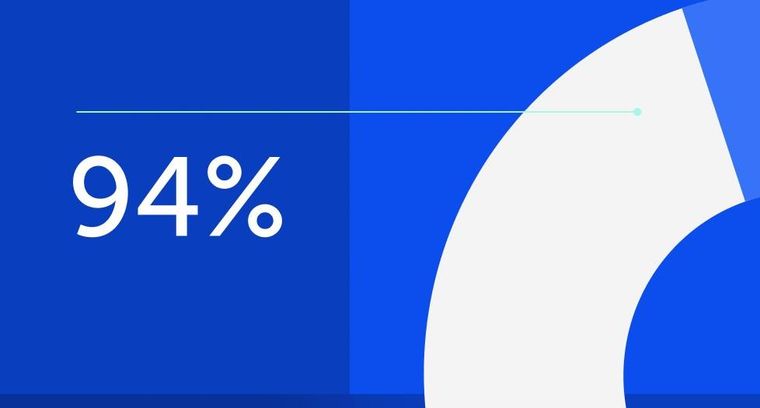
94% of researchers rate our articles as excellent or good
Learn more about the work of our research integrity team to safeguard the quality of each article we publish.
Find out more
ORIGINAL RESEARCH article
Front. Conserv. Sci., 26 March 2025
Sec. Global Biodiversity Threats
Volume 6 - 2025 | https://doi.org/10.3389/fcosc.2025.1487428
This article is part of the Research TopicImpacts of Anchor Scour, Vessel Moorings and Associated Infrastructure on Marine HabitatsView all articles
International shipping is the backbone of the global economy with ~80% of the world’s trade (by volume) transported by ship. The potential environmental impacts of this multi-billion-dollar industry have received considerable attention, particularly emissions into air and sea. Many of these large commercial vessels lay at anchor for extended periods while awaiting their turn to enter port, and yet the impacts associated with anchoring remain virtually unexamined. Anchors can exceed 20 tonnes, with chains up to hundreds of metres in length and individual links weighing up to 200kg; there is potential for significant effects on seafloor biota where anchoring is concentrated. Filling knowledge gaps in deep-water wave-exposed environments is logistically challenging and expensive. To do so we used sediment grabs collected offshore from the Port of Newcastle (SE Australia) – the world’s largest coal export terminal – to sample infaunal assemblages in anchor-affected locations relative to reference locations (30 to 55m water depth). Polychaetes and crustaceans were the most abundant biota in the samples (~85%), whereas molluscs were very low in abundance (<3%), despite being well represented in terms of diversity (11 families of bivalves and 9 families of gastropods). Invertebrate abundance almost doubled in areas exposed to anchoring compared to reference areas. In contrast, invertebrate diversity declined with increasing anchoring activity, however this relationship was weak. Importantly, we observed major shifts in the overall invertebrate assemblage at anchored-affected locations – with reductions in suspension feeders mirrored by increases in scavengers and predators. We assert that suspension feeders were negatively impacted by sediment mobilisation or direct physical damage from anchor chains, while opportunistic scavengers and mobile predators benefitted from this disturbance. We contend that anchor disturbance is a global issue given burgeoning marine trade. Data on the effects of anchoring are urgently required to better inform the management of environments regularly used as anchorages.
Marine soft sediments (including silt, mud, sand and gravel) are the most common ocean habitat worldwide, accounting for some 80% of the seafloor (Lenihan and Micheli, 2001). Often dismissed as featureless with little topographic relief and low biodiversity, soft sediment habitats are inhabited by burrowing ecosystem engineers; organisms which create diverse microhabitats, including aerobic environments and refugia, for a wide range of associated species (Gray, 1974; Woodin, 1978; Meadows and Meadows, 1991; Reise, 2002). In addition, the feeding activities of vertebrates and invertebrates commonly disturb sediments by creating pits or depressions (Oliver et al., 1984; Thrush et al., 1991; Lee, 2010). Disturbance is therefore an ecologically important structuring agent in soft sediments, particularly in unvegetated nearshore environments. In high-energy, open coast environments these habitats are also frequently exposed to abiotic sediment disturbance from waves, storms and fluvial discharge. These disturbances affect organisms both directly and indirectly via shifts in sediment grain size and nutrients (Oliver et al., 1980; Hall, 1994). Overlying these biotic and abiotic drivers of sediment disturbance and habitat structure are anthropogenic disturbances. For example, physical impacts associated with extractive fishing practices in soft sediments are well documented and include the elimination of epibiota as well as the removal of habitat complexity (Collie et al., 1997; Watling and Norse, 1998). Impacts from other anthropogenic disturbances such as anchoring large commercial vessels, however, are less well documented.
International shipping carries at least 80% of world trade. This industry has an international fleet of more than 68,000 vessels (DOT, 2024), and is tightly regulated (UNCTAD, 2023). Many of these commercial vessels anchor as they await their turn to berth. Soft sediments near ports are often used for anchorage as this habitat is extensive and offers a good holding substratum for heavy-tonnage vessels. In contrast to many of the internationally regulated impacts relating to shipping, including minimising pollution (including noise), biofouling, ballast water exchange and most recently vessel emissions relating to greenhouse gases (International Chamber of Shipping, 2008; UNCTAD, 2023), the effects of anchoring large commercial vessels on unvegetated soft sediments have not been scrutinised (Davis and Broad, 2016). It is evident from observations of cruise ships in the Caribbean, that heavy-tonnage vessels at anchor can be highly destructive (Davis, 1977; Smith, 1988; Flynn and Forrester, 2019; Davis et al., 2022) but much of our understanding of impacts on soft sediments relies on data from recreational vessels (Macolino et al., 2019; Broad et al., 2020). Numerous studies show dramatic damage to habitat and associated biota due to disturbance from shallow water anchoring and mooring (e.g. Herbert et al., 2009; Demers et al., 2013; see references within Broad et al., 2020) with negative outcomes for vagile taxa such as fishes (Kiggins et al., 2018; Lanham et al., 2018). Unfortunately, experimental examination of the effects resulting from the anchoring of recreational vessels on fauna in soft sediment are rare (but see Backhurst and Cole, 2000; Vázquez-Luis et al., 2015), while assessments of the impacts on fauna from high-tonnage commercial vessels anchoring on soft sediment in deeper water (>30m) are non-existent.
