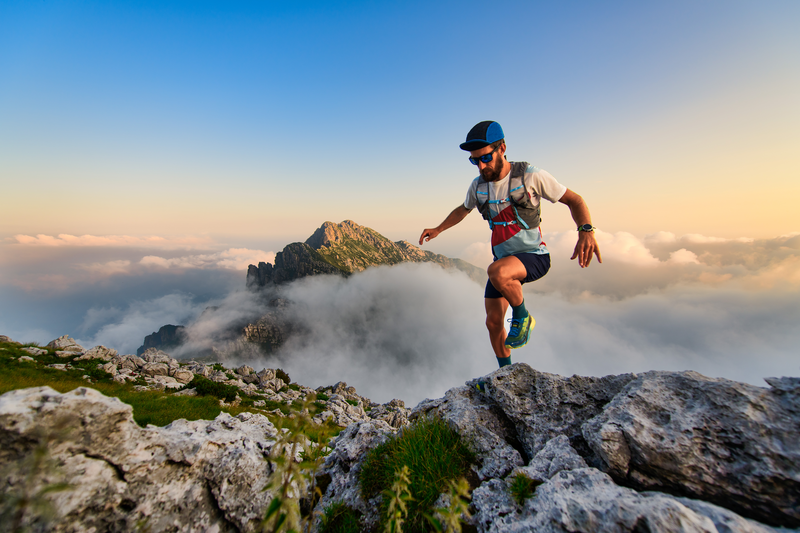
95% of researchers rate our articles as excellent or good
Learn more about the work of our research integrity team to safeguard the quality of each article we publish.
Find out more
REVIEW article
Front. Catal. , 06 April 2022
Sec. Heterogeneous Catalysis
Volume 2 - 2022 | https://doi.org/10.3389/fctls.2022.839072
This article is part of the Research Topic Horizons in Catalysis View all 9 articles
Solar energy transformation over semiconductor-based photocatalysis is an ideal solution to environmental problems and future sustainability. Layered bismuth oxyhalides (BiOX, X = Cl, Br or I) are very attractive and promising photocatalysts in the environment fields. This review summarizes recent advances on the design of BiOX to enhance energy converting efficiency. Especially, the emerging techniques to enhance the photocatalytic behaviors of BiOX are discussed, including non-metal/metal doping, heterojunction engineering, carbon interfacing, coupling with noble metals, defect engineering, and morphology tuning. The application of BiOX composites in wastewater remediation is also reviewed in terms of organic photocatalytic oxidation and heavy metal ion photocatalytic reduction. Finally, the future chances and challenges of BiOX photocatalysts for practical application are summarized. In all, this review well underlies the innovative preparation of BiOX products for environment-related purposes.
Water pollution and unavailability of clean and inexpensive water are among the biggest challenges of the 21st century (Qu et al., 2013). Water shortage attacks 1/3 of the global population, and clean drinking water is inaccessible to one billion people (WHO/UNICEF Joint Monitoring Programme for Water Supply and Sanitation and UNICEF, 2005). The remarkable enhancement in living standards since the industrial revolution increases both the demand for freshwater and the amount/variety of polluting chemicals in water (Tollefson, 2011; Richardson, 2012; Carlsen et al., 2013). The existence of emerging contaminants, including toxic heavy metal ions, antibiotics, and endocrine disrupting chemicals (Fatta-Kassinos et al., 2011; Bueno et al., 2012), in water resources made the EU Commission Services establish a new guidance on environmental quality criteria to guarantee the quality of drinking water and to preserve the aquatic environment. Among the existent technologies for water and wastewater treatment, the most common methods include adsorption, membranes, chemical oxidation, and biological treatments. However, these technologies are relatively inefficient in eliminating emerging and persistent organic compounds. The advanced oxidation processes (AOPs) based on in situ formation of largely reactive species (HO• and O2-•) can convert organic contaminants into less harmful substances (e.g., CO2 and H2O) (Lee and Park, 2013). Various AOPs such as photochemical oxidation (UV/O3 and UV/H2O2), chemical oxidation (O3 and Fenton reagents), and heterogeneous photocatalysis (UV/TiO2) (Hidalgo et al., 2007; Malato et al., 2007) are available for wastewater processing. Among the most explored and economical AOPs, photocatalysis needs only light energy without extra chemicals and runs at mild pressure and temperature (Lin et al., 2015; Landge et al., 2021). Semiconductor materials are by far the most common form of photocatalysts since they can be photoexcited by photons of energy equal to or above the bandgap energy to produce charge carriers for surface redox reactions. The overall process of photocatalysis takes five steps (Herrmann, 1999; Herrmann, 2005):
1) mass migration of reactants from the bulk medium to the catalyst–fluid interfacial region;
2) surface adsorption of the reactants;
3) photocatalyst activation and surface reaction:
a absorption of photons by the catalyst
b generation of electron-hole (e−-h+) pairs
c diffusion of photogenerated charges to the surface and
d surface transfer of the photocharges to the reactants (i.e., surface reaction)
4) product desorption from the photocatalyst surface; and
5) mass migration of the products from the interface to the bulk fluid.
In particular, for a semiconductor photocatalyst, when irradiated light arrives at its valance band (VB), a VB electron is excited to the conduction band (CB), forming electron-hole pairs. Most of the photocharges that fail to transport away very quickly will recombine. The photocharges can also be trapped by various bulk and surface sites during the diffusion to the surface. As a result, only a fraction of the photocharges will arrive at the surface to catalyze redox reactions (Hoffmann et al., 1995). Figure 1 displays all the reactions on the catalyst surface mentioned above.
FIGURE 1. Schematic map of the photocatalysis mechanism over an organic contaminant in water (Linsebigler et al., 1995).
Common photocatalysts (e.g., ZnO and TiO2) (Raizada et al., 2016) mostly can degrade organic pollutants in water under UV irradiation (<5% solar spectrum), but their applications are limited by the low light-gathering ability, fast photocharge carrier recombination, and narrow feasible range of pH. Therefore, photocatalysts driven by visible light (∼43% of solar light) with wide pH tolerance and fast division of photocharge carriers are needed. Recently, bismuth-based photocatalysts such as Bi2O3 (Gadhi et al., 2016; Jalalah et al., 2015; Oudghiri-Hassani et al., 2015), Bi2WO6 (Fu et al., 2005; Zhang and Zhu, 2005; Zhang et al., 2009), BiVO4 (Yu and Kudo, 2006; Luo et al., 2011), Bi2MoO6 (Bi et al., 2007; Zhang et al., 2010), BiPO4 (Pan and Zhu, 2011; Pan and Zhu, 2010), Bi2Ti2O7 (Yao et al., 2004), (BiO)2CO3 (Ni et al., 2016), BiOCOOH (Xiong et al., 2011a), and bismuth oxyhalides (BiOX, X = Cl, Br, I) (Henle et al., 2007; Huang and Zhu, 2008; Huizhong et al., 2008; Zhang et al., 2008; Chang et al., 2010; Chen et al., 2013; Cheng et al., 2014; Ye et al., 2014; Yang et al., 2018) have been used in visible-light–driven water purification. Especially, BiOX with its chemical inertness, photocorrosion tolerance, and harmlessness in aqueous media has received wide attention. The BiOX becomes crystals in matlockite (PbFCl-type) tetragons (space group P4/nmm). The crystal lattice comprises [Bi2O2]2+ slice interlayers between halogen anion dual-slabs, gathering along the z-axis by the nonbonding (van der Waals) interaction (Figure 2A) (Chen et al., 2013). This two-dimensional layered structure of BiOX facilitates the formation of an inner electric field between halogen and [Bi2O2]2+ slabs owing to the transformation of photoexcited electron-hole pairs (Zhang et al., 2006). The VB of BiOX is constituted by X p (X = Cl, Br or I) orbitals and O 2p orbitals (Tu et al., 2012; Zhang et al., 2012; Zhao et al., 2013) while the CB comprises Bi 6p orbitals (Cao J. et al., 2012; Zhang et al., 2013). Density functional theory (DFT) calculation indicates the distance between two slabs is elongated with the rise of the halogen atom count, following the narrowed bandgap, such as BiOCl (∼3.2 eV) (Ma Z. et al., 2017), BiOBr (∼2.6 eV) (Li K. L. et al., 2014; Kong et al., 2016), and BiOI (∼1.8 eV) (Xiao and Zhang, 2010; Ye et al., 2011) (Figure 2B). Thereby, the light absorbing range can be prolonged from ultraviolet to visible light.
FIGURE 2. (A) Crystal structures of BiOX (X = Cl, Br, I) (modified with permission (Ganose et al., 2016)). (B) Schematic VB and CB structures of BiOX (data from Ref (Guo et al., 2021)).
In 2006, Zhang et al. prepared the BiOCl using the hydrolysis method, and for the first time, they found that BiOCl had a better performance than TiO2 (P25, Degussa) on photocatalytic degradation of methyl orange (MO) dye (Zhang et al., 2006). In addition, Zhang and co-workers synthesized hierarchical BiOCl nanoplate microspheres using the one-pot solvothermal method. The as-obtained BiOCl showed 1.7 times higher photocatalytic activity than TiO2 toward MO degradation (Zhang et al., 2008). In 2015, Ding et al. prepared the BiOCl hierarchical microsphere (2–50 nm) through controlling pH in the reaction precursor, and the samples showed a preferable photocatalytic activity for rhodamine B (RhB) dye degradation (Ding et al., 2015). Although BiOCl has better performance than TiO2, the large band gap energy (∼3.2 eV) limited their application under visible light.
BiOBr had a narrower band gap (∼2.6 eV) than BiOCl and thus has been used under visible irradiation in recent years. Zhang et al. prepared the BiOBr microspheres using the ethylene glycol (EG) assisting solvothermal method (Zhang et al., 2008). Due to the narrow band gap of BiOBr, it shows 2.5 times and 3.5 times higher catalytic activity than TiO2 under UV and visible light irradiation. In addition, the flower-like hierarchical architectures of BiOBr was prepared by Jia et al., who employed tetrabutylammonium halide as halogen sources in ethanol solvent (Jia et al., 2015). The as-obtained BiOBr can degrade rhodamine B and salicylic acid under visible light.
The BiOI owned the narrowest band gap energy (∼1.8 eV) in BiOX and thus had the best performance under visible light in principle. However, the significant recombination of photocharges in pristine BiOI limited their photocatalytic activity. The BiOI hierarchical nanospheres show a better adsorption (57.4% removal) on MO dye than photocatalytic activity (∼42.6% removal) (Li R. et al., 2015). Therefore, the BiOI was always used as light harvesting for extending the light adsorption of composites. For example, Zhang et al. prepared the TiO2/BiOI p-n junction by using the hydrothermal-solvothermal route. The light absorption of composites can be extended to the visible region, and the separation efficiency of photocharges in TiO2 can be improved. As a result, the TiO2/BiOI composites show 16.6 times higher methylene blue degradation than TiO2 (Zhang Y. et al., 2021).
Although the favorable bandgap can be obtained by changing halogen atoms, the photocatalytic ability of pristine BiOX is still inefficient due to the fast electron-hole recombination and the decreased redox ability (Guo et al., 2021). In other words, BiOX can hardly keep responsive light sensitivity, rapid carrier movement, and high redox capability at the same time.
Many methods can be used to restrict charge carrier recombination in BiOX during heterogeneous photocatalysis. One common way is to decorate BiOX materials with another semiconductor to form type II heterojunctions, such as Bi2WO6 (Meng and Zhang, 2015; Xiang et al., 2016; Wang F. et al., 2018; Wang J. et al., 2019; Tahmasebi et al., 2019), TiO2 (Park et al., 2012; Yang J. et al., 2014; Choi et al., 2016; Yao et al., 2020), and Cu2O (Cao et al., 2015; Zhang Y. et al., 2020a). Another strategy is the deposition of noble metals: the generally lower Fermi energy of metal nanoparticles than that of BiOX promotes electron migration from the semiconductor to the metal via Schottky contact. The most common decorative metals include platinum (Pt), silver (Ag), and gold (Au) owing to their noble and/or catalytic properties (Yu et al., 2013; Gao et al., 2014; Bi et al., 2016; Liu et al., 2018; Zhou S. et al., 2019; Yadav et al., 2021). They all function as electron sinks/reservoirs, spatially isolating the electrons from the VC photoholes of BiOX, and as more-active sites and co-catalysts for photocatalytic reduction. Another useful method for extracting photoelectrons from BiOX to prevent charge carrier recombination is to prepare composites with carbon materials, particularly graphene-based nanomaterials (Chou et al., 2016; Dai and Zhao, 2017; Lee et al., 2017; Garg et al., 2018a; Su et al., 2018; Sharma N. et al., 2019; Zhang W. et al., 2019; Zhu et al., 2019; Yadav et al., 2020; Alansi et al., 2021; Sharma et al., 2022). Because of the fast electron motion in graphene, photoelectrons from the CB of BiOX to graphene are easily isolated from the BiOX surface. The other methods including defect engineering, Bi-rich strategy, morphology, and thickness control have also been reported and summarized in various review studies (Di et al., 2017; Li et al., 2018; Xiong et al., 2020). However, few studies focus on the intuitive comparison of photocatalytic activity of BiOX prepared by different methods for wastewater treatment.
Herein, we summarize recent advances in BiOX photocatalysts for wastewater remediation. First, we briefly probe into the preparation schemes for BiOX, which is regarded as essential from the application perspective. Then, various strategies to improve BiOX photocatalytic activity are discussed, such as facet management, element doping, heterojunction, defect engineering, and Schottky barriers. After that, the behaviors, decomposition routes of representative organics, and probable mechanisms during degradation by bismuth catalysts are assessed. Last, the problems of BiOX-based photocatalytic processes in water environmental use are listed.
The hydro/solvothermal method is the most widely used for producing diverse micro/nanostructured BiOX. Specifically, a chemical reaction proceeds in a sealed pressure vessel at high temperature and pressure in water or an organic solvent. The products often possess high crystallinity without calcination. Huo et al. prepared BiOBr nanosheets by hydrothermally treating Bi(NO3)3∙5H2O and KBr at 160°C for 12 h (Li K. L. et al., 2014). A similar procedure was extended to the preparation of BiOCl (Xiong et al., 2011b) and BiOI (Li H. et al., 2013). Different from the hydrothermal route which uses a precursor water solution, the solvothermal route adopts various solvents (e.g., methanol (Vadivel et al., 2014), ethylene glycol (Liu et al., 2014), and glycerol (Liu Z. et al., 2012)) to synthesize the precursor solution. This route allows the increment of pressure and temperature relative to the hydrothermal route (Liu Z. et al., 2012). Zhang et al. first produced 3D BiOX microspheres by using the solvothermal route with ethylene glycol (Figure 3) (Zhang et al., 2008) Furthermore, difference in solvents during this route impacts the growing direction of BiOX crystals. Xiong et al. studied the effect of alcohol chain length on the thermal preparation of BiOBr by using methanol, n-butanol, and n-hexanol as solvents at 180°C for 5 h in a stainless steel vessel (Xiong et al., 2016). When the carbon chain length of n-alcohol was prolonged, the characteristic diffraction peaks of BiOBr at the (102) and (110) facets were sharper and stronger, indicating the improvement of BiOBr crystallization.
FIGURE 3. SEM images of BiOCl (A), BiOBr (B), and BiOI microspheres (C) (reproduced with permission (Zhang et al., 2008)).
The adoption of precursors during preparation directly affects the BiOX facets. As reported, BiOBr with exposed (001) facet is photocatalytically more active, because the (001) facet has much lower surface energy than other facets and BiOBr materials have an intraelectric field along the [001] direction (Zhao et al., 2012). The use of ionic liquid Br instead of KBr led to the generation of BiOBr with exposed (001) facet, because the ionic liquid is passivating and can lower the facet surface energy (Mao et al., 2014).
The addition of surfactants such as polyvinylpyrrolidone (PVP) and hexadecyl-trimethyl-ammonium bromide can reportedly regulate morphology. Liu et al. made BiOBr flower-nanospheres with 32.9 m2/g surface area via the solvothermal procedure using Bi(NO3)3⋅5H2O, PVP (M = 45,000∼58,000), bromide [C16min]Br, and ethylene glycol as precursors while keeping the reaction at 140°C for 24 h (Liu and Wu, 2017). Hao et al. compared the effect of PVP on BiOI photocatalytic activity (Hao et al., 2012). With PVP, the BiOI nanosheets self-aggregated to form 3D hierarchical microspheres, which exhibited a higher photocatalytic activity in tetracycline hydrochloride (TCHC) degradation than 2D-BiOI nanoplates (removal rate 94 vs. 44%).
