- 1Department of Nuclear Sciences and Engineering, Nanjing University of Aeronautics and Astronautics, Nanjing, China
- 2JYAMS PET Research and Development Limited, Nanjing, China
Background: Myocardial perfusion imaging modalities, such as cardiac magnetic resonance (CMR), single-photon emission computed tomography (SPECT), and positron emission tomography (PET), are well-established non-invasive diagnostic methods to detect hemodynamically significant coronary artery disease (CAD). The aim of this meta-analysis is to compare CMR, SPECT, and PET in the diagnosis of CAD and to provide evidence for further research and clinical decision-making.
Methods: PubMed, Web of Science, EMBASE, and Cochrane Library were searched. Studies that used CMR, SPECT, and/or PET for the diagnosis of CAD were included. Pooled sensitivity, specificity, positive likelihood ratio, negative likelihood ratio, diagnostic odds ratio with their respective 95% confidence interval, and the area under the summary receiver operating characteristic (SROC) curve were calculated.
Results: A total of 203 articles were identified for inclusion in this meta-analysis. The pooled sensitivity values of CMR, SPECT, and PET were 0.86, 0.83, and 0.85, respectively. Their respective overall specificity values were 0.83, 0.77, and 0.86. Results in subgroup analysis of the performance of SPECT with 201Tl showed the highest pooled sensitivity [0.85 (0.82, 0.88)] and specificity [0.80 (0.75, 0.83)]. 99mTc-tetrofosmin had the lowest sensitivity [0.76 (0.67, 0.82)]. In the subgroup analysis of PET tracers, results indicated that 13N had the lowest pooled sensitivity [0.83 (0.74, 0.89)], and the specificity was the highest [0.91 (0.81, 0.96)].
Conclusion: Our meta-analysis indicates that CMR and PET present better diagnostic performance for the detection of CAD as compared with SPECT.
Introduction
Coronary artery disease (CAD) is one of the main causes of mortality worldwide, which is mainly attributed to coronary artery atherosclerosis (1). It is noteworthy that the burden of atherosclerotic cardiovascular disease in low- and middle-income countries is still increasing (2). Based on Global Burden of Disease program, statistics revealed that the global prevalence of CAD had reached ~154 million in the year 2016 (3). The overriding goal of common treatment approaches is to relieve symptom through drug therapy and vascular remodeling and to avoid potential cardiovascular events in the future (4–7).
At present, it is important to assess the degree of coronary artery stenosis accurately, so as to provide the basis for the upcoming treatment. Fractional flow reserve (FFR) has been regarded as a mature and precise approach to evaluate the hemodynamic relevance of a coronary artery stenosis; nevertheless, FFR is also an invasive approach (8). Non-invasive myocardial perfusion imaging (MPI) modalities, such as cardiac magnetic resonance (CMR), single-photon emission computed tomography (SPECT), and positron emission tomography (PET), are well-established methods for the detection of hemodynamically significant CAD (9–12). CMR characterized by high spatial resolution images of myocardial perfusion has been verified to be of assistance in guiding patients with CAD (13–15). On the other hand, SPECT is the most commonly performed diagnostic method in patients with stable coronary heart disease. Although SPECT has been reported that the sensitivity of detection of high-risk subgroups is not sounding, there are certain inaccuracies in screening patients for invasive coronary angiography (CA) (16–18). Common SPECT radiotracers include 99mTc-sestamibi (99mTc-MIBI), 99mTc-tetrofosmin, and 201Tl (19–21). PET is also a widely used MPI modality for the detection of hemodynamic significance in CAD. It offers better diagnostic performance, improved resolution of images, and inherent attenuation correction (22–24). Furthermore, compared with SPECT protocol, PET imaging provides lower radiation exposure to patients due to the physical property of radiotracers including 82Rb, 13N-ammonia, and 15O-H2O (25– 27).
To our knowledge, accumulated studies and meta-analysis have evaluated diagnostic performance of both invasive and non-invasive approaches for the confirmation of CAD (28–34). The aim of this meta-analysis was to generate a more comprehensive comparison of CMR, SPECT, and PET in the detection of CAD by collating the available evidence and subsequently to provide meaningful and hints for not only the field of implement research but also for the implementation and decision-making in clinical settings.
Materials and Methods
Each process of this meta-analysis was conducted based on preferred reporting items for systematic reviews and meta-analysis (PRISMA) (35).
