- 1Interventional Cardiology Unit, Cardio-Thoracic-Vascular Department, San Raffaele Scientific Institute, Milan, Italy
- 2Department of Cardiology, Hammersmith Hospital, Imperial College Healthcare NHS Trust, London, United Kingdom
- 3Department of Cardiology, Montefiore Medical Center, New York, NY, United States
- 4Division of Cardiology, Department of Medicine, University of Cape Town, Cape Town, South Africa
Transcatheter aortic valve replacement is the therapy of choice for patients with severe aortic stenosis who have prohibitive or high surgical risk. However, the benefit of TAVR is attenuated by the occurrence of major disabling stroke which is associated with increased mortality and early-reduced quality of life. Despite advances in TAVR technology, stroke remains a serious complication that is associated with significant negative outcomes. The majority of these occur in the acute phase following TAVR where cerebral embolic events are frequent. Cerebral embolic protection devices (CEPD) have been developed to minimize the risk of peri-procedural ischemic stroke during TAVR. CEPD have the potential to reduce intraprocedural burden of new silent ischemic injury. In this review we outline the etiology and incidence of stroke in TAVR population, and systematically review current evidence for cerebral embolic protection devices.
Introduction
Aortic stenosis (AS) is the most common valvular pathology in the elderly and its prevalence is expected to increase rapidly over the next decade due to an aging population (1, 2). Transcatheter aortic valve replacement (TAVR) has revolutionized the treatment of symptomatic AS with over 300,000 TAVR procedures performed worldwide, to-date. This resulted following a number of registries (3, 4) and randomized controlled trials (5–11) demonstrating mortality benefits of TAVR in inoperable and high-risk surgical patients. However, the benefit of TAVR is attenuated by the occurrence of major disabling stroke which is associated with increased mortality and in the short term reduced quality of life. Despite the evolution in TAVR technology, cerebrovascular events remain one of the most serious complications with long-term negative sequelae. Cerebral embolic protection devices (CEPD) have been developed to minimize the risk of peri-procedural ischemic stroke during TAVR. Furthermore, with the anticipated expansion of TAVR into intermediate risk and younger patients, the prevention of TAVR-related stroke and understanding the role of CEPD in this will become essential (12).
In this review we outline the etiology and incidence of stroke in TAVR population, and systematically review current evidence for cerebral embolic protection devices.
Etiology
The temporal pattern of stroke following aortic valve intervention is similar between surgical and transcatheter aortic valve replacement. However, the main disparity between these occurs in the acute peri-procedural period with up to half of strokes occurring within the first 24-h after TAVR (13, 14). The PARTNER trial (6) observed a significantly increased risk of peri-procedural stroke (6.7%) compared to medical therapy (1.7%). Subsequent meta-analyses (15, 16) demonstrated 30-days stroke incidence of 3.1–3.3%, and that it confers a 3.5-fold increase in mortality at 1-year. After the initial 2 months, known as the late phase, there is a similar incidence of stroke between surgical and transcatheter aortic valve replacement groups that is likely to reflect the baseline risk profiles of the populations (13, 17).
Acute Stroke
Acute strokes are ischemic in the vast majority (95%) of patients and thought to be secondary to procedural factors (Table 1). These peri-procedural strokes occur due to embolization phenomenon arising from disruption of the vasculature, especially the aortic arch, degenerate aortic leaflets, or the left ventricular outflow tract. This causes calcific material or atheromatous plaque embolization (18). The passage of stiff guidewires, large caliber TAVR delivery systems, and prolonged procedural time have previously been associated with cerebral embolization in AS patients (19). Furthermore, repeated attempts to the cross calcified aortic valve, manipulation of the calcified aortic valve annulus, mechanical force of valve deployment, and pre- or post-dilatation may all be associated with further anatomical disruption leading to cerebral embolization. In addition, thrombotic cerebral microembolization has been observed in patients acutely following TAVR, potentially developing on guidewires or catheters. Lastly, CEPD studies have demonstrated the presence of myocardial tissue and plastic from TAVR delivery system as sources of cerebral embolization (18).
Hemodynamic instability occurring during TAVR can lead to systemic hypotension and consequently cerebral hypoperfusion. The effect of cerebral microemboli under these conditions is amplified, due to impairment of clearance and cementation of microemboli within small vessels (20). Rapid ventricular pacing constitutes the greatest risk of hemodynamic instability during TAVR, this is required during balloon valvuloplasty before or after TAVR, and in balloon expandable TAVR prostheses, or in cases where difficultly is encountered in precise positioning of self-expanding TAVR prostheses to minimize the risk of migration/embolization. In general, rapid ventricular pacing is well tolerated however in certain patients this can be associated with greater risk of hemodynamic instability, for example those with impaired left ventricular function or in those with marked left ventricular hypertrophy (17). Hemodynamic instability can also occur as a consequence of anesthetic complications, or secondary to hemorrhage.