Both hydrolysis and alcoholysis methods offer mild conditions for synthesis of BiOX, but the products have nonuniform dimensions. For instance, BiOCl nanostructures were synthesized from 6 h of BiCl3 hydrolysis at 65°C using acetylacetone as an assisting solvent under acidic conditions (Armelao et al., 2012). Song et al. prepared 21- to 85-nm-thick BiOCl nanosheets from hydrolysis of Bi(NO3)3 with HCl and Na2CO3 by keeping it at ∼ pH2 for 30 min at ambient temperature (Song et al., 2017). Zhang et al. compared the photocatalytic activity of BiOBr prepared by hydrolysis and alcoholysis and added 0.5 g of BiBr3 to 15 ml of solvent of water or isopropyl alcohol at room temperature (Li R. et al., 2015). Then the suspension was heated at 20, 40, or 60°C for 10 min, and the pH was kept at 9. The BiOBr from hydrolysis was nanosheet-shaped and composed of the best crystallinity and unique (102) facets, while the BiOBr from alcoholysis was flower-like with exposed (110) facets. Both showed a superior photocatalytic activity toward methyl orange (MO) degradation, but the BiOBr from hydrolysis had higher photostability after 4 cycles. Huang et al. prepared BiOBr with different shapes (0D spherical quantum dots, 1D nanorods, 2D nanosheets, and 3D hierarchical architectures) through room-temperature hydrolysis (Figure 4) (Han et al., 2020) Different bismuth or bromine sources, solvents, capping agents, or surfactants affected the morphology of BiOBr during hydrolysis. The direct hydrolysis of BiI3 was used to prepare BiOI by Su et al., who used the as-obtained BiOI to degrade Rhodamine B under simulated solar light (Su et al., 2014).
FIGURE 4. Schematic map of the probable formation of 0D, 1D, 2D, or 3D BiOBr (Modified from Ref (Han et al., 2020)).
This method alters the morphology of BiOX mainly by modulating the crystal nucleation and growth during the synthesis. Nanomaterial synthesis by the template method basically takes three steps (Xie et al., 2016). 1) A template is produced. 2) The target product is synthesized under the action of the template via precipitation, sol-gel, hydrothermal, or other common synthetic method. 3) The template is removed. Cui et al. adopted carbonaceous microspheres as a template to adsorb Bi3+ and Cl−, followed by sintering up to 400°C to form hollow BiOCl microspheres, which were in a shell thickness of 40 nm and a diameter of 200 nm (Cui et al., 2016). With a butterfly wing as a biological template, Yan et al. prepared hierarchical BiOCl (Yan et al., 2019). BiOI was also used as a self-sacrifice template to construct Bi4O5I2/Bi5O7I and BiOI/Bi4O5I2 heterojunctions from simple calcination (Cheng et al., 2020). The BiOBr self-sacrifice template was also used for the formation of BiOBr/Bi4O5Br2 (Li P. et al., 2020).
Microwave, co-precipitation, and calcination can also be used to synthesize BiOX. For example, Chen et al. prepared BiOBr with a 3D flower-like structure after 27 min of microwave processing (Li et al., 2012). The BiOBr showed a Brunauer, Emmett and Teller (BET) surface area of 63.5 m2/g and an outstanding removal ability and fast adsorbing rate over Cr6+ in a wide pH range. Reportedly, the presence of surfactants during microwave processing improved the BiOBr photocatalytic activity (Chen et al., 2017). The BiOCl was also prepared by microwave with the same procedure as BiOBr and showed an excellent visible-light photocatalytic activity for dye Rhodamine B (RhB) (He et al., 2015). Ai et al. prepared the hierarchical porous BiOI using the microwave method. The as-obtained BiOI shows an excellent adsorption ability toward Congo red in water and it can be easily regenerated under light irradiation (Ai et al., 2014).
During preparation of BiOBr by co-precipitation (Lu et al., 2012), Bi(NO3)3 and KBr were mixed in acetic acid water solution, stirred, and aged for 6 h. The BiOCl microflowers were successfully prepared by using a simple co-precipitation method, and it had a better photocatalytic activity under acidic conditions than neutral and alkaline conditions (Wang and Zhang, 2020). The BiOI was also prepared by co-precipitation and EDTA was introduced as the retarder of the reaction and a structure-directing agent in aqueous media. The as-obtained BiOI exhibited a large BET surface area (∼47.5 m2 g−1) and thus had an excellent photocatalytic activity for NO removal (98%) in the gaseous phase (Montoya-Zamora et al., 2017).
The unstable halogen atoms in BiOX can easily escape due to the weak van der Waals forces. Therefore, phase transformation can be achieved by directly heating BiOX. Plate-like Bi24O31Br10 was prepared via sintering of BiOBr at 750°C (Yu C. et al., 2012). A similar process was extended to the synthesis of hollow Bi24O31Cl10 microspheres at 600°C (Cui et al., 2016). The 3D hierarchical bismuth oxyiodides was prepared using the ethylene glycol–assisted in situ hydrolysis method and the subsequent calcination at high temperature. The multiform bismuth oxyiodides obtained at different temperatures exhibit very distinct microstructure and band structure, and their photoabsorption was tuned from 700 to 400 nm in an orderly manner, rendering the adjustable oxidation and reduction ability of band energy levels (Huang et al., 2017).
Like any semiconductor-mediated photocatalysis, the maximum efficiency is decided by charge separation/transportation and surface catalytic performance. Inappropriate use of photoelectrons with low thermodynamic energy due to the presence of a positive CB will deteriorate the charge isolation of BiOBr and BiOI. Although BiOCl has a more negative CB, the large bandgap (∼3.22 eV) limits its application under visible light. Therefore, to enhance the visible light and photocatalytic capabilities of BiOX, diverse approaches like heterojunction, metal/nonmetal doping, noble metal deposition, interfacing with carbon materials, defect engineering, etc. can be adopted to strengthen the organic photodegradation efficiency.
Heterojunction basically refers to the band alignment caused by the interface between two types of semiconductors with unequal band structures. Heterojunction is divided by the band positions of the semiconductors into three types (Yang, 2021). Type II, the most widely used one, offers the best band positions for effective charge carrier isolation, which results in higher photocatalytic activity. The photoelectrons will be transported from the CB of semiconductor A to the CB of semiconductor B for favorable energetics of the relative positions of the CBs (Figure 5A). Holes are transferred at the same time from the VB of semiconductor B to the VB of semiconductor A. Under light illumination, the electrons and holes are spatially isolated from each other, lowering the recombination possibility (Ning et al., 2016). Yang et al. prepared type II Bi2WO6/BiOBr heterojunction via a one-step solvothermal route for degradation of rhodamine B (RhB), methylene blue, and colorless TCHC (Figure 6A) (Ren et al., 2019) Guo et al. prepared BiVO4/BiOCl heterojunction with a higher charge separation rate than pure BiVO4 or BiOCl (Liu et al., 2019). More research on BiOX-based Type II heterojunction can be found in Table 1.
FIGURE 5. (A) Type II photocatalysts; (B) p-n junction; and (C) Z-scheme strategy for higher photocatalytic activity.
FIGURE 6. (A) Photocatalytic mechanism of pollution by Bi2WO6/BiOBr catalyst (Type II heterojunction) (Ren et al., 2019). (B) Diagram of BiOBr/SnO2 p-n heterojunction charge transfer at the interface, electron location function, and charge difference distribution (Wu et al., 2020); (C) Charge transfer in Z-scheme CdS/BiOCl catalyst (Tang et al., 2020).
Though type-II heterojunction can perfectly isolate electrons-holes in space, the improvement in such isolation across this heterojunction is unable to prevent the ultrafast hole-electron recombination on the semiconductor. Therefore, p-n junction is proposed for greatly effective charge acquisition and isolation. Generally, p- and n-type semiconductors upon contact will constitute a p-n junction with an interfacial space-charge zone due to the electron and hole diffusion and thus generate a built-in electrical potential that can guide the electron and hole migration in the opposite direction (Figure 5B) (Jiang L. et al., 2012; Li L. et al., 2014; Jiang and Li, 2015) When incident light with an energy at least equal to the bandgap values irradiates the p-n junction, both p- and n-type semiconductors can be excited to form electron-hole pairs (Lin et al., 2011; Cao J. et al., 2012; Peng et al., 2014). The electrons are stimulated by the electric field to move to the CB of the n-type semiconductor, and the holes to the VB of the p-type semiconductor. Consequently, the electron-hole pairs are separated in p-n heterojunctions more quickly than in type-II heterojunctions due to the cooperation between the inner electric field and band arrangement (Yu et al., 2010). Wang et al. prepared p-type BiOBr and interfaced with n-type Bi2O2CO3 by precipitation conversion. The photoactivity of BiOBr/Bi2O2CO3 p-n junction is 14.8 folds that of bare Bi2O2CO3 (Wang J. J. et al., 2020). Sun et al. prepared BiOBr/SnO2 p-n heterojunctions to eliminate NO in visible light. Importantly, the charge transfer channels and directions at the BiOBr/SnO2 interface were determined by theoretical calculations (Figure 6B) and experiments (using XPS) (Wu et al., 2020). Similar research was extended to BiOCl and BiOI (Table 1).
Although the above heterojunctions are advantageous in separating photoelectron-hole pairs, the redox ability of these systems is weakened and thus cannot provide sufficient driving force for a specific photocatalytic reaction. Direct Z-scheme heterojunction photocatalysts have been proposed for spatial division of photoelectrons and holes whilst preserving the strong redox ability of two semiconductors (Figure 5C). Under irradiation, the CB photoelectrons of semiconductor A with strong reducing ability and the VB holes of semiconductor B with strong oxidizing ability are preserved. The CB photoelectrons of semiconductor B with low reducing ability and the VB holes of semiconductor A with low oxidizing ability recombine. The 4% CdS/BiOCl heterojunction prepared by Lin et al (Lin et al., 2017) showed a 10% higher photoactivity than pristine BiOCl in elimination of RhB, which was due to the direct Z-scheme mechanism (Figure 6C). In a new Z-scheme BiOBr/MnFe2O4 nanocomposite, spontaneous transfer of photoelectron carriers contributed to the photoelectron-hole division and decelerated the electron-hole recombination (Sin et al., 2020). Other Z-scheme photocatalysts were also reported before (Table 1).
Incorporation of transition metal ions into BiOX lattices to generate or modify the electronic states has gained much attention. Furthermore, it may induce lattice defects in BiOX or change crystallinity to prevent electron-hole combination, forming long-lasting carriers. Doping with special metal ions can also enhance light absorption region, which remarkably influences the photoelectrochemical (PEC) performance of semiconductors. Doping with transition metal ions (e.g., Fe3+, Cu2+, and Mn3+) will form impurity energy levels and may induce a redshift in the energy band, which is attributed to the electron transition from impurity bands to the CB or VB. Cu2+ was doped into BiOBr by using the solvothermal method to form the doping level (Figure 7), which strengthened both light absorption and electron-hole isolation efficiency (Lv et al., 2020). Consequently, the Cu2+-doped BiOBr showed a 2.28 times higher visible-light–driven photocatalytic activity relative to pristine BiOBr. A similar method was used to prepare Fe2+-doped BiOBr (Li W. et al., 2017). The doping of tungsten resulted in a bandgap reduction and the generation of an impurity band in the forbidden zone of BiOCl, as confirmed by first-principle theoretical computation and experimental findings. (Yang W. et al., 2014). Other commonly used transitional metal dopants reported in BiOX include Zn2+ (Li J. et al., 2015; Zhou et al., 2019b), Ti4+ (Jiang G. et al., 2012), Al3+ (Weng et al., 2014a), Sn4+ (Tu et al., 2015), and Mn3+ (Pare et al., 2011).
FIGURE 7. Mechanistics of photocharge transport and surface transfer on BiOBr (A,D) and Cu-BiOBr (B,E) with norfloxacin and dissolved oxygen under full (A,B) arc and visible light (D,E) irradiation. The corresponding degradation kinetics under full arc (C) and visible light (F) (Lv et al., 2020). (G) Photocatalytic bacterial inactivation enhancement of B-BiOBr nanosheets (Wu et al., 2016). (H–J) Organic degradation over La3+-doped BiOBr and corresponded degradation kinetic for RhB and CIP (Yin et al., 2017).
The incorporation of non-metal dopants can distribute extra extrinsic electronic levels in the band gap, which greatly affects light adsorption and charge carrier isolation. The main hypothesis is that the VB shifts upward to its maximum level after valence orbitals and local states hybridize from valence orbitals (Chen et al., 2015). Many non-metals such as N, Fen-Qiang Ma et al. (2017), Zhancheng Zhang et al. (2019), Obeid et al. (2020), Liu et al. (2021), and López-Velázquez et al. (2021) were doped into BiOX to improve the photocatalytic activity. Wang et al. prepared the boron-doped BiOBr nanosheets, in which the B dopants form an impurity level to accelerate charge separation (Figure 7G) (Wu et al., 2016) Carbon doping can enhance the internal electric field of Bi3O4Cl and thereby increase bulk-charge separation to 80% (Li et al., 2015). This homogeneous carbon-doped Bi3O4Cl was prepared by using a glucose-hydrothermal method, followed by thermal treatment to allow the diffusion of the carbon dopant in host lattices. A similar method was employed by Jin et al. to prepare C-doped Bi24O31Cl10 from oxytetracycline hydrochloride as a carbon and chlorine source (Jin et al., 2018).
Rare earth metals with a 4f electron shell are rich in electronic energy levels, which function as electron trapping centers for photocharge carriers. Hence, doping with rare earth metals may promote charge separation and overall photocatalytic performance. In the La3+-doped BiOBr microspheres produced by using an ionic liquid ([C16mim] Br)-assisted solvothermal route, (Yin et al., 2017), the substitution of Bi3+ by La3+ was monitored by XRD peak shift, while no La2O3 or another phase of La was found. The La-doped BiOBr significantly increased the photodegradation of ciprofloxacin, which was because La may trap photoelectrons and thus inhibit the electron-hole recombination (Figures 7H–J). Similarly, Y-doped BiOCl improved the visible-light photocatalytic activity in tetracycline decomposition (Zhong et al., 2020). Moreover, europium and erbium were also used as dopants in BiOX recently (Xia et al., 2016).
Deposition of noble metals (e.g., Ag, Pt, Rh, or Pd) on the surface of BiOX to build a space-charge dividing region (namely, the Schottky barrier) is another effective method to promote photo-charge separation. At the interface between a noble metal and BiOX, the electron transfer from the higher-Fermi-level BiOX to the lower-Fermi-level metal will arrange the Fermi energy levels. The presence of the Schottky barrier endows the metal and the semiconductor with excessive negative and positive charges, respectively. The Schottky barrier also acts as an effective electron trap against electron-hole reconnection in photocatalysis, which often leads to a higher photocatalytic capability. More recently, the deposition of Ag nanoparticles (NPs) on BiOX was found to remarkably improve dye decomposition efficiency under UV or visible light illumination (Yu et al., 2011; Yu C. L. et al., 2012). Li et al. prepared Pd/BiOBr by photodeposition (Figure 8A) and found the existence of Pd initiated the formation of more oxygen vacancies on the surface of BiOBr due to the electron interaction at the Pd–BiOBr interface. The coexistence of Pd and oxygen vacancies on BiOBr facilitates the adsorption of O2 and toluene molecules (Figure 8B). As a result, the Pd/BiOBr interface controls the charge division and activates O2 and toluene, improving the photocatalytic activity of BiOBr by 1.5 times in 99% selective oxidation of toluene to benzaldehyde (Figure 8C) (Li X. et al., 2020) Yu et al. investigated how noble metals (Rh, Pd, and Pt) deposition affected the photocatalytic behaviors of BiOX. The impact of the noble metals ranks as Pt > Pd > Rh under UV and as Rh > Pt > Pd under visible light. The noble metal NPs also function as electron traps to quicken electron-hole division and thus improve the visible light activity (Yu et al., 2013).
FIGURE 8. (A) Schematic scheme for preparation of Pd/BiOBr; (B) photocatalytic selective toluene oxidation to benzaldehyde over Pd/BiOBr and (C) comparison of toluene conversion and selectivity among photocatalysts (reproduced with permission (Li X. et al., 2020)).