Search Strategy and Selection Criteria
We conducted a systematic search of the electronic databases, PubMed, Web of Science, EMBASE, and Cochrane Library from inception to July 31, 2020, with articles in English language considered. The following key terms were used for the database research: “cardiac magnetic resonance,” “CMR,” “single-photon emission computed tomography,” “SPECT,” “positron emission tomography,” “PET,” “myocardial perfusion imaging,” “MPI,” and “coronary artery disease.” The bibliographies of these articles were also screened for any eligible studies. Inclusion criteria were as follows: (1) CMR, SPECT, and/or PET were used for the diagnosis of CAD in patients with suspected or confirmed CAD; (2) either CA or FFR was referred as the gold standard to assess diagnostic performance; (3) absolute numbers of participants with true positive (TP), false positive (FP), true negative (TN), and false negative (FN) outcomes could be excerpted directly in the original article or calculated on the basis of information presented in the literature. If studies were conducted by the same group, those with the largest sample size or the most sufficient information were enrolled. Articles were excluded if they were case report, review, letters, news, conference abstract, animal study, or studies without the necessary variables mentioned above.
Two independent investigators (Jianfeng Xu and Fei Cai) conducted the process of literature search and study inclusion. Discrepancies were resolved by discussion. If no consensus was reached, a third author (Changran Geng) was involved.
Data Extraction and Quality Assessments
Two reviewers (Zheng Wang and Xiaobin Tang) independently performed the title and abstract screening according to the inclusion criteria. Full-text reading of the literature was conducted for the final inclusion. The following information was extracted from each study: first author's name, year of publication, number of patients analyzed, reference standard (CA or FFR), level of analysis (patient-based or vessel-based), MPI modality and radiotracers used in the study, and absolute number of patients with TP, TN, FP, and FN results.
To assess the quality of the included studies, we used the Quality Assessment of Diagnostic Accuracy Studies-2 (QUADAS-2) criteria. This method contains components in terms of participant selection, index test, and reference standard, as well as flow and timing (36).
Statistical Analysis
The Stata 15.0 software and Review Manager 5.3 software were employed for all statistical analyses at the study level. p < 0.05 was considered to be of statistical significance. We calculated pooled sensitivity, specificity, positive likelihood ratio, negative likelihood ratio, diagnostic odds ratio, and the 95% confidence intervals and the area under the summary receiver operating characteristic curve (AUC). We used Cochran Q and the I2 statistics to detect the heterogeneity of studies included. I2 values of 0–25%, 25–50%, 50–75%, and 75–100% indicated insignificant, low, moderate, and high heterogeneity, respectively (37). Metaregression was performed to explore the possible source of heterogeneity between the included studies. A Hanley and McNeil method was used to assess for potential differences in AUCs. χ2 tests were employed to compare the differences in sensitivities and specificities using the bivariate model. We created funnel plots to assess potential bias of publication. Deeks' method was used to statistically check the asymmetry of the funnel plot and detect publication bias. We conducted sensitivity analysis to evaluate the impacts of single study on the overall outcomes.
Results
Study Selection and Characteristics
A total of 4,052 articles were identified from the databases searched. Among them, 581 duplicates were removed, and 2,801 studies were excluded through an initial screening. After a full text assessment for eligibility of the remaining 670 articles, 203 articles with 215 studies, 23,942 patients, and 20,213 artery territories were identified for inclusion in this meta-analysis. No additional studies were found through reference screening of the included articles. Figure 1 shows the flow of the database search and literature selection process. The results of quality evaluation of the included studies manifested that the high quality of the included studies (see Figure 2).
Diagnostic Performance of MPI Modalities
The numbers of articles included in the analysis of CMR, SPECT, and PET were 56, 134, and 25, respectively. The pooled sensitivities of CMR, SPECT, and PET were 0.86 (0.84, 0.88), 0.83 (0.81, 0.85), and 0.85 (0.80, 0.89), respectively (p = 0.109). The overall specificities were 0.83 (0.81, 0.86), 0.77 (0.74, 0.80), and 0.86 (0.81, 0.89) for CMR, SPECT, and PET, respectively (SPECT vs. PET, p < 0.01; SPECT vs. CMR, p < 0.01; PET vs. CMR, p = 0.716). The AUC values of CMR, SPECT, and PET were 0.92 (0.89, 0.94), 0.87 (0.84, 0.90), and 0.92 (0.89, 0.94) (SPECT vs. PET, p < 0.01; SPECT vs. CMR, p < 0.01; PET vs. CMR, p = 0.215) (Table 1 and Figure 3). Two studies (Dekker et al. and Driessen et al.) reported evaluation of quantitative myocardial blood flow (MBF), and results showed that MBF was lower in patients with CAD than that in the non-CAD participants in both studies.
