- 1Department of Cardiology, Beijing Jishuitan Hospital, Peking University Fourth Hospital, Beijing, China
- 2Department of Cardiology, Beijing Anzhen Hospital, Capital Medical University, Beijing, China
- 3Beijing Key Laboratory of Precision Medicine of Coronary Atherosclerotic Disease, Clinical Center for Coronary Heart Disease, Beijing Institute of Heart Lung and Blood Vessel Disease, Capital Medical University, Beijing, China
Coronavirus disease 2019 (COVID-19), caused by severe acute respiratory syndrome coronavirus 2, is a global pandemic. It has resulted in considerable morbidity and mortality around the world. The respiratory system is the main system invaded by the virus involved in COVID-19. In addition to typical respiratory manifestations, a certain proportion of severe COVID-19 cases present with evidence of myocardial injury, which is associated with excessive mortality. With availability of an increasing amount of imaging data, right ventricular (RV) damage is prevalent in patients with COVID-19 and myocardial injury, while left ventricular damage is relatively rare and lacks specificity. The mechanisms of RV damage may be due to increased RV afterload and decreased RV contractility caused by various factors, such as acute respiratory distress syndrome, pulmonary thrombosis, direct viral injury, hypoxia, inflammatory response and autoimmune injury. RV dysfunction usually indicates a poor clinical outcome in patients with COVID-19. Timely and effective treatment is of vital importance to save patients' lives as well as improve prognosis. By use of echocardiography or cardiovascular magnetic resonance, doctors can find RV dilatation and dysfunction early. By illustrating the phenomenon of RV damage and its potential pathophysiological mechanisms, we will guide doctors to give timely medical treatments (e.g., anticoagulants, diuretics, cardiotonic), and device-assisted therapy (e.g., mechanical ventilation, extracorporeal membrane oxygenation) when necessary for these patients. In the paper, we examined the latest relevant studies to investigate the imaging features, potential mechanisms, and treatments of myocardial damage caused by COVID-19. RV damage may be an association between myocardial damage and lung injury in COVID-19. Early assessment of RV geometry and function will be helpful in aetiological determination and adjustment of treatment options.
Introduction
Coronavirus disease 2019 (COVID-19) has spread rapidly and triggered a terrible global pandemic that involves more than 200 countries/regions. On 6 December 2020, there were more than 66.9 million confirmed cases and 1,534,954 deaths internationally (1). Although respiratory symptoms are usually predominant in COVID-19, elevated troponin levels have been found at the early stage in some cases, indicating that COVID-19 also affects the heart. In particular, there is an increased prevalence of cardiovascular complications, including new or worsening heart failure, arrhythmia, acute myocarditis, and myocardial infarction, in severe and critically ill patients with COVID-19. Recent studies have shown that the incidence of acute myocardial injury in hospitalized patients with COVID-19 is ~20–28% (2–4). With an increase in imaging evidence, such as echocardiography and magnetic resonance imaging (MRI), right ventricular (RV) involvement has been observed more commonly than left ventricular (LV) involvement in patients with COVID-19, with ~40% of patients experiencing RV dilatation and RV dysfunction (5, 6). RV damage is associated with a higher incidence of myocardial damage in COVID-19 and generally predicts a worse prognosis (7). This review aims to describe involvement of RV damage in patients with COVID-19, to determine the association of RV damage with COVID-19 and its plausible mechanisms, and to summarize the existing appropriate treatment strategies to improve patients' prognosis.
Myocardial Injury in Covid-19 is Common
Previous influenza-related studies have shown that elevated cardiac enzymes are relatively uncommon (8). Cardiac abnormalities associated with influenza are usually subclinical and/or transient (9). However, COVID-9-related cardiac injury is significantly different from influenza. In a review of 26 studies that included 11,685 patients, the overall prevalence of COVID-19-related acute myocardial injury ranged from 5 to 38% (10). N-terminal pro-brain natriuretic peptide and cardiac troponin-I levels were shown to be significantly higher in critically ill patients with COVID-19 than in non-critically ill patients (2). These findings suggest that the magnitude of elevated cardiac troponin levels may be related to the severity and prognosis of the disease (11). Monitoring cardiac troponin-I levels is important for judging the status of COVID-19, while understanding myocardial injury in patients with COVID-19. Chinese guidelines recommend myocardial enzyme monitoring in patients who are admitted for COVID-19 (12). Troponins are often associated with LV ischaemia and infarction. However, previous studies have shown that the most common mechanism of elevated troponin levels in patients with COVID-19 is acute RV damage rather than LV functional impairment (5). Specific manifestations of myocardial structural damage require assessment of cardiac imaging. Early retrospective analysis did not show any specificity between electrocardiography and echocardiography (13). However, with publication of more imaging study results, there are particularities in cardiac structural changes. Therefore, imaging assessment of cardiac injury in COVID-19 is important and helpful for differential diagnosis of cardiac events.
RV Involvement From Cardiac Images in Patients With Covid-19
With the discovery of COVID-19-related myocardial damage, cardiac imaging is becoming more common, and it can help to better understand the structural characteristics of COVID-19-related myocardial damage. Imaging studies can not only detect lesions, but also guide further treatment. We searched PubMed, EMBASE, and Web of Science until August 2020 for RV clinical research. “Snowball sampling” by searching reference lists and citation tracking was performed in each retrieved article. No language restrictions were applied. Following search terms were used: (“magnetic resonance imaging” OR “echocardiography” OR “myocardial injury” OR “cardiac manifestations” OR “cardiac function” OR “right ventricular damage/injury” OR “right ventricular dysfunction” OR “right ventricular dilatation”) AND (“coronavirus” OR “SARS-COV-2” OR “COVID-19”). Recent findings on imaging assessment of cardiac injury in COVID-19 were summarized in Tables 1, 2.