Due to the excellent electrical conductivity, flexibility, and specific surface, carbon materials are ideal supporting scaffolds for homogeneous anchoring of functional nanomaterials. Xu et al. coated hierarchical BiOX (X = Cl or I) on the newly prepared functional carbon nanotubes through ionic layer adsorption and reaction. The nanotubes with outstanding mechanical properties and pollutant absorbing ability were used to tailor the energy gaps of BiOX via covalent bonds (Figure 9A) (Weng et al., 2014b). Similarly, the BiOI/multi-walled carbon nanotube composites prepared by Xiong et al. using the solvothermal method photocatalytically outperformed pure BiOI owing to the interfacial charge-transfer (Figure 9B) (Su et al., 2012). Graphene is another common carbon material to couple with BiOX. Li et al. prepared a BiOBr/graphene composite by an in situ solvothermal route using graphene oxide (Figure 9C). The graphene oxide was reduced to graphene, and BiOBr NPs were formed on the surface. This composite has higher photocatalytic activity in degrading RhB (Tu et al., 2012). Reduced graphene oxide (RGO)/BiOCl hybrids were synthesized by Zhang et al. with graphene oxide as the precursor (Li Z. et al., 2017). The RGO/BiOCl was 8.4 and 3.8 times more photocatalytically active than BiOCl in MO degradation and water oxidation, respectively. This was because the chemical bonding of BiOCl with RGO led to faster charge separation and longer carrier lifetime. It is indicated that chloride vacancies may act as recombination centers to impede photocharge separation (Figure 9D). Carbon quantum dots (CQDs) with quasi-spherical nanoparticles usually <10 nm in diameter have been introduced to BiOX recently. Li et al. prepared the CQD-modified BiOCl which showed the enhanced photocatalytic activity due to superior electron transfer ability of CQDs (Di et al., 2015). Furthermore, the nitrogen-doped CQD–modified BiOBr was prepared by the same group to boost the photodegradation toward ciprofloxacin, rhodamine B, tetracycline hydrochloride, and bisphenol A (Di et al., 2016).
FIGURE 9. (A) Mechanism of photocatalytic reaction over BiOX/CNT (Weng et al., 2014b). (B) Electron transfer between BiOI and MWCNT (Su et al., 2012). (C) Photodegradation over BiOBr/graphene (Tu et al., 2012). (D) Charge transfer of RGO/BiOCl photoanodes before and after phosphate modification (Li Z. et al., 2017) (all reproduced with permission).
The creation of defects permits the adjustment of micro/electron structure, atom coordination number, and charge transfer in BiOX. Zhang et al. prepared the [001] facet exposed BiOBr with oxygen vacancy (OV) by using an ethylene glycol–assisted solvothermal method and used it to fix atmospheric N2 to NH3 (Figures 10A,B) (Li et al., 2015d). The sample without OV was also synthesized by further sintering in an O2 atmosphere, and the color turned white (Figure 10C). NH3 was detected over BiOBr with OV, but not in BiOBr without OV (Figure 10D). This was because the OV in BiOBr was the catalytic activation center and can chemisorb N2 on the surface. Similarly, Xue et al. used BiOBr nanosheets with OV to realize N2 to NH3 (Xue et al., 2018). The Bi3O4Br nanosheets with single-unit-cell thickness and confined defects have been controllably prepared by Di et al., who demonstrated that the bismuth vacancy can greatly facilitate the generation of oxygen defects, accounting for the formed oxygen defects in the bismuth oxyhalides (Di et al., 2019). Wang et al. prepared OV-rich S-doped BiOBr by one-step solvothermal treatment. The optimal BB-5S degraded 98% 4-chlorophenol (4-CP) under visible light within 120 min, which was 18.0 and 4.9 times those of OV-poor sulfur-doped BiOBr and pristine ultrathin BiOBr, respectively. Experiments and DFT computation showed a sub-band was formed under the synergy of OVs and S doping, which largely facilitated visible-light absorption capability and inhibited photocharge recombination, thus improving photocatalytic activity (Wang Q. et al., 2019). Cui et al. solvothermally prepared OV-rich BiOCl nanosheets (Cui et al., 2018). A new energy level from OV was verified both theoretically and experimentally. BiOCl nanosheets with OV were capable of photocatalytic oxygen evolution under visible light. Additionally, OV-rich BiOCl displayed a higher visible-light photocurrent and more efficient photocharge division and migration than BiOCl with fewer OVs.
FIGURE 10. (A) Schematic map of photocatalytic N2 fixation over BiOBr with OV. (B) Photocatalytic N2 fixation model with water as both the solvent and proton source. (C) Colors of BiOBr with or without OV. (D) Quantitative monitoring of NH3 formed over different photocatalysts (Li et al., 2015d). (E) Illustration of the formation process of Bi24O31Br10 with Bi-O vacancy pairs. Electrostatic potential of (F) Bi24O31Br10 with Bi-O vacancy pairs and (G) perfect Bi24O31Br10. Electron localization function of (H) Bi24O31Br10 with Bi-O vacancy pairs and (I) perfect Bi24O31Br10 (Di et al., 2021).
Apart from the single vacancies, the effect of surface vacancy pairs in BiOX on photocatalytic activity has been explored recently. When the thickness of BiOX decreased to atomically thin, the atomic-escape energy will be smaller and then surface atoms will escape from the 2D lattice and create vacancies more easily (Di et al., 2018a). Liu and co-workers prepared the Bi24O31Br10 atomic layer with “Bi-O” vacancy pairs by a mild polyvinylpyrrolidone (PVP) self-assembly strategy coupled with pH adjusting (see Figure 10E). In contrast to the perfect surface, Bi24O31Br10 with Bi-O vacancy pairs own large local electric field intensity (Figures 10F,G). Furthermore, the presence of Bi-O vacancy pairs would create an electron delocalization hot spot (Figures 10H,I), where both inflow and outflow of electrons are better favored. Therefore, the separated charges can be effciently delivered to target reactants, significantly enhancing the catalytic reaction rate (Di et al., 2021).
Semiconductor photocatalysts with specific exposed crystal surfaces can improve photo-charge separation and transportation, which further strengthens the photocatalytic performances. In general, surfactants and H+ are used to modulate the exposed crystal surface. Glucose can be the capping and structure-directing agent in preparing one-dimensional rod-like BiOBr with exposed (110) facets (BiOBr-110) (Figure 11A) (Peng et al., 2017). Glucose can interact with the (001) facet of BiOBr NPs to prevent the development of these planes, leading to the generation of ultrathin BiOBr nanosheets with exposed (001) facets. In the meantime, glucose drives the ultrathin nanosheets to gather along the [001] orientation, forming BiOBr nanorods with exposed (110) facets. BiOBr-110 photocatalytically outperforms BiOBr-001 owing to the lower resistance and higher efficiency of charge transfer. Zhao et al. prepared the BiOCl with high ratios of [001] and (Li et al., 2012) facets (BiOCl-H1 and BiOCl-H2, respectively) under various acid conditions (Figure 11D) (Zhao et al., 2020), which remarkably raised the RhB photodegradation efficiency. The apparent rate constant of BiOCl-H2 was 0.0961 min−1, about 2.7 times that of BiOCl-H1 (Figures 11E,F). The Z-scheme facet heterojunction was constructed to accelerate charge separation and transportation (Figure 11D). Zhang et al. found that single-crystal BiOCl nanosheets with exposed [001] facets were more active in direct semiconductor photoexcited MO removal under UV light, but the counterpart with exposed [010] facets was highly active in indirect dye photosensitive decomposition under visible light (Jiang J. et al., 2012).
FIGURE 11. (A) Schematic map of the proposed formation mechanism of BiOBr-110. (B) RhB and (C) MO degradation over BiOBr-110 and BiOBr-001 (Peng et al., 2017). (D) Facet junction interface charge transportation. (E) Photodegradation of RhB and (F) dynamic fitting by using a first-order model (Zhao et al., 2020) (all reproduced with permission).
Thickness tuning is another strategy for improving the photocatalytic activity of BiOX. When the thickness of BiOX is reduced, the quantum confinement can be realized to tune the band edge structure, which is favorable for meeting the potential requirement of various catalytic reactions. In addition, the charge migration distance can be shortened from the inside to the surface which improves the separation efficiency of charges. Low-coordinated atoms with dangling bonds can provide active sites to participate in the catalytic reactions (Di et al., 2018b; Xiong et al., 2018). The thickness-dependent photocatalytic activity toward salicylic acid degradation was reported by Li et al., who prepared the thinner and thicker Bi3O4Cl through the liquid phase exfoliation under isopropanol or further recrystallization (Li J. et al., 2013). The thinnest Bi3O4Cl endows the highest photodegradation activity due to the highest internal electric field. The thinner thickness of Bi24O31Br10 was prepared when a larger amount of NH4Br was used. The higher charge separation efficiency can be realized in thinner Bi24O31Br10 nanosheets, thus leading to the formation of higher reactive oxygen species concentration, favoring the degradation of tetracycline hydrochloride (Wang C. Y. et al., 2017).
The morphology control is a classic but effective strategy for boosting the photocatalytic activity of BIOX (Garg et al., 2018b; Bárdos et al., 2019; Bárdos et al., 2020). It is well known that porous and hollow solids have excellent adsorptive properties, which is a prerequisite for highly efficiently utilizing photo-induced electron-hole pairs during photocatalysis. BiOI hollow microspheres were synthesized using a facile reactable ionic liquid 1-butyl-3-methylimidazolium iodine ([Bmim]I)-assisted microemulsion method at room temperature (Di et al., 2014). The as-obtained BiOI hollow microspheres shows enhanced photocatalytic activity, which was attributed to the shortened diffusion pathways, lower energy band gap, higher BET surface area, faster interfacial charge transfer rate, and higher separation efficiency of photogenerated electrons and holes. Liu and co-workers prepared the Bi12O17Br2 nanotubes, exhibiting 14.4 times more activity than Bi12O17Br2 nanoplates (Di et al., 2020). The enhanced activity is attributed to better CO2 adsorption and activation, increased carrier separation, and CO desorption, which was induced by the surface tensile strain in Bi12O17Br2 nanotubes.
By adjusting the elemental constituents, the energy band structures of BiOX materials can be tuned to meet the requirement of various applications. For example, the bismuth-rich BiOCl and BiOBr can narrow the band gap compared to pristine BiOCl and BiOBr while bismuth-rich BiOI shows an increased band gap (Li J. et al., 2017; Wang Z. et al., 2019). In addition, the bismuth-rich BiOX materials own more abundant tunable surface atomic configurations including surface atomic types, atomic quantity, and atomic distance. Bi24O31Br10 materials display outstanding photocatalytic hydrogen evolution behavior, while the pristine BiOBr has been demonstrated to be inactive for photocatalytic hydrogen evolution (Shang et al., 2014).
The very positive VB and the modification of BiOX endow photoholes with sufficient thermodynamic energy to be used in organic oxidation. The organics can be degraded directly through hole-oxidation or indirectly by the newly formed oxidative radicals such as hydroxyl radicals (OH−). During this process, the photoelectrons will be removed by dissolved oxygen to maximize the net charge separation efficiency and can also be used to reduce heavy metal ions.
Photooxidative degradation of many organic pollutants over modified BiOX has been extensively reviewed (Yang et al., 2018; Sharma K. et al., 2019; Garg et al., 2019). Both the decomposition mechanism and the number of intermediates depend on the properties of organic compounds. The abundant organic dyes in textile, apparel, and paper industrial wastewater cause severe environmental pollution. The dye-polluted wastewater carries refractory pigments that are highly harmful and carcinogenic to humans (Brown and De Vito, 1993; Chen et al., 2010). Dyes even at extremely low levels (below 1 ppm) are highly visible in water and severely worsen water environments (Ledakowicz and Gonera, 1999; Grzechulska and Morawski, 2002; Daneshvar et al., 2004). In general, organic dyes are either cationic or anionic according to their chemical structures. Cationic dyes (e.g., RhB, methylene blue, and malachite green) carry cationic functional groups (mostly N+) that can dissociate into cations in aqueous solutions. In contrast, anionic dyes including acid orange 7 and MO contain sulfonic or carboxylic acid groups. Both anionic and cationic functional groups can interact with BiOX with a hydrophilic surface, which is favorable for the oxidation by hole or hydroxyl radicals. For instance, RhB was mainly degraded by hole and hydroxyl radicals in BiOBr/BiOF (Jiang et al., 2017). However, the RhB degradation by BiOBr/TiO2 heterojunction was mediated by superoxide radical anions (O2−), which was produced by single electron reduction of dissolved oxygen (Yang et al., 2021). Organic dye degradation was also investigated using different BiOX-based photocatalysts, namely, BiOCl/CeO2 (Zhang Y. et al., 2020b), W-doped BiOCl (Mokhtari and Tahmasebi, 2021), Bi2WO6/BiOX (X = Cl, Br, I) (Derikvand and Tahmasebi, 2021), and BiVO4/BiOX (X = F, Cl, Br, I) (Razavi-Khosroshahi et al., 2019).
The release of antibacterial agents into the aquatic environment has caused more concerns about their potential environmental risks, especially the occurrence and spread of antibiotic resistance and chronic toxicity to microbial species. Fluoroquinolones (e.g., norfloxacin, ciprofloxacin, moxifloxacin, and ofloxacin) are extensively used as human and veterinary medicine owing to their wide-spectrum activity against Gram- and Gram-negative bacteria (Brain et al., 2004; Liu C. et al., 2012). However, fluoroquinolones cannot be fully metabolised in the body and are largely excreted in their pharmacologically active forms, which make them the most-detected antibiotics in wastewater and surface water (Robinson et al., 2005). In general, the degradation of fluoroquinolones mainly involves amine side-chain degradative oxidation, defluorination, and decarboxylation (Chen and Chu, 2015). Of them, the degradation of the electron-rich amine side-chain to oxidized products is extremely important (Sturini et al., 2012). Different BiOX-based photocatalysts were also designed to reduce antibiotics. For example, Cu2+-doped BiOBr (Lv et al., 2020), Nb5+ -doped BiOBr (Wei et al., 2020), and N-doped BiOCl (Maimaitizi et al., 2020) were used in the degradation of norfloxacin. Type II heterojunctions such as BiVO4/BiOCl (Ma X. et al., 2017), BiOBr/Fe2O3 (Guo et al., 2017), and BiOBr/CdS (Senasu et al., 2021) can reportedly enhance the photodegradation of antibiotics.
Apart from the oxidation degradation of organic dyes and antibiotics, modified BiOX can also be applied to other common organics, including pharmaceutical active compounds (Heidari et al., 2020; Gao et al., 2021) and endocrine-disrupting chemicals (López-Velázquez et al., 2021; Wu et al., 2021; Zhang H. et al., 2021). However, only organics transformation occurs in most cases. Therefore, future works shall focus on the development of modified BiOX with strong oxidation ability to degrade organics and mineralize their byproducts.
Heavy metal water pollution is a global environmental problem that threatens human health and the ecology. Hexavalent chromium (Cr(VI)) is an essential component in many industrial applications, including electroplating, leather tanning, and anti-corrosion steel, despite its carcinogenicity and mutagenicity (Shi et al., 1994; Salnikow and Zhitkovich, 2008). Compared to other oxidation states of Cr ions, Cr(III) is relatively benign with significantly lower toxicity since it can be excreted from the human body. Hence, photocatalytic reduction of Cr(IV) to Cr(III) is considered as an effective route. Long et al. prepared BiOBr-Bi2S3 heterojunctions via ion-exchange (Long et al., 2021). The performance of photo-reduced Cr(VI) was about 28.9 and 184.6 times higher than those of pure Bi2S3 and BiOBr, respectively. More importantly, the novel composite had a good Cr(III) adsorption efficiency, suggesting that it can be a bifunctional photocatalyst. Similar Cr (VI) removal was also finished with CoFe2O4/BiOBr/graphene, (Li et al., 2019), BiOCl/Bi2S3 (Lu et al., 2020), and BiOClxBr1-x (Hussain et al., 2020). However, there is no example of using BiOX as photocatalysts into the reduction of other heavy metal ions.
Although numerous modified BiOX had been developed and used on photocatalytic degradation, they still faced limitation during the practical application. One of the major challenges that the photocatalysts confront is the inefficient separation and recovery from the aqueous medium after being utilized in the wastewater treatment process. An et al. collected the Cu-doped BiOBr from suspension by centrifuge and then regenerated it by heating at 200°C to remove the residual organic (Lv et al., 2020). Although this method is effective for the recovery of photocatalysts, the process is time consuming and that inevitably raises the treatment cost. The immobilization of photocatalysts on a certain supporting substrate provides a dual advantage; the first is the efficient separation and easy recovery of reused photocatalysts, and the second is the prevention of agglomeration of the photocatalysts for higher photocatalytic performance (Tettey et al., 2010; Cao C. Y. et al., 2012). A wide range of substrates have been employed for the immobilization of BiOX, such as activated carbon, ceramic fibers, and ceramic paper (Yadav et al., 2019; Kása et al., 2020; Yadav et al., 2020). The novel green BiOX (Cl, Br and I) was homogeneously immobilized on Al2O3-based ceramic fiber sheets, which exhibited an excellent photocatalytic performance and recyclability for MO, bisphenol A, and ampicillin disintegration under visible-light irradiation (Yadav et al., 2019).
BiOX-based materials are prospective photocatalysts for environmental treatments, such as waste water remediation, organic pollutant degradation, and heavy metal removal. Therefore, the literature on BiOX (X = Cl, Br, I) was reviewed and discussed to provide some guidance for future studies.
Until now, heat treatments including solvothermal and hydrothermal methods are the most widely used to prepare BiOX. These routes allow researchers to control the morphology, specific surface area, dimension, and pore size and volume. However, this is a time- and energy-consuming process. Therefore, future studies shall focus on a facile method for large-scale synthesis of more attractive BiOBr photocatalysts. The microwave-assisted method allows for the rapid production of BiOX, but the research of this process is still in its infancy.
