- Department of Physiology and Cell Biology, Dorothy M. Davis Heart and Lung Research Institute, The Ohio State University Wexner Medical Center, Columbus, OH, United States
Obesity is a complex disease that affects whole body metabolism and is associated with an increased risk of cardiovascular disease (CVD) and Type 2 diabetes (T2D). Physical exercise results in numerous health benefits and is an important tool to combat obesity and its co-morbidities, including cardiovascular disease. Exercise prevents both the onset and development of cardiovascular disease and is an important therapeutic tool to improve outcomes for patients with cardiovascular disease. Some benefits of exercise include enhanced mitochondrial function, restoration and improvement of vasculature, and the release of myokines from skeletal muscle that preserve or augment cardiovascular function. In this review we will discuss the mechanisms through which exercise promotes cardiovascular health.
Introduction
Obesity and its associated co-morbidities are increasing at rapid rates across the United States and worldwide (1). Obesity is associated with many adverse health effects, including increased risks of cardiovascular disease (CVD), type 2 diabetes (T2D), certain cancers, and death (2–6). As obesity rates continue to rise, the prevalence of associated comorbidities including T2D and CVD increase concomitantly (7); overweight people are twice as likely, and severely obese people are ten times more likely to develop cardiovascular diseases than individuals of a healthy weight (8).
Regular physical exercise has several beneficial effects on overall health. While decreasing body mass and adiposity are not the primary outcomes of exercise, exercise can mediate several diseases that accompany obesity including T2D and CVD (9–14). Several recent studies have shown that sustained physical activity is associated with decreased markers of inflammation, improved metabolic health, decreased risk of heart failure, and improved overall survival (15–17). Exercise improves overall metabolic health and reduces the development of T2D (18) by improving glucose tolerance (19), insulin sensitivity (20), and decreasing circulating lipid concentrations (21). This occurs primarily through adaptations to the skeletal muscle, liver, and adipose tissue (16, 22, 23). Physical exercise can also improve cardiovascular function through adaptations to the heart and vascular system (17, 24–27). Regular physical exercise decreases resting heart rate, blood pressure, and atherogenic markers, and increases physiological cardiac hypertrophy (13–15, 28). Exercise improves myocardial perfusion and increases high-density lipoprotein (HDL) cholesterol levels, all of which reduce stress on the heart and improve cardiovascular function in healthy and diseased individuals (11, 15, 29, 30). Given the increasing interest in exercise-based therapies, we will discuss the benefits of exercise on cardiovascular health and the potential mechanisms through which they occur.
Cardiovascular Disease
Cardiovascular disease (CVD) is the leading cause of morbidity and mortality worldwide (31, 32). Almost half of all adults in the United States have at least one key risk factor for development of CVD (i.e., high blood pressure, high cholesterol, or smoking) (33). CVD encompasses a wide range of conditions that affect the heart and vasculature including arrhythmias, dilated, hypertrophic, or idiopathic cardiomyopathies, heart failure and atherosclerosis (34, 35). These conditions can lead to potentially fatal cardiac events such as stroke, myocardial infarction (heart attack), or cardiac arrest (31, 36). Thus, determining various therapeutic tools to prevent or reduce the incidence of CVD is vital.
Although cardiovascular disease can arise in response to multiple factors, the prevalence of obesity-related CVD is rapidly increasing (8). This can occur for several reasons, one being that a high fat diet or obesity can lead to hypertension. In obesity, angiotensin II and aldosterone secretion from abdominal subcutaneous adipose tissue drives activation of the renin-angiotensin system (37–41). Angiotensin II induces vasoconstriction in arterioles, causing arteriolar resistance and increased systemic blood pressure, in addition to stimulating the release of anti-diuretic hormone, which increases water reabsorption in the kidneys. Aldosterone increases the reabsorption of water and sodium into the blood, resulting in increased extracellular fluid volume, thus increasing blood pressure. The renin-angiotensin system also affects the sympathetic nervous system through inhibition of norepinephrine reuptake in the pre-synaptic sympathetic nerve terminals, increasing resting norepinephrine concentration (42), which can cause an increased resting heart rate and eventually development of hypertension (43, 44). Therefore, the renin-angiotensin system and sympathetic nervous system create a positive feedback loop to increase hypertension in obese individuals (40).
Sustained hypertension increases left ventricular afterload, forcing the left ventricle to work harder (45). This leads to pathologic hypertrophy of the ventricular walls and ventricular chamber dilation, eventually culminating in decreased myocardial function and the onset of heart failure (46, 47). As myocardial function declines, the cardiovascular system becomes impaired, resulting in insufficient blood flow. Oxygen and nutrients are then unable to meet the physiological demands of the body, resulting in tachycardia and extreme fatigue, as well as compounding health issues such as pulmonary congestion, fluid retention, and arrhythmias (48, 49).
Another potential cause of obesity-related CVD is metabolic overload of the heart, which can occur independent of hypertension. The heart is a “metabolic omnivore” (50), but in the obesogenic state, and particularly with insulin resistance, fatty acid uptake and utilization is significantly increased (51). This can lead to inefficient β-oxidation and intramyocardial lipid accumulation (52). Because the heart has limited storage capacity, abundant accumulation of excess lipids and toxic lipid metabolites results in “lipotoxicity” which contributes to cardiac dysfunction (53–55). Indeed, several studies have demonstrated that metabolic changes precede structural changes in the heart (56, 57). Cardiac metabolism is also altered in T2D patients who are not obese. As in obesity, T2D is associated with elevated circulating free fatty acids, increased myocardial fatty acid uptake and utilization, and myocardial insulin resistance leading to decreased glucose uptake and utilization in the heart (58–61).
Atherosclerosis is the most common form of CVD, and the development of atherosclerosis progresses slowly in response to persistent exposure to an unhealthy, sedentary lifestyle, including obesity (34, 62). In an obese state, circulating levels of triglycerides and LDL cholesterol are increased (63), causing small plaques to form under endothelial cells of the innermost surface of artery walls (34, 62, 64). While normal endothelial cells can prevent adhesion of these plaques by leukocytes, under obese conditions LDL molecules are oxidized causing endothelial cells to instead express adhesion molecules and chemoattractants (65–67). In response, macrophages take up oxidized LDL and are transformed into foam cells (64, 65) which localize to the fatty plaques within arteries and secrete factors that further promote plaque formation (67, 68). Resulting plaques cause vessel walls to thicken and stiffen, inhibiting blood flow (69). If the plaques become large enough or thrombosis occurs, the inhibition of blood flow can lead to ischemic conditions and cardiac events including stroke, myocardial infarction (MI), or cardiac arrest (70), all of which can be fatal.
Exercise Training Improves Cardiovascular Health
There are several risk factors leading to the development and progression of CVD, but one of the most prominent is a sedentary lifestyle (34, 35, 71). A sedentary lifestyle can be characterized by both obesity and consistently low levels of physical activity. Thus, lifestyle interventions that aim to increase physical activity and decrease obesity are attractive therapeutic methods to combat most non-congenital types of CVD.
Physical Activity Decreases Cardiovascular Risk Factors
Regular physical exercise is associated with numerous health benefits to reduce the progression and development of obesity, T2D, and CVD (9–14). Several randomized clinical trials have demonstrated that lifestyle interventions including moderate exercise and a healthy diet improve cardiovascular health in at-risk populations (72, 73). Individuals with metabolic syndrome who participated in a 4 month program of either a diet (caloric restriction) or exercise intervention had reduced adiposity, decreased systolic, diastolic and mean arterial blood pressure, and lower total and low-density lipoprotein (LDL) cholesterol lipid profiles compared to the control group (12). Both the diet and exercise intervention improve these cardiovascular outcomes to a similar extent (74).
Several previous studies have investigated the effects of diet and exercise, independently or in combination, on metabolic and cardiovascular health and have determined that diet, exercise, or a combination of diet and exercise induces weight loss, decreases visceral adiposity, lowers plasma triglycerides, plasma glucose, HDL levels, and blood pressure, and improves VO2max (75–78). Importantly, several of these beneficial effects of exercise are evident independent of weight loss (79). Studies have shown that exercise can improve metabolic and cardiovascular health independent of changes in body weight, including improved glucose homeostasis (80, 81), endothelial function (82), blood pressure (83), and HDL levels (84, 85). These data indicate exercise, independent of changes in body mass, results in significant improvements in cardiovascular and metabolic health. Although a detailed analysis of the vast impact of diet on cardiometabolic health is outside the scope of this review, the importance of diet and exercise in tandem should not be ignored, as many studies have shown that cardiometabolic health is improved to a higher extent in response to a combined diet and exercise programs compared to either intervention alone (86–89).
Exercise has a similar effect on cardiovascular improvements in lean and overweight normoglycemic subjects. In a 1 year study of non-obese individuals, a 16–20% increase in energy expenditure (of any form of exercise) with no diet intervention resulted in a 22.3% decrease in body fat mass and reduced LDL cholesterol, total cholesterol/HDL ratio, and C-reactive protein concentrations, all risk factors associated with CVD (74). In overweight individuals, 7–9 months of low-intensity exercise (walking ~19 km per week at 40–55% VO2peak) significantly increased cardiorespiratory fitness compared to sedentary individuals (90). Together these data indicate that exercise interventions decrease the risk or severity of CVD in subjects who are lean, obese, or have type 2 diabetes (12, 74, 90).
Physical Activity Improves Cardiovascular Function in Patients With CVD
Exercise is also an important therapeutic treatment for patients who have cardiovascular diseases (14). A systematic review of 63 studies found that exercise-based cardiac rehabilitation improved cardiovascular function (91). These studies consisted of various forms of aerobic exercise at a range of intensities (from 50 to 95% VO2), over a multitude of time periods (1–47 months). Overall, exercise significantly reduced CVD-related mortality, decreased risk of MI, and improved quality of life (91). Another study looked specifically in patients with atherosclerosis post-revascularization surgery. Patients who underwent 60 min of exercise per day on a cycle ergometer for 4 weeks had an increase blood flow reserve (29%) and improved endothelium-dependent vasodilatation (10). A recent study provided personalized aerobic exercise rehabilitation programs for patients who had an acute myocardial infarction for 1 year after a coronary intervention surgery (92). The patients who underwent the exercise rehabilitation program had increased ejection fraction (60.81 vs. 53% control group), increased exercise tolerance, and reduced cardiovascular risk factors 6 months after starting the exercise rehabilitation program (92). This improvement in cardiovascular health in patients with atherosclerosis or post-MI is likely the result of increased myocardial perfusion in response to exercise, however more research is required to fully understand these mechanisms (10).
