- Radiation Institute for Science and Engineering, A Texas A&M Chancellor's Research Initiative, Prairie View A&M University, Prairie View, TX, United States
Radiation Therapy (RT) has been critical in cancer treatment regimens to date. However, it has been shown that ionizing radiation is also associated with increased risk of damage to healthy tissues. At high radiation doses, varied effects including inactivation of cells in treated tissue and associated functional impairment are seen. These range from direct damage to the heart; particularly, diffuse fibrosis of the pericardium and myocardium, adhesion of the pericardium, injury to the blood vessels and stenosis. Cardiac damage is mostly a late responding end-point, occurring anywhere between 1 and 10 years after radiation procedures. Cardiovascular disease following radiotherapy was more common with radiation treatments used before the late 1980s. Modern RT regimens with more focused radiation beams, allow tumors to be targeted more precisely and shield the heart and other healthy tissues for minimizing the radiation damage to normal cells. In this review, we discuss radiation therapeutic doses used and post-radiation damage to the heart muscle from published studies. We also emphasize the need for early detection of cardiotoxicity and the need for more cardio-protection approaches where feasible.
Introduction
Cancer associated heart disease has become a prominent cause of mortality in the industrialized world (1). Modern treatment using radiotherapy has resulted in a dramatic improvement in the chances of cancer patient's survival. While the high energy ionized radiation treatment successfully kill cancer cells, they at the same time harm healthy cells, leading to several side effects including increased cardiovascular disease in cancer survivors (2).
It is well known that nuclear industry workers and survivors of nuclear catastrophes have a significantly higher incidence of cardiovascular diseases than the general population (3–5). For the last couple of decades, it had been found that radio therapy (RT) increases the risk of associated radiation related cardiac damage in cancer survivors (6). However, a significant increase of death rate in the follow up after 10 year was found in patients post radiation therapy (7). Later studies also revealed that radiotherapy increased the cardiovascular mortality in women treated for left breast compared to those who are treated only to the right breast from earlier studies during 1970s and 1980s (8). Several population studies show that RT induced heart disease develops very slowly and often seen around 15 years after the first exposure to radiation (9).
Subsequent studies have focused on the risk of radiation-induced heart mortality as a linear-quadratic function at moderate dose levels (10) and at high dose levels a more linear response (11–13). However, no threshold dose studies have been reported; we therefore suggest that the radiation dose exposed to the heart must be minimized and limited as there is no such thing as safe radiation dose to the heart.
Studies to-date show that radiation-associated cardiac disease emerged from studies of breast cancer (14) and Hodgkin's lymphoma (15, 16) There exists enough scientific evidence to now support radiation-related heart injury as a direct effect of RT to the chest (8) (Early Breast Cancer Trialists' Collaborative Group, EBCTCG-2000). At doses above 30 Gy, heart disease may occur within a year or two of radiation exposure with concomitant increase in the risk factors for cardiovascular disease with higher radiotherapy doses. At lower doses, the latency period is longer and can extend to more than a decade (17). Cardiovascular disease as a direct side effect of radiation was more common with radiation treatment regimens used before the late 1980s. Newer radiation protocols with lower radiation doses and more focused radiation beams allow tumors to be targeted more precisely and shield the heart and other healthy tissue from direct impact of radiation. In this review we discuss radiation induced damages to the heart tissue and effectiveness of current approaches to minimize the damage.
Radiation Induced Cardiac Damage
A study of radiation doses used between the 1950s and the 1990s comparing whole heart doses for left vs. right-sided breast cancer indicate that heart doses for left-sided were higher than that for the right. The dose range was shown to be 13–17 Gy for the left breast and 2–10 Gy for the right (18). Breast radiotherapy practiced in the 1970s and 1980s resulted in more exposure to the myocardium of the heart and thereby damage, which was higher when left breast was treated (Table 1). Higher cardiovascular mortality following irradiation of the left breast as opposed to the right has been attributed to this difference (19). Swedish cancer registry documents increased mortality from myocardial infarction for patients treated for left compared with right sided tumors during 1970 and 1985 (20).

Table 1. Relative risk of Cardiac mortality after radiation for left vs. right breast cancer laterality at 95% Confidence Interval (CMR, Cardiac Mortality Ratio).
A correlation exists between RT to the thoracic region and ischemic cardiac disease with older clinical trials that are perhaps no longer standards for radiation treatment care (Table 2). It is also noted that RT for Hodgkin's lymphoma and breast cancer increases the risk factor for cardiovascular disease (26). A 3–5-fold greater incidence of cardiovascular disease has been observed in patients treated for Hodgkin's lymphoma and thereafter followed for a median of 18 years (27).
In contrast, most of these complications are reduced significantly with recent modern radiotherapeutic approaches that are designed to minimize direct cardiac dose such as three dimensional conformal radiotherapy (3DCRT) [(28–30)] and field-in-field techniques (31). Modern advances also contain better imaging technology approaches that help minimize the radiation doses to critical organs including the exposure to the heart. Among these, image guided radiotherapy (IGRT) (32), intensity modulated radiotherapy (IMRT) (33) and stereotactic body radiotherapy (SBRT) (34) provide more efficient conformation around the tumor volume, sparing organs at risk. IMRT (35–38) and accelerated partial breast irradiation (39, 40) along with practices such as deep inspiration breath hold (DIBH) vs. free breathing reduce the mean heart dose by about 50% with mean heart doses 2–3 Gy (41–46).
In a study investigating the linkage of radiotherapy to cardiovascular associated deaths, the absolute risk was seen to increase within the first 10 years for coronary disease and from the next 10 for mortality (47). To this effect, earlier measurement of cardiac damage becomes crucial to better clinical therapeutic intervention (Figure 1).
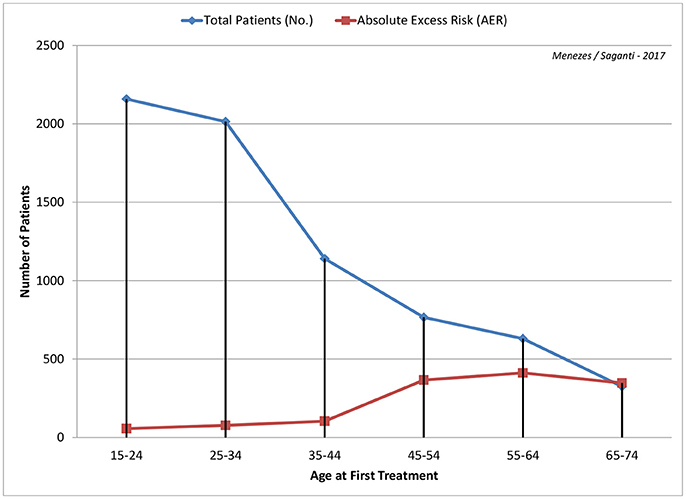
Figure 1. Age at first radiation treatment from 15 years through 74 years are shown with calculated Absolute Excess Risk (AER) per 1,000 patients is depicted with data from Swerdlow et al. (48). Higher the age, the greater the risk with about 50% around age 45 years and almost 100% by age 65 years.