MRI Findings
MRI can be used to quantitatively assess myocardial fibrosis and oedema (28, 29). This technique is currently the gold standard for evaluating cardiac morphology and function (30). MRI analysis includes conventional sequences and quantitative mapping sequences. Conventional sequences include short-axis and long-axis cine, T2-weighted imaging (T2WI), and late gadolinium-enhanced scanning (LGE). Quantitative mapping sequences include native T1/T2 mapping and post-contrast T1 mapping. T1 mapping is mainly applied to quantitatively assess diffuse fibrosis, while T2 mapping enables the quantification of edema. Post-contrast T1 mapping can better obtain extracellular volume fraction, which can be used as the most sensitive biomarker of myocardial fibrosis and is highly consistent with histopathological findings (31). Myocardial oedema is assessed on T2WI images, and LV and RV functional parameters are calculated by changes in endocardial and epicardial contours (14). A study of competitive athletes recovered from COVID-19 found that cardiac MRI (CMR) was more sensitive to identify myocarditis, helping to identify the high-risk population. CMR has a negative predictive value for exclusion of myocarditis (16). Two other studies, analyzing of patients who had already recovered from COVID-19 when undergoing MRI, showed increased T1 and T2 signals, positive LGE and/or pericardial enhancement in 58–78% of the population (14, 15). In Huang's study, 26 patients without previous cardiac diseases were all recovered and isolated for 14 days, and myocardial edema was found in 54% of patients (14). In Puntmann's study, mostly non-hospitalized patients recovered from COVID-19, 60% of them found myocardial inflammation (16). While COVID-19 patients had cardiac injury, regardless of preexisting disease, severity and overall course of COVID-19 manifestations, time since initial diagnosis, or presence of cardiac symptoms (16). Decreased RV functional parameters, including the RV ejection fraction, cardiac output, the cardiac index, and stroke volume, were found in patients with positive cardiac MRI findings compared with healthy controls (P < 0.05). These findings suggest that sustained cardiac involvement, including oedema, fibrosis, and impaired RV contractile function, may remain in patients who recover from COVID-19. Similarly, Puntmann et al. showed that the RV ejection fraction was decreased in patients with COVID-19 compared with healthy controls (15). They also found a reduction in the LV ejection fraction in the recovered COVID-19 cohort. However, Huang et al. showed that LV function was hyperdynamic or normal in the same subgroup (14). The outcomes were inconsistent between these two studies. Regardless of the discrepancy, Puntmann et al. considered that native T1 and T2 were the best indicators with the ability to detect COVID-19-related myocardial pathology (15). Further investigation on the long-term cardiovascular consequences of COVID-19 is required (16).
Echocardiographic Findings
Echocardiography is commonly used for assessing cardiac damage. This technique is easier to perform than cardiac MRI. Conventional echocardiographic evaluation includes cardiac structural assessment, myocardial systolic and diastolic function, and valvular hemodynamics. According to the American Society of Echocardiography, RV dysfunction is present when the following parameters used to quantify RV function are less than low values in the normal range: pulsed Doppler systolic myocardial velocity <9.5 cm/s, tricuspid annular plane systolic excursion <17 mm, RV ejection fraction <45%, and RV fractional area change <35% (32, 33). RV dilatation is usually observed early in the pressure-overloaded right ventricle. Typically, in the RV-focused view, a basal diameter > 41 mm and an intermediate horizontal diameter > 35 mm indicate RV dilatation (32).
Most inpatients with COVID-19 have RV dilatation or dysfunction. However, LV dysfunction is less common. In a study of 74 patients with COVID-19, 27% presented with RV dysfunction, but LV function was hyperdynamic or normal in 89% (6). Szekely et al. (5) showed that RV dysfunction was more common in patients with elevated troponin levels and a poor clinical grade, whereas the total number of patients with an impaired LV function was relatively smaller. Notably, in several other studies, LV dysfunction was not rare in patients with COVID-19 (18, 23, 25). This discrepancy among studies may be due to differences in the study populations, but RV damage is still universally found by echocardiography in patients with COVID-19. We summarized the results of recent cardiac imaging studies (Table 2). Among patients with COVID-19-related myocardial injury, the proportion of RV dilatation ranged from 13.3 to 48.9% (5, 6, 17, 20, 23). RV dilatation associated with elevated D-dimer levels and C-reactive protein levels was more common in patients with COVID-19 (6, 17, 18, 20). There was no significant difference in the incidence of major comorbidities (hypertension, diabetes and known coronary artery disease), laboratory markers of inflammation (white blood cell count, C-reactive protein) or myocardial injury (troponin) in patients with right ventricular dilatation (17).
Conventional echocardiographic parameters are not sensitive to early RV systolic dysfunction, and therefore, cannot be used for early diagnosis (34). Two-dimensional speckle tracking echocardiography can more accurately evaluate myocardial function and detect subclinical cardiac functional impairment earlier than conventional echocardiography (35, 36), which can measure LV global longitudinal strain (LVGLS), RV longitudinal strain (RVLS), RV free wall strain (RVFWS), and RV global strain (RVGS). In a retrospective study, RVLS was found to predict mortality in patients with COVID-19 more accurately. Therefore, there is potential value of RVLS for risk stratification in COVID-19. The optimal cut-off values for prediction of outcome were calculated to be −23% for RVLS, 43.5% for RV fractional area change, and 23 mm for tricuspid annular systolic displacement (7). Baycan et al. (24) and Krishnamoorthy et al. (25) also evaluated the prognostic value of strain indices. RVGS and RVFWS were significantly reduced in patients with poor clinical outcomes. RVLS is an independent predictor of in-hospital mortality in patients with COVID, while the predictive value of LVGLS for mortality varies in different studies. However, speckle-tracking echocardiography is demanding on image quality. The structure of the chest wall in different patients has a large effect on imaging, and critically ill patients are unable to cooperate in adjusting positions, both of which affect the results.
RV Dysfunction and Prognosis in COVID-19
Cardiac imaging findings have shown that RV damage is common in patients with COVID-19. Concomitant RV damage usually indicates a poor prognosis and affects the clinical outcome of patients. In a study of 120 COVID-19 cases, non-survivors showed elevated pulmonary artery systolic pressure, dilated right heart chambers, and diminished RV function compared with survivors (7). In another study where 28 patients died of COVID-19, 14 had a RV abnormality, but only 2 had LV impairment (6). Indeed, these outcomes all indicate a strong relation between RV dysfunction and poor prognosis. One multivariate analysis revealed that RV enlargement was the only factor significantly associated with mortality (17). Patients with COVID-19 and RV dysfunction often have more severe symptoms (19). Argulian et al. found that renal dysfunction was more common in patients with RV dilatation than those without (17). Therefore, RV dysfunction often predicts the presence of some severe complications, and they may partly account for the high mortality in this population. Additionally, Pagnesi et al. (21) showed that pulmonary hypertension, instead of RV dysfunction, was associated with worse in-hospital clinical outcomes in patients with COVID-19. However, because their study population was non-intensive care unit patients without mechanical ventilation, this may have eliminated the association between COVID-19 and RV involvement.
Although CMR imaging is the gold standard for assessing RV function (30), the high infectivity of COVID-19 and the inability of patients to hold their breath for a long time limit its application. Patients without pre-existing cardiovascular diseases are more likely to have normal echocardiography than those with pre-existing cardiovascular diseases (21). RV dysfunction is more common than LV dysfunction in COVID-19 (23). Patients with RV dysfunction had a higher rate of cardiac comorbidities compared with patients without RV dysfunction (37). The main reasons for performing echocardiography in the previous study were suspected heart failure and elevated cardiac biomarker concentrations (5, 21, 23). Independent predictors of RV abnormalities are suspected RV failure and moderate or severe COVID-19 symptoms (21). To minimize the risk of the spread of infection, at least echocardiography should be performed in patients with suspected heart failure, more cardiac comorbidities, elevated cardiac biomarkers, and severe COVID-19 symptoms. Abnormal transthoracic echocardiography ultimately affects decision-making of clinicians in 16–33.3% of patients (18, 19). It also showed that clinical management was altered in 24.2% of patients because of acute cardiovascular events observed with transthoracic echocardiography (18).
