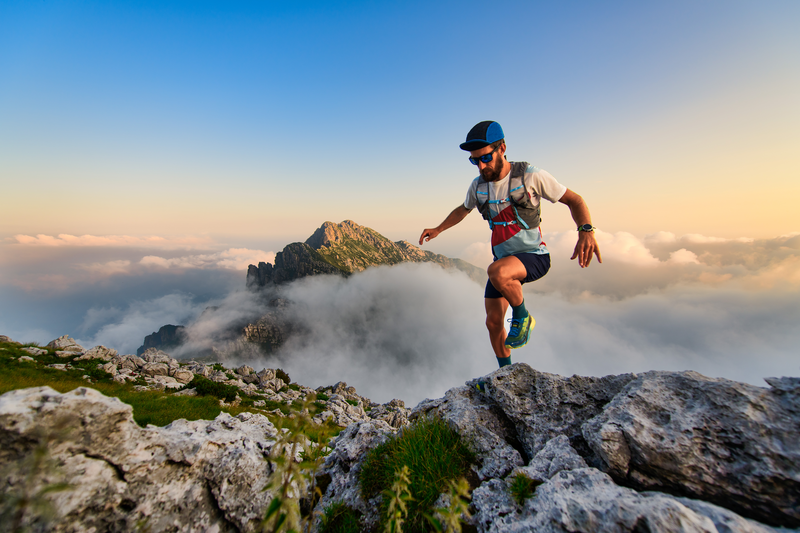
94% of researchers rate our articles as excellent or good
Learn more about the work of our research integrity team to safeguard the quality of each article we publish.
Find out more
ORIGINAL RESEARCH article
Front. Conserv. Sci. , 10 December 2024
Sec. Animal Conservation
Volume 5 - 2024 | https://doi.org/10.3389/fcosc.2024.1503408
This article is part of the Research Topic Long-Term Research on Avian Conservation Ecology in the Age of Global Change and Citizen Science View all 8 articles
Introduction: Urbanization, characterized by the rapid expansion of human settlements and development, greatly impacts biodiversity, especially where developments and human settlements are not guided by proper environmental consideration.
Methods: We used data collected through citizen science projects under the African Bird Atlas Project, based on standardized protocols to gather bird occurrence data. Species’ ecological traits, related to foraging behavior, habitat speciality, and body mass, were analyzed to assess functional richness and functional diversity (FD) represented by Rao’s quadratic entropy. Geospatial data, including the Global Human Settlement Layer and Human Modification Index (HMI), along with the probability of urban expansion up to 2030, were integrated to examine the impact of urbanization using Bayesian models.
Results: Our findings reveal a marked decline in taxonomic richness, diversity, and functional richness associated with increasing urbanization and human modification, with a similar pattern observed along the gradient from mostly uninhabited areas to cities. However, FD increased with urbanization from uninhabited areas to cities. The relationship between FD and HMI was nonlinear, showing an initial negative trend that became positive as HMI increased. This suggests a transition in bird communities, where generalist species thrive in urban environments, potentially replacing specialized species and leading to functional redundancy.
Discussion: Despite the complex relationships observed, urbanization has a predominantly negative impact on the richness and diversity of Afrotropical bird communities. The decline in avian diversity and functional richness has important implications for ecosystem functions and services, crucial for biodiversity and human well-being. Our research provides valuable insights into the ecological impacts of urban expansion and emphasizes the importance of preserving natural habitats amidst growing urban landscapes.
Graphical Abstract. Summary of taxonomic and functional richness and diversity trends along the urbanization gradient. Taxonomic Richness, Functional Richness, and Taxonomic Diversity decrease with increasing urbanization, as indicated by the downward red arrows (highest in uninhabited and rural areas). Functional Diversity, as measured by Rao's Quadratic Entropy, increases in more urbanized environments, as shown by the upward blue arrow (highest in cities).