One defining characteristic of heart failure is exercise intolerance (93), which resulted in a prescription of bed rest for these patients until the 1950s (94). However, it has now been shown that a monitored rehabilitation program using moderate intensity exercise is safe for heart failure patients, and this has now become an important therapeutic for patients with heart failure (95–97). Meta-analyses and systemic reviews have shown that exercise training in heart failure patients is associated with improved quality of life, reduced risk of hospitalization and decreased rates of long-term mortality (93, 98–102). One study of heart failure patients found that aerobic exercise (walking or cycling) at 60–70% of heart rate reserve 3–5 times per week for over 3 years led to improved health and overall quality of life (determined by a self-reported Kansas City Cardiomyopathy Questionnaire, a 23-question disease-specific questionnaire) (103). Other studies have shown that exercise-based rehabilitation at a moderate intensity in heart failure patients improves cardiorespiratory fitness and increases both exercise endurance capacity and VO2max (12–31% increase) (101, 104).
More recent studies have examined the effects of high-intensity exercise on patients with heart failure. A recent study found that 12 weeks of high intensity interval training (HIIT) in heart failure patients (with reduced ejection fraction) was well-tolerated and had similar benefits compared to patients who underwent moderate continuous exercise (MCE) training, including improved left ventricular remodeling and aerobic capacity (105). A separate study found that 4 weeks of HIIT in heart failure patients with preserved ejection fraction improved VO2peak and reduced diastolic dysfunction compared to both pre-training values and compared to the MCE group (78). These studies indicate that both moderate and high intensity exercise training improve cardiovascular function in heart failure patients, likely related to increased endothelium-dependent vasodilation (106) and improved aerobic capacity (78, 101, 105).
Mechanisms Regulating Exercise-Induced Benefits on Cardiovascular Health
Multiple mechanisms mediate the benefits of regular physical exercise on cardiovascular health (13, 14) (Figure 1). Exercise represents a major challenge to whole-body homeostasis, and provokes widespread changes in numerous cells, tissues, and organs in response to the increased metabolic demand (121), including adaptations to the cardiovascular system (13, 14).
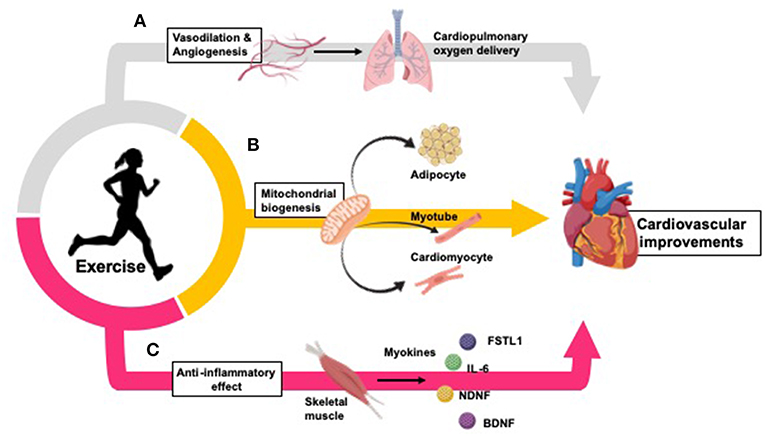
Figure 1. Exercise improves cardiovascular health by inducing changes in oxygen delivery, vasculature, peripheral tissues, and inflammation. (A) Exercise improves oxygen delivery throughout the body through promotion of vasodilation and angiogenesis (107–110). (B) Exercise increases mitochondrial biogenesis in adipocytes (104, 111, 112), skeletal muscle myotubes (113), and cardiomyocytes (14, 114, 115). (C) Exercise causes a long-term anti-inflammatory effect (which is inversely related to the increased inflammation typically seen in CVD and obesity) (116). Myokines released from skeletal muscle during physical exercise partially mediate these anti-inflammatory effects, and promote inter-tissue cross talk to mediate further cardiovascular benefits (117–120).
Exercise induces adaptations in several cell types and tissues throughout the body. Exercise increases mitochondrial biogenesis in adipocytes (104, 111, 112), skeletal muscle myocytes (113), and cardiomyocytes (14, 114, 115), increasing aerobic respiration within these tissues. Additionally, exercise improves oxygen delivery throughout the body through vasodilation and angiogenesis (107–110), protecting against ischemia-reperfusion injury in the heart (122, 123). Further, exercise causes a long-term anti-inflammatory effect which is inversely related to the increased inflammation typically seen in CVD and obesity (116). Myokines released from skeletal muscle during physical exercise partially mediate these anti-inflammatory effects, and promote inter-tissue cross talk to mediate further cardiovascular benefits (117–120).
Exercise Improves Mitochondrial Biogenesis and Function
Many of the benefits sustained by exercise are due to mitochondrial adaptations throughout the body. For example, exercise improves long-term cardiorespiratory fitness (VO2) by increasing the mitochondrial content and desaturation of myoglobin in skeletal muscle tissue, improving the oxidative capacity of skeletal muscle (113, 124, 125). The increase of oxygen uptake and utilization by skeletal muscle (as indicated by arteriovenous oxygen difference; a-vO2) in response to regular exercise (126) is protective against a decrease in obesity-related a-vO2, resulting in individuals to require more blood to receive the same amount of oxygen (127).
Mitochondrial biogenesis is augmented in cardiomyocytes in response to exercise (14, 114, 115, 128). This is likely due to enhanced activation of AMP-activated protein kinase (AMPK) and subsequent increase mitochondrial PGC-1α expression (109, 114) Exercise also increases the ability of mitochondria to oxidize fatty acids (the predominant substrate utilized in healthy myocardium), thus increasing the capacity for ATP synthesis (14, 129–133). These exercise-induced enhancements of mitochondrial function are important in preventing cardiovascular dysfunctions often caused by obesity.
Obesity is associated with defective mitochondrial biogenesis in the myocardium (134) and reduced mitochondrial capacity for oxidative phosphorylation and ATP synthesis (135, 136). In heart failure, fatty acid uptake, and utilization is decreased (137), likely causing the heart failure associated shift toward glucose metabolism in order to preserve cardiovascular function (130, 137, 138). However, in advanced heart failure, diabetes, or obesity, myocardial insulin resistance may develop, impairing glucose uptake and accelerating cardiovascular dysfunction (139–141). Importantly, insulin sensitivity is improved in response to regular exercise (142) which is vital in reducing the risk of obesity-related insulin resistance. Insulin has also been indicated to directly regulate mitochondrial metabolism by promoting induction of OPA1, a GTPase that controls mitochondrial cristae integrity, energetics and mitochondrial DNA maintenance (143, 144), thus indicating another potential mechanism of exercise-induced improvements in cardiovascular health through mitochondrial function enhancement.
Reactive oxygen species (ROS) are physiological byproducts of aerobic mitochondrial metabolism and while necessary for initiating cellular repair or apoptosis, increased levels of ROS are associated with inflammation and several forms of CVD (145). While exercise increases the direct production of ROS by mitochondria, the net cellular ROS load is reduced by exercise due to increased action of antioxidant systems (146). Essentially, exercise creates a system in which cells exhibit a “favorable” response within low exposures of ROS, allowing antioxidant systems to work effectively (147).
By increasing the ability of mitochondria to prevent oxidative damage, exercise-induced modifications to mitochondria protect against ischemia-reperfusion damage to the heart. During ischemia, the absence of oxygen from the heart creates an environment in which the return of oxygenated blood flow leads to the induction of inflammation and oxidative stress rather than restoration of normal function (148). In contrast, exercise-induced adaptations to cardiomyocyte mitochondria dampen oxidative damage caused by ischemia-reperfusion, resulting in reduced cardiac injury and decreasing the risk of ischemia-related cardiac dysfunction or death (149–151).
Exercise Improves Vasculature and Myocardial Perfusion
Exercise training induces vascular adaptations to several tissues (107, 108). In the heart, the increase in vascularization protects against vascular stress and reduces the likelihood of a cardiac event (24–26). These adaptations are mediated through increased expression of vascular endothelial nitric oxide synthase (eNOS). Exercise increases the intensity of physiological shear stress, inducing the shear stress-dependent activity of c-Src in endothelial cells and increasing expression of eNOS (27, 152). In the vascular endothelium, eNOS catalyzes the production of nitric oxide (NO) which causes vasodilation, inhibits platelet aggregation and prevents leukocyte adhesion to vessel walls, thus reducing the onset of atherosclerosis, thrombosis, ischemia, or other cardiac events (152, 153).
Exercise also induces angiogenesis, however the mechanisms regulating this process are unclear. It has been hypothesized that the increase in nitric oxide (NO) production after exercise upregulates pro-angiogenic factors, particularly vascular endothelial growth factor (VEGF) (154). One recent study determined that male rats who underwent exercise training for 10 weeks after MI had increased Akt phosphorylation of eNOS, and reactivation of cardiac VEGF pathway activity, resulting in increased angiogenesis (155). While the mechanisms are not completely defined, it is clear that exercise induces arteriogenesis, increases angiogenesis and protects against vascular stress, thus decreasing the possibility of a cardiac event (107–110, 122, 123).
Exercise Reduces Chronic Inflammation
Inflammation is a complex yet normal biological reaction to damaging stimuli (156). Chronic inflammation is associated with multiple diseases including obesity, T2D, and CVD (116, 157). Excess consumption of nutrients causes cells including adipocytes (158), hepatocytes (159), islet cells (160), and skeletal muscle cells (161) to activate the transcription factors nuclear factor kappa-light-chain-enhancer of activated B cells (NF-κB) and activator protein 1 (AP-1), increase expression of toll-like receptor 4 (TLR4) (162, 163), and stimulate the release of cytokines such as TNF-α, IL-6, IL-1β, and CCL2 (158, 164). The subsequent inflammation is modest in comparison to inflammatory responses during infection or injury (165) but remains as a chronic response to obesity termed “meta-inflammation” (163). Exercise, however, results in a long-term anti-inflammatory effect (116, 156). The exercise-induced reduction of meta-inflammation during disease is hypothesized by some to be related to downregulation of NF-κB (166–168), but exercise also decreases monocyte accumulation and suppresses the release of TNF-α and other pro-inflammatory adipokines, creating an anti-inflammatory effect (169–172).
Excess immune activation caused by obesity is of particular concern for vascular health, as activation of TLR4 causes monocyte recruitment and conversion to foam cells, driving the progression of atherosclerosis (67, 173). Exercise prevents the development of atherosclerosis by reducing expression of TLRs on monocytes and macrophages, which subsequently decreases the availability of TLR4 ligands and inhibiting pro-inflammatory cytokine production (170, 171, 174). Exercise also decreases pro-inflammatory N-terminal pro b-type natriuretic (NT-proBNP) and high-sensitivity C-reactive protein (hsCRP) within the heart, both of which are predictors of heart failure in atherosclerosis (175, 176).
Exercise Enhances Inter-tissue Communication Through Release of Myokines
Skeletal muscle can act as a secretory organ by stimulating the production, secretion, and expression of specific myokines after contraction (177–179). Myokines are chemical messengers that function in an autocrine, paracrine, or endocrine manner to influence crosstalk between different organs including skeletal muscle, liver, and adipose tissue (180–185). They are of great interest with regards to cardiovascular health because the well-known protective actions of exercise on cardiovascular function are at least partially mediated by increased secretion of myokines (Figure 2) (195). Some myokines that impact cardiovascular health include IL-6, myonectin, Fstl1, and NDNF (196).