Male was an independent predictor of prognosis (7), while age, weight, and ethnicity were not significantly different in COVID-19 patients with cardiac injury. Patients with a history of established cardiovascular disease or elevated cardiac biomarkers have an increased susceptibility to infection and an increased risk of severe disease progression and death (4, 37, 38). These patients are more likely to have RV dysfunction and pulmonary hypertension, which are independent risk factors for poor prognosis (21, 22). The proportions of echocardiographic abnormalities and serious heart disease are similar after excluding patients with pre-existing heart disease (heart failure, valvular disease, or ischemic heart disease), suggesting that cardiac abnormalities are associated with COVID-19 infection in this population (19).
Aetiology of COVID-19 With RV Functional Changes May Involve Multiple Facets
The Right Ventricle Is More Susceptible to Lung Injury Than the Left Ventricle
The transverse section of the right ventricle is crescent-shaped compared with the thick wall of the left ventricle, and the relative surface area of the right ventricle is higher and the volume is lower. The thin RV free wall has greater compliance than the left ventricle. These anatomical features allow acute dilatation of the right ventricle when there is a sharp increase in afterload. RV systolic function is sensitive to increased pressure, and a slight rise in pulmonary circulation resistance causes RV overload and impaired systolic function. The primary target organ of severe acute respiratory syndrome coronavirus-2 is the lungs. The right ventricle is vulnerable to a slight increase in pulmonary vascular resistance (39), making it more vulnerable to injury than the left ventricle. As the right ventricle continues to expand, RV geometry changes, and the tricuspid annulus dilates insufficiently, resulting in tricuspid regurgitation. Tricuspid regurgitation leads to further RV dilatation and volume overload, which shifts the interventricular septum to the left and affects LV filling and contraction. RV pressure overload increases wall tension, increases myocardial oxygen consumption, and decreases RV oxygen supply during systole. This further leads to myocardial ischaemia and reduces RV contractility. RV dilatation may precede development of acute cor pulmonale (40).
Acute Respiratory Distress Syndrome and RV Dysfunction
COVID-19 mainly affects the respiratory system and the incidence of acute respiratory distress syndrome (ARDS) reported in COVID-19 ranges from 19.6 to 31% (37, 38, 41). ARDS is a severe form of COVID-19, which leads to a dramatic increase in RV afterload and delayed contraction owing to its own pathological effects and mechanical ventilation with a high positive end-expiratory pressure (PEEP). This then reverses the end-systolic transseptal pressure gradient. The incidence of RV dysfunction in ARDS has been reported to be 22–50% (33). There is no robust evidence to verify a definitive causal relationship between RV dysfunction and mortality in ARDS. However, RV dysfunction is undoubtedly associated with increased mortality and poorer prognosis in patients with COVID-19-related ARDS (42). In the setting of ARDS, numerous factors can destroy the pulmonary circulation, including mechanical compression by interstitial oedema, microvascular thrombosis, hypoxic or mediator-induced pulmonary vasoconstriction, and pulmonary vascular muscular remodeling. These factors raise pulmonary arterial pressure and further rapidly increase RV afterload. Pulmonary vascular resistance abates RV ejection and LV pulmonary venous return, while RV dilatation results in LV compression by a septal shift because of an inextensible pericardium. Both of these mechanisms account for the decrease in LV ejection and RV coronary blood flow. Therefore, ARDS-derived pulmonary circulation injury in COVID-19 has a deleterious effect on RV dysfunction (43, 44).
RV dilatation secondary to mechanical ventilation during hospitalization for ARDS requires attention. In the ARDS population, a lung protective ventilation strategy is recommended and mainly refers to PEEP. High PEEP levels cause overinflation of the normal alveoli and compression of intra-alveolar vessels, which lead to high pulmonary vascular resistance and increased RV afterload (43). Therefore, RV dysfunction can be a haemodynamically significant and deleterious consequence of COVID-19-related mechanical ventilation. Notably, Sud et al. showed that there was no meaningful correlation between PEEP and RV dilation on echocardiography in their COVID-19 infection cohort (17). However, they did not deny the possible contribution on RV dilatation from mechanical ventilation.
Pulmonary Embolism and RV Dysfunction
Owing to risk factors, such as virus-induced endothelial injury, vascular inflammation, and hospitalization-related prolonged immobilization, most patients with COVID-19 stay in a hypercoagulable state, and they are vulnerable to venous thrombosis. Poissy et al. studied 107 patients with COVID-19 who were admitted to the intensive care unit (45). They reported a high incidence of pulmonary embolism (20.4%), which was significantly higher than the contemporaneous average level in patients with influenza and in in-hospital patients. An autopsy of patients with COVID-19 showed a high incidence of deep venous thrombosis (58%) and death-causing pulmonary embolism (33%) (46). When thrombus enters pulmonary vessels, it produces mechanical obstruction and stimulates endothelial cells and platelets to release vasoactive mediators (e.g., thromboxane A2, serotonin). This triggers obstruction-related vasoconstriction and increases RV afterload and pulmonary arterial pressure in patients. Oxygen demand from the right ventricle increases, while embolism-associated hypoxemia and hypotension decrease myocardial oxygen supply. This imbalance finally leads to RV dysfunction (47).
Myocardial Injury and a Cytokine Storm
Myocardial injury was recognized early in patients with COVID-19 in China, and it also partly accounts for RV dysfunction. Myocarditis can occur before pulmonary symptoms of shock (48). The possible mechanisms for myocardial injury are as follows. Angiotensin-converting enzyme 2 (ACE2) is highly expressed not only in the lungs, but also in the cardiovascular system, thus possibly mediating viral entry into cardiomyocytes to cause direct damage. Cardiac elevation of troponin-I levels is accompanied by an increase in other inflammatory markers, such as lactate dehydrogenase, ferritin, tumor necrosis factor-α (TNF-α), interleukin-6 (IL-6) and interleukin-8 (IL-8). This could represent a cytokine storm syndrome or secondary haemophagocytic lymphohistiocytosis, which may result in cardiac involvement (49). After viral invasion into the body, T cells become activated, and they produce and release amounts of antiviral cytokines. Because of an imbalanced response among subtypes of T helper cells, a cytokine storm release is induced, which attributes to hyperactivation of monocytes/macrophages. This then leads to tissue damage to multiple organs and causes complications, such as ARDS and cardiac insufficiency.
