- 1VCU Pauley Heart Center, Virginia Commonwealth University, Richmond, VA, United States
- 2Department of Pharmacotherapy and Outcome Sciences, Virginia Commonwealth University, Richmond, VA, United States
- 3Department of Physical Therapy, College of Applied Health Sciences, University of Illinois, Chicago, IL, United States
Background: Impaired cardiorespiratory fitness (CRF) is a hallmark of heart failure (HF). Serum levels of C-reactive protein (CRP), a systemic inflammatory marker, and of N-terminal pro-brain natriuretic peptide (NT-proBNP), a biomarker of myocardial strain, independently predict adverse outcomes in HF patients. Whether CRP and/or NT-proBNP also predict the degree of CRF impairment in HF patients across a wide range of ejection fraction is not yet established.
Methods: Using retrospective analysis, 200 patients with symptomatic HF who completed one or more treadmill cardiopulmonary exercise tests (CPX) using a symptom-limited ramp protocol and had paired measurements of serum high-sensitivity CRP and NT-proBNP on the same day were evaluated. Univariate and multivariate correlations were evaluated with linear regression after logarithmic transformation of CRP (log10) and NT-proBNP (logN).
Results: Mean age of patients was 57 ± 10 years and 55% were male. Median CRP levels were 3.7 [1.5–9.0] mg/L, and NT-proBNP levels were 377 [106–1,464] pg/ml, respectively. Mean peak oxygen consumption (peak VO2) was 16 ± 4 mlO2•kg−1•min−1. CRP levels significantly correlated with peakVO2 in all patients (R = −0.350, p < 0.001) and also separately in the subgroup of patients with reduced left ventricular ejection fraction (LVEF) (HFrEF, N = 109) (R = −0.282, p < 0.001) and in those with preserved EF (HFpEF, N = 57) (R = −0.459, p < 0.001). NT-proBNP levels also significantly correlated with peak VO2 in all patients (R = −0.330, p < 0.001) and separately in patients with HFrEF (R = −0.342, p < 0.001) and HFpEF (R = −0.275, p = 0.032). CRP and NT-proBNP did not correlate with each other (R = 0.05, p = 0.426), but independently predicted peak VO2 (R = 0.421, p < 0.001 and p < 0.001, respectively).
Conclusions: Biomarkers of inflammation and myocardial strain independently predict peak VO2 in HF patients. Anti-inflammatory therapies and therapies alleviating myocardial strain may independently improve CRF in HF patients across a large spectrum of LVEF.
Introduction
Heart failure (HF) is a syndrome that presents clinically with dyspnea, fatigue, and/or edema caused by structural or functional cardiac defects that lead to reduced cardiac output and/or increased cardiac pressure at rest or during stress. Impaired cardiorespiratory fitness (CRF) is a hallmark of heart failure (HF) (1). CRF is defined as the ability of the circulatory, respiratory, and muscular systems to supply oxygen during sustained physical activity (2). CRF is expressed in metabolic equivalents (METs) and measured by peak oxygen uptake (peak VO2) using exercise tests (3). CRF is both an objective measure of habitual physical activity, as well as a prognostic indicator in HF.
C-reactive protein (CRP), a marker for systemic inflammation, is produced by the hepatocytes upon inflammation, infection, or tissue injury (4). Patients with HF show signs of chronic systemic inflammation, as shown by elevated serum levels of CRP (5). Increased levels of CRP are associated with an increased risk for CVD events and for mortality (6, 7). Higher CRP levels are also associated with worse cardiopulmonary exercise performance in patients with ischemic heart disease and systolic HF (8, 9).
Natriuretic peptides are peptide hormones that function as counter-regulatory mechanisms for the Renin-Angiotensin-Aldosterone-system (RAAS), and therefore cause a decrease in arterial pressure, central venous pressure, pulmonary capillary wedge pressure, cardiac output, and total blood volume through natriuresis and diuresis (10). Brain natriuretic peptide (BNP) is produced by the ventricles in response to an increase in myocardial stretch, damage, or ischemia (10). N-terminal pro-brain natriuretic peptide (NT-proBNP) is the biologically inactive peptide that is cleaved off the pro-hormone, proBNP (11). The diagnostic and prognostic power of both BNP and NT-proBNP is similar, however, NT-proBNP is less sensitive to breakdown than BNP is, which results in a more accurate measurement and reproducibility (11). Plasma levels of NT-proBNP have shown to relate to a low peak oxygen uptake (peak VO2) in HF patients (12).
Serum levels of CRP and NT-proBNP have shown to predict adverse outcomes in patients with HF, both in HF with reduced ejection fraction (HFrEF, LVEF <50%) and in HF with preserved ejection fraction (HFpEF, LVEF >50%). Although CRP and NT-proBNP provide independent and complementary insight into CRF, whether CRP and/or NT-proBNP also independently predict the degree of CRF impairment in HF patients is not yet established. We hypothesize that two biomarkers, CRP, and NT-proBNP, by acting as surrogates for different pathophysiologic mechanisms, inflammation and myocardial strain, respectively, will independently predict the degree of CRF impairment in patients with HF across the spectrum of LVEF including both HFrEF and HFpEF. The objective of this study was to investigate whether CRP and/or NT-proBNP can independently predict CRF impairment, defined as reduced peak VO2 in patients with HF across a wide range of ejection fraction.
Materials and Methods
Study Design
We retrospectively queried a database of de-identified data that was prospectively collected data from patients with symptomatic HF who completed one or more cardiopulmonary exercise tests (CPX) using a symptom-limited ramp protocol on a treadmill and had paired measurements of serum high-sensitivity CRP and NT-proBNP on the same day. All members of the research team have completed training on the ethical conduct of research on human subjects. The VCU Institutional Review Board approved of the study, which was conducted according to the International Conference on Harmonization Good Clinical Practice Guidelines and the Declaration of Helsinki.
Cardiopulmonary Exercise Testing
All patients underwent maximal CPX with a certified exercise physiologist under the supervision of a physician with a metabolic cart connected to a treadmill (Vmax Encore, Viasys, Yora Linda, CA) using a ramp protocol, as described before (13). Oxygen and carbon dioxide sensors were calibrated before the test with known oxygen, nitrogen, and carbon dioxide concentrations and the flow sensor was calibrated using a 3-L syringe. Subjects were asked to exercise to maximal fatigue. Twelve-lead ECG measurements were done at baseline, throughout the test, and during recovery. Every 2 min blood pressure was measured using an automated exercise compatible device (Tango, SunTech Medical). Expired gases were sampled during exercise with a mouthpiece-mounted sensor and were analyzed continuously to measure oxygen consumption (VO2), carbon dioxide production (VCO2), and minute ventilation (VE). The peak VO2 (mlO2•kg−1•min−1) during exercise was defined as the highest 10-s average value for VO2 during the last 30 s of the exercise. Peak VO2 measured during exercise is the most objective variable for assessment of functional capacity as is an important prognostic indicator (3). American Heart Association/American College of Cardiology guidelines for exercise testing contraindications and termination criteria were followed.