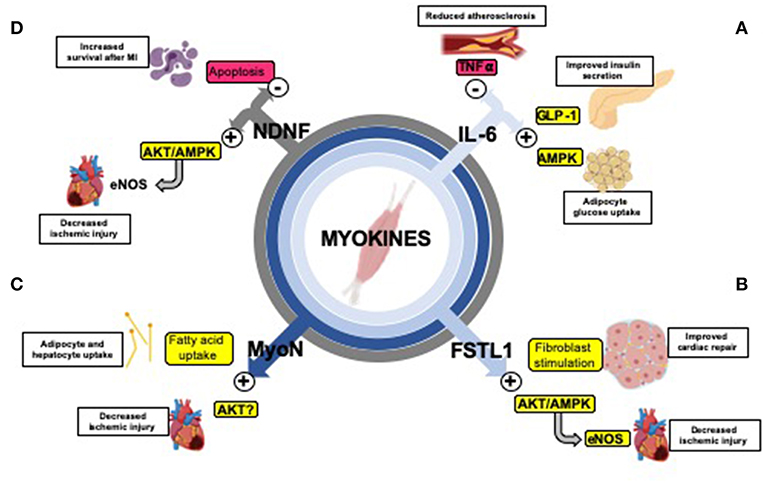
Figure 2. Exercise-induced myokines mediate organ cross-talk and improve cardiometabolic health. (A) The myokine IL-6 inhibits TNF-α (186), reducing inflammation and protecting against the formation of atherosclerosis (187); stimulates GLP-1 secretion causing improved insulin secretion (188); increases lipolysis and fatty acid oxidation in adipose tissue (189) and increases glucose uptake through the AMPK signaling pathway (190, 191). (B) Fstl1 decreases ischemic injury size through activation of the Akt/AMPK pathway (activating eNOS and enhancing revascularization) (118, 119) and early fibroblast stimulation (which aids in repair after ischemia-reperfusion) (192). (C) Myonectin (MyoN) increases fatty acid uptake in adipocytes and hepatocytes (117), and promotes protects against ischemic injury in the heart, possibly through Akt activation (193). (D) NDNF improves survival after myocardial infarction (MI) by reducing apoptosis (120) through stimulation of the Akt/AMPK/eNOS pathway (enhancing revascularization) (194).
Interleukin-6 (IL-6)
IL-6 was introduced as the first myokine over a decade ago (197). Circulating levels of IL-6 are increased in response an acute bout of aerobic exercise (198, 199) and can act in an endocrine fashion to improve metabolic and cardiovascular health. Exercise-induced elevated concentrations of IL-6 can stimulate glucagon-like peptide-1 (GLP-1) secretion from intestinal L cells and pancreatic α cells, leading to improvements in insulin secretion and glycemia (188). IL-6 also increases lipolysis and fatty acid oxidation in adipose tissue (189) and can increase glucose uptake through stimulation of the AMP-activated protein kinase (AMPK) signaling pathway (190, 191). With regard to cardiovascular function, IL-6 can reduce inflammation by inhibiting tumor necrosis factor-α (TNF- α) (186). This results in a protective effect on cardiovascular health because TNF- α is involved in the formation of atherosclerosis, development of heart failure, and subsequent complications, including myocardial infarction (MI) (187). More investigation is required to determine the direct effects of IL-6 action on cardiovascular health.
Myonectin
Myonectin (or CTRP15) is abundantly expressed in skeletal muscle and is increased in response to chronic aerobic exercise (117). Importantly, injection of myonectin into wild-type mice decreases circulating free fatty acids levels by increasing fatty acid uptake in adipocytes and hepatocytes (117). Myonectin has also been identified to have protective effects on cardiovascular health; mice deficient in Myonectin had enhanced ischemic injury in response to MI while systemic delivery of myonectin attenuated ischemic injury (200). Further work is needed to determine whether these benefits are observed in response to an increase in myonectin after exercise.
Follistatin-Like 1 (Fstl1)
Fstl1, also referred to as TSC-36, is a secreted glycoprotein that belongs to the follistatin family of proteins and is upregulated in skeletal muscle in response to exercise (194, 201, 202). Expression of Fstl1 is also increased in ischemic and hypertrophic hearts of mice and functions in a protective manner (118). Systemic administration of Fstl1 in both mouse and swine models led to reduced apoptosis, inflammation and injury size following ischemia-reperfusion (118, 119). In vitro, treatment of cultured cardiomyocytes with Fstl1 reduces apoptosis in response to hypoxia-reoxygenation by activating Akt and AMPK (118, 119). One recent study demonstrated that Fstl1 stimulates early fibroblast activation, which is required for acute repair and protects the heart from rupture after ischemia-reperfusion (192). While the exact role of an exercise-induced increase in Flst1 on cardiovascular function has not been defined, these data indicate that Fstl1 is increased in response to exercise, and an increase in circulating Fstl1 functions to repair cardiovascular damage and improve cardiovascular function (202).
Neuron-Derived Neurotrophic Factor (NDNF)
NDNF is a glycosylated protein secreted from the endothelial cells of skeletal muscle (203). Although initially identified as a neurotrophic factor expressed in mouse brain and spinal cord (204), NDNF is also released from skeletal muscle in response to exercise (203) and acts as a hypoxia-induced pro-angiogenic factor that stimulates endothelial cell network formation through activation of the Akt/eNOS signaling pathway (194). This pro-angiogenic affect is an important component in the recovery from MI; intramuscular administration of NDNF using an adenoviral vector improved systolic function in a mouse model after MI (120). Increased NDNF levels are also associated with reduced myocardial hypertrophy and apoptosis in post-MI hearts (120). Another study showed that down-regulation of NDNF by siRNA impairs recovery from ischemia-reperfusion injury (205). Treatment of NDNF in cardiomyocytes also reduces hypoxia-induced apoptosis via activation of the focal adhesion kinase/Akt-dependent pathway (120). Additionally, increased levels of NDNF released from skeletal muscle in response to exercise enhance fatty acid oxidation through activation of AMPK (203). These data demonstrate the importance of NDNF as an endogenous ischemia- and exercise inducible factor that can enhance revascularization and therefore have a cardiovascular protective effect.
Conclusions
The rate of obesity-related cardiovascular disease is rapidly increasing, and often associated with additional co-morbidities including type 2 diabetes (3, 6, 8). It is clear that exercise reduces cardiovascular risk factors, and this reduction in risk factors is independent of changes to body weight or incidence of type 2 diabetes (75–77, 79, 206, 207). Exercise is also an important therapeutic treatment for patients who have cardiovascular diseases (14), further demonstrating the protective and restorative properties of exercise. In patients with CVD, exercise improved endothelium-dependent vasodilatation, increased ejection fraction and exercise tolerance, improved quality of life, and reduced CVD-related mortality (10, 91, 92, 101, 103, 208–211). Exercise improves cardiovascular health by several mechanisms including increased mitochondrial biogenesis and fatty acid oxidation (14, 114, 115, 128–130) dilation of blood vessels causing improved myocardial perfusion (9–11), and reduction of inflammation providing protection against the development of atherosclerosis (67, 116, 156). Myokines released from skeletal muscle during exercise also mediate systemic and cardiovascular health benefits through an anti-inflammatory action, increased fatty acid oxidation, increased glucose uptake, and improved insulin secretion and sensitivity (117, 186, 193, 196, 212–214). Importantly, several myokines (IL-6, Myonectin, Fstl1, and NDNF) have also been shown to have cardiovascular protective effects in response to ischemia-reperfusion injury (117–120, 186, 187).
While it is clear that exercise is important, the mechanistic pathways behind exercise-induced benefits on cardiovascular health are still being identified. Further understanding of the molecular mechanisms through which exercise improves cardiovascular function will lead to the development of therapeutics which can act in conjunction with exercise programs, and for individuals whom are unable or unwilling to exercise to amplify the beneficial effects of exercise.
Future research will investigate the effects of cardiac specific proteins on cardiovascular health, expanding research into the areas of system cross-talk will help delineate how other tissues, skeletal muscle in particular, can mediate cardiovascular improvements via myokine release. How these myokines affect cardiovascular function, including adaptations to mitochondrial activity, angiogenesis and inflammatory responses will provide insight into new mechanisms for the beneficial effects of exercise on cardiovascular function. Accordingly, myokines may act as potential targets for heart disease prevention and therapies. Recent studies have investigated the use of gene therapies, including the use of adeno-associated virus, on cardiovascular function. While these therapies have not been fully optimized with remaining issues in immunogenicity, efficacy and genotoxicity (215), their development provides excitement for the potential therapies focused on exercise-induced myokines that improve cardiovascular function as a treatment for patients who are unable, or perhaps unwilling, to exercise. Together these data highlight the importance of exercise and exercise-related therapies to both prevents the development of cardiovascular disease and promotes recovery and improved health in patients with CVD.
Author Contributions
KP, KKB, and KIS outlined, drafted, and contributed to the writing of the manuscript. All authors approved the final version of the manuscript.
Funding
This work was supported by National Institutes of Health Grants R01-HL138738 and 17CSA33610078 to KIS and K01-DK116916 to KKB.
Conflict of Interest Statement
The authors declare that the research was conducted in the absence of any commercial or financial relationships that could be construed as a potential conflict of interest.
References
1. Hales CM, Carroll MD, Fryar CD, Ogden CL. Prevalence of Obesity Among Adults and Youth: United States, 2015−2016. U.S. Department of Health and Human Services; National Center for Health Statistics; Centers for Disease Control and Prevention (2017).
