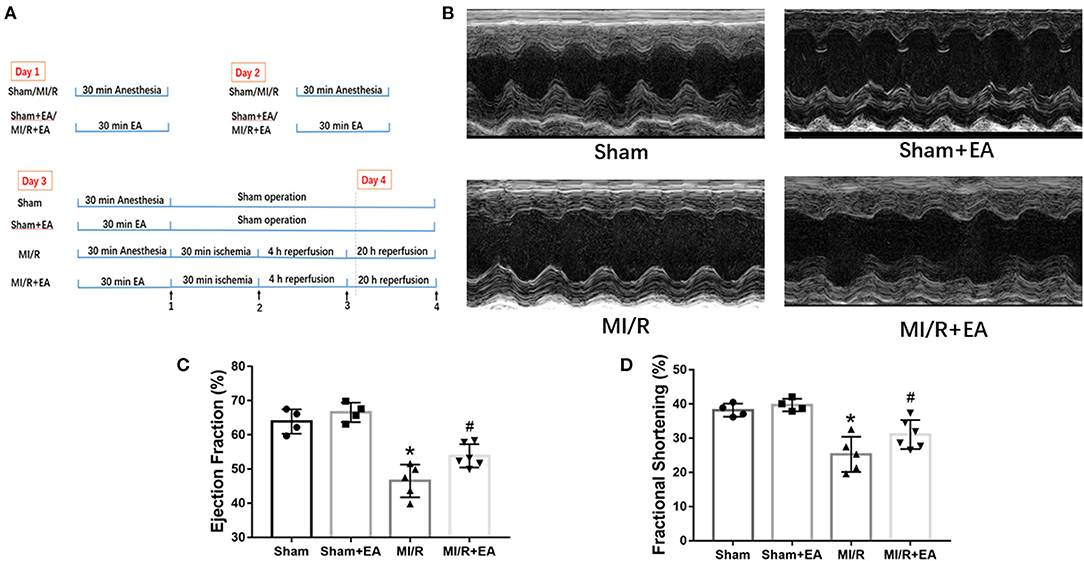
Figure 1. EA pretreatment mitigated MI/R-induced cardiac dysfunction. (A) A schematic illustration of the study groups and in vivo experimental protocols. Time point 1: ischemic phase. Time point 2: reperfusion phase. Time point 3: heart tissue collection for protein analysis. Time point 4: echo analysis and TTC staining. (B) Representative images of M-mode echocardiography in MI/R-induced cardiac injury following EA pretreatment. Images of M-mode echocardiography were recorded from horizontal direction of the heart and then were analyzed to assess cardiac systolic function. (C) Ejection fraction. (D) Fractional shortening. n = 4–6 in each group. EA, electroacupuncture; MI/R, myocardial ischemia/reperfusion; TTC, triphenyltetrazolium chloride. *P < 0.05 vs. Sham group, #P < 0.05 vs. MI/R group.
Electroacupuncture Pretreatment Reduced Myocardial Infarct Size and the Activities of Lactate Dehydrogenase and Creatine Kinase-MB
To further investigate whether EA pretreatment could affect MI/R-induced myocardial infarct size and myocardial cell death, TTC/Evans blue double staining was used to assess infarct size; and LDH and CK-MB activities were determined to evaluate myocardial cell death. Our results showed that MI/R led to a significant increase of myocardial infarct size compared with sham group, while EA pretreatment dramatically reduced myocardial infarct size compared with MI/R group as shown in Figure 2. The above result clearly showed that EA pretreatment significantly reduced MI/R-induced myocardial infarct size. Furthermore, the activities of LDH and CK-MB in the serum were increased in response to MI/R injury compared with sham group, while EA pretreatment decreased the activities of LDH and CK-MB in EA+MI/R group compared with MI/R group as shown in Figure 2. These data revealed that myocardial cell death caused by MI/R injury was inhibited following EA pretreatment. Taken together, these results indicated that EA pretreatment decreased myocardial infarct size and LDH and CK-MB release to protect hearts from MI/R injury.