Urbanization, characterized by the rapid expansion of human settlements and infrastructure, has become a global phenomenon, transforming natural environments with significant implications for biodiversity (Grimm et al., 2008; Mckinney, 2008). By 2010, over 50% of the world’s population resided in urban areas, and projections indicate that by 2050, this figure will rise to 70% (UN, 2012). For Africa, the projected tripling of urban land cover by 2030, is particularly concerning as it will lead to substantial habitat loss across the continent (Seto et al., 2012). This ongoing urban development will result in increased predator presence (Baker et al., 2008; Fischer et al., 2012), elevated noise levels (Proppe et al., 2013), and forest fragmentation (Zipperer et al., 2012), with substantial influence on community composition (Marzluff, 2001), and ecosystem functioning (e.g. Blair, 1996; Ferenc et al., 2014; Lim and Sodhi, 2004; Marzluff, 2001; Pautasso et al., 2011).
The composition of bird communities in urban areas is a function of species tolerance and adaptive capacity. Species sensitive to habitat disturbances are classified as “urban avoiders” (McKinney, 2002) or “urban-sensitive” (Garden et al., 2007), while those thriving in urban environments are called “urban exploiters” (McKinney, 2002) or “synanthropes” (Marzluff et al., 2001; Cresswell et al., 2020). Both categories are impacted differently by urbanization (DeGraaf and Wentworth, 1986; Isaksson, 2018), with specialized species (because of their life-history and ecological traits) being impacted the most (Evans et al., 2011; Sol et al., 2014). The negative effects of urbanization on bird communities have been extensively documented globally and in Africa (see Chamberlain et al., 2017; Oliviera et al., 2017; Ibáñez-Álamo et al., 2017; Lee et al., 2021; Sol et al., 2020). Considering the growing human population and the subsequent increased urbanization globally (United Nations Department of Economic and Social Affairs, 2019), understanding species’ responses to anthropogenic pressures are paramount for guiding conservation measures (Newbold et al., 2018).
Because birds are well known, and are good indicators of environmental health (Fraixedas et al., 2020), they are invaluable when studying the impacts of urbanization on habitat structure and composition (Chace and Walsh, 2006; MacGregor-Fors et al., 2009; Reis et al., 2012; Bregman et al., 2014; Marzluff, 2016). Numerous studies have documented varying trends in how urbanization affects bird abundance and diversity (Palacio et al., 2018; Carvajal-Castro et al., 2019; Korányi et al., 2021). While some studies report minimal influence of urbanization on bird diversity (Korányi et al., 2021), others link increased urbanization to reduced diversity (Sol et al., 2017, 2020). Conversely, a few studies report increased bird diversity with rising urbanization (Batáry et al., 2018; Filloy et al., 2019). Beyond bird abundance and diversity, it is essential to consider birds for the ecological roles they play in seed dispersal, pollination, pest control, nutrient cycling, and scavenging (Lundberg and Moberg, 2003; Pigot et al., 2016; S̜ekercioğlu et al., 2016; Sekercioglu, 2006). These roles not only sustain ecosystems but also ensure human wellbeing (Cardinale et al., 2012); for example, loss of scavengers, has been shown to lead to an increase in diseases (García-Jiménez et al., 2021, 2022).
The functional traits that support bird’s roles in environments have been extensively documented in recent studies (Pigot et al., 2020; Tobias and Pigot, 2019), allowing for detailed characterization of bird communities with unmatched precision. To better understand impacts of urbanization on vital ecosystem functions and services (Sol et al., 2020), an effective method is to examine functional diversity. Functional diversity encompasses the identity, variety, and relative abundance of phenotypic traits in organisms that affect key ecosystem processes (Díaz et al., 2007; Petchey and Gaston, 2006; Tilman, 2001). Studies are particularly needed in the tropical regions including Africa, where rapid population growth has led to accelerated urbanization (Seto et al., 2011, 2012). However, only a few studies have investigated how urbanization affects bird ecological roles in Africa (Chamberlain et al., 2017; Lee et al., 2021; Njoroge et al., 2014; van Rensburg et al., 2009; Awoyemi et al., 2024). Awoyemi et al. (2024) examined the impact of urbanization on biodiversity metrics within cities in a single country, utilizing a paired sampling approach to compare urban and non-urban areas. To gain a more detailed insights, future studies could explore the effects of urbanization on bird community and ecosystem functions across a finer urbanization gradient and at a broader scale.