A suggested protocol to identify cardiac damage - Methods that would reliably predict the progression from radiotherapy to late, irreversible cardiac damage would facilitate the development of better therapeutic measures to cardiac safety. A way to identify patients at risk for cardiac failure would help generation of some early preventive measures, individualized toward the patient. Methods could be set in place to detect and/or measure early cardiac damage such as biochemical tests. Studies for improving prediction and preventing lesions to cardiac tissue surrounding tumors such as BACCARAT (BreAst Cancer and Cardiotoxicity induced by RAdioTherapy) could improve patient care and overall quality of life (49). Atrial natriuretic peptide (ANP) levels are seen as increased in patients irradiated for Hodgkin's disease and breast cancer. This alludes to the possibility that ANP plasma levels may be an identification marker for radiation induced cardiac dysfunction (50).
Radiation Induced Vascular Changes
It is well documented that RT induces vascular endothelial dysfunction, which ultimately results in clinical cardiovascular events, manifesting many years after completion of therapy (51). Radiation induced heart conditions are described in selected studies (Table 3). The linkage of senescence of endothelial cells and atherosclerosis has been well established (68). In the preclinical setting, irradiation of the heart has been associated with endothelial cell dysfunction leading to accelerated atherosclerosis (69).
A more focused study with rodent models indicate that the radiation causes microvascular damage. Microvascular damage is manifested by a decrease in capillary density, resulting in chronic myocardial ischemia and fibrosis, whereas macrovascular disease is due to an accelerated onset of age-related atherosclerosis (70). Experimental data (53) lead to formulation of two possibilities for a mechanistic explanation of increased death from coronary artery dysfunction that follows exposure to radiation. The first, being radiation increases the frequency of myocardial dysfunction by affecting the biological pathway of age-related atherosclerosis. The second that radiation reduces the heart's tolerance to acute infarctions due to damage to the microvasculature, thereby increasing lethality. These two possible explanations may be contiguous and not necessarily exclusive acting together to produce heart disease.
Charged Particle Therapy and Heart
Particle radiation therapy applied today uses more advanced techniques and safer approaches. About 137,518 (by 2014) patients worldwide were treated with particle therapy between 1954 and 2014, 86% of which were treated with protons and 14% with carbon ions and with other particles (71). Between 2014 and 2016, in just 2 years, the total number of patients treated with particle therapy increased by 27% or 36,994 new patients to a total of 174,512 (by 2016), about 27% increase. This includes a 37% increase in new carbon ion therapy patients from 15,736 (in 2014) to 21,580 (in 2016) by 5,844. On the other hand, proton therapy patients were increased by about 26% from 118,195 (in 2014) to 149,345 by about 31,150 patients worldwide. This is a significant increase in the total number of patients who are treated with more precise radiation treatment options. A study for the late effects of radiotoxicity to the heart from this new class of patient database after 5 and 10 years is of great importance for detailed studies and assessment. Such studies are anticipated and expected to dominate the published literature in the next few years. More details of the ion therapy data worldwide are shown in Figure 3 for protons and carbon ions and in Table 4 for all other particle therapy patients.
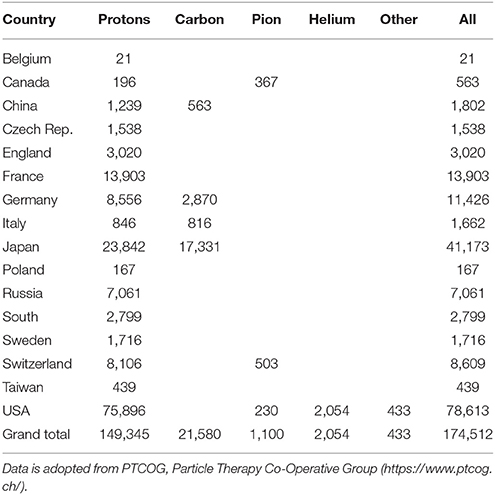
Table 4. Total number of patients who received treatment with protons, carbon, pion, helium, and other ions around the world through 2017.
Adjuvant breast radiotherapy dramatically reduced radiation dose to the heart and substantially decreased the risk of death from cardiovascular heart disease (72, 73). More efficient planning with CT scanners and accurate delivery with IMRT could be ways to protect the heart and lungs from unintentional radiation (74).
Radiation treatment with x-rays and gamma particles, which emit high energy electromagnetic radiation is absorbed completely into the target tissue, resulting in an increase of radiation dose per tissue depth. Proton and heavy ions such as carbon ions which constitute charged particles, deposit minimal energy at the entrance of the body where their velocity is greater and deposit most of the energy at the end of its range (as planned and calculated for the Bragg peak) in the tumor. Charged particles therefore present a newer advancement to RT to achieve lower and more targeted dose to tumor and reduce organ at risk (OAR). Since cardiac damage is a late event, long term follow-up data to study its effects on the heart are limiting. Charged particles operate by delivering high energy more effectively than x-rays or gamma particles, therefore they have an advantage of exhibiting a higher control of the tumor, lower probability of damage to healthy tissue, low risk of complications and a good prognosis for a rapid recovery after therapy (75); thus it is most promising for cardio-protection than conventional radio therapy.
Proton therapy may spare radiation exposure to the heart and reduce cardiotoxicity (18). The main benefit of proton therapy in breast cancer is to spare the heart from direct radiation exposure (76). The heart dose is dramatically reduced in proton therapy. A study on left breast cancer treatment using intensity radiotherapy and proton therapy using normal tissue probability showed that proton therapy has less radiation dose and damage to the heart (77). However, whether the cardiovascular disease is reduced in breast cancer survivors from proton therapy remains unclear. An undergoing study will reveal whether proton therapy decreases radiation induced cardiovascular disease in breast cancers.