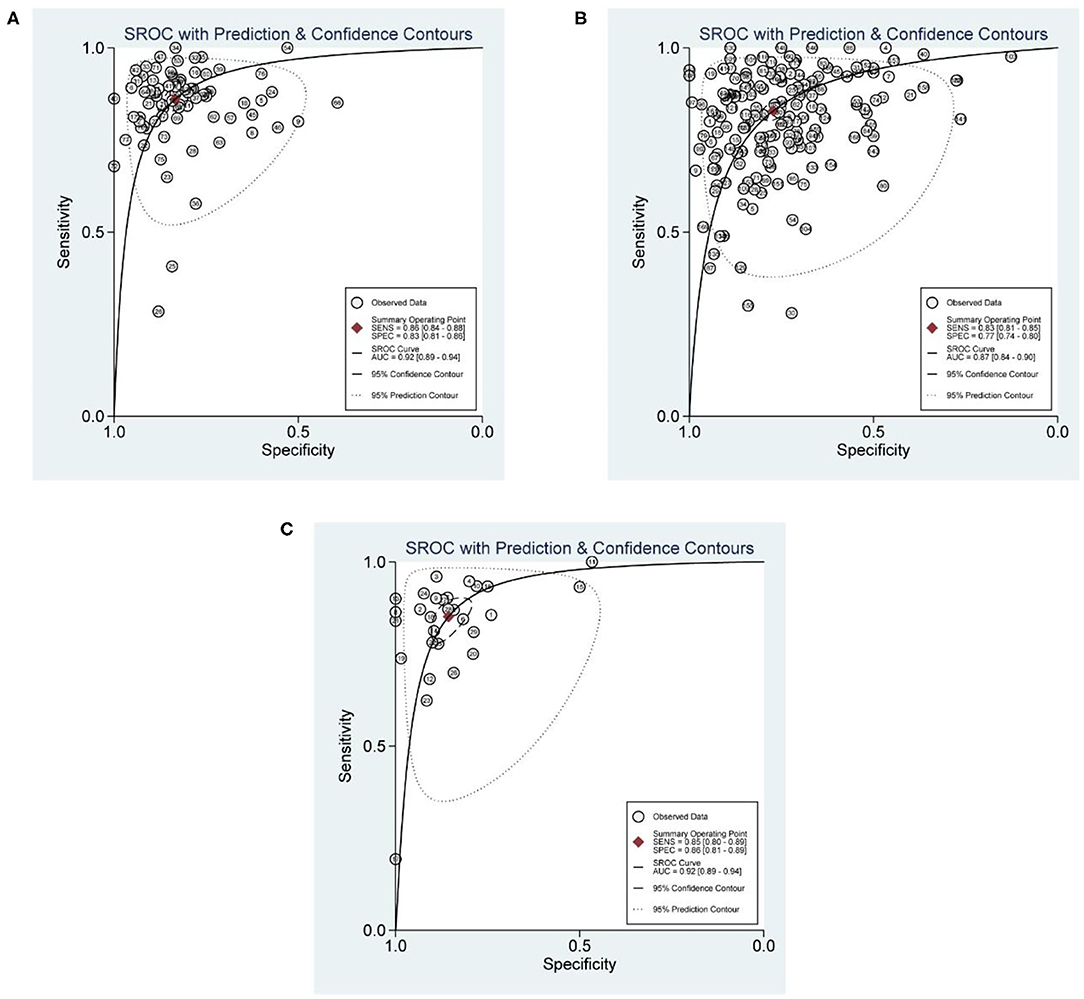
Figure 3. SROC curves for diagnostic performance of CMR, SPECT, and PET. (A) SROC curve for diagnostic performance of CMR. (B) SROC curves for diagnostic performance of SPECT. (C) SROC curves for diagnostic performance of PET.
Subgroup Analysis of the Performance of CMR
With regard to the reference standard, the overall sensitivity of CMR was 0.87 (0.83, 0.89) when CA was the reference standard, it yielded a specificity of 0.82 (0.79, 0.85), and the AUC value was 0.91 (0.88, 0.93). When FFR was used as the reference standard, the pooled sensitivity and specificity were 0.85 (0.81, 0.88) and 0.86 (0.81, 0.89), with an AUC value of 0.91 (0.88, 0.93). The pooled sensitivity was higher at the patient level [0.88 (0.86, 0.90)] than that at the vessel level [0.83 (0.78, 0.86)]. The pooled specificities at the patient level and the territory level were 0.81 (0.78, 0.84) and 0.86 (0.82, 0.90), respectively. The AUCs were equal at the two levels (Table 2). The pooled sensitivity and specificity were 0.89 (0.85, 0.91) and 0.80 (0.76, 0.83) in prospective CMR studies. Results of subgroup analyses (type of study, data assessment, prevalence of CAD and multivessel disease, and patient selection) for the diagnostic performance of CMR on patient-based level are listed in Supplementary Table 4.