2. Brown CD, Higgins M, Donato KA, Rohde FC, Garrison R, Obarzanek E, et al. Body mass index and the prevalence of hypertension and dyslipidemia. Obes Res. (2000) 8:605–19. doi: 10.1038/oby.2000.79
3. Guh DP, Zhang W, Bansback N, Amarsi Z, Birmingham CL, Anis AH. The incidence of co-morbidities related to obesity and overweight: a systematic review and meta-analysis. Bmc Public Health. (2009) 9:88. doi: 10.1186/1471-2458-9-88
4. LamonFava S, Wilson PWF, Schaefer EJ. Impact of body mass index on coronary heart disease risk factors in men and women - The Framingham Offspring Study. Arterioscl Throm Vas. (1996) 16:1509–15. doi: 10.1161/01.ATV.16.12.1509
5. Wilson PWF, D'Agostino RB, Sullivan L, Parise H, Kannel WB. Overweight and obesity as determinants of cardiovascular risk - The Framingham experience. Arch Inter Med. (2002) 162:1867–72. doi: 10.1001/archinte.162.16.1867
6. Van Gaal LF, Mertens IL, De Block CE. Mechanisms linking obesity with cardiovascular disease. Nature. (2006) 444:875–80. doi: 10.1038/nature05487
7. Moore JX, Chaudhary N, Akinyemiju T. Metabolic syndrome prevalence by race/ethnicity and sex in the united states. national health and nutrition examination survey, 1988-2012. Prev Chronic Dis. (2017) 14:160287. doi: 10.5888/pcd14.160287
8. Kivimaki M, Kuosma E, Ferrie JE, Luukkonen R, Nyberg ST, Alfredsson L, et al. Overweight. obesity, and risk of cardiometabolic multimorbidity: pooled analysis of individual-level data for 120 813 adults from 16 cohort studies from the USA and Europe. Lancet Public Health. (2017) 2:e277–85. doi: 10.1016/S2468-2667(17)30074-9
9. Ashor AW, Lara J, Siervo M, Celis-Morales C, Oggioni C, Jakovljevic DG, et al. Exercise modalities and endothelial function: a systematic review and dose-response meta-analysis of randomized controlled trials. Sports Med. (2015) 45:279–96. doi: 10.1007/s40279-014-0272-9
10. Hambrecht R, Wolf A, Gielen S, Linke A, Hofer J, Erbs S, et al. Effect of exercise on coronary endothelial function in patients with coronary artery disease. N Engl J Med. (2000) 342:454–60. doi: 10.1056/NEJM200002173420702
11. Laughlin MH, Bowles DK, Duncker DJ. The coronary circulation in exercise training. Am J Physiol-Heart C. (2012) 302:H10–23. doi: 10.1152/ajpheart.00574.2011
12. Pettman TL, Buckley JD, Misan GM, Coates AM, Howe PR. Health benefits of a 4-month group-based diet and lifestyle modification program for individuals with metabolic syndrome. Obes Res Clin Pract. (2009) 3:221–35. doi: 10.1016/j.orcp.2009.06.002
13. Platt C, Houstis N, Rosenzweig A. Using exercise to measure and modify cardiac function. Cell Metab. (2015) 21:227–36. doi: 10.1016/j.cmet.2015.01.014
14. Vega RB, Konhilas JP, Kelly DP, Leinwand LA. Molecular mechanisms underlying cardiac adaptation to exercise. Cell Metab. (2017) 25:1012–26. doi: 10.1016/j.cmet.2017.04.025
15. Che L, Li D. The effects of exercise on cardiovascular biomarkers: new Insights, recent data, and applications. Adv Exp Med Biol. (2017) 999:43–53. doi: 10.1007/978-981-10-4307-9_3
16. Stanford KI, Goodyear LJ. Exercise and type 2 diabetes: molecular mechanisms regulating glucose uptake in skeletal muscle. Adv Physiol Educ. (2014) 38:308–14. doi: 10.1152/advan.00080.2014
17. Nystoriak MA, Bhatnagar A. Cardiovascular Effects and Benefits of Exercise. Front Cardiovasc Med. (2018) 5:135. doi: 10.3389/fcvm.2018.00135
18. Egan B, Zierath JR. Exercise metabolism and the molecular regulation of skeletal muscle adaptation. Cell Metab. (2013) 17:162–84. doi: 10.1016/j.cmet.2012.12.012
19. Slentz CA, Bateman LA, Willis LH, Granville EO, Piner LW, Samsa GP, et al. Effects of exercise training alone vs. a combined exercise and nutritional lifestyle intervention on glucose homeostasis in prediabetic individuals: a randomised controlled trial. Diabetologia. (2016) 59:2088–98. doi: 10.1007/s00125-016-4051-z
20. Conn VS, Koopman RJ, Ruppar TM, Phillips LJ, Mehr DR, Hafdahl AR. Insulin sensitivity following exercise interventions: systematic review and meta-analysis of outcomes among healthy adults. J Prim Care Community Health. (2014) 5:211–22. doi: 10.1177/2150131913520328
21. Lin X, Zhang X, Guo J, Roberts CK, McKenzie S, Wu WC, et al. Effects of exercise training on cardiorespiratory fitness and biomarkers of cardiometabolic health: a systematic review and meta-analysis of randomized controlled trials. J Am Heart Assoc. (2015) 4:e002014. doi: 10.1161/JAHA.115.002014
22. Ruderman NB, Park H, Kaushik VK, Dean D, Constant S, Prentki M, et al. AMPK as a metabolic switch in rat muscle, liver and adipose tissue after exercise. Acta Physiol Scand. (2003) 178:435–42. doi: 10.1046/j.1365-201X.2003.01164.x
23. Petridou A, Nikolaidis MG, Matsakas A, Schulz T, Michna H, Mougios V. Effect of exercise training on the fatty acid composition of lipid classes in rat liver, skeletal muscle, and adipose tissue. Eur J Appl Physiol. (2005) 94:84–92. doi: 10.1007/s00421-004-1294-z
24. Hambrecht R, Adams V, Erbs S, Linke A, Krankel N, Shu Y, et al. Regular physical activity improves endothelial function in patients with coronary artery disease by increasing phosphorylation of endothelial nitric oxide synthase. Circulation. (2003) 107:3152–8. doi: 10.1161/01.CIR.0000074229.93804.5C
25. Leung FP, Yung LM, Laher I, Yao XQ, Chen ZY, Huang Y. Exercise, vascular wall and cardiovascular diseases an update (Part 1). Sports Med. (2008) 38:1009–24. doi: 10.2165/00007256-200838120-00005
26. Fiuza-Luces C, Garatachea N, Berger NA, Lucia A. Exercise is the real polypill. Physiology. (2013) 28:330–58. doi: 10.1152/physiol.00019.2013
27. Davis ME, Cai H, McCann L, Fukai T, Harrison DG. Role of c-Src in regulation of endothelial nitric oxide synthase expression during exercise training. Am J Physiol-Heart C. (2003) 284:H1449–53. doi: 10.1152/ajpheart.00918.2002
28. Ellison GM, Waring CD, Vicinanza C, Torella D. Physiological cardiac remodelling in response to endurance exercise training: cellular and molecular mechanisms. Heart. (2012) 98:5–10. doi: 10.1136/heartjnl-2011-300639
29. Fontana L. Interventions to promote cardiometabolic health and slow cardiovascular ageing. Nat Rev Cardiol. (2018) 15:566–77. doi: 10.1038/s41569-018-0026-8
30. Duncker DJ, Bache RJ. Regulation of coronary blood flow during exercise. Physiol Rev. (2008) 88:1009–86. doi: 10.1152/physrev.00045.2006
31. Virani SS Benjamin EJ, Callaway CW, Chang AR, Cheng S, Chiuve SE, et al. Heart Disease and Stroke Statistics 2018 At-a-Glance. American Heart Association (2018).
32. Division for Heart Disease and Stroke Prevention. Heart Disease Fact Sheet Centers for Disease Control and Prevention (2017). (accessed January 19, 2019).
34. Heidenreich PA, Trogdon JG, Khavjou OA, Butler J, Dracup K, Ezekowitz MD, et al. Forecasting the future of cardiovascular disease in the United States: a policy statement from the American Heart Association. Circulation. (2011) 123:933–44. doi: 10.1161/CIR.0b013e31820a55f5
35. American Heart Association. American Heart Disease and Stroke Statistics - 2018 At a Glance (2018). (accessed January 6, 2019).
36. Chen Y, Rollins J, Paigen B, Wang X. Genetic and genomic insights into the molecular basis of atherosclerosis. Cell Metab. (2007) 6:164–79. doi: 10.1016/j.cmet.2007.07.001
37. Harte A, McTernan P, Chetty R, Coppack S, Katz J, Smith S, et al. Insulin-mediated upregulation of the renin angiotensin system in human subcutaneous adipocytes is reduced by rosiglitazone. Circulation. (2005) 111:1954–61. doi: 10.1161/01.CIR.0000161954.17870.5D
38. Briones AM, Nguyen Dinh Cat A, Callera GE, Yogi A, Burger D, He Y, et al. Adipocytes produce aldosterone through calcineurin-dependent signaling pathways: implications in diabetes mellitus-associated obesity and vascular dysfunction. Hypertension. (2012) 59:1069–78. doi: 10.1161/HYPERTENSIONAHA.111.190223
39. DeMarco VG, Aroor AR, Sowers JR. The pathophysiology of hypertension in patients with obesity. Nat Rev Endocrinol. (2014) 10:364–76. doi: 10.1038/nrendo.2014.44
40. Cabandugama PK, Gardner MJ, Sowers JR. The renin angiotensin aldosterone system in obesity and hypertension: roles in the cardiorenal metabolic syndrome. Med Clin North Am. (2017) 101:129–37. doi: 10.1016/j.mcna.2016.08.009
41. Schutten MT, Houben AJ, de Leeuw PW, Stehouwer CD. The link between adipose tissue renin-angiotensin-aldosterone system signaling and obesity-associated hypertension. Physiology. (2017) 32:197–209. doi: 10.1152/physiol.00037.2016
42. Raasch W, Betge S, Dendorfer A, Bartels T, Dominiak P. Angiotensin converting enzyme inhibition improves cardiac neuronal uptake of noradrenaline in spontaneously hypertensive rats. J Hypertens. (2001) 19:1827–33. doi: 10.1097/00004872-200110000-00017
43. Rocchini AP, Yang JQ, Gokee A. Hypertension and insulin resistance are not directly related in obese dogs. Hypertension. (2004) 43:1011–6. doi: 10.1161/01.HYP.0000123073.48855.e9
44. Jiang SZ, Lu W, Zong XF, Ruan HY, Liu Y. Obesity and hypertension. Exp Ther Med. (2016) 12:2395–9. doi: 10.3892/etm.2016.3667
46. Aurigemma GP, de Simone G, Fitzgibbons TP. Cardiac remodeling in obesity. Circ Cardiovasc Imaging. (2013) 6:142–52. doi: 10.1161/CIRCIMAGING.111.964627
47. Halade GV, Kain V. Obesity and Cardiometabolic Defects in Heart Failure Pathology. Compr Physiol. (2017) 7:1463–77. doi: 10.1002/cphy.c170011
