- 1School of Physiology, Pharmacology and Neuroscience, University of Bristol, Bristol, United Kingdom
- 2Liverpool Heart and Chest Hospital NHS Foundation Trust, Liverpool, United Kingdom
- 3Gloucestershire Hospitals NHS Foundation Trust, Gloucester, United Kingdom
The COVID-19 pandemic is an unprecedented challenge and will require novel therapeutic strategies. Affected patients are likely to be at risk of arrhythmia due to underlying comorbidities, polypharmacy and the disease process. Importantly, a number of the medications likely to receive significant use can themselves, particularly in combination, be pro-arrhythmic. Drug-induced prolongation of the QT interval is primarily caused by inhibition of the hERG potassium channel either directly and/or by impaired channel trafficking. Concurrent use of multiple hERG-blocking drugs may have a synergistic rather than additive effect which, in addition to any pre-existing polypharmacy, critical illness or electrolyte imbalance, may significantly increase the risk of arrhythmia and Torsades de Pointes. Knowledge of these risks will allow informed decisions regarding appropriate therapeutics and monitoring to keep our patients safe.
Introduction
The novel coronavirus disease of 2019 (COVID-19) pandemic is uncharted territory for clinicians and healthcare systems alike. The potential for a high volume of severely unwell patients creates a significant challenge. In particular, the combination of severe infection, respiratory dysfunction, sepsis, shock, and/or haemodynamic instability (1–3) present significant potential for myocardial injury and potentially dangerous arrhythmia. Not only do these pathophysiological states promote the formation of arrhythmia, but unstable arrhythmia could present a significant threat to this cohort of patients who are at risk of haemodynamic instability.
Due to the potential strain on healthcare systems, it is likely that non-specialists will be involved in the management of COVID-19 patients including those experiencing arrhythmia. Similarly, clinicians may face situations where they are using multiple concurrent medications with which they may not be entirely familiar. Here, we attempt to summarize how the use of multiple different types of medication could contribute (synergistically, in some instances) to increase arrhythmic risk. In particular, we focus on the risk of drug-induced QT interval prolongation (commonly referred to as acquired long QT syndrome; aLQTS) which is a serious issue for many of the medications which are likely to be used.
While the use of drugs known to prolong the QT interval may well be necessary and unavoidable, an awareness and understanding of this risk should guide additional safety measures such as monitoring of the corrected QT interval (QTc) on 12-lead electrocardiogram (ECG) measurement. For cases of a prolonged QT interval there is a risk, particularly with a rate-corrected QT interval (QTc) of >500 ms (4), of dangerous arrhythmia including Torsades de Pointes (TdP); close monitoring and involvement of specialist cardiology input should be sought, as well as minimizing, where possible, the use of other known QT-prolonging medication.
Increased Baseline Arrhythmic Risk in COVID-19 Patients
From the data available, COVID-19 seems to cause more serious disease in older patients and those with comorbidities (1–3, 5–7). Zhou et al. (3) described hypertension, diabetes and coronary heart disease as the three most prevalent comorbidities in COVID-19 patients from two hospitals at the epicenter of the initial outbreak in Wuhan. Not only were these comorbidities associated with a significantly worse outcome, they are also, in combination with advanced age, significant risk factors for arrhythmia. Increasing age and co-morbidities also increase the likelihood of pre-existing polypharmacy, which may prove problematic in the context of additional potentially QT-prolonging medication. Unfortunately, published data of COVID-19 patient cohorts to date do not seem to include any ECG or specific arrhythmia data, although no doubt we will gain a better picture as our understanding of the disease and its management evolves.
Increased Arrhythmic Risk of COVID-19 Infection
SARS-CoV-2 is thought to gain entry to human host cells via the angiotensin converting enzyme 2 (ACE2) receptor, which is highly expressed in the heart and lungs and to which the viral spike protein has high affinity (8, 9). Interestingly, data during the previous SARS-CoV outbreak demonstrated ACE2-dependent cardiac infection and inflammation in both mouse and human hearts (10). The down-regulation of affected ACE2 receptors was suggested as a potential contributory factor to SARS-associated myocarditis and subsequent cardiomyopathy, with inflammation and fibrosis likely to provide a substrate for arrhythmia.