Subacute/Late Stroke
The etiology of delayed stroke after TAVR remains poorly characterized and is probably due to multiple factors, the primary cause thought to be thromboembolic. This can occur due to numerous reasons: valve crimping and balloon dilatation of prosthetic valve leaflets can cause structural damage that result in prothrombotic state with platelet and fibrin aggregation (14); the valve delivery system may scrape the diffuse atherosclerosis inside the aorta and some particles be dislodged later with the increased cardiac output and increased flow; increased risk of thrombus formation on the TAVR prosthesis as endothelialization can take over 1 year (21); and new onset atrial arrhythmias, predominantly atrial fibrillation, have been revealed to confer increased risk of ischemic stroke and mortality (22). In addition, TAVR prothesis leaflet thickening and leaflet thrombus have recently been reported following an acutely successful procedure. Makkar et al. (23) reported on reduced aortic-valve leaflet motion in 55 patients from a clinical trial of TAVR and two single-center registries that included 132 patients who were undergoing either TAVR or surgical aortic-valve bioprosthesis implantation. From the clinical trial arm, reduced leaflet motion was noted in 22 of 55 patients (40%) on computed tomography (CT) imaging approximately 1-month after TAVR. Of note, all the patients with reduced leaflet motion CT had hypoattenuating opacities noted in the corresponding leaflets on two-dimensional CT. The findings on transesophageal echocardiography were consistent with a hyperechogenic, homogeneous mass located on the aortic aspect of the prosthetic leaflets that prevented normal leaflet excursion. There was no significant difference between patients with reduced leaflet motion and those with normal leaflet motion with respect to the mean aortic-valve gradient. Importantly, there was no significant difference in the incidence of stroke or TIA between patients with reduced leaflet motion and those with normal leaflet motion in the clinical trial (2 of 22 patients and 0 of 33 patients, respectively; P = 0.16). However, in the pooled registries including surgical aortic valve replacement, a significant difference was detected (3 of 17 patients and 1 of 115 patients, respectively; P = 0.007). Chakravarty et al. (24) reported on 890 patients from two large registries who had CT imaging following either TAVR or surgical aortic valve replacement. It was demonstrated that 106 (12%) of 890 patients had subclinical leaflet thrombosis, including five (4%) of 138 with thrombosis of surgical valves vs. 101 (13%) of 752 with thrombosis of transcatheter valves (p = 0.001).Subclinical leaflet thrombosis was less frequent among patients receiving anticoagulants. Subclinical leaflet thrombosis resolved in 36 (100%) of 36 patients receiving anticoagulants, whereas it persisted in 20 (91%) of 22 patients not receiving anticoagulants (p < 0.0001). Although stroke rates were not different between those with (4·12 strokes per 100 person-years) or without (1.92 strokes per 100 person-years) reduced leaflet motion (p = 0.10), subclinical leaflet thrombosis was associated with increased rates of transient ischaemic attacks (TIAs; 4.18 TIAs per 100 person-years vs. 0.60 TIAs per 100 person-years; p = 0.0005) and all strokes or TIAs (7.85 vs. 2.36 per 100 person-years; p = 0.001). Although, CEPD have no role in the prevention of leaflet thrombosis, prosthetic valve thrombosis may potentially have a tangible deleterious effect on rates of cerebrovascular accidents, but further data from the randomized low risk TAVR trials are awaited which should help clarify this issue.
Silent Cerebrovascular Events
The incidence of subclinical new cerebral ischemic lesions has been identified in as many as 93% of patients post-TAVR and recent pooled analysis reported an incidence of 77.5% (25). These are up to double of that seen in isolated surgical aortic valve replacement (26). Subclinical acute cerebral ischemic lesions can be accurately identified on diffusion-weighted magnetic resonance imaging (DW-MRI) with these regions demonstrating hyperintense signal as a result of reduction in water diffusion rate (27). Hyperintense signals on DW-MRI are well-established surrogate parameters for cerebral embolization and have already been investigated after catheter-based or cardiothoracic surgical interventions (28). In addition, cognitive function testing can be utilized to screen patients to determine those who may have had subclinical strokes (29). In various clinical contexts the occurrence of small brain infarcts has been linked to a higher incidence of stroke (30, 31) or cognitive impairment and dementia (32–34). Cerebral emboli detected on DWI-MRI increases the risk of clinically overt stroke by 2-4 fold and the greater the volume of lesions seen on DW-MRI, the greater the long-term risk of cognitive dysfunction and long-term dementia (32, 35). However, the prognostic significance of these subclinical brain injuries remains contentious and the correlation between new cerebral infarcts post TAVR and long-term cognitive decline or behavioral changes remain uncertain. Performing neurocognitive assessment immediately following TAVR is challenging in elderly patients since results can be influenced by the degree of alertness and fatigue, which is common in elderly patients peri-procedurally especially if sedation or general anesthetic have been administered (36). The BRAVO-3 MRI study investigated the role of intra-procedural parenteral anticoagulation (heparin vs. bivalirudin) during TAVR in reducing risk of cerebral emboli during TAVR (37). In this study, there was no difference in rates of with new cerebral emboli between the bivalirudin (54.5%) and heparin (58.1%) groups. Of note, all patients that presented with clinically overt stroke showed evidence of new emboli on MRI. The total volume of emboli, the volume of single embolus per patient, and the volume of the largest embolus per patient were higher in patients presenting with vs. without stroke at 30-days.
Transcarotid Doppler studies have shown a high incidence of high-intensity transient signal (HITS) throughout the entire TAVR procedure, especially during valve positioning and implantation (38), highlighting the high embolic risk during these phases of the procedure. Therefore, the use of CEPD might play an important role during these at high risk phases of the TAVR procedure. All studies evaluating CEPD have focused on the assessment and characterization of new brain ischemic lesions on DW-MRI as the main efficacy endpoints (39). The relatively small incidence of clinically apparent cerebrovascular events makes them difficult to use as endpoints in clinical trials, shifting the attention to subclinical cerebral injury (17).
Cerebral Embolic Protection Devices
Cerebral embolic protection devices are filters designed to capture or deflect emboli traveling to the brain during TAVR procedures in order to protect the supra-aortic vessels from embolic debris. These filters are normally positioned across the origin of supra-aortic vessels before the advancement of the TAVR system across the aortic valve and is retrieved at the end of the procedure (Figure 1). The positioning of these devices can be challenging particularly if atherosclerotic plaques are located in the vicinity of the ostium of supra-aortic vessels or aortic arch, hampering the implantation and positioning of CEPD which may even promote plaque disruption and consequently cerebral embolization (40). Initial in-human experiences have shown the feasibility and safety of CEPD during TAVR (41, 42). Currently there are three devices commercially available with studies that have evaluated their efficacy (Table 2).