Doppler Echocardiography
Subjects underwent transthoracic Doppler echocardiogram. Echocardiography was performed according to the American Society of Echocardiography measurement guidelines and provides information on both cardiac dimensions and function (14). LV end-diastolic and end-systolic volumes (LVEDV, LVESV), EF, and early transmitral E wave velocities were obtained. Early mitral annulus (e′) velocities obtained by tissue Doppler were averaged between lateral and septal e′ and tricuspid annulus plane systolic excursion. The E/e′ ratio provides information on diastolic function and was calculated to estimate LV filling pressures (15, 16).
Biomarker Analysis
We analyzed differential comprehensive metabolic profile and plasma levels of biomarkers including high-sensitivity CRP and NT-proBNP. CRP, a marker for systemic inflammation, is increased in HF and has shown to relate to poor exercise performance (8, 9). CRP values <3.0 mg/L were considered within normal range. CRP values >3.0 mg/L have shown to be associated with an increased risk of cardiovascular disease (6). N-terminal pro-brain natriuretic peptide (NT-proBNP), a biomarker of myocardial strain, correlates with exercise capacity in HF (12). NT-proBNP values <300 ng/ml were considered within normal range (17). Both CRP and NT-proBNP independently predict adverse outcomes in patients with HF (7, 18, 19). Furthermore, we analyzed White Blood Cell (WBC) count, absolute neutrophils, and leukocyte count. Neutrophil to leukocyte ratio (NLR) is a measure for systemic inflammation (20). A NLR >4 has prognostic value in cardiovascular disease (21).
Data Analysis
For the data analysis, each of the tests were considered as a separate measurement for data analysis. We assessed the correlation between different clinical parameters and peak oxygen consumption (peak VO2), as the preferred measures of CRF obtained during effort-limited maximal cardiopulmonary exercise testing (CPX). This was done for the entire HF group and then analyzed separately for 2 groups of patients stratified according to LVEF <50% (HFrEF) or ≥50% (HFpEF). According to the Fick principle, peak VO2 is determined by stroke volume, heart rate, and arterial-venous oxygen difference and therefore reflects both cardiac, vascular, and peripheral skeletal muscle components. The cause of exercise intolerance has been proposed to be different in HFrEF and HFpEF patients, therefore we stratified according to LVEF (22).
Statistical Analysis
Data was tested for deviation from Gaussian distribution using the Kolmogorov–Smirnov test, and due to a lack of deviation, is presented as mean and standard deviation. Data that deviated from Gaussian distribution is presented as median and interquartile range. For normally distributed continuous variables, differences between groups were evaluated using independent-samples t-test, and for not normally distributed variables with the Mann–Whitney U-test. Correlations between continuous variables were assessed using linear regression. Multivariate analysis using stepwise linear regression was used to assess predictors of CRF with available clinical parameters. A ROC curve analysis was performed to evaluate whether the biomarkers had discriminative value for reduced CRF defined as peak VO2 <10 mlO2•kg−1•min−1and peak VO2 <14 mlO2•kg−1•min−1. SPSS Statistics 24.0 (IBM, Armonk, NY) statistical software package was used for all analyses. No missing data imputation method used. A p-value < 0.05 was considered statistically significant.
Results
We evaluated a total of 366 CPX from 200 different patients (2.0 ± 1.3 studies per patient) of which the clinical characteristics are shown in Table 1 for all patients. Clinical characteristics are shown separately for patients with HF with reduced LVEF (LVEF <50%) and for patients with HF with preserved LVEF HFpEF (LVEF ≥50%) in Table 2. Mean age of patients was 57 ± 10 years and 110 (55%) were male. Mean LVEF was 44 ± 14% (HFrEF, N = 109 and HFpEF, N = 57) (Figure 1), with a mean LVEF of 36 ± 11% in HFrEF, and 58 ± 6% in HFpEF. Median high-sensitivity CRP levels were 3.7 [1.5–9.0] mg/L, respectively and median NT-proBNP levels were 377 [106–1,464] pg/ml, respectively. In HFrEF, median CRP levels were 4.2 [1.9–9.2] mg/L, and median NT-proBNP levels were 1,029 [280–2,263] pg/ml. In HFpEF, median CRP levels were 3.7 [0.6–10.8] mg/L and median NT-proBNP levels were 102 [46–183] pg/ml, respectively (P < 0.001 for NT-proBNP, P = 0.03 for CRP between HFpEF and HFrEF). The distribution of LVEF, CRP, and NT-proBNP is shown in Figure 1. Mean White Blood Cell (WBC) count and median Neutrophil to Leukocyte Ratio (NLR) was 7.2 (2.4) × 109/L and 2.3 [1.6–3.1], with a mean WBC count and median NLR of 7.4 (2.6) × 109/L and 2.0 [1.5–2.7] in HFpEF, and 6.9 (2.1) × 109/L and 2.3 [1.6–3.2] in HFrEF, respectively, (P = 0.198 for WBC count, P = 0.07 for NLR). Mean peak oxygen consumption (peak VO2) was 16 ± 4 mlO2•kg−1•min−1and mean treadmill exercise time (TET) was 8.7 ± 2.8 min.
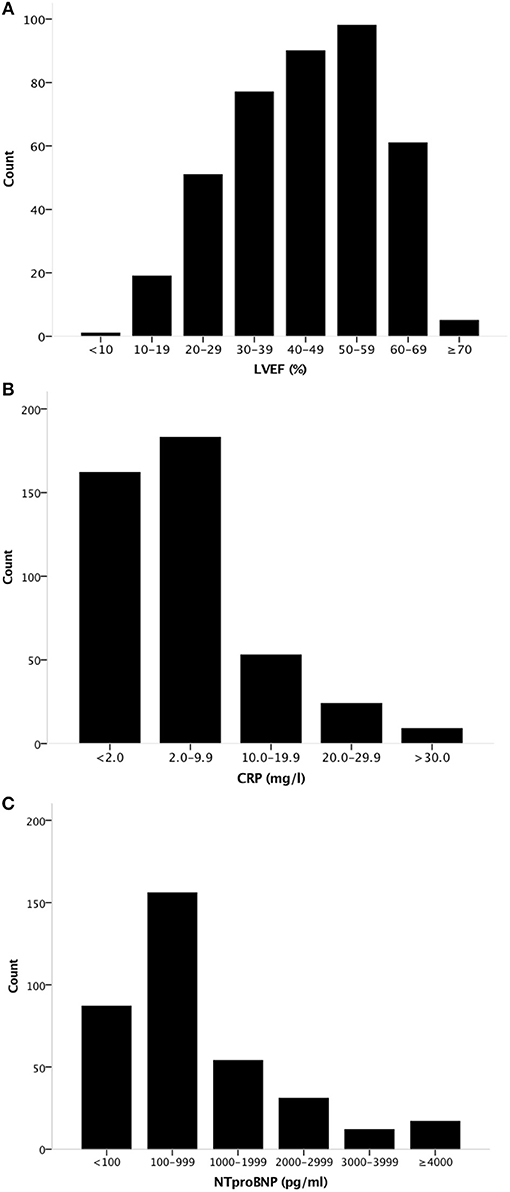
Figure 1. Distribution of LVEF, CRP, and NT-proBNP levels in the population. Histogram showing the distribution of (A) LVEF, (B) CRP levels, and (C) NT-proBNP levels in the population. LVEF, Left Ventricular Ejection Fraction.