48. Mayo Clinic. Diseases & Conditions: Heart Failure (2018). Mayo Clinic Staff (accessed February 10, 2019).
49. Friedman DJ, Wang N, Meigs JB, Hoffmann U, Massaro JM, Fox CS, et al. Pericardial fat is associated with atrial conduction: the Framingham Heart Study. J Am Heart Assoc. (2014) 3:e000477. doi: 10.1161/JAHA.113.000477
50. Taegtmeyer H, Lubrano G. Rethinking cardiac metabolism: metabolic cycles to refuel and rebuild the failing heart. F1000Prime Rep. (2014) 6:90. doi: 10.12703/P6-90
51. Lopaschuk GD, Folmes CD, Stanley WC. Cardiac energy metabolism in obesity. Circ Res. (2007) 101:335–47. doi: 10.1161/CIRCRESAHA.107.150417
52. Abel ED, Litwin SE, Sweeney G. Cardiac remodeling in obesity. Physiol Rev. (2008) 88:389–419. doi: 10.1152/physrev.00017.2007
53. Harmancey R, Wilson CR, Taegtmeyer H. Adaptation and maladaptation of the heart in obesity. Hypertension. (2008) 52:181–7. doi: 10.1161/HYPERTENSIONAHA.108.110031
54. Fukushima A, Lopaschuk GD. Cardiac fatty acid oxidation in heart failure associated with obesity and diabetes. Biochim Biophys Acta. (2016) 1861:1525–34. doi: 10.1016/j.bbalip.2016.03.020
55. Park TS, Yamashita H, Blaner WS, Goldberg IJ. Lipids in the heart: a source of fuel and a source of toxins. Curr Opin Lipidol. (2007) 18:277–82. doi: 10.1097/MOL.0b013e32814a57db
56. Zhong M, Alonso CE, Taegtmeyer H, Kundu BK. Quantitative PET imaging detects early metabolic remodeling in a mouse model of pressure-overload left ventricular hypertrophy in vivo. J Nucl Med. (2013) 54:609–15. doi: 10.2967/jnumed.112.108092
57. Li J, Kemp BA, Howell NL, Massey J, Minczuk K, Huang Q, et al. Metabolic changes in spontaneously hypertensive rat hearts precede cardiac dysfunction and left ventricular hypertrophy. J Am Heart Assoc. (2019) 8:e010926. doi: 10.1161/JAHA.118.010926
58. Lopaschuk GD, Ussher JR, Folmes CD, Jaswal JS, Stanley WC. Myocardial fatty acid metabolism in health and disease. Physiol Rev. (2010) 90:207–58. doi: 10.1152/physrev.00015.2009
59. Taegtmeyer H, McNulty P, Young ME. Adaptation and maladaptation of the heart in diabetes: part I: general concepts. Circulation. (2002) 105:1727–33. doi: 10.1161/01.CIR.0000012466.50373.E8
60. Young ME, McNulty P, Taegtmeyer H. Adaptation and maladaptation of the heart in diabetes: part II: potential mechanisms. Circulation. (2002) 105:1861–70. doi: 10.1161/01.CIR.0000012467.61045.87
61. Brahma MK, Pepin ME, Wende AR. My sweetheart is broken: role of glucose in diabetic cardiomyopathy. Diabetes Metab J. (2017) 41:1–9. doi: 10.4093/dmj.2017.41.1.1
62. Rocha VZ, Libby P. Obesity, inflammation, and atherosclerosis. Nat Rev Cardiol. (2009) 6:399–409. doi: 10.1038/nrcardio.2009.55
63. Klop B, Elte JW, Cabezas MC. Dyslipidemia in obesity: mechanisms and potential targets. Nutrients. (2013) 5:1218–40. doi: 10.3390/nu5041218
65. Bentzon JF, Otsuka F, Virmani R, Falk E. Mechanisms of plaque formation and rupture. Circ Res. (2014) 114:1852–66. doi: 10.1161/CIRCRESAHA.114.302721
66. Libby P. Inflammation and cardiovascular disease mechanisms. Am J Clin Nutr. (2006) 83:456s−60s. doi: 10.1093/ajcn/83.2.456S
67. Tsoupras A, Lordan R, Zabetakis I. Inflammation, not Cholesterol, Is a Cause of Chronic Disease. Nutrients. (2018) 10:604. doi: 10.3390/nu10050604
68. Bobryshev YV, Ivanova EA, Chistiakov DA, Nikiforov NG, Orekhov AN. Macrophages and their role in atherosclerosis: pathophysiology and transcriptome analysis. Biomed Res Int. (2016). 2016:9582430. doi: 10.1155/2016/9582430
69. Patzelt J, Verschoor A, Langer HF. Platelets and the complement cascade in atherosclerosis. Front Physiol. (2015) 6:49. doi: 10.3389/fphys.2015.00049
70. Thygesen K, Alpert JS, Jaffe AS, Simoons ML, Chaitman BR, White HD, et al. Third universal definition of myocardial infarction. Eur Heart J. (2012) 33:2551–67. doi: 10.1093/eurheartj/ehs184
71. Young DR, Hivert MF, Alhassan S, Camhi SM, Ferguson JF, Katzmarzyk PT, et al. Sedentary behavior and cardiovascular morbidity and mortality: a science advisory from the american heart association. Circulation. (2016) 134:e262–79. doi: 10.1161/CIR.0000000000000440
72. Miele EM, S.Headley AE. The effects of chronic aerobic exercise on cardiovascular risk factors in persons with diabetes mellitus. Curr Diab Rep. (2017) 17:97. doi: 10.1007/s11892-017-0927-7
73. Drenowatz C, Sui X, Fritz S, Lavie CJ, Beattie PF, Church TS, et al. The association between resistance exercise and cardiovascular disease risk in women. J Sci Med Sport. (2015) 18:632–6. doi: 10.1016/j.jsams.2014.09.009
74. Fontana L, Villareal DT, Weiss EP, Racette SB, Steger-May K, Klein S, et al. C.G. Washington University School of Medicine, Calorie restriction or exercise: effects on coronary heart disease risk factors. A randomized, controlled trial. Am J Physiol Endocrinol Metab. (2007) 293:E197–202. doi: 10.1152/ajpendo.00102.2007
75. Wing RR, Lang W, Wadden TA, Safford M, Knowler WC, Bertoni AG, et al. Benefits of modest weight loss in improving cardiovascular risk factors in overweight and obese individuals with type 2 diabetes. Diabetes Care. (2011) 34:1481–6. doi: 10.2337/dc10-2415
76. Dandanell S, Skovborg C, Praest CB, Kristensen KB, Nielsen MG, Lionett S, et al. Maintaining a clinical weight loss after intensive lifestyle intervention is the key to cardiometabolic health. Obes Res Clin Pract. (2017) 11:489–98. doi: 10.1016/j.orcp.2016.09.009
77. Lean ME, Leslie WS, Barnes AC, Brosnahan N, Thom G, McCombie L, et al. Primary care-led weight management for remission of type 2 diabetes (DiRECT): an open-label, cluster-randomised trial. Lancet. (2018) 391:541–51. doi: 10.1016/S0140-6736(17)33102-1
78. Angadi SS, Mookadam F, Lee CD, Tucker WJ, Haykowsky MJ, Gaesser GA. High-intensity interval training vs. moderate-intensity continuous exercise training in heart failure with preserved ejection fraction: a pilot study. J Appl Physiol. (2015) 119:753–8. doi: 10.1152/japplphysiol.00518.2014
79. Gaesser GA, Angadi SS, Sawyer BJ. Exercise and diet, independent of weight loss, improve cardiometabolic risk profile in overweight and obese individuals. Physician Sportsmed. (2011) 39:87–97. doi: 10.3810/psm.2011.05.1898
80. Church TS, Blair SN, Cocreham S, Johannsen N, Johnson W, Kramer K, et al. Effects of aerobic and resistance training on hemoglobin A1c levels in patients with type 2 diabetes: a randomized controlled trial. JAMA. (2010) 304:2253–62. doi: 10.1001/jama.2010.1710
81. Sigal RJ, Kenny GP, Boule NG, Wells GA, Prud'homme D, Fortier M, et al. Effects of aerobic training, resistance training, or both on glycemic control in type 2 diabetes - A Randomized trial. Ann Inter Med. (2007) 147:357–69. doi: 10.7326/0003-4819-147-6-200709180-00005
82. Swift DL, Earnest CP, Blair SN, Church TS. The effect of different doses of aerobic exercise training on endothelial function in postmenopausal women with elevated blood pressure: results from the DREW study. Br J Sport Med. (2012) 46:753–8. doi: 10.1136/bjsports-2011-090025
83. Cornelissen VA, Fagard RH. Effects of endurance training on blood pressure, blood pressure-regulating mechanisms, and cardiovascular risk factors. Hypertension. (2005) 46:667–75. doi: 10.1161/01.HYP.0000184225.05629.51
84. Kodama S, Tanaka S, Saito K, Shu M, Sone Y, Onitake F, et al. Effect of aerobic exercise training on serum levels of high-density lipoprotein cholesterol - A meta-analysis. Arch Inter Med. (2007) 167:999–1008. doi: 10.1001/archinte.167.10.999
85. Swift DL, Johannsen NM, Lavie CJ, Earnest CP, Church TS. The role of exercise and physical activity in weight loss and maintenance. Progr Cardiovasc Dis. (2014) 56:441–7. doi: 10.1016/j.pcad.2013.09.012
86. Sanchez-Aguadero N, Alonso-Dominguez R, Garcia-Ortiz L, Agudo-Conde C, Rodriguez-Martin C, de Cabo-Laso A, et al. Diet and physical activity in people with intermediate cardiovascular risk and their relationship with the health-related quality of life: results from the MARK study. Health Qual Life Out. (2016) 14:169. doi: 10.1186/s12955-016-0572-x
87. Ross R, Dagnone D, Jones PJH, Smith H, Paddags A, Hudson R, et al. Reduction in obesity and related comorbid conditions after diet-induced weight loss or exercise-induced weight loss in men - A randomized, controlled trial. Ann Inter Med. (2000) 133:92–103. doi: 10.7326/0003-4819-133-2-200007180-00008
88. Wu T, Gao X, Chen M, van Dam RM. Long-term effectiveness of diet-plus-exercise interventions vs. diet-only interventions for weight loss: a meta-analysis. Obes Rev. (2009) 10:313–23. doi: 10.1111/j.1467-789X.2008.00547.x