Physical disturbance associated with anchoring commercial vessels, many exceeding 300m in length, we predict to be significant. As vessels swing at anchor with shifting winds and tides, lengths of heavy anchor chain are dragged across the seafloor, scouring it and displacing large quantities of sediment (Watson et al., 2022). In addition to the crushing of epifauna and infauna, this movement will also mobilise sediments, changing sediment grain size as well as increasing turbidity and the probability of smothering biota (Davis et al., 2016). Shifts in the trophic structure of assemblages may therefore also be expected, with increases in scavenger-dominated guilds (Backhurst and Cole, 2000). We note though that fauna associated with soft sediment habitat are frequently exposed to disturbance (Probert, 1984) and therefore may be relatively resilient to anthropogenic activities such as anchoring.
Here we examine the effects of anchoring by commercial heavy-tonnage vessels (i.e. bulk carriers ~ 300m in length) on offshore (>5km from the shoreline) soft sediment environments in 30-55m of water. We use the world’s largest coal export terminal as a case study; the Port of Newcastle (SE Australia), which receives more than 2000 vessel visits per year. Anchoring in this region is not tightly regulated, but is at the discretion of the ship’s master. There are no designated anchorages, but it is recommended that vessels anchor three nautical miles offshore. The aim of this study was to examine soft sediment infaunal assemblages exposed to anchoring by heavy-tonnage vessels relative to sites where anchoring did not occur. We used vessel positional information (AIS – Automated Information Systems - data) to infer levels of anchor activity (i.e. differentiating highly disturbed and less disturbed areas within a defined anchorage). In our assessment we also accounted for other variables that may influence the infaunal assemblage, such as depth and sediment grain size. We believe that this is the first attempt to examine the effects of anchoring on soft-sediment macrobenthic assemblages by commercial heavy-tonnage vessels. This is important as the shipping fleet is expected to grow substantially, with world trade volumes increasing on average at more than 7% annually until very recently (UNCTAD, 2023). Our research contributes to UN Sustainable Development Goal SDG14 - Conserve and sustainably use the oceans, seas and marine resources for sustainable development (UNDP, 2019) and we hope will lead to improved management of global anchoring activities.
The Port of Newcastle is on Australia’s SE coast 100km north of Sydney. It is the world’s largest coal exporting Port, moving in excess of 144 million tonnes of coal annually with >2,000 ship movements per year (data for 2023 https://www.portofnewcastle.com.au/wp-content/uploads/2024/05/PON_2023_AnnualTradeReport.pdf, accessed 3 Feb. 2025). The Port began commercial operations in 1799, with the shipment of lime (https://www.portofnewcastle.com.au/the-port/history-and-heritage/, accessed 3 Feb 2025). In June 2010 the Port of Newcastle introduced a vessel arrival system – for safety and supply chain reasons (Heaver, 2021). This drastically reduced the number of vessels waiting at anchor from a maximum of 70 ships anchored at any one time. It also reduced time at anchor, which averaged 11.1 days in 2010 to just 2.7 days in the 32 months from September 2012 (Davis et al., 2016; Heaver, 2021). Anchoring still occurs in waters under federal jurisdiction (beyond three nautical miles at 30-60 m depth) while vessels wait their turn to berth and load; the roadstead or area considered to provide safe anchorage extends at least 50km in a linear fashion from the Hunter River to the south (see Figure 4 in Davis et al., 2016). We reasoned that the heavily industrialised Port of Newcastle may impact the invertebrate assemblage. To assess the potential impacts of anchoring on invertebrate habitat, we established three locations within the anchor roadstead (North, Central and South) which included anchored and reference (non-anchored) areas (Figure 1). We used a Smith-MacIntyre grab (0.0625m2) to sample anchor-affected and reference (non-anchor) locations within each region (n=7) for a total of 42 grabs between 31 May and 2 June 2016. Grab material was processed in accord with Przeslawski et al. (2024). Grabs penetrated to a depth of ~10 cm, although this was contingent on the bulk density of the sediment. A sub-sample of sediment was removed from the grab and frozen on board for later grain-size analysis (see below). The remaining grab sample was wet sieved (500 µm) on board and resultant biota immediately fixed in ethanol (70%) then returned to the laboratory for processing.
Figure 1. Heatmap of anchoring activity within the Newcastle anchoring roadstead within the period Sept. 2012 – July 2016. Each point or circle represents a grab sample with the total anchored days for this period depicted by circle size. Note the three locations North (N and RN), Central (C and RC) and South (S and RS) with reference areas (R) lacking anchoring activity. Insets focus on each of the anchor-affected locations – N, C, S. Note that no anchoring was observed in the reference areas and that anchoring activity extends for at least 25km and may extend to 50km south of the Port (Davis et al., 2016).
On return to the laboratory, animals were sorted from sediment under a dissecting microscope, enumerated and identified to the lowest taxonomic level by an experienced benthic biologist. Vouchers of the Operational Taxonomic Units were then lodged at the Australian Museum Sydney. It is worth emphasising that infauna from non-vegetated sediments in >35m are not well characterised in this region, presenting a challenge to identify the feeding guild to which they belong.