CRP as a Predictor of CRF
CRP levels significantly and inversely correlated with peak VO2 (R = −0.350, p < 0.001, N = 316), and with TET (R = −0.342, p < 0.001, N = 314) as shown in Figure 2. The association between CRP and peak VO2 and between CRP and TET remained significant when the analysis was limited to HFrEF patients (R = −0.282, p < 0.001, N = 192 for peak VO2 and R = 0.336, p < 0.001, N = 190 for TET) and to HFpEF patients (R = −0.459, p < 0.001, N = 90 for peak VO2 and R = −0.345, p < 0.01, N = 90 for TET).
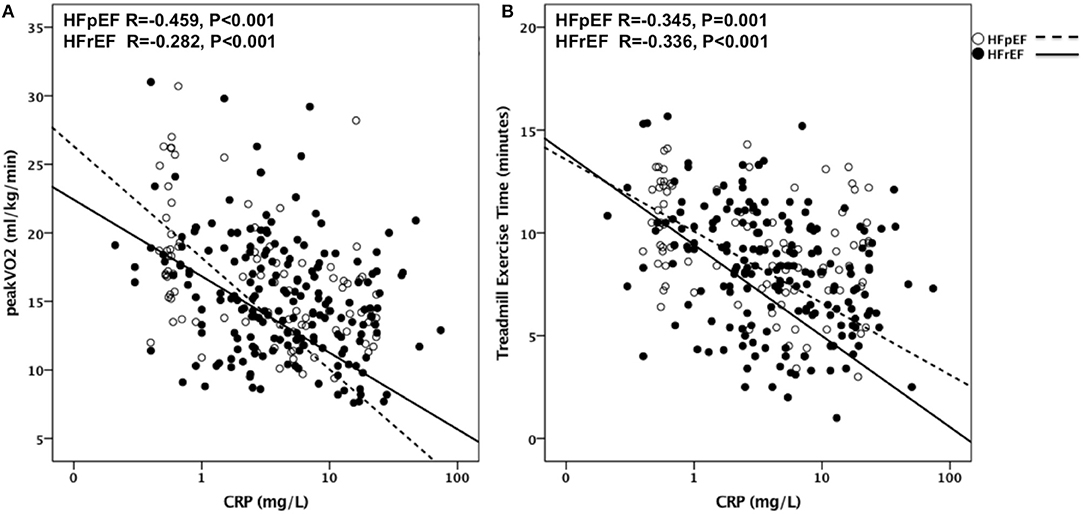
Figure 2. CRP as predictor of CRF in HFrEF and HFpEF. Correlations are shown in (A) between CRP and Peak VO2 in patients with HFpEF (R = −0.459, P < 0.001) and with HFrEF (R = −0.282, P < 0.001) and in (B) between CRP and TET in patients with HFpEF (R = −0.345, P < 0.001) and with HFrEF (R = −0.336, P < 0.001). VO2, Oxygen uptake; HFpEF, Heart Failure with preserved Ejection Fraction; HFrEF, Heart Failure with reduced Ejection Fraction; TET, Treadmill Exercise Time.
NT-proBNP as a Predictor of CRF
NT-proBNP levels significantly and inversely correlated with peak VO2 (R = −0.330, p < 0.001, N = 258) and with TET (R = −0.412, p < 0.001, N = 256) as shown in Figure 3. The association between NT-proBNP and peak VO2 and between NT-proBNP and TET remained significant when the analysis was limited to HFrEF patients (R = −0.354, p < 0.001, N = 168 for peak VO2 and R = −0.437, p < 0.001, N = 166 for TET) as well as in HFpEF patients (R = −0.275, p = 0.032, N = 61 for peak VO2 and R = −0.459, p < 0.001, N = 61 for TET).
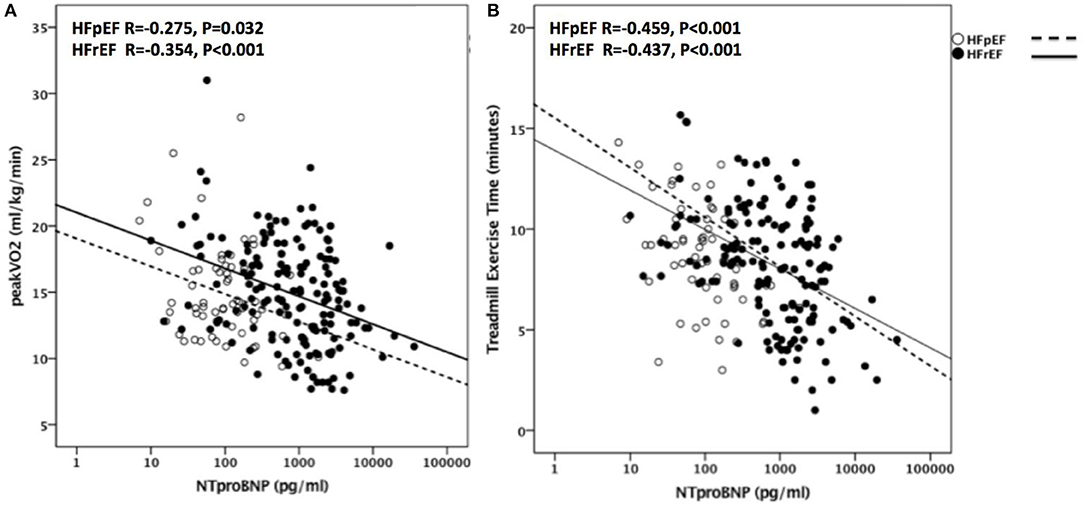
Figure 3. NT-proBNP as predictor of CRF in HFrEF and HFpEF. Correlations are shown in (A) between NT-proBNP and Peak VO2 in patients with HFpEF (R = −0.275, P = 0.032) and with HFrEF (R = −0.354, P < 0.001) and in (B) between NT-proBNP and TET in patients with HFpEF (R = −0.459, P < 0.001) and with HFrEF (R = −0.437, P < 0.001). VO2, oxygen uptake; HFpEF, Heart Failure with preserved Ejection Fraction; HFrEF, Heart Failure with reduced Ejection Fraction; TET, Treadmill Exercise Time.