Subgroup Analysis of the Performance of SPECT
The numbers of studies using 99mTc-tetrofosmin, 99mTc-MIBI, and 201Tl for SPECT tracers were 21, 53, and 40, respectively. Studies that used two or more tracers were not rerolled in this pooled analysis. Results manifested that 201Tl showed the highest pooled sensitivity [0.85 (0.82, 0.88)] and specificity [0.80 (0.75, 0.83)]. 99mTc-tetrofosmin had the lowest sensitivity [0.76 (0.67, 0.82)]. The pooled specificity of 99mTc-MIBI was the lowest [0.76 (0.71, 0.80)]. The AUC values of 99mTc-tetrofosmin, 99mTc-MIBI, 201Tl were 0.85 (0.81, 0.88), 0.87 (0.8, 0.90), and 0.90 (0.87, 0.92), respectively. Analysis based on reference standard (CA or FFR) showed that the sensitivity was higher but the specificity was lower using CA as reference. Besides, analysis on the analytic level presented higher sensitivity at the patient level and higher specificity at the artery territory level. See more details in Table 3. The pooled sensitivity and specificity were 0.88 (0.86, 0.90) and 0.73 (0.69, 0.77) in prospective SPECT studies. Results of subgroup analyses (type of study, data assessment, prevalence of CAD and multivessel disease, and patient selection) for the diagnostic performance of SPECT are listed in Supplementary Table 5.
Subgroup Analysis of the Performance of PET
The numbers of studies utilizing 82Rb, 13N-ammonia, and 15O for PET tracers were 15, 5, and 3, respectively. One study that used 62Cu was not included in this pooled analysis. 82Rb demonstrated the highest pooled sensitivity [0.87 (0.79, 0.93)]. Although 13N showed the lowest pooled sensitivity [0.83 (0.74, 0.89)], its pooled specificity was the highest [0.91 (0.81, 0.96)]. The AUC values of 82Rb, 13N-ammonia, and 15O were 0.91 (0.89, 0.94), 0.92 (0.89, 0.94), and 0.88 (0.85, 0.91), respectively. Moreover, analysis on the basis of reference standard showed that the specificity was higher using CA as reference. Besides, with respect to analytic level, the pooled analysis yielded higher sensitivity at the patient level (Table 4). In prospective PET studies, the pooled sensitivity and specificity were 0.88 (0.74, 0.95) and 0.88 (0.66, 0.96). Results of subgroup analyses (type of study, data assessment, prevalence of CAD and multivessel disease, and patient selection) for the diagnostic performance of PET are listed in Supplementary Table 6.
Heterogeneity and Publication Bias
Deeks' tests for publication bias yielded p values of 0.81, <0.01, and 0.13 for CMR, SPECT, and PET, which revealed that there was possible publication bias in the analysis of SPECT.
Sensitivity Analysis
We performed the sensitivity analysis to assess the impacts of a single study on the overall outcomes. No outlier of CMR, SPECT, or PET was identified.
Discussion
CAD has become the primary cause of deaths worldwide (38). CA and FFR are commonly regarded as the reference standard for the detection and evaluation of prognosis of CAD. However, they are an invasive approach and have potential risks for human body (28, 39). In contrast, non-invasive techniques such as CMR, SPECT, and PET embraced higher spatial resolution and low dose of radiation (CMR is free of nuclear radiation); they are undergoing an increase in clinical utility despite the relatively high cost (12, 40, 41). Nowadays, they remain to be the most reliable approaches for diagnosis of CAD with hemodynamic significance and guide the choice of treatment and assessment prognosis (27).
We conducted a meta-analysis to estimate the performance of CMR, SPECT, and PET for the diagnosis of obstructive CAD. The analysis was based on study design, type of analysis performed in the individual studies, type of radiotracers for SPECT and PET, reference standard, analysis level, and patient selection, some of which have not been discussed in previous meta-analyses (27, 29, 42). This is one of the strengths of this study. Results revealed that CMR, SPECT, and PET presented high sensitivity and specificity for the detection of CAD. There were no statistically significant differences in sensitivities between CMR, SPECT, and PET (p = 0.109). The differences in specificities were statistically significant (SPECT vs. PET, p < 0.01; SPECT vs. CMR, p < 0.01; PET vs. CMR, p = 0.716). The AUC values of CMR, SPECT, and PET indicated that CMR and PET showed better diagnostic performance for the detection of CAD as compared with SPECT (SPECT vs. PET, p < 0.01; SPECT vs. CMR, p < 0.01; PET vs. CMR, p = 0.215). Results manifested that 201Tl showed the highest pooled sensitivity [0.85 (0.82, 0.88), p < 0.01] and specificity [0.80 (0.75, 0.83), p < 0.01]. 13N revealed the biggest AUC [0.92 (0.89, 0.94), p = 0.087]. For the three modalities, when CA was the reference standard, the overall sensitivity of CMR was higher, but the specificity was lower. Besides, the pooled analysis yielded higher sensitivity at the patient level than vessel level. The diagnostic performance in this analysis was not quite similar to previous meta-analyses (27, 29). In this study, when FFR was utilized as the reference standard, the AUCs for both CMR and PET were equal. The reason may be the different source of heterogeneity between studies included. Although other imaging techniques such as echocardiography and CT have been proven sensitive in previous studies, in consideration of the aim of this study and the considerable amount of articles included, we did not consider including the two modalities (43–45).