The risk of arrhythmia is likely to increase with the development of significant infection, and increase as the severity of the infection and/or systemic inflammatory response increases (11). Significant myocardial damage and fulminant myocarditis have been described (2, 3, 5), and cardiac arrest associated with ventricular arrhythmia (as well as non-shockable rhythms) is reported (7). Du et al. reported some form of arrhythmia present in 60% of a group of fatal cases, with cardiac arrest or malignant arrhythmia listed as the cause of death for over 10% of cases (12).
There is increasing awareness of the development in severely unwell patients of a hyperinflammatory state or cytokine storm (13) which can lead to multi-organ failure. Recent work strongly suggests this hypercytokinemia (in particular elevated levels of interleukin-6) further increases arrhythmic risk via multiple mechanisms, including, notably, hERG blockade (14) and QT prolongation (15, 16). Myocarditis itself is a heterogenous condition associated with a number of arrhythmic states including bradyarrhythmia and atrial or ventricular tachyarrhythmia (17). Data are urgently needed to describe the unique arrhythmic challenges of COVID-19 myocarditis.
Additionally, the multiple medications likely to receive significant use (antibiotics, anesthetic agents, anti-arrhythmic agents, and potentially specific agents to target COVID-19 such as anti-malarial or anti-viral medications) may indeed contribute to a pro-arrhythmic state in a patient group already at risk. Importantly, the additional risk of QT prolongation with some potential combinations of these medications may be synergistic rather than simply additive, due largely to their unique mechanisms of ion channel blockade. Finally, significant electrolyte disturbances, common in unwell patients, will further exacerbate arrhythmic risk (18).
Clinical Management of COVID-19: Significant Potential for hERG Blockade and QT Prolongation
The hERG (or “Kv11.1”) potassium channel (encoded by human Ether-à-go-go Related Gene; alternative nomenclature KCNH2) mediates the rapid component of cardiac delayed rectifier K+ current (also known as IKr). Briefly, this channel is crucial to the repolarization phase of the cardiac action potential; we recommend an excellent review article (19). Inhibition of hERG is considered to be the most common and important mechanism of drug-induced QT prolongation (20, 21) and occurs through direct pharmacological channel block and/or impaired trafficking of hERG channels to the cell membrane. Consequently, hERG testing is a requirement during novel drug development: indeed, prolongation of the QT interval (and its association with dangerous TdP) has been the biggest cause of restriction or withdrawal of drugs already on the market (21–23).
Importantly, recent data including work from our group suggests that the effect on hERG (and thus on QT-prolongation) of multiple drugs may not be simply additive but synergistic (24, 25) (i.e., an effect in excess of the sum of their individual parts). This is particularly relevant when considering an older patient group who may already be taking hERG-blocking medication. In particular, the antimalarial medications chloroquine and hydroxychloroquine (26, 27) are receiving particular attention as antiviral agents: these drugs are multichannel blockers with particular effects on hERG and Kir2.1 and are likely to cause significant QT prolongation at the concentrations effective against SARS-CoV-2 in vitro (28–30), especially when combined with other antivirals (such as lopinavir/ritonavir) or antibiotics (macrolides and fluroquinolones being particularly notable) (26). Further information should be sought regarding novel antiviral agents (31) currently undergoing clinical trials; for example, favipiravir has been associated with QT interval prolongation in a case report (32) and remdesivir awaits comprehensive testing.
Combination therapy with azithromycin and hydroxychloroquine is undergoing testing at present (33, 34). Notably, part of a Brazilian study comparing low vs. high dose chloroquine, in combination with ceftriaxone and azithromycin with or without oseltamivir, was terminated early due to safety concerns; with 25% of those in the high-dose arm showing QT prolongation (vs. 11% in the low-dose arm) and two patients in the high-dose arm experiencing ventricular tachycardia prior to death (35). Similarly, the head of a French cardiology unit reported they had prematurely terminated their hydroxychloroquine-azithromycin COVID-19 trial due to unacceptable QT prolongation (36). The randomized DisCoVeRy (NCT04315948), SOLIDARITY (EudraCT Number 2020-000982-18), and RECOVERY (EudraCT Number 2020-001113-21) studies will provide important evidence regarding the effectiveness and safety of various antiviral and antimalarial drugs in the treatment of COVID-19 patients (37).