Multivariate Analysis
Multivariate analysis including CRP and NT-proBNP showed that both CRP and NT-proBNP independently predicted peak VO2 (R = 0.421, p < 0.001 and p < 0.001, respectively), and TET (R = 0.478, p < 0.001, and p < 0.001, respectively). CRP and NT-proBNP did not exhibit collinearity (R = +0.05, p = 0.426, N = 255). When the analysis was limited to HFrEF patients, CRP and NT-proBNP predicted peak VO2 (R = 0.432, p < 0.001, p < 0.001, respectively) and TET (R = 0.496, p < 0.001, p < 0.001, respectively). When the analysis was limited to HFpEF patients, NT-proBNP predicted peak VO2 independent from CRP (R = 0.287, p = 0.5 for CRP, p = 0.04 for NT-proBNP) and TET (R = 0.459, p = 0.988 for CRP, p < 0.001 for NT-proBNP). Furthermore, multivariate analysis including NT-proBNP, BMI, age, and sex significantly predicted peak VO2 (R = 0.631, p < 0.001, p < 0.001, p = 0.04, respectively, with a trend for sex (p = 0.08). NT-proBNP, CRP, BMI, age, and sex significantly predicted TET (R = 0.651, p < 0.001, p = 0.006, p < 0.001, p < 0.001, p = 0.029, respectively). When the analysis was limited to HFrEF patients, BMI, age, sex, NT-proBNP, and CRP predicted Peak VO2 (R = 0.669, p < 0.001, p < 0.001, p < 0.001, p < 0.001, p = 0.03, respectively) and TET (R = 0.716, p < 0.001, p < 0.001, p < 0.001, p < 0.001, p = 0.002, respectively). When the analysis was limited to HFpEF patients, BMI, and age significantly predicted peak VO2 (R = 0.669, p < 0.001, and p < 0.001, respectively) and BMI, age, and NT-proBNP predicted TET (R = 0.680 p < 0.001, p = 0.017, p = 0.03, respectively).
ROC Curve Analysis
A ROC curve analysis was performed to evaluate whether CRP and NT-proBNP have discriminative value for reduced CRF, defined as peak VO2 <10 mlO2•kg−1•min−1and peak VO2 <14 mlO2•kg−1•min−1(Figure 4). For peak VO2 <10 mlO2•kg−1•min−1, the area under the curve (AUC) = 0.660 95% CI [0.544–0.776], P = 0.014 for CRP and AUC = 0.749 95% CI [0.669–0.829], P < 0.001 for NT-proBNP. For peak VO2 <14 mlO2•kg−1•min−1, AUC = 0.658 95% CI [0.597–0.718], P < 0.001 for CRP and AUC = 0.608 95% CI [0.537–0.678] P = 0.003 for NT-proBNP.
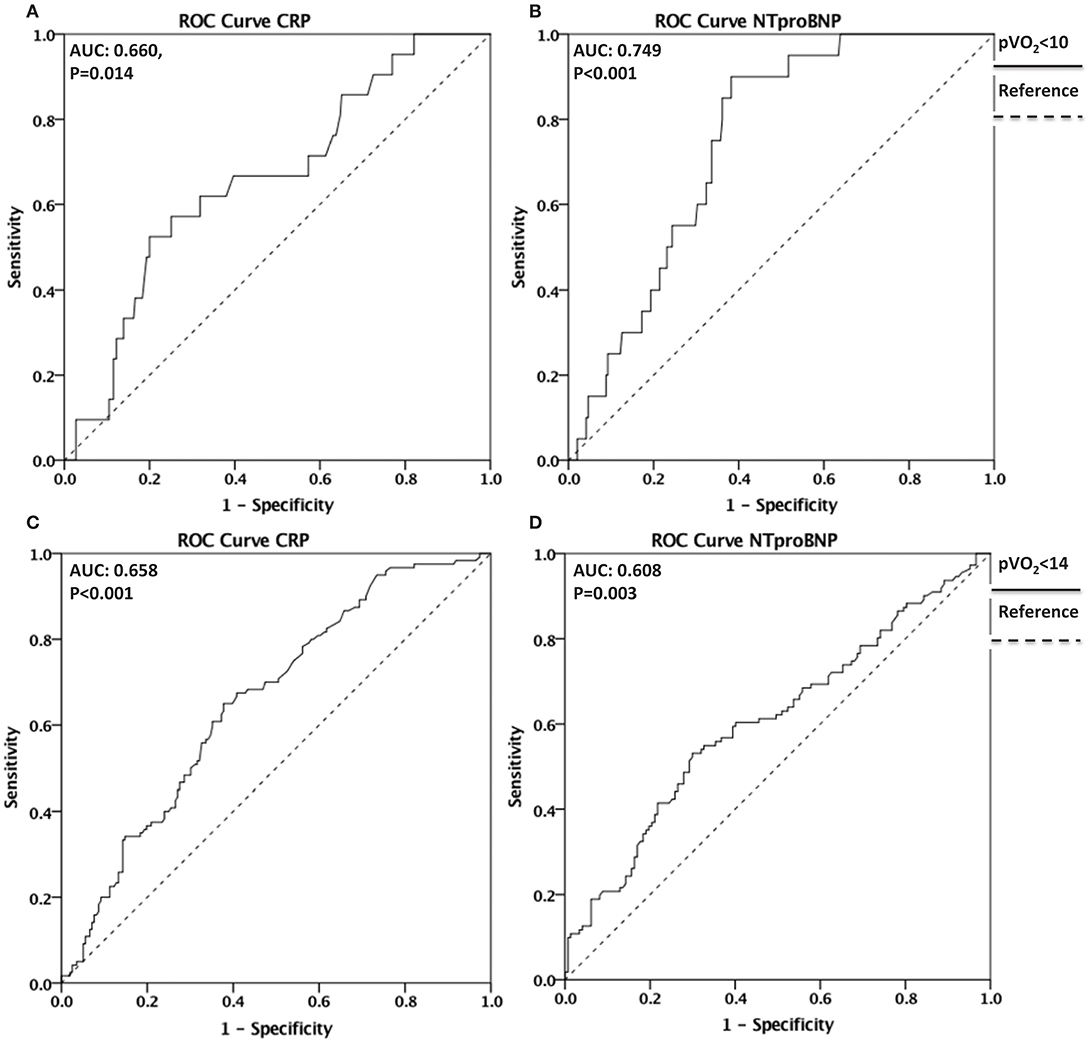
Figure 4. Discriminative value of CRP and NT-proBNP for reduced CRF. ROC curves depicting the discriminative value of CRP in (A) and of NT-proBNP in (B) for reduced CRF defined as Peak VO2 <10 mlO2•kg−1•min−1(AUC = 0.660, P = 0.014 for CRP and AUC = 0.749, P < 0.001 for NT-proBNP). ROC curves depicting the discriminative value of CRP in (C) and NT-proBNP in (D) for reduced CRF defined as Peak VO2 <14 mlO2•kg−1•min−1 (AUC = 0.658, P < 0.001 for CRP and AUC = 0.608, P = 0.003 for NT-proBNP). ROC, Receiver operating characteristic; CRF, Cardiorespiratory Fitness; VO2, oxygen uptake; AUC. Area Under the Curve.