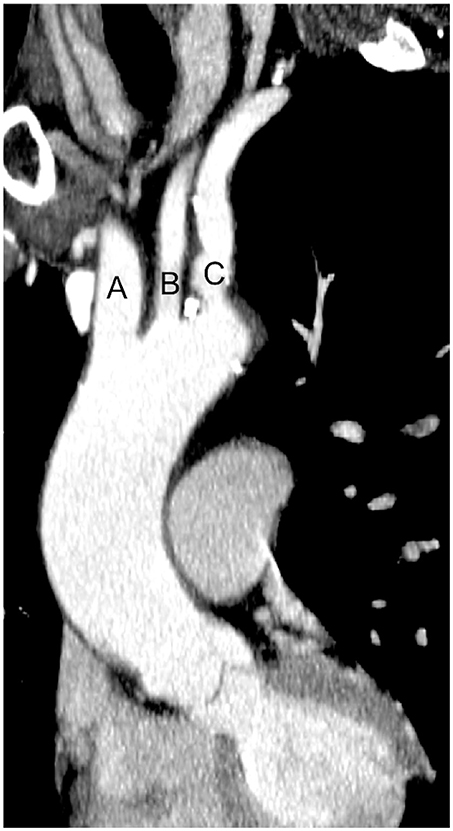
Figure 1. Computed tomography image of ascending aorta and supra-aortic vessels. (A) Brachiocephalic artery; (B) Left common carotid artery; (C) Left subclavian artery.
Embrella Device
The Embrella Embolic Deflector device (EED) (Edwards Lifesciences; Irvine, California, United States) is a filter designed to deflect debris traveling to the brain during the positioning and implantation of the TAVR valve. The distal end of the deflector consists of an oval shaped nitinol frame (length 59 mm, width 25.5 mm) covered with a porus polyurethane membrane (100 microns pore size). The frame of the device has two opposing petals that are positioned along the greater curve of the aorta, covering the ostia of both the brachiocephalic and the left common carotid arteries (45). The device is inserted via the right radial or brachial approach using a 6-French delivery system. The EED system is deployed at the beginning of the TAVR procedure just before any attempt to cross the native aortic valve. Nietlispach et al. reported the first in-human experience with the EED device showing the feasibility and safety of device implantation in a preliminary series of 4 patients (1 aortic valvuloplasty, 3 TAVR procedures) (41). Subsequently, the PROTAVI-C study (45) evaluated the procedural safety, technical feasibility, and exploratory efficacy of the EED. This prospective non-randomized study included 54 patients, with 42 patients receiving the EED device and 12 patients not receiving it (control group). TAVR procedures were performed by transfemoral approach with Edwards Sapiens XT. The PROTAVI-C study demonstrated that EED use during TAVR is feasible and safe with minimal procedural complications related to the device (1 radial thrombosis with no clinical consequences and 1 pseudoaneurysm of the brachial artery that required surgical repair). The EED system did not prevent the occurrence of cerebral microemboli during TAVR as evaluated by transcranial Doppler during the procedure. The number of HITS was actually higher in the EED group than in the control group, 632 [interquartile range, 347-893] vs. 279 [interquartile range, 0–505], respectively (p < 0.001). Therefore, suggesting that EED manipulation may also represent a potential source of embolic debris. In addition, the use of EED had no effect on the occurrence and number of new ischemic lesions as evaluated by DW-MRI at 7 days after the procedure. These ischemic lesions disappeared within few weeks (as evaluated by DW-MRI at around 30 days) and were not associated with any neurological and cognitive impairment. However, the use of a EED was associated with a reduction in lesion volume compared to the control group. Fundamentally, this study was limited by the low number of patients and lack of randomization. However, the EED device is currently not available commercially.
Claret Device
The Claret embolic protection device (CD) (Claret Medical, Inc.; Santa Rosa, California, United States) is designed to capture debris dislodged during TAVR and it is the first device with FDA approval (46). The system consists of a dual filter system deployed via the right radial or brachial approach to the brachiocephalic and left common carotid arteries. It consists of a proximal filter (sized 9–15 mm in diameter) delivered in the brachiocephalic artery covering all areas of the brain supplied by the right vertebral and right carotid artery and a distal filter (sized 6.5–10 mm in diameter) delivered in the left common carotid artery. The left vertebral artery, which usually originates from the left subclavian artery, remains unprotected, as does the cerebral regions fed by this vessel. At the start of the procedure the system is advanced through a 6F sheath and it is deployed in the aortic arch and withdrawn following removal of the TAVR delivery system (42). The CLEAN TAVI study (43) was a single center, blinded, randomized clinical trial that evaluated the efficacy of the Claret device in reducing the number of cerebral lesions in patients undergoing TAVR with Medtronic CoreValve. The primary endpoint was the reduction in number of lesions on DW-MRI at 2 days post-TAVR. The secondary outcome was the difference in volume of new lesions after TAVR in potentially protected territories. The study included 100 patients randomized 1:1 to the control or filter group. This showed a reduction in the number of new ischemic cerebral lesions [difference 5.00 (IQR, 2.00-8.00); p < 0.001] and volume of cerebral lesions in the filter group compared to the control group [difference 234 mm3 (95% CI, 91-406); p = 0.001]. These changes were observed largely within cerebral territories that were protected by the filter (day 2 post-TAVR: 246 vs. 527 mL, p = 0.002). The MISTRAL-C study (47), a multicenter, double-blind, randomized trial that confirmed the efficacy of CD in reducing the number of new ischemic cerebral lesions and the volume of these lesions in 65 patients randomized 1:1 to CD vs. non-CD. The main limitation of this study is that only 57% of the randomized patients underwent follow-up DW-MRI. The SENTINEL trial (48) is the largest randomized clinical trial evaluating the safety and efficacy of a transcatheter CD system during TAVR. The SENTINEL trial enrolled 363 patients, who were randomized 1:1:1 into a safety arm (n = 123), an imaging device arm (n = 121), and an imaging control arm (n = 119). In this study a significant reduction of median total new lesion volume in protected territories, evaluated by DW-MRI 2-7 days after TAVR, was not observed (102.8 mm3, IQR 36.9-423.2 mm3 in the device arm vs. 178.0 mm3, IQR 34.3-482.5 mm3 in the control arm; p = 0.33), However, the use of the Sentinel device during TAVR was safely performed and histopathological debris was found within filters in 99% of patients, confirming the embolic risk during TAVR with frequent embolization of non-thrombotic material (vascular material in 94% of cases). Importantly, it was demonstrated for the first time that there is a correlation between new lesion volume and neurocognitive decline. Latib et al. (36) identified some challenges related to this trial that can be extended to other CEPD trials. Firstly, the need of baseline MRI to detect previous neurological damage and their impact on new cerebral lesions. Post-hoc multivariable analysis in the SENTINEL trial identified pre-existing lesion volume as main predictor of new lesion volumes. In addition, after adjusting for baseline T2/FLAIR lesion volume, there was a reduction in new lesion volume in both protected and all territories in the device vs. control arms. Secondly, the time points of evaluation of 2–7 days after TAVR might create too much heterogeneity in terms of detected volumes of ischemic lesions because of the time-dependent sensitivity of DW-MRI. As a matter of fact, the time point for performing DW-MRI post-TAVR could affect the sensitivity for detecting silent cerebral infarcts, as these lesions tend to disappear over time, being totally absent at 30 days following the procedure (49, 50). Thirdly, the evaluation of cognitive dysfunction might be misleading in elderly patients in the first few days after TAVR, therefore a simpler and more focused battery of tests may be repeated later in time. Furthermore, the Claret device can only protect completely 9 out of 28 brain territories because of the dual blood supply of the posterior circulation. Thus, if we believe that cerebral protection is important, it is not acceptable that these areas of the brain remain unprotected. The even embolic distribution shown on DW-MRI validates the need of a comprehensive brain protection (51, 52).