Online resources can be consulted to clarify which drugs are associated with QT prolongation (see www.crediblemeds.org). Table 1 lists medications associated with QT prolongation which may be commonly used in the management of COVID-19 [as taken from the updated WHO management guidance (38) with additions from authors' clinical experience from UK practice]. The list is not exhaustive but attempts to highlight potential areas of risk when using these medications, as well as their use in combination.
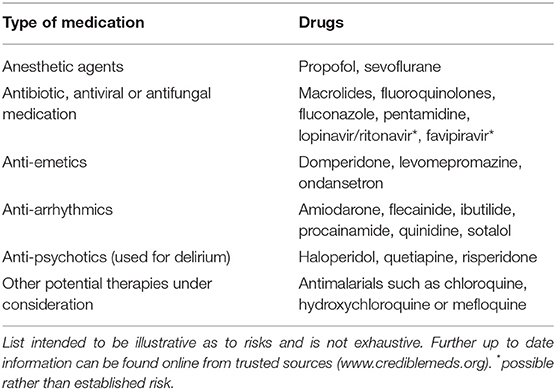
Table 1. A list of medications which could be used in the management of COVID-19 which are also associated with risk of QT prolongation.
Discussion
The recent publication by Ackerman et al. of urgent guidance and a practical flow-chart regarding safe use of QT-prolonging medication is very welcome, and should be consulted as an aid to manage risk in the setting of QT prolongation (39), as should the Heart Rhythm Society (HRS) Task Force update (40). Other resources provide valuable guidance for clinicians dealing with specific patient populations: those with congenital heart disease (41) or inherited arrhythmia syndromes (42). Of note, a case report has reported significant QT prolongation (620 ms) in a patient with COVID-19 treated with multiple hERG blocking medications (including levofloxacin, hydroxychloroquine and azithromycin), which was successfully managed with drug cessation and intravenous lignocaine (43). Separately, mexiletine has also been suggested to be effective in treating TdP associated with acquired long QT syndrome (44).
COVID-19 represents a step into the unknown: not only are we grappling to come to terms with effective management of this new disease, but so too with safe use of these treatments. Effective therapy will be welcome, but the use of multiple drugs in combination has to be exercised with caution as it may increase the risk of QT prolongation and Torsades de Pointes, largely via pharmacological hERG blockade. Knowledge of this risk enables clinicians to ensure adequate monitoring of the QT interval and management of arrhythmic risk, maximizing safety for our patients in this challenging time.
Author Contributions
All authors contributed to the concept, planning and writing of this mini-review, and approved the manuscript prior to submission.
Funding
AC receives an MRC Clinical Research Training Fellowship for (MR/S021299/1) unrelated work. OH receives a BHF Clinical Research Training Fellowship (FS/19/44/34424) for unrelated hERG work.
Conflict of Interest
The handling editor is currently organizing a Research Topic with one of the authors AJ, and confirms the absence of any other collaboration.
The remaining authors declare that the research was conducted in the absence of any commercial or financial relationships that could be construed as a potential conflict of interest.
References
1. Guan W, Ni Z, Hu Y, Liang W, Ou C, He J, et al. Clinical characteristics of coronavirus disease 2019 in China. N Engl J Med. (2020). doi: 10.1101/2020.02.06.20020974. [Epub ahead of print].
2. Yang X, Yu Y, Xu J, Shu H, Xia A, Liu H, et al. Clinical course and outcomes of critically ill patients with SARS-CoV-2 pneumonia in Wuhan, China: a single-centered, retrospective, observational study. Lancet Respir. (2020). doi: 10.1016/S2213-2600(20)30079-5. [Epub ahead of print].
3. Zhou F, Yu T, Du R, Fan G, Liu Y, Liu Z, et al. Clinical course and risk factors for mortality of adult inpatients with COVID-19 in Wuhan, China: a retrospective cohort study. Lancet. (2020). doi: 10.1016/S0140-6736(20)30566-3. [Epub ahead of print].
4. Gibbs C, Thalamus J, Kristoffersen DT, Svendsen MV, Holla ØL, Heldal K, et al. QT prolongation predicts short-term mortality independent of comorbidity. Europace. (201) 21:1254–60. doi: 10.1093/europace/euz058
5. Ruan Q, Yang K, Wang W, Jiang L, Song J. Clinical predictors of mortality due to COVID-19 based on an analysis of data of 150 patients from Wuhan, China. Intens Care Med. (2020) 1–3. doi: 10.1007/s00134-020-05991-x. [Epub ahead of print].