We investigated whether anchoring was an important driver of impacts on the infauna. We interrogated monthly AIS data (ships position) offshore of Newcastle (Sept. 2012 – July 2016) to assess the spatial and temporal extent of anchor activity and overlaid this information on bathymetric charts from echosounder surveys. AIS data, generated hourly, were acquired from the Australian Maritime Safety Authority (www.amsa.gov.au: monthly data accessed January 15, 2021) and imported to ArcMap. Data were treated using the approach of Deter et al. (2017), applying a range of filters including vessel speed, type, and residence time (≥6 h) to determine those vessels laying at anchor. Filtered GPS points were then used to define a polygon (‘convex-hull’ function in ArcMap) for each vessel and for each anchoring event. Davis et al. (2022) have used anchor polygons to calculate anchor activity at another location and provide a depiction of their use (see their Figure 2). The length of chain in contact with the seabed while a vessel is at anchor is unknown, as is the exact location of the anchor itself (not recorded in AIS), although we assumed that the position of the anchor was within the anchor ‘polygon’. The location of the vessel’s GPS beacon is also uncertain and, for the purposes of this approach, it is assumed to be located near the helm, i.e. at the stern. To accommodate these unknowns and so as not over-estimate the extent of anchor scour, the area of the defined anchor polygon was then systematically reduced, in a concentric manner, by 1/3 in keeping with the approach of Deter et al. (2017). The processing of the AIS data created two predictors related to anchoring. The first, ‘anchoring days’ was a measure of anchor intensity, the total number of days at which vessels lay at anchor at the grab locations across the entire three year 11 month period of monitoring. The second metric was ‘treatment’, which was a categorical predictor of whether the grab locations were anchor-affected or outside of the anchoring zone i.e. reference areas.
Figure 2. Important predictors of macrobenthic faunal abundance and diversity across the study region. (A, B) variables associated with the top generalised additive model for predicting the mean total abundance of fauna; (C, D) the variables associated with predicting macroinvertebrate diversity (richness); (E) relative importance scores of explanatory variables to predict total abundance and richness. Note, the most parsimonious model for diversity only contains the predictor % mud. Error bars and banding represent 95% confidence intervals.
We assembled a dataset of other abiotic measures likely to influence the biotic data to be included as covariates in our statistical analyses (McArthur et al., 2010). The abiotic data set comprised three elements: (i) depth, (ii) sediment grain size and (iii) variables related to the spatial structure and location of samples. Depth (m) was recorded in situ using the research vessel sounder for each grab. For the sediment analyses, a subsample of sediment (~100g) was removed from the grab on the vessel and frozen to allow subsequent determination of sediment grain size in the laboratory. Sediment was processed at Geoscience Australia following the procedure outlined in Przeslawski et al. (2024). The analytical methods included wet sieve separation into mud, sand and gravel fractions, as well as laser granulometry of the mud fraction (1–63 um diameter). Digital grain size imagery analysis (Camsizer) was undertaken on the sand (63–2000 um diameter) and gravel fractions (2000–16000 um diameter). The combined Camsizer and Laser datasets were summarily binned into 1 phi intervals and normalized to 100%. Finally, spatial covariates included the factor ‘Location’ to account for the spatial clustering of samples in the northern, central and southern regions (Figure 1), while Latitude of each grab sample was included as a proxy for distance to the entrance of the Hunter River.
Prior to statistical analysis, data exploration was performed to examine potential outliers, homogeneity and collinearity of explanatory variables. First, we used histogram plots to visualise the distribution of each abiotic variable. The variables ‘anchor days’, ‘% mud’ and ‘% gravel’ were square root transformed to improve homogeneity (see Supplementary Table 1). Examination of the sediment data revealed that % sand and % gravel were highly negatively correlated (r= -0.997), so we elected to use % gravel as a proxy, while % mud did not covary with % sand or % gravel. Importantly, several explanatory variables likely to influence the biotic assemblage were collinear. For example, depth was correlated with the two anchoring variables and % mud (Supplementary Table 2).
To overcome issues of collinearity we used a full-subsets information theoretic approach (Burnham and Anderson, 2004). Specifically, we employed full-subsets generalised additive modelling following Fisher et al. (2018). This approach constructs all possible combinations of models except for models containing collinear variables. Model selection criterion such as Akaike information criterion (AIC), can then be used to determine a ‘best’ or most ‘parsimonious’ model and if multiple candidate models have high AIC weight, information theoretic approaches allow model averaging and multimodel inference (Fisher et al., 2018). Further, by summing model weights for each explanatory variable, the relative importance of different variables can be assessed (Burnham and Anderson, 2004). To assess impacts of anchoring on the total abundance and diversity of macrobenthic taxa we fitted a series of GAMs using the function; FSSGAM 1.11 (Fisher et al., 2018) in R (R Core Development Team, 2021). GAMs were chosen over generalised linear models to examine potentially non-linear relationships using smoothing splines.
A negative binomial distribution was fitted for the total abundance models, while a gaussian distribution was fitted for species richness models. The maximum number of explanatory variables for each model was limited to three and all continuous variables were fitted with smoothing splines with the number of knots k = 4. These parameters were set to prevent overfitting and create conservative, ecologically interpretable models (Fisher et al., 2018). Explanatory variables with Pearson’s correlation coefficients > 0.28 could not be included in the same model. Models were compared using Akaike information criterion corrected for small sample sizes (AICc). The model with the lowest AICc or models within ± 2 AICc units of the lowest AICc score were considered the ‘top’ model(s) (Burnham and Anderson, 2004). If there were multiple ‘top’ models the relative importance of each explanatory variable was determined by summing model weights (Burnham and Anderson, 2004). This process assisted with disentangling the relative importance of collinear explanatory variables. Both ‘anchor days’ and anchor ‘treatment’ were included as candidate predictors in the full-subset GAMs to assess the relative importance of anchoring intensity versus the presence/absence of anchoring activity on macrobenthic fauna.