In this meta-analysis, we did a detailed literature retrieval to enhance the probability of searching as much related studies as we can. Two independent reviewers performed the entire process of data extraction using a standardized spreadsheet. Furthermore, we evaluated the heterogeneity between the studies included. There were significant heterogeneities among studies. Distinctions in the year of publication, study methodology and patient characteristics, and reference standard may contribute to the heterogeneity. Metaregression was performed to investigate the likely cause of heterogeneity. As was shown in the metaregression, for CMR, year of publication, reference standard, and analytic basis (patient or vessel based) were the possible source of heterogeneity. For the analysis of SPECT and PET, the source of heterogeneity may be attributed to the type of radiotracers, reference standard, and analytic basis. The inclusion of covariates in this meta-analysis was based on the information extracted from the included articles, and the potential role of covariates in scientific and clinical application was also considered. Accordingly, not all potential sources of heterogeneity were analyzed. This may bias our conclusion for heterogeneity assessment in the study. Sensitivity analysis indicated that after omitting one study after another, the pooled outcomes were robust in this meta-analysis. Despite the existence of heterogeneity and publication bias, the results of this analysis may provide hints and assistances for the field of further research and clinical decision in the diagnosis of CAD. As for research purpose, quantitative assessments and new radiotracers with a lower dose of nuclear exposure to patients and higher spatial resolution need to be further investigated in the context of the results of this meta-analysis. Besides, results of the International Study of Comparative Health Effectiveness With Medical and Invasive Approaches (ISCHEMIA) revealed that, as in comparison with the control group (medical therapy only), the intervention group (angiography, revascularization, and medical therapy) did not reduce the risk of ischemic cardiovascular events or death from any cause over a follow-up of 3.2 years (46, 47). Nevertheless, considering the significant limitations of the study, non-invasive testing will continue to be important to diagnose the etiology of chest pain syndromes, provide prognostic information, guide management decisions, and assess the effectiveness of therapy. In terms of clinical application, physicians' decisions should be made on the basis of level of expertise and the attainability of infrastructure in different clinical settings. Moreover, joint utilization of different detection techniques is recommended to improve diagnostic accuracy.
Data Availability Statement
The data analyzed in this study is subject to the following licenses/restrictions: The datasets during and/or analyzed during the current study available from the corresponding author on reasonable request. Requests to access these datasets should be directed to Xiaobin Tang, tangxiaobin@nuaa.edu.cn.
Author Contributions
JX and FC conceived and designed this study. JX and CG were responsible for the collection, extraction, and analysis of the data. JX was responsible for writing the paper. ZW and XT performed the quality evaluation and completed data analysis. XT polished the English language. All authors and participants reviewed the paper and reached an agreement to approve the final manuscript.
Conflict of Interest
JX and ZW were employed by the company JYAMS PET Research and Development Limited.
The remaining authors declare that the research was conducted in the absence of any commercial or financial relationships that could be construed as a potential conflict of interest.
Supplementary Material
The Supplementary Material for this article can be found online at: https://www.frontiersin.org/articles/10.3389/fcvm.2021.621389/full#supplementary-material
Abbreviations
CAD, coronary artery disease; ARBs, angiotensin receptor blockers; ACE, angiotensin-converting enzyme; FFR, fractional flow reserve; MPI, myocardial perfusion imaging; CMR, cardiac magnetic resonance; SPECT, single-photon emission computed tomography; PET, positron emission tomography; CT, computed tomography; PRISMA, preferred reporting items for systematic reviews and meta-analysis; TP, true positive; FP, false positive; TN, true negative; FN, false negative; +LR, positive likelihood ratio; –LR, negative likelihood ratio; DOR, diagnostic odds ratio; CI, confidence interval; SROC, summary receiver operating characteristic; AUC, area under the SROC curve; MBF, myocardial blood flow; QUADAS-2, Quality Assessment of Diagnostic Accuracy Studies-2; ISCHEMIA, International Study of Comparative Health Effectiveness With Medical and Invasive Approaches.