In addition to the advantage of proton therapy, carbon therapy delivers higher linear energy transfer radiation (LET). High LET radiation increases radiation sensitivity to radioresistant cancer and overcomes the oxygen enhancement ratio (OER). Carbon ion therapy has also been used to stage I breast cancer without surgery at National Institute of Radiological Science (NIRS; Chiba, Japan) (78). Significant sparing of normal tissue has been demonstrated with IMRT (Intensity-modulated radiation therapy) proton treatment (79, 80), such that the dose delivered to 90% of the cochlea was reduced from 101.2% with conventional x-rays to 33.4% for IMRT beams and 2.4% for proton beams. Dose calculations to the heart recorded a reduction from 72.2% with conventional x-rays to 29.5% with IMRT and merely 0.5% with protons (Figure 2).
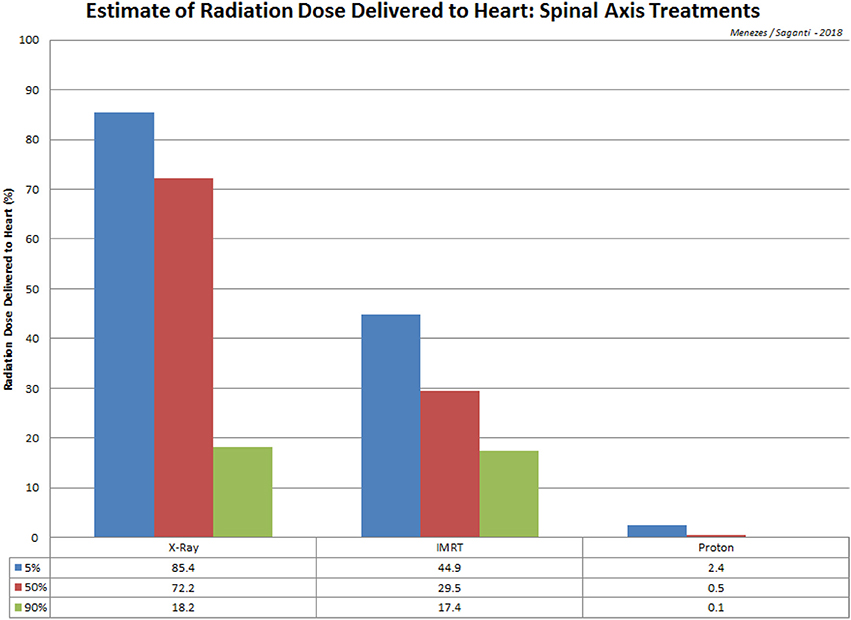
Figure 2. A comparison of radiation treatment via spinal axis and the estimated dose received at the heart for X-Ray, IMRT, and Proton procedures. Data is adopted from St Clair et al. (80).
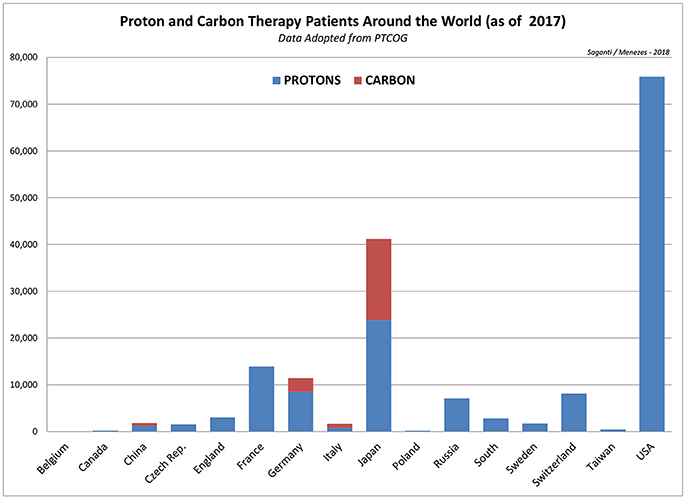
Figure 3. Depiction of worldwide patients treated with protons and carbon ions as of 2017 indicating largest number patients treated with protons (75,896) in the US and patients treated with carbon ions (17,331) in Japan. Data is adopted from PTCOG, Particle Therapy Co-Operative Group (https://www.ptcog.ch/).
Non Radiation Approaches for Preventing Damage to the Heart
Just as any other decease-prevention, mitigation, and treatment of radiation-induced cardiac injury also demands early detection. The sequences of events leading to cardiac damage that result from radiation are of several facets. To identify an early detection marker to predict risk of radiation induced cardiovascular disease is a key to prevent the late effects. Ionizing radiation induce premature aging in cultured endothelial cells (ECs) can be seen as increased apoptosis and expression of inflammatory markers (81) which in vivo are associated with EC dysfunction and atherosclerotic plaque formation (82). It has been also reported that the biological effects of ionizing radiation exposure activate NF-κB, and reduces anti-inflammatory gene expression, which in vivo are pro-atherogenic conditions (83). Also, p90RSK is a unique serine/threonine kinase with two distinct functional kinase domains (84) that has been well characterized for its role in heart failure (85, 86). Perhaps, phenomena can be used as an early detection marker of radiation induced late cardiovascular diseases.
Pharmaceutical approach to prevent RT-related cardiac injury - since the endothelium of the vasculature is thought to be one target for injury induced by radiation, pharmaceutical interventions to maintain endothelial functions are one potential strategy to mitigate and treat radiation-induced cardiac damage. The pharmaceutical drug Captopril, which is currently used to treat hypertension and congestive heart failure because of its function as angiotensin-converting enzyme (ACE) inhibitor, has been known to be able to prevent structural changes to the heart, when administered after radiation exposure (20 Gy), but there is no evidence seen in its ability to prevent the decline in cardiac function (87). However, ACE inhibitors are not evaluated for cardio protective ability with lower doses of radiation (10 Gy or lower). Similarly, the drug Simvastatin, a lipid-lowering medication for lowering cholesterol has been observed to be capable of decreasing the radiation-associated injury to rats (88). However, critical data is lacking for understanding the ability of Simvastatin to mitigate cardiac damage following radiation (89). The plant polyphenol curcumin has been shown to have a potent anti-inflammatory and antioxidant properties (90).
Cardiac muscle toxicity can result in a concomitant loss of cardiac muscle and deterioration of the vasculature, ultimately resulting in cardiac failure. Current heart failure care can alleviate symptoms but cardiac myocytes that are killed during cancer therapies cannot be replaced or regenerated with current pharmaceuticals administered to-date. In light of the fact that most pharmaceutical interventions have not yet been demonstrated to be effective to repair cardiac damage, there arises a need for early detection of cardiac toxicity (91) and development of a new generation of therapeutics that are better able to more effectively prevent the cardiac injury caused by existing cancer therapeutics (92).