Discussion
CRF is an important determinant of quality of life and prognostic indicator in patients with HF (23, 24). In the current study, we show that systemic inflammation, as measured by elevated CRP levels, and myocardial strain, as indicated by elevated NT-proBNP levels, independently predict impaired CRF in patients with HF, reflected in reduced peak VO2. Both CRP and NT-proBNP show a high discriminative value for reduced CRF as defined by peak VO2 <10 mlO2•kg−1•min−1and peak VO2 <14 mlO2•kg−1•min−1.
Systemic Inflammation in Heart Failure
HF is characterized by systemic inflammation, as shown by elevated circulating levels of inflammatory biomarkers in patients that increase with progression of the disease (25). For one, systemic inflammation may be the result of HF by means of tissue hypoperfusion and neurohormonal activation (26), or inflammation may play a pathophysiologic role in HF (27, 28). It is suggested that the pro-inflammatory state contributes to the development and progression of HF, not only by impairing myocardial function but also by affecting other organs and tissues and thereby adding to other aspects of the HF syndrome including cachexia and anemia (25).
The preferred inflammatory biomarker in cardiovascular disease is CRP (29, 30). HF patients have elevated CRP levels and such levels tend to increase with clinical decompensation and predict worse outcomes (5, 31, 32). Elevated CRP levels reflect inflammatory and immune deregulation in HF (31, 33). Elevated CRP levels also correlate with worse cardiac function (34, 35), and worse functional capacity in patients with ischemic heart disease and systolic HF (8, 9). The association between elevated CRP levels and HF is however complex and likely only incompletely understood. CRP is produced in the liver in response to cytokines such as Interleukin-6 (IL-6) (36). IL-6 is considered a secondary cytokine produced by myeloid cells in response to Interleukin-1 (IL-1) and Tumor Necrosis Factor-α (TNF-α) (36). Elevated IL-1 and TNF-α levels have been reported in patients with HF (33, 37). Moreover, IL-1 and TNF-α are also known as soluble cardiodepressant factors in patients with sepsis (38). More recently enhanced IL-1 activity has been described in patients with acute decompensated HF (31). IL-1 induces a reversible left ventricular systolic dysfunction in the mouse that is characterized by β-adrenergic receptor desensitization and impaired contractile reserve (39). This data points to an active role of IL-1 in the pathophysiology of HF.
Myocardial Strain
Elevated NT-proBNP levels reflect myocardial strain due to increased pressure, however, levels may also increase in response to other insults such as ischemia or inflammatory cytokines. NT-proBNP has shown to predict adverse outcomes in HF patients (10). Although BNP exerts protective effects on the heart during HF, the circulating levels of BNP or NT-proBNP reflect worse hemodynamics (elevated filling pressures) and neurohormonal activation (40). A lack of endogenous BNP response facilitates the onset of HF in animal experimental models (41), whereas potentiation of the BNP effects using recombinant BNP or neprylisin inhibitors help prevent HF (42).
Limitations
The retrospective nature of the study limited the power of our analyses, specifically in the HFpEF cohort for which the sample size was smaller than for HFrEF patients. Another limitation of our study is that every visit in the data analysis was used as separate data point, which results in some patients being represented more than once in the database. Having patients represented more than once can alter the representation of the patient population, as a single patient could have provided more than one data entry leading to an over-representation of their specific clinical characteristics. Lastly, we were not able to address the role of other biomarkers for inflammation in HF in our study.
Potential Implications for Diagnosis, Risk Stratification, and Treatment
According to our findings, both elevated CRP and NT-proBNP levels are independently predictive of impaired CRF in HF and predictive of moderately or severe reduction in peak VO2. A scoring system that would include both biomarkers is therefore likely to yield a better discrimination than only one of the markers. In non-ST elevation ACS, CRP, and BNP in combination with troponin I were predictive of mortality, MI and CHF. Further, a combination of the biomarkers provided additional prognostic value (43). These observations were validated in a cohort of 1,635 patients in the TACTICS-TIMI 18 study, and after adjustment for known clinical predictors, the number of elevated biomarkers remained predictive of the composite end point. Specifically, patients with one, two, and three elevated biomarkers had a 2.1, 3.1, and 3.7-fold increase in the risk of death, MI, or development of CHF after 6 months (43). A scoring system that could utilize the prognostic power of both CRP and NT-proBNP would allow for risk stratification beyond that solely provided by each of the markers used separately to predict CRF in patients with HF across a wide range of LVEF.
Inhibiting systemic inflammation with anti-inflammatory therapies and alleviating myocardial strain may represent two independent therapeutic strategies to improve CRF in patients with HF. Phase II studies have started exploring the effects of Interleukin-1 blockers in HF. A pilot feasibility study in 7 patients was conducted to test the efficacy of Anakinra on CP exercise performance in patients with HF and evidence of CRP. CRP levels were greatly reduced and peak VO2 significantly improved (31). In the REDHART sub study, 60 patients with HFrEF and elevated CRP were randomly assigned to daily subcutaneous injections of Anakinra 100 mg for weeks, 12 weeks, or placebo (44). Treatment with Anakinra did not affect peak VO2 or VE/VCO2 slope at 2 weeks; however, patients showed improvement in peak VO2 when assigned to the 12-week group. Further, the incidence of death or rehospitalization for HF at 24 weeks was 6, 31, and 30% for the Anakinra 12-week, Anakinra 2-week, and placebo groups, respectively. In the D-HART pilot study, the effects of IL-1 blockade with Anakinra on aerobic exercise capacity and CRP in patients with HFpEF were examined (45). Anakinra led to a statistically significant improvement in peak VO2 consumption and a significant reduction in plasma CRP levels.