6. Young BE, Ong SWX, Kalimuddin S, Low JG, Tan SY, Loh J, et al. Epidemiologic features and clinical course of patients infected with SARS-CoV-2 in Singapore. JAMA. (2020) 323:1488–94. doi: 10.1001/jama.2020.3204
7. Chen N, Zhou M, Dong X, Qu J, Gong F, Han Y, et al. Epidemiological and clinical characteristics of 99 cases of 2019 novel coronavirus pneumonia in Wuhan, China: a descriptive study. Lancet. (2020) 395:507–13. doi: 10.1016/S0140-6736(20)30211-7
8. Zhang H, Penninger JM, Li Y, Zhong N, Slutsky AS. Angiotensin-converting enzyme 2 (ACE2) as a SARS-CoV-2 receptor: molecular mechanisms and potential therapeutic target. Intens Care Med. (2020) 46:586–90. doi: 10.1007/s00134-020-05985-9
9. Crackower MA, Sarao R, Oliveira-dos-Santos AJ, Da Costa J, Zhang L. Angiotensin-converting enzyme 2 is an essential regulator of heart function. Nature. (2002) 417:822–8. doi: 10.1038/nature00786
10. Oudit GY, Kassiri Z, Jiang C, Liu PP, Poutanen SM, Penninger JM, et al. SARS-coronavirus modulation of myocardial ACE2 expression and inflammation in patients with SARS. Eur J Clin Invest. (2009) 39:618–25. doi: 10.1111/j.1365-2362.2009.02153.x
11. Shahreyar M, Fahhoum R, Akinseye O, Bhandari S, Dang G, Khouzam RN. Severe sepsis and cardiac arrhythmias. Ann Transl Med. (2018) 6:6. doi: 10.21037/atm.2017.12.26
12. Du Y, Tu L, Zhu P, Mu M, Wang R, Yang P, et al. Clinical features of 85 fatal cases of COVID-19 from Wuhan: a retrospective observational study. Am J Respir Crit Care Med. (2020). doi: 10.1164/rccm.202003-0543OC. [Epub ahead of print].
13. Mehta P, McAuley DF, Brown M, Sanchez E, Tattersall RS, Manson JJ. COVID-19: consider cytokine storm syndromes and immunosuppression. Lancet. (2020) 395:1033–4. doi: 10.1016/S0140-6736(20)30628-0
14. Aromolaran AS, Srivastava U, Alí A, Chahine M, Lazaro D, El-Sherif N, et al. Interleukin-6 inhibition of hERG underlies risk for acquired long QT in cardiac and systemic inflammation. PLoS ONE. (2018) 13:e0208321. doi: 10.1371/journal.pone.0208321
15. Lazzerini PE, Boutjdir M, Capecchi PL. COVID-19, arrhythmic risk and inflammation: mind the gap! Circulation. (2020). doi: 10.1161/CIRCULATIONAHA.120.047293. [Epub ahead of print].
