- State Power Investment Corporation Hydrogen Energy Company, Ltd. Co., Beijing, China
Single-atom catalysts (SACs) have emerged as a hot research topic in recently years, and have been intensively investigated for energy storage and conversion applications. Significant advances in the synthesis of SACs have been achieved through enormous efforts in this area, however, their application is hindered by the low active site loading and poor long-term stability. In contrast with other methods, atomization, in which the SACs are synthesized from transformation of the nanoparticles to atomic sites, is a very attractive and innovative top-down approach to achieve high-density supported active sites with outstanding stability. However, limited attention has been paid to this area, despite the significant advances achieved in the past two years. In this short review, we discuss in detail the latest advances in atomization approaches for the synthesis of SACs and highlight the associated advantages and opportunities.
Introduction
Nanomaterials are widely employed in environmental protection, energy storage/conversion, petrochemical engineering, and other fields (Amin et al., 2014; Abdalla et al., 2018; Hodges et al., 2018). In particular, they exhibit excellent properties and play a critical role in energy-related areas (Bevilacqua et al., 2015; Mao and Hatton, 2015; Cheng et al., 2016a; Cheng et al., 2018). The typical size of these materials is around 10 nm, which endows them with a larger specific surface area and exposed catalytic sites (Cheng et al., 2014; Cheng et al., 2015). Owing to the high cost and low abundance of precious metals, scientists and engineers are directing their efforts to increase the catalytic efficiency and utilization of the individual precious metal atoms by decreasing the nanoparticle (NP) size (Shao et al., 2011; Cargnello et al., 2013). Nevertheless, this reduced sizes cannot meet the demands of green chemistry applications, which require that most atoms of the nanomaterial participate in the catalytic reactions, in order to maximize their utilization (Yang et al., 2013). Noble metal reduction has attracted enormous attention, and approaches to reduce the NP size to clusters or even single atoms have been investigated (Shao et al., 2011; Reske et al., 2014; Cheng et al., 2016b). In contrast with supported NPs, which contain surface-active centers with different coordination environments, single-atom catalysts (SACs) consist of separated atoms, which endows these materials with very good uniformity (Zhang and Zheng, 2016; Kim et al., 2018). This reduces the complexity of the NPs and provides an effective approach to obtain homogenous catalysts.
SACs have attracted wide attention in recent years because of optimal atom utilization, controlled metal coordination environments, unique electronic properties, and homogeneous distribution of catalytic active site (Liu, 2017; Chen et al., 2018a; Cheng et al., 2018; Zhang et al., 2018). The electronic configurations of the highly dispersed atoms can be tuned by modifying the coordination environments, resulting in a class of materials with unique properties (Huang et al., 2012; Deng et al., 2015; Fei et al., 2015; Xiao et al., 2019; Lei et al., 2020). The distinctive properties of the SACs have attracted considerable interest, especially in the area of catalysis.
Owing to their high surface energy, SACs are loaded on supports and are normally referred to as supported SACs (Liu, 2017; Chen et al., 2018a; Cheng et al., 2018; Zhang et al., 2018). The use of SACs has been explored for various applications, such as oxygen reduction/evolution reactions (Zhang et al., 2017; Pan et al., 2018; Yang et al., 2018), carbon dioxide reduction (Cheng et al., 2019b; Cheng et al., 2019c), nitrogen reduction (Zhang R. et al., 2019), and organic synthesis (Zhang et al., 2016). In the past several years, substantial efforts have been dedicated to develop new SACs for a variety of applications, and novel methods have been employed for their synthesis (Szuromi, 2016; Liu, 2017; Cheng et al., 2019b). Recent studies found that SACs can be synthesized by atomization of NPs; this novel strategy provides a route to synthesize SACs with high loading and superior stability. In this review, we first summarize the synthesis methods for SACs and the chemical interaction between the single atoms and their supports. Then, we describe atomization approaches for SAC synthesis and discuss the associated opportunities.
The Road to Atomization Approaches
The synthesis of SACs remains a hot topic, a large number of methods have been developed. These include wet-chemistry, pyrolysis, atomic layer deposition, special confinement (Yang et al., 2013; Wang et al., 2018a; Zhang et al., 2018). The key challenge in SAC synthesis is to avoid the aggregation of the metal atoms resulting from the high surface energy of the atomically dispersed metal atoms. The atomic sites are stabilized through strong metal–support interactions on supports such as metals, metal oxides, chalcogenides, and carbon materials (Huang et al., 2012; Wang et al., 2018c; Xiao et al., 2019; Lei et al., 2020). A strong interaction between metal atoms and support is required to stabilize the dispersed metal atoms (Cheng et al., 2019b). Moreover, a strong coordination environment is the prerequisite to obtain stable SACs. The strong interaction between metal atoms and support will unavoidably affect the properties of both components (Yang et al., 2013; Cheng et al., 2019b), thus offering great opportunities for designing catalysts with controlled characteristics (Yang et al., 2013; Cheng et al., 2019b).
The key step in SAC synthesis is to control the metal–support interactions (Liu, 2017). This interaction is highly dependent on the anchoring sites, such as defects or functionalities introduced to immobilize the metal atoms (Liu, 2016). The ultimate goal in the synthesis process is to create anchoring sites for the metal atoms (Lee et al., 2008). These sites can be created before supporting the metal atoms, or formed in situ at high temperature, simultaneously with the single-metal atomic sites (Figure 1A). In the case of the initial preparation of anchoring sites for stabilizing the metal atoms, a number of methods have been developed since the advent of SACs. They include wet chemistry (Chen et al., 2018a; Liu et al., 2020), atomic layer deposition (Cheng and Sun, 2017), and photochemical reduction (Wei et al., 2017) strategies. In these methods, the supports are initially treated by etching or functionalization to provide defect sites able to stabilize the isolated metal atoms (Zhang Y. et al., 2019). The introduction of defects or ligands of complexes on the support surface facilitates the chemical combination between single atoms and substrate. Therefore, regardless of the wet chemistry, atomic layer deposition, or photochemical reduction approach employed, the success of the synthesis does not depend on how the precursor is introduced, e.g., via solvent or vapor, but relies on the anchoring sites provided. In order to stabilize the atomically dispersed metal atoms, the SACs should normally be annealed at high temperature to create a coordination strong enough to stabilize the atoms during the catalytic process; however, the annealing process unavoidably leads to aggregation of the metal atoms. More importantly, surface defect engineering normally provides a limited amount of surface anchoring sites and leads to weak binding before annealing; this inevitably results in aggregation of metal atoms and low loading of single atoms, which limits the application of this approach in catalysis.