To boost the activity of BiOX-based semiconductor photocatalysts, researchers are using diverse solutions, such as heterojunction engineering, ion doping, interfacing with carbon materials, coupling with noble metals, and facet control. Although much effort has been dedicated to regulating BiOX-based materials and optimizing their photocatalytic performance, the research in this area is still faced with a lot of challenges, and it is also filled with opportunities. In view of 2D materials, the reduction of atomic layer thickness may bring new properties that the bulk materials do not possess. The layered structure of BiOX enables it to readily form a single layer or few-layer structure. Therefore, researchers may pay attention to control preparation of BiOX with atomic thickness and explore their photocatalytic performance. The defect engineering has been verified to regulate the photocatalytic activity of BiOX, but most of the defect type in BiOX is still oxygen vacancy. More defects have not been constructed in BiOX including halogen vacancies and dual vacancies, which will interact with surface adsorbates during photocatalysis. Furthermore, in the presence of these defects, new electronic states may be induced and affect the electron transfer. Therefore, the investigation of the relationship between defect types and photocatalytic activity is a promising work in the future.
Apart from the modification of BiOX, the exploration of catalytic mechanisms at the atomic level during multiple photocatalytic applications is much needed. The catalytic sites are crucially important and thus it should be determined through calculation or direct experimental observation. More in situ characterization should be performed to gain atomic-level insight into the relationship between active sites and photocatalytic activity, which will guide the design direction of BiOX.
Last, photocatalytic oxidation of organic molecules was reviewed, but most of the works focused on the organic dye degradation at the laboratory scale. However, for practical application of BiOX, the treatment of organics in real water/wastewater is still a challenge, which is mainly due to the existence of dissolved inorganic ions. The photoreduction of heavy metals over BiOX is another important field that shall be investigated in the future. Taking the practical application into consideration, the immobilization techniques for the recovery of material is also important for the development.
XL prepared and wrote the article, and FL and XH further edited the manuscript and checked the English writing.
The authors declare that the research was conducted in the absence of any commercial or financial relationships that could be construed as a potential conflict of interest.
All claims expressed in this article are solely those of the authors and do not necessarily represent those of their affiliated organizations, or those of the publisher, the editors, and the reviewers. Any product that may be evaluated in this article, or claim that may be made by its manufacturer, is not guaranteed or endorsed by the publisher.
Ai, L., Zeng, Y., and Jiang, J. (2014). Hierarchical Porous BiOI Architectures: Facile Microwave Nonaqueous Synthesis, Characterization and Application in the Removal of Congo Red from Aqueous Solution. Chem. Eng. J. 235, 331–339. doi:10.1016/j.cej.2013.09.046
Alansi, A. M., Qahtan, T. F., and Saleh, T. A. (2021). Solar‐Driven Fixation of Bismuth Oxyhalides on Reduced Graphene Oxide for Efficient Sunlight‐Responsive Immobilized Photocatalytic Systems. Adv. Mater. Inter. 8, 2001463. doi:10.1002/admi.202001463
Armelao, L., Bottaro, G., Maccato, C., and Tondello, E. (2012). Bismuth Oxychloride Nanoflakes: Interplay between Composition-Structure and Optical Properties. Dalton Trans. 41, 5480–5485. doi:10.1039/c2dt12098d
Bárdos, E., Király, A. K., Pap, Z., Baia, L., Garg, S., and Hernádi, K. (2019). The Effect of the Synthesis Temperature and Duration on the Morphology and Photocatalytic Activity of BiOX (X= Cl, Br, I) Materials. Appl. Surf. Sci. 479, 745–756. doi:10.1016/j.apsusc.2019.02.136
Bárdos, E., Márta, V., Baia, L., Todea, M., Kovács, G., Baán, K., et al. (2020). Hydrothermal Crystallization of Bismuth Oxybromide (BiOBr) in the Presence of Different Shape Controlling Agents. Appl. Surf. Sci. 518, 146184. doi:10.1016/j.apsusc.2020.146184
Bi, C., Cao, J., Lina, H., Wang, Y., and Chen, S. (2016). Enhanced Photocatalytic Activity of Bi12O17Cl2 through Loading Pt Quantum Dots as a Highly Efficient Electron Capturer. Appl. Catal. B: Environ. 195, 132–140. doi:10.1016/j.apcatb.2016.05.011
Bi, J., Wu, L., Li, J., Li, Z., Wang, X., and Fu, X. (2007). Simple Solvothermal Routes to Synthesize Nanocrystalline Bi2MoO6 Photocatalysts with Different Morphologies. Acta Materialia 55, 4699–4705. doi:10.1016/j.actamat.2007.04.034
Brain, R. A., Johnson, D. J., Richards, S. M., Sanderson, H., Sibley, P. K., and Solomon, K. R. (2004). Effects of 25 Pharmaceutical Compounds to Lemna Gibba Using a Seven-Day Static-Renewal Test. Environ. Toxicol. Chem. 23, 371–382. doi:10.1897/02-576
Brown, M. A., and De Vito, S. C. (1993). Predicting Azo Dye Toxicity. Crit. Rev. Environ. Sci. Techn. 23, 249–324. doi:10.1080/10643389309388453
Bueno, M. J. M., Gomez, M. J., Herrera, S., Hernando, M. D., Agüera, A., and Fernández-Alba, A. R. (2012). Occurrence and Persistence of Organic Emerging Contaminants and Priority Pollutants in Five Sewage Treatment Plants of Spain: Two Years Pilot Survey Monitoring. Environ. Pollut. 164, 267–273. doi:10.1016/j.envpol.2012.01.038
Cao, C.-Y., Qu, J., Wei, F., Liu, H., and Song, W.-G. (2012). Superb Adsorption Capacity and Mechanism of Flowerlike Magnesium Oxide Nanostructures for lead and Cadmium Ions. ACS Appl. Mater. Inter. 4, 4283–4287. doi:10.1021/am300972z
Cao, C., Xiao, L., Chen, C., and Cao, Q. (2015). Synthesis of Novel Cu2O/BiOCl Heterojunction Nanocomposites and Their Enhanced Photocatalytic Activity under Visible Light. Appl. Surf. Sci. 357, 1171–1179. doi:10.1016/j.apsusc.2015.09.121
Cao, F., Wang, Y., Wang, J., Lv, X., Liu, D., Ren, J., et al. (2018). Oxygen Vacancy Induced superior Visible-Light-Driven Photodegradation Pollutant Performance in BiOCl Microflowers. New J. Chem. 42, 3614–3618. doi:10.1039/c7nj04041e
Cao, J., Li, X., Lin, H., Chen, S., and Fu, X. (2012). In Situ preparation of Novel P-N junction Photocatalyst BiOI/(BiO)2CO3 with Enhanced Visible Light Photocatalytic Activity. J. Hazard. Mater. 239-240, 316–324. doi:10.1016/j.jhazmat.2012.08.078
Carlsen, L., Bruggemann, R., and Sailaukhanuly, Y. (2013). Application of Selected Partial Order Tools to Analyze Fate and Toxicity Indicators of Environmentally Hazardous Chemicals. Ecol. Indicators 29, 191–202. doi:10.1016/j.ecolind.2012.12.028
Chai, B., Yan, J., Fan, G., Song, G., and Wang, C. (2019). In-situ Construction of Bi2SiO5/BiOBr Heterojunction with Significantly Improved Photocatalytic Activity under Visible Light. J. Alloys Compd. 802, 301–309. doi:10.1016/j.jallcom.2019.06.195
Chang, A.-M., Chen, Y.-H., Lai, C.-C., and Pu, Y.-C. (2021). Synergistic Effects of Surface Passivation and Charge Separation to Improve Photo-Electrochemical Performance of BiOI Nanoflakes by Au Nanoparticle Decoration. ACS Appl. Mater. Inter. 13, 5721–5730. doi:10.1021/acsami.0c18430
Chang, L., Pu, Y., Shen, G., Cui, Y., Wei, X., Cao, B., et al. (2020). Excellent Adsorption-Photocatalysis Synergistic Activity of 3D-3D Flower-like BiOBr/graphene Hydrogel Composite and the Removal of Potassium Butyl Xanthate. New J. Chem. 44, 2479–2488. doi:10.1039/c9nj06060j
Chang, X., Huang, J., Cheng, C., Sui, Q., Sha, W., Ji, G., et al. (2010). BiOX (X = Cl, Br, I) Photocatalysts Prepared Using NaBiO3 as the Bi Source: Characterization and Catalytic Performance. Catal. Commun. 11, 460–464. doi:10.1016/j.catcom.2009.11.023
Chen, L., Huang, R., Xiong, M., Yuan, Q., He, J., Jia, J., et al. (2013). Room-Temperature Synthesis of Flower-like BiOX (X═Cl, Br, I) Hierarchical Structures and Their Visible-Light Photocatalytic Activity. Inorg. Chem. 52, 11118–11125. doi:10.1021/ic401349j
Chen, M., and Chu, W. (2015). Photocatalytic Degradation and Decomposition Mechanism of Fluoroquinolones Norfloxacin over Bismuth Tungstate: experiment and Mathematic Model. Appl. Catal. B: Environ. 168-169, 175–182. doi:10.1016/j.apcatb.2014.12.023
Chen, S., Zhang, J., Zhang, C., Yue, Q., Li, Y., and Li, C. (2010). Equilibrium and Kinetic Studies of Methyl orange and Methyl Violet Adsorption on Activated Carbon Derived from Phragmites Australis. Desalination 252, 149–156. doi:10.1016/j.desal.2009.10.010
Chen, X., Liu, L., and Huang, F. (2015). Black Titanium Dioxide (TiO2) Nanomaterials. Chem. Soc. Rev. 44, 1861–1885. doi:10.1039/c4cs00330f
Chen, Z. G., Zhu, L., Xia, J. X., Xu, L., Li, H. M., Zhang, J., et al. (2014). Synthesis of Flower-like Pd/BiOCl Composites via Reactable Ionic Liquid and Their Enhanced Photocatalytic Properties. Mater. Techn. 29, 245–251. doi:10.1179/1753555714y.0000000146
Chen, Z., Liao, J., Chen, Y., Zhang, J., Fan, W., and Huang, Y. (2016). Synthesis of Oxygen Deficient BiOI for Photocatalytic Degradation of Methyl orange. Inorg. Chem. Commun. 74, 39–41. doi:10.1016/j.inoche.2016.10.035
Chen, Z., Zeng, J., Di, J., Zhao, D., Ji, M., Xia, J., et al. (2017). Facile Microwave-Assisted Ionic Liquid Synthesis of Sphere-like BiOBr Hollow and Porous Nanostructures with Enhanced Photocatalytic Performance. Green. Energ. Environ. 2, 124–133. doi:10.1016/j.gee.2017.01.005
Cheng, H., Huang, B., and Dai, Y. (2014). Engineering BiOX (X = Cl, Br, I) Nanostructures for Highly Efficient Photocatalytic Applications. Nanoscale 6, 2009–2026. doi:10.1039/c3nr05529a
Cheng, H., Huang, Y., Wu, J., Ling, Y., Dong, L., Zha, J., et al. (2020). Controllable Design of Bismuth Oxyiodides by In-Situ Self-Template Phase Transformation and Heterostructure Construction for Photocatalytic Removal of Gas-phase Mercury. Mater. Res. Bull. 131, 110968. doi:10.1016/j.materresbull.2020.110968
Choi, Y. I., Jeon, K. H., Kim, H. S., Lee, J. H., Park, S. J., Roh, J. E., et al. (2016). TiO2/BiOX (X=Cl, Br, I) Hybrid Microspheres for Artificial Waste Water and Real Sample Treatment under Visible Light Irradiation. Separat. Purif. Techn. 160, 28–42. doi:10.1016/j.seppur.2016.01.009
Chou, S.-Y., Chung, W.-H., Chen, L.-W., Dai, Y.-M., Lin, W.-Y., Lin, J.-H., et al. (2016). A Series of BiOxIy/GO Photocatalysts: Synthesis, Characterization, Activity, and Mechanism. RSC Adv. 6, 82743–82758. doi:10.1039/c6ra12482h
Cui, D., Wang, L., Xu, K., Ren, L., Wang, L., Yu, Y., et al. (2018). Band-gap Engineering of BiOCl with Oxygen Vacancies for Efficient Photooxidation Properties under Visible-Light Irradiation. J. Mater. Chem. A. 6, 2193–2199. doi:10.1039/c7ta09897a
Cui, P., Wang, J., Wang, Z., Chen, J., Xing, X., Wang, L., et al. (2016). Bismuth Oxychloride Hollow Microspheres with High Visible Light Photocatalytic Activity. Nano Res. 9, 593–601. doi:10.1007/s12274-015-0939-z
Dai, W.-W., and Zhao, Z.-Y. (2017). Understanding the Interfacial Properties of Graphene-Based materials/BiOI Heterostructures by DFT Calculations. Appl. Surf. Sci. 406, 8–20. doi:10.1016/j.apsusc.2017.02.079
Daneshvar, N., Salari, D., and Khataee, A. R. (2004). Photocatalytic Degradation of Azo Dye Acid Red 14 in Water on ZnO as an Alternative Catalyst to TiO2. J. Photochem. Photobiol. A: Chem. 162, 317–322. doi:10.1016/s1010-6030(03)00378-2
Derikvand, L., and Tahmasebi, N. (2021). Synthesis and Photocatalytic Performance of Bi2WO6/BiOX (X=Cl, Br, I) Composites for RhB Degradation under Visible Light. Korean J. Chem. Eng. 38, 163–169. doi:10.1007/s11814-020-0687-y
