- 1Department of Thoracic and Cardiovascular Surgery, Shenzhen Baoan Women's and Children's Hospital, Jinan University, Guangzhou, China
- 2Center for Cardiac Intensive Care, Beijing Anzhen Hospital, Beijing, China
Objective: Randomized controlled trials (RCTs) evaluating the influence of remote ischemic preconditioning (RIPC) on acute kidney injury (AKI) after cardiac surgery showed inconsistent results. We performed a meta-analysis to evaluate the efficacy of RIPC on AKI after cardiac surgery.
Methods: Relevant studies were obtained by search of PubMed, Embase, and Cochrane's Library databases. A random-effect model was used to pool the results. Meta-regression and subgroup analyses were used to determine the source of heterogeneity.
Results: Twenty-two RCTs with 5,389 patients who received cardiac surgery −2,702 patients in the RIPC group and 2,687 patients in the control group—were included. Moderate heterogeneity was detected (p for Cochrane's Q test = 0.03, I2 = 40%). Pooled results showed that RIPC significantly reduced the incidence of AKI compared with control [odds ratio (OR): 0.76, 95% confidence intervals (CI): 0.61–0.94, p = 0.01]. Results limited to on-pump surgery (OR: 0.78, 95% CI: 0.64–0.95, p = 0.01) or studies with acute RIPC (OR: 0.78, 95% CI: 0.63–0.97, p = 0.03) showed consistent results. Meta-regression and subgroup analyses indicated that study characteristics, including study design, country, age, gender, diabetic status, surgery type, use of propofol or volatile anesthetics, cross-clamp time, RIPC protocol, definition of AKI, and sample size did not significantly affect the outcome of AKI. Results of stratified analysis showed that RIPC significantly reduced the risk of mild-to-moderate AKI that did not require renal replacement therapy (RRT, OR: 0.76, 95% CI: 0.60–0.96, p = 0.02) but did not significantly reduce the risk of severe AKI that required RRT in patients after cardiac surgery (OR: 0.73, 95% CI: 0.50–1.07, p = 0.11).
Conclusions: Current evidence supports RIPC as an effective strategy to prevent AKI after cardiac surgery, which seems to be mainly driven by the reduced mild-to-moderate AKI events that did not require RRT. Efforts are needed to determine the influences of patient characteristics, procedure, perioperative drugs, and RIPC protocol on the outcome.
Introduction
Acute kidney injury (AKI) is common for patients after cardiac surgery, particularly for patients who receive cardiac surgery with complex procedures, such as on-pump surgery, concomitant coronary artery bypass graft (CABG) with valvular surgery, longer aortic cross-clamp time during cardiopulmonary bypass, and surgeries of open total aortic arch replacement (1–3). Previous studies showed that patients with postoperative AKI, even of mild degree, had worse clinical outcome after cardiac surgery (4). Currently, multiple criteria have been applied to define AKI after cardiac surgery, while consensus regarding the optimal definition of AKI remains lacking (5). Among which, the Acute Kidney Injury Network (AKIN) and the Risk, Injury, Failure, Loss, End Stage Kidney Disease (RIFLE) have been the mostly used criteria to define AKI, while the Kidney Disease: Improving Global Outcomes (KDIGO) criteria for AKI staging has been shown to demonstrate greater sensitivity to detect AKI and to predict associated in-hospital mortality, than do the RIFLE or AKIN criteria (5). Since treatment options for postoperative AKI remain limited, identification of strategies to prevent the incidence of AKI after cardiac surgery are clinically important (6). Remote ischemic preconditioning (RIPC), which is known as a strategy that protects the target organ by inducing brief episodes of ischemia and reperfusion in distant tissue, has been suggested to be effective for preventing AKI (7–9). However, randomized clinical trials (RCTs) evaluating the influence of RIPC on AKI after cardiac surgery showed inconsistent results (10–31). Since most of these studies were of limited sample size, the individual studies may have been statistically insufficient to detect a significant influence of RIPC on postoperative AKI. Furthermore, several previous meta-analyses that pooled studies published through 2016 showed that RIPC was not effective at preventing AKI in patients after on-pump cardiac surgery (32–34). Although, these results were also inconsistent (35). Many RCTs have been published since 2016 (22, 24–31), warranting an updated meta-analysis. In addition, the potential impacts of study and patient features on the efficacy of RIPC on postoperative AKI in these patients were rarely analyzed. Therefore, we performed an updated meta-analysis with comprehensive subgroup analyses to investigate the role of RIPC to prevent AKI after cardiac surgery.
Methods
The Preferred Reporting Items for Systematic Reviews and Meta-Analyses (PRISMA) statement (36) and the Cochrane Handbook guidelines (37) were followed during the designing and implementation of the study.
