- 1China University of Geosciences, School of Earth Sciences and Resources, Beijing, China
- 2School of Basic Education Department or Faculty, Beijing Polytechnic College, Beijing, China
The composite photocatalyst can be constructed by using natural porous minerals with a wide range of sources, low prices and stable chemical properties as the titanium dioxide catalyst carrier, which can not only reduce the cost of catalyst preparation and application, but also effectively improve the dispersion, recyclability and catalytic performance of the catalyst. In recent years, a large number of scientific researchers have conducted a lot of research on the preparation and performance of porous mineral-supported composite catalytic materials. This paper describes the catalytic mechanism of titanium dioxide, as well as the research and application progress of various nonmetallic mineral materials supported by titanium dioxide, and prospects the development trend in the future.
Introduction
With the rapid development of industry, environmental crises, which have blocked the further development of human civilization, is becoming more and more serious (Lin et al., 2019; Qiao et al., 2019; Xue et al., 2018). The use of photocatalytic technology in the advanced oxidation process is an effective method to solve the problem (Gaya and Abdullah 2007; Herrmann 1999). Photocatalytic technology evolves gradually developed in the 1970s. It is a technology that can convert solar energy into chemical energy to treat pollutants. In 1972, Fujishima and Honda discovered that titanium dioxide can split water to produce hydrogen when exposed to ultraviolet light (Fujishima and Honda 1972), which paved way for the research on semiconductor photocatalysis technology. Since then, semiconductor photocatalyst technology has been extensively researched, and it has been discovered that the technology can be used to split water for hydrogen evolution (Singh et al., 2014; Zhang et al., 2018; Li Shuang et al., 2021; Mohamed et al., 2021; Li Shuang et al., 2021), remove pollutants (Habibi et al., 2014; Frank and Bard 1977; Schrauzer and Guth 2002; Mastunaga 1985; Abdelraheem et al., 2019), reduce CO2(Li Kaining et al., 2021; Sun J et al., 2020; Yu et al., 2014; Low et al., 2017), Nitrogen Fixation (Wang et al., 2021; Wang et al., 2019; Wu et al., 2021). TiO2 photocatalysis technology has been demonstrated to be an effective method for treating organic pollutants in both air and water (Tsoukleris D S et al., 2007; Singh T et al., 2021). It has the advantages of low cost, stable chemical properties, appropriate conduction band valence band potential, no secondary pollution, and low photo-corrosion, making it a research hotspot in the field of photocatalysis (Yan et al., 2019; Ding et al., 2019; Prakash et al., 2018; Selcuk S et al., 2018). However, several problems limit the applications of pure TiO2, including prone to agglomeration, Low specific surface area, difficulty recycling, high photogenerated electron–hole recombination rate, and limited light utilization wavelength range (Lavanya et al., 2014). It often requires immobilized load and visible light modification to improve its application performance. The crystal structure and morphology of TiO2 are intended to improve the inherent optical properties, specific surface area, recycling problems, and charge transfer efficiencies, such as element doping (Akbarzadeh et al., 2010; Ranganathan et al., 2016), compound semiconductors (Székely et al., 2021; Ding et al., 2019; Prakash et al., 2018; Selcuk et al., 2018), Nobel-metal deposition (Sato and White 1980; Gianmario 2000), and loading cocatalysts (Li Kaining et al., 2021; Ridhwan Samsudin et al., 2018). Among them, there are numerous types of research on loading cocatalysts, and the industrial application of photocatalysts can be realized by modifying the load.
Based on the rapid development of TiO2, we believe that it is necessary to summarize the carrier of TiO2 photocatalyst and point out its development trend through further review. This article examines the most recent advances in titanium dioxide photocatalyst supports research and introduces the role of various nonmetallic mineral carriers. Furthermore, the photocatalytic performance improvement strategy and the use of titanium dioxide photocatalyst are summarized. Furthermore, we also discussed the current challenges facing TiO2-based composites as well as key issues that need to be resolved in future research. We hope that this review will provide a better understanding of TiO2 and help solve its problems.
Principle of TiO2 Photocatalysis
TiO2 is an N-type semiconductor, and there are three main crystal structures: Anatase TiO2, Rutile TiO2, and Brookite TiO2 (Hoffmann et al., 1995). The three crystal types are allotropes with each other, the most fundamental crystal structure unit is the same, composed of titanium oxide octahedron [TiO6], and the crystal structure of the three crystals is shown in Figure 1 (Pelaez et al., 2012). When compared to the rutile phase, anatase phase TiO2 demonstrated greater photocatalytic activity for the degradation of all substances (Lavanya et al., 2014). The higher photocatalytic activity in anatase TiO2 is attributed to parameters such as the band-gap, number of hydroxyl groups, surface area, and porosity of the catalyst (Tayade et al., 2007). Anatase titanium dioxide has a strong ability to adsorb and capture oxygen, and has a large specific surface area. Its electrons and holes are difficult to recombine, so its activity is high. Meanwhile, studies have shown that the particle size of titanium dioxide has a decisive effect on the stability of the crystal phase (Song et al., 2021; Lee et al., 2019; Gong and Selloni 2005).
When TiO2 is illuminated with an energy greater than or equal to its forbidden bandwidth (for anatase TiO2, it must receive light with a wavelength less than 387.5 nm, limiting the use of photocatalysts), the electrons on the valence band are excited and undergo transition. When transferred to the conduction band, the photogenerated electron (e−)-hole (h+) pairs are separated, and such photogenerated electron (e−)-hole (h+) pairs are collectively called carriers. One of these photogenerated carriers will recombine within a few picoseconds (ps), while the other will migrate to the surface of TiO2 within a few nanoseconds (ns) to immediately undergo redox reactions and react with oxygen and water to form highly active oxides (Tachikawa et al., 2007), oxidize substances that do not react with light into hydroxyl radicals (·OH), while photogenerated electrons will generate strong reducing superoxide radicals (·O2−) in the redox reaction. (·OH) has strong oxidizing properties, which can destroy carbon-carbon bonds (C—C), carbon-hydrogen bonds (C—H), carbon-nitrogen bonds (C—N), and carbon-oxygen bonds (C—O) in organic matter. Nitrogen-hydrogen bonds (N-H), for example, eventually degrade organic pollutants into CO2, H2O, and other simple inorganic substances that can be emitted (Gaya and Abdullah, 2008). Because of the presence of hydroxyl radicals and superoxide radicals, the general redox reaction produces intermediate products but does not stop there, resulting in no intermediate products remaining in the reaction. The reaction mechanism of titanium dioxide photocatalyst is shown in Figure 2 (Pelaez et al., 2012).
The specific reaction equation of the titanium dioxide photocatalyst is:
When TiO2 is exposed to light with a wavelength of less than 387.5 nm, it generates photo-generated electrons and holes:
Superoxide radicals (·O2−) and hydroxyl radicals (·OH) are generated on the conduction band and valence band respectively:
The generated superoxide radicals (·O2−) and hydroxyl radicals (·OH) will react with O2, H2O and other groups to produce strong oxidizing free radicals (·OOH) and H2O2:
These radical groups with strong redox properties can indiscriminately oxidize the pollutants adsorbed on the surface of titanium dioxide into non-toxic water, carbon dioxide and other simple inorganic substances:
The method of preparing TiO2 can be roughly divided into three methods: gas phase method, liquid phase method, and solid phase method, including sol-gel method (Dikici et al., 2018; Shimelis et al., 2017), hydrolysis precipitation method (Zhu et al., 2019), hydrothermal method, immersion method, mechanochemical method, microemulsion method, Magnetron sputtering method, etc.