89. Blumenthal JA, Babyak MA, Hinderliter A, Watkins LL, Craighead L, Lin PH, et al. Effects of the DASH diet alone and in combination with exercise and weight loss on blood pressure and cardiovascular biomarkers in men and women with high blood pressure: the ENCORE study. Arch Intern Med. (2010) 170:126–35. doi: 10.1001/archinternmed.2009.470
90. Duscha BD, Slentz CA, Johnson JL, Houmard JA, Bensimhon DR, Knetzger KJ, et al. Effects of exercise training amount and intensity on peak oxygen consumption in middle-age men and women at risk for cardiovascular disease. Chest. (2005) 128:2788–93. doi: 10.1378/chest.128.4.2788
91. Anderson L, Thompson DR, Oldridge N, Zwisler AD, Rees K, Martin N, et al. Exercise-based cardiac rehabilitation for coronary heart disease. Cochr Database Syst Rev. (2016) 1:CD001800. doi: 10.1002/14651858.CD001800.pub3
92. Zhang Y, Cao H, Jiang P, Tang H. Cardiac rehabilitation in acute myocardial infarction patients after percutaneous coronary intervention: a community-based study. Medicine. (2018) 97:e9785. doi: 10.1097/MD.0000000000009785
93. Alvarez P, Hannawi B, Guha A. Exercise and heart failure: advancing knowledge and improving care. Methodist Debakey Cardiovasc J. (2016) 12:110–5. doi: 10.14797/mdcj-12-2-110
94. Silverman ME. A view from the millennium: the practice of cardiology circa 1950 and thereafter. J Am Coll Cardiol. (1999) 33:1141–51. doi: 10.1016/S0735-1097(99)00027-3
95. Haykowsky MJ, Daniel KM, Bhella PS, Sarma S, Kitzman DW. Heart Failure: Exercise-Based Cardiac Rehabilitation: who, when, and how intense? Can J Cardiol. (2016) 32(10 Suppl. 2):S382–7. doi: 10.1016/j.cjca.2016.06.001
96. Upadhya B, Haykowsky MJ, Eggebeen J, Kitzman DW. Exercise intolerance in heart failure with preserved ejection fraction: more than a heart problem. J Geriatr Cardiol. (2015) 12:294–304. doi: 10.1007/s11897-015-0257-5
97. Ades PA, Keteyian SJ, Balady GJ, Houston-Miller N, Kitzman DW, Mancini DM, et al. Cardiac rehabilitation exercise and self-care for chronic heart failure. JACC Heart Fail. (2013) 1:540–7. doi: 10.1016/j.jchf.2013.09.002
98. Piepoli MF, Davos C, Francis DP, Coats AJ, ExTra MC. Exercise training meta-analysis of trials in patients with chronic heart failure (ExTraMATCH). BMJ. (2004) 328:189. doi: 10.1136/bmj.37938.645220.EE
99. Davies EJ, Moxham T, Rees K, Singh S, Coats AJ, Ebrahim S, et al. Exercise training for systolic heart failure: cochrane systematic review and meta-analysis. Eur J Heart Fail. (2010) 12:706–15. doi: 10.1093/eurjhf/hfq056
100. Sagar VA, Davies EJ, Briscoe S, Coats AJ, Dalal HM, Lough F, et al. Exercise-based rehabilitation for heart failure: systematic review and meta-analysis. Open Heart. (2015) 2:e000163. doi: 10.1136/openhrt-2014-000163
101. Taylor RS, Sagar VA, Davies EJ, Briscoe S, Coats AJ, Dalal H, et al. Exercise-based rehabilitation for heart failure. Cochrane Database Syst Rev. (2014) 4:CD003331. doi: 10.1002/14651858.CD003331.pub4
102. O'Connor CM, Whellan DJ, Lee KL, Keteyian SJ, Cooper LS, Ellis SJ, et al. Efficacy and safety of exercise training in patients with chronic heart failure: HF-ACTION randomized controlled trial. JAMA. (2009) 301:1439–50. doi: 10.1001/jama.2009.454
103. Flynn KE, Pina IL, Whellan DJ, Lin L, Blumenthal JA, Ellis SJ, et al. Effects of exercise training on health status in patients with chronic heart failure: HF-ACTION randomized controlled trial. JAMA. (2009) 301:1451–9. doi: 10.1001/jama.2009.457
104. Asrar Ul Haq M, Goh CY, Levinger I, Wong C, Hare DL. Clinical utility of exercise training in heart failure with reduced and preserved ejection fraction. Clin Med Insights Cardiol. (2015) 9:1–9. doi: 10.4137/CMC.S21372
105. Ellingsen O, Halle M, Conraads V, Stoylen A, Dalen H, Delagardelle C, et al. High-intensity interval training in patients with heart failure with reduced ejection fraction. Circulation. (2017) 135:839–49. doi: 10.1161/CIRCULATIONAHA.116.022924
106. Pearson MJ, Smart NA. Effect of exercise training on endothelial function in heart failure patients: a systematic review meta-analysis. Int J Cardiol. (2017) 231:234–43. doi: 10.1016/j.ijcard.2016.12.145
107. Hoier B, Hellsten Y. Exercise-induced capillary growth in human skeletal muscle and the dynamics of VEGF. Microcirculation. (2014) 21:301–14. doi: 10.1111/micc.12117
108. Olver TD, Ferguson BS, Laughlin MH. Molecular mechanisms for exercise training-induced changes in vascular structure and function: skeletal muscle, cardiac muscle, and the brain. Prog Mol Biol Transl Sci. (2015) 135:227–57. doi: 10.1016/bs.pmbts.2015.07.017
109. Tao L, Bei Y, Zhang H, Xiao J, Li X. Exercise for the heart: signaling pathways. Oncotarget. (2015) 6:20773–84. doi: 10.18632/oncotarget.4770
110. Black MA, Cable NT, Thijssen DH, Green DJ. Impact of age, sex, and exercise on brachial artery flow-mediated dilatation. Am J Physiol Heart Circ Physiol. (2009) 297:H1109–16. doi: 10.1152/ajpheart.00226.2009
111. Stanford KI, R. Middelbeek JW, Townsend KL, Lee MY, Takahashi H, So K, et al. A novel role for subcutaneous adipose tissue in exercise-induced improvements in glucose homeostasis. Diabetes. (2015) 64:2002–14. doi: 10.2337/db14-0704
112. Stanford KI, Goodyear LJ. Exercise regulation of adipose tissue. Adipocyte. (2016) 5:153–62. doi: 10.1080/21623945.2016.1191307
113. Lundby C, Jacobs RA. Adaptations of skeletal muscle mitochondria to exercise training. Exp Physiol. (2016) 101:17–22. doi: 10.1113/EP085319
114. Riehle C, Wende AR, Zhu Y, Oliveira KJ, Pereira RO, Jaishy BP, et al. Insulin receptor substrates are essential for the bioenergetic and hypertrophic response of the heart to exercise training. Mol Cell Biol. (2014) 34:3450–60. doi: 10.1128/MCB.00426-14
115. Vettor R, Valerio A, Ragni M, Trevellin E, Granzotto M, Olivieri M, et al. Exercise training boosts eNOS-dependent mitochondrial biogenesis in mouse heart: role in adaptation of glucose metabolism. Am J Physiol Endocrinol Metab. (2014) 306:E519–28. doi: 10.1152/ajpendo.00617.2013
116. Kasapis C, Thompson PD. The effects of physical activity on serum C-reactive protein and inflammatory markers - A systematic review. J Am Coll Cardiol. (2005) 45:1563–9. doi: 10.1016/j.jacc.2004.12.077
117. Seldin MM, Peterson JM, Byerly MS, Wei Z, Wong GW. Myonectin (CTRP15), a novel myokine that links skeletal muscle to systemic lipid homeostasis. J Biol Chem. (2012) 287:11968–80. doi: 10.1074/jbc.M111.336834
118. Oshima Y, Ouchi N, Sato K, Izumiya Y, Pimentel DR, Walsh K. Follistatin-like 1 is an Akt-regulated cardioprotective factor that is secreted by the heart. Circulation. (2008) 117:3099–108. doi: 10.1161/CIRCULATIONAHA.108.767673
119. Ogura Y, Ouchi N, Ohashi K, Shibata R, Kataoka Y, Kambara T, et al. Therapeutic impact of follistatin-like 1 on myocardial ischemic injury in preclinical models. Circulation. (2012) 126:1728–38. doi: 10.1161/CIRCULATIONAHA.112.115089
120. Joki Y, Ohashi K, Yuasa D, Shibata R, Kataoka Y, Kambara T, et al. Neuron-derived neurotrophic factor ameliorates adverse cardiac remodeling after experimental myocardial infarction. Circ-Heart Fail. (2015) 8:342–51. doi: 10.1161/CIRCHEARTFAILURE.114.001647
121. Hawley JA, Hargreaves M, Joyner MJ, Zierath JR. Integrative biology of exercise. Cell. (2014) 159:738–49. doi: 10.1016/j.cell.2014.10.029
122. Borges JP, da Silva Verdoorn K. Cardiac ischemia/reperfusion injury: the beneficial effects of exercise. Adv Exp Med Biol. (2017) 999:155–179. doi: 10.1007/978-981-10-4307-9_10
123. Sattelmair J, Pertman J, Ding EL, Kohl HW III, Haskell W, Lee IM. Dose response between physical activity and risk of coronary heart disease: a meta-analysis. Circulation. (2011) 124:789–95. doi: 10.1161/CIRCULATIONAHA.110.010710
124. Irving BA, Lanza IR, Henderson GC, Rao RR, Spiegelman BM, Nair KS. Combined training enhances skeletal muscle mitochondrial oxidative capacity independent of age. J Clin Endocrinol Metab. (2015) 100:1654–63. doi: 10.1210/jc.2014-3081
125. Konopka AR, Suer MK, Wolff CA, Harber MP. Markers of human skeletal muscle mitochondrial biogenesis and quality control: effects of age and aerobic exercise training. J Gerontol A Biol Sci Med Sci. (2014) 69:371–8. doi: 10.1093/gerona/glt107
126. Glynn A, Fiddler H. Introduction to Exercise Physiology. In: Chambers G, editor. The Physiotherapist's Pocket Guide to Exercise: Assessment, Prescription and Training. London: Churchill Livingstone (2009).