Search Strategy
PubMed, Embase, and the Cochrane Library (Cochrane Center Register of Controlled Trials) databases were searched for relevant studies with a combined strategy of: [1] “ischemic preconditioning” OR “remote ischemic preconditioning” OR “RIPC”; [2] “cardiac surgery” OR “coronary artery bypass” OR “surgical coronary revascularization” OR “valve surgery” OR “valve replacement”; and [3] “random” OR “randomized” OR “randomized” OR “randomly.” Only clinical studies were considered. The references of related reviews and original articles were also searched as a complementation. The latest database search was conducted on 5th May 2020.
Study Selection
Inclusion criteria were as follows: [1] peer-reviewed articles in English or Chinese; [2] designed as parallel-group RCTs; [3] included adult patients scheduled for open heart surgery who were randomly allocated to a RIPC treatment group or a control group; and [4] reported the incidence of AKI in the perioperative periods. Reviews, studies including children or neonates, preclinical studies, observational studies, and repeated reports were excluded.
Data Extraction and Quality Assessment
Study search, data extraction, and quality evaluation were achieved by two independent authors. If disagreement occurred, it was resolved by consensus between the two authors. We extracted data regarding study information (first author, publication year, and study country), study design (blind or open-label), patient information (number of participants, mean age, gender, diabetic status, and patients with severe cardiac systolic dysfunction), surgery type, perioperative anesthetics (use of propofol or volatile anesthetics, and cross-clamp time), RIPC protocol, and definition of AKI. Quality evaluation was achieved using the Cochrane's Risk of Bias Tool (37) according to the following aspects: [1] random sequence generation; [2] allocation concealment; [3] blinding of participants and personnel; [4] blinding of outcome assessors; [5] incomplete outcome data; [6] selective outcome reporting; and [7] other potential bias.
Statistical Analysis
Incidence of AKI in each arm was evaluated via odds ratio (OR) and its 95% confidence intervals (CIs). We used the Cochrane's Q test to detect the heterogeneity, and significant heterogeneity was suggested if p < 0.10 (38). The I2 statistic was also calculated, and an I2 > 50% reflected significant heterogeneity. Pooled analyses were calculated using a random-effect model because this method incorporates the influence of potential heterogeneity and retrieves a more generalized result (37). Stratified analyses comparing the results in complex and simple cardiac surgeries were also performed. We defined complex surgeries as double-valve or triple-valve surgery, mitral valve surgery, coronary artery bypass graft (CABG) plus valve(s), open total aortic arch replacement, or any “redo” operation. Accordingly, CABG or single-valve surgeries were defined as simple surgeries. Predefined meta-regression analyses, sensitivity analyses, and subgroup analyses were performed to explore the potential influences of study characteristics on the outcome. These characteristics included study design, country, age, gender, diabetic status, surgery type, use of propofol, or volatile anesthetics, cross-clamp time, RIPC protocol, definition of AKI, and sample size of the RCT (39). For continuous variables, medians were used for cut-off. To evaluate the influence of RIPC on the AKI events with different severity, we defined patients with mild-to-moderate AKI as those that did not require renal replacement therapy (RRT) and those with severe AKI as patients that required RRT. Stratified analysis was performed accordingly. Publication bias was evaluated by visual inspection of funnel plots, and the Egger's regression asymmetry test (40). p < 0.05 were considered statistically significant. The RevMan (Version 5.1; Cochrane, Oxford, UK) and Stata software (Version 12.0; Stata, College Station, TX) were applied for statistical analyses.
Results
Search Results
In summary, 1,035 articles were obtained through the database search. After exclusion of duplicate studies, 956 articles were screened. Among them, 871 articles were subsequently excluded based on titles and abstracts primarily because these studies were irrelevant. Among the 85 potentially relevant articles, 63 were further excluded via full-text review based on reasons listed in Figure 1. Finally, 22 RCTs (10–31) were included.
Study Characteristics
Table 1 shows the characteristics of the included studies. Overall, 22 RCTs (10–31) with 5,389 patients who received cardiac surgery −2,702 patients in the RIPC group and 2,687 patients in the control group—were included. These studies were published between 2010 and 2020. Patients with normal kidney function at baseline were included except one study that included patients with chronic kidney disease (CKD) who were scheduled for cardiac surgery (17). With regard to the surgery procedures, 20 studies included patients who received on-pump surgeries (10–28, 30), while the remaining two studies included patients who received off-pump surgeries (29, 31). Proportions of diabetic patients varied among the included studies, and patients with severe cardiac systolic function (left ventricular ejection fraction <30%) were rarely included. The use of propofol and volatile anesthetics varied among the patients of the included studies. RIPC was performed after anesthesia induction and before CPB in most of the included studies (acute RIPC), except in one study in which RIPC was performed 24–48 h before the surgery (chronic RIPC) (25). The protocol of RIPC included 3–4 cycles of upper or lower limb ischemia (5–10 min blood pressure cuff inflation to a pressure of 200 mmHg or at least a pressure that was 20 mmHg higher than the systolic arterial pressure), followed by 5–10 min reperfusion (with the cuff deflated). Uninflated cuffs were used on patients in the control group. Most of the studies used the AKIN definition of AKI, while the RIFLE (13, 19, 28) and the KDIGO (18, 20, 30, 31) definitions were used in three and four studies, respectively.