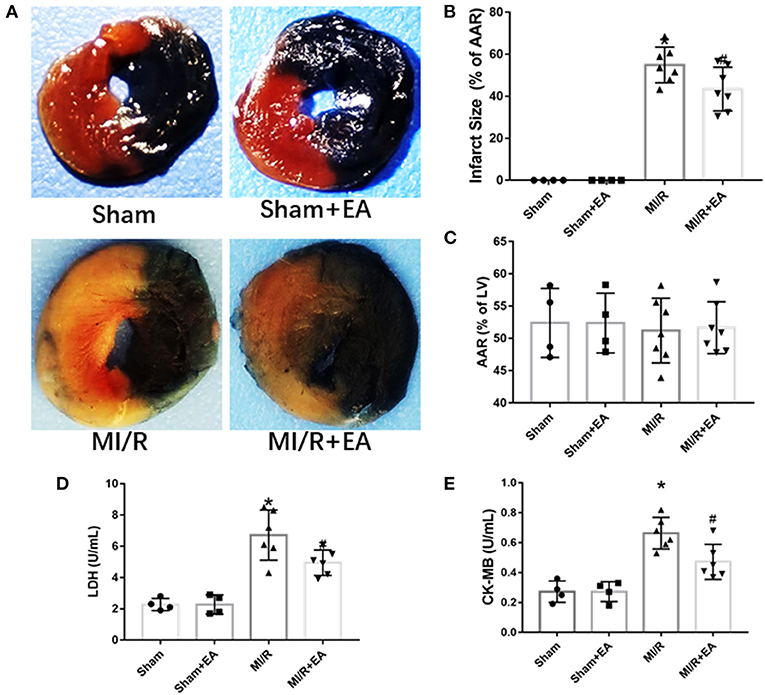
Figure 2. The effects of EA pretreatment on myocardial infarct size, LDH, and CK-MB activities in MI/R-induced cardiac injury. (A) Representative images of cardiac slices by TTC/Evans blue double staining in MI/R-induced cardiac injury following EA pretreatment. (B) Myocardial infarct size of different groups. (C) Myocardial area at risk (AAR) following MI/R procedure and EA treatment. n = 4–7 in each group for TTC/Evans blue double staining. (D) Serum LDH activity. (E) Serum CK-MB activity. n = 4–6 in each group for enzyme activity measurements. LDH, lactate dehydrogenase; CK-MB, creatine kinase-MB isoform; EA, electroacupuncture; MI/R, myocardial ischemia/reperfusion; TTC, triphenyltetrazolium chloride. *P < 0.05 vs. Sham group, #P < 0.05 vs. MI/R group.
Electroacupuncture Reduced Myocardial Ischemia/Reperfusion-Induced Cell Apoptosis and Activated XBP1/GRP78/Akt Pathway
To elucidate the role of cell apoptosis in EA protection against MI/R injury, cell apoptosis among different groups was determined. As shown in Figure 3, MI/R significantly enhanced myocardial apoptosis compared with sham group. Moreover, EA pretreatment decreased MI/R-induced cell apoptosis by 27.6% compared with MI/R group, implying the protective role of EA on reducing cell apoptosis during MI/R injury.
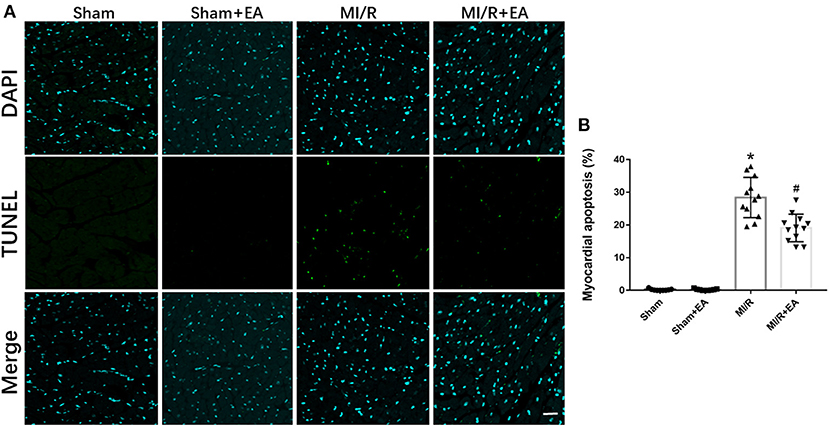
Figure 3. The effects of EA pretreatment on myocardial apoptosis in MI/R-induced cardiac injury. (A) Representative images of myocardial apoptosis by TUNEL staining in MI/R-induced cardiac injury following EA pretreatment. (B) Myocardial apoptosis of mice from different groups. n = 3–4 for each group. Three to four hearts in each group were used for TUNEL staining, and five random fields in three sections of each heart were used for further analysis. Bar, 100 μm. *P < 0.05 vs. Sham group, #P < 0.05 vs. MI/R group. EA, electroacupuncture; MI/R, myocardial ischemia/reperfusion.
To further investigate the potential molecular mechanisms underlying EA protection against MI/R injury, the expressions of XBP1/GRP78/Akt signaling pathway and apoptotic proteins were assessed. As shown in Figure 4, our data demonstrated that MI/R injury resulted in increase of XBP1, GRP78, and phosphorylated Akt (p-Akt) expressions, while the pro-apoptotic proteins including Bax and cleaved Caspase 3 were upregulated compared with sham group. The results showed that the expressions of XBP1, GRP78, and Akt were further increased, and the expressions of Bax and cleaved Caspase 3 were decreased following EA pretreatment. These results indicated that the molecular mechanism of EA protection against MI/R injury was at least partly via activation of XBP1/GRP78/Akt signaling pathway, therefore ultimately reducing cell apoptosis.