Non-Metallic Minerals Supported TiO2
In the process of using TiO2 photocatalyst, to improve the photocatalytic activity, the photocatalyst is often made into nano-powder to increase the specific surface area of the powder and increase the number of photocatalytic active sites (Shi et al., 2009). When powder TiO2 is used directly as a functional material for water treatment, however, defects such as easy agglomeration and recovery difficulty limit its application in advanced wastewater treatment (Kumar and Devi 2011). Rong Wang et al. discovered that when nano-TiO2 comes into contact with water, it will produce a highly hydrophilic and lipophilic surface when exposed to ultraviolet light, and the contact angle quickly approaches 0°(Wang et al., 1997).
According to research, the grain size of titanium dioxide has a direct impact on its photocatalytic performance (Song et al., 2021; Lee et al., 2019). The photocatalytic degradation rate of anatase TiO2 nanoparticles increased significantly when the grain size was increased from 6.6 to 26.6 nm (Wang et al., 2014). The photo-reactivity was found to increase with increasing crystallite size but to be independent of the crystallinity. The shape of the primary particles can also have a significant impact on titania nanoparticles’ photocatalytic activity. Compared to spherical, cubic or hexagonal shapes have better photocatalytic activity. (Scotti et al., 2009). After TiO2 is loaded on a suitable carrier, it can avoid the agglomeration of nano-TiO2 particles, and solve the problem of difficulty in separating titanium dioxide from liquid, and will not cause secondary pollution. Simultaneously, the loaded TiO2 can achieve a larger specific surface area and adsorption performance, realize pollutant enrichment on the carrier’s surface, and increase the possibility of contact between the photocatalyst and organic pollutants, thereby improving TiO2’s photocatalytic efficiency. As a photocatalytic carrier, non-metallic minerals have the following advantages: wide sources, low price, stable chemical properties, and natural porous structure. After loading photocatalyst, it can not only reduce the cost of preparation and application of composite photocatalyst, but also improve the dispersion, recoverability and catalytic performance of photocatalyst. Teh C M’s research shows that a higher specific surface area can promote the adsorption of more reactant molecules to the surface of the catalyst, thereby avoiding the recombination of photogenerated electron–hole pairs (Teh and Mohamed 2011). Table 1 shows the research progress of TiO2/non-metallic mineral composite photocatalytic materials in the treatment of pollutants. (Unless otherwise specified, it is the contaminants in the treatment solution).
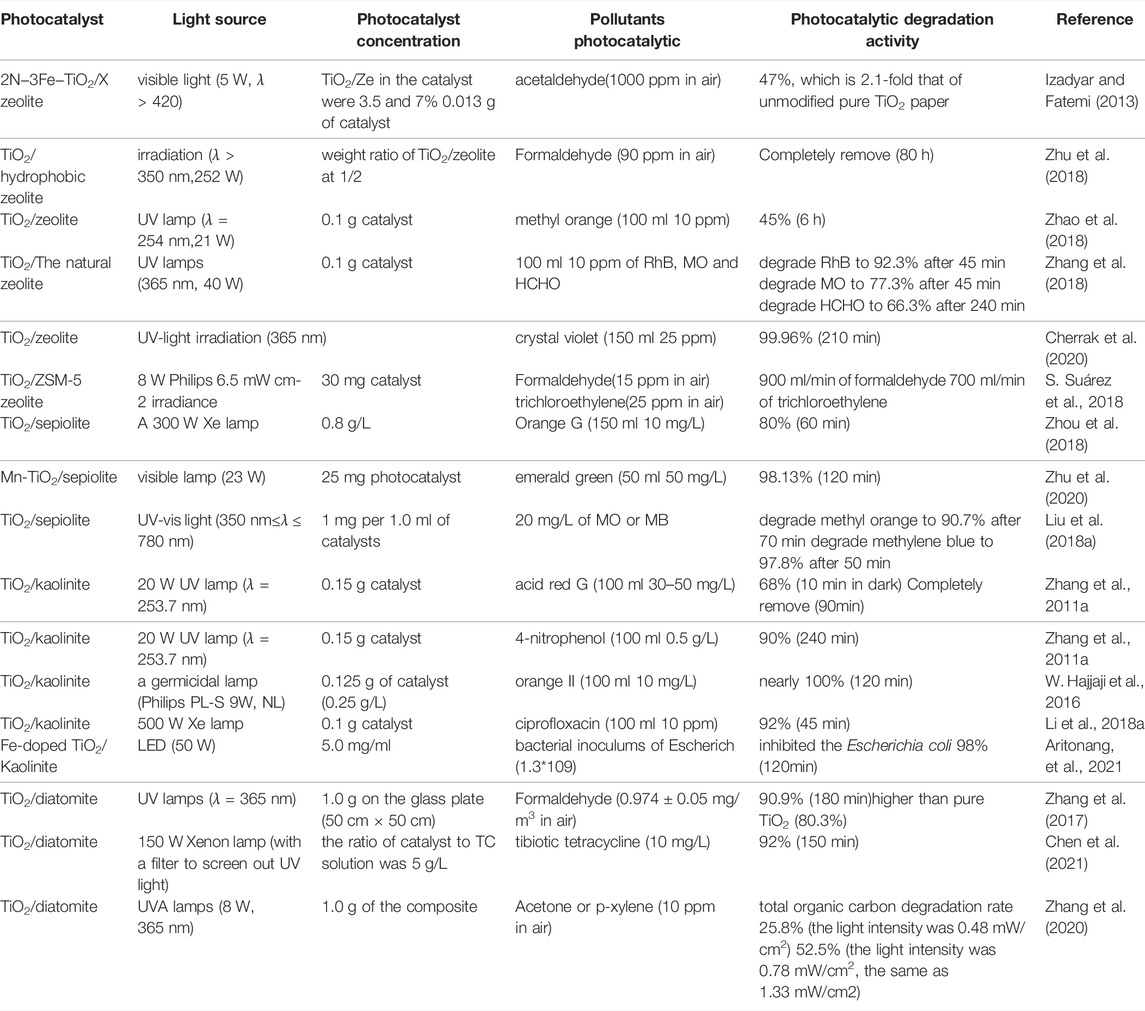
TABLE 1. Research progress of TiO2/non-metallic mineral composite photocatalytic materials in the treatment of pollutant.
Layered Silicate Mineral Kaolinite Supported Nano-TiO2
Kaolin is a kind of aluminum-containing layered silicate mineral. Its structural unit layer is made up of a 1:1 structural unit layer of silicon-oxygen tetrahedral sheets and aluminum-oxygen octahedral sheets (Vimonses et al.,2010). The calcination of kaolin is a dehydroxylation and aluminum activation process, and the crystal structure changes from layered kaolinite to amorphous metakaolinite at the same time. Kaolinite as a TiO2 photocatalyst carrier can improve the agglomeration of nano-TiO2 particles on the one hand, and realize the uniform loading of nano-TiO2 particles on the surface of kaolinite (Li CQ, 2018). On the other hand, the highly temporary surface is densely concentrated with negatively charged hydroxyl groups, which can promote carrier separation and improve quantum efficiency. Figure 3 shows the diagram description of the photocatalytic degradation enhancement mechanism of TiO2/kaolinite composite (Li et al., 2018b).
