- Institute of Physiology at Komi Science Center of the Ural Branch of the Russian Academy of Sciences, Syktyvkar, Russia
The purpose of this study is to assess the production of nitric oxide in professional cross-country skiers with normotensive and hypertensive responses to physical activity at maximum load. The observation group included professional cross-country skiers (22.2 ± 7.1 years, = 107) who were current members of the national team of the Komi Republic. All the examined athletes performed the exercise test on a cycle ergometer “until exhaustion.” The following parameters were determined for each participant while they were sitting at rest, while at their anaerobic threshold level, during peak load, and during the recovery period (5th min): systolic blood pressure, diastolic blood pressure, heart rate, and the level of stable nitric oxide metabolites (nitrites, nitrates) in capillary blood samples. According to the blood pressure results, the cross-country skiers were divided into two groups. Group I included athletes with a normotensive response to stress. Group II was composed of individuals with a hypertensive response to stress. During the performance of the test “until exhaustion,” a significant (p < 0.05) increase in the amount of stable metabolites of nitric oxide was observed in the group of athletes with a normotensive response to the load compared with the group with a hypertensive response to the load. In athletes with a normotensive reaction to the load during exercise at maximum load and in the early recovery period, nitrate was prioritized in the regulation of vascular tone. The exercise test on a cycle ergometer “until exhaustion,” combined with the assessment of the levels of stable nitric oxide metabolites in plasma, can be considered a test for the early diagnosis of endothelial dysfunction in professional athletes.
Introduction
One of the factors limiting the functional capabilities of the bodies of athletes is overstraining of the cardiovascular system (1). Physical exercise leads to a progressive increase in heart rate (HR), which subsequently increases blood flow and vascular shear stress. Nitric oxide (NO), a signaling molecule, plays an important role in the regulation of many physiological functions, including regulating circulation and blood pressure by vasodilation (2, 3). The potential mechanisms through which vascular control may be beneficially modified in response to repeated exposure to shear stress include increased endothelium-dependent dilator capacity (4) and higher production of endothelial NO and endothelial NO synthase (eNOS) expression (5). In athletes, peripheral vasoconstriction during maximal exercise is prevented by improved endothelium-dependent dilator capacity (as indicated by plasma levels of nitrite oxide), which may increase skeletal muscle oxidative capacity during exercise (5). An imbalance in the NO synthesis system and formation of endothelial dysfunction play an important role in the development of cardiovascular pathology. Prolonged and intense aerobic training can result in a decrease in NO bioavailability, which weakens endothelial-dependent vasodilation (6). According to the literature (7), systolic blood pressure (SBP) >200 mmHg and/or diastolic pressure (DBP) >100 mmHg serve as a predictor for the termination of the exercise test “until exhaustion” in professional cross-country skiers.
Impaired NO generation is important in the development of endothelial dysfunction and hypertension (3, 6). Endothelial dysfunction leads to prioritized production of vasoconstrictor substances (tissue AII, endothelin, and thromboxane A2), decreased production of depressant compounds (bradykinin, NO, and prostacyclin), and an inadequate regulatory response of the vascular wall. As a result, endothelium-dependent vasoconstriction prevails over endothelium-dependent vasodilation (8).
Thus, the mechanisms of the development of endothelial dysfunction may vary, and the significance of the contribution of the features of NO synthesis as an early marker remains unresolved. According to the literature (9), SBP increases significantly and proportionally to workload increases during exercise tests in healthy adults, which can be useful in the diagnosis of cardiovascular diseases. In this regard, it can be assumed that the cardiovascular response of the body of a professional athlete to physical load of maximum load may depend not only on the mechanisms of hypertension formation but also on the specific features of NO synthesis.
The purpose of this study was to assess NO production in professional cross-country skiers with normotensive and hypertensive responses to physical activity at maximum load.
Methods
Ethical Approval
The experimental design and protocol of the study were approved by the Ethics Committee of the Institute of Physiology, Russian Academy of Sciences, Syktyvkar. The study conformed to the Code of Ethics of the World Medical Association (Declaration of Helsinki). The participants were given all the information about the experimental protocol, experimental procedures, and possible risks and discomforts associated with performing the exercise test on a cycle ergometer “until exhaustion.” The participants were informed that they were free to withdraw from the study at any time and without consequence. After the required explanations, the volunteers gave their written informed consent for participating in the experiment.
Participants
The observation group included well-trained cross-country skiers (n = 107 males, 22.2 ± 7.1 years old) who were current members of the national team of the Komi Republic, had no signs or history of chronic diseases, and volunteered to participate in this study. All athletes had extensive experience in endurance events and had a minimum of 5 years of cross-country skiing practice as part of their main training schedule.