Data Quality
Table 2 shows the details of study quality evaluation. Most of the included RCTs were double blinded except for four studies, which were single blinded (10, 12, 17, 23). Methods of random sequence generation were reported in 19 studies, and information of allocation concealment was reported in 14 studies. The overall quality score varied between 4 and 7, which suggested a generally good study quality.
Meta-Analysis Results
Moderate heterogeneity was detected (p for Cochrane's Q test = 0.03, I2 = 40%) among the included RCTs. Pooled results with a random-effect model showed that RIPC significantly reduced the incidence of AKI after cardiac surgery compared with the control (OR: 0.76, 95% CI: 0.61–0.94, p = 0.01; Figure 2). Stratified analyses indicated that the effect of RIPC on postoperative AKI was not significantly different in studies with simple, complex, or mixed procedures (p for subgroup difference = 0.88, Figure 3). Results of the univariate meta-regression analyses suggested that study sample size, age, gender, diabetic status, volatile anesthetic use, and cross-clamp time in CPB did not significantly affect the outcome (p all >0.05; Table 3). Moreover, sensitivity analyses confirmed a potential preventative efficacy of RIPC on postoperative AKI in patients with normal renal function at baseline (21 studies, OR: 0.75, 95% CI: 0.60–0.93, p = 0.01), in studies with on-pump surgery only (20 studies, OR: 0.78, 95% CI: 0.64–0.95, p = 0.01), and in studies with acute RIPC (21 studies, OR: 0.78, 95% CI: 0.63–0.97, p = 0.03; Table 4). Further subgroup analyses indicated that study characteristics, including study design, country, age, gender, diabetic status, surgery type, use of propofol or volatile anesthetics, cross-clamp time, RIPC protocol, definition of AKI, or sample size of the RCT did not significantly affect AKI outcome (p for subgroup difference all >0.10; Table 4). Stratified analysis according to the severity of AKI showed that RIPC significantly reduced the risk of mild-to-moderate AKI that did not require RRT (OR: 0.76, 95% CI: 0.60–0.96, p = 0.02) but did not significantly reduce the risk of severe AKI that required RRT in patients after cardiac surgery (OR: 0.73, 95% CI: 0.50–1.07, p = 0.11; Figure 4).
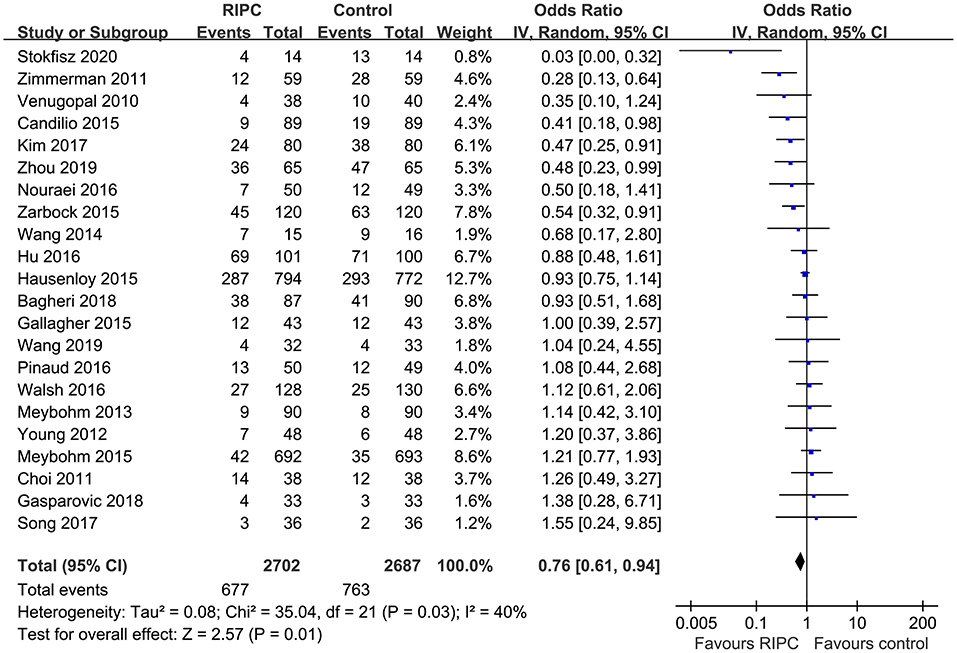
Figure 2. Forest plots for the meta-analysis comparing the efficacy of RIPC and control on AKI after cardiac surgery.