Cell based therapy to prevent RT-related cardiac injury—it has been investigated as a possible future treatment strategy for heart failure patients. Co-culturing stem cells with primary cells in vitro followed by injecting in vivo have demonstrated the ability of stem cells to engraft and differentiate into cells of cardiac nature. Myocytes isolated from cardiac tissue of rats have been shown as capable of inducing cardio- myogenic differentiation of endothelial progenitor cells (93, 94) and mesenchymal stem cells (95, 96). Mesenchymal stem cells injected into hearts of pig (97) or sheep (98) following myocardial infarction, have been shown to engraft long-term, express muscle-specific proteins as well as cells of vascular and smooth muscle origin (98). Despite the expression of cardiac proteins which are good indicators of cardiac differentiation, data is lacking for the stem cell's ability for differentiating into heart cells in vivo, alluding to the fact that merely injecting stem cells into heart may not be the best approach for cardiac muscle regeneration.
Activating stem cells residing within the heart may hold more promise as a therapeutic intervention strategy for heart regeneration. Scientific data exists for the ability of resident cardiac stem cells toward differentiating into the cardiac lineage. More specifically, the percentage of this population of dividing cardiac stem cells are shown to be increased in hearts undergoing acute infarction and those with end-stage cardiomyopathy when compared with normal cardiac tissue. Additionally, these cardiac stem cells display an increased commitment toward differentiation to the cardiac myocyte, smooth muscle and endothelial cell lineages within the infarcted and end-stage hearts as compared to hearts without abnormality or disease (99–101). Ongoing research is currently aimed at this differentiation process for understanding how to selectively increase the population of cells capable of regeneration which have highly sought after value for their functionality. Therefore, perhaps the best cell source for heart muscle regeneration is most likely the resident, cardiac stem cells if the proportion that becomes a thriving functioning heart cells could be enhanced. Further studies are needed to develop the cell based therapy specially targeted RT-induced cardiac injury.
Conclusions
From various studies reviewed for this publication, it is evident that age at first radiation exposure plays a prominent role in cardiovascular related damage. The younger the age at first treatment, the greater the protection of the heart tissue and hence the lower is the risk. On the other hand, the older the age at first treatment the risk is significantly higher and the repercussions onset at an earlier time. It is also noted that by age 45–50 years, the risk of cardiovascular related damage risk increases by about 50%. This is of significant importance for general public and further studies and assessment by sex and treated conditions are to be published at a later time. We recommend more comprehensive long-term studies to be considered and evaluated as a function of time (up to ten years and beyond), sex (M/F), and radiation dose and type administered for various target sites.
A new class of radiation treatment procedures with particle therapy will be of greater challenge ahead in the years to come. At a rapid pace, nearly 20,000 patients per year during recent five years with ion therapy (protons and carbon) pose a potential challenge of cardio toxicity studies in near future. It is essential to establish the radiation related toxicity to the heart from particle therapy; it is believed that particle therapy is a rapidly growing approach for most cancer treatment protocols around the world.
Very likely it would be desirable for oncology research to encourage both medical and scientific explorations within the cardiac care and research communities to extend their follow-up for a greater period of time to discern any unforeseen cardiac complications which at present are most likely under-reported. Nonetheless, current radiation protocols far surpass the previous regimens in providing more radioprotection to critical organs including the heart. Much of the radiation related cardiotoxicity is associated with the use of traditional radiation approaches and older methods whereas the advanced modern therapies including particle therapy might reduce the immediate cardiac damage drastically. Advanced particle radiotherapy holds the promise for moving forward toward enhancing the efficacy of tumor cell killing and lowering the risk of cardiac complications from traditional radiation treatment approaches.
Author Contributions
All authors listed have made a substantial, direct and intellectual contribution to the work, and approved it for publication.
Conflict of Interest Statement
The authors declare that the research was conducted in the absence of any commercial or financial relationships that could be construed as a potential conflict of interest.
Acknowledgments
This research is supported by the Texas A&M University (TAMU) Chancellor's Research Initiative (CRI)—Radiation Institute for Science and Engineering (RaISE) at the Prairie View A&M University CRI/RaISE, Dr. Saganti (PI).
References
1. Benjamin EJ, Blaha MJ, Chiuve SE, Cushman M, Das SR, Deo R. Heart disease and stroke statistics-2017 update: a report from the American heart association. Circulation (2017) 135:e146–603. doi: 10.1161/CIR.0000000000000485
2. Abe J, Martin JF, Yeh ET. The future of onco-cardiology: we are not just side effect hunters. Circ Res. (2016) 119:896–9. doi: 10.1161/CIRCRESAHA.116.309573
3. McGeoghegan D, Binks K, Gillies M, Jones S, Whaley S. The non-cancer mortality experience of male workers at British nuclear fuels plc, 1946–2005. Int J Epidemiol. (2008) 37:506–18. doi: 10.1093/ije/dyn018
4. Wakeford R. Radiation in the workplace-a review of studies of the risks of occupational exposure to ionising radiation. J Radiol Prot. (2009) 29:A61–79. doi: 10.1088/0952-4746/29/2A/S05
5. Azimzadeh O, Azizova T, Merl-Pham J, Subramanian V, Bakshi MV, Moseeva M, et al. A dose-dependent perturbation in cardiac energy metabolism is linked to radiation-induced ischemic heart disease in Mayak nuclear workers. Oncotarget (2017) 8:9067–78. doi: 10.18632/oncotarget.10424
6. Cohn KE, Stewart JR, Fajardo LF, Hancock EW. Heart disease following radiation. Medicine (1967) 46:281–98. doi: 10.1097/00005792-196705000-00003
7. Cuzick J, Stewart H, Peto R, Baum M, Fisher B, Host H, et al. Overview of randomized trials of postoperative adjuvant radiotherapy in breast cancer. Cancer Treat Rep. (1987) 71:15–29.