A multivariate PERMANOVA was used to assess the influence of anchoring on the macrobenthic assemblage. The PERMANOVA included the factor Location (Fixed with three levels: North, Central and South) to account for the spatial layout of the sampling sites as well as the anchor ‘treatment’. This model design was chosen as the full subset analysis identified these factors as the most important for the total abundance of macrobenthic fauna. Assemblage data were based on a Bray-Curtis similarity matrix and were fourth root transformed to account for common taxa. The PERMANOVA was performed in R using the ‘adonis’ function from the ‘vegan’ package with the number of permutations set to 9999 (Oksanen et al., 2025). This package was also used to generate a non-metric Multidimensional Scaling plot (nMDS) to visualise differences in the assemblage between replicates from anchor-affected and anchor-free locations. The nMDS included ordinations of fifteen taxa that contributed significantly to differences in the composition of the assemblage.
To assess anchoring effects on the abundance of individual taxa, we selected a small number of taxa that were present in >35% of all samples and could be unequivocably placed within the filter feeder or scavenger/predator guilds. We assessed the impacts of anchoring using GAMs including ‘location’ and ‘anchor treatment’ as factors. As with the multivariate analysis, this model design was employed as the full subset analysis identified these factors as the most important in explaining the variation in total abundance of the assemblage. All models were initially fitted with a Poisson distribution then checked for overdispersion. If overdispersion was observed models were then fitted with a negative binomial distribution.
The sample locations (anchor-affected and reference areas) were dominated by coarse sands; sand constituted from 72.5 to 99.2% of the sediment sample, while gravel composed up to 27.4%. Biologically, a total of 138 taxa (Operational Taxonomic Units) were recorded from 42 grabs, with more than 3300 individuals counted; 37 taxa were documented just once (Supplementary Table 3). Predictably, the fauna was dominated by polychaetes, amphipods, along with tanaid crustaceans (>77% of the sample). Molluscs were diverse in the samples, with 11 families of bivalves and 9 families of gastropods, but their abundance was limited, constituting <3% of the sample. Just ten taxa accounted for almost two thirds (~65%) of the overall abundance recorded. Anchoring activity was monitored for 3yrs and 11 months (1430 days). Over this period, no anchoring occurred at reference locations. Where anchoring did occur, the minimum time period was 26 hrs to a maximum of almost 35 days (Supplementary Table 4). Anchored areas averaged 11.9 (± 8.3) days (standard deviation) of anchor disturbance over the entire period of monitoring. Where anchoring did occur the maximum time since the last anchoring event was 45 days (Supplementary Table 4). As evident in Figure 1, four of the anchor-affected lacked anchoring and were coded as anchor-free in our subsequent analysis.
Anchoring had a clear impact on the total abundance of macrobenthic taxa, appearing in two of the three ‘best’ models. The categorical predictor of anchoring (treatment) displayed the second highest variable importance score after location (Table 1; Figure 2). In reference (anchor-free) areas the mean total abundance was approximately 57 individuals per grab sample, which increased to ~100 individuals per grab sample in anchor-affected locations (Figure 2). Location was the most important predictor of the total abundance of macrobenthic fauna (Table 1; Figure 2), with greater abundances observed in the north compared to the south (Figure 2). In contrast to total abundance, the anchoring variables were not strong predictors of diversity. Although, increasing anchor intensity (anchor days) resulted in a decrease in diversity from ~25 to ~20 taxa, there was substantial variation around the predicted relationship (Figure 2). The most parsimonious model for diversity only included the variable % mud, where samples with greater proportion of mud displayed greater diversity (Table 1; Figure 2). Importantly, the full-subsets approached identified anchoring activity variables over depth as the more important predictor of the macrobenthic assemblage across the study region.
Table 1. Top generalised additive models (GAMs) predicting the total abundance and richness of the macrobenthic assemblage.
The entire assemblage showed a statistically significant shift in response to the treatment variable – anchoring (Table 2; Figure 3). Ordinations indicate the shift in the overall assemblage was consistent with a reduction in filter-feeders at sites exposed to anchoring while the scavenger and predatory guild increased in abundance in these areas (Figure 3). Of the 15 OTU’s (taxa) that were significantly affected by anchoring, ten increased in anchor affected locations, while the remaining five mirrored this pattern in a marked dichotomy within the assemblage. We could not confidently ascribe each of these taxa to a feeding guild and so we focussed on six taxa for which we were confident in their assignment. Generalised additive models confirmed that the presence of anchoring was an important predictor of the abundance of the filter feeding spionid polychaetes – Prionospio sp. and Dipolydora sp. The abundance of Prionospio sp. and Dipolydora sp. was 3.3 and ~20 times greater in anchor-free reference locations compared to the anchor-affected locations, respectively (Figure 4). In sharp contrast, anchoring had no effect on the abundance of the filter feeding motile bryozoan – Cupuladria guineensis, with similar abundances across anchor-affected and reference locations (Figure 4).
Table 2. PERMANOVA table of multivariate responses of invertebrates to Anchoring (Treatment) and location.
Figure 3. Non metric multidimensional scaling (nMDS) plot of infaunal assemblages among grabs in anchor- affected (black circles) and reference (anchor-free) locations (grey circles). Vectors depict taxa significantly influenced by anchoring.
Figure 4. Responses of individual infaunal taxa from the filter feeding guild to anchoring – Predicted mean abundance of filter feeding spionid polychaetes – (A) Dipolydora sp. 1 and (B) Prionospio sp. 1. along with the mobile bryozoan (C) Cupuladria guineensis, from generalised additive models. Error bars are 95% confidence intervals.
Shifts in the filter-feeding guild were reflected in changes in the scavenger/predator guild. Generalised additive models confirmed that anchoring was an important predictor of the abundance of several key taxa comprising the scavenger/predator guild. The presence of anchoring increased the abundance of the polychaete Onuphis c.f. holobranchiata and amphipods in the families Melitidae and Aoridae. The abundance of O. c.f. holobranchiata, Melitidae and Aoridae were ~2.2, ~7.5 and ~12 times greater in anchor-affected locations compared to reference locations (Figure 5).
Figure 5. Responses of individual infaunal taxa from the scavenger/predator guild to anchoring – Predicted mean abundance for (A) the polychaete Onuphis c.f. holobranchiata, (B) a melitid amphipod and (C) an aorid amphipod from generalised additive models. Error bars are 95% confidence intervals.