Publication Bias
The funnel plots were symmetrical, suggesting low-risk of publication bias (Figure 5). Egger's regression tests showed similar results (p = 0.28).
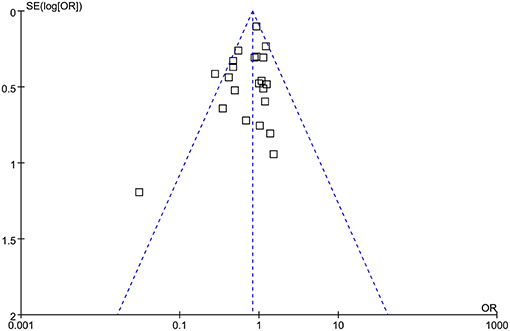
Figure 5. Funnel plots for the meta-analysis comparing the efficacy of RIPC and control on AKI after cardiac surgery.
Discussion
In this updated meta-analysis, we pooled the results of 22 RCTs that involved 5,389 patients who received cardiac surgery and found that RIPC significantly reduced the incidence of postoperative AKI in these patients. Sensitivity analyses showed consistent results in studies with on-pump surgery only, in studies of patients with normal renal function at baseline, and in studies evaluating the acute efficacy of RIPC. In addition, results of meta-regression and subgroup analyses indicated that characteristics, including study design, country, age, gender, diabetic status, surgery type, use of propofol or volatile anesthetics, cross-clamp time, RIPC protocol, definition of AKI, and sample size of the RCT did not significantly impact the efficacy of RIPC on postoperative AKI. Moreover, stratified analysis showed that RIPC significantly reduced the risk of mild-to-moderate AKI that did not require RRT but did not significantly reduce the risk of severe AKI that required RRT in patients after cardiac surgery. To sum up, these results suggest that RIPC is effective for preventing AKI after cardiac surgery, which seems to be mainly driven by the reduced mild-to-moderate AKI events that did not require RRT. Further efforts are needed to determine the influences of patient characteristics, procedure, perioperative drugs, and RIPC protocol on AKI outcome.
Some meta-analyses have been previously published evaluating the use of RIPC for preventing AKI after cardiac surgery (32–35). Compared with these studies, our updated meta-analysis has the following strengths. First, we included the most recent RCTs with more than 5,000 patients, which is a much larger sample population than was included in the previous meta-analyses. The relatively large number of available RCTs and sample size of this meta-analysis may overcome the potential statistical inadequacy of previously published individual RCTs and meta-analyses. Secondly, some RCTs (41, 42) evaluating the efficacy of combined remote ischemic pre- and post-conditioning, rather than RIPC alone, on AKI were included in previous meta-analyses (34, 35), which may have confounded the results. In our meta-analysis, only studies that compared RIPC and control on AKI after cardiac surgery were included, which therefore reflects the potential benefits of RIPC only. Finally, the relative larger number of available RCTs enables us to perform comprehensive meta-regression and subgroup analyses of the influences of study characteristics on AKI outcome. This is important because these factors were rarely considered in previous meta-analyses, probably due to the limited RCTs available. In this study, we synthesized the current evidence from RCTs and showed that RIPC is effective for preventing AKI after cardiac surgery. Besides the potential preventative efficacy on AKI, previous studies have shown that RIPC is also effective to attenuate perioperative myocardial injury (43) and reduce the risk of new onset atrial fibrillation (44) in patients undergoing cardiac surgery and may reduce mortality in patients receiving volatile inhalational agent anesthesia (34). Taken together, these findings support the benefits of RIPC use for patients undergoing cardiac surgeries.
Moderate heterogeneity was detected among the included studies in our meta-analysis. Although we aimed to evaluate the potential contribution of study characteristics on AKI outcome, results of meta-regression and subgroup analyses did not reveal any significant relationship between these factors and the efficacy of RIPC on postoperative AKI. It has been suggested by previous meta-analyses that patient age, complexity of the surgical procedure, and use of propofol may significantly modify the efficacy of RIPC on postoperative AKI (32, 35). However, by including more eligible RCTs, we found that these factors played no significant role on the outcome of AKI following RIPC. In fact, some recently published experimental studies suggest that the potential interaction between these factors, such as propofol, with organ protective efficacy of RIPC may be more complicated than expected. A recent study in a rat model found that the timing of propofol administration could affect RIPC-induced cardioprotection (45), and that different doses of propofol used in the studies may have also affected the results. Future studies are needed to determine the influences of patient characteristics, procedure, perioperative drugs, and RIPC protocol on the efficacy of RIPC on AKI prevention.