127. Vella CA, Ontiveros D, Zubia RY. Cardiac function and arteriovenous oxygen difference during exercise in obese adults. Eur J Appl Physiol. (2011) 111:915–23. doi: 10.1007/s00421-010-1554-z
128. Judge S, Jang YM, Smith A, Selman C, Phillips T, Speakman JR, et al. Exercise by lifelong voluntary wheel running reduces subsarcolemmal and interfibrillar mitochondrial hydrogen peroxide production in the heart. Am J Physiol-Reg I. (2005) 289:R1564–72. doi: 10.1152/ajpregu.00396.2005
129. Dufour CR, Wilson BJ, Huss JM, Kelly DP, Alaynick WA, Downes M, et al. Genome-wide orchestration of cardiac functions by the orphan nuclear receptors ERRalpha and gamma. Cell Metab. (2007) 5:345–56. doi: 10.1016/j.cmet.2007.03.007
130. Tao L, Bei Y, Lin S, Zhang H, Zhou Y, Jiang J, et al. Exercise training protects against acute myocardial infarction via improving myocardial energy metabolism and mitochondrial biogenesis. Cell Physiol Biochem. (2015) 37:162–75. doi: 10.1159/000430342
131. Ferreira R, Nogueira-Ferreira R, Trindade F, Vitorino R, Powers SK, Moreira-Goncalves D. Sugar or fat: the metabolic choice of the trained heart. Metabolism. (2018) 87:98–104. doi: 10.1016/j.metabol.2018.07.004
132. Kolwicz SC Jr. An exercise in cardiac metabolism. Front Cardiovasc Med. (2018) 5:66. doi: 10.3389/fcvm.2018.00066
133. Burelle Y, Wambolt RB, Grist M, Parsons HL, Chow JC, Antler C, et al. Regular exercise is associated with a protective metabolic phenotype in the rat heart. Am J Physiol Heart Circ Physiol. (2004) 287:H1055–63. doi: 10.1152/ajpheart.00925.2003
134. Nisoli E, Clementi E, Carruba MO, Moncada S. Defective mitochondrial biogenesis: a hallmark of the high cardiovascular risk in the metabolic syndrome? Circ Res. (2007) 100:795–806. doi: 10.1161/01.RES.0000259591.97107.6c
135. Casademont J, Miro O. Electron transport chain defects in heart failure. Heart Fail Rev. (2002) 7:131–9. doi: 10.1023/A:1015372407647
136. Neubauer S. The failing heart–an engine out of fuel. N Engl J Med. (2007) 356:1140–51. doi: 10.1056/NEJMra063052
137. Doenst T, Nguyen TD, Abel ED. Cardiac metabolism in heart failure: implications beyond ATP production. Circ Res. (2013) 113:709–24. doi: 10.1161/CIRCRESAHA.113.300376
138. Rosenblatt-Velin N, Montessuit C, Papageorgiou I, Terrand J, Lerch R. Postinfarction heart failure in rats is associated with upregulation of GLUT-1 and downregulation of genes of fatty acid metabolism. Cardiovasc Res. (2001) 52:407–16. doi: 10.1016/S0008-6363(01)00393-5
139. Velez M, Kohli S, Sabbah HN. Animal models of insulin resistance and heart failure. Heart Fail Rev. (2014) 19:1–13. doi: 10.1007/s10741-013-9387-6
140. Ashrafian H, Frenneaux MP, Opie LH. Metabolic mechanisms in heart failure. Circulation. (2007) 116:434–48. doi: 10.1161/CIRCULATIONAHA.107.702795
141. Abel ED, O'Shea KM, Ramasamy R. Insulin resistance: metabolic mechanisms and consequences in the heart. Arterioscler Thromb Vasc Biol. (2012) 32:2068–76. doi: 10.1161/ATVBAHA.111.241984
142. Bird SR, Hawley JA. Update on the effects of physical activity on insulin sensitivity in humans. BMJ Open Sport Exerc Med. (2016) 2:e000143. doi: 10.1136/bmjsem-2016-000143
143. Parra V, Verdejo HE, Iglewski M, Del Campo A, Troncoso R, Jones D, et al. Insulin stimulates mitochondrial fusion and function in cardiomyocytes via the Akt-mTOR-NFkappaB-Opa-1 signaling pathway. Diabetes. (2014) 63:75–88. doi: 10.2337/db13-0340
144. Riehle C, Abel ED. Insulin signaling and heart failure. Circ Res. (2016) 118:1151–69. doi: 10.1161/CIRCRESAHA.116.306206
145. Incalza MA, D'Oria R, Natalicchio A, Perrini S, Laviola L, Giorgino F. Oxidative stress and reactive oxygen species in endothelial dysfunction associated with cardiovascular and metabolic diseases. Vascul Pharmacol. (2018) 100:1–19. doi: 10.1016/j.vph.2017.05.005
146. Bloomer RJ, Goldfarb AH, Wideman L, McKenzie MJ, Consitt LA. Effects of acute aerobic and anaerobic exercise on blood markers of oxidative stress. J Strength Cond Res. (2005) 19:276–85. doi: 10.1519/00124278-200505000-00007
147. Radak Z, Chung HY, Goto S. Systemic adaptation to oxidative challenge induced by regular exercise. Free Radic Biol Med. (2008) 44:153–9. doi: 10.1016/j.freeradbiomed.2007.01.029
148. Kalogeris T, Baines CP, Krenz M, Korthuis RJ. Cell biology of ischemia/reperfusion injury. Int Rev Cell Mol Biol. (2012) 298:229–317. doi: 10.1016/B978-0-12-394309-5.00006-7
149. Lee Y, Min K, Talbert EE, Kavazis AN, Smuder AJ, Willis WT, et al. Exercise protects cardiac mitochondria against ischemia-reperfusion injury. Med Sci Sports Exerc. (2012) 44:397–405. doi: 10.1249/MSS.0b013e318231c037
150. Camara AK, Bienengraeber M, Stowe DF. Mitochondrial approaches to protect against cardiac ischemia and reperfusion injury. Front Physiol. (2011) 2:13. doi: 10.3389/fphys.2011.00013
151. Kwon JYI, Song W, Roltsch MH, Lee Y. Mitochondrial antioxidant enzymes and endurance exercise-induced cardioprotection against ischemia-reperfusion injury. Sport Exerc Med Open J. (2018) 4:9–15. doi: 10.17140/SEMOJ-4-155
152. Calvert JW, Condit ME, Aragon JP, Nicholson CK, Moody BF, Hood RL, et al. Exercise protects against myocardial ischemia-reperfusion injury via stimulation of beta(3)-adrenergic receptors and increased nitric oxide signaling: role of nitrite and nitrosothiols. Circ Res. (2011) 108:1448–58. doi: 10.1161/CIRCRESAHA.111.241117
153. Verhaar MC, Westerweel PE, van Zonneveld AJ, Rabelink TJ. Free radical production by dysfunctional eNOS. Heart. (2004) 90:494–5. doi: 10.1136/hrt.2003.029405
154. Prior BM, Yang HT, Terjung RL. What makes vessels grow with exercise training? J Appl Physiol. (2004) 97:1119–28. doi: 10.1152/japplphysiol.00035.2004
155. Leosco D, Rengo G, Iaccarino G, Golino L, Marchese M, Fortunato F, et al. Exercise promotes angiogenesis and improves beta-adrenergic receptor signalling in the post-ischaemic failing rat heart. Cardiovasc Res. (2008) 78:385–94. doi: 10.1093/cvr/cvm109
156. Beavers KM, Brinkley TE, Nicklas BJ. Effect of exercise training on chronic inflammation. Clin Chim Acta. (2010) 411:785–793. doi: 10.1016/j.cca.2010.02.069
157. You T, Arsenis NC, Disanzo BL, Lamonte MJ. Effects of exercise training on chronic inflammation in obesity : current evidence and potential mechanisms. Sports Med. (2013) 43:243–56. doi: 10.1007/s40279-013-0023-3
158. Berg AH, Scherer PE. Adipose tissue, inflammation, and cardiovascular disease. Circ Res. (2005) 96:939–49. doi: 10.1161/01.RES.0000163635.62927.34
159. Cai D, Yuan M, Frantz DF, Melendez PA, Hansen L, Lee J, et al. Local and systemic insulin resistance resulting from hepatic activation of IKK-beta and NF-kappaB, Nat Med. (2005) 11:183–90. doi: 10.1038/nm1166
160. Ehses JA, Perren A, Eppler E, Ribaux P, Pospisilik JA, Maor-Cahn R, et al. Increased number of islet-associated macrophages in type 2 diabetes. Diabetes. (2007) 56:2356–70. doi: 10.2337/db06-1650
161. Wu H, Ballantyne CM. Skeletal muscle inflammation and insulin resistance in obesity. J Clin Invest. (2017) 127:43–54. doi: 10.1172/JCI88880
162. Song MJ, Kim KH, Yoon JM, Kim JB. Activation of Toll-like receptor 4 is associated with insulin resistance in adipocytes. Biochem Biophys Res Commun. (2006) 346:739–45. doi: 10.1016/j.bbrc.2006.05.170
163. Rogero MM, Calder PC. Obesity, inflammation, toll-like receptor 4 and fatty acids. Nutrients. (2018) 10:e432. doi: 10.3390/nu10040432
164. Shoelson SE, Lee J, Goldfine AB. Inflammation and insulin resistance. J Clin Invest. (2006) 116:1793–801. doi: 10.1172/JCI29069
165. Gregor MF, Hotamisligil GS. Inflammatory mechanisms in obesity. Annu Rev Immunol. (2011) 29:415–45. doi: 10.1146/annurev-immunol-031210-101322
166. Liu T, Zhang L, Joo D, Sun SC. NF-kappaB signaling in inflammation. Signal Transduct Target Ther. (2017) 2:17023. doi: 10.1038/sigtrans.2017.23
167. Liu HW, Chang SJ. Moderate exercise suppresses NF-kappaB signaling and activates the SIRT1-AMPK-PGC1alpha axis to attenuate muscle loss in diabetic db/db Mice. Front Physiol. (2018) 9:636. doi: 10.3389/fphys.2018.00636
168. Sriwijitkamol A, Christ-Roberts C, Berria R, Eagan P, Pratipanawatr T, DeFronzo RA, et al. Reduced skeletal muscle inhibitor of kappa B beta content is associated with insulin resistance in subjects with type 2 diabetes: reversal by exercise training - Reversal by exercise training. Diabetes. (2006) 55:760–7. doi: 10.2337/diabetes.55.03.06.db05-0677
169. Flynn MG, McFarlin BK, Markofski MM. The anti-inflammatory actions of exercise training. Am J Lifestyle Med. (2007) 1:220–35. doi: 10.1177/1559827607300283
170. Gleeson M, Bishop NC, Stensel DJ, Lindley MR, Mastana SS, Nimmo MA. The anti-inflammatory effects of exercise: mechanisms and implications for the prevention and treatment of disease. Nat Rev Immunol. (2011) 11:607–15. doi: 10.1038/nri3041
171. Lancaster GI, Febbraio MA. The immunomodulating role of exercise in metabolic disease. Trends Immunol. (2014) 35:262–9. doi: 10.1016/j.it.2014.02.008
172. Vieira VJ, Valentine RJ, Wilund KR, Antao N, Baynard T, Woods JA. Effects of exercise and low-fat diet on adipose tissue inflammation and metabolic complications in obese mice. Am J Physiol Endocrinol Metab. (2009) 296:E1164–71. doi: 10.1152/ajpendo.00054.2009
173. Cole JE, Georgiou E, Monaco C. The expression and functions of toll-like receptors in atherosclerosis. Mediators Inflamm. (2010) 2010:393946. doi: 10.1155/2010/393946
174. McFarlin BK, Flynn MG, Campbell WW, Craig BA, Robinson JP, Stewart LK, et al. Physical activity status, but not age, influences inflammatory cytokine production and toll-like receptor 4. Med Sci Sport Exer. (2006) 38:S308. doi: 10.1249/00005768-200605001-02204
175. Harutyunyan MJ, Mathiasen AB, Winkel P, Gotze JP, Hansen JF, Hildebrandt P, et al. High-sensitivity C-reactive protein and N-terminal pro-B-type natriuretic peptide in patients with stable coronary artery disease: a prognostic study within the CLARICOR Trial. Scand J Clin Lab Inv. (2011) 71:52–62. doi: 10.3109/00365513.2010.538081
176. Creber RMM, Lee CS, Margulies K, Ellis S, Riegel B. Exercise in heart failure and patterns of inflammation and myocardial stress over time. Circulation. (2014) 130:A11902.