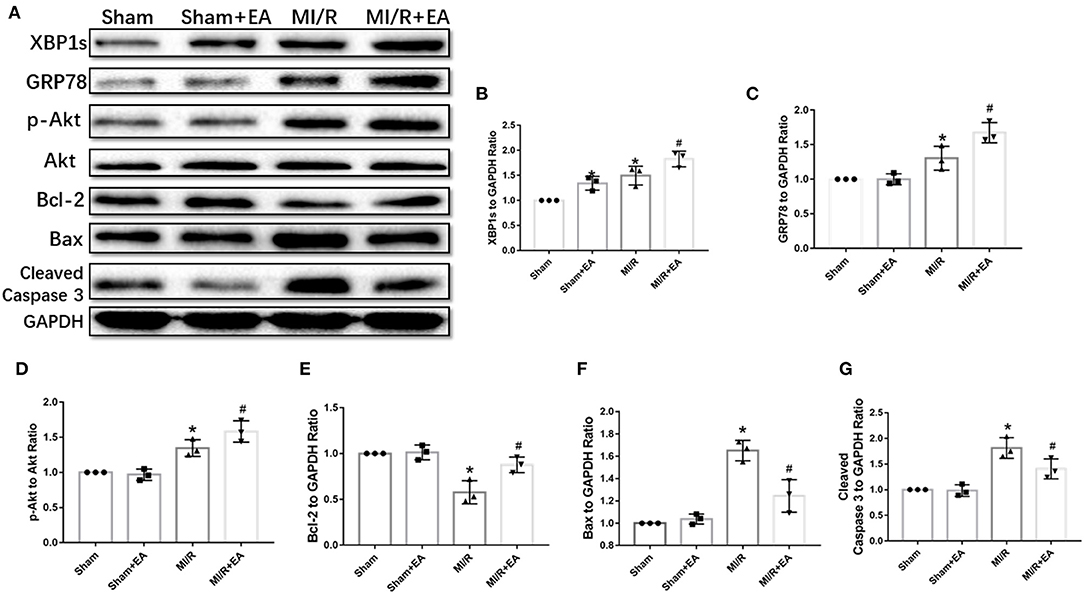
Figure 4. EA pretreatment activated XBP1/GRP78/Akt pathway and reduced apoptotic protein expressions. (A) Representative blot images of proteins related to XBP1/GRP78/Akt and apoptotic signaling pathways. (B–G) The quantitative analysis of XBP1, GRP78, p-Akt, Bcl-2, Bax, and cleaved Caspase 3 protein expressions. n = 3 in each group. *P < 0.05 vs. Sham group, #P < 0.05 vs. MI/R group. EA, electroacupuncture; MI/R, myocardial ischemia/reperfusion.
Inhibition of XBP1 Exacerbated Stimulated Ischemia/Reperfusion Injury and Cell Apoptosis in H9c2 Cells
To further investigate the role of XBP1-mediated signaling pathway in SI/R-induced cell injury, the inhibitor 4μ8C was used in in vitro study. The results in Figure 5 revealed that XBP1 inhibitor 4μ8C significantly exacerbated SI/R-induced H9c2 cell injury manifested by the increase of LDH and CK-MB activities and the elevation of cell apoptosis compared with the SI/R group. However, 4μ8C alone did not significantly affect the activities of LDH and CK-MB or cell apoptosis in normal conditions. These results showed that XBP1 inhibition could deteriorate SI/R-induced cell injury via increase of cell death.