Di, J., Chen, C., Zhu, C., Long, R., Chen, H., Cao, X., et al. (2021). Surface Local Polarization Induced by Bismuth‐Oxygen Vacancy Pairs Tuning Non‐Covalent Interaction for CO 2 Photoreduction. Adv. Energ. Mater. 11, 2102389. doi:10.1002/aenm.202102389
Di, J., Song, P., Zhu, C., Chen, C., Xiong, J., Duan, M., et al. (2020). Strain-Engineering of Bi12O17Br2 Nanotubes for Boosting Photocatalytic CO2 Reduction. ACS Mater. Lett. 2, 1025–1032. doi:10.1021/acsmaterialslett.0c00306
Di, J., Xia, J., Chisholm, M. F., Zhong, J., Chen, C., Cao, X., et al. (2019). Defect‐Tailoring Mediated Electron-Hole Separation in Single‐Unit‐Cell Bi 3 O 4 Br Nanosheets for Boosting Photocatalytic Hydrogen Evolution and Nitrogen Fixation. Adv. Mater. 31, 1807576. doi:10.1002/adma.201807576
Di, J., Xia, J., Ge, Y., Xu, L., Xu, H., He, M., et al. (2014). Reactable Ionic Liquid-Assisted Rapid Synthesis of BiOI Hollow Microspheres at Room Temperature with Enhanced Photocatalytic Activity. J. Mater. Chem. A. 2, 15864–15874. doi:10.1039/c4ta02400a
Di, J., Xia, J., Ji, M., Wang, B., Li, X., Zhang, Q., et al. (2016). Nitrogen-doped Carbon Quantum dots/BiOBr Ultrathin Nanosheets: In Situ strong Coupling and Improved Molecular Oxygen Activation Ability under Visible Light Irradiation. ACS Sustain. Chem. Eng. 4, 136–146. doi:10.1021/acssuschemeng.5b00862
Di, J., Xia, J., Ji, M., Wang, B., Yin, S., Zhang, Q., et al. (2015). Carbon Quantum Dots Modified BiOCl Ultrathin Nanosheets with Enhanced Molecular Oxygen Activation Ability for Broad Spectrum Photocatalytic Properties and Mechanism Insight. ACS Appl. Mater. Inter. 7, 20111–20123. doi:10.1021/acsami.5b05268
Di, J., Xia, J., Li, H., Guo, S., and Dai, S. (2017). Bismuth Oxyhalide Layered Materials for Energy and Environmental Applications. Nano Energy 41, 172–192. doi:10.1016/j.nanoen.2017.09.008
Di, J., Xiong, J., Li, H., and Liu, Z. (2018a). Ultrathin 2D Photocatalysts: Electronic-Structure Tailoring, Hybridization, and Applications. Adv. Mater. 30, 1704548. doi:10.1002/adma.201704548
Di, J., Yan, C., Handoko, A. D., Seh, Z. W., Li, H., and Liu, Z. (2018b). Ultrathin Two-Dimensional Materials for Photo- and Electrocatalytic Hydrogen Evolution. Mater. Today 21, 749–770. doi:10.1016/j.mattod.2018.01.034
Ding, L., Wei, R., Chen, H., Hu, J., and Li, J. (2015). Controllable Synthesis of Highly Active BiOCl Hierarchical Microsphere Self-Assembled by Nanosheets with Tunable Thickness. Appl. Catal. B: Environ. 172-173, 91–99. doi:10.1016/j.apcatb.2015.02.019
Fatta-Kassinos, D., Meric, S., and Nikolaou, A. (2011). Pharmaceutical Residues in Environmental Waters and Wastewater: Current State of Knowledge and Future Research. Anal. Bioanal. Chem. 399, 251–275. doi:10.1007/s00216-010-4300-9
Fu, H., Pan, C., Yao, W., and Zhu, Y. (2005). Visible-Light-Induced Degradation of Rhodamine B by Nanosized Bi2WO6. J. Phys. Chem. B 109, 22432–22439. doi:10.1021/jp052995j
Gadhi, T. A., Hernández-Gordillo, A., Bizarro, M., Jagdale, P., Tagliaferro, A., and Rodil, S. E. (2016). Efficient α/β-Bi 2 O 3 Composite for the Sequential Photodegradation of Two-Dyes Mixture. Ceramics Int. 42, 13065–13073. doi:10.1016/j.ceramint.2016.05.087
Ganose, A. M., Cuff, M., Butler, K. T., Walsh, A., and Scanlon, D. O. (2016). Interplay of Orbital and Relativistic Effects in Bismuth Oxyhalides: BiOF, BiOCl, BiOBr, and BiOI. Chem. Mater. 28, 1980–1984. doi:10.1021/acs.chemmater.6b00349
Gao, P., Yang, Y., Yin, Z., Kang, F., Fan, W., Sheng, J., et al. (2021). A Critical Review on Bismuth Oxyhalide Based Photocatalysis for Pharmaceutical Active Compounds Degradation: Modifications, Reactive Sites, and Challenges. J. Hazard. Mater. 412, 125186. doi:10.1016/j.jhazmat.2021.125186
Gao, Y., Wang, L., Li, Z., Li, C., Cao, X., Zhou, A., et al. (2014). Microwave-assisted Synthesis of Flower-like Ag-BiOCl Nanocomposite with Enhanced Visible-Light Photocatalytic Activity. Mater. Lett. 136, 295–297. doi:10.1016/j.matlet.2014.08.026
Garg, S., Yadav, M., Chandra, A., Gahlawat, S., Ingole, P. P., Pap, Z., et al. (2018b). Plant Leaf Extracts as Photocatalytic Activity Tailoring Agents for BiOCl towards Environmental Remediation. Ecotoxicology Environ. Saf. 165, 357–366. doi:10.1016/j.ecoenv.2018.09.024
Garg, S., Yadav, M., Chandra, A., and Hernadi, K. (2019). A Review on BiOX (X= Cl, Br and I) Nano-/microstructures for Their Photocatalytic Applications. J. Nanosci. Nanotechnol. 19, 280–294. doi:10.1166/jnn.2019.15771
Garg, S., Yadav, M., Chandra, A., Sapra, S., Gahlawat, S., Ingole, P., et al. (2018a). Facile green Synthesis of BiOBr Nanostructures with superior Visible-Light-Driven Photocatalytic Activity. Materials 11, 1273. doi:10.3390/ma11081273
Grzechulska, J., and Morawski, A. W. (2002). Photocatalytic Decomposition of Azo-Dye Acid Black 1 in Water over Modified Titanium Dioxide. Appl. Catal. B: Environ. 36, 45–51. doi:10.1016/s0926-3373(01)00275-2
Guo, C., Gao, S., Lv, J., Hou, S., Zhang, Y., and Xu, J. (2017). Assessing the Photocatalytic Transformation of Norfloxacin by BiOBr/iron Oxides Hybrid Photocatalyst: Kinetics, Intermediates, and Influencing Factors. Appl. Catal. B: Environ. 205, 68–77. doi:10.1016/j.apcatb.2016.12.032
Guo, J., Li, X., Liang, J., Yuan, X., Jiang, L., Yu, H., et al. (2021). Fabrication and Regulation of Vacancy-Mediated Bismuth Oxyhalide towards Photocatalytic Application: Development Status and Tendency. Coord. Chem. Rev. 443, 214033. doi:10.1016/j.ccr.2021.214033
Han, L., Guo, Y., Lin, Z., and Huang, H. (2020). 0D to 3D Controllable Nanostructures of BiOBr via a Facile and Fast Room-Temperature Strategy. Colloids Surf. A: Physicochemical Eng. Aspects 603, 125233. doi:10.1016/j.colsurfa.2020.125233
Hao, R., Xiao, X., Zuo, X., Nan, J., and Zhang, W. (2012). Efficient Adsorption and Visible-Light Photocatalytic Degradation of Tetracycline Hydrochloride Using Mesoporous BiOI Microspheres. J. Hazard. Mater. 209-210, 137–145. doi:10.1016/j.jhazmat.2012.01.006
He, J., Wang, J., Liu, Y., Mirza, Z. A., Zhao, C., and Xiao, W. (2015). Microwave-assisted Synthesis of BiOCl and its Adsorption and Photocatalytic Activity. Ceramics Int. 41, 8028–8033. doi:10.1016/j.ceramint.2015.02.152
Heidari, S., Haghighi, M., and Shabani, M. (2020). Sunlight-activated BiOCl/BiOBr-Bi24O31Br10 Photocatalyst for the Removal of Pharmaceutical Compounds. J. Clean. Prod. 259, 120679. doi:10.1016/j.jclepro.2020.120679
Henle, J., Simon, P., Frenzel, A., Scholz, S., and Kaskel, S. (2007). Nanosized BiOX (X = Cl, Br, I) Particles Synthesized in Reverse Microemulsions. Chem. Mater. 19, 366–373. doi:10.1021/cm061671k
Herrmann, J.-M. (1999). Heterogeneous Photocatalysis: Fundamentals and Applications to the Removal of Various Types of Aqueous Pollutants. Catal. Today 53, 115–129. doi:10.1016/s0920-5861(99)00107-8
Herrmann, J.-M. (2005). Heterogeneous Photocatalysis: State of the Art and Present Applications in Honor of Pr. R.L. Burwell Jr. (1912-2003), Former Head of Ipatieff Laboratories, Northwestern University, Evanston (Ill). Top. Catal. 34, 49–65. doi:10.1007/s11244-005-3788-2
Hidalgo, M. C., Aguilar, M., Maicu, M., Navío, J. A., and Colón, G. (2007). Hydrothermal Preparation of Highly Photoactive TiO2 Nanoparticles. Catal. Today 129, 50–58. doi:10.1016/j.cattod.2007.06.053
Hoffmann, M. R., Martin, S. T., Choi, W., and Bahnemann, D. W. (1995). Environmental Applications of Semiconductor Photocatalysis. Chem. Rev. 95, 69–96. doi:10.1021/cr00033a004
Huang, H., Xiao, K., Zhang, T., Dong, F., and Zhang, Y. (2017). Rational Design on 3D Hierarchical Bismuth Oxyiodides via In Situ Self-Template Phase Transformation and Phase-junction Construction for Optimizing Photocatalysis against Diverse Contaminants. Appl. Catal. B: Environ. 203, 879–888. doi:10.1016/j.apcatb.2016.10.082
Huang, W. L., and Zhu, Q. (2008). Electronic Structures of Relaxed BiOX (X=F, Cl, Br, I) Photocatalysts. Comput. Mater. Sci. 43, 1101–1108. doi:10.1016/j.commatsci.2008.03.005
Huang, Y., Li, H., Balogun, M.-S., Liu, W., Tong, Y., Lu, X., et al. (2014). Oxygen Vacancy Induced Bismuth Oxyiodide with Remarkably Increased Visible-Light Absorption and superior Photocatalytic Performance. ACS Appl. Mater. Inter. 6, 22920–22927. doi:10.1021/am507641k
Huizhong, A., Yi, D., Tianmin, W., Cong, W., Weichang, H., and Zhang, J. (2008). Photocatalytic Properties of Biox (X= Cl, Br, and I). Rare Met. 27, 243–250. doi:10.1016/S1001-0521(08)60123-0
Hussain, M. B., Khan, M. S., Loussala, H. M., and Bashir, M. S. (2020). The Synthesis of a BiOClxBr1−x Nanostructure Photocatalyst with High Surface Area for the Enhanced Visible-Light Photocatalytic Reduction of Cr(vi). RSC Adv. 10, 4763–4771. doi:10.1039/c9ra10256f
Jalalah, M., Faisal, M., Bouzid, H., Park, J.-G., Al-Sayari, S. A., and Ismail, A. A. (2015). Comparative Study on Photocatalytic Performances of Crystalline α- and β-Bi2O3 Nanoparticles under Visible Light. J. Ind. Eng. Chem. 30, 183–189. doi:10.1016/j.jiec.2015.05.020
Jia, M., Hu, X., Wang, S., Huang, Y., and Song, L. (2015). Photocatalytic Properties of Hierarchical BiOXs Obtained via an Ethanol-Assisted Solvothermal Process. J. Environ. Sci. 35, 172–180. doi:10.1016/j.jes.2014.09.045
Jia, T., Wu, J., Song, J., Liu, Q., Wang, J., Qi, Y., et al. (2020a). In Situ self-growing 3D Hierarchical BiOBr/BiOIO3 Z-Scheme Heterojunction with Rich Oxygen Vacancies and Iodine Ions as Carriers Transfer Dual-Channels for Enhanced Photocatalytic Activity. Chem. Eng. J. 396, 125258. doi:10.1016/j.cej.2020.125258
Jia, T., Yang, J., Zhang, Y., Ji, L., Chen, Y., Wang, D., et al. (2020b). Facet-dependent Flower-like BiOBr with Exposed the (0 0 1) and (0 1 0) Facets for Enhanced Charge Carrier Transfer and Photocatalytic Oxidation Activity. Chem. Phys. Lett. 760, 138010. doi:10.1016/j.cplett.2020.138010
Jiang, D., and Li, X. (2015). Advanced Photocatalysts with Nanoheterostructures. Mater. Focus 4, 14–19. doi:10.1166/mat.2015.1216
Jiang, G., Wang, R., Wang, X., Xi, X., Hu, R., Zhou, Y., et al. (2012). Novel Highly Active Visible-Light-Induced Photocatalysts Based on BiOBr with Ti Doping and Ag Decorating. ACS Appl. Mater. Inter. 4, 4440–4444. doi:10.1021/am301177k
Jiang, J., Zhao, K., Xiao, X., and Zhang, L. (2012). Synthesis and Facet-dependent Photoreactivity of BiOCl Single-Crystalline Nanosheets. J. Am. Chem. Soc. 134, 4473–4476. doi:10.1021/ja210484t
Jiang, L., Zhou, G., Mi, J., and Wu, Z. (2012). Fabrication of Visible-Light-Driven One-Dimensional Anatase TiO2/Ag Heterojunction Plasmonic Photocatalyst. Catal. Commun. 24, 48–51. doi:10.1016/j.catcom.2012.03.017
Jiang, R., Lu, G., Yan, Z., Wu, D., Zhou, R., and Bao, X. (2019). Insights into a CQD-SnNb2O6/BiOCl Z-Scheme System for the Degradation of Benzocaine: Influence Factors, Intermediate Toxicity and Photocatalytic Mechanism. Chem. Eng. J. 374, 79–90. doi:10.1016/j.cej.2019.05.176
Jiang, T., Li, J., Gao, Y., Li, L., Lu, T., and Pan, L. (2017). BiOBr/BiOF Composites for Efficient Degradation of Rhodamine B and Nitrobenzene under Visible Light Irradiation. J. Colloid Interf. Sci. 490, 812–818. doi:10.1016/j.jcis.2016.12.007
Jin, X., Lv, C., Zhou, X., Zhang, C., Zhang, B., Su, H., et al. (2018). Realizing the Regulated Carrier Separation and Exciton Generation of Bi24O31Cl10via a Carbon Doping Strategy. J. Mater. Chem. A. 6, 24350–24357. doi:10.1039/c8ta08598f
Juntrapirom, S., Tantraviwat, D., Suntalelat, S., Thongsook, O., Phanichphant, S., and Inceesungvorn, B. (2017). Visible Light Photocatalytic Performance and Mechanism of Highly Efficient SnS/BiOI Heterojunction. J. Colloid Interf. Sci. 504, 711–720. doi:10.1016/j.jcis.2017.06.019
Kása, Z., Orbán, E., Pap, Z., Ábrahám, I., Magyari, K., Garg, S., et al. (2020). Innovative and Cost-Efficient BiOI Immobilization Technique on Ceramic Paper-Total Coverage and High Photocatalytic Activity. Nanomaterials 10, 1959.