References
1. Shao C, Wang J, Tian J, Tang YD. Coronary artery disease: from mechanism to clinical practice. Adv Experimen Med Biol. (2020) 1177:1–36. doi: 10.1007/978-981-15-2517-9_1
2. Fowkes FG, Aboyans V, Fowkes FJ, McDermott MM, Sampson UK, Criqui MH. Peripheral artery disease: epidemiology and global perspectives. Nat Rev Cardiol. (2017) 14:156–70. doi: 10.1038/nrcardio.2016.179
3. Collaborators GDaIIaP. Global, regional, and national incidence, prevalence, and years lived with disability for 328 diseases and injuries for 195 countries, 1990-2016: a systematic analysis for the Global Burden of Disease Study 2016. Lancet. (2017) 390:1211–59. doi: 10.1016/S0140-6736(17)32154-2
4. Mancini GB, Gosselin G, Chow B, Kostuk W, Stone J, Yvorchuk KJ, et al. Canadian Cardiovascular Society guidelines for the diagnosis and management of stable ischemic heart disease. Can J Cardiol. (2014) 30:837–49. doi: 10.1016/j.cjca.2014.05.013
5. Gerhard-Herman MD, Gornik HL, Barrett C, Barshes NR, Corriere MA, Drachman DE, et al. 2016 AHA/ACC Guideline on the management of patients with lower extremity peripheral artery disease: a report of the American College of Cardiology/American Heart Association Task Force on Clinical Practice Guidelines. J Am Coll Cardiol. (2017) 69:e71–126. doi: 10.1016/j.jacc.2016.11.007
6. Bauersachs R, Zeymer U, Brière JB, Marre C, Bowrin K, Huelsebeck M. Burden of coronary artery disease and peripheral artery disease: a literature review. Cardiovasc Ther. (2019) 2019:8295054. doi: 10.1155/2019/8295054
7. Knuuti J, Wijns W, Saraste A, Capodanno D, Barbato E, Funck-Brentano C, et al. 2019 ESC Guidelines for the diagnosis and management of chronic coronary syndromes. Eur Heart J. (2020) 41:407–77. doi: 10.1093/eurheartj/ehz425
8. Pijls NH, De Bruyne B, Peels K, Van Der Voort PH, Bonnier HJ, Bartunek JKJJ, et al. Measurement of fractional flow reserve to assess the functional severity of coronary-artery stenoses. N Engl J Med. (1996) 334:1703–8. doi: 10.1056/NEJM199606273342604
9. Pereira E, Bettencourt N, Ferreira N, Schuster A, Chiribiri A, Primo J, et al. Incremental value of adenosine stress cardiac magnetic resonance in coronary artery disease detection. Int J Cardiol. (2013) 168:4160–7. doi: 10.1016/j.ijcard.2013.07.114
10. Sand NPR, Veien KT, Nielsen SS, Nørgaard BL, Larsen P, Johansen A, et al. Prospective comparison of FFR derived from coronary CT angiography with SPECT perfusion imaging in stable coronary artery disease: the ReASSESS Study. JACC Cardiovasc Imaging. (2018) 11:1640–50. doi: 10.1016/j.jcmg.2018.05.004
11. Danad I, Raijmakers PG, Driessen RS, Leipsic J, Raju R, Naoum C, et al. Comparison of coronary CT angiography, SPECT, PET, and hybrid imaging for diagnosis of ischemic heart disease determined by fractional flow reserve. JAMA Cardiol. (2017) 2:1100–7. doi: 10.1001/jamacardio.2017.2471
12. Schindler TH, Dilsizian V. Coronary microvascular dysfunction: clinical considerations and noninvasive diagnosis. JACC Cardiovasc Imaging. (2020) 13(1 Pt 1):140–55. doi: 10.1016/j.jcmg.2018.11.036
13. Hussain ST, Paul M, Plein S, McCann GP, Shah AM, Marber MS, et al. Design and rationale of the MR-INFORM study: stress perfusion cardiovascular magnetic resonance imaging to guide the management of patients with stable coronary artery disease. J Cardiovasc Magn Reson. (2012) 14:65. doi: 10.1186/1532-429X-14-S1-O19
14. Morton G, Schuster A, Perera D, Nagel E. Cardiac magnetic resonance imaging to guide complex revascularization in stable coronary artery disease. Eur Heart J. (2010) 31:2209–15. doi: 10.1093/eurheartj/ehq256
15. Nagel E, Klein C, Paetsch I, Hettwer S, Schnackenburg B, Wegscheider K, et al. Magnetic resonance perfusion measurements for the noninvasive detection of coronary artery disease. Circulation. (2003) 108:432–7. doi: 10.1161/01.CIR.0000080915.35024.A9
16. Shaw LJ, Marwick TH, Zoghbi WA, Hundley WG, Kramer CM, Achenbach S, et al. Why all the focus on cardiac imaging? JACC Cardiovasc Imaging. (2010) 3:789–94. doi: 10.1016/j.jcmg.2010.05.004
17. Patel MR, Peterson ED, Dai D, Brennan JM, Redberg RF, Anderson HV, et al. Low diagnostic yield of elective coronary angiography. N Engl J Med. (2010) 362:886–95. doi: 10.1056/NEJMoa0907272