The potential mechanisms underlying the preventative role of RIPC on AKI after cardiac surgery are likely multifactorial (8, 9). Generally, RIPC is considered to mediate organ protection via regulating both the sympathetic and parasympathetic components of the autonomic nervous system (46–48). Ischemia has been associated with activation of sensory (afferent) C-fiber neurons, which trigger the vagus nerve of a distant organ, such as the kidney, consequently exerting protective effects (49). Experimental studies showed that RIPC was associated with the release of various humoral factors, which confers anti-inflammatory and anti-oxidative efficacies (50), two predominant pathogenic mechanisms in AKI. However, the exact mechanisms and key molecular pathways involved in the potential renoprotective efficacy of RIPC remain to be determined.
Our study has some limitations. First, the definitions of AKI varied across the included studies. Although the difference between subgroups was not significantly different, our subgroup analyses suggest that RIPC reduced AKI after cardiac surgery in studies that applied the AKIN definition but not in those that used the RIFLE or KDIGO definition. Since the optimal definition of AKI has yet to be established (51), we could not exclude the possibility that difference in the definition of AKI may affect the outcome of the meta-analysis. Secondly, no access to individual patient data was obtained. Therefore, subgroup analyses were based on study-level data, and results of the subgroup analyses should be interpreted with caution. Future large-scale RCTs or meta-analysis based on individual patient data are needed to validate the results. Moreover, the sample sizes of the included RCTs varied significantly. The two RCTs with largest sample sizes comprised over half of the included patients of the meta-analysis, which may primarily contribute to the results of overall meta-analysis and therefore comprise the importance of the meta-analysis. Besides, variations in the details of protocols for RIPC may affect its influence on AKI. We have performed subgroup analysis to evaluate the potential difference of RIPC performed in upper and lower limbs. However, influences of other details of RIPC, such as number of cycles, pressure applied and durations, and more importantly, the combinations of these factors could not be evaluated in the current meta-analysis. Finally, we did not perform stratified analyses according to the degree of AKI since data regarding the severity of AKI were rare in the included studies. Future studies are warranted in this regard.
In conclusion, results of this updated meta-analysis suggest that RIPC is effective for preventing AKI after cardiac surgery, which seems to be mainly driven by the reduced mild-to-moderate AKI events that did not require RRT. More studies are warranted to determine the influence of patient characteristics, procedure, perioperative drugs, and RIPC protocol on AKI outcome.
Data Availability Statement
The raw data supporting the conclusions of this article will be made available by the authors, without undue reservation.
Author Contributions
ZL and YZ contributed to the conception and design of the study. ZL, YZ, ML, and GZ performed the statistical analysis. ZL, YZ, DL, and RS wrote the first draft of the manuscript. XL wrote sections of the manuscript. All authors contributed to manuscript revision and read and approved the submitted version.
Conflict of Interest
The authors declare that the research was conducted in the absence of any commercial or financial relationships that could be construed as a potential conflict of interest.
References
1. Harky A, Joshi M, Gupta S, Teoh WY, Gatta F, Snosi M. Acute kidney injury associated with cardiac surgery: a comprehensive literature review. Braz J Cardiovasc Surg. (2020) 35:211–24. doi: 10.21470/1678-9741-2019-0122
2. Li Y, Xu J, Wang Y, Zhang Y, Jiang W, Shen B, et al. A novel machine learning algorithm, Bayesian networks model, to predict the high-risk patients with cardiac surgery-associated acute kidney injury. Clin Cardiol. (2020) 43:752–61. doi: 10.1002/clc.23377
3. Gumbert SD, Kork F, Jackson ML, Vanga N, Ghebremichael SJ, Wang CY, et al. Perioperative acute kidney injury. Anesthesiology. (2020) 132:180–204. doi: 10.1097/ALN.0000000000002968
4. Pickering JW, James MT, Palmer SC. Acute kidney injury and prognosis after cardiopulmonary bypass: a meta-analysis of cohort studies. Am J Kidney Dis. (2015) 65:283–93. doi: 10.1053/j.ajkd.2014.09.008
5. Wang Y, Bellomo R. Cardiac surgery-associated acute kidney injury: risk factors, pathophysiology and treatment. Nat Rev Nephrol. (2017) 13:697–711. doi: 10.1038/nrneph.2017.119
6. Vives M, Hernandez A, Parramon F, Estanyol N, Pardina B, Munoz A, et al. Acute kidney injury after cardiac surgery: prevalence, impact and management challenges. Int J Nephrol Renovasc Dis. (2019) 12:153–66. doi: 10.2147/IJNRD.S167477
7. Hu J, Liu S, Jia P, Xu X, Song N, Zhang T, et al. Protection of remote ischemic preconditioning against acute kidney injury: a systematic review and meta-analysis. Crit Care. (2016) 20:111. doi: 10.1186/s13054-016-1272-y