8. Darby SC, McGale P, Taylor CW, Peto R. Long-term mortality from heart disease and lung cancer after radiotherapy for early breast cancer: prospective cohort study of about 300,000 women in US SEER cancer registries. Lancet Oncol. (2005) 6:557–65. doi: 10.1016/S1470-2045(05)70251-5
9. Roychoudhuri R, Robinson D, Putcha V, Cuzick J, Darby S, Moller H. Increased cardiovascular mortality more than fifteen years after radiotherapy for breast cancer: a population-based study. BMC Cancer (2007) 7:9. doi: 10.1186/1471-2407-7-9
10. Shimizu Y, Kodama K, Nishi N, Kasagi F, Suyama A, Soda M, et al. Radiation exposure and circulatory disease risk: Hiroshima and Nagasaki atomic bomb survivor data, 1950–2003. BMJ (2010) 340:b5349. doi: 10.1136/bmj.b5349
11. Bhattacharya S, Asaithamby A. Ionizing radiation and heart risks. Semin Cell Dev Biol. (2016) 58:14–25. doi: 10.1016/j.semcdb.2016.01.045
12. Darby SC, Ewertz M, McGale P, Bennet AM, Blom-Goldman U, Bronnum D, et al. Risk of ischemic heart disease in women after radiotherapy for breast cancer. N Engl J Med. (2013) 368:987–98. doi: 10.1056/NEJMoa1209825
13. van Nimwegen FA, Ntentas G, Darby SC, Schaapveld M, Hauptmann M, Lugtenburg PJ, et al. Risk of heart failure in survivors of Hodgkin lymphoma: effects of cardiac exposure to radiation and anthracyclines. Blood (2017) 129:2257–65. doi: 10.1182/blood-2016-09-740332
14. Little MP, Tawn EJ, Tzoulaki I, Wakeford R, Hildebrandt G, Paris F, et al. A systematic review of epidemiological associations between low and moderate doses of ionizing radiation and late cardiovascular effects, and their possible mechanisms. Radiation Res. (2008) 169:99–109. doi: 10.1667/RR1070.1
15. Hancock EW. Heart disease after radiation. N Engl J Med. (1983) 308:588. doi: 10.1056/NEJM198303103081010
16. Maraldo MV, Giusti F, Vogelius IR, Lundemann MM, van der Kaaij A, Ramadan S, et al. Cardiovascular disease after treatment for Hodgkin's lymphoma: an analysis of nine collaborative EORTC-LYSA trials. Lancet Haematol. (2015) 2:e492–502. doi: 10.1016/S2352-3026(15)00153-2
17. Hull MC, Morris CG, Pepine CJ, Mendenhall NP. Valvular dysfunction and carotid, subclavian, and coronary artery disease in survivors of hodgkin lymphoma treated with radiation therapy. JAMA (2003) 290:2831–7. doi: 10.1001/jama.290.21.2831
18. Taylor CW, Nisbet A, McGale P, Darby SC. Cardiac exposures in breast cancer radiotherapy: 1950s−1990s. Int J Radiat Oncol Biol Phys. (2007) 69:1484–95. doi: 10.1016/j.ijrobp.2007.05.034
19. Raghunathan D, Khilji MI, Hassan SA, Yusuf SW. Radiation-induced cardiovascular disease. Curr Atheroscler Rep. (2017) 19:22. doi: 10.1007/s11883-017-0658-x
20. Rutqvist LE, Johansson H. Mortality by laterality of the primary tumour among 55,000 breast cancer patients from the Swedish Cancer Registry. Br J Cancer (1990) 61:866–8. doi: 10.1038/bjc.1990.193
21. Brosius FC III, Waller BF, Roberts WC. Radiation heart disease: analysis of 16 young (aged 15 to 33 years) necropsy patients who received over 3,500 rads to the heart. Am J Med. (1981) 70:519–30.
22. Applefeld MM, Wiernik PH. Cardiac disease after radiation therapy for Hodgkin's disease: analysis of 48 patients. Am J Cardiol. (1983) 51:1679–81.
23. Orzan F, Brusca A, Conte MR, Presbitero P, Figliomeni MC. Severe coronary artery disease after radiation therapy of the chest and mediastinum: clinical presentation and treatment.Br Heart (1993) 69:496–500.
24. Veinot JP, Edwards WD. Pathology of radiation-induced heart disease: a surgical and autopsy study of 27 cases. Hum Pathol. (1996) 27:766–73.
25. Erven K, Florian A, Slagmolen P, Sweldens C, Jurcut R, Wildiers H, et al. Subclinical cardiotoxicity detected by strain rate imaging up to 14 months after breast radiation therapy. Int J Radiat Oncol Biol Phys. (2013) 85:1172–8. doi: 10.1016/j.ijrobp.2012.09.022
26. Adams MJ, Hardenbergh PH, Constine LS, Lipshultz SE. Radiation-associated cardiovascular disease. Crit Rev Oncol Hematol. (2003) 45:55–75. doi: 10.1016/S1040-8428(01)00227-X
27. Aleman BM, van den Belt-Dusebout AW, De Bruin ML, van 't Veer MB, Baaijens MH, de Boer JP, et al. Late cardiotoxicity after treatment for Hodgkin lymphoma. Blood (2007) 109:1878–86. doi: 10.1182/blood-2006-07-034405
28. Erven K, Jurcut R, Weltens C, Giusca S, Ector J, Wildiers HW, et al. Acute radiation effects on cardiac function detected by strain rate imaging in breast cancer patients. Int J Radiat Oncol Biol Phys. (2011) 79:1444–51. doi: 10.1016/j.ijrobp.2010.01.004
29. Vivekanandan N, Sriram P, Kumar SA, Bhuvaneswari N, and Saranya K. Volumetric modulated arc radiotherapy for esophageal cancer. Med Dosim. (2012) 37:108–13. doi: 10.1016/j.meddos.2011.01.008
31. Ercan T, Igdem S, Alco G, Zengin F, Atilla S, Dincer M, et al. Dosimetric comparison of field in field intensity-modulated radiotherapy technique with conformal radiotherapy techniques in breast cancer. Jpn J Radiol. (2010) 28:283–9. doi: 10.1007/s11604-010-0423-3
32. Jaffray DA. Image-guided radiotherapy: from current concept to future perspectives. Nat Rev Clin Oncol. (2012) 9:688–99. doi: 10.1038/nrclinonc.2012.194
33. Bortfeld T. IMRT: a review and preview. Phys Med Biol. (2006) 51:R363–79. doi: 10.1088/0031-9155/51/13/R21
34. Teh BS, Ishiyama H, Mathews T, Xu B, Butler EB, Mayr NA, et al. Stereotactic body radiation therapy (SBRT) for genitourinary malignancies. Discov Med. (2010) 10:255–62.