Experimental Protocol
The study was performed during the morning after a breakfast low in nitrates; therefore, all of the volunteers consumed a standardized meal (400–420 kcal) consisting of (in units of the percentage of the total energy supplied by the entire meal, En%) 78 En% carbohydrate, 14 En% fat, and 8 En% protein the night before the assessment. The participants' dietary intake was assessed using a food frequency questionnaire. Calories consumed at breakfast were not normalized by body weight. The low-nitrate dinner excluded foods and drinks that are the main sources of nitrates in human food (meat and fish products, vegetables (mainly beets, leafy green vegetables), marinades, spirits, fruit, and mineral drinks).
In each of the examined athletes, the following parameters were determined when sitting at rest, at the anaerobic threshold level, during peak load, and during the recovery period (5th min) (Figure 1): SBP, DBP, heart rate (HR), and the level of stable metabolites of NO in capillary blood samples. Blood pressure was measured by the Korotkov method on the right arm. Heart rate data were obtained from test protocols. The pulse pressure (PP) value was calculated as the difference between SBP and DBP. The maximum oxygen consumption per kilogram of body weight (VO2 max/kg) was estimated.
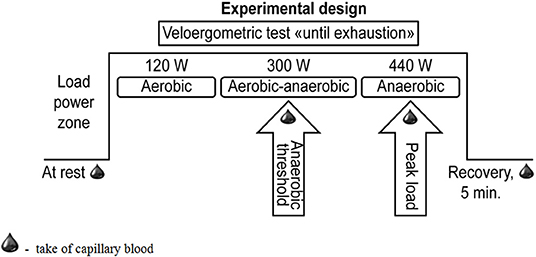
Figure 1. Overview of the study design incorporating the exercise test on a cycle ergometer “until exhaustion”.
According to the blood pressure results, the cross-country skiers were divided into two groups. Group I included athletes with a normotensive response to the load (SBP at a peak load of up to 200 mmHg and/or DBP at a peak load of up to 100 mmHg). Group II was composed of individuals with a hypertensive response to the load (SBP at a peak load of >200 mmHg and/or DBP at a peak load of >100 mmHg). The characteristics of the examined groups of athletes are presented in Table 1.
Exercise Test on a Cycle Ergometer “Until Exhaustion”
Aerobic capacity (VO2 max) testing was performed on an ergometer bike (“Ergoselect-100,” Ergoline GmbH, Germany) in the “breath by breath” mode, and the indicators were averaged over 15-s segments. The protocol included 1 min of cycling without load (for adaptation) followed by stepwise load increases of 40 W in 2-min increments starting with an initial load of 120 W. The pedaling speed was 60 rpm. The anaerobic threshold (AT) was determined by reaching a respiratory coefficient of one (10, 11).
Determination of NOx
The NO levels in the plasma were measured indirectly by evaluating the stable metabolites of NO, including nitrites [NO(2)(–)] and nitrates [NO(3)(–)], which were presorted as an index (NOx) of NO production using the Griess reaction. NO is rapidly converted to nitrite and nitrate, the terminal products of NO in human plasma. A strong correlation between endogenous NO production and NOx levels in plasma has been reported (12). Briefly, each of the examined athletes was collected capillary blood samples when sitting at rest, at the anaerobic threshold level, during peak load, and during the recovery period (5th min). One-milliliter blood samples were collected into tubes with heparin and immediately centrifuged for 20 min at 2,500 g. The separated plasma was aliquoted and stored at −40°C until analysis. Samples were measured twice against a standard nitrite curve with a known concentration. Plasma samples were deproteinized by precipitation in ethanol. After centrifugation, the supernatants were incubated for 30 min at 37°C with vanadium chloride to convert nitrate to nitrite. Next, the samples were mixed with the Griess reagent, and the light absorbance was measured at a wavelength of 540 nm using a Spectronic Genesys-6 Spectrophotometer (Thermo Electron Scientific Instruments LLC, Madison, WI USA). Total nitrite was then measured using the Griess reagent. The plasma nitrate concentration was calculated by subtracting the primary nitrite concentration from the total nitrite concentration. All chemicals used for NO determination were obtained from Sigma (St. Louis, MO, USA). The detection limit for NO was 0.001 μmol/l. The NO3/NO2 index was calculated as the ratio between NO3 and NO2.
Statistical Analysis
Statistical analyses were performed using Statistica software (version 6.0, StatSoft Inc., 2001, USA). Descriptive statistics were used to calculate the mean and standard deviation (SD). Differences in the dynamics of each parameter were tested by Friedman's ANOVA. The significance of variation between the groups was estimated by the Wilcoxon-test. The correlation coefficients between two variables were determined by Spearman rank analysis. A value of p < 0.05 was accepted as statistically significant.