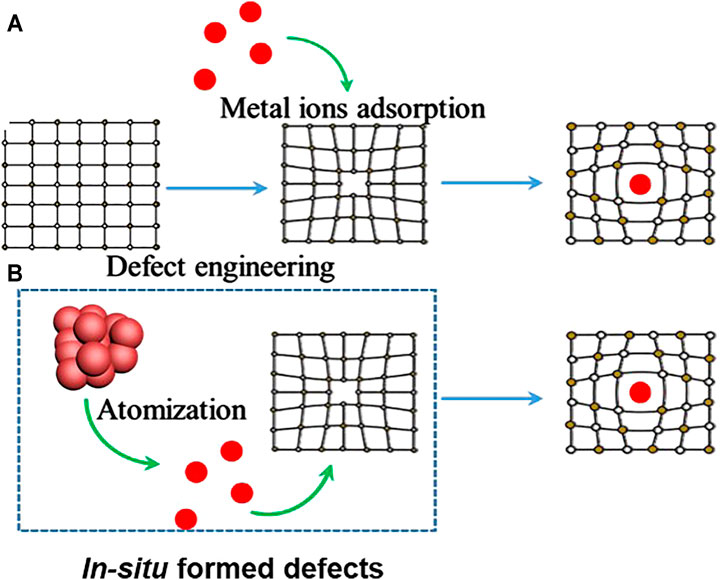
FIGURE 1. (A) Methods adopted for the synthesis of defects before adsorption of metal ions. (B) Atomization methods for the synthesis of SACs.
In practice, a good catalyst should have a high turnover frequency and a large number of accessible active sites, as well as good stability (Won-Jun et al., 2018). Normally, the turnover frequency is strongly dependent on the type of active sites, the coordination environment, and the support, which can affect the electronic structure of the active sites. Enormous efforts have been dedicated to develop new catalysts able to achieve a high turnover frequency through control of the coordination environments (Munnik et al., 2015; Gao et al., 2019; Li et al., 2020a). Because the high temperature can normally create more dynamic defects, the in situ formation of defects during the annealing process is attracting increasing attention (Hiroshi, 2014). Taking carbon-supported SACs as an example, non-metal dopants such as N, P, and S could form defects able to stabilize the single metal atoms and avoid their aggregation or migration during the annealing process (Zhang Y. et al., 2019). During the pyrolysis process, metal atoms can be anchored by the in situ-formed defects, providing a strategy to achieve high-density atomic sites. As a further route to avoid aggregation, spatial confinement strategies can be employed to further prevent the migration of single atoms, especially when large amounts of metal precursor are introduced (Li Z. et al., 2020). The materials employed for spatial confinement are porous systems such as metal–organic frameworks (MOFs), covalent organic frameworks (COFs), carbon nitrides, lamellar materials, graphdiyne, porous carbon, and zeolites (Li Z. et al., 2020). Unfortunately, these strategies normally leads to encapsulation of the active sites inside the structure rather than on the surface, which results in low utilization of metal atoms (Hou et al., 2020).
Although the development of SACs with a high density of surface-active sites is critically important, this is not an easy task. One of the important strategies to achieve a high weight content of single atoms over carbon is to simultaneously create defects for trapping single atoms (Wang J. et al., 2018). In this process, the in situ-created defects will be stabilized and thus increase the metal loading, because high-temperature annealing can create a huge number of dynamic defects. The latter can trap the metal atoms to form a stable coordination environment, leading to a high loading of surface-supported single atoms with high stability.
Intriguingly, recent research found that metal NPs can be dispersed into atoms at high temperatures (Hermenegildo, 2014). Conventionally, owing to the thermal migration of metal atoms, single metal atoms or ultrafine metal NPs with high surface free energy tend to aggregate into larger particles under high-temperature conditions, in a process called Ostwald ripening (Lochhead, 2017). Once these migrating atoms are effectively trapped by the dynamic defects at high temperature, atomically dispersed metal sites are formed. Therefore, anti-Ostwald ripening is an efficient way to obtain SACs, and the transformation of the support at high temperature can usually provide more dynamic spaces for anchoring metal atoms (Figure 1B), achieving a high surface density of single atoms. More importantly, the formation of single atom sites at high temperature can create a strong bond between the metal atoms and the substrate, thus endowing the materials with high stability and preventing the sintering of the dispersed atomic sites (Moliner et al., 2016). These advantages indicate an innovative route to achieve high-density single-atom active sites with outstanding stability.
Recent Advances in Atomization for SAC Synthesis
At high temperature, the presence of the defects in metal oxides is more dynamic and the defect concentration is significantly higher than at room temperature; this opens up opportunities to obtain high-density single atoms with enhanced stability (Sasaki and Maier, 1999). Metal oxides are ideal supports that can provide defects or vacancies, especially at high temperature, creating dynamic sites able to immobilize the migrating atoms (Jones et al., 2016). SACs based on noble metals such as Pt, Pd, and Au have been synthesized by mixing the NP source with metal oxide supports (Yu-Yao and Kummer, 1987). Under high-temperature conditions, Pt can be released as volatile PtO2 and transferred from the pristine metal nanoparticles to the metal oxide (Yu-Yao and Kummer, 1987; Bruix et al., 2014; Moliner et al., 2016). Ceria oxide has been shown to provide a type of “nanopocket” able to bind Pt2+ ions so strongly that they withstand sintering and bulk diffusion (Bruix et al., 2014). Neitzel et al. pointed out that the Pt cations remain stable on the surface of the substrate, instead of diffusing into the ceria bulk (Neitzel et al., 2016). Based on these findings, the Datye group found that aluminum oxide-supported Pt NPs can be converted into single Pt atoms and trapped on CeO2 (100) surfaces. Compared with ceria cubes, it is easier to trap Pt species on polyhedral ceria and ceria nanorods to form atomically dispersed catalysts, suggesting that the support plays an important role (Jones et al., 2016). Moliner et al. (2016) found that Pt NPs can transform into single Pt atoms in O2 atmosphere and high-temperature conditions. Reversible conversion of NPs and single atoms can be achieved efficiently in reducing and oxidizing atmospheres (Figure 2) (Moliner et al., 2016). Using N, N, N-trimethyl-1-adamantammonium and a Pt-mercapto complex as organic structure-directing agent and Pt precursor, respectively, single Pt atoms were synthesized in O2 atmosphere; these atoms were stable at 650°C. The Pt–O coordination number was found to be 2.71 after calcination at 500°C. Pt single atoms supported on highly siliceous chabazite could convert >80% of ethylene to ethane (Moliner et al., 2016).