Triguard Device
The TriGuard (TG) CEPD (Keystone Heart Ltd., Herzliya, Israel) is a mechanical system designed to deflect cerebral emboli during TAVR while allowing maximal blood flow to the brain and it is the only deflection device that covers all 3 cerebral vessels. The device is a single-wire nitinol frame and mesh filter with pore size of 130 μm and it is positioned across all 3 cerebral vessels and maintained by stabilizers in the innominate artery and the inner curvature of the aortic arch. At the start of the TAVR procedure, a 9 Fr arterial sheath is inserted in the contralateral femoral artery through which the TriGuard device is advanced to the aortic arch and deployed to cover the ostia of the three major cerebral vessel take-offs and it is withdrawn after completion of the TAVR procedure (44).
The DEFLECT I (44) and DEFLECT II (53) studies are single arm studies that confirmed the safety and performance of the first and second generation TriGuard device. In particular, the DEFLECT I formed the basis for TriGuard been granted CE mark in October 2013. The DEFLECT III (54) a prospective, multi-center, single-blind, randomized controlled trial evaluating the safety, efficacy and performance of the TriGuard device in subjects undergoing TAVR. The study included 85 patients randomized 1:1 to CEDP (46) or unprotected TAVR (39). This study showed that embolic protection during TAVR with TriGuard was safe and complete vessel coverage was achieved in 89% of the patients. The safety endpoint (including death, stroke, life-threatening or disabling bleeding, stage 2 or 3 acute kidney injury, or major vascular complications) was not different between the two groups. However, in the intention-to-treat analysis the use of TriGuard was associated with a greater freedom from new cerebral DWI lesions (21.2 vs. 11.5%), a reduction in “new neurologic impairment” defined as worsening in National Institutes of Health Stroke Scale score from baseline (2.6 vs. 12.1%) and a reduction in single and multiple lesions volume, especially for lesion volume of small and medium size (< 150 mm3). Additionally, it showed improved cognitive function in some domain at discharge: in the International Shopping List Test (a measure of episodic memory), significant differences were observed when patients were evaluated at discharge, favoring the interventional arm (65.4 vs. 30.4%, p = 0.022). The main limitations of this study are its lack of statistical power to evaluate the safety and efficacy endpoints and the high loss to follow-up (31% were lost to the post-interventional DWI evaluations and 26% were lost to the post-interventional cognitive and neurologic assessments). Lansky et al. (54) performed a pooled analysis on DEFLECT I, DEFLECT II studies, and the Neuro TAVR registry, this was a registry of 142 patients undergoing TAVR with TriGuard protection (n = 59) vs. no protection (n = 83). The study reported that TriGuard protection significantly reduced the incidence of in-hospital Valve Academic Research Consortium-2–defined stroke (VARC-2) (0 vs. 6%; P = 0.05), the incidence of stroke as defined by worsening National Institutes of Health Stroke Scale (NIHSS) with DW-MRI lesions (0 vs. 19%; P = 0.002), brain embolic lesion volume on MRI (315 + 620 mm3 vs. 511 + 893 mm3; P = 0.04) and demonstrated improved functioning on cognitive testing post-TAVR. The REFLECT-US (55) trial is an ongoing multicenter, randomized controlled trial evaluating the safety, efficacy, and performance of the TriGuard device in a larger cohort of patients undergoing TAVR compared to previous studies. This trial is including 285 TAVR subjects with 2:1 randomization to TriGuard (190 patients) and unprotected TAVR (95 patients).
New Cerebral Protection Devices
Several new CEPD are currently under development or early first-in-human analysis, these are outlined in Table 3. The Emblok Embolic Protection System (Innovative Cardiovascular Solutions, LLC) is one of the devices that has ongoing clinical feasibility study. This device provides full circumferential coverage of the aortic arch, hence protecting all supra-aortic vessels, utilizing a pore size of 125 μm. The system incorporates an integrated 4-Fr radiopaque pigtail catheter which provides constant visualization, from which aortagram can be performed both for CEPD and TAVR deployment. In addition, the radiopaque pigtail catheter aids in defining the non-coronary cusp hence it facilitates precise valve implantation while potentially decreasing contrast injections during TAVR positioning and deployment. The delivery system is 11–Fr compatible and allows two devices (i.e., embolic filter and pigtail catheter) to be deployed through a single access site supported by 0.035 guidewire. Clinical studies are currently underway to establish the safety and efficacy of Emblock in TAVR patient. In addition, there are other CEPD that currently under development or early first-in-human analysis, these are outlined in Table 3.