Results
Hemodynamic Parameters
SBP at rest was increased (p < 0.001) in individuals with a hypertensive response to the load compared with the group with a normotensive response to the load. When performing the test “until exhaustion,” all cross-country skiers showed a statistically significant increase in SBP during the passage of the anaerobic threshold (p = 0.001) compared with indicators at rest. During the maximum load (peak load), cross-country skiers continued to increase their SBP compared with the period of passage of the AT. At the level of the AT and peak load, the group that had a hypertensive response to the load was characterized by higher values of SBP (p < 0.001). During the recovery period, SBP-values significantly decreased in both groups (p < 0.001) (Table 2).
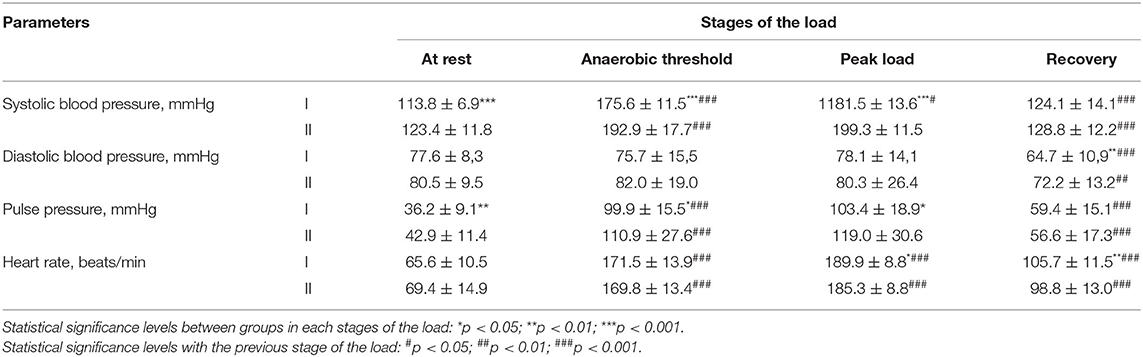
Table 2. Hemodynamic parameters at different stages of the load in professional cross-country skiers, Me ± SD.
DBP-values at rest, AT, and peak load did not significantly change in the study groups. However, during the recovery period, DBP was significantly (p < 0.001–0.01) decreased in both groups. Moreover, the decrease in DBP in the group with a normotensive response to the load was more pronounced than that in the opposite group (p < 0.01).
The dynamics of changes in the PP-value in the two groups were similar; specifically, significant increases during the period of the AT passage and a decrease 5 min after the end of the test were noted (p < 0.001). However, in the group with a hypertensive reaction to the load at rest, AT, and peak load, the value of this indicator was increased compared to a group of people with a normotensive reaction to the load (p < 0.01–0.05).
A significant (p < 0.001) increase in heart rate was found in both groups of cross-country skiers during the passage of the AT of the load compared with indicators at rest. A continued increase in heart rate was noted at the peak of the load compared with the period of passage of the AT (p < 0.001). During this load period, group I was characterized by increased heart rates compared to group II (p < 0.05). During recovery, athletes in the study groups showed a statistically significant decrease in heart rate compared with that noted during the peak load (p < 0.001).
Nitric Oxide Levels
The values of NOx at rest did not exhibit statistically significant differences between the study groups. In subjects with a normotensive response to the load, an increase in NOx was detected during the passage of the AT compared with values at rest (p < 0.05). During periods of peak load and recovery in this group, NOx tended to decrease (Table 3). In the group with a hypertensive reaction to the load during the passage of the AT, the level of NOx in the blood tended to increase. At the peak load and recovery, the group with a normotensive response to the load was characterized by higher values of NOx (p < 0.01).
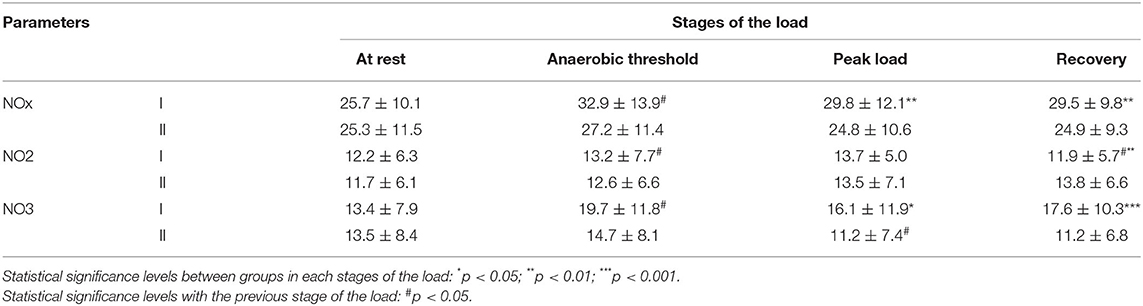
Table 3. Nitric oxide levels at different stages of the load in professional cross-country skiers, Me ± SD (μmol/L).