The anchoring of heavy-tonnage commercial vessels resulted in marked changes in soft sediment assemblages offshore from the Port of Newcastle in southeastern Australia. We saw marked increases in invertebrate abundance at anchor-affected locations. In contrast, anchoring had a weak negative effect on the diversity of the macrobenthic assemblage. Importantly, we saw shifts in feeding guilds in the assemblage, with reductions in suspension-feeding polychaetes, mirrored by increases in scavengers and predators at anchor-affected locations. The location of the study sites and % of mud were also strong predictors of the macrobenthic assemblage, with a clear north to south gradient in abundance, as well as increasing macroinvertebrate diversity with mud content. Although depth was correlated with anchoring activity in our analysis, our full subsets information theoretic approach suggests that anchoring is the more important factor influencing the macrobenthic assemblage across the study region.
We anticipated reductions in the diversity and abundance of the invertebrate assemblage associated with disturbance from anchor scour; akin to those impacts seen with demersal trawls (Frid et al., 1999). Indeed, in their global meta-analysis of experimental trawl impacts Sciberras and co-workers (2018) detail dramatic and statistically significant reductions in abundance and diversity following a single pass by fishing gear. Further, their meta-analysis revealed that reductions in biota were intensified with increasing depth of penetration into sediment – but rarely did any fishing technique exceed a penetration depth of 20cm. Anchors from heavy-tonnage vessels in unconsolidated sediment can excavate up to 80 cm, and penetration may even exceed 5m (see Figure 6 in Broad et al., 2020; Watson et al., 2022). In contrast, we observed only a weak negative effect of anchoring on invertebrate diversity and it was a function of anchoring intensity. Diversity rose at low anchoring intensity, plateaued and then fell by around 25% as anchor intensity increased (Figure 2). Such shifts in diversity are consistent with Connell’s Intermediate Disturbance Hypothesis (Connell, 1978). The dramatic increases in abundance we observed are more difficult to explain, but field tests on infaunal assemblages also report increases in abundance at intermediate levels of disturbance (Huxham et al., 2000). It is worth emphasising that the maximum span of time vessels at anchor over one of our sample points was just under 35 days, equating to <2.5% of the 1430 days we monitored anchor activity.
It has long been known that biogenic disturbance, associated with the burrowing and foraging activities of biota, operates over relatively small scales; usually centimetres to meters (Probert, 1984). Importantly, these frequent localised disturbances create heterogeneity and likely act to maintain diversity in soft sediment habitats. In contrast, anchoring activities occur over much larger scales – a single anchoring event in sediment may displace 2800 m3 of material (Watson et al., 2022). Estimates of the area disturbed by anchoring and mooring activities are in the order of square kilometres. Watson et al. (2020) estimate that anthropogenic activities disturb 6.4 km2 of seafloor in a single inlet in New Zealand, while in SE Australia, Davis et al. (2022) calculated non-overlapping anchor disturbance of ~88 km2 near Port Kembla in the 8 years to 2020. Rates of recovery following disturbance are expected to be rapid (<1 year), particularly for infauna with short lifespans such as polychaetes and crustaceans (Sciberras et al., 2018). However, on the removal of moorings in shallow water in the UK, Herbert et al. (2009) failed to see assemblage recovery after 15 months relative to controls that had never had moorings. Rates of recovery in our system remain unknown and the fauna poorly characterised; a high proportion (>50%) of the fauna is likely undescribed (Coleman et al., 1997). It may be premature to suggest that assemblages recover rapidly from such large-scale disturbance.
High wave energy open coastlines, such as our Newcastle study site, usually possess coarse sediments and are dominated by suspension feeding invertebrates (Byers and Grabowski, 2014). In anchor-affected locations, we saw a shift away from filter-feeders with, for example, marked reductions in the abundance of two species of spionid polychaetes. Feeding in close relatives of the spionids we encountered (Dipolydora sp. 1 and Prionospio sp. 1) can switch between filter feeding and deposit feeding, but in the habitat we examined are expected to be filter feeders (Jumars et al., 2015). We contend that the mobilisation of sediments from anchor scour, particularly the presence of fines, acts to smother or interfere with the feeding activities of these suspension feeders (Magris and Ban, 2019). The striking exception to reductions in suspension feeders was a mobile bryozoan - Cupuladria guineensis; this bryozoan was commonly encountered at both anchor-affected and reference areas. Its ability to reproduce clonally via fragmentation as well as clear sediment from its surface may explain why, as a suspension-feeder, it demonstrated such resilience to anchor activity in this region (Cook et al., 2018).
At the initiation of this project, we predicted a shift in the assemblage to one dominated by scavenging organisms. We anticipated this response as organisms are expected to be damaged and crushed by ships anchors and chains. In anchor-affected areas, we did indeed see increases in scavenging polychaetes and carrion feeding isopods and amphipods. It should be emphasised that infaunal sampling, however, will only provide a small snapshot of the large and diverse scavenging guild that characterises the open coast. The deployment of baited traps in eastern Australia has highlighted the presence of a rich epibenthic scavenging fauna, dominated by crustaceans – particularly cirolanid isopods (Keable, 1995; Lowry and Smith, 2003). Although our findings are consistent with a shift to scavengers, in the absence of data from baited traps it is premature to assert confidently that increases in the scavenging guild have occurred. It is also worth noting that the large hermit crab Strigopagurus strigimanus is a common epibenthic scavenger at the depths over which we sampled (Davis, unpublished data) but is unlikely be taken in infaunal sampling and was not detected in our samples.
Our work challenges the assumption that infaunal assemblages in high energy environments are relatively immune to disturbance associated with anchor scour, however, much more work remains. Foremost among this research is the need to generate additional datasets in other parts of the globe or preferably a manipulative experimental examination of anchoring impacts in these environments. Our data highlights a shift to scavengers in the assemblage, but this would be much better understood by deploying baited traps. Finally, the rate at which these assemblages recover from anchoring is also worthy of attention. We contend that this research will assist the shipping industry, considering the global scale of anchoring, in developing more sustainable practices.