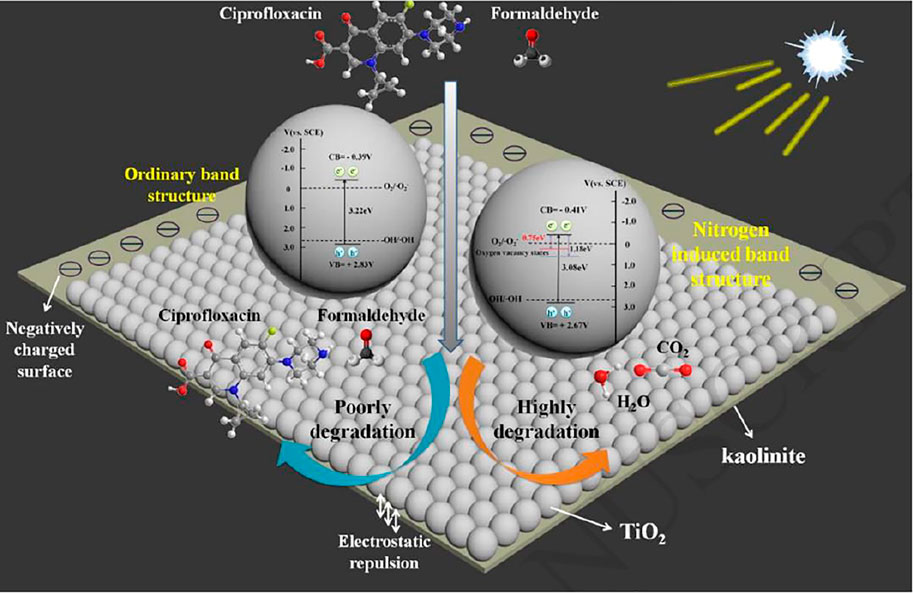
FIGURE 3. The diagram description of the photocatalytic degradation enhancement mechanism of TiO2/kaolinite composite (Li et al.,2018b).
Hongliang Xu et al. prepared the kaolinite/TiO2 composite material by the sol-gel method. The results show that intercalation and exfoliation reduce the size and thickness of the kaolinite particles. The acid treatment improves the distribution and loading of TiO2 crystal grains. Both acid treatment and kaolinite exfoliation improved the photocatalytic performance of the kaolinite/TiO2 composite photocatalyst, but the acid treatment is more conducive to the preparation of high performance kaolinite/TiO2 composite photocatalyst than exfoliation (Xu et al.,2018). Zhang Y et al. generated TiO2/Kaolin composite photocatalytic material with mixed crystal composition, and the specific surface area of the composite material reached 113.5 m2/g, demonstrating excellent removal effect on acid red G and p-nitrophenol under UV irradiation (Zhang et al., 2011a). Kibanova D et al. prepared TiO2/kaolin and TiO2/montmorillonite composite photocatalysts, respectively, to study the removal effect of gaseous formaldehyde under different light sources and humidity conditions, and compared them to Degussa P25 photocatalyst. It was found that the removal rate under UVA (365 nm) irradiation was not as high as that under UVC irradiation. When the humidity is 10% in the same light source, the removal effect of pollutants is optimal, but too much humidity results in a lower removal rate (Kibanova et al., 2009). Wang C et al. formed a TiO2/Kaolin nano-photocatalyst via titanium tetrachloride hydrolysis. The experimental results show that kaolin promotes anatase formation while inhibiting anatase transformation to rutile. Ti-o-si bond is formed in the composite material. The sample calcined at 200°C has the smallest grain size and an abundance of oxygen vacancies and defects on the surface, which can significantly reduce the composite efficiency of electron–hole pairs (Wang et al., 2011). Tokarský J et al. used hydrolytic precipitation to construct a TiO2/kaolinite composite photocatalytic material from titanyl sulfate. Kaolinite could be converted into metakaolinite by forging and burning at 600°C, and TiO2 nanoparticles on the surface of kaolinite were found to be in order by simulation with the empirical atomic field. As the target pollutant, acid orange 7 aqueous solutions were used. It degrades effectively. After urea intercalation and heat treatment (Tokarský et al., 2012). Matějka V et al. produced flake kaolinite. After the kaolinite was peeled off, the specific surface area increased from 14 to 24 m2/g. TiO2 particles were immobilized on the surface of kaolinite by titanyl sulfate was used as a titanium source, and azo dye acid orange 7 was desorbed under UV light. It is found that the composite was discovered to have high photocatalytic activity (Matějka and Neuwirthová 2014). Kočí K et al. prepared TiO2/kaolinite composite by hydrolyzing precipitation of titanyl sulfate. When applied to the photocatalytic reduction of CO2, it was discovered that the composite produced more methane and methanol than commercial P25 (Kočí et al., 2011). Zhang Y et al. prepared TiO2/Kaolinite composite by low-temperature hydrolysis precipitation method. Anatase and rutile phases, titanite and rutile phases, and a mixed phase of anatase, titanite, and rutile coexisted in the TiO2/Kaolinite composite. At 700°C, the composite degraded acid red G and p-nitrophenol immensely well (Zhang et al., 2011a).
Chain Silicate Mineral Sepiolite Loaded With TiO2
Sepiolite is a kind of magnesium-containing porous chain silicate mineral. It has a chain-like and layered transition-type structure in its crystal structure. Two layers of silicon-oxygen tetrahedra are joined by magnesia octahedra. Because of its abundant acid-base center, large specific surface area, and high mechanical strength, sepiolite is widely used in catalysis (De la Caillerie and Fripiat 1992). Figure 4 shows the catalytic degradation mechanism diagram of TiO2/sepiolite composite material (Liu et al., 2018a).
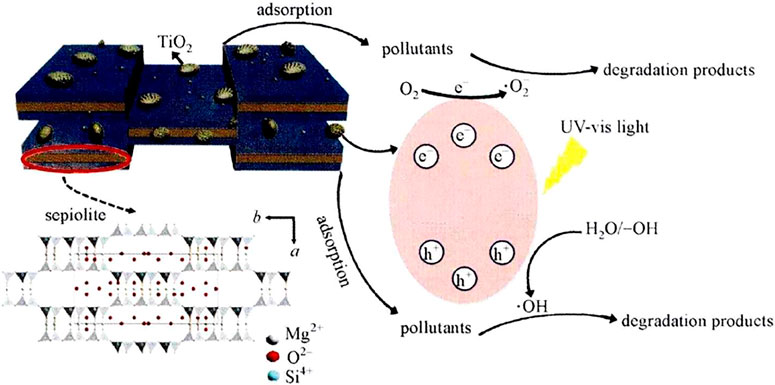
FIGURE 4. Catalytic degradation mechanism diagram of TiO2/sepiolite composite material (Liu R. et al., 2018)
Li et al. prepared a magnetic TiO2/sepiolite photocatalytic composite material by the sol-gel method, which has an outstanding synergistic degradation effect on pentavalent chromium ions and dichlorophenol (Li et al., 2015). Zhang et al. synthesized a kind of TiO2 supported acid-activated sepiolite fibers under low-temperature conditions. They discovered that the specific surface area of the composite material was significantly greater than that of the original sepiolite and that the photocatalytic activity of the TiO2/AAS composites is excellent and superior to that of the TiO2/sepiolite (raw sepiolite) and pure TiO2. The addition of sepiolite significantly improves the photocatalytic activity of titanium dioxide (Zhang et al., 2014; Zhang et al., 2011b).
Uğurlu M et al. synthesized a TiO2/sepiolite composite photocatalyst using sepiolite as a carrier, which has a good photocatalytic effect on phenol and lignin and investigated the pH value of the solution. The effect of the amount of catalyst and temperature on photocatalytic performance. The findings of this study show that using sepiolite as a carrier can raise the crystal transition temperature of TiO2 and inhibit crystal grain growth and agglomeration, and that sepiolite adsorption can promote the deep progress of a photocatalytic reaction. Zhang et al. used titanium tetrachloride as the titanium source and prepared an Au-doped nano-TiO2/sepiolite composite photocatalytic powder material by the sol-gel method. Methyl orange and Congo red have good degradation properties (Uğurlu and Karaoğlu 2011). Zhang D et al. used the microwave hydrothermal method to load TiO2 onto the surface of sepiolite fiber, with the anionic dye orange G serving as the target degradation product. The catalytic results revealed that the obtained composite material has a high specific surface area. It is distributed on the surface and has a good degradation effect on orange G (Zhang 2014).