8. Giannopoulos G, Vrachatis DA, Panagopoulou V, Vavuranakis M, Cleman MW, Deftereos S. Remote ischemic conditioning and renal protection. J Cardiovasc Pharmacol Ther. (2017) 22:321–9. doi: 10.1177/1074248417702480
9. Zarbock A, Kellum JA, Gourine AV, Ackland GL. Salvaging remote ischaemic preconditioning as a therapy for perioperative acute kidney injury. Br J Anaesth. (2020) 124:8–12. doi: 10.1016/j.bja.2019.09.009
10. Venugopal V, Laing CM, Ludman A, Yellon DM, Hausenloy D. Effect of remote ischemic preconditioning on acute kidney injury in nondiabetic patients undergoing coronary artery bypass graft surgery: a secondary analysis of 2 small randomized trials. Am J Kidney Dis. (2010) 56:1043–9. doi: 10.1053/j.ajkd.2010.07.014
11. Choi YS, Shim JK, Kim JC, Kang KS, Seo YH, Ahn KR, et al. Effect of remote ischemic preconditioning on renal dysfunction after complex valvular heart surgery: a randomized controlled trial. J Thorac Cardiovasc Surg. (2011) 142:148–54. doi: 10.1016/j.jtcvs.2010.11.018
12. Zimmerman RF, Ezeanuna PU, Kane JC, Cleland CD, Kempananjappa TJ, Lucas FL, et al. Ischemic preconditioning at a remote site prevents acute kidney injury in patients following cardiac surgery. Kidney Int. (2011) 80:861–7. doi: 10.1038/ki.2011.156
13. Young PJ, Dalley P, Garden A, Horrocks C, La Flamme A, Mahon B, et al. A pilot study investigating the effects of remote ischemic preconditioning in high-risk cardiac surgery using a randomised controlled double-blind protocol. Basic Res Cardiol. (2012) 107:256. doi: 10.1007/s00395-012-0256-6
14. Meybohm P, Renner J, Broch O, Caliebe D, Albrecht M, Cremer J, et al. Postoperative neurocognitive dysfunction in patients undergoing cardiac surgery after remote ischemic preconditioning: a double-blind randomized controlled pilot study. PLoS ONE. (2013) 8:e64743. doi: 10.1371/journal.pone.0064743
15. Wang Q, Luo W, Zhou Q. [Intervention of NGAL and HO-1 in valve replacement surgery-induced acute kidney injury]. Zhong Nan Da Xue Xue Bao Yi Xue Ban. (2014) 39:1001–7.
16. Candilio L, Malik A, Ariti C, Barnard M, Di Salvo C, Lawrence D, et al. Effect of remote ischaemic preconditioning on clinical outcomes in patients undergoing cardiac bypass surgery: a randomised controlled clinical trial. Heart. (2015) 101:185–92. doi: 10.1136/heartjnl-2014-306178
17. Gallagher SM, Jones DA, Kapur A, Wragg A, Harwood SM, Mathur R, et al. Remote ischemic preconditioning has a neutral effect on the incidence of kidney injury after coronary artery bypass graft surgery. Kidney Int. 2015 Feb;87(2):473-81. doi: 10.1038/ki.2014.259
18. Hausenloy DJ, Candilio L, Evans R, Ariti C, Jenkins DP, Kolvekar S, et al. Remote ischemic preconditioning and outcomes of cardiac surgery. N Engl J Med. (2015) 373:1408–17. doi: 10.1056/NEJMoa1413534
19. Meybohm P, Bein B, Brosteanu O, Cremer J, Gruenewald M, Stoppe C, et al. A multicenter trial of remote ischemic preconditioning for heart surgery. N Engl J Med. (2015) 373:1397–407. doi: 10.1056/NEJMoa1413579
20. Zarbock A, Schmidt C, Van Aken H, Wempe C, Martens S, Zahn PK, et al. Effect of remote ischemic preconditioning on kidney injury among high-risk patients undergoing cardiac surgery: a randomized clinical trial. JAMA. (2015) 313:2133–41. doi: 10.1001/jama.2015.4189
21. Hu Q, Luo W, Huang L, Huang R, Chen R, Gao Y. Multiorgan protection of remote ischemic perconditioning in valve replacement surgery. J Surg Res. (2016) 200:13–20. doi: 10.1016/j.jss.2015.06.053
22. Nouraei SM, Baradari AG, Jazayeri A. Does remote ischaemic preconditioning protect kidney and cardiomyocytes after coronary revascularization? A double blind controlled clinical trial. Med Arch. (2016) 70:373–8. doi: 10.5455/medarh.2016.70.373-378
23. Pinaud F, Corbeau JJ, Baufreton C, Binuani JP, De Brux JL, Fouquet O, et al. Remote ischemic preconditioning in aortic valve surgery: results of a randomized controlled study. J Cardiol. (2016) 67:36–41. doi: 10.1016/j.jjcc.2015.06.007
24. Walsh M, Whitlock R, Garg AX, Legare JF, Duncan AE, Zimmerman R, et al. Effects of remote ischemic preconditioning in high-risk patients undergoing cardiac surgery (Remote IMPACT): a randomized controlled trial. CMAJ. (2016) 188:329–36. doi: 10.1503/cmaj.150632
25. Kim TK, Min JJ, Cho YJ, Hausenloy DJ, Ahn H, Kim KH, et al. Effects of delayed remote ischemic preconditioning on peri-operative myocardial injury in patients undergoing cardiac surgery - a randomized controlled trial. Int J Cardiol. (2017) 227:511–5. doi: 10.1016/j.ijcard.2016.10.111
26. Song Y, Song JW, Lee S, Jun JH, Kwak YL, Shim JK. Effects of remote ischemic preconditioning in patients with concentric myocardial hypertrophy: a randomized, controlled trial with molecular insights. Int J Cardiol. (2017) 249:36–41. doi: 10.1016/j.ijcard.2017.08.073
27. Bagheri S, Shahbazi S, Shafa M, Borhani-Haghighi A, Kiani M, Sagheb MM. The effect of remote ischemic preconditioning on the incidence of acute kidney injury in patients undergoing coronary artery bypass graft surgery: a randomized controlled trial. Iran J Med Sci. (2018) 43:587–95.