Here, we investigate the impacts of urbanization and human modification on taxonomic and functional metrics both in Nigeria and Kenya. Nigeria and Kenya average population densities are estimated at 226 humans/km2 and 97 humans/km2, respectively (United Nations, 2019). Both countries fall within the Afrotropical region with rich but declining biodiversity (Cazzolla Gatti et al., 2015). Nigeria is situated on the East Atlantic Flyway, while Kenya sits on the East Asia-East Africa Flyway, making both countries crucial for the conservation of migratory birds. Yet, the impact of urbanization on biodiversity in these landscapes is relatively understudied (Ibáñez-Álamo et al., 2017; Magle et al., 2012), mainly due to limited local capacity and funds (Awoyemi and Ibáñez-Álamo, 2023).
Building on Oliviera et al. (2017), Newbold et al. (2015), and Sol et al. (2017), we compare bird diversity across four levels of urbanization (settlement types): cities, suburban areas (moderately urbanized environments), dispersed rural areas (slightly human-modified), and mostly uninhabited areas (MUAs) in Kenya and Nigeria. We hypothesize that urbanization negatively impacts taxonomic and functional richness and diversity of African bird communities. Specifically, as urbanization intensifies, we expect to observe a decline in both the number of species (taxonomic richness) and the unique ecological function of the species (functional richness). Additionally, we anticipate that urban expansion will reduce the overall diversity of bird communities, leading to more homogenized assemblages with fewer distinct species and functional roles. We also anticipate that uninhabited and rural areas will have higher taxonomic and functional richness and diversity than suburban areas and cities, reflecting the negative impact of urbanization on bird communities.
We used data collected through the African Bird Atlas Project (ABAP), specifically originating from the country-level citizen science projects: the Nigerian Bird Atlas Project (hereafter, NiBAP) established in 2015, and the Kenya Bird Map (hereafter, KBM) founded in 2013. NiBAP and KBM rely on citizens to collect and submit bird data through data collection cards, each representing a full observation record for a specific time and location (pentad), also known as checklists. These projects adhere to protocols established by the Southern African Bird Atlas Project (hereafter, SABAP; Underhill, 2016), ensuring standardized data collection and reporting procedures. Citizens participating in these projects survey and record birds within a 5-minute x 5-minute grid (approximately 9 km x 9 km square) referred to as a pentad. Survey lists of birds are reported as either full protocol cards or ad hoc cards. Full protocol cards are records from a minimum of two hours of focused survey over a maximum five days within each pentad, covering different vegetation types. Where citizen scientist spends less than 2 hours including reports of incidental bird observation, those submission are considered ad hoc cards (Tende et al., 2024). Both full protocol and ad hoc cards are essential for reporting species distribution, and provide presence data on species in a pentad (Figure 1).
Figure 1. Coverage maps of Nigeria (A) and Kenya (B), indicating the number of checklists submitted with Group 4+ checklists used for this analysis, shown in darker shades.
Full protocol card submission allows for calculation of species reporting rate as an index of abundance. The index tell how frequently a species is recorded in all full protocol cards submitted within a pentad, and ranges from 0 (never recorded) to 1 (recorded in all full protocol cards) (see Lee et al., 2017, 2021; Figure 1).
We extracted raw bird distribution records from NiBAP (932 species in 228,019 records) and KBM (1373 species in 266,708 records). To ensure data integrity, we used only vetted species lists available at Birdmap Africa - Nigeria and Birdmap Africa - Kenya, filtering the records to include only species present in these lists. After cleaning the data, we retained 914 unique species with 227,420 records for Nigeria and 1,069 species with 265,276 records for Kenya. We also filtered coverage grids in both countries to include only those pentads where at least four full protocol cards had been submitted over the years across the study period, following the methodology of previous studies (Lee et al., 2017). This step ensured that we focused on reliable data subsets. Finally, we integrated the functional traits dataset (see Supplementary Table S1) with the reporting rates dataset to enrich the ecological context.