In ARDS, increased levels of cytokines, such as IL-6, IL-8, TNF-α, can be tested. In particular, IL-6 is an important marker. A previous study reported that elevated circulating IL-6 levels were associated with increased mortality in COVID-19 (50). Targeted therapy against the IL-6 receptor with tocilizumab can be effective in severe COVID-19 cases. A cytokine storm is essentially a protective response to limit spread of the virus, but its exact mechanism of myocardial injury remains unclear. However, cardiomyocyte and endothelial cell death triggered by inflammatory cytokines, such as TNF-α, has been well-documented (51). Ventricular dilatation with a reduction in the ejection fraction may be an adaptive response to myocardial dysfunction. Myocardial depression results from the direct or indirect action of one or more cardioinhibitory substances. Besides, TNF-α and IL-1, which act as potent inducible nitric oxide synthase inducers, are associated with inhibition of cardiomyocyte function. For one thing, nitric oxide interferes with calcium metabolism in cardiomyocytes, which in turn impairs contractile function. For another, peroxynitrite generated by interaction of nitric oxide with superoxide ions is directly toxic to cardiomyocytes (52). Additionally, Hypoxemia caused by COVID-19 can also induce intracellular calcium overload, leading to apoptosis of cardiomyocytes (53). So, an inflammatory storm, as well as autoimmune activation, can induce extensive vascular and myocardial inflammation, while predisposing to diffuse thrombosis (54).
In summary, the mechanism of myocardial injury varies at different stages of COVID-19. Isolated RV dysfunction can be found in the presence of severe ARDS or pulmonary embolism (55), while diffuse myocardial damage caused by viral toxicity and the host immune response also partly weaken RV function (Figure 1). Because of ACE2 expression in the endothelium, virus-induced endothelial shedding and microvascular damage may lead to thrombosis and myocardial infarction (55). ACE2-mediated direct injury may be a major mechanism in the early stages of COVID-19. With aggravation of COVID-19, pulmonary and cardiac injury caused by hypoxia is gradually aggravated. Inflammatory reactions and autoimmune damage leading to exacerbation of disease play a major role in the later stages of COVID-19.
Treatment of RV Dysfunction With COVID-19
Medical Treatment
Medical treatment of RV functional impairment includes reducing volume load, enhancing RV contractility, and reducing pulmonary arterial pressure. Diuretics can reduce intravascular volume. The RV Starling curve is flat, and improvement in RV function can only be observed with a large negative fluid balance. Normally, the RV filling pressure needs to be maintained at a slightly increased level at ~8–12 mmHg. The volume status can be further adjusted on this basis to achieve optimal RV function and cardiac output (56). RV pressure monitoring is also important when circulating hypovolemia results in decreased blood pressure and the requirement for appropriate fluid replacement. Central venous pressure and mixed venous oxygen saturation help determine RV filling and oxygen supply. Echocardiography also helps determine the volume status. RV dilatation with restriction of LV filling indicate excessive preload.
Levosimendan is a novel calcium sensitizer that stabilizes the spatial configuration of myocardial fibrin and increases myocardial contractility. This calcium sensitizer has the advantages of no effect on diastolic function or arrhythmia, and does not increase myocardial oxygen consumption. Levosimendan improves RV myocardial contractility and reduces RV afterload. Morelli et al. showed that levosimendan was an effective treatment option for ARDS with acute right heart dilatation, and it was believed to dilate the pulmonary circulation and improve RV contractility (57). Norepinephrine might improve RV function by restoring RV perfusion pressure as suggested in an experimental model of massive pulmonary embolism (58). Intravenous epoprostenol can improve symptoms, hemodynamics, and the survival rate, and enhance RV systolic function (59). Bosentan is a specific endothelin receptor antagonist, which reduces mean pulmonary arterial pressure and increases the cardiac index. Inhaled nitric oxide can selectively dilate pulmonary vessels, improve the ventilation-blood flow ratio, significantly reduce pulmonary vascular resistance, and increase cardiac output, while it has a slight effect on systemic vascular resistance. In patients with pulmonary heart disease caused by ARDS, inhaled nitric oxide reduces pulmonary arterial pressure and pulmonary inflammatory responses (60). In patients with pulmonary embolism and ARDS, prostacyclin is as effective as inhaled nitric oxide in reducing pulmonary arterial pressure, improving gas exchange and oxygenation, increasing cardiac output, and improving RV function (61, 62).
To alleviate inflammation and fibrosis, corticosteroids are considered as potential therapeutic agents for ARDS, which reduce morbidity and mortality, but remain controversial. High-dose corticosteroid therapy can accelerate improvement of ARDS, reduce mortality, and shorten the duration of invasive mechanical ventilation (63). However, the World Health Organization recommends that systemic corticosteroids should not be routinely used in patients with COVID-19 or COVID-19-associated ARDS (64).
Severe COVID-19 is often associated with thrombosis, and disseminated intravascular coagulation may be present in the majority of fatal cases (65). Prolonged immobilization and hormonal therapy increases the risk of venous thromboembolism. Patients with right heart enlargement are also prone to cardiac thrombosis. Coagulopathy due to COVID-19 may be associated with bacterially-induced infectious coagulopathy. Overproduction of inflammatory cytokines, vascular endothelial injury, and increased levels of damage-associated molecular patterns contribute to thrombosis. Patients who meet the sepsis-induced coagulopathy score criteria or have significantly elevated D-dimer levels may benefit from anticoagulant therapy by mainly using low-molecular-weight heparin (66). Among 449 patients with severe COVID-19, 99 received heparin (mainly low-molecular-weight heparin) for 7 days or longer, and 28-day mortality was significantly lower in patients with sepsis-induced coagulopathy scores ≥ 4 or D-dimer levels > six times the upper limit of normal using heparin than in non-users (P = 0.029, P = 0.017). Lin et al. (67) also recommended the use of low-molecular-weight heparin in patients with D-dimer values > four times the upper limit of normal. Thrombotic coagulopathy is common in severe patients with COVID-19, and D-dimer is more useful than other coagulation markers for prediction of this disease. However, bleeding complications are relatively uncommon in COVID-19. Therefore, anticoagulant therapy is necessary.