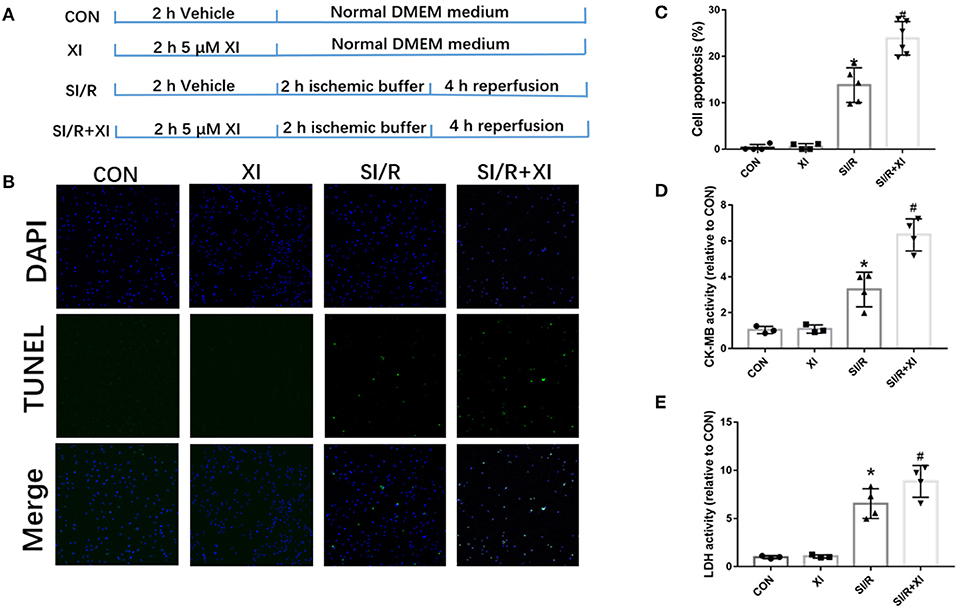
Figure 5. 4μ8C, an XBP1 inhibitor, increased H9c2 cell apoptosis LDH and CK-MB activities in vitro. (A) A schematic illustration of the study groups and in vitro experimental protocols. (B) Representative images of cell apoptosis by TUNEL staining in SI/R-induced cell injury following 4μ8C pretreatment. (C) The quantitative analysis of cell apoptosis following 4μ8C pretreatment. Eight random fields were selected for quantitation of cell apoptosis for each repeat experiment. (D) LDH activity. (E) CK-MB activity. n = 3–4 in each group. XI, XBP1 inhibitor; LDH, lactate dehydrogenase; CK-MB, creatine kinase-MB; SI/R, stimulated ischemia/reperfusion. Bar, 50 μm. *P < 0.05 vs. CON group, #P < 0.05 vs. SI/R group.
XBP1 Inhibition Downregulated GRP78/Akt Pathway in Stimulated Ischemia/Reperfusion-Injured H9c2 Cells
To demonstrate the effects of XBP1 inhibition on GRP78/Akt signaling pathway, we further explored the effects of 4μ8C on the XBP1/GRP78/Akt pathway. The western blotting in Figure 6 demonstrated that the XBP1 inhibitor 4μ8C downregulated XBP1, GRP78, Akt, and Bcl-2 expressions and elevated the expressions of Bax and cleaved Caspase 3 compared with MI/R group. Our in vitro results favored the notion that the inhibition of XBP1 could worsen SI/R-induced cell injury via regulation of GRP78/Akt pathway.
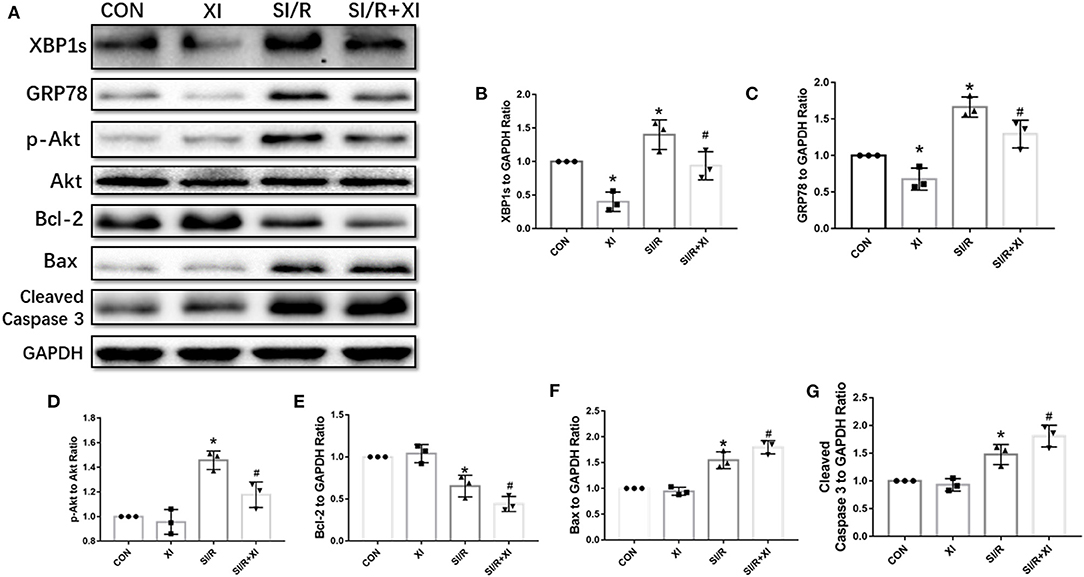
Figure 6. 4μ8C inhibited XBP1/GRP78/Akt pathway and enhanced apoptotic protein expressions in vitro. (A) Representative blot images of proteins related to XBP1/GRP78/Akt and apoptotic signaling pathways in H9c2 cells. (B–G) The quantitative analysis of XBP1, GRP78, p-Akt, Bcl-2, Bax, and cleaved Caspase 3 protein expressions. n = 3 in each group. *P < 0.05 vs. CON group, #P < 0.05 vs. SI/R group.