To describe the functional traits of our bird species, we compiled published data describing the foraging behavior, habitat specialization and masses for all the bird species. Each species was categorized based on 13 functional traits primarily related to diet, body mass, and habitat specialization (Appendix I). Dietary classifications followed the framework provided in (Child et al., 2009), which associates dietary categories with ecological roles. We did this because the functional role of a species in an ecosystem may be more directly inferred by how the species uses resources (Petchey and Gaston, 2006; Oliviera et al., 2017). For instance, scavengers contribute to carcass and waste disposal and help in disease control, while carnivores help manage rodent populations. Species that primarily rely on fruits (frugivores) aid in seed dispersal, whereas insectivores (insect eating birds) help control invertebrates (Whelan et al., 2015; Chan et al., 2016; Lee et al., 2021). Additional categories include piscivores, ecological engineers (such as woodpeckers and barbets), habitat specialists (including biome-restricted species and primary forest specialists), and species foraging in aquatic environments. Each classification was binary and non-exclusive, allowing species to belong to multiple dietary classes, particularly omnivores. A comprehensive list of species and their assigned functional traits is available in the Supplementary Data Sheet S1. The mass values were primarily sourced from “The Handbook of the Birds of the World”. We supplemented any missing mass data by referencing online resources such as the Global Biodiversity Information Facility (GBIF-www.gbif.org) or national museum ringing records. Functional richness is vital because it is a measure of the functional traits present in a community, thus presenting only presence/absence of species functional traits. However, previous studies have revealed abundance-based metrics to be more influential on ecosystem functions (Petchey and Gaston, 2006; Newbold et al., 2012). Rao’s quadratic entropy presents a perfect opportunity to bridge this bias. It accounts for species richness, while also capturing trait identity, abundance and variety (Ricotta et al., 2016).
We extracted the Global Human Settlement Layer (GHSL (Melchiorri, 2022), available for download from the Copernicus Emergency Management Service (https://human-settlement.emergency.copernicus.eu/download.php?ds=smod). This dataset identifies settlement typologies using two-digit codes: City (30), Dense Town (23), Semi-Dense Town (22), Suburban (21), Village (13), Dispersed Rural (12), Mostly Uninhabited Area (11), and Water Grid (10). These typologies are represented at a 1 km resolution.
To determine the dominant settlement type within each pentad (9 km x 9 km, encompassing 81 GHSL cells), we used the ‘geobgu::raster_extract’ function in R. By applying the base R modal function, we identified the most prevalent settlement type, resulting in five classes: City, Suburban, Dispersed Rural Area, and Mostly Uninhabited Area. Additionally, we obtained the Global Human Modification of Terrestrial Systems dataset, referred to as the Human Modification Index (HMI), from the Socioeconomic Data and Applications Center (SEDAC). This dataset quantifies human impact on terrestrial lands through 13 stressors, with values ranging from 0 (minimal impact) to 1 (intense modification) (Kennedy et al., 2019, 2020).
We also obtained a dataset offering a 2.5 arc-minute resolution probability of urban expansion from the year 2000 to 2030. This dataset utilizes a Monte Carlo model to forecast the likelihood of urbanization for non-urban grid cells by 2030, providing valuable insights into global urban land cover changes. Unlike the categorical GHSL layer, the HMI and urbanization layers provide numerical values, enabling a fine-scale gradient analysis. We resampled/upscaled these layers to a 5-minute × 5-minute resolution (approximately one-twelfth degree) to maintain spatial congruity with our avian data and ensure consistent analysis across all datasets.
The Directed Acyclic Graph (DAG) (Figure 2) illustrates the intricate causal relationships between human modification, human settlement, urban expansion, and their effects on avian taxonomic and functional diversity. The bidirectional connections indicate that urbanization and settlement patterns mutually influence each other. Additionally, the probability of urban expansion (P(Urban Expansion)) reflects the likelihood of further encroachment driven by existing modifications and settlements, which may adversely affect bird taxonomic and functional richness due to habitat fragmentation and resource reduction. Overall, this DAG underscores the feedback loops between human activities and ecological outcomes.
Figure 2. Directed Acyclic Graph (DAG) illustrating the interplay of human modification, settlements, and probability of urban expansion on taxonomic/functional richness and diversity.