The NO2 value between the study groups at rest, AT, and peak load did not exhibit statistically significant changes. An increase in NO2 was detected in group I during periods of the AT passage compared with values at rest (p < 0.05). It should be noted that during recovery 5 min after the end of the test, different dynamics of changes in the level of NO2 were observed. An increase in NO2 was detected in group II, while a decrease was noted in group I (p < 0.01).
A statistically significant (p < 0.05) increase in NO3 was noted in group I during the passage of the AT compared with the values at rest. In the group with a hypertensive reaction to the load at the peak of the load, the value of NO3 was lower compared with the values at the AT (p < 0.05). At the same time, at both the peak of the load and recovery timepoints, the value of NO3 was lower in group II compared with group I (p < 0.05–0.001). It should be noted that for the subjects with a normotensive response to the load, an increase in the NO3/NO2 index was observed during the test “until exhaustion” (Figure 2). In the athletes with a hypertensive reaction to the load, a decrease in the NO3/NO2 index was observed during the peak load and during the recovery period.
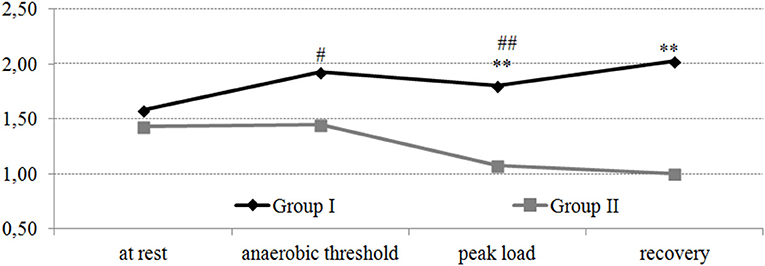
Figure 2. Dynamics of the NO3/NO2 index in professional cross-country skiers during the exercise test on a cycle ergometer “until exhaustion.” Statistical significance levels between groups: *p < 0.05; **p < 0.01; ***p < 0.001. Statistical significance levels between stages of the load: #p < 0.05; ##p < 0.01; ###p < 0.001.
Thus, in athletes with different hemodynamic responses to the load, the opposite dynamics of changes in nitric oxide indicators were noted during the test “until exhaustion.”
Interrelationship of Hemodynamic Parameters and Nitric Oxide Levels
After conducting a correlation analysis in the group with normotensive reactions, a negative relationship between NO3 values and SBP during recovery was revealed (r = −0.38, p < 0.05). At the peak load, positive relationships were observed between DBP and NOx, NO3, and the NO3/NO2 index (r = 0.48, p < 0.01; r = 0.59, p < 0.001; r = 0.53, p < 0.001, respectively). In the group with a normotensive response to the load, negative relationships among NO3, NO3/NO2, and pulse pressure indicators at different stages of the test were revealed. It should be noted that the relationship between heart rate values and indicators of nitric oxide at rest was negative (Table 4).
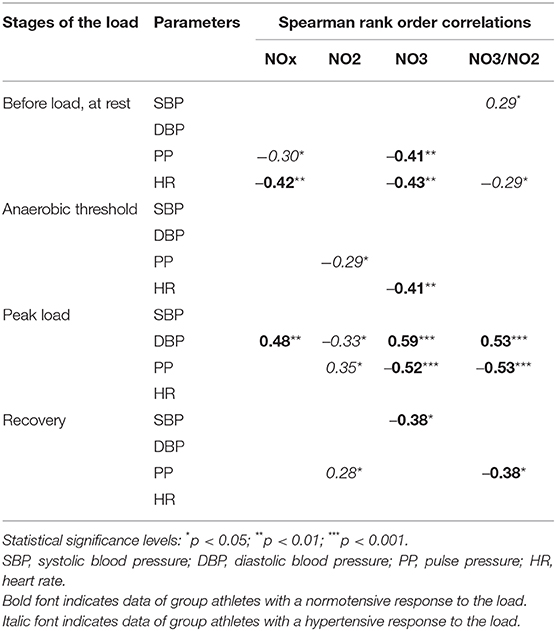
Table 4. Correlations between biochemical and hemodynamic parameters at different stages of the load in professional cross-country skiers in groups I and II.
In the group with a hypertensive reaction to the load, a negative relationship was noted between NO2 and DBP-values during the peak load (r = −0.33, p < 0.05), and a positive correlation was noted between the NO3/NO2 index and SBP at rest. Unlike in group I, a correlation between the PP and the level of NO2 was noted in the group with a hypertensive response to the load; the correlation was negative (r = −0.29, p < 0.05) during the anaerobic threshold and positive at peak load and during recovery (r = 0.35, p < 0.05; r = 0.28, p < 0.05, respectively). In this group, the heart rate indicator showed a correlation only with the NO3/NO2 index at rest (r = −0.29, p < 0.05).