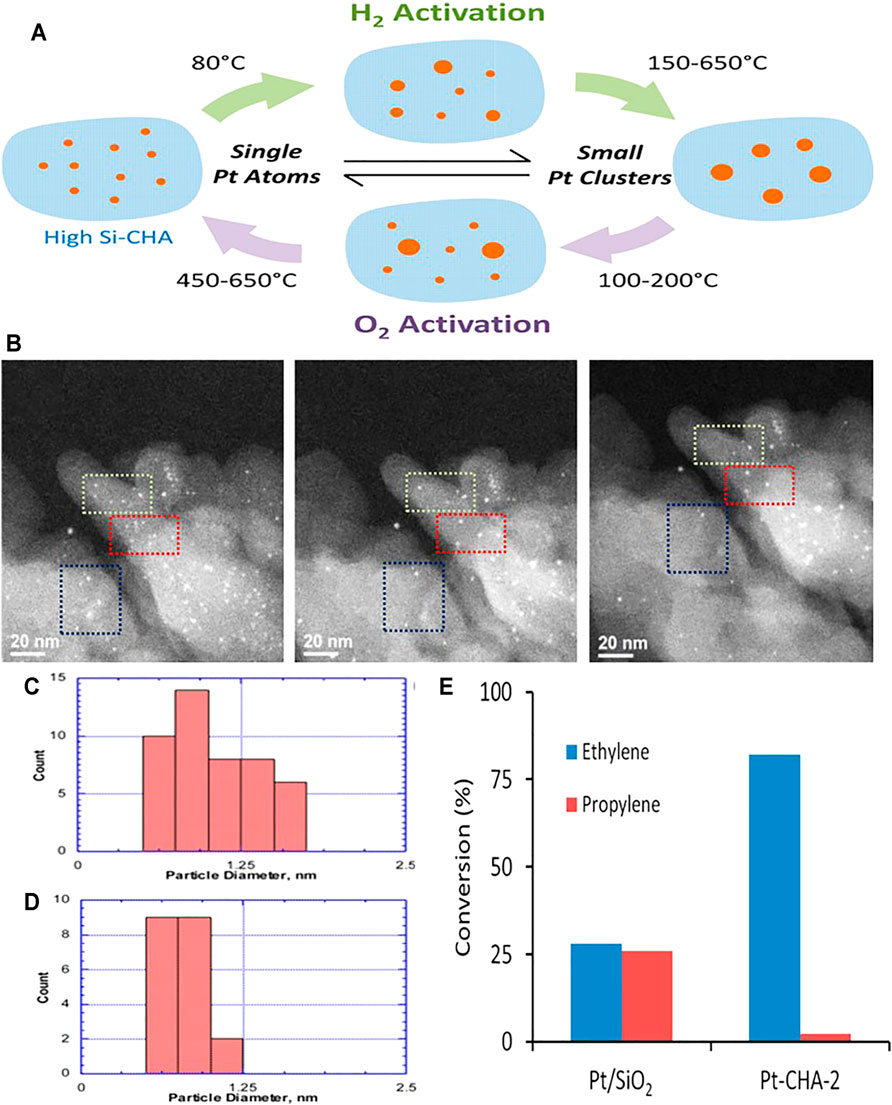
FIGURE 2. (A) Scheme of reversible conversion between Pt NPs and single atoms supported on high-silica chabazite (high Si-CHA) zeolite. (B) Aberration-corrected high-angle annular dark-field scanning transmission electron microscopy (HAADF-STEM) images of reduced Pt-chabazite. (C, D) particle size distributions of (C) reduced Pt-chabazite and (D) Sample subjected to 20-min in situ O 2 treatment. (E) Comparison of ethylene and propylene hydrogenation performances of Pt-highly siliceous chabazite and Pt/SiO2. Reproduced with permission from (Moliner et al., 2016). Copyright 2016, American Chemical Society.
Recently, Cai et al. (2019) reported an effective way to convert noble Au NPs to single atoms by annealing and used this approach to anchor Au single atoms on Ni2P (Figure 3). First, nanoscale Au@Ni2P yolk–shell structures were synthesized using chloroauric acid, oleylamine, octadecylene, and triphenylphosphine. Then, the Au@Ni2P yolk–shell structure was heated to 350°C and kept at that temperature for 1 h. The annealing procedure can change the morphology and atomic structure of Au@Ni2P nanoparticles, and the results of aberration-corrected in situ high-resolution STEM showed that single Au atoms could be well dispersed in the Ni2P matrix by diffusing into the Ni2P shell. At 1.47 V vs. RHE, the annealed sample exhibited a better oxygen evolution performance than fresh Au@Ni2P, pure Ni2P, and commercial IrO2, with 12-, 40-, and 16-fold increases in the oxygen evolution reaction (OER) rate, respectively (Cai et al., 2019).
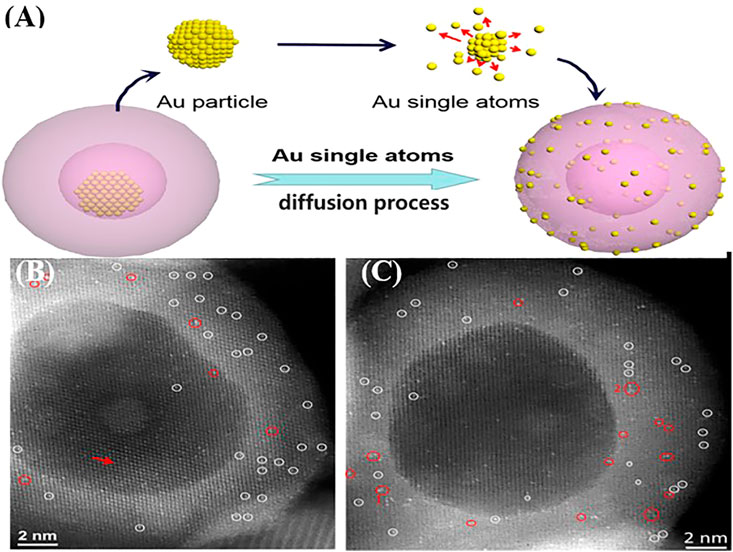
FIGURE 3. (A) Scheme of conversion of Au NPs to Au single atoms using a yolk-shell structure. (B,C) Images of Au single atoms in Ni2P matrix (single Au atoms and small Au cluster are represented as white and red circles, respectively). Reproduced with permission from (Cai et al., 2019). Copyright 2019, American Chemical Society.
Huang et al. (2012) synthesized Ag single atoms supported on hollandite-type manganese oxide by in situ atomization of supported Ag NPs. Typically, the Ag NPs were supported onto hollandite-type manganese oxide upon annealing at 230 °C, as the Ag NPs fragmented into Ag atoms and migrated to the inside of the hollandite-type manganese oxide. This was followed by the formation of single Ag atom chains, resulting in the Ag atoms being anchored at hollandite-type manganese oxide tunnel openings. The conversion of Ag NPs to single Ag atom chains by annealing was demonstrated by X-ray diffraction (XRD), high-resolution transmission electron microscopy (HRTEM), and Fourier-transform extended X-ray absorption fine structure (EXAFS) characterizations. The single Ag atom catalyst exhibited superior ability in the activation of lattice oxygen atoms and molecular oxygen, which promote the oxidation of formaldehyde (Huang et al., 2012). Lang et al. (2019) used covalent metal–support interactions to trap vaporized Pt atoms on the surface of Fe2O3 and synthesize a high-loading Pt SAC. As the temperature increased from 300 to 700°C, the Pt NPs were converted to Pt single atoms on Fe2O3 (Lang et al., 2019). The Pt NPs eventually disappeared and a high density of single Pt atoms was obtained by calcination at 800°C for 5 h. Calculation results showed that the formed Pt atoms coordinated four surface oxygen atoms, with an average Pt–O length of 1.94 Å. And the obtained catalyst methane combustion exhibited outstanding activity to promote methane combustion. (Lang et al., 2019).