35. Cozzi L, Fogliata A, Bolsi A, Nicolini G, Bernier J. Three-dimensional conformal vs. intensity-modulated radiotherapy in head-and-neck cancer patients: comparative analysis of dosimetric and technical parameters. Int J Radiat Oncol Biol Phys. (2004) 58:617–24. doi: 10.1016/j.ijrobp.2003.09.059
36. Jin X, Yi J, Zhou Y, Yan H, Han C, Xie C. Comparison of whole-field simultaneous integrated boost VMAT and IMRT in the treatment of nasopharyngeal cancer. Med Dosim. (2013) 38:418–23. doi: 10.1016/j.meddos.2013.05.004
37. Liem X, Chira C, Fourquet A, Campana F, Peurien D, Fournier-Bidoz N, et al. Preliminary results of whole breast helical tomotherapy with simultaneous integrated boost in the adjuvant treatment of breast cancer. Cancer Radiother. (2014) 18:15–22. doi: 10.1016/j.canrad.2013.07.149
38. Zhang X, Li X, Quan EM, Pan X, Li Y. A methodology for automatic intensity-modulated radiation treatment planning for lung cancer. Phys Med Biol. (2011) 56:3873–93. doi: 10.1088/0031-9155/56/13/009
39. Smith BD, Arthur DW, Buchholz TA, Haffty BG, Hahn CA, Hardenbergh PH, et al. Accelerated partial breast irradiation consensus statement from the American Society for Radiation Oncology (ASTRO). J Am Coll Surg. (2009) 209:269–77. doi: 10.1016/j.jamcollsurg.2009.02.066
40. Shah C, Badiyan S, Ben Wilkinson J, Vicini F, Beitsch P, Keisch M, et al. Treatment efficacy with accelerated partial breast irradiation (APBI): final analysis of the American society of breast surgeons MammoSite((R)) breast brachytherapy registry trial. Ann Surg Oncol. (2013) 20:3279–85. doi: 10.1245/s10434-013-3158-4
41. Remouchamps VM, Letts N, Vicini FA, Sharpe MB, Kestin LL, Chen PY, et al. Initial clinical experience with moderate deep-inspiration breath hold using an active breathing control device in the treatment of patients with left-sided breast cancer using external beam radiation therapy. Int J Radiat Oncol Biol Phys. (2003) 56:704–15. doi: 10.1016/S0360-3016(03)00010-5
42. Stranzl H, Zurl B, Langsenlehner T, Kapp KS. Wide tangential fields including the internal mammary lymph nodes in patients with left-sided breast cancer. Influence of respiratory-controlled radiotherapy (4D-CT) on cardiac exposure Strahlenther Onkol (2009). 185:155–60. doi: 10.1007/s00066-009-1939-2
43. McIntosh A, Shoushtari AN, Benedict SH, Read PW, Wijesooriya K. Quantifying the reproducibility of heart position during treatment and corresponding delivered heart dose in voluntary deep inhalation breath hold for left breast cancer patients treated with external beam radiotherapy. Int J Radiat Oncol Biol Phys. (2011) 81:e569–76. doi: 10.1016/j.ijrobp.2011.01.044
44. Hayden AJ, Rains M, Tiver K. Deep inspiration breath hold technique reduces heart dose from radiotherapy for left-sided breast cancer. J Med Imaging Radiat Oncol. (2012) 56:464–72. doi: 10.1111/j.1754-9485.2012.02405.x
45. Hjelstuen MH, Mjaaland I, Vikstrom J, Dybvik KI. Radiation during deep inspiration allows loco-regional treatment of left breast and axillary-, supraclavicular- and internal mammary lymph nodes without compromising target coverage or dose restrictions to organs at risk. Acta Oncol. (2012) 51:333–44. doi: 10.3109/0284186X.2011.618510
46. Swanson T, Grills IS, Ye H, Entwistle A, Teahan M, Letts N, et al. Six-year experience routinely using moderate deep inspiration breath-hold for the reduction of cardiac dose in left-sided breast irradiation for patients with early-stage or locally advanced breast cancer. Am J Clin Oncol. (2013) 36:24–30. doi: 10.1097/COC.0b013e31823fe481
47. Cheng Y-J, Nie X-Y, Ji C-C, Lin X-X, Liu L-J, Chen X-M, et al. Long-term cardiovascular risk after radiotherapy in women with breast cancer. J Am Heart Assoc. (2017) 6:e005633. doi: 10.1161/JAHA.117.005633
48. Swerdlow AJ, Higgins CD, Smith P, Cunningham D, Hancock BW, Horwich A, et al. Myocardial infarction mortality risk after treatment for Hodgkin disease: a collaborative British cohort study. J Natl Cancer Inst. (2007) 99:206–14. doi: 10.1093/jnci/djk029
49. Jacob S, Pathak A, Franck D, Latorzeff I, Jimenez G, Fondard O, et al. Early detection and prediction of cardiotoxicity after radiation therapy for breast cancer: the BACCARAT prospective cohort study. Radiat Oncol. (2016) 11:54. doi: 10.1186/s13014-016-0627-5
50. Wondergem J, Strootman EG, Frolich M, Leer JW, Noordijk EM. Circulating atrial natriuretic peptide plasma levels as a marker for cardiac damage after radiotherapy. Radiother Oncol. (2001) 58:295–301. doi: 10.1016/S0167-8140(00)00303-0
51. Stewart FA. Mechanisms and dose-response relationships for radiation-induced cardiovascular disease. Ann ICRP (2012) 41:72–9. doi: 10.1016/j.icrp.2012.06.031
52. Murros KE, Toole JF. The effect of radiation on carotid arteries: a review article. Arch Neurol. (1989) 46:449–55.
53. Stewart FA, Heeneman S, Te Poele J, Kruse J, Russell NS, Gijbels M, et al. Ionizing radiation accelerates the development of atherosclerotic lesions in ApoE-/- mice and predisposes to an inflammatory plaque phenotype prone to hemorrhage. Am J Pathol. (2006) 168:649–58. doi: 10.2353/ajpath.2006.050409
54. Gujral DM, Lloyd G, Bhattacharyya S. Radiation-induced valvular heart disease. Heart. (2016) 102:269–76. doi: 10.1136/heartjnl-2015-308765
55. Posner MR, Cohen GI, Skarin AT. Pericardial disease in patients with cancer: the differentiation of malignant from idiopathic and radiation-induced pericarditis. Am J Med. (1981) 71:407–13.