Anchoring is a common practice worldwide and with burgeoning world trade is set to increase dramatically. Impacts of anchoring, particularly large commercial vessels (e.g. bulk carriers) are only now becoming clear (e.g. Broad et al., 2023), but impacts on some habitats – such as unvegetated soft sediments – remain to be critically examined. Our focus on this habitat revealed that anchoring increased infaunal abundance while slightly reducing diversity. Importantly though, the infaunal assemblage shifted away from suspension feeders to an assemblage dominated by predators and scavengers. Further research with a focus on broader community-wide patterns, such as impacts on nutrient cycling and biogeomorphic development, needs to be prioritised. The immediate response of managers should be to reduce anchoring or at least the anchoring footprint; a vessel arrival system and the use of designated anchorages offer advantages in these regards. Charting a more sustainable path for the shipping industry will ensure the conservation of important marine resources.
The original contributions presented in the study are included in the article/Supplementary Material, further inquiries can be directed to the corresponding author/s.
The manuscript presents research on animals that do not require ethical approval for their study. Collections were made in accordance with NSW Scientific Collection Permit F95/269-8.2.
AD: Conceptualization, Funding acquisition, Project administration, Supervision, Writing – original draft, Writing – review & editing. AB: Conceptualization, Investigation, Resources, Writing – review & editing. CS: Conceptualization, Funding acquisition, Investigation, Methodology, Writing – review & editing. CW: Formal Analysis, Visualization, Writing – review & editing. RP: Data curation, Investigation, Resources, Writing – review & editing, Validation. WN: Data curation, Investigation, Resources, Validation, Writing – review & editing. WM: Data curation, Investigation, Resources, Validation, Writing – review & editing. FK: Data curation, Investigation, Resources, Validation, Writing – review & editing. BM: Data curation, Investigation, Resources, Visualization, Writing – review & editing. TI: Conceptualization, Funding acquisition, Investigation, Methodology, Project administration, Resources, Supervision, Validation, Writing – review & editing. SO’HM: Investigation, Visualization, Writing – review & editing. MR: Formal Analysis, Investigation, Validation, Visualization, Writing – review & editing.
The author(s) declare that financial support was received for the research and/or publication of this article. Three of us (AB, CS and CW) were supported by an Australian Government Research Training Program (AGRTP) scholarship.
We thank the Global Challenges Program, University of Wollongong for supporting our anchoring research from its inception. The crew of the RV Bombora – Michael Sutherland, Michelle Linklater and Jeff Miller - are acknowledged for their assistance in the field. Shona Marks (Benthic Australia Pty Ltd) is thanked for assessing the biota, while staff at the Australian Museum (Sydney), particularly Dr Claire Rowe, provided Catalogue data in a timely fashion.
The authors declare that the research was conducted in the absence of any commercial or financial relationships that could be construed as a potential conflict of interest.
All claims expressed in this article are solely those of the authors and do not necessarily represent those of their affiliated organizations, or those of the publisher, the editors and the reviewers. Any product that may be evaluated in this article, or claim that may be made by its manufacturer, is not guaranteed or endorsed by the publisher.
The Supplementary Material for this article can be found online at: https://www.frontiersin.org/articles/10.3389/fcosc.2025.1487428/full#supplementary-material
Backhurst M. K., Cole R. G. (2000). Biological impacts of boating at Kawau Island, north-eastern New Zealand. J. Environ. Manage. 60, 239–251. doi: 10.1006/jema.2000.0382
Broad A., Rees M. J., Davis A. R. (2020). Anchor and chain scour as disturbance agents in benthic environments: trends in the literature and charting a course to more sustainable boating and shipping. Mar. Poll. Bull. 161, 111683. doi: 10.1016/j.marpolbul.2020.111683
Broad A., Rees M., Knott N., Swadling D., Hammond M., Ingleton T., et al. (2023). Anchor scour from shipping and the defaunation of rocky reefs: A quantitative assessment. Sci Total Environ. 863, 160717.
Burnham K. P., Anderson D. R. (2004). Multimodel inference: understanding AIC and BIC in model selection. Soc Method Res. 33, 261–304. doi: 10.1177/0049124104268644
Byers J. E., Grabowski J. H. (2014). “Soft-sediment Communities, Chapter 10,” in Marine Community Ecology and Conservation. Eds. Bertness M. D., Bruno J. F., Silliman B. R., Stachowicz J. J. (Sinauer Associates, Massachusetts USA), 227–249.
Coleman N., Gason A. S., Poore G. C. (1997). High species richness in the shallow marine waters of south-east Australia. Mar. Ecol. Prog. Ser. 154, 17–26. doi: 10.3354/meps154017
Collie J. S., Escanero G. A., Valentine P. C. (1997). Effects of bottom fishing on the benthic megafauna of Georges Bank. Mar. Ecol. Prog. Ser. 155, 159–172. doi: 10.3354/meps155159
Connell J. H. (1978). Diversity in tropical rain forests and coral reefs: high diversity of trees and corals is maintained only in a nonequilibrium state. Science 199, 1302–1310. doi: 10.1126/science.199.4335.1302
Cook P. L., Bock P. E., Gordon D. P. (2018). “Chapter 3: Class Gymnolaemata, Order Cheilostomata,” in Australian Bryozoa Volume 2: Taxonomy of Australian Families. Eds. Cook P. L., Bock P. E., Gordon D. P., Weaver H. J. (Melbourne, Australia: CSIRO Publishing), 61–280.