Carbon materials are effective supports for achieving high loadings of single atoms, owing to their high surface area and tunable structure. The introduction of heteroatoms (e.g., N, B, and P) in carbon nanomaterials can alter their electronic structure, providing inhomogeneous anchoring sites for metal atoms that could break the inert C6 symmetry (Nistor et al., 2011; Dai et al., 2015). Owing to the ultrahigh doping levels that could be introduced, carbon supports provide an ideal pathway to achieve high loadings of single atoms (Cheng et al., 2018; Yan et al., 2018; Chang et al., 2019; Cheng et al., 2019a). Conventionally, the efficient way to synthesize carbon-supported SACs is to mix metal salt precursors with carbon and heteroatom precursors (Cheng et al., 2019b). During this process, metal atoms (M) coordinate nitrogen, phosphorus, sulfur, and/or carbon atoms, forming M–N/S/P/C-based single atoms (Chen et al., 2018b; Li et al., 2018). A recent study showed that using metal NPs annealed with carbon and nitrogen sources could be used to synthesize SACs. Yang et al. (Jian et al., 2018) prepared Ni atoms supported on the surface of carbon nanotubes (CNTs) and applied them to catalyze the electroreduction of CO2. Owing to thermal diffusion, Ni NPs trapped on the carbon nanotubes can be converted to single Ni atoms on the surface (Figure 4). The Ni NPs contribute to break C–C bonds on the carbon nanotube surface; then, the fragmented Ni atoms can be anchored by the N defects on the surface of the carbon matrix, after which the Ni NPs are gradually transformed into Ni single atoms. The conversion process was demonstrated by in situ environmental TEM. Quantitative EXAFS fitting results showed that the Ni atoms had a coordination number of 4.3 and a mean bond length of 1.89 Å, in contrast to the 12 and 2.48 Å values measured for Ni NPs, respectively. The Ni SACs showed a higher electrocatalytic current than N-doped carbon and carbon nanotubes for electrochemical reduction of carbon dioxide. Similarly, Fan et al. (2020) synthesized Ni single atoms on the surface of N-doped carbon nanotubes through conversion of Ni NPs to Ni SAs at high temperature, using a polymeric layer of resorcinol, melamine and formaldehyde. Thermal migration on the CNT surface facilitated migration of Ni NPs into N-doped carbon nanotubes to form the Ni–N coordination shell. The atomic dispersed Ni atoms provided highly efficient electrocatalytic carbon dioxide reduction at low overpotentials to procude CO, showing a CO faradaic efficiency over 90%, turnover frequency upto 12,000 h−1, outperforming current state-of-the-art single atom catalysts.
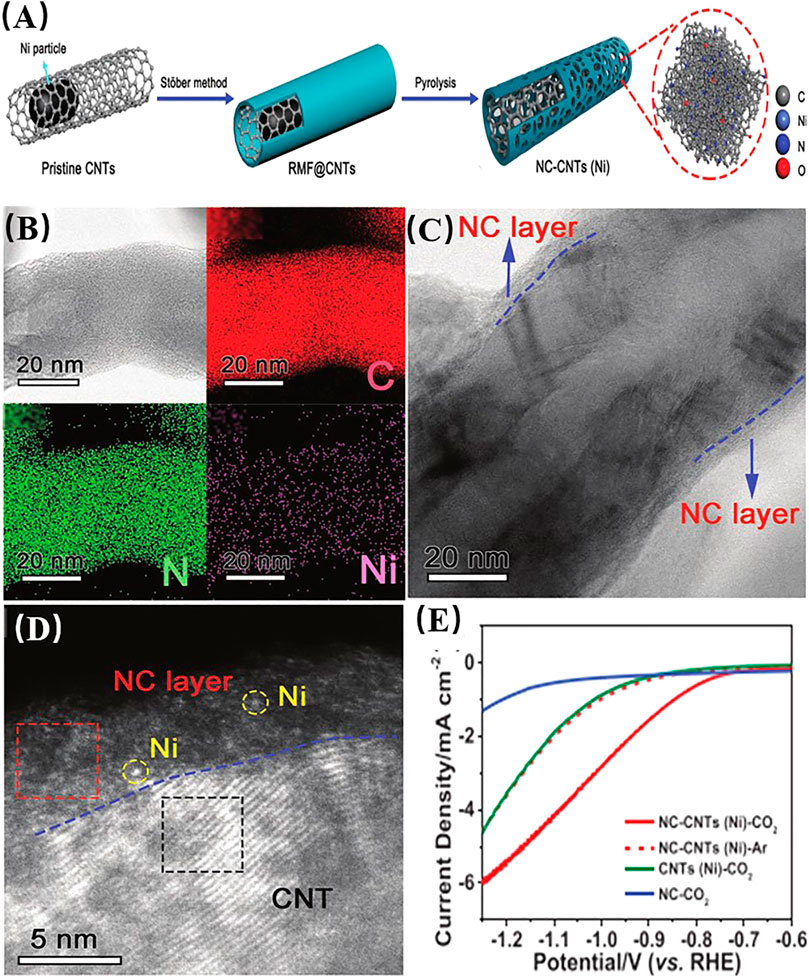
FIGURE 4. (A) Schematic illustration of the formation of single Ni atoms supported on N-doped carbon nanotubes (NC-CNTs) derived from resorcinol-melamine-formaldehyde resin (RMF). (B) HAADF-STEM image of individual single Ni atoms supported on N-doped carbon nanotubes and corresponding energy-dispersive X-ray spectroscopy (EDX) maps. (C) HAADF-STEM image. (D) High-magnification HAADF-STEM image. (E) Linear sweep voltammetry (LSV) curves acquired under CO2-saturated (solid line) or Ar-saturated (dashed line) conditions (Fan et al., 2019). Reproduced with permission from (Fan et al., 2019). Copyright 2018, Wiley-VCH.
Rong et al. synthesized a Bi SAC on N-doped carbon using in situ pyrolysis of a Bi-based metal–organic framework and dicyandiamide. The decomposition of dicyandiamide released NH3, which facilitated the atomization of Bi NPs. And the single-atom Bi-N4 site was demonstrated the dominating active sites for carbon dioxide activation. Similarly, N-doped carbon was used to stabilize Ni SACs by enhancing the surface area and porosity (Zhang E. et al., 2019). Wei et al. (2018) prevented the sintering effects in SACs and successfully transformed noble metal NPs (Pd, Pt, Au) into highly active SACs. Using the thermal pyrolysis of a metal–organic framework (zeolite imidazolate framework-8, ZIF-8) to prepare an N-doped carbon matrix, the noble metal atoms were anchored on N-doped carbon at 900°C (Figure 5). In situ environmental TEM results showed that the sintering effects could still be observed in SACs at high temperatures. However, the increasing size of the NPs resulted in a higher collision probability between enlarged Pd NPs and nanoclusters; thus, the Pd NPs became gradually smaller and finally transformed into Pd single atoms. The thermal migration of large Pd particles within carbon nitride had a significant effect on the size reduction of sintered Pd NPs (Wei et al., 2018). The thermally stable Pd single atoms exhibited even better activity and selectivity than nanoparticles (Pd-NPs) for semi-hydrogenation of acetylene.