56. Stewart JR, Fajardo LF, Gillette SM, Constine LS. Radiation injury to the heart. Int J Radiat Oncol Biol Phys. (1995) 31:1205–11. doi: 10.1016/0360-3016(94)00656-6
57. McChesney SL, Gillette EL, Powers BE. Radiation-induced cardiomyopathy in the dog. Radiat Res. (1988) 113:120–32.
58. Wright TL, Bresnan MJ. Radiation-induced cerebrovascular disease in children. Neurology (1976) 26:540–3.
59. Ivanov VK, Maksioutov MA, Chekin SY, Petrov AV, Biryukov AP, Kruglova ZG. The risk of radiation-induced cerebrovascular disease in Chernobyl emergency workers. Health Phys. (2006) 90:199–207. doi: 10.1097/01.HP.0000175835.31663.ea
60. Morris B1, Partap S, Yeom K, Gibbs IC, Fisher PG, King AA. Cerebrovascular disease in childhood cancer survivors: a Children's Oncology Group Report. Neurology (2009) 73:1906–13. doi: 10.1212/WNL.0b013e3181c17ea8
61. Smith GL, Smith BD, Buchholz TA, Giordano SH, Garden AS, Woodward WA. Cerebrovascular disease risk in older head and neck cancer patients after radiotherapy. J Clin Oncol. (2008) 26:5119–25. doi: 10.1200/JCO.2008.16.6546
62. McReynolds, Richard A, Gold GL, Roberts WR. Coronary heart disease after mediastinal irradiation for Hodgkin's disease. Am J Med. (1976) 60:39–45.
63. Gyenes G. Radiation-induced ischemic heart disease in breast cancer: a review. Acta Oncol. (1998) 37:241–6.
64. Morton DL, Kagan AR, Roberts WC, O'Brien KP, Holmes EC. Pericardiectomy for radiation-induced pericarditis with effusion. Ann Thorac Surg. (1969) 8:195–208.
65. Morton DL, Joseph WL, Ketcham AS, Geelhoed GW, Adkins PC. Surgical resection and adjunctive immunotherapy for selected patients with multiple pulmonary metastases. Ann Surg. (1973) 178:360.
66. Mill WB, Baglan RJ, Kurichety P, Prasad S, Lee JY, Moller R. Symptomatic radiation-induced pericarditis in Hodgkin's disease. Int J Radiat Oncol Biol Phys. (1984) 10:2061–5.
67. Stewart JR, Fajardo LF. Radiation-induced heart disease: an update. Prog Cardiovasc Dis. (1984) 27:173–94.
68. Minamino T, Miyauchi H, Yoshida T, Ishida Y, Yoshida H, Komuro I. Endothelial cell senescence in human atherosclerosis: role of telomere in endothelial dysfunction. Circulation (2002) 105:1541–4. doi: 10.1161/01.CIR.0000013836.85741.17
69. Gabriels K, Hoving S, Seemann I, Visser NL, Gijbels MJ, Pol JF, et al. Local heart irradiation of ApoE(-/-) mice induces microvascular and endocardial damage and accelerates coronary atherosclerosis. Radiother Oncol. (2012) 105:358–64. doi: 10.1016/j.radonc.2012.08.002
70. Darby SC, Cutter DJ, Boerma M, Constine LS, Fajardo LF, Kodama K, et al. Radiation-related heart disease: current knowledge and future prospects. Int J Radiat Oncol Biol Phys. (2010) 76:656–65. doi: 10.1016/j.ijrobp.2009.09.064
71. Jermann M. Particle therapy statistics in 2014. Int J Part Ther. (2015) 2:50–4. doi: 10.14338/IJPT-15-00013
72. Giordano SH, Kuo YF, Freeman JL, Buchholz TA, Hortobagyi GN, Goodwin JS. Risk of cardiac death after adjuvant radiotherapy for breast cancer. J Natl Cancer Inst. (2005) 97:419–24. doi: 10.1093/jnci/dji067
73. Cuaron JJ, Chon B, Tsai H, Goenka A, DeBlois D, Ho A, et al. Early toxicity in patients treated with postoperative proton therapy for locally advanced breast cancer. Int J Radiat Oncol Biol Phys. (2015) 92:284–91. doi: 10.1016/j.ijrobp.2015.01.005
74. Glide-Hurst CK, Chetty IJ. Improving radiotherapy planning, delivery accuracy, and normal tissue sparing using cutting edge technologies. J Thorac Dis. (2014) 6:303–18. doi: 10.3978/j.issn.2072-1439.2013.11.10
75. Loeffler JS, Durante M. Charged particle therapy–optimization, challenges and future directions. Nat Rev Clin Oncol. (2013) 10:411–24. doi: 10.1038/nrclinonc.2013.79
76. MacDonald SM. Proton therapy for breast cancer: getting to the heart of the matter. Int J Radiat Oncol Biol Phys. (2016) 95:46–8. doi: 10.1016/j.ijrobp.2015.11.035
77. Tommasino F, Durante M, D'Avino V, Liuzzi R, Conson M, Farace P, et al. Model-based approach for quantitative estimates of skin, heart, and lung toxicity risk for left-side photon and proton irradiation after breast-conserving surgery. Acta Oncol. (2017) 56:730–6. doi: 10.1080/0284186X.2017.1299218
78. Akamatsu H, Karasawa K, Omatsu T, Isobe Y, Ogata R, Koba Y. First experience of carbon-ion radiotherapy for early breast cancer. Jpn J Radiol. (2014) 32:288–95. doi: 10.1007/s11604-014-0300-6
79. Moon SH, Shin KH, Kim TH, Yoon M, Park S, Lee DH, et al. Dosimetric comparison of four different external beam partial breast irradiation techniques: three-dimensional conformal radiotherapy, intensity-modulated radiotherapy, helical tomotherapy, and proton beam therapy. Radiother Oncol. (2009) 90:66–73. doi: 10.1016/j.radonc.2008.09.027
80. St Clair WH, Adams JA, Bues M, Fullerton BC, La Shell S, Kooy HM, et al. Advantage of protons compared to conventional X-ray or IMRT in the treatment of a pediatric patient with medulloblastoma. Int J Radiat Oncol Biol Phys. (2004) 58:727–34. doi: 10.1016/S0360-3016(03)01574-8
81. Lowe D, Raj K. Premature aging induced by radiation exhibits pro-atherosclerotic effects mediated by epigenetic activation of CD44 expression. Aging Cell (2014) 13:900–10. doi: 10.1111/acel.12253
82. Heo KS, Fujiwara K, Abe J. Disturbed-flow-mediated vascular reactive oxygen species induce endothelial dysfunction. Circ J. (2011) 75:2722–30. doi: 10.1253/circj.CJ-11-1124