Rack-Shaped Silicate Mineral Zeolite Loaded With TiO2
A zeolite is a group of water-containing alkali metal and alkaline earth metal framework aluminosilicate minerals. The zeolite crystal lattice contains a large number of uniformly sized pores and channels. The pores are connected by open channels that allow them to communicate with the outside world. At the same time, these channels are easily occupied by Na, Ca, K, and other cations and water molecules. Among all TiO2 supporting carriers, the zeolite molecular sieve has the largest specific surface area, the most irregular inner surface layout, the highest chemical stability, the best particle exchange performance, and the ability to provide a unique nano-scale reaction field. It is widely used in the support of TiO2 photocatalysts. The supported zeolite molecular sieve can enrich the low-dose organic substrates in the liquid system on the surface of the catalyst and catalyze the concentrated catalysis, and increase the degradation rate of organic pollutants (Su et al., 2017; Yener et al., 2017).
Liu X et al. used a sol-gel method to generate a TiO2/zeolite (TiO2 loaded amount of 30%) composite material and investigated its adsorption and photocatalytic degradation performance for sulfadiazine (SDZ) under ultraviolet light irradiation. The results show that under neutral pH conditions, the TiO2/zeolite sample can remove % of SDZ in 120 min. It is because the synergistic effect of TiO2 photocatalyst and zeolite adsorbent in the composite material accelerates the photodegradation rate of SDZ in water. Figure 5 shows the catalytic degradation mechanism of TiO2/zeolite composite material (Liu X. et al., 2018).
Similarly, Kovalevskiy N S et al. studied the photocatalytic degradation efficiency of TiO2/zeolite composite materials for various VOCs was investigated, and it was discovered that TiO2/zeolite composite materials can inhibit secondary pollution and improve air purification efficiency when compared to pure TiO2 (Kovalevskiy et al., 2018). Zhang G et al. prepared a TiO2/zeolite composite material by a hydrolysis deposition method and chose formaldehyde and organic dyes in water (phenol, MO, and RhB) as target degradation products to study the material’s photocatalytic activity. The findings show that, when compared to pure TiO2, the TiO2/zeolite composite material has higher photocatalytic degradation activity and a higher degradation efficiency for organic pollutants (Zhang et al.,2018).
There are many types of zeolite molecular sieves used to support TiO2 photocatalysts. Different types of zeolite molecular sieves have different structures and properties, and their effects on TiO2 photocatalytic activity differ significantly. For example, X-type zeolite has a higher static adsorption capacity for CO2 gas than A-type zeolite,X-type zeolite is more suitable as a photocatalyst carrier because its ion exchange capacity is greater than that of Y-type zeolite. Different types of zeolite molecular sieve-supported TiO2 exhibit higher photocatalytic activity when compared to pure TiO2 powder. Wang J J et al. also reached the same conclusion as mentioned above (Wang et al., 2015).
Biogenic Sedimentary Rock Diatomite Loaded With TiO2
Diatomite with consists of amorphous silica (SiO2. nH2O) essentially derived from the skeletons of ancient diatoms. It is a biogenic siliceous sedimentary rock with a large surface area, high porosity, high permeability, small particle size, low density, thermal resistance, and chemical stability. Their surface contains numerous (Si─OH) silanol groups, which can form hydrogen bonds with a variety of contaminants. Diatomite could serve as an effective support material for nano titanium dioxide particles. Figure 6 shows a schematic diagram of the surface structure of diatomite (Cherrak et al., 2020).
Zhang G et al. used hydrolysis and deposition to create a TiO2/diatomite composite photocatalyst. The TiO2/diatomite composite material demonstrated higher photocatalytic activity than pure TiO2, demonstrating that Nano TiO2 particles have stronger formaldehyde adsorption capacity and good dispersibility after the addition of diatomite (Zhang et al., 2017). Zhang J prepared a TiO2/diatomite photocatalyst by hydrolytic deposition method using titanium tetrachloride as a precursor. It has been demonstrated that adding a small amount of sulfate ion promotes the formation of the anatase phase and inhibits the transformation from anatase to rutile (Gao and Zhang 2001; Zhang et al., 2016). At the same time, the sulfate ions immobilized on the surface of TiO2/diatomite have a strong affinity for electrons, capturing the photogenerated electrons, preventing electron–holes recombination (Zhang et al., 2016).
Carbon-Containing Mineral Black Talc Loaded With TiO2
As a kind of layered silicate mineral, black talc is widely used. Compared with other silicate minerals, black talc has good surface affinity, chemical stability, and thermal stability. Meanwhile, there is a carbon layer between the black talc layers and before the talc crystal grains that has a high capacity for organic pollutant adsorption. It can adsorb organic pollutants around the black talc, increasing the concentration of pollutants near the minerals and thus increasing the photocatalyst’s catalytic efficiency. The author prepares a titanium dioxide/black talc composite photocatalyst using black talc as a carrier, implements the indirect light degradation method, and completely exploits the adsorption-degradation coupling of black talc, which greatly reduces the time of light. Figure 7 shows schematic diagram of photocatalytic performance enhancement mechanism of TiO2/Black Talc composite material (Shuai et al., 2021).
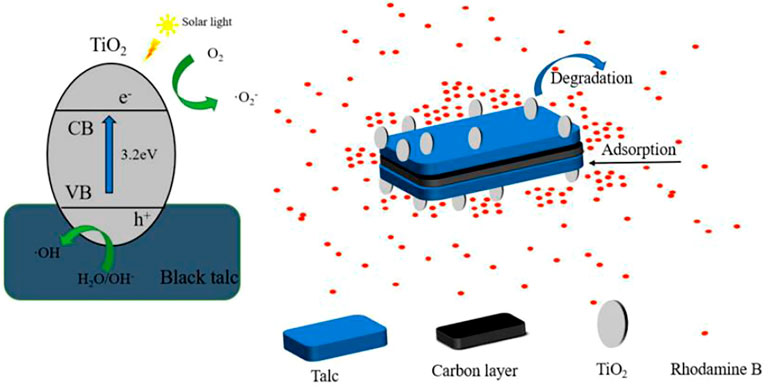
FIGURE 7. Schematic diagram of photocatalytic performance enhancement mechanism of TiO2/Black Talc composite material (Shuai et al., 2021).
Conclusion and Outlook
Nano-TiO2 composite catalyst supported by nonmetallic minerals combines the adsorption and capture function of mineral materials with the photocatalytic degradation function of nano-TiO2, which not only addresses the issues that pure nano-TiO2 is difficult to use and recycle but also improves the photocatalytic performance of nano-TiO2 materials and promotes the practical application of nano-TiO2 materials. In addition, the composite material also opens up a new way for the efficient utilization of silicate nonmetallic minerals. Nano-TiO2 composite catalysts supported by nonmetallic minerals are expected to have a successful potential application.
However, the current mineral material loaded TiO2 photocatalyst still has challenges to be solved:
1) The photocatalyst is still in the laboratory stage and is often treated for a single pollutant, which makes it difficult for materials to deal with pollutants in complex systems. Therefore, it is necessary to further build a mineral adsorption degradation synergistic catalytic system to prepare porous mineral supported composite catalytic materials with better performance.
2) There is still a certain gap between laboratory preparation and industrial production, and it is necessary to solve the problems of technology, equipment and production efficiency in the industrial production process.
3) The conversion efficiency of photocatalytic materials to visible light is relatively low, and the mechanism of action on mineral carriers is not clear enough. It is necessary to borrow more advanced analysis and testing methods to study its compounding principle and improve the utilization rate of light.