Discussion
The present study demonstrated that EA pretreatment attenuated MI/R-induced cardiac dysfunction and mitigated MI/R-induced damage by decreasing serum LDH, CK-MB, and myocardial apoptosis. The underlying molecular mechanism of EA protection was shown to be involved in the activation of XBP1/GRP78/Akt pathway. Further in vitro result revealed that inhibition of XBP1 decreased the downstream GRP78 and Akt expressions and elevated cell apoptosis, implying the importance of XBP1-mediated pathway against MI/R injury.
As a traditional Chinese medical therapy, EA is shown to be a beneficial treatment for several diseases including stress urinary incontinence, knee osteoarthritis, and acute ischemic cerebral apoplexy in clinical studies (23–25). Notably, EA significantly reduced myocardial injury induced by cardiac hypertrophy and ischemic insult in animal models and clinical studies (18, 19). EA pretreatment at Neiguan (PC6) acupoint mitigated cardiac hypertrophy via upregulation of ERK signaling pathway (26). Furthermore, Lujan et al. demonstrated that EA decreased the incidence of I/R-mediated ventricular tachyarrhythmias via lowering cardiac metabolic demand (27). Inhibition of cardiac norepinephrine release and regulation of opioid and PKC-dependent pathways by EA treatment attenuated MI/R injury in a rabbit model (17). Consistent with these previous studies, we observed that EA pretreatment for 3 consecutive days reduced myocardial infarct size, increased LVEF and LVFS, but suppressed the activities of LDH and CK-MB in MI/R injury. Moreover, EA pretreatment decreased the levels of expressions of Bax and Cleaved Caspase 3. These results clearly showed that EA pretreatment attenuated MI/R-induced cardiac contractile dysfunction and myocardial apoptosis.
Accumulating evidence has demonstrated that ER stress was markedly activated in I/R-injured myocardium (28, 29). ER stress is known to occur when the protein synthesis and protein process exceed its capacity in ER lumen under cellular stress. The UPR can be initiated by three signaling pathways including eIF2a-ATF4, IRE1a-XBP1, and ATF6 pathways to maintain ER homeostasis (30). Moreover, melatonin inhibited PERK-eIF2α-ATF4-mediated ER stress to protect cardiac function from MI/R injury (31). It was also revealed that ischemic preconditioning attenuated MI/R-induced injury through suppression of ER stress (32). All these data favored the notion that the inhibition of ER stress would be beneficial to mitigate myocardial ischemic injury. However, these protective effects were not observed by direct inhibition of key molecules in ER stress but the upstream regulators of ER stress. In contrast to these results, acute activation of ER stress by the key molecules involved in ER stress signaling pathway displayed cardioprotective roles in ischemic heart diseases, which was even attributed to the other molecular mechanisms beyond ER stress signaling pathways. ATF6 as a key mediator of one conserved branch of ER stress protected hearts from MI/R injury via inducing the expressions of catalase and protein disulfide isomerase (10, 11). Furthermore, ATF6 binds to the promoter of the protein disulfide isomerase associated 6 (pdia6) gene to protect cardiomyocytes against simulated I/R-induced death in vitro (33). GRP78 as a signal sensor of ER stress activated by ischemic preconditioning attenuated ischemic injury in cardiomyocytes via activation of Nrf2/HO-1 pathway (34, 35). Cardiomyocyte-specific overexpression of GRP78 protected hearts from MI/R injury in vivo and in vitro through stimulation of Akt signaling pathway (12). Moreover, this study revealed that GRP78 could interact with phosphatidylinositol 3-kinase and therefore lead to the stimulation of Akt (12). Thus, our present study was designed to investigate whether activation of XBP1 could activate GRP78/Akt signaling pathway to protect hearts from MI/R injury. Our results revealed that EA pretreatment markedly upregulated XBP1 expression and the downstream GRP78 expression during MI/R injury. Then GRP78 further enhanced Akt signaling to reduce MI/R-induced cell apoptosis. Additionally, in an in vitro SI/R model, inhibition of XBP1 could significantly reduce cell viability and increase cell apoptosis and the activities of LDH and CK-MB, indicating a vital role of XBP1 in the pathology of MI/R injury.
The key role of Akt signaling in the pathogenesis of MI/R injury has been illustrated in numerous previous studies. As an important anti-apoptotic pathway, promotion of Akt signaling pathway significantly reduced cell apoptosis to protect hearts and cardiomyocytes from I/R injury (36–38). Our data consistently showed that cell apoptosis determined by TUNEL staining and apoptotic protein expressions was significantly reduced by the induction of XBP1/GRP78/Akt axis. The inhibition of XBP1 in H9c2 cells inhibited Akt phosphorylation, thus execrating cell injury and apoptosis. Our study clearly showed that the inhibition of XBP1 worsened SI/R-induced cell injury.