In a follow up study, the Diastolic Heart Failure Anakinra Response Trial-2 (DHART-2), patients with stable symptomatic HFpEF were treated with Anakinra to confirm the effects on peak VO2 and CRP and observe its effects on serum NT-proBNP (46). Twenty-eight patients completed two visits or more and Anakinra was found to significantly reduce CRP as well as NT-proBNP levels. After 12 weeks of IL-1 blockade with Anakinra, NT-proBNP was reduced at a magnitude that correlated with CRP reduction. Anakinra however failed to increase peak VO2 in the DHART2 study. The potential benefits of IL-1 blockade in patients with heart disease is further supported by the results of the phase III Canakinumab Antiinflammatory Thrombosis Outcome Study (CANTOS) (47), in which patients with prior acute myocardial infarction were randomized to canakinumab, IL-1β blocker, or placebo, and showed a significant reduction in major adverse cardiac events. A small single-center sub-study of the CANTOS trial showed a significant improvement in peak VO2 in canakinumab-treated patients at 3 and 12 months (46).
Neprilysin inhibitors have also provided a novel therapeutic strategy to combat HF symptoms and promote CRF. Another study was completed to compare the effects of Candoxatril (novel neutral endopeptidase inhibitor) with those of Furosemide in the treatment of patients with mild HF (37). Male patients with mild HF were randomly assigned to treatment with 20 mg of Furosemide twice a day, 200 mg of Candoxatril twice a day, or 400 mg of Candoxatril twice a day, for 9 days. For patients assigned to Furosemide, treadmill exercise capacity decreased by 30 ± 26 s compared to an increase of 12 ± 35 and of 35 ± 31 s for 200 mg of Candoxatril twice a day and 400 mg of Candoxatril twice a day, respectively (37). A pilot study was completed to evaluate the short-term effects of sacubitril/valsartan on maximal exercise capacity evaluated by peak VO2 in stable patients with symptomatic HFrEF, with a secondary end point looking at changes in the VE/VCO2 slope. When compared with baseline peak VO2, patients experienced a significant increase in peak VO2 at 30 days (+0.92 mlO2•kg−1•min−1), which corresponded to a 7.9% increase (38). These beneficial effects on CRF, fit well with the overall favorable effects of sacubitril/valsartan on major adverse cardiovascular events and cardiac death in the Prospective Comparison of ARNI (Angiotensin Receptor-Neprilysin Inhibitor) with ACEI (Angiotensin-Converting-Enzyme Inhibitor) to Determine Impact on Global Mortality and Morbidity in Heart Failure (PARADIGM-HF) trial (48).
Conclusion
Biomarkers of inflammation and myocardial strain independently predict reduced peak VO2 in HF patients. Anti-inflammatory therapies and therapies alleviating myocardial strain may independently improve CRF in HF patients across a large spectrum of LVEF.
Ethics Statement
The study was completed with a de-identified database available at VCU. The research was considered exempt from Institutional Review Board review as per VCU IRB guidelines.
Author Contributions
JC, SC, CT, DK, LB, HB, GW, BV, and AA clinical data collection. JW, KR, and AA database analysis, drafting of the manuscript. LB, MB, MV, and RA critical revision of the manuscript.
Conflict of Interest Statement
AA has served as a consultant to Novartis, Swedish Orphan Biovitrum, Janssen, Merck, and Olatec. BV has served as consultant to Novartis.
The remaining authors declare that the research was conducted in the absence of any commercial or financial relationships that could be construed as a potential conflict of interest.
Acknowledgments
AA and BV are supported by the United States of America National Heart & Lung Institute award (1R61HL139943). AA has served as a consultant to Novartis, Swedish Orphan Biovitrum, Janssen, Merck, and Olatec. BV has served as consultant to Novartis.
Abbreviations
CRF, Cardiorespiratory Fitness; HF, Heart Failure; HFrEF, Heart Failure with Reduced Ejection Fraction; HFpEF, Heart Failure with Preserved Ejection Fraction; METs, Metabolic Equivalents; VO2, Oxygen Consumption; CRP, C-Reactive Protein; NT-proBNP, N-Terminal Pro-Brain Natriuretic Peptide; WBC, White Blood Cell; NLR, Neutrophil to Leukocyte Ratio; ANP, Atrial Natriuretic Peptide; CVD, Cardiovascular Disease; CVP, Central Venous Pressure; RAAS, Renin Angiotensin Aldosterone System; LVEF, Left Ventricular Ejection Fraction; LVEDV, Left Ventricular End Diastolic Volume; LVESV, Left Ventricular End Systolic Volume; CPX, Cardiopulmonary Exercise Test; TET, Treadmill Exercise Time; Peak VO2, Peak Oxygen Consumption; VE/VCO2, Ventilatory Efficiency; ACS, Acute Coronary Syndrome; MI, Myocardial Infarction; ARNI, Angiotensin Receptor-Neprilysin Inhibitor; ACEI, Angiotensin-Converting-Enzyme Inhibitor; PARADIGM-HF, Prospective Comparison of ARNI with ACEI to Determine Impact on Global Mortality and Morbidity in Heart Failure; CANTOS, Canakinumab Antiinflammatory Thrombosis Outcome Study; ROC, Receiver Operating Characteristic; AUC, Area Under the Curve.