Davis G. E. (1977). Anchor damage to a coral reef on the coast of Florida. Biol. Conserv. 11, 29–34. doi: 10.1016/0006-3207(77)90024-6
Davis A. R., Broad A. (2016). Environment: Curb anchor scour for green shipping. Nature 533, 36. doi: 10.1038/533036a
Davis A. R., Broad A., Gullett W., Reveley J., Steele C., Schofield C. (2016). Anchors away? The impacts of anchor scour by ocean-going vessels and potential response options. Mar. Policy 73, 1–7. doi: 10.1016/j.marpol.2016.07.021
Davis A. R., Broad A., Small M., Oxenford H. A., Morris B., Ingleton T. C. (2022). Mapping of benthic ecosystems: key to improving the management and sustainability of anchoring practices for ocean-going vessels. Continental Shelf Res. 247, 104834. doi: 10.1016/j.csr.2022.104834
Demers M.-C. A., Davis A. R., Knott N. A. (2013). A comparison of the impact of ‘seagrass-friendly’ boat mooring systems on Posidonia australis. Mar. Env. Res. 83, 54–62. doi: 10.1016/j.marenvres.2012.10.010
Deter J., Lozupone X., Inacio A., Boissery P., Holon F. (2017). Boat anchoring pressure on coastal seabed: quantification and bias estimation using AIS data. Mar. pollut. Bull. 123, 175–181. doi: 10.1016/j.marpolbul.2017.08.065
DOT (2024). Department of Transport, UK. Shipping Fleet Statistics: 2023. Available online at: https://www.gov.uk/government/statistics/shipping-fleet-statistics-2023/shipping-fleet-statistics-2023 (Accessed Aug. 2024).
Fisher R., Wilson S. K., Sin T. M., Lee A. C., Langlois T. J. (2018). A simple function for full-subsets multiple regression in ecology with R. Ecol. Evol. 8, 6104–6113. doi: 10.1002/ece3.2018.8.issue-12
Flynn R. L., Forrester G. E. (2019). Boat anchoring contributes substantially to coral reef degradation in the British Virgin Islands. PeerJ 7, e7010. doi: 10.7717/peerj.7010
Frid C. L. J., Clark R. A., Hall J. A. (1999). Long-term changes in the benthos on a heavily fished ground off the NE coast of England. Mar. Ecol. Prog. Ser. 188, 13–20. doi: 10.3354/meps188013
Hall S. J. (1994). Physical disturbance and marine benthic communities: life in unconsolidated sediments. Oceanography Mar. Biology: an Annu. review. 32, 179–239.
Heaver T. D. (2021). Reducing anchorage in ports: changing technologies, opportunities and challenges. Front. Future Transportation 2. doi: 10.3389/ffutr.2021.709762
Herbert R. J., Crowe T. P., Bray S., Sheader M. (2009). Disturbance of intertidal soft sediment assemblages caused by swinging boat moorings. Hydrobiologia 625, 105–116. doi: 10.1007/s10750-008-9700-x
Huxham M., Roberts I., Bremner J. (2000). A field test of the intermediate disturbance hypothesis in the soft-bottom intertidal. Int. Rev. Hydrobiology: A J. Covering all Aspects Limnology Mar. Biol. 85, 379–394.
International Chamber of Shipping (2008). Shipping and the Environment: A Code of Practice, 4th ed. (London: International Chamber of Shipping). doi: 10.1002/1522-2632(200008)85:4<379::AID-IROH379>3.0.CO;2-X
Jumars P. A., Dorgan K. M., Lindsay S. M. (2015). Diet of worms emended: an update of polychaete feeding guilds, appendix A family-by-family updates. Annu. Rev. Mar. Sci 7, 497–520. doi: 10.1146/annurev-marine-010814-020007
Keable S. J. (1995). Structure of the marine invertebrate scavenging guild of a tropical reef ecosystem: field studies at Lizard Island, Queensland, Australia. J. Natural History 29, 27–45. doi: 10.1080/00222939500770021
Kiggins R. S., Knott N. K., Davis A. R. (2018). Miniature Baited Remote Underwater Video (mini-BRUV) reveals the response of cryptic fishes to seagrass cover. Env. Biol. Fishes 101, 1717–1722. doi: 10.1007/s10641-018-0823-2
Lanham B. S., Vergés A., Hedge L. H., Johnston E. L., Poore A. G. (2018). Altered fish community and feeding behaviour in close proximity to boat moorings in an urban estuary. Mar. Poll. Bull. 129, 43–51. doi: 10.1016/j.marpolbul.2018.02.010
Lee W. J. (2010). Intensive use of an intertidal mudflat by foraging adult American horseshoe crabs Limulus polyphemus in the Great Bay estuary, New Hampshire. Curr. Zoology 56, pp.611–pp.617. doi: 10.1093/czoolo/56.5.611
Lenihan H. S., Micheli F. (2001). “Soft-sediment communities,” in Marine community ecology. Eds. Bertness M. D., Gaines S., Hay M. E. (Sinauer Associates, Sunderland MA), 253–287.
Lowry J. K., Smith S. D. A. (2003). “Invertebrate Scavenging Guilds along the Continental Shelf and Slope of Eastern Australia—General Description,” in Final report to the Fisheries Research Development Corporation, Project 96/280, 59 (Australian Museum, Sydney).
Macolino A. C., Dafforn K. A., Hedge L. H., Poore A. G. B., Johnston E. L. (2019). Fine-scale effects of boat moorings on soft sediment communities masked in large-scale comparisons. Front. Mar. Sci. 6. doi: 10.3389/fmars.2019.00101
Magris R. A., Ban N. C. (2019). A meta-analysis reveals global patterns of sediment effects on marine biodiversity. Global Ecol. Biogeogr. 28, 1879–1898. doi: 10.1111/geb.12990
McArthur M. A., Brooke B. P., Przeslawski R., Ryan D. A., Lucieer V. L., Nichol S., et al. (2010). On the use of abiotic surrogates to describe marine benthic biodiversity. Estuarine Coast. Shelf Sci. 88, 21–32. doi: 10.1016/j.ecss.2010.03.003
Meadows P. S., Meadows A. (1991). Environmental impact of burrowing animals and animal burrows (Oxford U.K: Clarendon Press).