However, there are some limitations in the present study. First, although we demonstrated that EA pretreatment could increase XBP1 expression, the mechanisms and the upstream regulators of XBP1 were still absent and need further exploration. We speculate that EA pretreatment may lead to the overall metabolic changes of the muscle and alter the profile of myokines. Second, the time point of EA treatment was before MI/R surgery. This will limit the clinical use, and the post-ischemia treatment is more reasonable for clinical application. Third, the in vitro data can only demonstrate that the XBP1/GRP78/Akt pathway participates in SI/R-induced cell injury since EA cannot directly treat cell in vitro.
In summary, the results of this study suggest for the first time that EA pretreatment upregulated XBP1/GRP78/Akt signaling pathway and improved cardiac function during MI/R injury. Clarification of upstream of XBP1 in the pathological process of MI/R injury will be required to better understand the action of EA protection, which may not only contribute to elucidation of the molecular mechanism but have potential clinical use.
Data Availability Statement
The raw data supporting the conclusions of this article will be made available by the authors, without undue reservation.
Ethics Statement
The animal study was reviewed and approved by the Animal Care Committee of Air Force Medical University.
Author Contributions
LY, JY, and MZ designed and supervised the study and revised the manuscript. NW, JM, YM, and CM conducted the experiments and collected the data. LL and PQ collected and analyzed the data. EG established the animal model. NW and JM wrote the draft. All authors approved the final manuscript.
Funding
This work was supported by grants from National Natural Science Foundation of China (81774415, 81600295), Shaanxi Province Science and Technology Innovation Team Project (2020TD-034), and the Subject Boosting Project of Xijing Hospital (XJZT18Z02, XJZT18MJ14). All the funds have no role in the design of the research.
Conflict of Interest
The authors declare that the research was conducted in the absence of any commercial or financial relationships that could be construed as a potential conflict of interest.
References
1. Nowbar AN, Gitto M, Howard JP, Francis DP, Al-Lamee R. Mortality from ischemic heart disease. Circ Cardiovasc Qual Outcomes. (2019) 12:e005375. doi: 10.1161/CIRCOUTCOMES.118.005375
2. Fewell SW, Travers KJ, Weissman JS, Brodsky JL. The action of molecular chaperones in the early secretory pathway. Annu Rev Genet. (2001) 35:149–91. doi: 10.1146/annurev.genet.35.102401.090313
3. Wang S, Binder P, Fang Q, Wang Z, Xiao W, Liu W, et al. Endoplasmic reticulum stress in the heart: insights into mechanisms and drug targets. Br J Pharmacol. (2018) 175:1293–304. doi: 10.1111/bph.13888
4. Sozen E, Karademir B, Ozer NK. Basic mechanisms in endoplasmic reticulum stress and relation to cardiovascular diseases. Free Radic Biol Med. (2015) 78:30–41. doi: 10.1016/j.freeradbiomed.2014.09.031
5. Yoshida H, Matsui T, Yamamoto A, Okada T, Mori K. XBP1 mRNA is induced by ATF6 and spliced by IRE1 in response to ER stress to produce a highly active transcription factor. Cell. (2001) 107:881–91. doi: 10.1016/S0092-8674(01)00611-0
6. Liu MQ, Chen Z, Chen LX. Endoplasmic reticulum stress: a novel mechanism and therapeutic target for cardiovascular diseases. Acta Pharmacol Sin. (2016) 37:425–43. doi: 10.1038/aps.2015.145
7. Okada K, Minamino T, Tsukamoto Y, Liao Y, Tsukamoto O, Takashima S, et al. Prolonged endoplasmic reticulum stress in hypertrophic and failing heart after aortic constriction: possible contribution of endoplasmic reticulum stress to cardiac myocyte apoptosis. Circulation. (2004) 110:705–12. doi: 10.1161/01.CIR.0000137836.95625.D4
8. Yao Y, Lu Q, Hu Z, Yu Y, Chen Q, Wang QK. A non-canonical pathway regulates ER stress signaling and blocks ER stress-induced apoptosis and heart failure. Nat Commun. (2017) 8:133. doi: 10.1038/s41467-017-00171-w
9. Doroudgar S, Thuerauf DJ, Marcinko MC, Belmont PJ, Glembotski CC. Ischemia activates the ATF6 branch of the endoplasmic reticulum stress response. J Biol Chem. (2009) 284:29735–45. doi: 10.1074/jbc.M109.018036