18. Berman DS, Kang X, Slomka PJ, Gerlach J, de Yang L, Hayes SW, et al. Underestimation of extent of ischemia by gated SPECT myocardial perfusion imaging in patients with left main coronary artery disease. J Nucl Cardiol. (2007) 14:521–8. doi: 10.1016/j.nuclcard.2007.05.008
19. Katayama T, Ogata N, Tsuruya Y. Diagnostic accuracy of supine and prone thallium-201 stress myocardial perfusion single-photon emission computed tomography to detect coronary artery disease in inferior wall of left ventricle. Ann Nucl Med. (2008) 22:317–21. doi: 10.1007/s12149-008-0118-3
20. Wu MC, Chin KC, Lin KH, Chiu NT. Diagnostic efficacy of a low-dose 32-projection SPECT 99mTc-sestamibi myocardial perfusion imaging protocol in routine practice. Nucl Med Commun. (2009) 30:140–7. doi: 10.1097/MNM.0b013e328319e65f
21. Kawai Y, Morita K, Nozaki Y, Ohkusa T, Sakurai M, Tamaki N. Diagnostic value of 123I-betamethyl-p-iodophenyl-pentadecanoic acid (BMIPP) single photon emission computed tomography (SPECT) in patients with chest pain. Comparison with rest-stress 99mTc-tetrofosmin SPECT and coronary angiography. Circ J. (2004) 68:547–52. doi: 10.1253/circj.68.547
22. Slart RH, Bax JJ, van Veldhuisen DJ, van der Wall EE, Dierckx RA, Jager PL. Imaging techniques in nuclear cardiology for the assessment of myocardial viability. Int J Cardiovasc Imaging. (2006) 22:63–80. doi: 10.1007/s10554-005-7514-8
23. Koepfli P, Hany TF, Wyss CA, Namdar M, Burger C, Konstantinidis AV, et al. CT attenuation correction for myocardial perfusion quantification using a PET/CT hybrid scanner. J Nucl Med. (2004) 45:537–42. Available online at: https://jnm.snmjournals.org/content/45/4/537
24. Klocke FJ, Baird MG, Lorell BH, Bateman TM, Messer JV, Berman DS, et al. ACC/AHA/ASNC guidelines for the clinical use of cardiac radionuclide imaging–executive summary: a report of the American College of Cardiology/American Heart Association Task Force on Practice Guidelines (ACC/AHA/ASNC Committee to Revise the 1995 Guidelines for the Clinical Use of Cardiac Radionuclide Imaging). Circulation. (2003) 108:1404–18. doi: 10.1161/01.CIR.0000080946.42225.4D
25. Singh V, Di Carli MF. SPECT Versus PET myocardial perfusion imaging in patients with equivocal CT. Curr Cardiol Rep. (2020) 22:43. doi: 10.1007/s11886-020-01287-0
26. Maaniitty T, Knuuti J, Saraste A. 15O-Water PET MPI: current status and future perspectives. Semin Nucl Med. (2020) 50:238–47. doi: 10.1053/j.semnuclmed.2020.02.011
27. Al Badarin FJ, Malhotra S. Diagnosis and prognosis of coronary artery disease with SPECT and PET. Curr Cardiol Rep. (2019) 21:57. doi: 10.1007/s11886-019-1146-4
28. Ullah W, Roomi S, Abdullah HM, Mukhtar M, Ali Z, Ye P, et al. Diagnostic accuracy of cardiac magnetic resonance versus fractional flow reserve: a systematic review and meta-analysis. Cardiol Res. (2020) 11:145–54. doi: 10.14740/cr1028
29. Jaarsma C, Leiner T, Bekkers SC, Crijns HJ, Wildberger JE, Nagel E, et al. Diagnostic performance of noninvasive myocardial perfusion imaging using single-photon emission computed tomography, cardiac magnetic resonance, and positron emission tomography imaging for the detection of obstructive coronary artery disease: a meta-analysis. J Am Coll Cardiol. (2012) 59:1719–28. doi: 10.1016/j.jacc.2011.12.040
30. Yang Z, Zheng H, Zhou T, Yang LF, Hu XF, Peng ZH, et al. Diagnostic performance of myocardial perfusion imaging with SPECT, CT and MR compared to fractional flow reserve as reference standard. Int J Cardiol. (2015) 190:103–5. doi: 10.1016/j.ijcard.2015.04.091
31. van Diemen PA, Driessen RS, Kooistra RA, Stuijfzand WJ, Raijmakers PG, Boellaard R, et al. Comparison between the performance of quantitative flow ratio and perfusion imaging for diagnosing myocardial ischemia. JACC Cardiovasc Imaging. (2020) 13:1976–85. doi: 10.1016/j.jcmg.2020.02.012
32. Strähl M, Schindler M, Keller LS, Holy E, Jakob P, Siegrist PT, et al. Diagnostic performance of angiography-based quantitative flow ratio for the identification of myocardial ischemia as assessed by (13)N-ammonia myocardial perfusion imaging positron emission tomography. Int J Cardiol. (2020) 314:13–9. doi: 10.1016/j.ijcard.2020.04.069
33. Ghekiere O, Dacher JN, Dewilde W, Mancini I, Cools W, Vanhoenacker PK, et al. Value of relative myocardial perfusion at MRI for fractional flow reserve-defined ischemia: a pilot study. AJR Am J Roentgenol. (2019) 212:1002–9. doi: 10.2214/ajr.18.20469