Oksanen J., Simpson G., Blanchet F., Kindt R., Legendre P., Minchin P., et al. (2025). vegan: Community Ecology Package (R package version 2.7-0). Available at: https://github.com/vegandevs/veganhttps://vegandevs.github.io/vegan/.
Oliver J. S., Slattery P. N., Hulberg L. W., Nybakken J. W. (1980). Relationships between wave disturbance and zonation of benthic invertebrate communities along a subtidal high-energy beach in Monterey Bay, California. Fishery Bull. 78, 437–454.
Oliver J. S., Slattery P. N., Silberstein M. A., O’Connor E. F. (1984). Gray whale feeding on dense ampeliscid amphipod communities near Bamfield, British Columbia. Can. J. Zoology 62, 41–49. doi: 10.1139/z84-009
Probert P. K. (1984). Disturbance, sediment stability, and trophic structure of soft-bottom communities. J. Mar. Res. 42, 893–921. doi: 10.1357/002224084788520837
Przeslawski R., Berents P., Clark M., Dittmann S., Edgar G., Frid C., et al. (2024).Marine sampling field manual for grabs and box corers. In: Field Manuals for Marine Sampling to Monitor Australian Waters, Version 3 (National Environmental Science Programme (NESP). Available online at: https://grabs-and-boxcorers-field-manual.github.io/ (Accessed 16 Aug 2024).
R Core Development Team (2021). R: A language and environment for statistical computing (Vienna: R Foundation for Statistical Computing). Available at: https://www.R-project.org/.
Reise K. (2002). Sediment mediated species interactions in coastal waters. J. Sea Res. 48, pp.127–pp.141. doi: 10.1016/S1385-1101(02)00150-8
Sciberras M., Hiddink J. G., Jennings S., Szostek C. L., Hughes K. M., Kneafsey B., et al. (2018). Response of benthic fauna to experimental bottom fishing: A global meta-analysis. Fish Fisheries 19, 698–715. doi: 10.1111/faf.2018.19.issue-4
Smith S. H. (1988). Cruise ships: a serious threat to coral reefs and associated organisms. Ocean Shoreline Manage. 11, 231–248. doi: 10.1016/0951-8312(88)90021-5
Thrush S. F., Pridmore R. D., Hewitt J. E., Cummings V. J. (1991). Impact of ray feeding disturbances on sandflat macrobenthos: Do communities dominated by polychaetes or shellfish respond differently? Mar. Ecol. Prog. series. Oldendorf 69, 245–252. doi: 10.3354/meps069245
UNCTAD (2023). Review of Maritime Transport (Geneva: United Nations Conference on Trade and Development). Available online at: https://unctad.org/publication/review-maritime-transport-2023 (Accessed Aug., 2024).
UNDP (2019). United Nations Development Programme, sustainable development goals. Goal 14, life below water. Available online at: www.undp.org/content/undp/en/home/sustainabledevelopment-goals/goal-14-life-below-water.html (Accessed 10 Jun 2021).
Vázquez-Luis M., Borg J. A., Morell C., Banach-Esteve G., Deudero S. (2015). Influence of boat anchoring on Pinna nobilis: a field experiment using mimic units. Mar. Freshw. Res. 66, 786–794. doi: 10.1071/MF14285
Watling L., Norse E. A. (1998). Disturbance of the seabed by mobile fishing gear: a comparison to forest clearcutting. Conserv. Biol. 12, 1180–1197. doi: 10.1046/j.1523-1739.1998.0120061180.x
Watson S. J., Neil H., Ribó M., Lamarche G., Strachan L. J., MacKay K., Wilcox S., et al (2020). What we do in the shallows: Natural and anthropogenic seafloor geomorphologies in a drowned river valley, New Zealand. Front Mar Sci. 7, 579626.
Watson S. J., Ribó M., Seabrook S., Strachan L. J., Hale R., Lamarche G. (2022). The footprint of ship anchoring on the seafloor. Sci. Rep. 12, 7500. doi: 10.1038/s41598-022-11627-5
Keywords: anchoring, infauna, marine invertebrates, human impacts, disturbance, AIS, SDG14, sediment grain size
Citation: Davis AR, Broad A, Steele C, Woods C, Przeslawki R, Nicholas WA(T), Maher W, Krikowa F, Morris B, Ingleton TC, O’Hea Miller S and Rees MJ (2025) Dragging the chain: anchor scour impacts from high-tonnage commercial vessels on a soft bottom macrobenthic assemblage. Front. Conserv. Sci. 6:1487428. doi: 10.3389/fcosc.2025.1487428
Received: 28 August 2024; Accepted: 05 March 2025;
Published: 26 March 2025.
Edited by:
Susana França, Center for Marine and Environmental Sciences (MARE), PortugalReviewed by:
Marta Ribó, Auckland University of Technology, New ZealandCopyright © 2025 Davis, Broad, Steele, Woods, Przeslawki, Nicholas, Maher, Krikowa, Morris, Ingleton, O’Hea Miller and Rees. This is an open-access article distributed under the terms of the Creative Commons Attribution License (CC BY). The use, distribution or reproduction in other forums is permitted, provided the original author(s) and the copyright owner(s) are credited and that the original publication in this journal is cited, in accordance with accepted academic practice. No use, distribution or reproduction is permitted which does not comply with these terms.
*Correspondence: Andrew R. Davis, YWRhdmlzQHVvdy5lZHUuYXU=
Disclaimer: All claims expressed in this article are solely those of the authors and do not necessarily represent those of their affiliated organizations, or those of the publisher, the editors and the reviewers. Any product that may be evaluated in this article or claim that may be made by its manufacturer is not guaranteed or endorsed by the publisher.
Research integrity at Frontiers
Learn more about the work of our research integrity team to safeguard the quality of each article we publish.