Author Contributions
XY: conceptualization, methodology, data curation, and writing ‐ original draft preparation. JW: conceptualization, investigation, methodology, and funding acquisition. FR and GD:conceptualization, writing ‐ review and editing, supervision. HS: conceptualization, investigation, and methodology. All authors contributed to the article and approved the submitted version.
Conflict of Interest
The authors declare that the research was conducted in the absence of any commercial or financial relationships that could be construed as a potential conflict of interest.
Publisher’s Note
All claims expressed in this article are solely those of the authors and do not necessarily represent those of their affiliated organizations, or those of the publisher, the editors and the reviewers. Any product that may be evaluated in this article, or claim that may be made by its manufacturer, is not guaranteed or endorsed by the publisher.
References
Abdelraheem, W. H. M., Patil, M. K., Nadagouda, M. N., and Dionysiou, D. D. (2019). Hydrothermal Synthesis of Photoactive Nitrogen- and boron- Codoped TiO2 Nanoparticles for the Treatment of Bisphenol A in Wastewater: Synthesis, Photocatalytic Activity, Degradation Byproducts and Reaction Pathways. Appl. Catal. B: Environ. 241, 598–611. doi:10.1016/j.apcatb.2018.09.039
Akbarzadeh, R., Umbarkar, S. B., and Sonawane, R. S. (2010). Vanadia–titania Thin Films for Photocatalytic Degradation of Formaldehyde in Sunlight[J]. Appl. Catal. A: Gen. 374 (1-2), 103–109. doi:10.1016/j.apcata.2009.11.035
Aritonang, A. B., Pratiwi, E., Warsidah, W., Nurdiansyah, S. I., and Risko, R. (2021). Fe-doped TiO2/Kaolinite as an Antibacterial Photocatalyst under Visible Light Irradiation. Bull. Chem. React. Eng. Catal. 16 (2), 293–301. doi:10.9767/bcrec.16.2.10325.293-301
Chen, Y., Wu, Q., Liu, L., Wang, J., and Song, Y. (2019). The Fabrication of Self-Floating Ti3+/N Co-doped TiO2/diatomite Granule Catalyst with Enhanced Photocatalytic Performance under Visible Light Irradiation. Appl. Surf. Sci. 467-468, 514–525. doi:10.1016/j.apsusc.2018.10.146
Cherrak, R., Hadjel, M., Benderdouche, N., Adjdir, Mi., Mokhtar, A., Khaldi, Ka., et al. (2020). Weidler. Preparation of Nano-TiO2/Diatomite Composites by Non-hydrolytic Sol–Gel Process and its Application in Photocatalytic Degradation of Crystal Violet. J. Silicon 12 (1–2), 6. doi:10.1007/s12633-019-00186-6
De la Caillerie, J.-B. d. E., and Fripiat, J. J. (1992). AL Modified Sepiolite as Catalyst or Catalyst Support. Catal. Today 14 (2), 125–140. doi:10.1016/0920-5861(92)80017-h
Dikici, B., Niinomi, M., Topuz, M., Say, Y., Aksakal, B., Yilmazer, H., et al. (2018). Synthesis and Characterization of Hydroxyapatite/TiO2 Coatings on the β-Type Titanium Alloys with Different Sintering Parameters Using Sol-Gel Method. Prot. Met. Phys. Chem. Surf. 54 (3), 457–462. doi:10.1134/s2070205118030255
Ding, X., Wang, W., Zhang, A., Zhang, L., and Yu, D. (2019). Efficient Visible Light Degradation of Dyes in Wastewater by Nickel-Phosphorus Plating-Titanium Dioxide Complex Electroless Plating Fabric. J. Mater. Res. 34 (6), 999–1010. doi:10.1557/jmr.2019.16
Fakhrul Ridhwan Samsudin, M., Sufian, S., Bashiri, R., Muti Mohamed, N., Tau Siang, L., and Mahirah Ramli, R. (2018). Optimization of Photodegradation of Methylene Blue over Modified TiO2/BiVo4 Photocatalysts: Effects of Total TiO2 Loading and Different Type of Co-catalyst. Mater. Today Proc. 5 (10), 21710–21717. doi:10.1016/j.matpr.2018.07.023
Frank, S. N., and Bard, A. J. (1977). Heterogeneous Photocatalytic Oxidation of Cyanide Ion in Aqueous Solutions at Titanium Dioxide Powder. J. Am. Chem. Soc. 99 (1), 303–304. doi:10.1021/ja00443a081
Fujishima, A., and Honda, K. (1972). Electrochemical Photolysis of Water at a Semiconductor Electrode. Nature 238 (5358), 37–38. doi:10.1038/238037a0
Gao, L., and Zhang, Q. (2001). The Promoting Effect of Sulfate Ions on the Nucleation of TiO2 (Anatase) Nanocrystals. Mater. Trans. 42 (8), 1676–1680. doi:10.2320/matertrans.42.1676
Gaya, U. I., and Abdullah, A. H. (2008). Heterogeneous Photocatalytic Degradation of Organic Contaminants over Titanium Dioxide: A Review of Fundamentals, Progress and Problems. J. Photochem. Photobiol. C: Photochem. Rev. 9 (1), 1–12. doi:10.1016/j.jphotochemrev.2007.12.003
Gaya, U. I., and Abdullah, A. H. (2007). Heterogeneous Photocatalytic Degradation of Organic Contaminants over Titanium Dioxide: A Review of Fundamentals, Progress and Problems[J]. J. Photochem. Photobiol. C: Photochem. Rev. 9 (1), 1–12.