28. Gasparovic H, Kopjar T, Rados M, Anticevic A, Malojcic B, Ivancan V, et al. Impact of remote ischemic preconditioning preceding coronary artery bypass grafting on inducing neuroprotection. J Thorac Cardiovasc Surg. (2019) 157:1466–76.e3. doi: 10.1016/j.jtcvs.2018.08.116
29. Wang H, Lyu Y, Liao Q, Jin L, Xu L, Hu Y, et al. Effects of remote ischemic preconditioning in patients undergoing off-pump coronary artery bypass graft surgery. Front Physiol. (2019) 10:495. doi: 10.3389/fphys.2019.00495
30. Zhou H, Yang L, Wang G, Zhang C, Fang Z, Lei G, et al. Remote ischemic preconditioning prevents postoperative acute kidney injury after open total aortic arch replacement: a double-blind, randomized, sham-controlled trial. Anesth Analg. (2019) 129:287–93. doi: 10.1213/ANE.0000000000004127
31. Stokfisz K, Ledakowicz-Polak A, Zagorski M, Jander S, Przybylak K, Zielinska M. The clinical utility of remote ischemic preconditioning in protecting against cardiac surgery-associated acute kidney injury: a pilot randomized clinical trial. Adv Clin Exp Med. (2020) 29:189–96. doi: 10.17219/acem/112610
32. Pierce B, Bole I, Patel V, Brown DL. Clinical outcomes of remote ischemic preconditioning prior to cardiac surgery: a meta-analysis of randomized controlled trials. J Am Heart Assoc. (2017) 6:e004666. doi: 10.1161/JAHA.116.004666
33. Yi B, Wang J, Yi D, Zhu Y, Jiang Y, Li Y, et al. Remote ischemic preconditioning and clinical outcomes in on-pump coronary artery bypass grafting: a meta-analysis of 14 randomized controlled trials. Artif Organs. (2017) 41:1173–82. doi: 10.1111/aor.12900
34. Xie J, Zhang X, Xu J, Zhang Z, Klingensmith NJ, Liu S, et al. Effect of remote ischemic preconditioning on outcomes in adult cardiac surgery: a systematic review and meta-analysis of randomized controlled studies. Anesth Analg. (2018) 127:30–8. doi: 10.1213/ANE.0000000000002674
35. Zhou C, Bulluck H, Fang N, Li L, Hausenloy DJ. Age and surgical complexity impact on renoprotection by remote ischemic preconditioning during adult cardiac surgery: a meta analysis. Sci Rep. (2017) 7:215. doi: 10.1038/s41598-017-00308-3
36. Moher D, Liberati A, Tetzlaff J, Altman DG. Preferred reporting items for systematic reviews and meta-analyses: the PRISMA statement. BMJ. (2009) 339:b2535. doi: 10.1136/bmj.b2535
37. Higgins J, Green S. Cochrane handbook for systematic reviews of interventions version 5.1.0. The Cochrane Collaboration. (2011). Available online at: www.cochranehandbook.org.