Kong, X. Y., Lee, W. P. C., Ong, W.-J., Chai, S.-P., and Mohamed, A. R. (2016). Oxygen-Deficient BiOBr as a Highly Stable Photocatalyst for Efficient CO2Reduction into Renewable Carbon-Neutral Fuels. ChemCatChem 8, 3074–3081. doi:10.1002/cctc.201600782
Landge, V. K., Sonawane, S. H., Sivakumar, M., Sonawane, S. S., Uday Bhaskar Babu, G., and Boczkaj, G. (2021). S-scheme Heterojunction Bi2O3-ZnO/Bentonite clay Composite with Enhanced Photocatalytic Performance. Sustainable Energ. Tech. Assessments 45, 101194. doi:10.1016/j.seta.2021.101194
Ledakowicz, S., and Gonera, M. (1999). Optimisation of Oxidants Dose for Combined Chemical and Biological Treatment of Textile Wastewater. Water Res. 33, 2511–2516. doi:10.1016/s0043-1354(98)00494-1
Lee, S.-Y., and Park, S.-J. (2013). TiO2 Photocatalyst for Water Treatment Applications. J. Ind. Eng. Chem. 19, 1761–1769. doi:10.1016/j.jiec.2013.07.012
Lee, Y.-H., Dai, Y.-M., Fu, J.-Y., and Chen, C.-C. (2017). A Series of Bismuth-Oxychloride/bismuth-Oxyiodide/graphene-Oxide Nanocomposites: Synthesis, Characterization, and Photcatalytic Activity and Mechanism. Mol. Catal. 432, 196–209. doi:10.1016/j.mcat.2017.01.002
Li, G., Qin, F., Yang, H., Lu, Z., Sun, H., and Chen, R. (2012). Facile Microwave Synthesis of 3D Flowerlike BiOBr Nanostructures and Their Excellent Cr VI Removal Capacity. Eur. J. Inorg. Chem. 2012, 2508–2513. doi:10.1002/ejic.201101427
Li, H., Jia, Q., Cui, Y., and Fan, S. (2013). Photocatalytic Properties of BiOI Synthesized by a Simple Hydrothermal Process. Mater. Lett. 107, 262–264. doi:10.1016/j.matlet.2013.06.019
Li, H., Li, J., Ai, Z., Jia, F., and Zhang, L. (2018). Oxygen Vacancy-Mediated Photocatalysis of BiOCl: Reactivity, Selectivity, and Perspectives. Angew. Chem. Int. Ed. 57, 122–138. doi:10.1002/anie.201705628
Li, H., Shang, J., Ai, Z., and Zhang, L. (2015). Efficient Visible Light Nitrogen Fixation with BiOBr Nanosheets of Oxygen Vacancies on the Exposed {001} Facets. J. Am. Chem. Soc. 137, 6393–6399. doi:10.1021/jacs.5b03105
Li, J., Zhang, L., Li, Y., and Yu, Y. (2013). Synthesis and Internal Electric Field Dependent Photoreactivity of Bi3O4Cl Single-Crystalline Nanosheets with High {001} Facet Exposure Percentages. Nanoscale 6, 167–171. doi:10.1039/c3nr05246j
Li, J., Li, H., Zhan, G., and Zhang, L. (2017). Solar Water Splitting and Nitrogen Fixation with Layered Bismuth Oxyhalides. Acc. Chem. Res. 50, 112–121. doi:10.1021/acs.accounts.6b00523
Li, J., Zhao, K., Yu, Y., and Zhang, L. (2015). Facet-Level Mechanistic Insights into General Homogeneous Carbon Doping for Enhanced Solar-To-Hydrogen Conversion. Adv. Funct. Mater. 25, 2189–2201. doi:10.1002/adfm.201404178
Li, K.-L., Lee, W. W., Lu, C.-S., Dai, Y.-M., Chou, S.-Y., Chen, H.-L., et al. (2014). Synthesis of BiOBr, Bi3O4Br, and Bi12O17Br2 by Controlled Hydrothermal Method and Their Photocatalytic Properties. J. Taiwan Inst. Chem. Eng. 45, 2688–2697. doi:10.1016/j.jtice.2014.04.001
Li, L., Salvador, P. A., and Rohrer, G. S. (2014). Photocatalysts with Internal Electric fields. Nanoscale 6, 24–42. doi:10.1039/c3nr03998f
Li, M., Song, C., Wu, Y., Wang, M., Pan, Z., Sun, Y., et al. (2019). Novel Z-Scheme Visible-Light Photocatalyst Based on CoFe2O4/BiOBr/Graphene Composites for Organic Dye Degradation and Cr(VI) Reduction. Appl. Surf. Sci. 478, 744–753. doi:10.1016/j.apsusc.2019.02.017
Li, P., Cao, W., Zhu, Y., Teng, Q., Peng, L., Jiang, C., et al. (2020). NaOH-induced Formation of 3D Flower-Sphere BiOBr/Bi4O5Br2 with Proper-Oxygen Vacancies via In-Situ Self-Template Phase Transformation Method for Antibiotic Photodegradation. Sci. Total Environ. 715, 136809. doi:10.1016/j.scitotenv.2020.136809
Li, R., Gao, X., Fan, C., Zhang, X., Wang, Y., and Wang, Y. (2015). A Facile Approach for the Tunable Fabrication of BiOBr Photocatalysts with High Activity and Stability. Appl. Surf. Sci. 355, 1075–1082. doi:10.1016/j.apsusc.2015.07.216
Li, W. T., Huang, W. Z., Zhou, H., Yin, H. Y., Zheng, Y. F., and Song, X. C. (2015). Synthesis of Zn2+ Doped BiOCl Hierarchical Nanostructures and Their Exceptional Visible Light Photocatalytic Properties. J. Alloys Compd. 638, 148–154. doi:10.1016/j.jallcom.2015.03.103
Li, W., Zou, Y., Geng, X., Xiao, F., An, G., and Wang, D. (2017). Constructing Highly Catalytic Oxidation over BiOBr-Based Hierarchical Microspheres: Importance of Redox Potential of Doped Cations. Mol. Catal. 438, 19–29. doi:10.1016/j.mcat.2017.05.017
Li, X., Wang, T., Tao, X., Qiu, G., Li, C., and Li, B. (2020). Interfacial Synergy of Pd Sites and Defective BiOBr for Promoting the Solar-Driven Selective Oxidation of Toluene. J. Mater. Chem. A. 8, 17657–17669. doi:10.1039/d0ta05733a
Li, Y., Qin, H., Sun, H., Zhang, M., Wang, J., Yao, H., et al. (2015). Synthesis of BiOI Hierarchical Nanospheres and Their Application in Photocatalysis. Mater. Lett. 152, 248–251. doi:10.1016/j.matlet.2015.03.126
Li, Z., Qu, Y., Hu, K., Humayun, M., Chen, S., and Jing, L. (2017). Improved Photoelectrocatalytic Activities of BiOCl with High Stability for Water Oxidation and MO Degradation by Coupling RGO and Modifying Phosphate Groups to Prolong Carrier Lifetime. Appl. Catal. B: Environ. 203, 355–362. doi:10.1016/j.apcatb.2016.10.045
Lin, J., Shen, J., Wang, R., Cui, J., Zhou, W., Hu, P., et al. (2011). Nano-p-n Junctions on Surface-Coarsened TiO2 Nanobelts with Enhanced Photocatalytic Activity. J. Mater. Chem. 21, 5106–5113. doi:10.1039/c0jm04131a
Lin, W., Yu, X., Shen, Y., Chen, H., Zhu, Y., Zhang, Y., et al. (2017). Carbon dots/BiOCl Films with Enhanced Visible Light Photocatalytic Performance. J. Nanopart. Res. 19, 56. doi:10.1007/s11051-017-3764-3
Lin, Z.-R., Zhao, L., and Dong, Y.-H. (2015). Quantitative Characterization of Hydroxyl Radical Generation in a Goethite-Catalyzed Fenton-like Reaction. Chemosphere 141, 7–12. doi:10.1016/j.chemosphere.2015.05.066
Linsebigler, A. L., Lu, G., and Yates, J. T. (1995). Photocatalysis on TiO2 Surfaces: Principles, Mechanisms, and Selected Results. Chem. Rev. 95, 735–758. doi:10.1021/cr00035a013
Liu, C., Nanaboina, V., Korshin, G. V., and Jiang, W. (2012). Spectroscopic Study of Degradation Products of Ciprofloxacin, Norfloxacin and Lomefloxacin Formed in Ozonated Wastewater. Water Res. 46, 5235–5246. doi:10.1016/j.watres.2012.07.005
Liu, C., Zhou, J., Su, J., and Guo, L. (2019). Turning the Unwanted Surface Bismuth Enrichment to Favourable BiVO4/BiOCl Heterojunction for Enhanced Photoelectrochemical Performance. Appl. Catal. B: Environ. 241, 506–513. doi:10.1016/j.apcatb.2018.09.060
Liu, H., Cao, W., Su, Y., Wang, Y., and Wang, X. (2012). Synthesis, Characterization and Photocatalytic Performance of Novel Visible-Light-Induced Ag/BiOI. Appl. Catal. B: Environ. 111-112, 271–279. doi:10.1016/j.apcatb.2011.10.008
Liu, J., Yuan, Q., Zhao, H., and Zou, S. (2018). Efficient Room-Temperature Selective Oxidation of Benzyl Alcohol into Benzaldehyde over Pt/BiOCl Nanocomposite. Catal. Lett. 148, 1093–1099. doi:10.1007/s10562-018-2337-0
Liu, Y., Hu, Z., and Yu, J. C. (2021). Photocatalytic Degradation of Ibuprofen on S-Doped BiOBr. Chemosphere 278, 130376. doi:10.1016/j.chemosphere.2021.130376
Liu, Y., and Wu, Q. (2017). One Novel Material with High Visible-Light Activity: Hexagonal Cu Flakelets Embedded in the Petals of BiOBr Flower-Nanospheres. J. Nanopart. Res. 19, 55. doi:10.1007/s11051-016-3734-1
Liu, Z., Wu, B., Xiang, D., and Zhu, Y. (2012). Effect of Solvents on Morphology and Photocatalytic Activity of BiOBr Synthesized by Solvothermal Method. Mater. Res. Bull. 47, 3753–3757. doi:10.1016/j.materresbull.2012.06.026
Liu, Z., Wu, B., Zhao, Y., Niu, J., and Zhu, Y. (2014). Solvothermal Synthesis and Photocatalytic Activity of Al-Doped BiOBr Microspheres. Ceramics Int. 40, 5597–5603. doi:10.1016/j.ceramint.2013.10.152
Long, Z., Zhang, G., Du, H., Zhu, J., and Li, J. (2021). Preparation and Application of BiOBr-Bi2S3 Heterojunctions for Efficient Photocatalytic Removal of Cr(VI). J. Hazard. Mater. 407, 124394. doi:10.1016/j.jhazmat.2020.124394
López-Velázquez, K., Guzmán-Mar, J. L., Montalvo-Herrera, T. J., Mendiola-Alvarez, S. Y., and Villanueva-Rodríguez, M. (2021). Efficient Photocatalytic Removal of Four Endocrine-Disrupting Compounds Using N-Doped BiOBr Catalyst under UV-Vis Radiation. J. Environ. Chem. Eng. 9, 106185. doi:10.1016/j.jece.2021.106185
Lu, L., Kong, L., Jiang, Z., Lai, H. H.-C., Xiao, T., and Edwards, P. P. (2012). Visible-light-driven Photodegradation of Rhodamine B on Ag-Modified BiOBr. Catal. Lett. 142, 771–778. doi:10.1007/s10562-012-0824-2
Lu, Y., Song, J., Li, W., Pan, Y., Fang, H., Wang, X., et al. (2020). Preparation of BiOCl/Bi2S3 Composites by Simple Ion Exchange Method for Highly Efficient Photocatalytic Reduction of Cr6+. Appl. Surf. Sci. 506, 145000. doi:10.1016/j.apsusc.2019.145000
Luo, W., Yang, Z., Li, Z., Zhang, J., Liu, J., Zhao, Z., et al. (2011). Solar Hydrogen Generation from Seawater with a Modified BiVO4 Photoanode. Energy Environ. Sci. 4, 4046–4051. doi:10.1039/c1ee01812d
Lv, X., Yan, D. Y. S., Lam, F. L.-Y., Ng, Y. H., Yin, S., and An, A. K. (2020). Solvothermal Synthesis of Copper-Doped BiOBr Microflowers with Enhanced Adsorption and Visible-Light Driven Photocatalytic Degradation of Norfloxacin. Chem. Eng. J. 401, 126012. doi:10.1016/j.cej.2020.126012
Lyu, J., Hu, Z., Li, Z., and Ge, M. (2019). Removal of Tetracycline by BiOBr Microspheres with Oxygen Vacancies: Combination of Adsorption and Photocatalysis. J. Phys. Chem. Sol. 129, 61–70. doi:10.1016/j.jpcs.2018.12.041
Ma, F.-Q., Yao, J.-W., Zhang, Y.-F., and Wei, Y. (2017). Unique Band Structure Enhanced Visible Light Photocatalytic Activity of Phosphorus-Doped BiOI Hierarchical Microspheres. RSC Adv. 7, 36288–36296. doi:10.1039/c7ra06261c
Ma, X., Ma, Z., Liao, T., Liu, X., Zhang, Y., Li, L., et al. (2017). Preparation of BiVO4/BiOCl Heterojunction Photocatalyst by In-Situ Transformation Method for Norfloxacin Photocatalytic Degradation. J. Alloys Compd. 702, 68–74. doi:10.1016/j.jallcom.2017.01.214
Ma, Z., Li, P., Ye, L., Zhou, Y., Su, F., Ding, C., et al. (2017). Oxygen Vacancies Induced Exciton Dissociation of Flexible BiOCl Nanosheets for Effective Photocatalytic CO2 Conversion. J. Mater. Chem. A. 5, 24995–25004. doi:10.1039/c7ta08766g
Maimaitizi, H., Abulizi, A., Kadeer, K., Talifu, D., and Tursun, Y. (2020). In Situ synthesis of Pt and N Co-doped Hollow Hierarchical BiOCl Microsphere as an Efficient Photocatalyst for Organic Pollutant Degradation and Photocatalytic CO2 Reduction. Appl. Surf. Sci. 502, 144083. doi:10.1016/j.apsusc.2019.144083
Malato, S., Blanco, J., Alarcón, D. C., Maldonado, M. I., Fernández-Ibáñez, P., and Gernjak, W. (2007). Photocatalytic Decontamination and Disinfection of Water with Solar Collectors. Catal. Today 122, 137–149. doi:10.1016/j.cattod.2007.01.034
Malefane, M. E., Feleni, U., Mafa, P. J., and Kuvarega, A. T. (2020). Fabrication of Direct Z-Scheme Co3O4/BiOI for Ibuprofen and Trimethoprim Degradation under Visible Light Irradiation. Appl. Surf. Sci. 514, 145940. doi:10.1016/j.apsusc.2020.145940
Mao, D., Lü, X., Jiang, Z., Xie, J., Lu, X., Wei, W., et al. (2014). Ionic Liquid-Assisted Hydrothermal Synthesis of Square BiOBr Nanoplates with Highly Efficient Photocatalytic Activity. Mater. Lett. 118, 154–157. doi:10.1016/j.matlet.2013.12.049
Meng, X., and Zhang, Z. Synthesis. (2015). Analysis, and Testing of BiOBr-Bi2WO6 Photocatalytic Heterojunction Semiconductors. Int. J. Photoenergy 2015. doi:10.1155/2015/630476
Mokhtari, F., and Tahmasebi, N. (2021). Hydrothermal Synthesis of W-Doped BiOCl Nanoplates for Photocatalytic Degradation of Rhodamine B under Visible Light. J. Phys. Chem. Sol. 149, 109804. doi:10.1016/j.jpcs.2020.109804
Montoya-Zamora, J. M., Martínez-de la Cruz, A., and López Cuéllar, E. (2017). Enhanced Photocatalytic Activity of BiOI Synthesized in Presence of EDTA. J. Taiwan Inst. Chem. Eng. 75, 307–316. doi:10.1016/j.jtice.2017.03.031
Ni, Z., Sun, Y., Zhang, Y., and Dong, F. (2016). Fabrication, Modification and Application of (BiO)2CO3-based Photocatalysts: A Review. Appl. Surf. Sci. 365, 314–335. doi:10.1016/j.apsusc.2015.12.231
Ning, S., Ding, L., Lin, Z., Lin, Q., Zhang, H., Lin, H., et al. (2016). One-pot Fabrication of Bi3O4Cl/BiOCl Plate-On-Plate Heterojunction with Enhanced Visible-Light Photocatalytic Activity. Appl. Catal. B: Environ. 185, 203–212. doi:10.1016/j.apcatb.2015.12.021
Obeid, M. M., Stampfl, C., Bafekry, A., Guan, Z., Jappor, H. R., Nguyen, C. V., et al. (2020). First-principles Investigation of Nonmetal Doped Single-Layer BiOBr as a Potential Photocatalyst with a Low Recombination Rate. Phys. Chem. Chem. Phys. 22, 15354–15364. doi:10.1039/d0cp02007a
Oudghiri-Hassani, H., Rakass, S., Al Wadaani, F. T., Al-Ghamdi, K. J., Omer, A., Messali, M., et al. (2015). Synthesis, Characterization and Photocatalytic Activity of α-Bi2O3 Nanoparticles. J. Taibah Univ. Sci. 9, 508–512. doi:10.1016/j.jtusci.2015.01.009
Pan, C., and Zhu, Y. (2010). New Type of BiPO4 Oxy-Acid Salt Photocatalyst with High Photocatalytic Activity on Degradation of Dye. Environ. Sci. Technol. 44, 5570–5574. doi:10.1021/es101223n
Pan, C., and Zhu, Y. (2011). Size-controlled Synthesis of BiPO4 Nanocrystals for Enhanced Photocatalytic Performance. J. Mater. Chem. 21, 4235–4241. doi:10.1039/c0jm03655b
Pare, B., Sarwan, B., and Jonnalagadda, S. B. (2011). Photocatalytic Mineralization Study of Malachite green on the Surface of Mn-Doped BiOCl Activated by Visible Light under Ambient Condition. Appl. Surf. Sci. 258, 247–253. doi:10.1016/j.apsusc.2011.08.040
Park, H., Bak, A., Ahn, Y. Y., Choi, J., and Hoffmannn, M. R. (2012). Photoelectrochemical Performance of Multi-Layered BiOx-TiO2/Ti Electrodes for Degradation of Phenol and Production of Molecular Hydrogen in Water. J. Hazard. Mater. 211-212, 47–54. doi:10.1016/j.jhazmat.2011.05.009
Peng, Y., Xu, J., Liu, T., and Mao, Y.-g. (2017). Controlled Synthesis of One-Dimensional BiOBr with Exposed (110) Facets and Enhanced Photocatalytic Activity. CrystEngComm 19, 6473–6480. doi:10.1039/c7ce01452j
Peng, Y., Yan, M., Chen, Q.-G., Fan, C.-M., Zhou, H.-Y., and Xu, A.-W. (2014). Novel One-Dimensional Bi2O3-Bi2WO6 P-N Hierarchical Heterojunction with Enhanced Photocatalytic Activity. J. Mater. Chem. A. 2, 8517–8524. doi:10.1039/c4ta00274a
Qin, H., Wang, K., Jiang, L., Li, J., Wu, X., and Zhang, G. (2020). Ultrasonic-assisted Fabrication of a Direct Z-Scheme BiOI/Bi2O4 Heterojunction with superior Visible Light-Responsive Photocatalytic Performance. J. Alloys Compd. 821, 153417. doi:10.1016/j.jallcom.2019.153417
Qu, X., Alvarez, P. J. J., and Li, Q. (2013). Applications of Nanotechnology in Water and Wastewater Treatment. Water Res. 47, 3931–3946. doi:10.1016/j.watres.2012.09.058
Raizada, P., Priya, B., Thakur, P., and Singh, P. (2016). “Solar Light Induced Photodegradation of Oxytetracyline Using Zr Doped TiO2/CaO Based Nanocomposite,” in Indian Journal of Chemistry (Chennai, Tamil Nadu: Council of Scientific & Industrial Research).