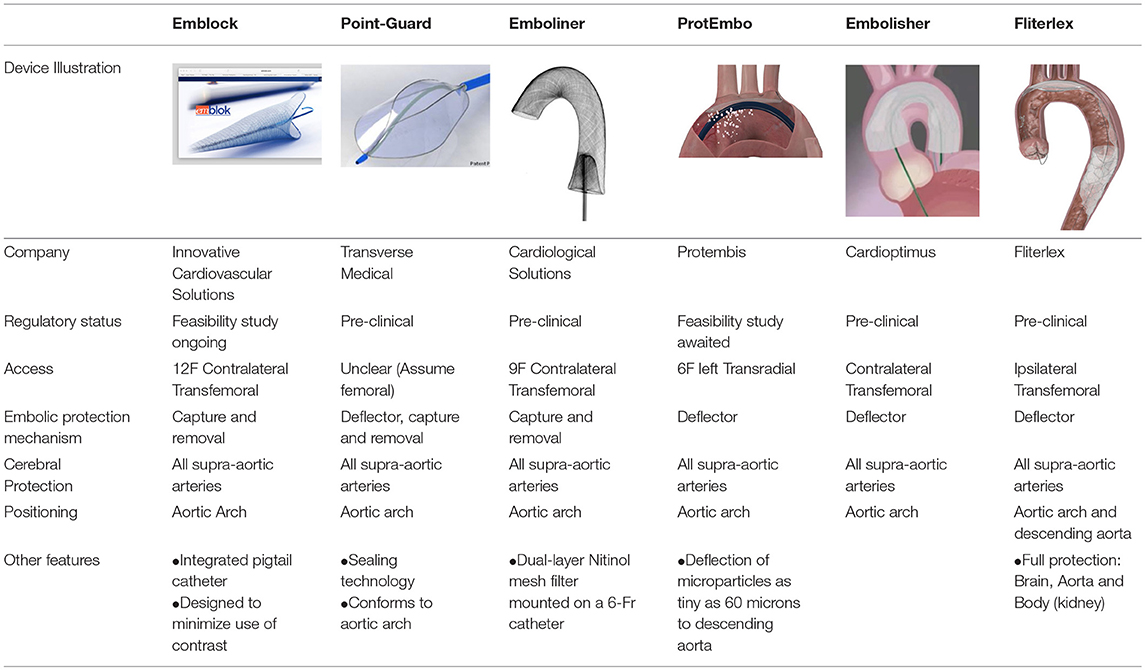
Table 3. Percutaneous cerebral protection devices currently under development or first-in-man study stage.
Discussion
Despite advances in TAVR technology, stroke remains a serious complication that is associated with significant negative outcomes. The majority of these occur in the acute phase following TAVR where cerebral embolic events are frequent. CEPD have the potential to reduce intraprocedural burden of new silent ischemic injury. Although individual CEPD studies have not yet demonstrated a reduction in rates of silent cerebral ischemic lesions evaluated by DW-MRI, they have demonstrated reductions in total cerebral ischemic volumes. Giustino et al. (56) performed a meta-analysis on 5 studies evaluating the efficacy of these 3 CEPD. A total of 625 patients were included, 376 with CEPD and 249 without CEPD, the CEPD group showed a lower risk in death or stroke, suggesting that CEPD may be a clinically relevant adjunctive strategy in patients undergoing TAVR. Aufrett e al. meta-analysis (sixty-four studies involving 72,318 patients) reported that female sex, chronic kidney disease, enrollment date, and new-onset atrial fibrillation were predictors of early CVE after TAVR. The main limitation of this meta-analysis is that most of the studies included were not powered for CVEs as main endpoint (57). A very large multicentre study, powered for early CVEs, is needed to identify which are the predictors of such events that will help us tailoring our preventive strategies. Preventing procedure-related cerebral injury remains a significant unmet clinical need with potentially important long-term sequelae. As we move to low-risk patients, the bar to ensuring good TAVR outcomes will become much higher. Hence, CEPD could potentially become standard of care if: (a) we accept as a community that silent cerebral infarction has a negative impact on long-term outcomes and that prevention of these is as important as preventing stroke; (b) CEPD devices are easy to use, safe, provide full protection of supra-aortic arteries during the procedure, and can be rapidly implanted without adding significant time to the procedure or interfering with valve positioning or deployment; (c) specific reimbursement for CEPD during TAVR become available and/or the cost of devices decrease significantly. If the previous conditions are satisfied, a very large multicentre randomized study might be conducted to evaluate the clinical benefit from CEPD devices.
Conclusion
Stroke remains one of the most serious complication following TAVR with associated worse outcomes that negate the benefit of TAVR procedure. Although CEPD have been demonstrated to reduce cerebral infarct volume, whether it decreases rates of both silent cerebral ischemic lesions and clinically evident ischemic strokes remains unclear. However, there will be greater emphasis on prevention of cerebral ischemic events as we move to low-risk patients. To elucidate the exact role of CEPD, a large randomized controlled trial with long-term follow-up with baseline and follow-up cerebral MRI imaging, and full neurological clinical evaluation, ideally using a device that protects all supra-aortic arteries, to establish the role of CEPD both in the short and long term.
Author Contributions
OD, GI, GW, and AL substantially contributed conception and design of the manuscript. OD and GI wrote the first draft of the manuscript. All authors contributed to manuscript revision, read and approved the submitted version.
Conflict of Interest Statement
The authors declare that the research was conducted in the absence of any commercial or financial relationships that could be construed as a potential conflict of interest.