Thus, athletes with a normotensive response to the load revealed more statistically significant and closer relationships between hemodynamic parameters and the level of nitric oxide in the blood. Typically, three indicators (NO, NO3, NO3/NO2) participate in the regulation of vascular tone at the peak of the load; however, only NO2 is involved in individuals with a hypertensive reaction to the load. The priority biochemical indicator is NO3 for athletes from group I and NO2 for athletes from group II.
Discussion
In the athletes examined in this study, the SBP at rest corresponded to the norm, and the DBP at rest was higher than that reported in literature based data obtained from students from a physical education department (64.0 ± 4.7 mmHg) (13). An increase in DBP at rest in both of the groups examined in this study could be associated with prolonged training in open, cold air, which could lead to an increase in peripheral vascular resistance (14). According to the literature (15), even short-term (1 h) cold exposure induces toward hypercoagulation in young healthy people, which can also cause a higher level of DBP. Additionally, cold air can indirectly lead to an increase in cardiovascular risks through its effect on the sympathetic and renin-angiotensin systems, blood pressure, and risk factors for atherosclerosis, such as blood viscosity and the amount of fibrinogen, lipids, and uric acid (16). In general, people who have been in open, cold air for a long time are characterized by increased blood pressure (17), which probably affects the value of SBP during exercise and limits its tolerance.
It is known that blood pressure increases during a load test, and the degree of its growth is proportional to the intensity and severity of the work performed (9). However, according to our data, in athletes with a normotensive response to the load, the power of the performed load was increased compared with that noted in a group of athletes with a hypertensive response to the load. Additionally, in athletes from group II, PP was higher at rest, the AT and peak load. These results also indicate the effect of the hypertensive component.
It is known (18) that in the body of professional athletes under intense and strenuous physical exertion, oxidative stress occurs, leading to the accumulation of lipid peroxidation products, including free radicals. Oxidative stress is the main reason for the decrease in the activity of NO synthase (NOS) through a decrease in the availability of the co-factor NOS-tetrahydrobiopterin and, subsequently, the inhibition of the enzymatic synthesis of NO. With a decrease in the level of NO in the tissues, the adaptive capacity of the body decreases, and pathological changes in metabolism are observed, leading to diseases. There is an opinion that the primary cause of the pathogenesis of coronary heart disease and atherosclerotic vascular damage is a deficiency of NO in the vascular endothelium and myocardium (19). In our study, a decrease in NOx levels was observed during exercise at maximum load, in a group of athletes with a hypertensive response to the load, which may suggest a decrease in the enzymatic synthesis of NO. This finding also confirms the previously obtained information that, in the pathology of the cardiovascular system, a decrease in NO levels in tissues is observed (20).
In particular, in most clinical studies (21–23), in patients with stage II hypertension (HT), a decrease in NOx levels was detected, which is quite common in the context of endothelial dysfunction. Deficiency of endothelial NO can be caused by several factors: a decrease in eNOS activity (21), destruction or capture of NO by free radicals, and/or a weakening of the effect of NO on smooth muscle (22). Reduced vasodilator function of the endothelium is an important link in the pathogenesis of HT. However, in a number of studies on hypertension, an increase in NO metabolites was found, which appeared during tress, humoral system activation, or a more severe course of hypertension (23).
According to our data, the athletes with a hypertensive reaction to the load show a decrease in the level of NO3 in the blood, and NO2 tended to increase during the peak and recovery periods. In these periods of the study, we also revealed correlations among NO2, DBP, and PP. That is, the higher the NO2, the lower the DBP-value and the higher the PP-value. At the same time, there were no correlations between NO3 and hemodynamic parameters.