Device-Assisted Therapy
Mechanical ventilation, sedation, and analgesia may lead to increased afterload, increased transpulmonary pressure, and decreased cardiac output. Therefore, mechanical ventilation indications need to be strictly followed. For critically ill patients requiring mechanical ventilation, appropriate mechanical ventilation measures should be implemented to avoid hypoxemia, hypercapnia, a low or high lung volume, and high PEEP. Protective ventilation strategies should also be used when necessary. The principle of mechanical ventilation in patients with right heart failure is to limit plateau pressure and offer PEEP, avoiding hypercapnia, hypoxemia, and pulmonary vasoconstriction. Respiratory settings are adjusted according to the tolerance of the right ventricle, as assessed by ultrasound, to coordinate the balance between recruitment and hyperventilation resulting from ventilation according to RV function (68). PEEP can dilate the alveoli, compress extra-alveolar capillaries, and cause an increase in pulmonary vascular resistance. This increases afterload and RV volume, resulting in RV dilatation, which in turn affects LV filling. Appropriate PEEP is important for treatment.
When optimized ventilation measures still do not improve hypoxemia in mechanically ventilated patients, extracorporeal membrane oxygenation (ECMO) can be considered. ECMO is used as a rescue therapy for COVID-19 with refractory hypoxemia in accordance with provisional guidelines established by the World Health Organization (69) in 2020. However, because of a lack of relevant trials on the use of ECMO in patients with COVID-19, there is insufficient evidence that these patients can benefit from ECMO. For acute RV failure caused by severe ARDS, extracorporeal carbon dioxide removal devices can be considered for super-protective lung ventilation (tidal volume: 4 mL/kg) (70). Additionally, increased work of breathing, pulmonary oedema, and endogenous PEEP caused by weaning increase RV afterload. Worsening of RV function is an important cause of weaning failure in mechanically ventilated patients. When RV function is impaired in combination with severely impaired LV function, adjunctive therapy with Impella device or intra-aortic balloon counterpulsation can be used. Continuous renal replacement therapy may be considered when volume overload and drug therapy are not effective. COVID-19-related myocardial injury treatments are summarized in Figure 2.
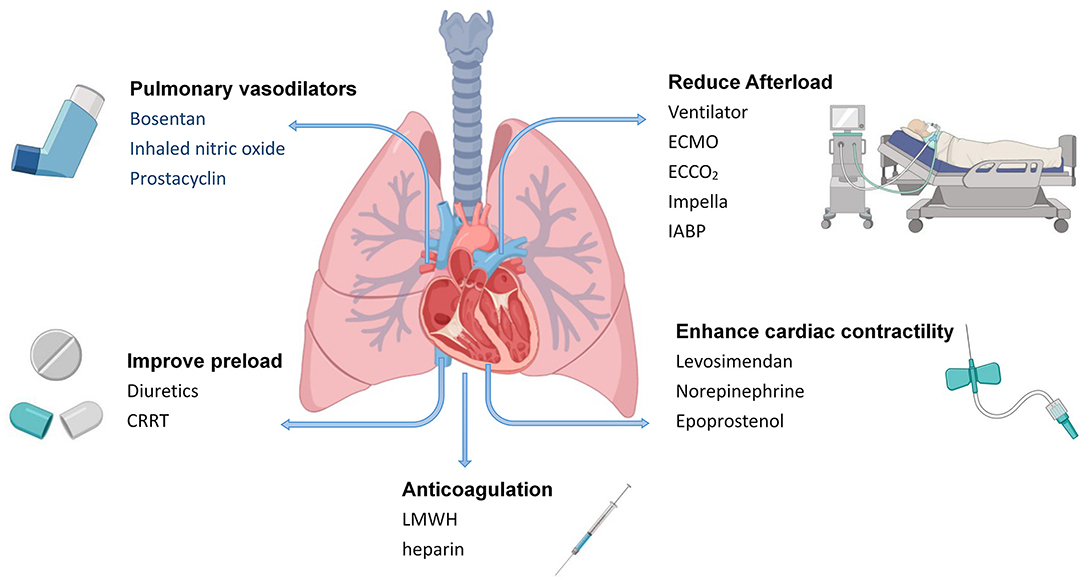
Figure 2. Treatment of RV dysfunction with COVID-19. ECCO2, extracorporeal carbon dioxide removal devices; IABP, intra-aortic balloon pump; LMWH, low molecular weight heparin.
Future Directions and Conclusions
RV dysfunction usually indicates a poor prognosis in the wide array of cardiopulmonary diseases. Assessment of RV function is essential for managing ARDS, acute pulmonary embolism, and pulmonary hypertension. RV dilatation is common in patients with COVID-19. A full understanding of COVID-19-related RV dysfunction is conducive for early identification and precise treatment, and to help improve the prognosis of severe cases and reduce mortality. Early recognition of RV dysfunction allows appropriate treatment to be provided as soon as possible. How to identify RV dysfunction early is important for stratification of disease risk and prognostic evaluation. Echocardiography, cardiac MRI, right heart catheterization, and other examinations are helpful for early identification of RV dysfunction. At the same time, monitoring of biological indicators related to RV function, such as troponins and brain natriuretic peptide, should not be ignored for the suggestive role in RV function. It is recommended to assess RV function as soon as possible, for COVID-19 patients with suspected cardiac injury, elevated cardiac biomarkers, severe respiratory symptoms. RV function is often monitored to optimize haemodynamic and respiratory parameter settings. Timely medical treatment should be delivered. And device assistance should be implemented if necessary. RV damage reflects an association between myocardial injury and COVID-19. In future medical care, clinicians need to further focus on the morbidity of RV dysfunction in patients with COVID-19. Using cardiac imaging to detect RV dysfunction will provide early information concerning the severity of COVID-19 infection. Performing an appropriate strategy of the right ventricle will be helpful to reduce mortality and improve prognosis in this persistent epidemic.
Author Contributions
WL contributed conception and constructing the overall structure and contents. YL wrote the draft and sections of the manuscript. All authors contributed to manuscript revision, read, and approved the submitted version.
Conflict of Interest
The authors declare that the research was conducted in the absence of any commercial or financial relationships that could be construed as a potential conflict of interest.
Acknowledgments
We thank Ellen Knapp, PhD, from Liwen Bianji, Edanz Group China (www.liwenbianji.cn/ac), for editing the English text of a draft of this manuscript.
Abbreviations
ARDS, acute respiratory distress syndrome; COVID-19, coronavirus disease 2019; ECMO, extracorporeal membrane oxygenation; LV, left ventricular; LVEF, left ventricular ejection fraction; LGE, late gadolinium enhancement; MRI, magnetic resonance imaging; PEEP, positive end-expiratory pressure; RV, right ventricular; RVLS, right ventricular longitudinal strain.
References
1. Medicine JHUa. COVID-19 Dashboard by the Center for Systems Science and Engineering (CSSE) at Johns Hopkins. (2020). Available online at: https://coronavirus.jhu.edu/map.html (accessed December 6, 2020).