References
1. Nkomo VT, Gardin JM, Skelton TN, Gottdiener JS, Scott CG, Enriquez-Sarano M. Burden of valvular heart diseases: a population-based study. Lancet (2006) 368:1005–11. doi: 10.1016/S0140-6736(06)69208-8
2. Osnabrugge LJ, Mylotte D, Head SJ, Van Mieghem NM, Nkomo VT, LeReun CM, et al. Aortic Stenosis in the elderly. J Am Coll Cardiol. (2013) 62:1002–12. doi: 10.1016/j.jacc.2013.05.015
3. Moat NE, Ludman P, De Belder MA, Bridgewater B, Cunningham AD, Young CP, et al. Long-term outcomes after transcatheter aortic valve implantation in high-risk patients with severe aortic stenosis: The U.K. TAVI (United Kingdom transcatheter aortic valve implantation) registry. J Am Coll Cardiol. (2011) 58:2130–8. doi: 10.1016/j.jacc.2011.08.050
4. Rodés-Cabau J, Dumont E, De LaRochellière R, Doyle D, Lemieux J, Bergeron S, et al. Feasibility and initial results of percutaneous aortic valve implantation including selection of the transfemoral or transapical approach in patients with severe aortic stenosis. Am J Cardiol. (2008) 102:1240–6. doi: 10.1016/j.amjcard.2008.06.061
5. Smith CR, Leon MB, Mack MJ, Miller DC, Moses JW, Svensson LG, et al. Transcatheter versus surgical aortic-valve replacement in high-risk patients. N Engl J Med. (2011) 364:2187–98. doi: 10.1056/NEJMoa1103510
6. Leon MB, Smith CR, Mack M, Miller DC, Moses JW, Svensson LG, et al. Transcatheter aortic-valve implantation for aortic stenosis in patients who cannot undergo surgery. N Engl J Med. (2010) 363:1597–607. doi: 10.1056/NEJMoa1008232
7. Adams DH, Popma JJ, Reardon MJ, Yakubov SJ, Coselli JS, Deeb GM, et al. Transcatheter aortic-valve replacement with a self-expanding prosthesis. N Engl J Med. (2014) 370:1790–8. doi: 10.1056/NEJMoa1400590
8. Kodali SK, Williams MR, Smith CR, Svensson LG, Webb JG, Makkar RR, et al. Two-year outcomes after transcatheter or surgical aortic-valve replacement. N Engl J Med. (2012) 366:1686–95. doi: 10.1056/NEJMoa1200384
9. Makkar RR, Fontana GP, Jilaihawi H, Kapadia S, Pichard AD, Douglas PS, et al. Transcatheter aortic-valve replacement for inoperable severe aortic stenosis. N Engl J Med. (2012) 366:1696–704. doi: 10.1056/NEJMoa1202277
10. Kapadia SR, Tuzcu EM, Makkar RR, Svensson LG, Agarwal S, Kodali S, et al. Long-term outcomes of inoperable patients with aortic stenosis randomly assigned to transcatheter aortic valve replacement or standard therapy. Circulation (2014) 130:1483–92. doi: 10.1161/CIRCULATIONAHA.114.009834
11. Reardon MJ, Van Mieghem NM, Popma JJ, Kleiman NS, Søndergaard L, Mumtaz M, et al. Surgical or transcatheter aortic-valve replacement in intermediate-risk patients. N Engl J Med. (2017) 376:1321–31. doi: 10.1056/NEJMoa1700456
12. Leon MB, Smith CR, Mack MJ, Makkar RR, Svensson LG, Kodali SK, et al. Transcatheter or surgical aortic-valve replacement in intermediate-risk patients. N Engl J Med. (2016) 374:1609–20. doi: 10.1056/NEJMoa1514616
13. Miller DC, Blackstone EH, Mack MJ, Svensson LG, Kodali SK, Kapadia S, et al. Transcatheter (TAVR) versus surgical (AVR) aortic valve replacement: Occurrence, hazard, risk factors, and consequences of neurologic events in the PARTNER trial. J Thorac Cardiovasc Surg. (2012) 143:832–43.e13. doi: 10.1016/j.jtcvs.2012.01.055
14. E. Tay LW, Gurvitch R, Wijesinghe N, Nielispach F, Wood D, Cheung A, et al. A high-risk period for cerebrovascular events exists after transcatheter aortic valve implantation. JACC Cardiovasc Interv. (2011) 4:1290–7. doi: 10.1016/j.jcin.2011.08.012
15. Eggebrecht H, Schmermund A, Voigtländer T, Kahlert P, Erbel R, Mehta RH, et al. Risk of stroke after transcatheter aortic valve implantation (TAVI): A meta-analysis of 10,037 published patients. EuroIntervention (2012) 8:129–38. doi: 10.4244/EIJV8I1A20
16. Muralidharan A, Thiagarajan K, Van Ham R, Gleason TG, Mulukutla S, Schindler JT, et al. Meta-analysis of perioperative stroke and mortality in transcatheter aortic valve implantation. Am J Cardiol. (2017) 118:1031–45. doi: 10.1016/j.amjcard.2016.07.011
17. Fanning JP, Walters DL, Platts DG, Eeles E, Bellapart J, Fraser JF Characterization of neurological injury in transcatheter aortic valve implantation: how clear is the picture?. Circulation (2014) 129:504–15. doi: 10.1161/CIRCULATIONAHA.113.004103
18. Van Mieghem NM, Schipper EI, Ladich E, Faqiri E, van der Boon R, Randjgari A, et al. Histopathology of embolic debris captured during transcatheter aortic valve replacement. Circulation (2013) 127:2194–201. doi: 10.1161/CIRCULATIONAHA.112.001091
19. Kahlert P, Knipp SC, Schlamann M, Thielmann M, Al-Rashid F, Weber M, et al. Silent and Apparent Cerebral Ischemia After Percutaneous Transfemoral Aortic Valve Implantation: A Diffusion-Weighted Magnetic Resonance Imaging Study. Circulation (2010) 121: 870–8. doi: 10.1161/CIRCULATIONAHA.109.855866
20. Caplan LR, Hennerici M. Impaired clearance of emboli (washout) is an important link between hypoperfusion, embolism, and ischemic stroke. Arch Neurol. (1998) 55:1475–82.