Until recently, NO2 and NO3 were considered relatively inert intermediate products of NO oxidation, and these molecules are synthesized in the body by the NO synthase enzyme. However, in recent years, unique properties of NO2 have been discovered, making it possible to recognize it as the most important biologically active signaling molecule (24). The significant vasodilator response observed in in vivo and in vitro experiments on the introduction of NO2 solutions suggested that it could be an alternative source of NO (25). Information had also been reported on the involvement of NO2 in the adaptation to physiological hypoxia conditions, such as those caused by physical exertion (26). Modern ideas about nitrite-dependent mechanisms of adaptation to hypoxia are based on data on the involvement of NO2 in oxygen-dependent and hypoxic-dependent nitrite reductase processes. The NO released as a result of these processes is involved in the regulation of vascular tone, modulation of mitochondrial redox reactions (27), changes in the sensitivity of cardiac contractile proteins to oxygen (28) and calcium ions (29), and inhibition of inducible NOS. Due to the cyclic metabolic transformations of NO, NO2, and NO3, the optimal level of NO necessary for the normal functioning of the cardiovascular system under conditions of impaired functioning of NO synthases is maintained. On the other hand, excess NO is removed via the formation of an NO depot in the form of NO2 that protects tissues from oxidative and nitrosative stress. It has now been established that the source of vasoactive NO is also NO2, which is always present in the blood and can be reduced to NO enzymatically, under the influence of xanthine oxidoreductase, and non-enzymatically, under conditions of low pH and pO2 (30). Nitrate is sequentially reduced to nitrite and nitric oxide, which activate soluble guanylate cyclase (31). In particular, exercise has been shown to increase plasma nitrite levels by increasing NO synthesis in endothelial cells (32, 33). Plasma nitrite is gradually oxidized to nitrate, a process that is greatly accelerated by the presence of heme proteins (34). Nitrate is stable in plasma until it is excreted in the urine. Circulating nitrite rather than nitrate reflects endothelial-dependent NO synthesis (35). The increased production of NO during exercise is probably controlled by increasing nitrate excretion as a possible mechanism to control plasmatic homeostasis (36). When oxygen tension decreases, the reduction of nitrite by deoxyhemoglobin produces NO. Erythrocyte NO generation and output, along with the oxygen concentration gradient, could be related to the role of nitrite bound to erythrocytes in vasodilatation processes in response to hypoxia. Thus, it can be assumed that in athletes with a hypertensive response to exercise, NO2 was used as an NO precursor for vasodilation, but it is possible that this compensatory mechanism for regulating cardiovascular tone was insufficient.
Thus, in the group of athletes with a hypertensive reaction to the load, an imbalance was established in the NO synthesis system, which led to a significant increase in blood pressure during exercise at maximum load. The observed imbalance in the NO synthesis system during exercise can subsequently lead to the occurrence of endothelial dysfunction and play an important role in the development of hypertension which will also be registered at rest, and cardiovascular pathology.
Conclusions
At rest, in athletes with normotensive and hypertensive responses to the load, the hemodynamic parameters and the level of stable metabolites of nitric oxide and their amounts in the blood corresponded to generally accepted standards, which was not informative for predicting endothelial dysfunction. However, during the performance of the test “until exhaustion” by athletes in the group with a normotensive response to the load, a significant (p < 0.05) increase in the amount of stable metabolites of nitric oxide was observed compared with the group with a hypertensive response to the load. In cross-country skiers with a normotensive reaction to the load during exercise at maximum load and in the early recovery period, nitrate is primarily involved in the regulation of vascular tone. The development of hypertensive reactions in these athletes during the test was due to nitrate deficiency. Thus, the exercise test on a cycle ergometer “until exhaustion” combined with the assessment of the levels of stable nitric oxide metabolites in plasma can be considered a test for the early diagnosis of endothelial dysfunction in professional athletes.
Data Availability Statement
The raw data supporting the conclusions of this article will be made available by the authors, without undue reservation.
Ethics Statement
The studies involving human participants were reviewed and approved by Ethics Committee of the Institute of Physiology, Russian Academy of Sciences, Syktyvkar. The patients/participants provided their written informed consent to participate in this study.
Author Contributions
OP participated in the experimental procedure, carried out the biochemical studies, performed the statistical analysis, and drafted the manuscript. NV participated in the experimental procedure, involved in data collection, and drafted the manuscript. BE oversaw the experimental procedures, provided coordination, and helped with drafting of the manuscript. All authors have read and approved the final version of the manuscript, agreed with the order of presentation of the authors, and participated in designing the experiment.
Funding
This work was carried out at the expense of the subsidy for the implementation of State task No. GR AAAA-A17-117012310157-7 and No. GR AAAA-A17-117012310153-9.
Conflict of Interest
The authors declare that the research was conducted in the absence of any commercial or financial relationships that could be construed as a potential conflict of interest.