2. Chen C, Chen C, Yan JT, Zhou N, Zhao JP, Wang DW. Analysis of myocardial injury in patients with COVID-19 and association between concomitant cardiovascular diseases and severity of COVID-19. Zhonghua Xin Xue Guan Bing Za Zhi. (2020) 48:567–71. doi: 10.3760/cma.j.cn112148-20200225-00123
3. Guo T, Fan Y, Chen M, Wu X, Zhang L, He T, et al. Cardiovascular implications of fatal outcomes of patients with coronavirus disease 2019 (COVID-19). JAMA Cardiol. (2020) 5:811–8. doi: 10.1001/jamacardio.2020.1017
4. Shi S, Qin M, Shen B, Cai Y, Liu T, Yang F, et al. Association of cardiac injury with mortality in hospitalized patients with COVID-19 in Wuhan, China. JAMA Cardiol. (2020) 5:802–10. doi: 10.1001/jamacardio.2020.0950
5. Szekely Y, Lichter Y, Taieb P, Banai A, Hochstadt A, Merdler I, et al. Spectrum of cardiac manifestations in COVID-19: a systematic echocardiographic study. Circulation. (2020) 142:342–53. doi: 10.1161/CIRCULATIONAHA.120.047971
6. Mahmoud-Elsayed HM, Moody WE, Bradlow WM, Khan-Kheil AM, Senior J, Hudsmith LE, et al. Echocardiographic findings in patients with COVID-19 pneumonia. Can J Cardiol. (2020) 36:1203–7. doi: 10.1016/j.cjca.2020.05.030
7. Li Y, Li H, Zhu S, Xie Y, Wang B, He L, et al. Prognostic value of right ventricular longitudinal strain in patients with COVID-19. JACC: Cardiovasc Imaging. (2020) 13:2287–99. doi: 10.1016/j.jcmg.2020.04.014
8. Matsuura H, Ichida F, Saji T, Ogawa S, Waki K, Kaneko M, et al. Clinical features of acute and fulminant myocarditis in children-2nd nationwide survey by japanese society of pediatric cardiology and cardiac surgery. Circ J. (2016) 80:2362–8. doi: 10.1253/circj.CJ-16-0234
9. Ito T, Akamatsu K, Ukimura A, Fujisaka T, Ozeki M, Kanzaki Y, et al. The Prevalence and findings of subclinical influenza-associated cardiac abnormalities among Japanese patients. Intern Med. (2018) 57:1819–26. doi: 10.2169/internalmedicine.0316-17
10. Bavishi C, Bonow RO, Trivedi V, Abbott JD, Messerli FH, Bhatt DL. Acute myocardial injury in patients hospitalized with COVID-19 infection: a review. Prog Cardiovasc Dis. (2020) 6:682–9. doi: 10.1016/j.pcad.2020.05.013
11. Bonow RO, Fonarow GC, O'Gara PT, Yancy CW. Association of coronavirus disease 2019 (COVID-19) with myocardial injury and mortality. JAMA Cardiol. (2020) 5:751–3. doi: 10.1001/jamacardio.2020.1105
12. Chinese Clinical Guidance for COVID-19 Pneumonia Diagnosis and Treatment (7th edition). Published by China National Health Commission on March 4, 2020. Available online at: http://kjfy.meeting.so/msite/news/show/cn/3337.html (accessed March 16, 2020).
13. Deng Q, Hu B, Zhang Y, Wang H, Zhou X, Hu W, et al. Suspected myocardial injury in patients with COVID-19: evidence from front-line clinical observation in Wuhan, China. Int J Cardiol. (2020) 311:116–21. doi: 10.1016/j.ijcard.2020.03.087
14. Huang L, Zhao P, Tang D, Zhu T, Han R, Zhan C, et al. Cardiac involvement in patients recovered from COVID-2019 identified using magnetic resonance imaging. JACC Cardiovasc Imaging. (2020). doi: 10.1016/j.jcmg.2020.05.004
15. Puntmann VO, Carerj ML, Wieters I, Fahim M, Arendt C, Hoffmann J, et al. Outcomes of cardiovascular magnetic resonance imaging in patients recently recovered from coronavirus disease 2019 (COVID-19). JAMA Cardiol. (2020) 5:1265–73. doi: 10.1001/jamacardio.2020.3557
16. Rajpal S, Tong MS, Borchers J, Zareba KM, Obarski TP, Simonetti OP, et al. Cardiovascular magnetic resonance findings in competitive athletes recovering from COVID-19 infection. JAMA Cardiol. (2020) 13:e204916. doi: 10.1001/jamacardio.2020.4916
17. Argulian E, Sud K, Vogel B, Bohra C, Garg VP, Talebi S, et al. Right ventricular dilation in hospitalized patients with COVID-19 infection. JACC Cardiovasc Imaging. (2020) 13:2459–61. doi: 10.1016/j.jcmg.2020.05.010
18. Jain SS, Liu Q, Raikhelkar J, Fried J, Elias P, Poterucha TJ, et al. Indications and findings on transthoracic echocardiography in COVID-19. J Am Soc Echocardiogr. (2020) 33:1278–84. doi: 10.1016/j.echo.2020.06.009
19. Dweck MR, Bularga A, Hahn RT, Bing R, Lee KK, Chapman AR, et al. Global evaluation of echocardiography in patients with COVID-19. Eur Heart J Cardiovasc Imaging. (2020) 2:949–58. doi: 10.1093/ehjci/jeaa178
20. Rath D, Petersen-Uribe A, Avdiu A, Witzel K, Jaeger P, Zdanyte M, et al. Impaired cardiac function is associated with mortality in patients with acute COVID-19 infection. Res Rep Clin Cardiol. (2020) 109:1491–9. doi: 10.1007/s00392-020-01683-0
21. Pagnesi M, Baldetti L, Beneduce A, Calvo F, Gramegna M, Pazzanese V, et al. Pulmonary hypertension and right ventricular involvement in hospitalised patients with COVID-19. Heart. (2020) 106:1324–31. doi: 10.1136/heartjnl-2020-317355
22. D'Andrea A, Scarafile R, Riegler L, Liccardo B, Crescibene F, Cocchia R, et al. Right ventricular function and pulmonary pressures as independent predictors of survival in patients with COVID-19 pneumonia. JACC: Cardiovasc Imaging. (2020) 13:2467–8. doi: 10.1016/j.jcmg.2020.06.004
23. Vasudev R, Guragai N, Habib H, Hosein K, Virk H, Goldfarb I, et al. The utility of bedside echocardiography in critically ill COVID-19 patients: early observational findings from three Northern New Jersey hospitals. Echocardiography. (2020) 37:1362–5. doi: 10.1111/echo.14825
24. Baycan OF, Barman HA, Atici A, Tatlisu A, Bolen F, Ergen P., et al. Evaluation of biventricular function in patients with COVID-19 using speckle tracking echocardiography. Int J Cardiovas Imag. (2020) 15:1–10. doi: 10.1007/s10554-020-01968-5