Razavi-Khosroshahi, H., Mohammadzadeh, S., Hojamberdiev, M., Kitano, S., Yamauchi, M., and Fuji, M. (2019). BiVO4/BiOX (X = F, Cl, Br, I) Heterojunctions for Degrading Organic Dye under Visible Light. Adv. Powder Techn. 30, 1290–1296. doi:10.1016/j.apt.2019.04.002
Ren, X., Wu, K., Qin, Z., Zhao, X., and Yang, H. (2019). The Construction of Type II Heterojunction of Bi2WO6/BiOBr Photocatalyst with Improved Photocatalytic Performance. J. Alloys Compd. 788, 102–109. doi:10.1016/j.jallcom.2019.02.211
Richardson, S. D. (2012). Environmental Mass Spectrometry: Emerging Contaminants and Current Issues. Anal. Chem. 84, 747–778. doi:10.1021/ac202903d
Robinson, A. A., Belden, J. B., and Lydy, M. J. (2005). Toxicity of Fluoroquinolone Antibiotics to Aquatic Organisms. Environ. Toxicol. Chem. 24, 423–430. doi:10.1897/04-210r.1
Salnikow, K., and Zhitkovich, A. (2008). Genetic and Epigenetic Mechanisms in Metal Carcinogenesis and Cocarcinogenesis: Nickel, Arsenic, and Chromium. Chem. Res. Toxicol. 21, 28–44. doi:10.1021/tx700198a
Senasu, T., Nijpanich, S., Juabrum, S., Chanlek, N., and Nanan, S. (2021). CdS/BiOBr Heterojunction Photocatalyst with High Performance for Solar-Light-Driven Degradation of Ciprofloxacin and Norfloxacin Antibiotics. Appl. Surf. Sci. 567, 150850. doi:10.1016/j.apsusc.2021.150850
Shang, J., Hao, W., Lv, X., Wang, T., Wang, X., Du, Y., et al. (2014). Bismuth Oxybromide with Reasonable Photocatalytic Reduction Activity under Visible Light. ACS Catal. 4, 954–961. doi:10.1021/cs401025u
Sharma, K., Dutta, V., Sharma, S., Raizada, P., Hosseini-Bandegharaei, A., Thakur, P., et al. (2019). Recent Advances in Enhanced Photocatalytic Activity of Bismuth Oxyhalides for Efficient Photocatalysis of Organic Pollutants in Water: a Review. J. Ind. Eng. Chem. 78, 1–20. doi:10.1016/j.jiec.2019.06.022
Sharma, N., Pap, Z., Garg, S., and Hernádi, K. (2019). Hydrothermal Synthesis of BiOBr and BiOBr/CNT Composites, Their Photocatalytic Activity and the Importance of Early Bi6O6(OH)3(NO3)3·1.5H2O Formation. Appl. Surf. Sci. 495, 143536. doi:10.1016/j.apsusc.2019.143536
Sharma, N., Pap, Z., Kornélia, B., Gyulavari, T., Karacs, G., Nemeth, Z., et al. (2022). Effective Removal of Phenol by Activated charcoal/BiOCl Composite under UV Light Irradiation. J. Mol. Struct. 1254, 132344. doi:10.1016/j.molstruc.2022.132344
Sharma, N., Pap, Z., Székely, I., Focsan, M., Karacs, G., Nemeth, Z., et al. (2021). Combination of Iodine-Deficient BiOI Phases in the Presence of CNT to Enhance Photocatalytic Activity towards Phenol Decomposition under Visible Light. Appl. Surf. Sci. 565, 150605. doi:10.1016/j.apsusc.2021.150605
Shi, X., Mao, Y., Knapton, A. D., Ding, M., Rojanasakul, Y., Gannett, P. M., et al. (1994). Reaction of Cr(VI) with Ascorbate and Hydrogen Peroxide Generates Hydroxyl Radicals and Causes DNA Damage: Role of a Cr(IV)-mediated Fenton-like Reaction. Carcinogenesis 15, 2475–2478. doi:10.1093/carcin/15.11.2475
Shi, X., Wang, P., Wang, L., Bai, Y., Xie, H., Zhou, Y., et al. (2018). Few Layered BiOBr with Expanded Interlayer Spacing and Oxygen Vacancies for Efficient Decomposition of Real Oil Field Produced Wastewater. ACS Sustain. Chem. Eng. 6, 13739–13746. doi:10.1021/acssuschemeng.8b01622
Sin, J.-C., Lam, S.-M., Zeng, H., Lin, H., Li, H., Kugan Kumaresan, A., et al. (2020). Z-scheme Heterojunction Nanocomposite Fabricated by Decorating Magnetic MnFe2O4 Nanoparticles on BiOBr Nanosheets for Enhanced Visible Light Photocatalytic Degradation of 2,4-dichlorophenoxyacetic Acid and Rhodamine B. Separat. Purif. Techn. 250, 117186. doi:10.1016/j.seppur.2020.117186
Song, J., Zhang, Z., Zhi, S., Niu, B., Dong, H., Wu, D., et al. (2021). Oxygen-vacancy-rich BiOCl with 3D Network Structure for Enhanced Photocatalytic CO2 Reduction and Antibiotic Degradation. J. Taiwan Inst. Chem. E 128, 380–387. doi:10.1016/j.jtice.2021.08.044
Song, Z., Dong, X., Wang, N., Zhu, L., Luo, Z., Fang, J., et al. (2017). Efficient Photocatalytic Defluorination of Perfluorooctanoic Acid over BiOCl Nanosheets via a Hole Direct Oxidation Mechanism. Chem. Eng. J. 317, 925–934. doi:10.1016/j.cej.2017.02.126
Sturini, M., Speltini, A., Maraschi, F., Pretali, L., Profumo, A., Fasani, E., et al. (2012). Photodegradation of Fluoroquinolones in Surface Water and Antimicrobial Activity of the Photoproducts. Water Res. 46, 5575–5582. doi:10.1016/j.watres.2012.07.043
Su, J., Xiao, Y., and Ren, M. (2014). Direct Hydrolysis Synthesis of BiOI Flowerlike Hierarchical Structures and It's Photocatalytic Activity under Simulated Sunlight Irradiation. Catal. Commun. 45, 30–33. doi:10.1016/j.catcom.2013.10.020
Su, M., He, C., Zhu, L., Sun, Z., Shan, C., Zhang, Q., et al. (2012). Enhanced Adsorption and Photocatalytic Activity of BiOI-MWCNT Composites towards Organic Pollutants in Aqueous Solution. J. Hazard. Mater. 229-230, 72–82. doi:10.1016/j.jhazmat.2012.05.061
Su, X., Yang, J., Yu, X., Zhu, Y., and Zhang, Y. (2018). In Situ grown Hierarchical 50% BiOCl/BiOI Hollow Flowerlike Microspheres on Reduced Graphene Oxide Nanosheets for Enhanced Visible-Light Photocatalytic Degradation of Rhodamine B. Appl. Surf. Sci. 433, 502–512. doi:10.1016/j.apsusc.2017.09.258
Sun, Y., Qi, X., Li, R., Xie, Y., Tang, Q., and Ren, B. (2020). Hydrothermal Synthesis of 2D/2D BiOCl/g-C3n4 Z-Scheme: For TC Degradation and Antimicrobial Activity Evaluation. Opt. Mater. 108, 110170. doi:10.1016/j.optmat.2020.110170
Tahmasebi, N., Maleki, Z., and Farahnak, P. (2019). Enhanced Photocatalytic Activities of Bi2WO6/BiOCl Composite Synthesized by One-step Hydrothermal Method with the Assistance of HCl. Mater. Sci. Semiconductor Process. 89, 32–40. doi:10.1016/j.mssp.2018.08.026
Tang, X., Liu, H., Yang, C., Jin, X., Zhong, J., and Li, J. (2020). In-situ Fabrication of Z-Scheme CdS/BiOCl Heterojunctions with Largely Improved Photocatalytic Performance. Colloids Surf. A: Physicochemical Eng. Aspects 599, 124880. doi:10.1016/j.colsurfa.2020.124880
Tettey, K. E., Yee, M. Q., and Lee, D. (2010). Photocatalytic and Conductive MWCNT/TiO2 Nanocomposite Thin Films. ACS Appl. Mater. Inter. 2, 2646–2652. doi:10.1021/am1004656
Tian, N., Huang, H., Wang, S., Zhang, T., Du, X., and Zhang, Y. (2020). Facet-charge-induced Coupling Dependent Interfacial Photocharge Separation: A Case of BiOI/g-C3n4 P-N junction. Appl. Catal. B: Environ. 267, 118697. doi:10.1016/j.apcatb.2020.118697
Tu, X., Luo, S., Chen, G., and Li, J. (2012). One-Pot Synthesis, Characterization, and Enhanced Photocatalytic Activity of a BiOBr-Graphene Composite. Chem. Eur. J. 18, 14359–14366. doi:10.1002/chem.201200892
Tu, X., Qian, S., Chen, L., and Qu, L. (2015). The Influence of Sn(II) Doping on the Photoinduced Charge and Photocatalytic Properties of BiOBr Microspheres. J. Mater. Sci. 50, 4312–4323. doi:10.1007/s10853-015-8983-3
Vadivel, S., Keerthi, P., Vanitha, M., Muthukrishnaraj, A., and Balasubramanian, N. (2014). Solvothermal Synthesis of Sm-Doped BiOBr/RGO Composite as an Efficient Photocatalytic Material for Methyl orange Degradation. Mater. Lett. 128, 287–290. doi:10.1016/j.matlet.2014.04.047
Vinoth, S., and Pandikumar, A. (2021). Ni Integrated S-gC3N4/BiOBr Based Type-II Heterojunction as a Durable Catalyst for Photoelectrochemical Water Splitting. Renew. Energ. 173, 507–519. doi:10.1016/j.renene.2021.03.121
Vinoth, S., Rajaitha, P. M., and Pandikumar, A. (2021). Modulating Photoelectrochemical Water Splitting Performance by Constructing a Type-II Heterojunction between G-C3n4 and BiOI. New J. Chem. 45, 2010–2018. doi:10.1039/d0nj05384h
Wang, C.-Y., Zhang, X., Qiu, H.-B., Huang, G.-X., and Yu, H.-Q. (2017). Bi24O31Br10 Nanosheets with Controllable Thickness for Visible-Light-Driven Catalytic Degradation of Tetracycline Hydrochloride. Appl. Catal. B: Environ. 205, 615–623. doi:10.1016/j.apcatb.2017.01.015
Wang, F., Gu, Y., Yang, Z., Xie, Y., Zhang, J., Shang, X., et al. (2018). The Effect of Halogen on BiOX (X = Cl, Br, I)/Bi2WO6 Heterojunction for Visible-Light-Driven Photocatalytic Benzyl Alcohol Selective Oxidation. Appl. Catal. A: Gen. 567, 65–72. doi:10.1016/j.apcata.2018.09.010
Wang, H., Liao, B., Lu, T., Ai, Y., and Liu, G. (2020). Enhanced Visible-Light Photocatalytic Degradation of Tetracycline by a Novel Hollow BiOCl@CeO2 Heterostructured Microspheres: Structural Characterization and Reaction Mechanism. J. Hazard. Mater. 385, 121552. doi:10.1016/j.jhazmat.2019.121552
Wang, J.-J., Zhai, X.-Y., Zhang, G.-Y., Zhang, J.-B., and Zhao, Y.-F. (2020). Facile Construction of BiOBr/Bi2O2CO3 P-N Heterojunction by Precipitation Conversion at Ambient Temperature for Charge Transfer and Photocatalytic Activity. Solid State. Sci. 105, 106288. doi:10.1016/j.solidstatesciences.2020.106288
Wang, J., Wei, Y., Yang, B., Wang, B., Chen, J., and Jing, H. (2019). In Situ grown Heterojunction of Bi2WO6/BiOCl for Efficient Photoelectrocatalytic CO2 Reduction. J. Catal. 377, 209–217. doi:10.1016/j.jcat.2019.06.007
Wang, J., and Zhang, Z. (2020). Co-precipitation Synthesis and Photocatalytic Properties of BiOCl Microflowers. Optik 204, 164149. doi:10.1016/j.ijleo.2019.164149
Wang, Q., Liu, Z., Liu, D., Wang, W., Zhao, Z., Cui, F., et al. (2019). Oxygen Vacancy-Rich Ultrathin Sulfur-Doped Bismuth Oxybromide Nanosheet as a Highly Efficient Visible-Light Responsive Photocatalyst for Environmental Remediation. Chem. Eng. J. 360, 838–847. doi:10.1016/j.cej.2018.12.038
Wang, S., Yang, X., Zhang, X., Ding, X., Yang, Z., Dai, K., et al. (2017). A Plate-On-Plate Sandwiched Z-Scheme Heterojunction Photocatalyst: BiOBr-Bi 2 MoO 6 with Enhanced Photocatalytic Performance. Appl. Surf. Sci. 391, 194–201. doi:10.1016/j.apsusc.2016.07.070
Wang, Y., Jin, J., Chu, W., Cahen, D., and He, T. (2018). Synergistic Effect of Charge Generation and Separation in Epitaxially Grown BiOCl/Bi2S3 Nano-Heterostructure. ACS Appl. Mater. Inter. 10, 15304–15313. doi:10.1021/acsami.8b03390
Wang, Z., Chen, M., Huang, D., Zeng, G., Xu, P., Zhou, C., et al. (2019). Multiply Structural Optimized Strategies for Bismuth Oxyhalide Photocatalysis and Their Environmental Application. Chem. Eng. J. 374, 1025–1045. doi:10.1016/j.cej.2019.06.018
Wei, Z., Dong, X., Zheng, N., Wang, Y., Zhang, X., and Ma, H. (2020). Novel Visible-Light Irradiation Niobium-Doped BiOBr Microspheres with Enhanced Photocatalytic Performance. J. Mater. Sci. 55, 16522–16532. doi:10.1007/s10853-020-05265-3
Weng, B., Liu, S., Zhang, N., Tang, Z.-R., and Xu, Y.-J. (2014a). A Simple yet Efficient Visible-Light-Driven CdS Nanowires-Carbon Nanotube 1D-1D Nanocomposite Photocatalyst. J. Catal. 309, 146–155. doi:10.1016/j.jcat.2013.09.013
Weng, B., Xu, F., and Xu, J. (2014b). Hierarchical Structures Constructed by BiOX (X= Cl, I) Nanosheets on CNTs/carbon Composite Fibers for Improved Photocatalytic Degradation of Methyl orange. J. Nanopart. Res. 16, 1–13. doi:10.1007/s11051-014-2766-7
WHO/UNICEF Joint Monitoring Programme for Water Supply and Sanitation, UNICEF (2005). Water for Life: Making it Happen. Geneva: World health organization.