Supplementary Material
The Supplementary Material for this article can be found online at: https://www.frontiersin.org/articles/10.3389/fcvm.2020.582021/full#supplementary-material
References
1. Ihsan M, Watson G, Abbiss CR. What are the physiological mechanisms for post-exercise cold water immersion in the recovery from prolonged endurance and intermittent exercise? Sports Med. (2016) 46:1095–109. doi: 10.1007/s40279-016-0483-3
2. Singel DJ, Stamler JS. Chemical physiology of blood flow regulation by red blood cells: the role of nitric oxide and S-nitrosohemoglobin. Annu Rev Physiol. (2005) 67:99–145. doi: 10.1146/annurev.physiol.67.060603.090918
3. Lewis NCS, Bain AR, Wildfong KW, Green DJ, Ainslie PN. Acute hypoxaemia and vascular function in healthy humans. Exp Physiol. (2017) 102:1635–46. doi: 10.1113/EP086532
4. Celec P, Yonemitsu Y. Vascular endothelial growth factor—basic science and its clinical implications. Pathophysiology. (2004) 11:69–75. doi: 10.1016/j.pathophys.2004.03.002
5. Bailey SJ., Vanhatalo A, Winyard PG, Jones AM. The nitrate-nitrite-nitric oxide pathway: its role in human exercise physiology. Eur J Sport Sci. (2012) 12:4309–20. doi: 10.1080/17461391.2011.635705
6. Bergholm R, Makimattila S, Valkonen M, Liu ML, Lahdenpera S, Taskinen MR, et al. Intense physical training decreases circulating antioxidants and endothelium-dependent vasodilation in vivo. Atherosclerosis. (1999) 145:341–9. doi: 10.1016/S0021-9150(99)00089-1
7. Varlamova NG, Loginova TP, Martynov NA, Chernykh AA, Rastorguev IA, Garnov IO, et al. Cardiorespiratory predictors for maximal load test termination in professional cross-country skiers. Sports Med Sci Pract. (2015) 2:53–60. doi: 10.17238/ISSN2223-2524.2015.2.53
8. Sandoo A, Veldhuijzen van Zanten JJCS, Metsios GS, Carroll D, Kitas GD. The Endothelium and its role in regulating vascular tone. Open Cardiovasc Med J. (2010) 4:302–12. doi: 10.2174/1874192401004010302
9. Wielemborek-Musial K, Szmigielska K, Leszczynska J, Jegier A. Blood pressure response to submaximal exercise test in adults. Biomed Res Int. (2016) 2016:1–8. doi: 10.1155/2016/5607507
10. Dickstein K, Barvik S, Aarsland T, Snapinn S, Karlsson J. A comparison of methodologies in detection of the anaerobic threshold. Circulation. (1990) 81:38–46.
11. Myers J, Ashley E. Dangerous curves. A perspective on exercise, lactate, and the anaerobic threshold. Chest. (1997) 111:787–95. doi: 10.1378/chest.111.3.787
12. Granger DL, Taintor RR, Boockvar KS, Hibbs JB Jr. Measurement of nitrate and nitrite in biological samples using nitrate reductase and Griess reaction. Methods Enzymol. (1996) 268:142–51. doi: 10.1016/S0076-6879(96)68016-1
13. Gjovaag T, Hjelmeland AK, Oygard JB, Vikne H, Mirtaheri P. Acute hemodynamic and cardiovascular responses following resistance exercise to voluntary exhaustion. Effects of different loadings and exercise durations. J Sports Med Phys Fitness. (2016) 56:616–23.
14. Varlamova NG, Zenchenko TA, Boyko ER. Annual blood pressure dynamics and weather sensitivity in women. Tterapevticheskii arkhiv. (2017) 89:56–63. doi: 10.17116/terarkh2017891256-63
15. Mercer JB, Osterud B, Tveita T. The effect of short-term cold exposure on risk factors for cardiovascular diseases from China. Thromb Res. (1999) 95:93–104. doi: 10.1016/S0049-3848(99)00028-6
16. Luo B, Zhang S, Ma S, Zhou J, Wang B. Artificial cold air increases the cardiovascular risks in spontaneously hypertensive rats. Int J Environ Res Public Health. (2012) 9:3197–208. doi: 10.3390/ijerph9093197
17. Evdokimov VG, Rogachevskaya OV, Varlamova NG. The Modulating Influence of the North Factors on Human Cardiorespiratory System in Ontogenesis. Ekaterinburg: Ural Branch of Russian Academy of Sciences (2007).