25. Krishnamoorthy P, Croft LB, Ro R, Anastasius M, Zhao W, Giustino G, et al. Biventricular strain by speckle tracking echocardiography in COVID-19: findings and possible prognostic implications. Future Cardiol. (2020). doi: 10.2217/fca-2020-0100
26. van den Heuvel FMA, Vos JL, Koop Y, van Dijk APJ, Duijnhouwer AL, de Mast Q, et al. Cardiac function in relation to myocardial injury in hospitalised patients with COVID-19. Neth Heart J. (2020) 28:410–7. doi: 10.1007/s12471-020-01458-2
27. Zeng JH, Wu WB, Qu JX, Wang Y, Dong CF, Luo YF, et al. Cardiac manifestations of COVID-19 in Shenzhen, China. Infection. (2020) 48:861–70. doi: 10.1007/s15010-020-01473-w
28. Ferreira VM, Schulz-Menger J, Holmvang G, Kramer CM, Carbone I, Sechtem U, et al. Cardiovascular magnetic resonance in non-ischemic myocardial inflammation: expert recommendations. J Am Coll Cardiol. (2018) 72:3158–76. doi: 10.1016/j.jacc.2018.09.072
29. Kammerlander AA, Marzluf BA, Zotter-Tufaro C, Aschauer S, Duca F, Bachmann A, et al. T1 mapping by cmr imaging: from histological validation to clinical implication. JACC Cardiovasc Imaging. (2016) 9:14–23. doi: 10.1016/j.jcmg.2015.11.002
30. Friedrich MG, Sechtem U, Schulz-Menger J, Holmvang G, Alakija P, Cooper LT, et al. Cardiovascular magnetic resonance in myocarditis: a JACC white paper. J Am Coll Cardiol. (2009) 53:1475–87. doi: 10.1016/j.jacc.2009.02.007
31. Puntmann VO, Valbuena S, Hinojar R, Petersen SE, Greenwood JP, Kramer CM, et al. Society for Cardiovascular Magnetic Resonance (SCMR) expert consensus for CMR imaging endpoints in clinical research: part I—analytical validation and clinical qualification. J Cardiovasc Magn Reson. (2018) 20:67. doi: 10.1186/s12968-018-0484-5
32. Lang RM, Badano LP, Mor-Avi V, Afilalo J, Armstrong A, Ernande L, et al. Recommendations for cardiac chamber quantification by echocardiography in adults: an update from the American Society of Echocardiography and the European Association of Cardiovascular Imaging. J Am Soc Echocardiogr. (2015) 28:1–39.e14. doi: 10.1016/j.echo.2014.10.003
33. Zochios V, Parhar K, Tunnicliffe W, Roscoe A, Gao F. The Right Ventricle in ARDS. Chest. (2017) 152:181–93. doi: 10.1016/j.chest.2017.02.019
34. Carluccio E, Biagioli P, Alunni G, Murrone A, Zuchi C, Coiro S, et al. Prognostic value of right ventricular dysfunction in heart failure with reduced ejection fraction: superiority of longitudinal strain over tricuspid annular plane systolic excursion. Circ Cardiovasc Imaging. (2018) 11:e006894. doi: 10.1161/CIRCIMAGING.117.006894
35. Li Y, Xie M, Wang X, Lu Q, Zhang L, Ren P. Impaired right and left ventricular function in asymptomatic children with repaired tetralogy of Fallot by two-dimensional speckle tracking echocardiography study. Echocardiography. (2015) 32:135–43. doi: 10.1111/echo.12581
36. Park SJ, Park JH, Lee HS, Kim MS, Park YK, Park Y, et al. Impaired RV global longitudinal strain is associated with poor long-term clinical outcomes in patients with acute inferior STEMI. Jacc-Cardiovasc Imag. (2015) 8:161–9. doi: 10.1016/j.jcmg.2014.10.011
37. Huang C, Wang Y, Li X, Ren L, Zhao J, Hu Y, et al. Clinical features of patients infected with 2019 novel coronavirus in Wuhan, China. Lancet. (2020) 395:497–506. doi: 10.1016/S0140-6736(20)30183-5
38. Zhou F, Yu T, Du R, Fan G, Liu Y, Liu Z, et al. Clinical course and risk factors for mortality of adult inpatients with COVID-19 in Wuhan, China: a retrospective cohort study. Lancet. (2020) 395:1054–62. doi: 10.1016/S0140-6736(20)30566-3
39. Repesse X, Charron C, Vieillard-Baron A. Right ventricular failure in acute lung injury and acute respiratory distress syndrome. Minerva Anestesiol. (2012) 78:941–8.
40. Boissier F, Katsahian S, Razazi K, Thille AW, Roche-Campo F, Leon R, et al. Prevalence and prognosis of cor pulmonale during protective ventilation for acute respiratory distress syndrome. Intensive Care Med. (2013) 39:1725–33. doi: 10.1007/s00134-013-2941-9
41. Wang D, Hu B, Hu C, Zhu F, Liu X, Zhang J, et al. Clinical characteristics of 138 hospitalized patients with 2019 novel coronavirus-infected pneumonia in Wuhan, China. JAMA. (2020) 323:1061–9. doi: 10.1001/jama.2020.1585
42. Li X, Ma X. Acute respiratory failure in COVID-19: is it “typical” ARDS? Crit Care. (2020) 24:198. doi: 10.1186/s13054-020-02911-9
43. Nobre C, Thomas B. Right ventricle in ARDS. Chest. (2017) 152:215–6. doi: 10.1016/j.chest.2017.04.163
44. Repesse X, Charron C, Vieillard-Baron A. Acute respiratory distress syndrome: the heart side of the moon. Curr Opin Crit Care. (2016) 22:38–44. doi: 10.1097/MCC.0000000000000267
45. Poissy J, Goutay J, Caplan M, Parmentier E, Duburcq T, Lassalle F, et al. Pulmonary embolism in patients with COVID-19: awareness of an increased prevalence. Circulation. (2020) 142:184–6. doi: 10.1161/CIRCULATIONAHA.120.047430
46. Wichmann D, Sperhake JP, Lutgehetmann M, Steurer S, Edler C, Heinemann A, et al. Autopsy findings and venous thromboembolism in patients With COVID-19: a prospective cohort study. Ann Intern Med. (2020) 173:268–77. doi: 10.7326/L20-1206
47. Huisman MV, Barco S, Cannegieter SC, Le Gal G, Konstantinides SV, Reitsma PH, et al. Pulmonary embolism. Nat Rev Dis Primers. (2018) 4:18028. doi: 10.1038/nrdp.2018.28
48. Inciardi RM, Lupi L, Zaccone G, Italia L, Raffo M, Tomasoni D, et al. Cardiac involvement in a patient with coronavirus disease 2019 (COVID-19). JAMA Cardiol. (2020) 5:819–24. doi: 10.1001/jamacardio.2020.1096