Gianmario, M. (2000). Base Sites at the Surface of Microcrystalline TiO2 Anatase: Relationships between Surface Morphology and Chemical Behavior[J]. Appl. Catal. A 200 (2), 275
Gong, X.-Q., and Selloni, A. (2005). Reactivity of Anatase TiO2 Nanoparticles: The Role of the Minority (001) Surface. J. Phys. Chem. B 109 (42), 19560–19562. doi:10.1021/jp055311g
Habibi, M. H., Nasr-Esfahani, M., and Egerton, T. A. (2014). Photochemical Characterization and Photocatalytic Properties of a Nanostructure Composite TiO2 Film[J]. Int. J. Photoenergy 2007 (10), 136–151. doi:10.1155/2007/13653
Hajjaji, W., Andrejkovičová, S., Pullar, R. C., Tobaldi, D. M., Lopez-Galindo, A., Jammousi, F., et al. (2016). Effective Removal of Anionic and Cationic Dyes by Kaolinite and TiO2/kaolinite Composites. Clay miner. 51 (1), 19–27. doi:10.1180/claymin.2016.051.1.02
Herrmann, J.-M. (1999). Heterogeneous Photocatalysis: Fundamentals and Applications to the Removal of Various Types of Aqueous Pollutants. Catal. Today 53 (1), 115–129. doi:10.1016/s0920-5861(99)00107-8
Hoffmann, M. R., Martin, S. T., Choi, W., and Bahnemann, D. W. (1995). Environmental Applications of Semiconductor Photocatalysis. Chem. Rev. 95 (1), 69–96. doi:10.1021/cr00033a004
Izadyar, S., and Fatemi, S. (2013). Fabrication of X Zeolite Based Modified Nano TiO2 Photocatalytic Paper for Removal of VOC Pollutants under Visible Light. Ind. Eng. Chem. Res. 52 (32), 10961–10968. doi:10.1021/ie400416e
Kibanova, D., Cervini-Silva, J., and Destaillats, H. (2009). Efficiency of Clay−TiO2 Nanocomposites on the Photocatalytic Elimination of a Model Hydrophobic Air Pollutant. Environ. Sci. Technol. 43 (5), 1500–1506. doi:10.1021/es803032t
Kočí, K., Matějka, V., and Kovář, P. (2011). Comparison of the Pure TiO2 and kaolinite/TiO2 Composite as Catalyst for CO2 Photocatalytic Reduction[J]. Catal. Today 161 (1), 105. doi:10.1016/j.cattod.2010.08.026
Kovalevskiy, N. S., Lyulyukin, M. N., Selishchev, D. S., and Kozlov, D. V. (2018). Analysis of Air Photocatalytic Purification Using a Total hazard index: Effect of the Composite TiO2/zeolite Photocatalyst. J. Hazard. Mater. 358, 302–309. doi:10.1016/j.jhazmat.2018.06.035
Kumar, S. G., and Devi, L. G. (2011). Review on Modified TiO2Photocatalysis under UV/Visible Light: Selected Results and Related Mechanisms on Interfacial Charge Carrier Transfer Dynamics. J. Phys. Chem. A. 115, 13211–13241. doi:10.1021/jp204364a
Lavanya, T., Satheesh, K., Dutta, M., Victor Jaya, N., and Fukata, N. (2014). Superior Photocatalytic Performance of Reduced Graphene Oxide Wrapped Electrospun Anatase Mesoporous TiO 2 Nanofibers. J. alloys Compd. 615, 643–650. doi:10.1016/j.jallcom.2014.05.088
Lee, D. G., Kim, M.-c., Kim, B. J., Kim, D. H., Lee, S. M., Choi, M., et al. (2019). Effect of TiO2 Particle Size and Layer Thickness on Mesoscopic Perovskite Solar Cells. Appl. Surf. Sci. 477, 131–136. doi:10.1016/j.apsusc.2017.11.124
Li, C., Sun, Z., Song, A., Dong, X., Zheng, S., Dionysiou, D. D., et al. (2018a). Flowing Nitrogen Atmosphere Induced Rich Oxygen Vacancies Overspread the Surface of TiO2/kaolinite Composite for Enhanced Photocatalytic Activity within Broad Radiation Spectrum. Appl. Catal. B: Environ. 236, 76–87. doi:10.1016/j.apcatb.2018.04.083
Li, C., Sun, Z., Zhang, W., Yu, C., and Zheng, S. (2018b). Highly Efficient G-C3N4/TiO2/kaolinite Composite with Novel Three-Dimensional Structure and Enhanced Visible Light Responding Ability towards Ciprofloxacin and S. aureus. Appl. Catal. B: Environ. 220, 272–282. doi:10.1016/j.apcatb.2017.08.044
Li, F., Dai, Y., Gong, M., Yu, T., and Chen, X. (2015). Synthesis, Characterization of Magnetic-Sepiolite Supported with TiO2, and the Photocatalytic Performance over Cr(VI) and 2,4-dichlorophenol Co-existed Wastewater. J. Alloys Compd. 638, 435–442. doi:10.1016/j.jallcom.2015.03.070
Li, J., Li, J., Liu, X., Chen, J., Tian, P., Dai, S., et al. (2021). Probing the Role of Surface Hydroxyls for Bi, Sn and in Catalysts during CO2 Reduction. Appl. Catal. B: Environ. 298, 120581. doi:10.1016/j.apcatb.2021.120581
Li, X., Yu, J., Jaroniec, M., and Chen, X. (2019). Cocatalysts for Selective Photoreduction of CO2 into Solar Fuels. Chem. Rev. 119 (6), 3962–4179. doi:10.1021/acs.chemrev.8b00400
Li, Kaining, K., Zhang, S., Li, Y., Fan, J., and Lv, K. (2021). MXenes as noble-metal-alternative Co-catalysts in Photocatalysis. Chin. J. Catal. 42 (1), 3–14. doi:10.1016/s1872-2067(20)63630-0
Li, shuang, S., Yun Hau, N., Zhu, R., Lv, S., Wu, Co., Liu, Y., et al. (2021). In Situ construction of Elemental Phosphorus Nanorod-Modified TiO2 Photocatalysts for Efficient Visible-Light-Driven H2 Generation[J]. Appl. Catal. B: Environ., 297. doi:10.1016/j.apcatb.2021.120412
Liu, R., Ji, Z., Wang, J., and Zhang, J. (2018a). Mesocrystalline TiO2/sepiolite Composites for the Effective Degradation of Methyl orange and Methylene Blue. Front. Mater. Sci. 12 (3), 292–303. doi:10.1007/s11706-018-0429-9
Liu, X., Liu, Y., Lu, S., Guo, W., and Xi, B. (2018b). Performance and Mechanism into TiO2/Zeolite Composites for Sulfadiazine Adsorption and Photodegradation. Chem. Eng. J. 350, 131–147. doi:10.1016/j.cej.2018.05.141
Low, J., Cheng, B., and Yu, J. (2017). Surface Modification and Enhanced Photocatalytic CO2 Reduction Performance of TiO2: a Review. Appl. Surf. Sci. 392, 658–686. doi:10.1016/j.apsusc.2016.09.093
Mastunaga, T. (1985). Photoelectrochemical Sterilization of Microbial Cells by Semiconductor Powders[J]. FEMS Microbiol. Lett. 29, 211
Matějka, V., and Neuwirthová, L. (2014). Effect of Kaolinite Exfoliation on the Photocatalytic Properties of Kaolinite/TiO2 Composite[J]. Adv. Sci. Eng. Med. 6 (4), 490. doi:10.1166/asem.2014.1525
Mohamed, E., ElHamza, H., Rafat, T., Wipakorn, J., Nao, T., Naoki, F., et al. (2021). Nitrogen Doping-Mediated Oxygen Vacancies Enhancing Co-catalyst-free Solar Photocatalytic H2 Production Activity in Anatase TiO2 Nanosheet Assembly[J] 285, 119755.
Pelaez, M., Nolan, N. T., Pillai, S. C., Seery, M. K., Falaras, P., Kontos, A. G., et al. (2012). A Review on the Visible Light Active Titanium Dioxide Photocatalysts for Environmental Applications. Appl. Catal. B: Environ. 125 (0), 331–349. doi:10.1016/j.apcatb.2012.05.036
Prakash, J., Sun, S., Swart, H. C., and Gupta, R. K. (2018). Noble Metals-TiO2 Nanocomposites: From Fundamental Mechanisms to Photocatalysis, Surface Enhanced Raman Scattering and Antibacterial Applications. Appl. Mater. Today 11, 82–135. doi:10.1016/j.apmt.2018.02.002
Qiao, B., Chen, Y., Tian, M., Wang, H., Yang, F., Shi, G., et al. (2019). Characterization of Water Soluble Inorganic Ions and Their Evolution Processes during PM2.5 Pollution Episodes in a Small City in Southwest China. Sci. Total Environ. 650, 2605–2613. doi:10.1016/j.scitotenv.2018.09.376
Ranganathan, K., Morais, A., Nongwe, I., Longo, C., Nogueira, A. F., and Coville, N. J. (2016). Study of Photoelectrochemical Water Splitting Using Composite Films Based on TiO 2 Nanoparticles and Nitrogen or boron Doped Hollow Carbon Spheres as Photoanodes. J. Mol. Catal. A: Chem. 422, 165–174. doi:10.1016/j.molcata.2015.10.024
Sato, S., and White, J. M. (1980). Photodecomposition of water over Pt/TiO2 catalysts[J]. Chem. Phys. Letter. 72 (1), 83–86.