21. Linke A, Höllriegel R, Walther T, Schierle K, Wittekind C, Ender J, et al. Ingrowths of a percutaneously implanted aortic valve prosthesis (corevalve) in a patient with severe aortic stenosis. Circ Cardiovasc Interv. (2008) 1:155–8. doi: 10.1161/CIRCINTERVENTIONS.108.796094
22. Jørgensen TH, Thyregod GH, Tarp JB, Svendsen JH, Søndergaard L. Temporal changes of new-onset atrial fibrillation in patients randomized to surgical or transcatheter aortic valve replacement. Int J Cardiol. (2017) 234:16–21. doi: 10.1016/j.ijcard.2017.02.098
23. Makkar RR, Fontana G, Jilaihawi H, Chakravarty T, Kofoed KF, De Backer O, et al. Possible subclinical leaflet thrombosis in bioprosthetic aortic valves. N Engl J Med. (2015) 373:2015–24. doi: 10.1056/NEJMoa1509233
24. Chakravarty T, Søndergaard L, Friedman J, De Backer O, Berman D, Kofoed KF, et al. Subclinical leaflet thrombosis in surgical and transcatheter bioprosthetic aortic valves: an observational study. Lancet (2017) 389:2383–92. doi: 10.1016/S0140-6736(17)30757-2
25. Pagnesi M, Martino EA, Chiarito M, Mangieri A, Jabbour RJ, Van Mieghem NM, et al. Silent cerebral injury after transcatheter aortic valve implantation and the preventive role of embolic protection devices: a systematic review and meta-analysis. Int J Cardiol. (2016) 221:97–106. doi: 10.1016/j.ijcard.2016.06.143
26. Astarci P, Glineur D, Kefer J, D'Hoore W, Renkin J, Vanoverschelde JL, et al. Magnetic resonance imaging evaluation of cerebral embolization during percutaneous aortic valve implantation: comparison of transfemoral and trans-apical approaches using Edwards Sapiens valve. Eur J Cardiothorac Surg. (2011) 40:475–9. doi: 10.1016/j.ejcts.2010.11.070
27. Spaziano M, Francese DP, Leon MB, Généreux P. Imaging and functional testing to assess clinical and subclinical neurological events after transcatheter or surgical aortic valve replacement: a comprehensive review. J Am Coll Cardiol. (2014) 64:1950–63. doi: 10.1016/j.jacc.2014.07.986
28. Gress DR. The problem with asymptomatic cerebral embolic complications in vascular procedures: what if they are not asymptomatic?. J Am Coll Cardiol. (2012) 60:1614–6. doi: 10.1016/j.jacc.2012.06.037
29. Ghanem A, Dörner J, Schulze-Hagen L, Müller A, Wilsing M, Sinning JM, et al. Subacute subclinical brain infarctions after transcatheter aortic valve implantation negatively impact cognitive function in long-term follow-up. PLoS ONE (2017) 12:e0168852. doi: 10.1371/journal.pone.0168852
30. Bernick C, Kuller L, Dulberg C, Longstreth WT, Manolio T, Beauchamp N, et al. Cardiovascular Health Study Collaborative Reseach Group, Silent MRI infarcts and the risk of future stroke: the cardiovascular health study. Neurology (2001) 57:1222–9. doi: 10.1212/WNL.57.7.1222
31. Vermeer SE, Hollander M, van Dijk EJ, Hofman A, Koudstaal PJ, Breteler MB, Rotterdam Scan Study. Silent brain infarcts and white matter lesions increase stroke risk in the general population: the Rotterdam Scan Study. Stroke (2003) 34:1126–9. doi: 10.1161/01.STR.0000068408.82115.D2
32. Vermeer SE, Longstreth WT, Koudstaal PJ. Silent brain infarcts: a systematic review. Lancet Neurol. (2007) 6:611–9. doi: 10.1016/S1474-4422(07)70170-9
33. Barber PA, Hach S, Tippett LJ, Ross L, Merry AF, Milsom P. Cerebral ischemic lesions on diffusion-weighted imaging are associated with neurocognitive decline after cardiac surgery. Stroke (2008) 39:1427–33. doi: 10.1161/STROKEAHA.107.502989
34. Vermeer SE, Prins ND, den Heijer T, Hofman A, Koudstaal PJ, Breteler MB. Silent brain infarcts and the risk of dementia and cognitive decline. N Engl J Med. (2003) 348:1215–22. doi: 10.1056/NEJMoa022066
35. Sacco RL, Kasner SE, Broderick JP, Caplan LR, Connors JB, Culebras A, et al. An updated definition of stroke for the 21st century: a statement for healthcare professionals from the American Heart Association/American Stroke Association. Stroke (2013) 44:2064–89. doi: 10.1161/STR.0b013e318296aeca
36. Latib A, Pagnesi M. Cerebral embolic protection during transcatheter aortic valve replacement: a disconnect between logic and data?. J Am Coll Cardiol. (2017) 69:378–80. doi: 10.1016/j.jacc.2016.10.036
37. Van Belle E, Hengstenberg C, Lefevre T, Kupatt C, Debry N, Husser O, et al. BRAVO-3 MRI study investigators, cerebral embolism during transcatheter aortic valve replacement: the BRAVO-3 MRI study. J Am Coll Cardiol. (2016) 68:589–99. doi: 10.1016/j.jacc.2016.05.006
38. Kahlert P, Al-Rashid F, Döttger P, Mori K, Plicht B, Wendt D, et al. Cerebral embolization during transcatheter aortic valve implantation: a transcranial Doppler study. Circulation (2012) 126:1245–55. doi: 10.1161/CIRCULATIONAHA.112.092544
39. Rossow CF, McCabe JM. Protection from cerebral embolic events during transcatheter aortic valve replacement. Curr Cardiol Rep. (2016) 18:16. doi: 10.1007/s11886-015-0692-7
40. Abdul-Jawad Altisent O, Puri R, Rodés-Cabau J. Embolic protection devices during TAVI: current evidence and uncertainties. Rev Esp Cardiol. (2016) 69:962–72. doi: 10.1016/j.rec.2016.04.056
41. Nietlispach F, Wijesinghe N, Gurvitch R, Tay E, Carpenter JP, Burns C, et al. An embolic deflection device for aortic valve interventions. JACC Cardiovasc Interv. (2010) 3:1133–8. doi: 10.1016/j.jcin.2010.05.022
42. Naber CK, Ghanem A, Abizaid AA, Wolf A, Sinning JM, Werner N, et al. First-in-man use of a novel embolic protection device for patients undergoing transcatheter aortic valve implantation. EuroIntervention (2012) 8:43–50. doi: 10.4244/EIJV8I1A8
43. Haussig S, Mangner N, Dwyer MG, Lehmkuhl L, Lücke C, Woitek F, et al. Effect of a cerebral protection device on brain lesions following transcatheter aortic valve implantation in patients with severe aortic stenosis the CLEAN-TAVI randomized clinical trial. JAMA (2016) 316:592–601. doi: 10.1001/jama.2016.10302
44. Baumbach A, Mullen M, Brickman AM, Aggarwal SK, Pietras CG, Forrest JK, et al. Safety and performance of a novel embolic deflection device in patients undergoing transcatheter aortic valve replacement: results from the DEFLECT I study. EuroIntervention (2015) 11: 75–84. doi: 10.4244/EIJY15M04_01
45. Rodés-Cabau J, Kahlert P, Neumann FJ, Schymik G, Webb JG, Amarenco P, et al. Feasibility and exploratory efficacy evaluation of the Embrella Embolic Deflector system for the prevention of cerebral emboli in patients undergoing transcatheter aortic valve replacement: the PROTAVI-C pilot study. JACC Cardiovasc Interv. (2014) 7:1146–55. doi: 10.1016/j.jcin.2014.04.019