83. Frodin M, Gammeltoft S. Role and regulation of 90 kDa ribosomal S6 kinase (RSK) in signal transduction. Mol Cell Endocrinol. (1999) 151:65–77. doi: 10.1016/S0303-7207(99)00061-1
84. Fisher TL, Blenis J. Evidence for two catalytically active kinase domains in pp90rsk. Mol Cell Biol. (1996) 16:1212–9. doi: 10.1128/MCB.16.3.1212
85. Itoh S, Ding B, Shishido T, Lerner-Marmarosh N, Wang N, Maekawa N, et al. Role of p90 ribosomal S6 kinase-mediated prorenin-converting enzyme in ischemic and diabetic myocardium. Circulation (2006) 113:1787–98. doi: 10.1161/CIRCULATIONAHA.105.578278
86. Maekawa N, Abe J, Shishido T, Itoh S, Ding B, Sharma VK, et al. Inhibiting p90 ribosomal S6 kinase prevents (Na+)-H+ exchanger-mediated cardiac ischemia-reperfusion injury. Circulation (2006) 113:2516–23. doi: 10.1161/CIRCULATIONAHA.105.563486
87. Yarom R, Harper IS, Wynchank S, van Schalkwyk D, Madhoo J, Williams K, et al. Effect of captopril on changes in rats' hearts induced by long-term irradiation. Radiat Res. (1993) 133:187–97. doi: 10.2307/3578356
88. Inano H, Suzuki K, Onoda M, Wakabayashi K. Anti-carcinogenic activity of simvastatin during the promotion phase of radiation-induced mammary tumorigenesis of rats. Carcinogenesis (1997) 18:1723–7. doi: 10.1093/carcin/18.9.1723
89. Baker JE, Moulder JE, Hopewell JW. Radiation as a risk factor for cardiovascular disease. Antioxid Redox Signal. (2011) 15:1945–56. doi: 10.1089/ars.2010.3742
90. Lubbad A, Oriowo MA, Khan I. Curcumin attenuates inflammation through inhibition of TLR-4 receptor in experimental colitis. Mol Cell Biochem. (2009) 322:127–35. doi: 10.1007/s11010-008-9949-4
91. Altena R, Perik PJ, van Veldhuisen DJ, de Vries EG, Gietema JA. Cardiovascular toxicity caused by cancer treatment: strategies for early detection. Lancet Oncol. (2009) 10:391–9. doi: 10.1016/S1470-2045(09)70042-7
92. Begg AC, Stewart FA, Vens C. Strategies to improve radiotherapy with targeted drugs. Nat Rev Cancer (2011) 11:239–53. doi: 10.1038/nrc3007
93. Condorelli G, Borello U, De Angelis L, Latronico M, Sirabella D, Coletta M, et al. Cardiomyocytes induce endothelial cells to trans-differentiate into cardiac muscle: implications for myocardium regeneration. Proc Natl Acad Sci USA. (2001) 98:10733–8. doi: 10.1073/pnas.191217898
94. Badorff C, Brandes RP, Popp R, Rupp S, Urbich C, Aicher A, et al. Transdifferentiation of blood-derived human adult endothelial progenitor cells into functionally active cardiomyocytes. Circulation (2003) 107:1024–32. doi: 10.1161/01.CIR.0000051460.85800.BB
95. Shim WS, Jiang S, Wong P, Tan J, Chua YL, Tan YS, et al. Ex vivo differentiation of human adult bone marrow stem cells into cardiomyocyte-like cells. Biochem Biophys Res Commun. (2004) 324:481–8. doi: 10.1016/j.bbrc.2004.09.087
96. Li X, Yu X, Lin Q, Deng C, Shan Z, Yang M, et al. Bone marrow mesenchymal stem cells differentiate into functional cardiac phenotypes by cardiac microenvironment. J Mol Cell Cardiol. (2007) 42:295–303. doi: 10.1016/j.yjmcc.2006.07.002
97. Amado LC, Saliaris AP, Schuleri KH, St John M, Xie JS, Cattaneo S, et al. Cardiac repair with intramyocardial injection of allogeneic mesenchymal stem cells after myocardial infarction. Proc Natl Acad Sci USA. (2005) 102:11474–9. doi: 10.1073/pnas.0504388102
98. Liechty KW, MacKenzie TC, Shaaban AF, Radu A, Moseley AM, Deans R, et al. Human mesenchymal stem cells engraft and demonstrate site-specific differentiation after in utero transplantation in sheep. Nat Med. (2000) 6:1282–6. doi: 10.1038/81395
99. Urbanek K, Torella D, Sheikh F, De Angelis A, Nurzynska D, Silvestri F, et al. Myocardial regeneration by activation of multipotent cardiac stem cells in ischemic heart failure. Proc Natl Acad Sci USA. (2005) 102:8692–7. doi: 10.1073/pnas.0500169102
100. Crile G. Jr.. Results of simple mastectomy without irradiation in the treatment of operative stage I cancer of the breast. Ann Surg. (1968) 168:330–6. doi: 10.1097/00000658-196809000-00003
Keywords: radiation therapy, proton therapy, heavy ion radiotherapy, ionizing radiation cardiotoxicity, charged particle therapy, cardiovascular disease, radiation damage to the heart
Citation: Menezes KM, Wang H, Hada M and Saganti PB (2018) Radiation Matters of the Heart: A Mini Review. Front. Cardiovasc. Med. 5:83. doi: 10.3389/fcvm.2018.00083
Received: 31 January 2018; Accepted: 12 June 2018;
Published: 09 July 2018.
Edited by:
Giovanni Esposito, Università degli Studi di Napoli Federico II, ItalyReviewed by:
Feixiong Cheng, Northeastern University, United StatesDavid A. Gewirtz, Virginia Commonwealth University, United States
Copyright © 2018 Menezes, Wang, Hada and Saganti. This is an open-access article distributed under the terms of the Creative Commons Attribution License (CC BY). The use, distribution or reproduction in other forums is permitted, provided the original author(s) and the copyright owner(s) are credited and that the original publication in this journal is cited, in accordance with accepted academic practice. No use, distribution or reproduction is permitted which does not comply with these terms.
*Correspondence: Premkumar B. Saganti, cGJzYWdhbnRpQHB2YW11LmVkdQ==