16. Lazzerini PE, Laghi-Pasini F, Boutjdir M, Capecchi PL. Cardioimmunology of arrhythmias: the role of autoimmune and inflammatory cardiac channelopathies. Nat Rev Immunol. (2019) 19:63–4. doi: 10.1038/s41577-018-0098-z
17. Peretto G, Sala S, Rizzo S, De Luca G, Campochiaro C, Sartorelli S, et al. Arrhythmias in myocarditis: state of the art. Heart Rhythm. (2019) 16:793–801. doi: 10.1016/j.hrthm.2018.11.024
18. Zeltser D, Justo D, Halkin A, Prokhorov V, Heller K, Viskin S. Torsade de Pointes due to noncardiac drugs. Medicine. (2003) 82:282–90. doi: 10.1097/01.md.0000085057.63483.9b
19. Vandenberg JI, Perry MD, Perrin MJ, Mann SA, Ke Y, Hill AP. hERG K + channels: structure, function, and clinical significance. Physiol Rev. (2012) 92:1393–478. doi: 10.1152/physrev.00036.2011
20. Recanatini M, Poluzzi E, Masetti M, Cavalli A, De Ponti F. QT prolongation through hERG K+ channel blockade: current knowledge and strategies for the early prediction during drug development. Med Res Rev. (2005) 25:133–66. doi: 10.1002/med.20019
21. Hancox JC, McPate MJ, El Harchi A, Zhang Y. The hERG potassium channel and hERG screening for drug-induced torsades de pointes. Pharmacol Ther. (2008) 119:118–32. doi: 10.1016/j.pharmthera.2008.05.009
22. Lasser KE, Allen PD, Woolhandler SJ, Himmelstein DU, Wolfe SM, Bor DH. Timing of new black box warnings and withdrawals for prescription medications. J Am Med Assoc. (2002) 287:2215–20. doi: 10.1001/jama.287.17.2215
23. Roden DM. Drug-induced prolongation of the QT interval. N Engl J Med. (2004) 350:1013–22. doi: 10.1056/NEJMra032426
24. Wiśniowska B, Lisowski B, Kulig M, Polak S. Drug interaction at hERG channel: In vitro assessment of the electrophysiological consequences of drug combinations and comparison against theoretical models. J Appl Toxicol. (2018) 38:450–8. doi: 10.1002/jat.3552
25. El Harchi A, Butler AS, Zhang Y, Dempsey CE, Hancox JC. The macrolide drug erythromycin does not protect the hERG channel from inhibition by thioridazine and terfenadine. Physiol Rep. (2020) 8:e14385. doi: 10.14814/phy2.14385
26. Cortegiani A, Ingoglia G, Ippolito M, Giarratano A, Einav S. A systematic review on the efficacy and safety of chloroquine for the treatment of COVID-19. J Crit Care. (2020). doi: 10.1016/j.jcrc.2020.03.005. [Epub ahead of print].
27. Colson P, Rolain J-M, Lagier J-C, Brouqui P, Raoult D. Chloroquine and hydroxychloroquine as available weapons to fight COVID-19. Int J Antimicrob Agents. (2020) 105932. doi: 10.1016/j.ijantimicag.2020.105932. [Epub ahead of print].
28. Rodríguez-Menchaca AA, Navarro-Polanco RA, Ferrer-Villada T, Rupp J, Sachse FB, Tristani-Firouzi M, et al. The molecular basis of chloroquine block of the inward rectifier Kir2.1 channel. Proc Natl Acad Sci USA. (2008) 105:1364–8. doi: 10.1073/pnas.0708153105
29. Traebert M, Dumotier B, Meister L, Hoffmann P, Dominguez-Estevez M, Suter W. Inhibition of hERG K+ currents by antimalarial drugs in stably transfected HEK293 cells. Eur J Pharmacol. (2004) 484:41–8. doi: 10.1016/j.ejphar.2003.11.003
30. Vincent MJ, Bergeron E, Benjannet S, Erickson BR, Rollin PE, Ksiazek TG, et al. Chloroquine is a potent inhibitor of SARS coronavirus infection and spread. Virol J. (2005) 2:69. doi: 10.1186/1743-422X-2-69
31. Dong L, Hu S, Gao J. Discovering drugs to treat coronavirus disease 2019 (COVID-19). Drug Discov Ther. (2020) 14:58–60. doi: 10.5582/ddt.2020.01012
32. Chinello P, Petrosillo N, Pittalis S, Biava G, Ippolito G, Nicastri E. QTc interval prolongation during favipiravir therapy in an Ebolavirus-infected patient. PLoS Negl Trop Dis. (2017) 11:e0006034. doi: 10.1371/journal.pntd.0006034
33. Gautret P, Lagier J-C, Parola P, Hoang VT, Meddeb L, Mailhe M, et al. Hydroxychloroquine and azithromycin as a treatment of COVID-19: results of an open-label non-randomized clinical trial. Int J Antimicrob Agents. (2020) 105949. doi: 10.1016/j.ijantimicag.2020.105949. [Epub ahead of print].
34. Molina JM, Delaugerre C, Le Goff J, Mela-Lima B, Ponscarme D, Goldwirt L, et al. No evidence of rapid antiviral clearance or clinical benefit with the combination of hydroxychloroquine and azithromycin in patients with severe COVID-19 infection. Médecine Mal Infect. (2020). doi: 10.1016/j.medmal.2020.03.006. [Epub ahead of print].