38. Higgins JP, Thompson SG. Quantifying heterogeneity in a meta-analysis. Stat Med. (2002) 21:1539–58. doi: 10.1002/sim.1186
39. Higgins JP, Thompson SG, Deeks JJ, Altman DG. Measuring inconsistency in meta-analyses. BMJ. (2003) 327:557–60. doi: 10.1136/bmj.327.7414.557
40. Egger M, Davey Smith G, Schneider M, Minder C. Bias in meta-analysis detected by a simple, graphical test. BMJ. (1997) 315:629–34. doi: 10.1136/bmj.315.7109.629
41. Hong DM, Lee EH, Kim HJ, Min JJ, Chin JH, Choi DK, et al. Does remote ischaemic preconditioning with postconditioning improve clinical outcomes of patients undergoing cardiac surgery? Remote ischaemic preconditioning with postconditioning outcome trial. Eur Heart J. (2014) 35:176–83. doi: 10.1093/eurheartj/eht346
42. Min JJ, Bae JY, Kim TK, Kim JH, Hwang HY, Kim KH, et al. Pulmonary protective effects of remote ischaemic preconditioning with postconditioning in patients undergoing cardiac surgery involving cardiopulmonary bypass: a substudy of the remote ischaemic preconditioning with postconditioning outcome trial. Heart Lung Circ. (2016) 25:484–92. doi: 10.1016/j.hlc.2015.09.008
43. Yang L, Wang G, Du Y, Ji B, Zheng Z. Remote ischemic preconditioning reduces cardiac troponin I release in cardiac surgery: a meta-analysis. J Cardiothorac Vasc Anesth. (2014) 28:682–9. doi: 10.1053/j.jvca.2013.05.035
44. Kumar A, Singh H, Shariff M. Remote ischemic preconditioning and its role in the prevention of new onset atrial fibrillation post-cardiac surgery. A meta-analysis of randomized control trials. J Arrhythm. (2019) 35:789–94. doi: 10.1002/joa3.12252
45. Chen K, Yu J, Wang Q, Wu L, Liu X, Wong GTC, et al. The timing of propofol administration affects the effectiveness of remote ischemic preconditioning induced cardioprotection in rats. J Cell Biochem. (2020) 121:4535–41. doi: 10.1002/jcb.29671
46. Mastitskaya S, Marina N, Gourine A, Gilbey MP, Spyer KM, Teschemacher AG, et al. Cardioprotection evoked by remote ischaemic preconditioning is critically dependent on the activity of vagal pre-ganglionic neurones. Cardiovasc Res. (2012) 95:487–94. doi: 10.1093/cvr/cvs212
47. Randhawa PK, Bali A, Jaggi AS. RIPC for multiorgan salvage in clinical settings: evolution of concept, evidences and mechanisms. Eur J Pharmacol. (2015) 746:317–32. doi: 10.1016/j.ejphar.2014.08.016
48. Khaliulin I, Fleishman AN, Shumeiko NI, Korablina T, Petrovskiy SA, Ascione R, et al. Neuro-autonomic changes induced by remote ischemic preconditioning (RIPC) in healthy young adults: implications for stress. Neurobiol Stress. (2019) 11:100189. doi: 10.1016/j.ynstr.2019.100189
49. Donato M, Buchholz B, Rodriguez M, Perez V, Inserte J, Garcia-Dorado D, et al. Role of the parasympathetic nervous system in cardioprotection by remote hindlimb ischaemic preconditioning. Exp Physiol. (2013) 98:425–34. doi: 10.1113/expphysiol.2012.066217
50. Pickard JM, Davidson SM, Hausenloy DJ, Yellon DM. Co-dependence of the neural and humoral pathways in the mechanism of remote ischemic conditioning. Basic Res Cardiol. (2016) 111:50. doi: 10.1007/s00395-016-0568-z
Keywords: remote ischemic preconditioning, acute kidney injury, cardiac surgery, off-pump, meta-analysis
Citation: Liu Z, Zhao Y, Lei M, Zhao G, Li D, Sun R and Liu X (2021) Remote Ischemic Preconditioning to Prevent Acute Kidney Injury After Cardiac Surgery: A Meta-Analysis of Randomized Controlled Trials. Front. Cardiovasc. Med. 8:601470. doi: 10.3389/fcvm.2021.601470
Received: 01 September 2020; Accepted: 15 February 2021;
Published: 18 March 2021.
Edited by:
Sarah Longnus, Bern University Hospital, SwitzerlandReviewed by:
Pradeep Narayan, Rabindranath Tagore International Institute of Cardiac Sciences (RTIICS), IndiaMaria Nieves Sanz, Bern University Hospital, Switzerland
Copyright © 2021 Liu, Zhao, Lei, Zhao, Li, Sun and Liu. This is an open-access article distributed under the terms of the Creative Commons Attribution License (CC BY). The use, distribution or reproduction in other forums is permitted, provided the original author(s) and the copyright owner(s) are credited and that the original publication in this journal is cited, in accordance with accepted academic practice. No use, distribution or reproduction is permitted which does not comply with these terms.
*Correspondence: Zigang Liu, bGl1emlnYW5nZG9jQDE2My5jb20=; Xian Liu, Nzg1NTUyMTQ4QHFxLmNvbQ==
†These authors have contributed equally to this work