Schrauzer, G. N., and Guth, T. D. (2002). Photolysis of Water and Photoreduction of Nitrogen on Titanium Dioxide. J. Am. Chem. Soc. 99 (22), 7189–7193. doi:10.1021/ja00464a015
Scotti, R., D’Arienzo, M., and Morazzoni, F. (2009). Immobilization of Hydrothermally Produced TiO2 with Different Phase Composition for Photocatalytic Degradation of Phenol[J]. Appl. Catal. B: Environ. 88 (3-4), 323–330. doi:10.1016/j.apcatb.2008.11.012
Selcuk, S., Zhao, X., and Selloni, A. (2018). Structural Evolution of Titanium Dioxide during Reduction in High-Pressure Hydrogen. Nat. Mater 17 (10), 923–928. doi:10.1038/s41563-018-0135-0
Shi, J.-w., Zheng, J.-t., and Wu, P. (2009). Preparation, Characterization and Photocatalytic Activities of Holmium-Doped Titanium Dioxide Nanoparticles. J. Hazard. Mater. 161 (1), 416–422. doi:10.1016/j.jhazmat.2008.03.114
Shimelis, K. K., Kiflie, Ze., Dong, W. S., and Young, Rn. (2017). Optimization of Sol-Gel Synthesis Parameters in the Preparationof N-Doped TiO 2 Using Surface Response Methodology[J]. J. Sol-Gel Sci. Techn. 82 (2), 322. doi:10.1007/s10971-017-4322-2
Shuai, H., Wang, Y., Wang, J., Du, G., Chen, D., and Liang, Y. (2021). Preparation of TiO2/Black Talc Composite Photocatalyst and the Research on its Adsorption-Degradation Coupling Effects. Materials 14 (20), 6038. doi:10.3390/ma14206038
Singh, G. P., Shrestha, K. M., Nepal, A., Klabunde, K. J., and Sorensen, C. M. (2014). Graphene Supported Plasmonic Photocatalyst for Hydrogen Evolution in Photocatalytic Water Splitting. Nanotechnology 25 (26), 265701. doi:10.1088/0957-4484/25/26/265701
Singh, T., Pal, D. B., Bhatiya, A. K., et al. (2021). Integrated Process Approach for Degradation of P-Cresol Pollutant under Photocatalytic Reactor Using Activated carbon/TiO2 Nanocomposite: Application in Wastewater Treatment[J]. Environ. Sci. Pollut. Res., 1–10.
Song, E., Kim, D. H., Jeong, E. J., Choi, M., Kim, Y., Jung, H. J., et al. (2021). Effects of Particle Size and Polymorph Type of TiO2 on the Properties of BaTiO3 Nanopowder Prepared by Solid-State Reaction. Environ. Res. 202, 111668. doi:10.1016/j.envres.2021.111668
Su, H., Ma, Z., Hu, T., Zhang, W., and He, H. (2017). Sol-gel Synthesis of χTiO2/HZSM-5 Composite Photocatalyst on Degradation of Reactive Brilliant Red X3B. Cnano 13 (3), 292–298. doi:10.2174/1573413713666170201123624
Suárez, S., Jansson, I., Ohtani, B., and Sánchez, B. (2018). From Titania Nanoparticles to Decahedral Anatase Particles: Photocatalytic Activity of TiO 2/zeolite Hybrids for VOC Oxidation[J]. Catal. Today 326, 2. doi:10.1016/j.cattod.2018.09.004
Sun, J., Bian, J., Li, J., Zhang, Z., Li, Z., Qu, Y., et al. (2020). Efficiently Photocatalytic Conversion of CO2 on Ultrathin Metal Phthalocyanine/g-C3n4 Heterojunctions by Promoting Charge Transfer and CO2 Activation. Appl. Catal. B: Environ. 277, 119199. doi:10.1016/j.apcatb.2020.119199
Székely, I., Kedves, E. Z., and Pap, Z. (2021). Synthesis Design of Electronegativity Dependent WO3 and WO3∙ 0.33 H2O Materials for a Better Understanding of TiO2/WO3 Composites’ Photocatalytic Activity. [J]. Catalysts 11 (7), 779
Tachikawa, T., Fujitsuka, M., and Majima, T. (2007). Mechanistic Insight into the TiO2 Photocatalytic Reactions: Design of New Photocatalysts. J. Phys. Chem. C 111 (14), 5259–5275. doi:10.1021/jp069005u
Tayade, R. J., Surolia, P. K., Kulkarni, R. G., and Jasra, R. V. (2007). Photocatalytic Degradation of Dyes and Organic Contaminants in Water Using Nanocrystalline Anatase and Rutile TiO2. Sci. Techn. Adv. Mater. 8 (6), 455–462. doi:10.1016/j.stam.2007.05.006
Teh, C. M., and Mohamed, A. R. (2011). Roles of Titanium Dioxide and Ion-Doped Titanium Dioxide on Photocatalytic Degradation of Organic Pollutants (Phenolic Compounds and Dyes) in Aqueous Solutions: A Review. J. Alloys Compd. 509 (5), 1648–1660. doi:10.1016/j.jallcom.2010.10.181
Tokarský, J., Čapková, P., and Burda, J. V. (2012). Structure and Stability of kaolinite/TiO 2 Nanocomposite: DFT and MM Computations[J]. J. Mol. Model. 18 (6), 2689. doi:10.1007/s00894-011-1278-y
Tsoukleris, D. S., Maggos, T., Vassilakos, C., et al. (2007). Photocatalytic Degradation of Volatile Organics on TiO2 Embedded Glass Spherules[J]. Catal. Today 129 (1-2), 96–101. doi:10.1016/j.cattod.2007.06.047
Uğurlu, M., and Karaoğlu, M. H. (2011). TiO2 Supported on Sepiolite: Preparation, Structural and thermal Characterization and Catalytic Behaviour in Photocatalytic Treatment of Phenol and Lignin from Olive Mill Wastewater[J]. Chem. Eng. J. 166 (3), 859. doi:10.1016/j.cej.2010.11.056
Vimonses, V., Chong, M. N., and Jin, B. (2010). Evaluation of the Physical Properties and Photodegradation Ability of Titania Nanocrystalline Impregnated onto Modified Kaolin[J]. Microporous Mesoporous Mater. 132 (1-2), 201–209. doi:10.1016/j.micromeso.2010.02.021
Wang, C., Shi, H., Zhang, P., and Li, Y. (2011). Synthesis and Characterization of kaolinite/TiO2 Nano-Photocatalysts. Appl. Clay Sci. 53 (4), 646–649. doi:10.1016/j.clay.2011.05.017
Wang, J.-J., Jing, Y.-H., Ouyang, T., and Chang, C.-T. (2015). Preparation of 13X from Waste Quartz and Photocatalytic Reaction of Methyl Orange on TiO2/ZSM-5, 13X and Y-Zeolite. j nanosci nanotechnol 15 (8), 6141–6149. doi:10.1166/jnn.2015.10202
Wang, J., Fang, Y., Zhang, W., Yu, X., Wang, L., and Zhang, Y. (2021). TiO2/BiOBr 2D-2D Heterostructure via In-Situ Approach for Enhanced Visible-Light Photocatalytic N2 Fixation. Appl. Surf. Sci. 567, 150623. doi:10.1016/j.apsusc.2021.150623
Wang, R., Hashimoto, K., Fujishima, A., Chikuni, M., Kojima, E., Kitamura, A., et al. (1997). Light-induced Amphiphilic Surfaces. Nature 388 (6641), 431–432. doi:10.1038/41233
Wang, X., Sø, L., Su, R., Wendt, S., Hald, P., Mamakhel, A., et al. (2014). The Influence of Crystallite Size and Crystallinity of Anatase Nanoparticles on the Photo-Degradation of Phenol. J. Catal. 310, 100–108. doi:10.1016/j.jcat.2013.04.022
Wang, Y., Craven, M., Yu, X., Ding, J., Bryant, P., Huang, J., et al. (2019). Plasma-Enhanced Catalytic Synthesis of Ammonia over a Ni/Al2O3 Catalyst at Near-Room Temperature: Insights into the Importance of the Catalyst Surface on the Reaction Mechanism. ACS Catal. 9 (12), 10780–10793. doi:10.1021/acscatal.9b02538