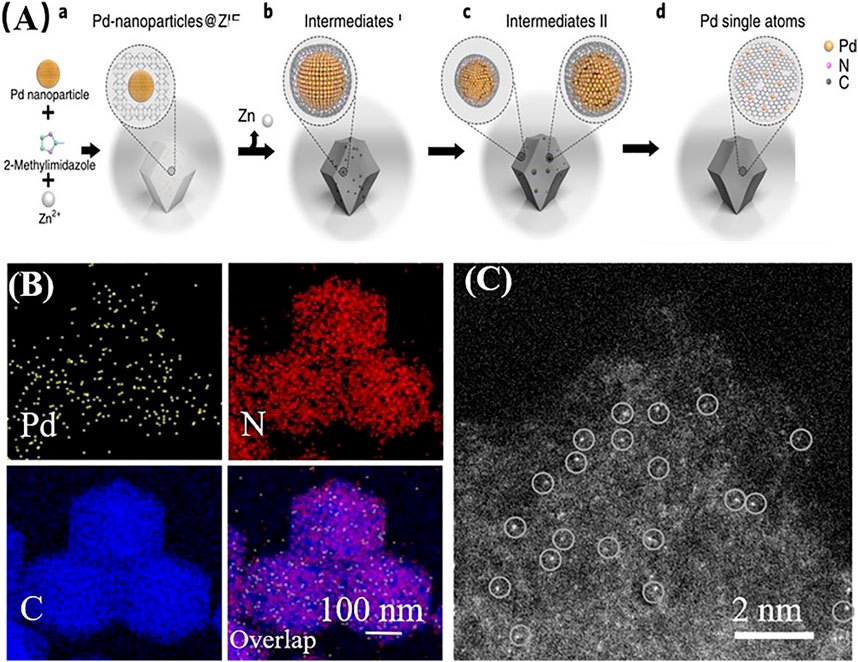
FIGURE 5. (A) Schematic illustration of the conversion of Pd NPs to single Pd atoms supported on N-doped carbon. (B) EDX elemental mapping of Pd single atoms supported on N-doped carbon. (C) High-resolution HAADF-STEM image of Pd single atoms supported on N-doped carbon (Wei et al., 2018a; Wei et al., 2018b). Reproduced with permission from (Wei et al., 2018a) Copyright 2018, Nature Publishing Group.
Despite the great progress made in this area, the atomization of NPs still faces several challenges at this stage, which include: a) operation conditions involving high temperatures; b) lack of a universal and simple approach; and c) a degree of atomization not high enough to achieve a high loading, due to the Ostwald ripening limitations. In order to achieve rapid atomization and suitable synthesis conditions, new agents have been introduced to promote the atomization process under mild conditions. Guo et al (Zhou et al., 2019) demonstrated that PH3 could promote the effective conversion of noble metal NPs (M-NPs, M = Ru, Rh, Pd) at 400 °C to M-P single atoms with good thermal stability on g-C3N4 nanosheets. Experimental and theoretical results revealed that single Pd atoms loaded on g-C3N4 nanosheets exist in the form of Pd-P2.
Qu et al. developed a novel gas migration strategy to directly obtain SACs through conversion of bulk nanomaterials, resulting a material active for oxygen reduction reaction. During the gas migration process, ZIF-8 was pyrolyzed in Ar atmosphere at a high temperature (1,173 K) to obtain empty zinc nodes and abundant defects. At the same time, ammonia molecules combined with the Cu atoms from the Cu foam surface and formed volatile Cu(NH3)x species in NH3 atmosphere. Finally, the Cu(NH3)x species were trapped by the defects of the N-doped carbon support, generating Cu SACs. EXAFS and XPS results revealed that the Cu single atoms were coordinated to 4 N atoms. Furthermore, Qu et al. showed that this gas migration strategy can be applied to synthesize other Co. or Ni SACs (Qu et al., 2018).
Feng et al. reported the transformation of Ru, Rh, Pd, Ag, Ir, and Pt NPs into single atoms supported on carbon with high dispersion (5 wt%), assisted by a mixture of CH3I and CO. Taking Rh as an example, the I• radicals and CO molecules could promote the breaking of Rh–Rh bonds to form mononuclear complexes. The isolated Rh mononuclear complexes were stabilized by the oxygen-containing functional groups. This method provides a general strategy for the development of single-site catalysts, also for other metals (Feng et al., 2019).
Discussion
The preparation of SACs requires a high surface density of active sites and stable coordination. Atomization provides an efficient way to obtain stable supported SACs with high-density surface-active sites. The volatile metal species and defects are critical for the transformation of NPs into single atoms. The atomization of the metal nanoparticles is closely related with the atmosphere that applied to synthesis SACs, thus control the atomization environment is the key to achieve efficient atomization. Noticeably, some metal nanoparticles are stable and not easy to form atomic moistures, this limits the general applicability of this strategy for SAC synthesis, because the breaking of metal–metal bonds to form volatile species is an energy-demanding process (Rodriguez and Goodman, 1992; Berry and Lu, 2017), and the required energy varies significantly from metal to metal. For example, the Fe–Fe bond energy is 418 kJ mol−1, which is 3.2 times that of Zn–Zn bonds (131 kJ mol−1). This makes it particularly hard to develop general methods for atomization, and these universal approaches are urgently required. The introduction of new agents can enhance the mobilization or vaporization of metal atoms, providing new routes for the atomization process. However, high-temperature annealing is still required. Thus, the identification of new agents to assist the atomization process is a task of great significance.
Furthermore, loading metal NPs on the supports followed by atomization at high temperature in the presence of agents normally requires a multistep process. However, simple methods for this purpose are lacking, and further investigations are required. The Li group developed a new approach involving the use of bulk copper as precursor to provide volatile Cu species in the presence of NH3; this simple approach offers great opportunities for bulk synthesis (Qu et al., 2018). More importantly, the atomization process and mechanism need to be further explored in order to guide the development of novel strategies.
Conclusion
In summary, SACs are attracting enormous interest, and significant advances have been achieved in the development of new strategies to obtain highly active SACs. Compared with conventional approaches such as wet chemistry, atomic layer deposition, and photochemical reduction methods, the atomization of metal NPs provides a more effective route to achieve highly dispersed and stable SACs. Interestingly, the atomization of supported metal NPs normally takes place on the surface of the supports, thus providing an effective way to achieve a high surface density of active sites with superior stability. More importantly, this atomization method are easy scale up, thus offer opportunities to promote the practical application of SACs. This unconventional approach offers new opportunities for the synthesis of highly efficient SACs.
Author Contributions
AZ drafted the manuscript. SJ revised the manuscript. All authors discussed the manuscript and approved the final version.
Funding
This work has received financial support from the Beijing Municipal Science and Technology Project (Z191100004719006).
Conflict of Interest
Authors AZ, MZ, SL, MC, and SJ was employed by State Power Investment Corporation Hydrogen Energy Company, Ltd. Co., Beijing, P.R.China.
Publisher’s Note
All claims expressed in this article are solely those of the authors and do not necessarily represent those of their affiliated organizations, or those of the publisher, the editors and the reviewers. Any product that may be evaluated in this article, or claim that may be made by its manufacturer, is not guaranteed or endorsed by the publisher.