177. Pedersen L, Hojman P. Muscle-to-organ cross talk mediated by myokines. Adipocyte. (2012) 1:164–7. doi: 10.4161/adip.20344
178. Hoffmann C, Weigert C. Skeletal muscle as an endocrine organ: the role of myokines in exercise adaptathions. Cold Spring Harb Perspect Med. (2017) 7:a029793. doi: 10.1101/cshperspect.a029793
179. Neufer PD, Bamman MM, Muoio DM, Bouchard C, Cooper DM, Goodpaster BH, et al. Understanding the cellular and molecular mechanisms of physical activity-induced health benefits. Cell Metab. (2015) 22:4–11. doi: 10.1016/j.cmet.2015.05.011
180. Pedersen BK. The diseasome of physical inactivity - and the role of myokines in muscle-fat cross talk. J Physiol-London. (2009) 587:5559–68. doi: 10.1113/jphysiol.2009.179515
181. Pedersen BK, Akerstrom TC, Nielsen AR, Fischer CP. Role of myokines in exercise and metabolism. J Appl Physiol. (2007) 103:1093–8. doi: 10.1152/japplphysiol.00080.2007
182. Pedersen BK. Muscles and their myokines. J Exp Biol. (2011) 214:337–46. doi: 10.1242/jeb.048074
183. Schnyder S, Handschin C. Skeletal muscle as an endocrine organ: PGC-1alpha, myokines and exercise. Bone. (2015) 80:115–25. doi: 10.1016/j.bone.2015.02.008
184. Ahima RS, Park HK. Connecting Myokines and Metabolism. Endocrinol Metab. (2015) 30:235–45. doi: 10.3803/EnM.2015.30.3.235
185. Baskin KK, Winders BR, Olson EN. Muscle as a “mediator” of systemic metabolism. Cell Metab. (2015) 21:237–48. doi: 10.1016/j.cmet.2014.12.021
186. Keller C, Hellsten Y, Steensberg A, Pedersen BK. Differential regulation of IL-6 and TNF-alpha via calcineurin in human skeletal muscle cells. Cytokine. (2006) 36:141–7. doi: 10.1016/j.cyto.2006.10.014
187. Kleinbongard P, Heusch G, Schulz R. TNFalpha in atherosclerosis, myocardial ischemia/reperfusion and heart failure. Pharmacol Ther. (2010) 127:295–314. doi: 10.1016/j.pharmthera.2010.05.002
188. Ellingsgaard H, Hauselmann I, Schuler B, Habib AM, Baggio LL, Meier DT, et al. Interleukin-6 enhances insulin secretion by increasing glucagon-like peptide-1 secretion from L cells and alpha cells. Nat Med. (2011) 17:1481–9. doi: 10.1038/nm.2513
189. van Hall G, Steensberg A, Sacchetti M, Fischer C, Keller C, Schjerling P, et al. Interleukin-6 stimulates lipolysis and fat oxidation in humans. J Clin Endocrinol Metab. (2003) 88:3005–10. doi: 10.1210/jc.2002-021687
190. Kelly M, Keller C, Avilucea PR, Keller P, Luo Z, Xiang X, et al. AMPK activity is diminished in tissues of IL-6 knockout mice: the effect of exercise. Biochem Biophys Res Commun. (2004) 320:449–54. doi: 10.1016/j.bbrc.2004.05.188
191. Carey AL, Steinberg GR, Macaulay SL, Thomas WG, Holmes AG, Ramm G, et al. Interleukin-6 increases insulin-stimulated glucose disposal in humans and glucose uptake and fatty acid oxidation in vitro via AMP-activated protein kinase. Diabetes. (2006) 55:2688–97. doi: 10.2337/db05-1404
192. Maruyama S, Nakamura K, Papanicolaou KN, Sano S, Shimizu I, Asaumi Y, et al. Follistatin-like 1 promotes cardiac fibroblast activation and protects the heart from rupture. EMBO Mol Med. (2016) 8:949–66. doi: 10.15252/emmm.201506151
193. Otaka N, Shibata R, Ohashi K, Uemura Y, Kambara T, Enomoto T, et al. Myonectin is an exercise-induced myokine that protects the heart from ischemia-reperfusion injury. Circ Res. (2018) 123:1326–38. doi: 10.1161/CIRCRESAHA.118.313777
194. Ouchi N, Ohashi K, Shibata R, Murohara T. Protective roles of adipocytokines and myokines in cardiovascular disease. Circ J. (2016) 80:2073–80. doi: 10.1253/circj.CJ-16-0663
195. Pedersen BK, Fischer CP. Beneficial health effects of exercise - the role of IL-6 as a myokine. Trends Pharmacol Sci. (2007) 28:152–6. doi: 10.1016/j.tips.2007.02.002
196. Pedersen BK, Febbraio MA. Muscles, exercise and obesity: skeletal muscle as a secretory organ. Nat Rev Endocrinol. (2012) 8:457–65. doi: 10.1038/nrendo.2012.49
197. Mathur N, Pedersen BK. Exercise as a mean to control low-grade systemic inflammation. Mediat Inflamm. (2008) 2008:109502. doi: 10.1155/2008/109502
198. Pedersen BK, Febbraio MA. Muscle as an endocrine organ: focus on muscle-derived interleukin-6. Physiol Rev. (2008) 88:1379–406. doi: 10.1152/physrev.90100.2007
199. Fischer CP. Interleukin-6 in acute exercise and training: what is the biological relevance? Exerc Immunol Rev. (2006) 12:6–33.
200. Yuasa D, Ohashi K, Shibata R, Mizutani N, Kataoka Y, Kambara T, et al. C1q/TNF-related protein-1 functions to protect against acute ischemic injury in the heart. Faseb J. (2016) 30:1065–75. doi: 10.1096/fj.15-279885
201. Gorgens SW, Raschke S, Holven KB, Jensen J, Eckardt K, Eckel J. Regulation of follistatin-like protein 1 expression and secretion in primary human skeletal muscle cells. Arch Physiol Biochem. (2013) 119:75–80. doi: 10.3109/13813455.2013.768270
202. Xi Y, Gong DW, Tian ZJ. FSTL1 as a Potential mediator of exercise-induced cardioprotection in post-myocardial infarction rats. Sci Rep. (2016) 6:32424. doi: 10.1038/srep32424
203. Matthews VB, Astrom MB, Chan MHS, Bruce CR, Krabbe KS, Prelovsek O, et al. Brain-derived neurotrophic factor is produced by skeletal muscle cells in response to contraction and enhances fat oxidation via activation of AMP-activated protein kinase. Diabetologia. (2009) 52:1409–18. doi: 10.1007/s00125-009-1364-1
204. Kuang XL, Zhao XM, Xu HF, Shi YY, Deng JB, Sun GT. Spatio-temporal expression of a novel neuron-derived neurotrophic factor (NDNF) in mouse brains during development. BMC Neurosci. (2010) 11:137. doi: 10.1186/1471-2202-11-137
205. Ohashi K, Enomoto T, Joki Y, Shibata R, Ogura Y, Kataoka Y, et al. Neuron-derived neurotrophic factor functions as a novel modulator that enhances endothelial cell function and revascularization processes. J Biol Chem. (2014) 289:14132–44. doi: 10.1074/jbc.M114.555789
206. Pandey A, Suskin N, Poirier P. The impact of burst exercise on cardiometabolic status of patients newly diagnosed with type 2 diabetes. Can J Cardiol. (2017) 33:1645–51. doi: 10.1016/j.cjca.2017.09.019
207. Chudyk A, Petrella RJ. Effects of exercise on cardiovascular risk factors in type 2 diabetes: a meta-analysis. Diabetes Care. (2011) 34:1228–37. doi: 10.2337/dc10-1881
208. Meyer K, Schwaibold M, Westbrook S, Beneke R, Hajric R, Gornandt L, et al. Effects of short-term exercise training and activity restriction on functional capacity in patients with severe chronic congestive heart failure. Am J Cardiol. (1996) 78:1017–22. doi: 10.1016/S0002-9149(96)00527-9
209. Maiorana A, O'Driscoll G, Cheetham C, Collis J, Goodman C, Rankin S, et al. Combined aerobic and resistance exercise training improves functional capacity and strength in CHF. J Appl Physiol. (2000) 88:1565–70. doi: 10.1152/jappl.2000.88.5.1565
210. Pena LL, Apstein CS, Balady GJ, Belardinelli R, Chaitman BR, Duscha BD, et al. Exercise and heart failure - A statement from the American Heart Association Committee on Exercise, Rehabilitation, and Prevention. Circulation. (2003) 107:1210–25. doi: 10.1161/01.CIR.0000055013.92097.40
211. Maugeri FS, Campana M, Ferrari R, Giordano A, Scalvini S, Volterrani M, et al. Experience from controlled trials of physical training in chronic heart failure - Protocol and patient factors in effectiveness in the improvement in exercise tolerance. Eur Heart J. (1998) 19:466–475. doi: 10.1053/euhj.1997.0736
212. Busquets S, Figueras M, Almendro V, Lopez-Soriano FJ, Argiles JM. Interleukin-15 increases glucose uptake in skeletal muscle. An antidiabetogenic effect of the cytokine. Biochim Biophys Acta. (2006) 1760:1613–7. doi: 10.1016/j.bbagen.2006.09.001
213. Barra NG, Chew MV, Holloway AC, Ashkar AA. Interleukin-15 treatment improves glucose homeostasis and insulin sensitivity in obese mice. Diabetes Obes Metab. (2012) 14:190–3. doi: 10.1111/j.1463-1326.2011.01495.x
214. Alvarez B, Carbo N, Lopez-Soriano J, Drivdahl RH, Busquets S, Lopez-Soriano FJ, et al. Effects of interleukin-15 (IL-15) on adipose tissue mass in rodent obesity models: evidence for direct IL-15 action on adipose tissue. Biochim Biophys Acta. (2002) 1570:33–7. doi: 10.1016/S0304-4165(02)00148-4
Keywords: exercise, obesity—complications, cardiovascular, type 2 diabetes, myokines
Citation: Pinckard K, Baskin KK and Stanford KI (2019) Effects of Exercise to Improve Cardiovascular Health. Front. Cardiovasc. Med. 6:69. doi: 10.3389/fcvm.2019.00069
Received: 05 March 2019; Accepted: 07 May 2019;
Published: 04 June 2019.
Edited by:
Jacob Haus, University of Michigan, United StatesReviewed by:
Jared Dickinson, Arizona State University, United StatesBruno Guigas, Leiden University, Netherlands
Copyright © 2019 Pinckard, Baskin and Stanford. This is an open-access article distributed under the terms of the Creative Commons Attribution License (CC BY). The use, distribution or reproduction in other forums is permitted, provided the original author(s) and the copyright owner(s) are credited and that the original publication in this journal is cited, in accordance with accepted academic practice. No use, distribution or reproduction is permitted which does not comply with these terms.
*Correspondence: Kristin I. Stanford, a3Jpc3Rpbi5zdGFuZm9yZCYjeDAwMDQwO29zdW1jLmVkdQ==