References
1. Kondamudi N, Haykowsky M, Forman DE, Berry JD, Pandey A. Exercise training for prevention and treatment of heart failure. Prog Cardiovasc Dis. (2017) 60:115–20. doi: 10.1016/j.pcad.2017.07.001
2. Lavie CJ, Arena R, Swift DL, Johannsen NM, Sui X, Lee DC, et al. Exercise and the cardiovascular system: clinical science and cardiovascular outcomes. Circ Res. (2015) 117:207–19. doi: 10.1161/CIRCRESAHA.117.305205
3. Balady GJ, Arena R, Sietsema K, Myers J, Coke L, Fletcher GF, et al. Clinician's guide to cardiopulmonary exercise testing in adults: a scientific statement from the American heart association. Circulation (2010) 122:191–225. doi: 10.1161/CIR.0b013e3181e52e69
4. Libby P, Ridker PM, Maseri A. Inflammation and atherosclerosis. Circulation (2002) 105:1135–43. doi: 10.1161/hc0902.104353
5. Van Tassell BW, Abouzaki NA, Oddi Erdle C, Carbone S, Trankle CR, Melchior RD, et al. Interleukin-1 blockade in acute decompensated heart failure: a randomized, double-blinded, placebo-controlled pilot study. J Cardiovasc Pharmacol. (2016) 67:544–51. doi: 10.1097/FJC.0000000000000378
6. Emerging Risk Factors Collaboration, Kaptoge S, Di Angelantonio E, Lowe G, Pepys MB, Thompson SG, et al. C-reactive protein concentration and risk of coronary heart disease, stroke, and mortality: an individual participant meta-analysis. Lancet (2010) 375:132–40. doi: 10.1016/S0140-6736(09)61717-7
7. Ridker PM, MacFadyen JG, Everett BM, Libby P, Thuren T, Glynn RJ, et al. Relationship of C-reactive protein reduction to cardiovascular event reduction following treatment with canakinumab: a secondary analysis from the CANTOS randomised controlled trial. Lancet (2017) 391:319–28. doi: 10.1016/S0140-6736(17)32814-3
8. Canada JM, Fronk DT, Cei LF, Carbone S, Erdle CO, Abouzaki NA, et al. Usefulness of C-reactive protein plasma levels to predict exercise intolerance in patients with chronic systolic heart failure. Am J Cardiol. (2016) 117:116–20. doi: 10.1016/j.amjcard.2015.10.020
9. Rahimi K, Secknus MA, Adam M, Hayerizadeh BF, Fiedler M, Thiery J, et al. Correlation of exercise capacity with high-sensitive C-reactive protein in patients with stable coronary artery disease. Am Heart J. (2005) 150:1282–9. doi: 10.1016/j.ahj.2005.01.006
10. de Lemos JA, McGuire DK, Drazner MH. B-type natriuretic peptide in cardiovascular disease. Lancet (2003) 362:316–22. doi: 10.1016/S0140-6736(03)13976-1
11. Pan Y, Li D, Ma J, Shan L, Wei M. NT-proBNP test with improved accuracy for the diagnosis of chronic heart failure. Medicine (2017) 96:e9181. doi: 10.1097/MD.0000000000009181
12. Felker GM, Whellan D, Kraus WE, Clare R, Zannad F, Donahue M, et al. N-terminal pro-brain natriuretic peptide and exercise capacity in chronic heart failure: data from the heart failure and a controlled trial investigating outcomes of exercise training (HF-ACTION) study. Am Heart J. (2009) 158(4 Suppl.):S37–44. doi: 10.1016/j.ahj.2009.07.011
13. Arena R, Humphrey R, Peberdy MA, Madigan M. Predicting peak oxygen consumption during a conservative ramping protocol implications for the heart failure population. J Cardiopulm Rehabil. (2003) 23:183–9. doi: 10.1097/00008483-200305000-00004
14. Porter TR, Shillcutt SK, Adams MS, Desjardins G, Glas KE, Olson JJ, et al. Guidelines for the use of echocardiography as a monitor for therapeutic intervention in adults: a report from the american society of echocardiography. J Am Soc Echocardiogr. (2015) 28:40–56. doi: 10.1016/j.echo.2014.09.009
15. Barmeyer A, Müllerleile K, Mortensen K, Meinertz T. Diastolic dysfunction in exercise and its role for exercise capacity. Heart Fail Rev. (2009) 14:125–34. doi: 10.1007/s10741-008-9105-y
16. Terzi S, Sayar N, Bilsel T, Enc Y, Yildirim A, Ciloglu F, et al. Tissue doppler imaging adds incremental value in predicting exercise capacity in patients with congestive heart failure. Heart Vessels (2007) 22:237–44. doi: 10.1007/s00380-006-0961-x
17. van Kimmenade RR, Pinto YM, Bayes-Genis A, Lainchbury JG, Richards AM, Januzzi JL. Usefulness of intermediate amino-terminal pro-brain natriuretic peptide concentrations for diagnosis and prognosis of acute heart failure. Am J Cardiol. (2006) 98:386–90. doi: 10.1016/j.amjcard.2006.02.043
18. Yin WH, Chen JW, Jen HL, Chiang MC, Huang WP, Feng AN, et al. Independent prognostic value of elevated high-sensitivity C-reactive protein in chronic heart failure. Am Heart J. (2004) 147:931–8. doi: 10.1016/j.ahj.2003.11.021
19. Park JJ, Choi DJ, Yoon CH, Oh IY, Jeon ES, Kim JJ, et al. Prognostic value of C-reactive protein as an inflammatory and n-terminal probrain natriuretic peptide as a neurohumoral marker in acute heart failure (from the Korean heart failure registry). Am J Cardiol. (2014) 113:511–7. doi: 10.1016/j.amjcard.2013.10.022
20. Ackland GL, Minto G, Clark M, Whittle J, Stephens RCM, Owen T, et al. Autonomic regulation of systemic inflammation in humans: a multi-center, blinded observational cohort study. Brain Behav Immun. (2018) 67:47–53. doi: 10.1016/j.bbi.2017.08.010
21. Uthamalingam S, Patvardhan EA, Subramanian S, Ahmed W, Martin W, Daley M, et al. Utility of the neutrophil to lymphocyte ratio in predicting long-term outcomes in acute decompensated heart failure. Am J Cardiol. (2011) 107:433–8. doi: 10.1016/j.amjcard.2010.09.039
22. Dhakal BP, Malhotra R, Murphy RM, Pappagianopoulos PP, Baggish AL, Weiner RB, et al. Mechanisms of exercise intolerance in heart failure with preserved ejection fraction: the role of abnormal peripheral oxygen extraction. Circ Hear Fail. (2015) 8:286–94. doi: 10.1161/CIRCHEARTFAILURE.114.001825
23. Kupsky DF, Ahmed AM, Sakr S, Qureshi WT, Brawner CA, Blaha MJ, et al. Cardiorespiratory fitness and incident heart failure: the henry ford exercise testing (FIT) project. Am Heart J. (2017) 185:35–42. doi: 10.1016/j.ahj.2016.12.006
24. Szlachcic J, Massie BM, Kramer BL, Topic N, Tubau J. Correlates and prognostic implication of exercise capacity in chronic congestive heart failure. Am J Cardiol. (1985) 55:1037–42. doi: 10.1016/0002-9149(85)90742-8
25. Yndestad A, Damås JK, Oie E, Ueland T, Gullestad L, Aukrust P. Systemic inflammation in heart failure - the whys and wherefores. Heart Fail Rev. (2006) 11:83–92. doi: 10.1007/s10741-006-9196-2
26. Van Linthout S, Tschöpe C. Inflammation—cause or consequence of heart failure or both? Curr Heart Fail Rep. (2017) 14:251–65. doi: 10.1007/s11897-017-0337-9
27. Buckley LF, Abbate A. Interleukin-1 blockade in cardiovascular diseases: a clinical update. Eur Heart J. (2018) 39:2063–9. doi: 10.1093/eurheartj/ehy128
28. Buckley LF, Abbate A. Interleukin-1 blockade in cardiovascular diseases: from bench to bedside. BioDrugs (2018) 32:111–8. doi: 10.1007/s40259-018-0274-5
29. Pearson TA, Mensah GA, Wayne R, Anderson JL, CannonIII RO, Criqui M, et al. Markers of inflammation and cardiovascular disease. Circulation (2003) 107:499–511. doi: 10.1161/01.cir.0000052939.59093.45.