References
Abdalla, A. M., Hossain, S., Azad, A. T., Petra, P. M. I., Begum, F., Eriksson, S. G., et al. (2018). Nanomaterials for Solid Oxide Fuel Cells: A Review. Renew. Sustainable Energ. Rev. 82, 353–368. doi:10.1016/j.rser.2017.09.046
Amin, M. T., Alazba, A. A., and Manzoor, U. (2014). A Review of Removal of Pollutants from Water/Wastewater Using Different Types of Nanomaterials. Adv. Mater. Sci. Eng. 2014, 825910. doi:10.1155/2014/825910
Berry, J. F., and Lu, C. C. (2017). Metal-Metal Bonds: From Fundamentals to Applications. Inorg. Chem. 56, 7577–7581. doi:10.1021/acs.inorgchem.7b01330
Bevilacqua, M., Filippi, J., Miller, H. A., and Vizza, F. (2015). Recent Technological Progress in CO2Electroreduction to Fuels and Energy Carriers in Aqueous Environments. Energ. Technology 3, 197–210. doi:10.1002/ente.201402166
Bruix, A., Lykhach, Y., Matolínová, I., Neitzel, A., Skála, T., Tsud, N., et al. (2014). Maximum Noble-Metal Efficiency in Catalytic Materials: Atomically Dispersed Surface Platinum. Angew. Chem. Int. Ed. 53, 10525–10530. doi:10.1002/anie.201402342
Cai, C., Han, S., Wang, Q., and Gu, M. (2019). Direct Observation of Yolk-Shell Transforming to Gold Single Atoms and Clusters with Superior Oxygen Evolution Reaction Efficiency. ACS Nano 13, 8865–8871. doi:10.1021/acsnano.9b02135
Cargnello, M., Doan-Nguyen, V. V. T., Gordon, T. R., Diaz, R. E., Stach, E. A., Gorte, R. J., et al. (2013). Control of Metal Nanocrystal Size Reveals Metal-Support Interface Role for Ceria Catalysts. Science 341, 771–773. doi:10.1126/science.1240148
Chang, X., Zhou, X., Ou, X., Lee, C. S., Zhou, J., and Tang, Y. (2019). Ultrahigh Nitrogen Doping of Carbon Nanosheets for High Capacity and Long Cycling Potassium Ion Storage. Adv. Energ. Mater. 9, 1902672. doi:10.1002/aenm.201902672
Chen, Y., Ji, S., Chen, C., Peng, Q., Wang, D., and Li, Y. (2018a). Single-Atom Catalysts: Synthetic Strategies and Electrochemical Applications. Joule 2, 1242–1264. doi:10.1016/j.joule.2018.06.019
Chen, Y., Ji, S., Zhao, S., Chen, W., Dong, J., Cheong, W.-C., et al. (2018b). Enhanced Oxygen Reduction with Single-Atomic-Site Iron Catalysts for a Zinc-Air Battery and Hydrogen-Air Fuel Cell. Nat. Commun. 9, 5422. doi:10.1038/s41467-018-07850-2
Cheng, N., and Sun, X. (2017). Single Atom Catalyst by Atomic Layer Deposition Technique. Chin. J. Catal. 38, 1508–1514. doi:10.1016/s1872-2067(17)62903-6
Cheng, Y., Zhao, S., Johannessen, B., Veder, J. P., Saunders, M., Rowles, M. R., et al. (2018). Atomically Dispersed Transition Metals on Carbon Nanotubes with Ultrahigh Loading for Selective Electrochemical Carbon Dioxide Reduction. Adv. Mater. 30, e1706287. doi:10.1002/adma.201706287
Cheng, Y., Dou, S., Saunders, M., Zhang, J., Pan, J., Wang, S., et al. (2016a). A Class of Transition Metal-oxide@MnOx Core-Shell Structured Oxygen Electrocatalysts for Reversible O2 Reduction and Evolution Reactions. J. Mater. Chem. A. 4, 13881–13889. doi:10.1039/c6ta04758k
Cheng, Y., He, S., Lu, S., Veder, J. P., Johannessen, B., Thomsen, L., et al. (2019a). Iron Single Atoms on Graphene as Nonprecious Metal Catalysts for High‐Temperature Polymer Electrolyte Membrane Fuel Cells. Adv. Sci. 6, 1802066. doi:10.1002/advs.201802066
Cheng, Y., Pan, J., Saunders, M., Yao, S., Shen, P. K., Wang, H., et al. (2016b). Structurally Confined Ultrafine NiO Nanoparticles on Graphene as a Highly Efficient and Durable Electrode Material for Supercapacitors. RSC Adv. 6, 51356–51366. doi:10.1039/c6ra04880c
Cheng, Y., Shen, P. K., Saunders, M., and Jiang, S. P. (2015). Core-Shell Structured PtRuCo X Nanoparticles on Carbon Nanotubes as Highly Active and Durable Electrocatalysts for Direct Methanol Fuel Cells. Electrochimica Acta 177, 217–226. doi:10.1016/j.electacta.2015.01.137
Cheng, Y., Xu, C., Shen, P. K., and Jiang, S. P. (2014). Effect of Nitrogen-Containing Functionalization on the Electrocatalytic Activity of PtRu Nanoparticles Supported on Carbon Nanotubes for Direct Methanol Fuel Cells. Appl. Catal. B: Environ. 158-159, 140–149. doi:10.1016/j.apcatb.2014.04.017
Cheng, Y., Yang, S., Jiang, S. P., and Wang, S. (2019b). Supported Single Atoms as New Class of Catalysts for Electrochemical Reduction of Carbon Dioxide. Small Methods 3, 1800440. doi:10.1002/smtd.201800440
Cheng, Y., Zhao, S., Li, H., He, S., Veder, J.-P., Johannessen, B., et al. (2019c). Unsaturated Edge-Anchored Ni Single Atoms on Porous Microwave Exfoliated Graphene Oxide for Electrochemical CO2. Appl. Catal. B: Environ. 243, 294–303. doi:10.1016/j.apcatb.2018.10.046
Dai, L., Xue, Y., Qu, L., Choi, H.-J., and Baek, J.-B. (2015). Metal-Free Catalysts for Oxygen Reduction Reaction. Chem. Rev. 115, 4823–4892. doi:10.1021/cr5003563
Deng, D., Chen, X., Yu, L., Wu, X., Liu, Q., Liu, Y., et al. (2015). A Single Iron Site Confined in a Graphene Matrix for the Catalytic Oxidation of Benzene at Room Temperature. Sci. Adv. 1, e1500462. doi:10.1126/sciadv.1500462
Fan, Q., Hou, P., Choi, C., Wu, T. S., Hong, S., Li, F., et al. (2020). Activation of Ni Particles into Single Ni-N Atoms for Efficient Electrochemical Reduction of CO 2. Adv. Energ. Mater. 10, 1903068. doi:10.1002/aenm.201903068
Fei, H., Dong, J., Arellano-Jiménez, M. J., Ye, G., Dong Kim, N., Samuel, E. L. G., et al. (2015). Atomic Cobalt on Nitrogen-Doped Graphene for Hydrogen Generation. Nat. Commun. 6, 8668. doi:10.1038/ncomms9668
Feng, S., Song, X., Liu, Y., Lin, X., Yan, L., Liu, S., et al. (2019). In Situ formation of Mononuclear Complexes by Reaction-Induced Atomic Dispersion of Supported noble Metal Nanoparticles. Nat. Commun. 10, 5281. doi:10.1038/s41467-019-12965-1