35. Borba M, de Val FA, Sampaio VS, Alexandre MA, Melo GC, Brito M, et al. Chloroquine diphosphate in two different dosages as adjunctive therapy of hospitalized patients with severe respiratory syndrome in the context of coronavirus (SARS-CoV-2) infection: preliminary safety results of a randomized, double-blinded, phase IIb cl. medRxiv. (2020). doi: 10.1101/2020.04.07.20056424. [Epub ahead of print].
36. NEWSWEEK. French Hospital Stops Hydroxychloroquine Treatment for COVID-19 Patient Over Major Cardiac Risk. Available online at: https://www.newsweek.com/hydroxychloroquine-coronavirus-france-heart-cardiac-1496810 (accessed April 19, 2020).
37. Taccone FS, Gorham J, Vincent J-L. Hydroxychloroquine in the management of critically ill patients with COVID-19: the need for an evidence base. Lancet. (2020) doi: 10.1016/S2213-2600(20)30172-7. [Epub ahead of print].
38. World Health Organization. Clinical Management of Severe Acute Respiratory Infection (SARI) When COVID-19 Disease Is Suspected: Interim Guidance (2020).
39. Giudicessi JR, Noseworthy PA, Friedman PA, Ackerman MJ. Urgent guidance for navigating and circumventing the QTc-prolonging and torsadogenic potential of possible pharmacotherapies for Coronavirus Disease 19 (COVID-19). Mayo Clin Proc. (2020). doi: 10.1016/j.mayocp.2020.03.024. [Epub ahead of print].
40. Heart Rhythm Society. HRS COVID-19 Task Force Update. Heart Rhythm Society. Available online at: https://www.hrsonline.org/COVID19-Challenges-Solutions/hrs-covid-19-task-force-message-qtc-guidance (accessed April 16, 2020).
41. Tan W, Aboulhosn J. The cardiovascular burden of coronavirus disease 2019 (COVID-19) with a focus on congenital heart disease. Int J Cardiol. (2020). doi: 10.1016/j.ijcard.2020.03.063. [Epub ahead of print].
42. Wu C-I, Postema PG, Arbelo E, Behr ER, Bezzina CR, Napolitano C, et al. SARS-CoV-2, COVID-19 and inherited arrhythmia syndromes. Heart Rhythm. (2020). doi: 10.1016/j.hrthm.2020.03.024. [Epub ahead of print].
43. Mitra RL, Greenstein SA, Epstein LM. An algorithm for managing QT prolongation in Coronavirus Disease 2019 (COVID-19) patients treated with either chloroquine or hydroxychloroquine in conjunction with azithromycin: possible benefits of intravenous lidocaine. Heart Case Rep. (2020). doi: 10.1016/j.hrcr.2020.03.016. [Epub ahead of print].
Keywords: COVID-19, QT, arrhythmia, hERG, drug safety, QTc
Citation: Carpenter A, Chambers OJ, El Harchi A, Bond R, Hanington O, Harmer SC, Hancox JC and James AF (2020) COVID-19 Management and Arrhythmia: Risks and Challenges for Clinicians Treating Patients Affected by SARS-CoV-2. Front. Cardiovasc. Med. 7:85. doi: 10.3389/fcvm.2020.00085
Received: 25 March 2020; Accepted: 20 April 2020;
Published: 05 May 2020.
Edited by:
Hendrik Tevaearai Stahel, Bern University Hospital, SwitzerlandReviewed by:
Antonio Sorgente, Cleveland Clinic Abu Dhabi, United Arab EmiratesMarina Cerrone, New York University, United States
Copyright © 2020 Carpenter, Chambers, El Harchi, Bond, Hanington, Harmer, Hancox and James. This is an open-access article distributed under the terms of the Creative Commons Attribution License (CC BY). The use, distribution or reproduction in other forums is permitted, provided the original author(s) and the copyright owner(s) are credited and that the original publication in this journal is cited, in accordance with accepted academic practice. No use, distribution or reproduction is permitted which does not comply with these terms.
*Correspondence: Alexander Carpenter, YWxleGFuZGVyLmNhcnBlbnRlckBicmlzdG9sLmFjLnVr