Wang, Z. (2018). Recent developments in heterogeneous photocatalysts for solar-driven overall water splitting[J]. Chemical Society Reviews 48 (7), 2109–2125. doi:10.1039/C8CS00542G
Wu, D., Tian, J., Xing, Y., Jin, X., and Ni, G. (2021). Fabrication of Z-Scheme ZnO/Bi2O4 Heterojunction Photocatalyst with superior Photocatalytic Nitrogen Fixation under Visible Light Irradiation. Solid State. Sci. 119, 106709. doi:10.1016/j.solidstatesciences.2021.106709
Xu, H., Sun, S., Jiang, S., Wang, H., Zhang, R., and Liu, Q. (2018). Effect of Pretreatment on Microstructure and Photocatalytic Activity of kaolinite/TiO2 Composite. J. Sol-gel Sci. Technol. 87 (3), 676–684. doi:10.1007/s10971-018-4760-5
Xue, J., and Kannan, K. (2019). Mass Flows and Removal of Eight Bisphenol Analogs, Bisphenol A Diglycidyl Ether and its Derivatives in Two Wastewater Treatment Plants in New York State, USA. Sci. Total Environ. 648, 442–449. doi:10.1016/j.scitotenv.2018.08.047
Yan, X., Yuan, X., Wang, J., Wang, Q., Zhou, C., Wang, D., et al. (2019). Construction of Novel Ternary Dual Z-Scheme Ag3VO4/C3N4/reduced TiO2 Composite with Excellent Visible-Light Photodegradation Activity. J. Mater. Res. 34 (12), 2024–2036. doi:10.1557/jmr.2019.164
Yener, H. B., Yılmaz, M., Deliismail, Ö., Özkan, S. F., and Helvacı, Ş. Ş. (2017). Clinoptilolite Supported Rutile TiO2 Composites: Synthesis, Characterization, and Photocatalytic Activity on the Degradation of Terephthalic Acid. Sep. Purif. Techn. 173, 17–26. doi:10.1016/j.seppur.2016.09.010
Yu, J., Low, J., Xiao, W., Zhou, P., and Jaroniec, M. (2014). Enhanced Photocatalytic CO2-Reduction Activity of Anatase TiO2 by Coexposed {001} and {101} Facets. J. Am. Chem. Soc. 136 (25), 8839–8842. doi:10.1021/ja5044787
Zhang, D. (2014). Photocatalytic Degradation of Organic Compounds by Au-TiO2/sepiolite Composites as the Highly Efficient Catalysts. Russ. J. Phys. Chem. 88 (12), 2252–2259. doi:10.1134/s003602441412005x
Zhang, G., Song, A., Duan, Y., and Zheng, S. (2018). Enhanced Photocatalytic Activity of TiO2/zeolite Composite for Abatement of Pollutants. Microporous Mesoporous Mater. 255, 61–68. doi:10.1016/j.micromeso.2017.07.028
Zhang, G., Sun, Z., Duan, Y., Ma, R., and Zheng, S. (2017). Synthesis of Nano-TiO 2/diatomite Composite and its Photocatalytic Degradation of Gaseous Formaldehyde. Appl. Surf. Sci. 412, 105–112. doi:10.1016/j.apsusc.2017.03.198
Zhang, G., Liu, Y., Hashisho, Z., Sun, Z., and Zheng, S. (2020). Lexuan .Adsorption and Photocatalytic Degradation Performances of TiO2/diatomite Composite for Volatile Organic Compounds: Effects of Key Parameters. [J].Applied Surf. Sci. 525. doi:10.1016/j.apsusc.2020.146633
Zhang, G., Xiong, Q., Xu, W., and Guo, S. (2014). Synthesis of Bicrystalline TiO2 Supported Sepiolite Fibers and Their Photocatalytic Activity for Degradation of Gaseous Formaldehyde. Appl. clay Sci. 102, 231–237. doi:10.1016/j.clay.2014.10.001
Zhang, J., Wang, X., Wang, J., Wang, J., and Ji, Z. (2016). Effect of Sulfate Ions on the Crystallization and Photocatalytic Activity of TiO2/diatomite Composite Photocatalyst. Chem. Phys. Lett. 643, 53–60. doi:10.1016/j.cplett.2015.11.020
Zhang, Y., Gan, H., and Zhang, G. (2011a). A Novel Mixed-phase TiO2/kaolinite Composites and Their Photocatalytic Activity for Degradation of Organic Contaminants[J]. Chem. Eng. J. 172 (2-3), 936–943. doi:10.1016/j.cej.2011.07.005
Zhang, Y., Wang, D., and Zhang, G. (2011b). Photocatalytic Degradation of Organic Contaminants by TiO2/sepiolite Composites Prepared at Low Temperature. Chem. Eng. J. 173 (1), 1–10. doi:10.1016/j.cej.2010.11.028
Zhao, Y., Li, J., Wang, L., Hao, Y., Yang, L., He, P., et al. (2018). Preparation and Characterization of Sulfated TiO2/Zeolite Composite Catalysts with Enhanced Photocatalytic Activity. Nano 13 (10), 1850117. doi:10.1142/s1793292018501175
Zhou, F., Song, H., Wang, H., Komarneni, S., and Yan, C. (2018). N-doped TiO2/sepiolite Nanocomposites with Enhanced Visible-Light Catalysis: Role of N Precursors. Appl. Clay Sci. 166, 9–17. doi:10.1016/j.clay.2018.08.025
Zhu, J., Liang, W., Qingxun, H., and Feng-Shou, X. (2018). Hydrophobic Zeolite Containing Titania Particles as Wettability-Selective Catalyst for Formaldehyde Removal(Article)[J]. ACS Catal. 8 (6), 5250.
Zhu, P., Ren, Z., Wang, R., Duan, M., Xie, L., Xu, J., et al. (2020). Preparation and Visible Photocatalytic Dye Degradation of Mn-TiO2/sepiolite Photocatalysts[J]. Front. Mater. Sci. 14 (7), 1–10. doi:10.1007/s11706-020-0486-8
Zhu, X., Wen, G., Liu, H., Han, S., Chen, S., Kong, Q., et al. (2019). One-step Hydrothermal Synthesis and Characterization of Cu-Doped TiO2 Nanoparticles/nanobucks/nanorods with Enhanced Photocatalytic Performance under Simulated Solar Light. J. Mater. Sci. Mater. Electron. 30 (14), 13826–13834. doi:10.1007/s10854-019-01766-3
Keywords: nano-titanium dioxide, photocatalysis, non-metallic minerals, loading, outlook
Citation: Xie Y, Wang J, Ren F, Shuai H and Du G (2022) Nonmetallic Mineral as the Carrier of TiO2 Photocatalyst: A Review. Front. Catal. 2:806316. doi: 10.3389/fctls.2022.806316
Received: 03 November 2021; Accepted: 31 January 2022;
Published: 30 March 2022.
Edited by:
Ji-Jun Zou, Tianjin University, ChinaReviewed by:
Diana Sannino, University of Salerno, ItalyJingxiang Low, University of Science and Technology of China, China
Copyright © 2022 Xie, Wang, Ren, Shuai and Du. This is an open-access article distributed under the terms of the Creative Commons Attribution License (CC BY). The use, distribution or reproduction in other forums is permitted, provided the original author(s) and the copyright owner(s) are credited and that the original publication in this journal is cited, in accordance with accepted academic practice. No use, distribution or reproduction is permitted which does not comply with these terms.
*Correspondence: Jiao Wang, wj@bgy.edu.cn; Fengguo Ren, rfg@bgy.edu.cn; Gaoxiang Du, dgx@cugb.edu.cn