46. Novo D FDA Executive Summary Claret Medical Inc. ' s Sentinel® Cerebral Protection System Table of Contents (2017).
47. Van Mieghem NM, Van Gils L, Ahmad H, Van Kesteren F, Van Der Werf HW, Brueren G, et al. Filter-based cerebral embolic protection with transcatheter aortic valve implantation: the randomised MISTRAL-C trial. EuroIntervention (2016) 12:499–507. doi: 10.4244/EIJV12I4A84
48. Kapadia SR, Kodali S, Makkar R, Mehran R, Lazar RM, Zivadinov R, et al. Protection against cerebral embolism during transcatheter aortic valve replacement. J Am Coll Cardiol. (2017) 69:367–77. doi: 10.1016/j.jacc.2016.10.023
49. Gass A, Ay H, Szabo K, Koroshetz WJ. Diffusion-weighted MRI for the “small stuff”: the details of acute cerebral ischaemia. Lancet Neurol. (2004) 3:39–45. doi: 10.1016/S1474-4422(03)00621-5
50. Lansberg MG, Thijs VN, O'Brien MW, Ali JO, de Crespigny AJ, Tong DC, et al. Evolution of apparent diffusion coefficient, diffusion-weighted, and T2-weighted signal intensity of acute stroke. Am J Neuroradiol. (2001) 22:637–44.
51. Layton KF, Kallmes DF, Cloft HJ, Lindell EP, Cox VS. Bovine aortic arch variant in humans: clarification of a common misnomer. Am J Neuroradiol. (2006) 27:1541–2.
52. Arnold M, Schulz-Heise S, Achenbach S, Ott S, Dörfler A, Ropers D, et al. Embolic cerebral insults after transapical aortic valve implantation detected by magnetic resonance imaging. JACC Cardiovasc Interv. (2010) 311:26–32. doi: 10.1016/j.jcin.2010.09.008
53. Samim MB., van der Worp Agostoni P, Hendrikse JR, Budde PJ, Nijhoff F, et al. TriGuardTM HDH embolic deflection device for cerebral protection during transcatheter aortic valve replacement. Catheter Cardiovasc Interv. (2017) 89: 470–7. doi: 10.1002/ccd.26566
54. Lansky AJ, Schofer J, Tchetche D, Stella P, Pietras CG, Parise H, et al. A prospective randomized evaluation of the TriGuardTM HDH embolic DEFLECTion device during transcatheter aortic valve implantation: results from the DEFLECT III trial. Eur. Heart J. (2015) 36:2070–8. doi: 10.1093/eurheartj/ehv191
55. Moses JW. A Description of US REFLECT Randomized Clinical Trial Disclosure Statement of Financial Interest (2017). Available online at: https://clinicaltrials.gov/ct2/show/study/NCT02536196
56. Giustino G, Mehran R, Veltkamp R, Faggioni M, Baber U, Dangas GD. Neurological outcomes with embolic protection devices in patients undergoing transcatheter aortic valve replacement. JACC Cardiovasc. Interv. (2016) 9:2124–33. doi: 10.1016/j.jcin.2016.07.024
57. Auffret V, Regueiro A, Del Trigo M, Abdul-Jawad Altisent O, Campelo-Parada F, Chiche O, Puri R, et al. Predictors of early cerebrovascular events in patients with aortic stenosis undergoing transcatheter aortic valve replacement. J Am Coll Cardiol. (2016) 68:673–84. doi: 10.1016/j.jacc.2016.05.065
Keywords: stroke, embolic protection devices, aortic stenosis, TAVI, TAVR
Citation: Demir OM, Iannopollo G, Mangieri A, Ancona MB, Regazzoli D, Mitomo S, Colombo A, Weisz G and Latib A (2018) The Role of Cerebral Embolic Protection Devices During Transcatheter Aortic Valve Replacement. Front. Cardiovasc. Med. 5:150. doi: 10.3389/fcvm.2018.00150
Received: 28 March 2018; Accepted: 03 October 2018;
Published: 23 October 2018.
Edited by:
Crochan John O'Sullivan, Triemli Hospital, SwitzerlandReviewed by:
Gidon Yehuda Perlman, Hadassah Medical Center, IsraelDavid Chistian Reineke, Inselspital, Switzerland
Copyright © 2018 Demir, Iannopollo, Mangieri, Ancona, Regazzoli, Mitomo, Colombo, Weisz and Latib. This is an open-access article distributed under the terms of the Creative Commons Attribution License (CC BY). The use, distribution or reproduction in other forums is permitted, provided the original author(s) and the copyright owner(s) are credited and that the original publication in this journal is cited, in accordance with accepted academic practice. No use, distribution or reproduction is permitted which does not comply with these terms.
*Correspondence: Azeem Latib, alatib@gmail.com