30. Ridker PM. Clinical application of C-reactive protein for cardiovascular disease detection and prevention. Circulation (2003) 107:363–9. doi: 10.1161/01.CIR.0000053730.47739.3C
31. Van Tassell BW, Arena RA, Toldo S, Mezzaroma E, Azam T, Seropian IM, et al. Enhanced interleukin-1 activity contributes to exercise intolerance in patients with systolic heart failure. PLoS ONE (2012) 7:e33438. doi: 10.1371/journal.pone.0033438
32. Elster SK, Braunwald E, Wood HF. A study of C-reactive protein in the serum of patients with congestive heart failure. Am Heart J. (1956) 51:533–41. doi: 10.1016/0002-8703(56)90099-0
33. Testa M, Yeh M, Lee P, Fanelli R, Loperfido F, Berman JW, et al. Circulating levels of cytokines and their endogenous modulators in patients with mild to severe congestive heart failure due to coronary artery disease or hypertension. J Am Coll Cardiol. (1996) 28:964–71. doi: 10.1016/S0735-1097(96)00268-9
34. Shah SJ, Marcus GM, Gerber IL, McKeown BH, Vessey JC, Jordan MV, et al. High-sensitivity C-reactive protein and parameters of left ventricular dysfunction. J Card Fail. (2006) 12:61–5. doi: 10.1016/j.cardfail.2005.08.003
35. Tang WH, Shrestha K, Van Lente F, Troughton RW, Martin MG, Borowski AG, et al. Usefulness of C-reactive protein and left ventricular diastolic performance for prognosis in patients with left ventricular systolic heart failure. Am J Cardiol. (2008) 101:370–3. doi: 10.1016/j.amjcard.2007.08.038
36. Ridker PM. C-Reactive protein: Eighty eighty years from discovery to emergence as a major risk marker for cardiovascular disease. Clin Chem. (2009) 55:209–15. doi: 10.1373/clinchem.2008.119214
37. Vasan RS, Sullivan LM, Roubenoff R, Dinarello CA, Harris T, Benjamin EJ, et al. Inflammatory markers and risk of heart failure in elderly subjects without prior myocardial infarction: the framingham heart study. Circulation (2003) 107:1486–91. doi: 10.1161/01.CIR.0000057810.48709.F6
38. Kumar A, Thota V, Dee L, Olson J, Uretz E, Parrillo JE. Tumor necrosis factor alpha and interleukin 1 beta are responsible for in vitro myocardial cell depression induced by human septic shock serum. J Exp Med. (1996) 183:949–58. doi: 10.1084/jem.183.3.949
39. Van Tassell BW, Seropian IM, Toldo S, Mezzaroma E, Abbate A. Interleukin-1β induces a reversible cardiomyopathy in the mouse. Inflamm Res. (2013) 62:637–40. doi: 10.1007/s00011-013-0625-0
40. Díez J. Chronic heart failure as a state of reduced effectiveness of the natriuretic peptide system: implications for therapy. Eur J Heart Fail. (2017) 19:167–76. doi: 10.1002/ejhf.656
41. Tamura N, Ogawa Y, Chusho H, Nakamura K, Nakao K, Suda M, et al. Cardiac fibrosis in mice lacking brain natriuretic peptide. Proc Natl Acad Sci U.S.A. (2000) 97:4239–44. doi: 10.1073/pnas.070371497
42. Gong X, Mou Z, Shao L, Zou Y, Gu Y, Sun S. Human recombinant-B-type natriuretic peptide protect ventricular function and structure in ST-elevation myocardial infarction. Int J Clin Exp Pathol. (2015) 8:11622–8.
43. Sabatine MS, Morrow DA, de Lemos JA, Gibson CM, Murphy SA, Rifai N, et al. Multimarker approach to risk stratification in non-ST elevation acute coronary syndromes. Circulation (2002) 105:1760–3. doi: 10.1161/01.CIR.0000015464.18023.0A
44. Van Tassell BW, Canada J, Carbone S, Trankle C, Buckley L, Oddi Erdle C, et al. Interleukin-1 blockade in recently decompensated systolic heart failure: Results from REDHART (Recently Decompensated Heart Failure Anakinra Response Trial). Circ Hear Fail. (2017) 10:e004373. doi: 10.1161/CIRCHEARTFAILURE.117.004373
45. Van Tassell BW, Arena R, Biondi-Zoccai G, Canada JM, Oddi C, Abouzaki NA, et al. Effects of interleukin-1 blockade with anakinra on aerobic exercise capacity in patients with heart failure and preserved ejection fraction (from the D-HART Pilot Study). Am J Cardiol. (2014) 113:321–7. doi: 10.1016/j.amjcard.2013.08.047
46. Trankle C, Canada J, Carbone S, Buckley LF, Del Buono MG, Christopher S, et al. Interleukin-1 blockade reduces Nt-probnp serum levels in patients with stable heart failure with preserved ejection fraction. J Am Coll Cardiol. (2018) 71:A869. doi: 10.1016/S0735-1097(18)31410-4
47. Ridker PM, Everett BM, Thuren T, MacFadyen JG, Chang WH, Ballantyne C, et al. Antiinflammatory therapy with canakinumab for atherosclerotic disease. N Engl J Med. (2017) 377:1119–31. doi: 10.1056/NEJMoa1707914
Keywords: heart failure, biomarker, systemic inflammation, myocardial strain, cardiorespiratory fitness, cardiopulmonary exercise testing
Citation: van Wezenbeek J, Canada JM, Ravindra K, Carbone S, Trankle CR, Kadariya D, Buckley LF, Del Buono M, Billingsley H, Viscusi M, Wohlford GF, Arena R, Van Tassell B and Abbate A (2018) C-Reactive Protein and N-Terminal Pro-brain Natriuretic Peptide Levels Correlate With Impaired Cardiorespiratory Fitness in Patients With Heart Failure Across a Wide Range of Ejection Fraction. Front. Cardiovasc. Med. 5:178. doi: 10.3389/fcvm.2018.00178
Received: 12 September 2018; Accepted: 30 November 2018;
Published: 21 December 2018.
Edited by:
Pietro Enea Lazzerini, Università degli Studi di Siena, ItalyReviewed by:
Gaetano Ruocco, Università degli Studi di Siena, ItalyCarl Lavie, Ochsner Medical Center, United States
Copyright © 2018 van Wezenbeek, Canada, Ravindra, Carbone, Trankle, Kadariya, Buckley, Del Buono, Billingsley, Viscusi, Wohlford, Arena, Van Tassell and Abbate. This is an open-access article distributed under the terms of the Creative Commons Attribution License (CC BY). The use, distribution or reproduction in other forums is permitted, provided the original author(s) and the copyright owner(s) are credited and that the original publication in this journal is cited, in accordance with accepted academic practice. No use, distribution or reproduction is permitted which does not comply with these terms.
*Correspondence: Antonio Abbate, YW50b25pby5hYmJhdGVAdmN1aGVhbHRoLm9yZw==
†These authors have contributed equally to this work