Gao, L., Li, X., Yao, Z., Bai, H., Lu, Y., Ma, C., et al. (2019). Unconventional P-D Hybridization Interaction in PtGa Ultrathin Nanowires Boosts Oxygen Reduction Electrocatalysis. J. Am. Chem. Soc. 141, 18083–18090. doi:10.1021/jacs.9b07238
Hermenegildo, G. (2014). Allotropic Carbon Nanoforms as Advanced Metal-free Catalysts or as Supports. Adv. Chem. 2014, 1–20. doi:10.1155/2014/906781
Hiroshi, N. (2014). Defects in Metals. Phys. Metall. 2014, 561–637. doi:10.1016/b978-0-444-53770-6.00006-x
Hodges, B. C., Cates, E. L., and Kim, J.-H. (2018). Challenges and Prospects of Advanced Oxidation Water Treatment Processes Using Catalytic Nanomaterials. Nat. Nanotech 13, 642–650. doi:10.1038/s41565-018-0216-x
Hou, C.-C., Wang, H.-F., Li, C., and Xu, Q. (2020). From Metal-Organic Frameworks to Single/dual-Atom and Cluster Metal Catalysts for Energy Applications. Energy Environ. Sci. 13, 1658–1693. doi:10.1039/c9ee04040d
Huang, Z., Gu, X., Cao, Q., Hu, P., Hao, J., Li, J., et al. (2012). Catalytically Active Single-Atom Sites Fabricated from Silver Particles. Angew. Chem. Int. Ed. 51, 4198–4203. doi:10.1002/anie.201109065
Jian, Y., Zongyang, Q., Changming, Z., Weichen, W., Wenxing, C., Zhijun, L., et al. (2018). In-situ Thermal Atomization to Transfer Supported Metal Nanoparticles to Surface Enriched Ni Single Atom Catalyst. Angew. Chem. Int. Edition 130 (43), 14291–14296. doi:10.1002/ange.201808049
Jones, J., Xiong, H., Delariva, A. T., Peterson, E. J., Pham, H., Challa, S. R., et al. (2016). Thermally Stable Single-Atom Platinum-On-Ceria Catalysts via Atom Trapping. Science 353, 150–154. doi:10.1126/science.aaf8800
Kim, J., Kim, H.-E., and Lee, H. (2018). Single-Atom Catalysts of Precious Metals for Electrochemical Reactions. ChemSusChem. 11, 104–113. doi:10.1002/cssc.201701306
Lang, R., Xi, W., Liu, J.-C., Cui, Y.-T., Li, T., Lee, A. F., et al. (2019). Non Defect-Stabilized Thermally Stable Single-Atom Catalyst. Nat. Commun. 10, 234. doi:10.1038/s41467-018-08136-3
Lee, K., Park, I., Cho, Y., Jung, D., Jung, N., Park, H., et al. (2008). Electrocatalytic Activity and Stability of Pt Supported on Sb-Doped SnO2 Nanoparticles for Direct Alcohol Fuel Cells. J. Catal. 258, 143–152. doi:10.1016/j.jcat.2008.06.007
Lei, Y., Wang, Y., Liu, Y., Song, C., Li, Q., Wang, D., et al. (2020). Realizing the Atomic Active center for Hydrogen Evolution Electrocatalysts. Angew. Chem. Int. Ed. Engl. 59, 47. doi:10.1002/ange.201914647
Li, H., Zhang, H.-X., Yan, X.-L., Xu, B.-S., and Guo, J.-J. (2018). Carbon-supported Metal Single Atom Catalysts. New Carbon Mater. 33, 1–11. doi:10.1016/s1872-5805(18)60322-1
Li, X., Li, X., Liu, C., Huang, H., Gao, P., Ahmad, F., et al. (2020a). Atomic-Level Construction of Tensile-Strained PdFe Alloy Surface toward Highly Efficient Oxygen Reduction Electrocatalysis. Nano Lett. 20, 1403–1409. doi:10.1021/acs.nanolett.9b05024
Li, Z., Ji, S., Liu, Y., Cao, X., Tian, S., Chen, Y., et al. (2020b). Well-Defined Materials for Heterogeneous Catalysis: From Nanoparticles to Isolated Single-Atom Sites. Chem. Rev. 120, 623–682. doi:10.1021/acs.chemrev.9b00311
Liu, J. (2017). Catalysis by Supported Single Metal Atoms. ACS Catal. 7, 34–59. doi:10.1021/acscatal.6b01534
Liu, J. (2016). Catalysis by Supported Metal Single Atoms. Acs Catal. 7, 34–59. doi:10.1021/acscatal.6b01534
Liu, W., Zhang, H., Li, C., Wang, X., Liu, J., and Zhang, X. (2020). Non-noble Metal Single-Atom Catalysts Prepared by Wet Chemical Method and Their Applications in Electrochemical Water Splitting. J. Energ. Chem. 47, 333–345. doi:10.1016/j.jechem.2020.02.020
Lochhead, R. Y. (2017). “Basic Physical Sciences for the Formulation of Cosmetic Products,” in Chapter 3 - Basic Physical Sciences for the Formulation of Cosmetic Products. Cosmetic Science and Technology. Editors K. Sakamoto, R. Y. Lochhead, H. I. Maibach, and Y. Yamashita (Amsterdam: Elsevier), 39–76. doi:10.1016/b978-0-12-802005-0.00003-3
Mao, X., and Hatton, T. A. (2015). Recent Advances in Electrocatalytic Reduction of Carbon Dioxide Using Metal-free Catalysts. Ind. Eng. Chem. Res. 54, 4033–4042. doi:10.1021/ie504336h
Moliner, M., Gabay, J. E., Kliewer, C. E., Carr, R. T., Guzman, J., Casty, G. L., et al. (2016). Reversible Transformation of Pt Nanoparticles into Single Atoms inside High-Silica Chabazite Zeolite. J. Am. Chem. Soc. 138, 15743–15750. doi:10.1021/jacs.6b10169
Munnik, P., de Jongh, P. E., and de Jong, K. P. (2015). Recent Developments in the Synthesis of Supported Catalysts. Chem. Rev. 115, 6687–6718. doi:10.1021/cr500486u
Neitzel, A., Figueroba, A., Lykhach, Y., Skála, T., Vorokhta, M., Tsud, N., et al. (2016). Atomically Dispersed Pd, Ni, and Pt Species in Ceria-Based Catalysts: Principal Differences in Stability and Reactivity. J. Phys. Chem. C 120, 9852–9862. doi:10.1021/acs.jpcc.6b02264
Nistor, R. A., Newns, D. M., and Martyna, G. J. (2011). The Role of Chemistry in Graphene Doping for Carbon-Based Electronics. ACS Nano 5, 3096–3103. doi:10.1021/nn200225f
Pan, Y., Liu, S., Sun, K., Chen, X., Wang, B., Wu, K., et al. (2018). A Bimetallic Zn/Fe Polyphthalocyanine‐Derived Single‐Atom Fe‐N 4 Catalytic Site:A Superior Trifunctional Catalyst for Overall Water Splitting and Zn-Air Batteries. Angew. Chem. Int. Ed. 57, 8614–8618. doi:10.1002/anie.201804349
Qu, Y., Li, Z., Chen, W., Lin, Y., Yuan, T., Yang, Z., et al. (2018). Direct Transformation of Bulk Copper into Copper Single Sites via Emitting and Trapping of Atoms. Nat. Catal. 1, 781–786. doi:10.1038/s41929-018-0146-x