49. Khan IH, Zahra SA, Zaim S, Harky A. At the heart of COVID-19. J Cardiac Surg. (2020) 35:1287–94. doi: 10.1111/jocs.14596
50. Ruan QR, Yang K, Wang WX, Jiang LY, Song JX. Clinical predictors of mortality due to COVID-19 based on an analysis of data of 150 patients from Wuhan, China. Intensive Care Medicine. (2020) 46:846–8. doi: 10.1007/s00134-020-05991-x
51. Zhu H, Rhee JW, Cheng P, Waliany S, Chang A, Witteles RM, et al. Cardiovascular complications in patients with COVID-19: consequences of viral toxicities and host immune response. Curr Cardiol Rep. (2020) 22:32. doi: 10.1007/s11886-020-01302-4
52. Nduka OO, Parrillo JE. The pathophysiology of septic shock. Crit Care Clin. (2009) 25:677–702. doi: 10.1016/j.ccc.2009.08.002
53. Clerkin KJ, Fried JA, Raikhelkar J, Sayer G, Griffin JM, Masoumi A, et al. COVID-19 and cardiovascular disease. Circulation. (2020) 141:1648–55. doi: 10.1161/CIRCULATIONAHA.120.046941
54. Zheng YY, Ma YT, Zhang JY, Xie X. COVID-19 and the cardiovascular system. Nat Rev Cardiol. (2020) 17:259–60. doi: 10.1038/s41569-020-0360-5
55. Boukhris M, Hillani A, Moroni F, Annabi MS, Addad F, Ribeiro MH, et al. Cardiovascular implications of the COVID-19 pandemic: a global perspective. Can J Cardiol. (2020) 36:1068–80. doi: 10.1016/j.cjca.2020.05.018
56. Ventetuolo CE, Klinger JR. Management of acute right ventricular failure in the intensive care unit. Ann Am Thorac Soc. (2014) 11:811–22. doi: 10.1513/AnnalsATS.201312-446FR
57. Morelli A, Teboul JL, Maggiore SM, Vieillard-Baron A, Rocco M, Conti G, et al. Effects of levosimendan on right ventricular afterload in patients with acute respiratory distress syndrome: a pilot study. Crit Care Med. (2006) 34:2287–93. doi: 10.1097/01.CCM.0000230244.17174.4F
58. Hirsch LJ, Rooney MW, Wat SS, Kleinmann B, Mathru M. Norepinephrine and phenylephrine effects on right ventricular function in experimental canine pulmonary embolism. Chest. (1991) 100:796–801. doi: 10.1378/chest.100.3.796
59. Kisch-Wedel H, Kemming G, Meisner F, Flondor M, Kuebler WM, Bruhn S, et al. The prostaglandins epoprostenol and iloprost increase left ventricular contractility in vivo. Intensive Care Med. (2003) 29:1574–83. doi: 10.1007/s00134-003-1891-z
60. Hunt JL, Bronicki RA, Anas N. Role of inhaled nitric oxide in the management of severe acute respiratory distress syndrome. Front Pediatr. (2016) 4:74. doi: 10.3389/fped.2016.00074
61. Searcy RJ, Morales JR, Ferreira JA, Johnson DW. The role of inhaled prostacyclin in treating acute respiratory distress syndrome. Ther Adv Respir Dis. (2015) 9:302–12. doi: 10.1177/1753465815599345
62. Lang IM, Gaine SP. Recent advances in targeting the prostacyclin pathway in pulmonary arterial hypertension. Eur Respir Rev. (2015) 24:630–41. doi: 10.1183/16000617.0067-2015
63. Meduri GU, Siemieniuk RAC, Ness RA, Seyler SJ. Prolonged low-dose methylprednisolone treatment is highly effective in reducing duration of mechanical ventilation and mortality in patients with ARDS. J Intensive Care Med. (2018) 6:53. doi: 10.1186/s40560-018-0321-9
64. Organization WH. Infection Prevention and Control During Health Care When Novel Coronavirus (ncov) Infection is Suspected. (2020). Available online at: https://www.who.int/publications/i/item/10665-331495 (accessed March 19, 2020).
65. Tang N, Li D, Wang X, Sun Z. Abnormal coagulation parameters are associated with poor prognosis in patients with novel coronavirus pneumonia. J Thromb Haemost. (2020) 18:844–7. doi: 10.1111/jth.14768
66. Tang N, Bai H, Chen X, Gong JL, Li DJ, Sun ZY. Anticoagulant treatment is associated with decreased mortality in severe coronavirus disease 2019 patients with coagulopathy. J Thromb Haemost. (2020) 18:1094–9. doi: 10.1111/jth.14817
67. Lin L, Lu LF, Cao W, Li TS. Hypothesis for potential pathogenesis of SARS-CoV-2 infection-a review of immune changes in patients with viral pneumonia. Emerg Microbes Infec. (2020) 9:727–32. doi: 10.1080/22221751.2020.1746199
68. Repesse X, Charron C, Vieillard-Baron A. Acute cor pulmonale in ARDS: rationale for protecting the right ventricle. Chest. (2015) 14:259–65. doi: 10.1378/chest.14-0877
69. Organization WH. Clinical Management of Severe Acute Respiratory Infection When Novel Coronavirus (nCoV) Infection Is Suspected: Interim Guidance. (2020). Available online at: https://www.who.int/publications/i/item/10665-332299 (accessed January 12, 2020).
Keywords: COVID-19, right ventricular damage, myocardial injury, cardiovascular magnetic resonance, echocardiography, ARDS
Citation: Lan Y, Liu W and Zhou Y (2021) Right Ventricular Damage in COVID-19: Association Between Myocardial Injury and COVID-19. Front. Cardiovasc. Med. 8:606318. doi: 10.3389/fcvm.2021.606318
Received: 14 September 2020; Accepted: 26 January 2021;
Published: 16 February 2021.
Edited by:
Shuyang Zhang, Peking Union Medical College Hospital, ChinaReviewed by:
Bastiaan Geelhoed, University Medical Center Groningen, NetherlandsSander W. van der Laan, University Medical Center Utrecht, Netherlands
Copyright © 2021 Lan, Liu and Zhou. This is an open-access article distributed under the terms of the Creative Commons Attribution License (CC BY). The use, distribution or reproduction in other forums is permitted, provided the original author(s) and the copyright owner(s) are credited and that the original publication in this journal is cited, in accordance with accepted academic practice. No use, distribution or reproduction is permitted which does not comply with these terms.
*Correspondence: Wei Liu, liuwei@ccmu.edu.cn