34. Fathala A, Aboulkheir M, Shoukri MM, Alsergani H. Diagnostic accuracy of (13)N-ammonia myocardial perfusion imaging with PET-CT in the detection of coronary artery disease. Cardiovasc Diagn Ther. (2019) 9:35–42. doi: 10.21037/cdt.2018.10.12
35. Moher D, Liberati A, Tetzlaff J, Altman DG. Preferred reporting items for systematic reviews and meta-analyses: the PRISMA statement. Int J Surgery. (2010) 8:336–41. doi: 10.1016/j.ijsu.2010.02.007
36. Reitsma JB, Moons KG, Bossuyt PM, Linnet K. Systematic reviews of studies quantifying the accuracy of diagnostic tests and markers. Clin Chem. (2012) 58:1534–45. doi: 10.1373/clinchem.2012.182568
37. Cumpston M, Li T, Page MJ, Chandler J, Welch VA, Higgins JP, et al. Updated guidance for trusted systematic reviews: a new edition of the Cochrane Handbook for Systematic Reviews of Interventions. Cochrane Database Syst Rev. (2019) 10:Ed000142. doi: 10.1002/14651858.ED000142
38. Benjamin EJ, Virani SS, Callaway CW, Chamberlain AM, Chang AR, Cheng S, et al. Heart disease and stroke statistics-2018 update: a report from the American Heart Association. Circulation. (2018) 137:e67–492. doi: 10.1161/CIR.0000000000000573
39. Collet C, Grundeken MJ, Asano T, Onuma Y, Wijns W, Serruys PW. State of the art: coronary angiography. EuroIntervention. (2017) 13:634–43. doi: 10.4244/EIJ-D-17-00465
40. Chung KS, Nguyen PK. Non-invasive measures of coronary microcirculation: taking the long road to the clinic. J Nucl Cardiol. (2018) 25:2112–5. doi: 10.1007/s12350-017-0972-2
41. Piekarski E, Manrique A, Rouzet F, Le Guludec D. Current status of myocardial perfusion imaging with new SPECT/CT cameras. Semin Nucl Med. (2020) 50:219–26. doi: 10.1053/j.semnuclmed.2020.02.009
42. Yang K, Yu SQ, Lu MJ, Zhao SH. Comparison of diagnostic accuracy of stress myocardial perfusion imaging for detecting hemodynamically significant coronary artery disease between cardiac magnetic resonance and nuclear medical imaging: a meta-analysis. Int J Cardiol. (2019) 293:278–85. doi: 10.1016/j.ijcard.2019.06.054
43. Tweet MS, Arruda-Olson AM, Anavekar NS, Pellikka PA. Stress echocardiography: what is new and how does it compare with myocardial perfusion imaging and other modalities? Curr Cardiol Rep. (2015) 17:43. doi: 10.1007/s11886-015-0600-1
44. Camen S, Haeusler KG, Schnabel RB. Cardiac imaging after ischemic stroke: echocardiography, CT, or MRI? Herz. (2019) 44:296–303. doi: 10.1007/s00059-019-4803-x
45. Ammon F, Bittner D, Hell M, Mansour H, Achenbach S, Arnold M, et al. CT-derived left ventricular global strain: a head-to-head comparison with speckle tracking echocardiography. Int J Cardiovasc Imaging. (2019) 35:1701–7. doi: 10.1007/s10554-019-01596-8
46. Maron DJ, Hochman JS, Reynolds HR, Bangalore S, O'Brien SM, Boden WE, et al. Initial invasive or conservative strategy for stable coronary disease. N Engl J Med. (2020) 382:1395–407. doi: 10.1056/NEJMoa1915922
Keywords: myocardial perfusion imaging, coronary artery disease, diagnostic performance, meta-analysis, non-invasive modality
Citation: Xu J, Cai F, Geng C, Wang Z and Tang X (2021) Diagnostic Performance of CMR, SPECT, and PET Imaging for the Identification of Coronary Artery Disease: A Meta-Analysis. Front. Cardiovasc. Med. 8:621389. doi: 10.3389/fcvm.2021.621389
Received: 26 October 2020; Accepted: 23 February 2021;
Published: 07 May 2021.
Edited by:
Constantinos Anagnostopoulos, Biomedical Research Foundation of the Academy of Athens (BRFAA), GreeceReviewed by:
Alessia Gimelli, Gabriele Monasterio Tuscany Foundation (CNR), ItalyGeorgios Benetos, National and Kapodistrian University of Athens, Greece
Copyright © 2021 Xu, Cai, Geng, Wang and Tang. This is an open-access article distributed under the terms of the Creative Commons Attribution License (CC BY). The use, distribution or reproduction in other forums is permitted, provided the original author(s) and the copyright owner(s) are credited and that the original publication in this journal is cited, in accordance with accepted academic practice. No use, distribution or reproduction is permitted which does not comply with these terms.
*Correspondence: Xiaobin Tang, tangxiaobin@nuaa.edu.cn