Wu, D., Yue, S., Wang, W., An, T., Li, G., Yip, H. Y., et al. (2016). Boron Doped BiOBr Nanosheets with Enhanced Photocatalytic Inactivation of Escherichia coli. Appl. Catal. B: Environ. 192, 35–45. doi:10.1016/j.apcatb.2016.03.046
Wu, H., Yuan, C., Chen, R., Wang, J., Dong, F., Li, J., et al. (2020). Mechanisms of Interfacial Charge Transfer and Photocatalytic NO Oxidation on BiOBr/SnO2 P-N Heterojunctions. ACS Appl. Mater. Inter. 12, 43741–43749. doi:10.1021/acsami.0c12628
Wu, H., Zhou, P., Alagarasan, J. K., Jing, J., Zhou, T., and Xu, Y. (2021). Construction of Novel PTh-BiOBr Composite with Enhanced Photocatalytic Degradation of Bisphenol A. Adv. Powder. Technol. 32 (7), 2390–2397. doi:10.1016/j.apt.2021.05.022
Wu, X., Ng, Y. H., Wang, L., Du, Y., Dou, S. X., Amal, R., et al. (2017). Improving the Photo-Oxidative Capability of BiOBr via crystal Facet Engineering. J. Mater. Chem. A. 5, 8117–8124. doi:10.1039/c6ta10964k
Xia, J., Ji, M., Li, W., Di, J., Xu, H., He, M., et al. (2016). Synthesis of Erbium Ions Doped BiOBr via a Reactive Ionic Liquid with Improved Photocatalytic Activity. Colloids Surf. A: Physicochemical Eng. Aspects 489, 343–350. doi:10.1016/j.colsurfa.2015.10.037
Xiang, Y., Ju, P., Wang, Y., Sun, Y., Zhang, D., and Yu, J. (2016). Chemical Etching Preparation of the Bi2WO6/BiOI P-N Heterojunction with Enhanced Photocatalytic Antifouling Activity under Visible Light Irradiation. Chem. Eng. J. 288, 264–275. doi:10.1016/j.cej.2015.11.103
Xiao, W., Su, Y., Luo, J., Jiang, L., Wu, X., Liu, Z., et al. (2020). Flower-like Hierarchical Architecture of BiOI/ZnO P-N junction Composites with High-Efficient Visible-Light Photodegradation Activities. Solid State. Sci. 108, 106432. doi:10.1016/j.solidstatesciences.2020.106432
Xiao, X., and Zhang, W.-D. (2010). Facile Synthesis of Nanostructured BiOI Microspheres with High Visible Light-Induced Photocatalytic Activity. J. Mater. Chem. 20, 5866–5870. doi:10.1039/c0jm00333f
Xie, Y., Kocaefe, D., Chen, C., and Kocaefe, Y. (2016). Review of Research on Template Methods in Preparation of Nanomaterials. J. Nanomater. 2016, 1–10. doi:10.1155/2016/2302595
Xiong, J., Cheng, G., Lu, Z., Tang, J., Yu, X., and Chen, R. (2011a). BiOCOOH Hierarchical Nanostructures: Shape-Controlled Solvothermal Synthesis and Photocatalytic Degradation Performances. CrystEngComm 13, 2381–2390. doi:10.1039/c0ce00705f
Xiong, J., Cheng, G., Li, G., Qin, F., and Chen, R. (2011b). Well-crystallized Square-like 2D BiOCl Nanoplates: Mannitol-Assisted Hydrothermal Synthesis and Improved Visible-Light-Driven Photocatalytic Performance. RSC Adv. 1, 1542–1553. doi:10.1039/c1ra00335f
Xiong, J., Di, J., and Li, H. (2018). Atomically Thin 2D Multinary Nanosheets for Energy-Related Photo, Electrocatalysis. Adv. Sci. 5, 1800244. doi:10.1002/advs.201800244
Xiong, J., Song, P., Di, J., and Li, H. (2020). Bismuth-rich Bismuth Oxyhalides: a New Opportunity to Trigger High-Efficiency Photocatalysis. J. Mater. Chem. A. 8, 21434–21454. doi:10.1039/d0ta06044e
Xiong, X., Ding, L., Wang, Q., Li, Y., Jiang, Q., and Hu, J. (2016). Synthesis and Photocatalytic Activity of BiOBr Nanosheets with Tunable Exposed {0 1 0} Facets. Appl. Catal. B: Environ. 188, 283–291. doi:10.1016/j.apcatb.2016.02.018
Xue, X., Chen, R., Chen, H., Hu, Y., Ding, Q., Liu, Z., et al. (2018). Oxygen Vacancy Engineering Promoted Photocatalytic Ammonia Synthesis on Ultrathin Two-Dimensional Bismuth Oxybromide Nanosheets. Nano Lett. 18, 7372–7377. doi:10.1021/acs.nanolett.8b03655
Yadav, M., Garg, S., Chandra, A., Gläser, R., and Hernadi, K. (2020). Green BiOI Impregnated 2-dimensional Cylindrical Carbon Block: A Promising Solution for Environmental Remediation and Easy Recovery of the Photocatalyst. Separat. Purif. Techn. 240, 116628. doi:10.1016/j.seppur.2020.116628
Yadav, M., Garg, S., Chandra, A., and Hernadi, K. (2019). Immobilization of green BiOX (X= Cl, Br and I) Photocatalysts on Ceramic Fibers for Enhanced Photocatalytic Degradation of Recalcitrant Organic Pollutants and Efficient Regeneration Process. Ceramics Int. 45, 17715–17722. doi:10.1016/j.ceramint.2019.05.340
Yadav, M., Garg, S., Chandra, A., Jyoti, P. P., Ingole, P. P., Bardos, E., et al. (2021). Quercetin-mediated 3-D Hierarchical BiOI-Q and BiOI-Q-Ag Nanostructures with Enhanced Photodegradation Efficiency. J. Alloys Compd. 856, 156812. doi:10.1016/j.jallcom.2020.156812
Yan, X., Zhao, H., Li, T., Zhang, W., Liu, Q., Yuan, Y., et al. (2019). In Situ synthesis of BiOCl Nanosheets on Three-Dimensional Hierarchical Structures for Efficient Photocatalysis under Visible Light. Nanoscale 11, 10203–10208. doi:10.1039/c9nr02304f
Yang, H. (2021). A Short Review on Heterojunction Photocatalysts: Carrier Transfer Behavior and Photocatalytic Mechanisms. Mater. Res. Bull. 142, 111406. doi:10.1016/j.materresbull.2021.111406
Yang, J., Wang, X., Lv, X., Xu, X., Mi, Y., and Zhao, J. (2014). Preparation and Photocatalytic Activity of BiOX-TiO2 Composite Films (X=Cl, Br, I). Ceramics Int. 40, 8607–8611. doi:10.1016/j.ceramint.2014.01.077
Yang, L., Chen, J., Liu, X., Que, M., Zhao, Y., Zheng, H., et al. (2021). 2D/2D BiOBr/(001)-TiO2 Heterojunction toward Enhanced Photocatalytic Degradation Activity of Rhodamine B. J. Alloys Compd. 884, 161064. doi:10.1016/j.jallcom.2021.161064
Yang, W., Wen, Y., Chen, R., Zeng, D., and Shan, B. (2014). Study of Structural, Electronic and Optical Properties of Tungsten Doped Bismuth Oxychloride by DFT Calculations. Phys. Chem. Chem. Phys. 16, 21349–21355. doi:10.1039/c4cp02801e
Yang, Y., Zhang, C., Lai, C., Zeng, G., Huang, D., Cheng, M., et al. (2018). BiOX (X = Cl, Br, I) Photocatalytic Nanomaterials: Applications for Fuels and Environmental Management. Adv. Colloid Interf. Sci. 254, 76–93. doi:10.1016/j.cis.2018.03.004
Yao, S., Wang, J., Zhou, X., Zhou, S., Pu, X., and Li, W. (2020). One-pot Low-Temperature Synthesis of BiOX/TiO2 Hierarchical Composites of Adsorption Coupled with Photocatalysis for Quick Degradation of Colored and Colorless Organic Pollutants. Adv. Powder Techn. 31, 1924–1932. doi:10.1016/j.apt.2020.02.023
Yao, W., Wang, H., Xu, X. H., Zhou, J. T., Yang, X. N., Zhang, Y., et al. (2004). Photocatalytic Property of Bismuth Titanate Bi2Ti2O7. Appl. Catal. A: Gen. 259, 29–33. doi:10.1016/j.apcata.2003.09.004
Ye, L., Su, Y., Jin, X., Xie, H., and Zhang, C. (2014). Recent Advances in BiOX (X = Cl, Br and I) Photocatalysts: Synthesis, Modification, Facet Effects and Mechanisms. Environ. Sci. Nano 1, 90–112. doi:10.1039/c3en00098b
Ye, L., Tian, L., Peng, T., and Zan, L. (2011). Synthesis of Highly Symmetrical BiOI Single-crystal Nanosheets and Their {001} Facet-dependent Photoactivity. J. Mater. Chem. 21, 12479–12484. doi:10.1039/c1jm11005e
Yin, S., Fan, W., Di, J., Wu, T., Yan, J., He, M., et al. (2017). La3+ Doped BiOBr Microsphere with Enhanced Visible Light Photocatalytic Activity. Colloids Surf. A: Physicochemical Eng. Aspects 513, 160–167. doi:10.1016/j.colsurfa.2016.10.012
Yu, C.-L., Cao, F.-F., Shu, Q., Bao, Y.-L., Xie, Z.-P., Yu, J., et al. (2012). Preparation, Characterization and Photocatalytic Performance of Ag/BiOX (X= Cl, Br, I) Composite Photocatalysts. Acta Phys-chim. Sin. 28, 647–653. doi:10.3866/PKU.WHXB201201051
Yu, C., Cao, F., Li, G., Wei, R., Yu, J. C., Jin, R., et al. (2013). Novel noble Metal (Rh, Pd, Pt)/BiOX(Cl, Br, I) Composite Photocatalysts with Enhanced Photocatalytic Performance in Dye Degradation. Separat. Purif. Techn. 120, 110–122. doi:10.1016/j.seppur.2013.09.036
Yu, C., Fan, C., Meng, X., Yang, K., Cao, F., and Li, X. (2011). A Novel Ag/BiOBr Nanoplate Catalyst with High Photocatalytic Activity in the Decomposition of Dyes. Reac Kinet Mech. Cat 103, 141–151. doi:10.1007/s11144-011-0291-6
Yu, C., Zhou, W., Yu, J., Cao, F., and Li, X. (2012). Thermal Stability, Microstructure and Photocatalytic Activity of the Bismuth Oxybromide Photocatalyst. Chin. J. Chem. 30, 721–726. doi:10.1002/cjoc.201280018
Yu, H., Cao, C., Wang, X., and Yu, J. (2017). Ag-modified BiOCl Single-crystal Nanosheets: Dependence of Photocatalytic Performance on the Region-Selective Deposition of Ag Nanoparticles. J. Phys. Chem. C 121, 13191–13201. doi:10.1021/acs.jpcc.7b03213
Yu, H., Huang, B., Wang, H., Yuan, X., Jiang, L., Wu, Z., et al. (2018). Facile Construction of Novel Direct Solid-State Z-Scheme AgI/BiOBr Photocatalysts for Highly Effective Removal of Ciprofloxacin under Visible Light Exposure: Mineralization Efficiency and Mechanisms. J. Colloid Interf. Sci. 522, 82–94. doi:10.1016/j.jcis.2018.03.056
Yu, J., and Kudo, A. (2006). Effects of Structural Variation on the Photocatalytic Performance of Hydrothermally Synthesized BiVO4. Adv. Funct. Mater. 16, 2163–2169. doi:10.1002/adfm.200500799
Yu, J., Wang, W., and Cheng, B. (2010). Synthesis and Enhanced Photocatalytic Activity of a Hierarchical Porous Flowerlike P-N Junction NiO/TiO2 Photocatalyst. Chem. Asian J. 5, 2499–2506. doi:10.1002/asia.201000550
Zhang, C., and Zhu, Y. (2005). Synthesis of Square Bi2WO6 Nanoplates as High-Activity Visible-Light-Driven Photocatalysts. Chem. Mater. 17, 3537–3545. doi:10.1021/cm0501517
Zhang, D., Su, C., Yao, S., Li, H., Pu, X., and Geng, Y. (2020). Facile In Situ Chemical Transformation Synthesis, Boosted Charge Separation, and Increased Photocatalytic Activity of BiPO4/BiOCl P-N Heterojunction Photocatalysts under Simulated Sunlight Irradiation. J. Phys. Chem. Sol. 147, 109630. doi:10.1016/j.jpcs.2020.109630
Zhang, H., Tee, J. C. L., Jaenicke, S., Gondal, M. A., Dastageer, M. A., Basheer, C., et al. (2021). BiOBrnI1-n Solid Solutions as Versatile Photooxidation Catalysts for Phenolics and Endocrine Disrupting Chemicals. Catal. Today 375, 547–557. doi:10.1016/j.cattod.2020.01.009
Zhang, K., Liu, C., Huang, F., Zheng, C., and Wang, W. (2006). Study of the Electronic Structure and Photocatalytic Activity of the BiOCl Photocatalyst. Appl. Catal. B: Environ. 68, 125–129. doi:10.1016/j.apcatb.2006.08.002
Zhang, K., Zhang, D., Liu, J., Ren, K., Luo, H., Peng, Y., et al. (2012). A Novel Nanoreactor Framework of Iodine-Incorporated BiOCl Core-Shell Structure: Enhanced Light-Harvesting System for Photocatalysis. CrystEngComm 14, 700–707. doi:10.1039/c1ce05755c
Zhang, L.-W., Wang, Y.-J., Cheng, H.-Y., Yao, W.-Q., and Zhu, Y.-F. (2009). Synthesis of Porous Bi2WO6Thin Films as Efficient Visible-Light-Active Photocatalysts. Adv. Mater. 21, 1286–1290. doi:10.1002/adma.200801354
Zhang, L., Xu, T., Zhao, X., and Zhu, Y. (2010). Controllable Synthesis of Bi2MoO6 and Effect of Morphology and Variation in Local Structure on Photocatalytic Activities. Appl. Catal. B: Environ. 98, 138–146. doi:10.1016/j.apcatb.2010.05.022
Zhang, W., Fu, J., Wang, Y., Zhang, X., and Li, J. (2019). A Facile Solvothermal Method Synthesis of Nitrogen-Doped Graphene Quantum dots/BiOX (X=Br, Cl) Hybrid Material for Enhanced Visible-Light Photoactivity. Optik 176, 448–456. doi:10.1016/j.ijleo.2018.08.085
Zhang, W., Zhang, Q., and Dong, F. (2013). Visible-Light Photocatalytic Removal of NO in Air over BiOX (X = Cl, Br, I) Single-Crystal Nanoplates Prepared at Room Temperature. Ind. Eng. Chem. Res. 52, 6740–6746. doi:10.1021/ie400615f
Zhang, X., Ai, Z., Jia, F., and Zhang, L. (2008). Generalized One-Pot Synthesis, Characterization, and Photocatalytic Activity of Hierarchical BiOX (X = Cl, Br, I) Nanoplate Microspheres. J. Phys. Chem. C 112, 747–753. doi:10.1021/jp077471t
Zhang, Y., Sun, A., Xiong, M., Macharia, D. K., Liu, J., Chen, Z., et al. (2021). TiO2/BiOI P-N junction-decorated Carbon Fibers as Weavable Photocatalyst with UV-Vis Photoresponsive for Efficiently Degrading Various Pollutants. Chem. Eng. J. 415, 129019. doi:10.1016/j.cej.2021.129019
Zhang, Y., Wang, Q., Liu, D., Wang, Q., Li, T., and Wang, Z. (2020a). Cu2O-BiOI Isotype (P-p) Heterojunction: Boosted Visible-Light-Driven Photoelectrochemical Activity for Non-enzymatic H2O2 Sensing. Appl. Surf. Sci. 521, 146434. doi:10.1016/j.apsusc.2020.146434
Zhang, Y., Shao, Q., Jiang, H., Liu, L., Wu, M., Lin, J., et al. (2020b). One-step Co-precipitation Synthesis of Novel BiOCl/CeO2composites with Enhanced Photodegradation of Rhodamine B. Inorg. Chem. Front. 7, 1345–1361. doi:10.1039/c9qi01524h
Zhang, Z., Wang, B., Guo, J., He, Y., Song, P., and Wang, R. (2019). In Situ Synthesis of C-Doped BiOBr Micron‐Flower by Structural Induction of Sodium Alginate for Rapid Removal Tetracycline. ChemistrySelect 4, 14007–14011. doi:10.1002/slct.201903827
Zhao, H., Liu, X., Dong, Y., Xia, Y., Wang, H., and Zhu, X. (2020). Fabrication of a Z-Scheme {001}/{110} Facet Heterojunction in BiOCl to Promote Spatial Charge Separation. ACS Appl. Mater. Inter. 12, 31532–31541. doi:10.1021/acsami.0c08687
Zhao, K., Zhang, L., Wang, J., Li, Q., He, W., and Yin, J. J. (2013). Surface Structure-dependent Molecular Oxygen Activation of BiOCl Single-Crystalline Nanosheets. J. Am. Chem. Soc. 135, 15750–15753. doi:10.1021/ja4092903
Zhao, L., Zhang, X., Fan, C., Liang, Z., and Han, P. (2012). First-principles Study on the Structural, Electronic and Optical Properties of BiOX (X=Cl, Br, I) Crystals. Physica B: Condensed Matter 407, 3364–3370. doi:10.1016/j.physb.2012.04.039
Zhong, S., Wang, X., Wang, Y., Zhou, F., Li, J., Liang, S., et al. (2020). Preparation of Y3+-Doped BiOCl Photocatalyst and its Enhancing Effect on Degradation of Tetracycline Hydrochloride Wastewater. J. Alloys Compd. 843, 155598. doi:10.1016/j.jallcom.2020.155598
Zhou, J., Zhou, J., Hu, Z., and Wang, L. (2019). Enhancement of Adsorption and Visible Light Photocatalytic Activity of the Zn2+-Doped BiOBr/PVP Modified Microspheres for RhB. Mater. Sci. Semiconductor Process. 90, 112–119. doi:10.1016/j.mssp.2018.10.012
Zhou, S., Shi, T., Chen, Z., Kilin, D., Shui, L., Jin, M., et al. (2019). First-Principles Study of Optoelectronic Properties of the Noble Metal (Ag and Pd) Doped BiOX (X = F, Cl, Br, and I) Photocatalytic System. Catalysts 9, 198. doi:10.3390/catal9020198
Keywords: bismuth oxyhalide, photocatalysis, strategies for photoactivity enhancement, charge separation, wastewater remediation
Citation: Lv X, Lam FLY and Hu X (2022) A Review on Bismuth Oxyhalide (BiOX, X=Cl, Br, I) Based Photocatalysts for Wastewater Remediation. Front. Catal. 2:839072. doi: 10.3389/fctls.2022.839072
Received: 19 December 2021; Accepted: 10 March 2022;
Published: 06 April 2022.
Edited by:
Maria Olea, University of Cambridge, United KingdomReviewed by:
Jun Di, Nanjing University of Science and Technology, ChinaCopyright © 2022 Lv, Lam and Hu. This is an open-access article distributed under the terms of the Creative Commons Attribution License (CC BY). The use, distribution or reproduction in other forums is permitted, provided the original author(s) and the copyright owner(s) are credited and that the original publication in this journal is cited, in accordance with accepted academic practice. No use, distribution or reproduction is permitted which does not comply with these terms.
*Correspondence: Frank Leung Yuk Lam, a2VmcmFua0B1c3QuaGs=; Xijun Hu, a2V4aHVAdXN0Lmhr
Disclaimer: All claims expressed in this article are solely those of the authors and do not necessarily represent those of their affiliated organizations, or those of the publisher, the editors and the reviewers. Any product that may be evaluated in this article or claim that may be made by its manufacturer is not guaranteed or endorsed by the publisher.
Research integrity at Frontiers
Learn more about the work of our research integrity team to safeguard the quality of each article we publish.