18. Heitzer T, Krohn K, Albers S, Meinertz T. Tetrahydrobiopterin improves endothelium-dependent vasodilation by increasing nitric oxide activity in patients with type II diabetes mellitus. Diabetologia. (2000) 43:1435–3148. doi: 10.1007/s001250051551
19. Besedina A. NO-synthase activity in patients with coronary heart disease associated with hypertension of different age groups. J Med Biochem. (2016) 35:43–9. doi: 10.1515/jomb-2015-0008
20. Tesauro M, Mauriello A, Rovella V, Annicchiarico-Petruzzelli M, Cardillo C, Melino G, et al. Arterial ageing: from endothelial dysfunction to vascular calcification. J Intern Med. (2017) 281:471–82. doi: 10.1111/joim.12605
21. Chou TC, Yen MH, Li CY, Ding YA. Alterations of nitric oxide synthase expression with aging and hypertension in rats. Hypertension. (1998) 31:643–8. doi: 10.1161/01.HYP.31.2.643
22. Kizub IV, Kharchenko OI, Kostiuk OS, Ostapchenko LI, Soloviev AI. Protein kinase C (PKC) involved in enhancement of alpha(1)-adrenoceptor-mediated responses of the main pulmonary artery in rats with diabetes mellitus. Regul Mech Biosyst. (2017) 8:287–92. doi: 10.15421/021745
23. Bahadoran Z, Mirmiran P, Tahmasebinejad Z, Azizi F, Ghasemi A. Serum nitric oxide metabolites and hard clinical endpoints: a population-based prospective study. Scand Cardiovasc J. (2019) 53:176–82. doi: 10.1080/14017431.2019.1618493
24. Mazzone M, Carmeliet P. Drug discovery: a lifeline for suffocating tissues. Nature. (2008) 453:1194–5. doi: 10.1038/4531194a
25. Schulman IH, Hare JM. Regulation cardiovascular processes by S-nitrosylation. Biochim Biophys Acta. (2012) 1820:752–62. doi: 10.1016/j.bbagen.2011.04.002
26. Gladwin M, Shelhamer J, Schechter A, Pease-Fye M, Waclawei M, Panza J, et al. Role of circulating nitrite and S-nitrosohemoglobin in the regulation of the regional blood floow in humans. Proc Natl Acad Sci USA. (2000) 97:11482–6. doi: 10.1073/pnas.97.21.11482
27. Gladwin MT, Kim-Shapiro DB. The functional nitrite reductase activity of the heme globins. Blood. (2008) 112:2636–47. doi: 10.1182/blood-2008-01-115261
28. Khan SA, Skaf MN, Harrison RW, Lee K, Minhas KM, Kumor A, et al. Nitric oxide regulation of myocardial contractility and calcium cycling. Circ Res. (2003) 92:1322–9. doi: 10.1161/01.RES.0000078171.52542.9E
29. Layland J, Li J, Shah AM. Role cyclic GMP-dependent protein kinase in the contractile response to exogenous nitric oxide in rat cardiac myocytes. J Physiol. (2002) 540:457–67. doi: 10.1113/jphysiol.2001.014126
30. Godber BL, Doel JJ, Sapkota GP, Blake DR, Stevens CR, Eisenthal R, et al. Reduction of nitrite to nitric oxide catalyzed by xanthine oxidoreductase. J Biol Chem. (2000) 275:7757–63. doi: 10.1074/jbc.275.11.7757
31. Zebrowska A, Mizia-Stec K, Mizia M, Gasiorb Z, Poprzecki S. Omega-3 fatty acids supplementation improves endothelial function and maximal oxygen uptake in endurance-trained athletes. Eur J Sport Sci. (2015) 15:305–14. doi: 10.1080/17461391.2014.949310
32. Wang JS, Chow SE, Chen JK, Strenuous acute exercise a Vects reciprocal modulation of platelet and polymorphonuclear leukocyte activities under shear Xow in men. J Thromb Haemost. (2003) 1:2031–7. doi: 10.1046/j.1538-7836.2003.00350.x
33. Delp MD, Laughlin MH. Time course of enhanced endothelium mediated dilation in aorta of trained rats. Med Sci Sports Exerc. (1997) 29:1454–61. doi: 10.1097/00005768-199711000-00011
34. Allen JD, Cobb FR, Gow AJ. Regional and whole-body markers of nitric oxide production following hyperemic stimuli. Free Radic Biol Med. (2005) 38:1164–69. doi: 10.1016/j.freeradbiomed.2004.12.018
35. Kleinbongard P, Dejam A, Lauer T, Rassaf T, Picker SAO, Scheeren T, et al. Plasma nitrite resects constitutive nitric oxide synthase activity in mammals. Free Radic Biol Med. (2003) 35:790–6. doi: 10.1016/S0891-5849(03)00406-4
Keywords: nitric oxide, cardiovascular system, endothelial dysfunction, exercise test on a cycle ergometer, cross-country skiers
Citation: Parshukova OI, Varlamova NG and Bojko ER (2020) Nitric Oxide Production in Professional Skiers During Physical Activity at Maximum Load. Front. Cardiovasc. Med. 7:582021. doi: 10.3389/fcvm.2020.582021
Received: 10 July 2020; Accepted: 13 November 2020;
Published: 14 December 2020.
Edited by:
Djuro Kosanovic, I. M. Sechenov First Moscow State Medical University, RussiaReviewed by:
Tharmarajan Ramprasath, Georgia State University, United StatesAmin Shah, The University of Texas Rio Grande Valley, United States
Copyright © 2020 Parshukova, Varlamova and Bojko. This is an open-access article distributed under the terms of the Creative Commons Attribution License (CC BY). The use, distribution or reproduction in other forums is permitted, provided the original author(s) and the copyright owner(s) are credited and that the original publication in this journal is cited, in accordance with accepted academic practice. No use, distribution or reproduction is permitted which does not comply with these terms.
*Correspondence: Olga I. Parshukova, b2xnYS1wYXJzaHVrb3ZhJiN4MDAwNDA7bWFpbC5ydQ== orcid.org/0000-0003-1862-6936