10. Martindale JJ, Fernandez R, Thuerauf D, Whittaker R, Gude N, Sussman MA, et al. Endoplasmic reticulum stress gene induction and protection from ischemia/reperfusion injury in the hearts of transgenic mice with a tamoxifen-regulated form of ATF6. Circ Res. (2006) 98:1186–93. doi: 10.1161/01.RES.0000220643.65941.8d
11. Jin JK, Blackwood EA, Azizi K, Thuerauf DJ, Fahem AG, Hofmann C, et al. ATF6 decreases myocardial ischemia/reperfusion damage and links ER stress and oxidative stress signaling pathways in the heart. Circ Res. (2017) 120:862–75. doi: 10.1161/CIRCRESAHA.116.310266
12. Bi X, Zhang G, Wang X, Nguyen C, May HI, Li X, et al. Endoplasmic reticulum chaperone GRP78 protects heart from ischemia/reperfusion injury through Akt activation. Circ Res. (2018) 122:1545–54. doi: 10.1161/CIRCRESAHA.117.312641
13. Cunha DA, Gurzov EN, Naamane N, Ortis F, Cardozo AK, Bugliani M, et al. JunB protects beta-cells from lipotoxicity via the XBP1-AKT pathway. Cell Death Differ. (2014) 21:1313–24. doi: 10.1038/cdd.2014.53
14. Zhang S, Wu B, Liu M, Li N, Zeng X, Liu H, et al. Acupuncture efficacy on ischemic stroke recovery: multicenter randomized controlled trial in China. Stroke. (2015) 46:1301–6. doi: 10.1161/STROKEAHA.114.007659
15. Bonica JJ. Therapeutic acupuncture in the People's Republic of China implications for American medicine. JAMA. (1974) 228:1544–51. doi: 10.1001/jama.228.12.1544
16. Huo ZJ, Li Q, Tian GH, Zhou CM, Wei XH, Pan CS, et al. The ameliorating effects of long-term electroacupuncture on cardiovascular remodeling in spontaneously hypertensive rats. BMC Complement Altern Med. (2014) 14:118. doi: 10.1186/1472-6882-14-118
17. Zhou W, Ko Y, Benharash P, Yamakawa K, Patel S, Ajijola OA, et al. Cardioprotection of electroacupuncture against myocardial ischemia-reperfusion injury by modulation of cardiac norepinephrine release. Am J Physiol Heart Circ Physiol. (2012) 302:H1818–25. doi: 10.1152/ajpheart.00030.2012
18. Zhang H, Liu L, Huang G, Zhou L, Wu W, Zhang T, et al. Protective effect of electroacupuncture at the Neiguan point in a rabbit model of myocardial ischemia-reperfusion injury. Can J Cardiol. (2009) 25:359–63. doi: 10.1016/S0828-282X(09)70095-9
19. Yang L, Yang J, Wang Q, Chen M, Lu Z, Chen S, et al. Cardioprotective effects of electroacupuncture pretreatment on patients undergoing heart valve replacement surgery: a randomized controlled trial. Ann Thorac Surg. (2010) 89:781–6. doi: 10.1016/j.athoracsur.2009.12.003
20. Gao E, Lei YH, Shang X, Huang ZM, Zuo L, Boucher M, et al. A novel and efficient model of coronary artery ligation and myocardial infarction in the mouse. Circ Res. (2010) 107:1445–53. doi: 10.1161/CIRCRESAHA.110.223925
21. Zhang J, Song J, Xu J, Chen X, Yin P, Lv X, et al. ERK1/2-Egr-1 signaling pathway-mediated protective effects of electroacupuncture in a mouse model of myocardial ischemia-reperfusion. Evid Based Complement Alternat Med. (2014) 2014:253075. doi: 10.1155/2014/253075
22. Lu L, Ma J, Sun M, Wang X, Gao E, Lu L, et al. Melatonin ameliorates MI-induced cardiac remodeling and apoptosis through a JNK/p53-dependent mechanism in diabetes mellitus. Oxid Med Cell Longev. (2020) 2020:1535201. doi: 10.1155/2020/1535201
23. Liu Z, Liu Y, Xu H, He L, Chen Y, Fu L, et al. Effect of electroacupuncture on urinary leakage among women with stress urinary incontinence: a randomized clinical trial. JAMA. (2017) 317:2493–01. doi: 10.1001/jama.2017.7220
24. Chen N, Wang J, Mucelli A, Zhang X, Wang C. Electro-acupuncture is beneficial for knee osteoarthritis: the evidence from meta-analysis of randomized controlled trials. Am J Chin Med. (2017) 45:965–85. doi: 10.1142/S0192415X17500513
25. Wang C, Wu Z, Li N, Zhao Y, Tian F, Zhou X, et al. Clinical curative effect of electric acupuncture on acute cerebral infarction: a randomized controlled multicenter trial. J Tradit Chin Med. (2014) 34:635–40. doi: 10.1016/S0254-6272(15)30075-3
26. Li J, Li J, Liang F, Hong Y, Wu S, Tang H, et al. Electroacupuncture at PC6 (Neiguan) improves extracellular signal-regulated kinase signaling pathways through the regulation of neuroendocrine cytokines in myocardial hypertrophic rats. Evid Based Complement Alternat Med. (2012) 2012:792820. doi: 10.1155/2012/792820