Reske, R., Mistry, H., Behafarid, F., Roldan Cuenya, B., and Strasser, P. (2014). Particle Size Effects in the Catalytic Electroreduction of CO2 on Cu Nanoparticles. J. Am. Chem. Soc. 136, 6978–6986. doi:10.1021/ja500328k
Rodriguez, J. A., and Goodman, D. W. (1992). The Nature of the Metal-Metal Bond in Bimetallic Surfaces. Science 257, 897–903. doi:10.1126/science.257.5072.897
Sasaki, K., and Maier, J. (1999). Low-temperature Defect Chemistry of Oxides. I. General Aspects and Numerical Calculations. J. Appl. Phys. 86, 5422–5433. doi:10.1063/1.371541
Shao, M., Peles, A., and Shoemaker, K. (2011). Electrocatalysis on Platinum Nanoparticles: Particle Size Effect on Oxygen Reduction Reaction Activity. Nano Lett. 11, 3714–3719. doi:10.1021/nl2017459
Wang, A., Li, J., and Zhang, T. (2018a). Heterogeneous Single-Atom Catalysis. Nat. Rev. Chem. 2, 65–81. doi:10.1038/s41570-018-0010-1
Wang, J., Li, Z., Wu, Y., and Li, Y. (2018b). Fabrication of Single-Atom Catalysts with Precise Structure and High Metal Loading. Adv. Mater. 30, 1801649. doi:10.1002/adma.201801649
Wang, Y., Chen, Z., Han, P., Du, Y., Gu, Z., Xu, X., et al. (2018c). Single-Atomic Cu with Multiple Oxygen Vacancies on Ceria for Electrocatalytic CO2 Reduction to CH4. ACS Catal. 8, 7113–7119. doi:10.1021/acscatal.8b01014
Wei, H., Huang, K., Wang, D., Zhang, R., Ge, B., Ma, J., et al. (2017). Iced Photochemical Reduction to Synthesize Atomically Dispersed Metals by Suppressing Nanocrystal Growth. Nat. Commun. 8, 1490. doi:10.1038/s41467-017-01521-4
Wei, S., Ang, L., Jin-Cheng, L., Zhi, L., Wenxing, C., Yue, G., et al. (2018). Direct Observation of Noble Metal Nanoparticles Transforming to Thermally Stable Single Atoms. Nat. Nanotechnol. 13, 856–861. doi:10.1038/s41565-018-0197-9
Won-Jun, J., Jae-Oh, S., Hak-Min, K., Seong-Yeun, Y., and Hyun-Seog, R. (2018). A Review on Dry Reforming of Methane in Aspect of Catalytic Properties. Catal. Today 324, 15–26. S092058611830083X-. doi:10.1016/j.cattod.2018.07.032
Xiao, M., Gao, L., Wang, Y., Wang, X., Zhu, J., Jin, Z., et al. (2019). Engineering Energy Level of Metal Center: Ru Single-Atom Site for Efficient and Durable Oxygen Reduction Catalysis. J. Am. Chem. Soc. 141, 19800–19806. doi:10.1021/jacs.9b09234
Yan, C., Li, H., Ye, Y., Wu, H., Cai, F., Si, R., et al. (2018). Coordinatively Unsaturated Nickel-Nitrogen Sites towards Selective and High-Rate CO2electroreduction. Energ. Environ. Sci. 11, 1204–1210. doi:10.1039/c8ee00133b
Yang, L., Cheng, D., Xu, H., Zeng, X., Wan, X., Shui, J., et al. (2018). Unveiling the High-Activity Origin of Single-Atom Iron Catalysts for Oxygen Reduction Reaction. Proc. Natl. Acad. Sci. USA. 115, 6626–6631. doi:10.1073/pnas.1800771115
Yang, X.-F., Wang, A., Qiao, B., Li, J., Liu, J., and Zhang, T. (2013). Single-Atom Catalysts: A New Frontier in Heterogeneous Catalysis. Acc. Chem. Res. 46, 1740–1748. doi:10.1021/ar300361m
Yuyao, Y., and Kummer, J. T. (1987). Low-concentration Supported Precious Metal Catalysts Prepared by thermal Transport. J. Catal. 106, 307–312. doi:10.1016/0021-9517(87)90237-5
Zhang, C., Chen, L., Cheng, H., Zhu, X., and Qi, Z. (2016). Atomically Dispersed Pd Catalysts for the Selective Hydrogenation of Succinic Acid to γ-butyrolactone. Catal. Today 276, 55–61. doi:10.1016/j.cattod.2016.01.028
Zhang, E., Wang, T., Yu, K., Liu, J., Chen, W., Li, A., et al. (2019a). Bismuth Single Atoms Resulting from Transformation of Metal-Organic Frameworks and Their Use as Electrocatalysts for CO2 Reduction. J. Am. Chem. Soc. 141, 16569–16573. doi:10.1021/jacs.9b08259
Zhang, H., Hwang, S., Wang, M., Feng, Z., Karakalos, S., Luo, L., et al. (2017). Single Atomic Iron Catalysts for Oxygen Reduction in Acidic Media: Particle Size Control and Thermal Activation. J. Am. Chem. Soc. 139, 14143–14149. doi:10.1021/jacs.7b06514
Zhang, L., Ren, Y., Liu, W., Wang, A., and Zhang, T. (2018). Single-Atom Catalyst: A Rising Star for Green Synthesis of Fine Chemicals. Natl. Sci. Rev. 5, 653–672. doi:10.1093/nsr/nwy077
Zhang, R., Jiao, L., Yang, W., Wan, G., and Jiang, H.-L. (2019b). Single-atom Catalysts Templated by Metal-Organic Frameworks for Electrochemical Nitrogen Reduction. J. Mater. Chem. A. 7, 26371–26377. doi:10.1039/c9ta10206j
Zhang, W., and Zheng, W. (2016). Single Atom Excels as the Smallest Functional Material. Adv. Funct. Mater. 26, 2988–2993. doi:10.1002/adfm.201600240
Zhang, Y., Guo, L., Tao, L., Lu, Y., and Wang, S. (2019c). Defect‐Based Single‐Atom Electrocatalysts. Small Methods 3, 1800406. doi:10.1002/smtd.201800406
Keywords: single atom catalysts, synthesis, nanoparticles, atomization, stability
Citation: Zhang A, Zhou M, Liu S, Chai M and Jiang S (2021) Synthesis of Single-Atom Catalysts Through Top-Down Atomization Approaches. Front. Catal. 1:754167. doi: 10.3389/fctls.2021.754167
Received: 06 August 2021; Accepted: 04 October 2021;
Published: 20 October 2021.
Edited by:
Stefania Specchia, Politecnico di Torino, ItalyReviewed by:
Biswarup Chakraborty, Indian Institute of Technology Delhi, IndiaGui-Rong Zhang, Tianjin Polytechnic University, China
Copyright © 2021 Zhang, Zhou, Liu, Chai and Jiang. This is an open-access article distributed under the terms of the Creative Commons Attribution License (CC BY). The use, distribution or reproduction in other forums is permitted, provided the original author(s) and the copyright owner(s) are credited and that the original publication in this journal is cited, in accordance with accepted academic practice. No use, distribution or reproduction is permitted which does not comply with these terms.
*Correspondence: Shengjuan Jiang, amlhbmdzajE5ODZAc2luYS5jb20=