27. Lujan HL, Kramer VJ, DiCarlo SE. Electroacupuncture decreases the susceptibility to ventricular tachycardia in conscious rats by reducing cardiac metabolic demand. Am J Physiol Heart Circ Physiol. (2007) 292:H2550–5. doi: 10.1152/ajpheart.00979.2006
28. Wang XB, Huang XM, Ochs T, Li XY, Jin HF, Tang CS, et al. Effect of sulfur dioxide preconditioning on rat myocardial ischemia/reperfusion injury by inducing endoplasmic reticulum stress. Basic Res Cardiol. (2011) 106:865–78. doi: 10.1007/s00395-011-0176-x
29. Zhao GL, Yu LM, Gao WL, Duan WX, Jiang B, Liu XD, et al. Berberine protects rat heart from ischemia/reperfusion injury via activating JAK2/STAT3 signaling and attenuating endoplasmic reticulum stress. Acta Pharmacol Sin. (2016) 37:354–67. doi: 10.1038/aps.2015.136
30. Wang X, Xu L, Gillette TG, Jiang X, Wang ZV. The unfolded protein response in ischemic heart disease. J Mol Cell Cardiol. (2018) 117:19–25. doi: 10.1016/j.yjmcc.2018.02.013
31. Yu L, Li B, Zhang M, Jin Z, Duan W, Zhao G, et al. Melatonin reduces PERK-eIF2alpha-ATF4-mediated endoplasmic reticulum stress during myocardial ischemia-reperfusion injury: role of RISK and SAFE pathways interaction. Apoptosis. (2016) 21:809–24. doi: 10.1007/s10495-016-1246-1
32. Liu M, Wang Y, Zhu Q, Zhao J, Wang Y, Shang M, et al. Protective effects of circulating microvesicles derived from ischemic preconditioning on myocardial ischemia/reperfusion injury in rats by inhibiting endoplasmic reticulum stress. Apoptosis. (2018) 23:436–48. doi: 10.1007/s10495-018-1469-4
33. Vekich JA, Belmont PJ, Thuerauf DJ, Glembotski CC. Protein disulfide isomerase-associated 6 is an ATF6-inducible ER stress response protein that protects cardiac myocytes from ischemia/reperfusion-mediated cell death. J Mol Cell Cardiol. (2012) 53:259–67. doi: 10.1016/j.yjmcc.2012.05.005
34. Shintani-Ishida K, Nakajima M, Uemura K, Yoshida K. Ischemic preconditioning protects cardiomyocytes against ischemic injury by inducing GRP78. Biochem Biophys Res Commun. (2006) 345:1600–5. doi: 10.1016/j.bbrc.2006.05.077
35. Ji H, Xiao F, Li S, Wei R, Yu F, Xu J. GRP78 effectively protect hypoxia/reperfusion-induced myocardial apoptosis via promotion of the Nrf2/HO-1 signaling pathway. J Cell Physiol. (2020) 236:1228–36. doi: 10.1002/jcp.29929
36. Miao W, Luo Z, Kitsis RN, Walsh K. Intracoronary, adenovirus-mediated Akt gene transfer in heart limits infarct size following ischemia-reperfusion injury in vivo. J Mol Cell Cardiol. (2000) 32:2397–402. doi: 10.1006/jmcc.2000.1283
37. Fujio Y, Nguyen T, Wencker D, Kitsis RN, Walsh K. Akt promotes survival of cardiomyocytes in vitro and protects against ischemia-reperfusion injury in mouse heart. Circulation. (2000) 101:660–7. doi: 10.1161/01.CIR.101.6.660
Keywords: electroacupuncture, myocardial ischemia/reperfusion injury, XBP1s, apoptosis, Akt
Citation: Wang N, Ma J, Ma Y, Lu L, Ma C, Qin P, Gao E, Zuo M, Yang J and Yang L (2021) Electroacupuncture Pretreatment Mitigates Myocardial Ischemia/Reperfusion Injury via XBP1/GRP78/Akt Pathway. Front. Cardiovasc. Med. 8:629547. doi: 10.3389/fcvm.2021.629547
Received: 15 November 2020; Accepted: 09 April 2021;
Published: 14 June 2021.
Edited by:
Christophe Piot, Clinique du Millénaire, FranceReviewed by:
Tom Van Agtmael, University of Glasgow, United KingdomStéphanie Barrere-Lemaire, INSERM U1191 Institut de Génomique Fonctionnelle (IGF), France
Copyright © 2021 Wang, Ma, Ma, Lu, Ma, Qin, Gao, Zuo, Yang and Yang. This is an open-access article distributed under the terms of the Creative Commons Attribution License (CC BY). The use, distribution or reproduction in other forums is permitted, provided the original author(s) and the copyright owner(s) are credited and that the original publication in this journal is cited, in accordance with accepted academic practice. No use, distribution or reproduction is permitted which does not comply with these terms.
*Correspondence: Lifang Yang, eWFuZ2xpZmFuZzYmI3gwMDA0MDtob3RtYWlsLmNvbQ==
†These authors have contributed equally to this work