All our analyses were conducted using R version 4.2.3 (R Core Team, 2023). We calculated standard measures of functional diversity, including functional richness (FRic) and Rao’s Q, using the ‘fundiversity’ package (Grenié and Gruson, 2023). Reporting rate in pentads was used as a proxy for abundance measures (Underhill, 2016). Functional diversity, represented by Rao’s Q, describes the variability in ecological functions across species within a community, while functional richness (FRic) reflects the range of ecological traits, unweighted by abundance (see Table 1 for further metrics definitions). Additionally, we calculated taxonomic richness as the count of distinct species in each pentad and used the Shannon-Weiner diversity index to measure taxonomic diversity, utilizing the ‘vegan’ package (Oksanen et al., 2018). For each metric of taxonomic and functional richness and diversity, we employed Bayesian hierarchical (mixed) models fitted in STAN (Carpenter et al., 2017) using the ‘brms’ package (Bürkner, 2021) to compare across settlement types. These models included random effects at the pentad level to account for spatial variability. The ‘modelr’ and ‘tidybayes’ packages were used to create a grid of predictor values, add posterior predictive draws, and compute and visualize contrasts between settlement levels using ‘ggplot2’. We then conducted pairwise contrast analyses of the predicted posterior distributions across the settlement types (City, Semi-Urban, Rural Area, and Uninhabited Area). We further investigated the relationship between each metric of taxonomic and functional richness and diversity and human modification of the environment, as well as the potential impact of urban expansion on avian communities. The sampling process involved four chains with 2000 iterations each, with the first 1000 iterations used for warm-up. Convergence and model fit were assessed through posterior predictive checks, effective sample size, and Rhat values close to 1.
A total of 914 species across 89 bird families were recorded in Nigeria, while 1069 species across 94 families were recorded in Kenya. In both countries, the majority of species (>90%) are classified as Least Concern, with 27 Threatened species recorded in Nigeria (3 Critically Endangered, 11 Endangered, and 13 Vulnerable) and 44 in Kenya (5 Critically Endangered, 20 Endangered, and 19 Vulnerable) according to the IUCN Red List (BirdLife International, 2024). Kenya supports 225 habitat specialists, compared to 179 in Nigeria. Additionally, both countries host urban-adaptive species, with 40 in Nigeria and 50 in Kenya. A detailed list of species is provided in Supplementary Data Sheet S2.
Taxonomic richness (Figure 3A) and functional richness (Figure 3B) decreased across the gradient from rural to suburban to city areas, indicating fewer species and traits in more urbanized environments. However, taxonomic diversity (Figure 3C) was highest in rural areas, followed by suburban, uninhabited, and city areas. Conversely, functional diversity (Figure 3D) was highest in city areas and lowest in uninhabited areas, with intermediate functional diversity values in rural and suburban areas.
Figure 3. Conditional effects plot of the differences in taxonomic richness (A), functional richness (B), taxonomic diversity (C), and functional diversity (D) across different levels of urbanization. Each plot shows the 95% credible intervals for the respective metric.
Our analysis revealed strong evidence that taxonomic richness decreased with increasing urbanization such that rural areas had higher taxonomic richness (i.e., a greater variety of species) than cities (median difference ± SD: Rural – City: 14.885 ± 15.520 [95% CI: -2.493, 57.996]; Posterior Probability (PP) = 0.951, Evidence Ratio (ER01) = 19.202, Table 2A). Additionally, both suburban areas and uninhabited areas had higher richness than cities (Suburban – City: 13.659 ± 14.702 [95% CI: -4.347, 53.868]; PP = 0.926, ER01 = 12.605, and Uninhabited – City: 9.233 ± 10.593 [95% CI: -4.262, 37.579], PP = 0.910, ER01 = 10.173, respectively, Table 2A). However, we did not find substantial differences between Suburban – Rural, Uninhabited – Rural, and Uninhabited – Suburban contrasts (Table 2A), as their distributions, including the 95% credible intervals, closely intersected the zero-effect line (Figure 4A).
Table 2. Results of contrast analyses on taxonomic and functional richness and diversity between different settlements, including the median, standard deviation (SD), and 95% credible intervals (CI) of contrasts from posterior estimates.
Figure 4. Predicted posterior contrasts in taxonomic and functional richness and diversity. (A) Taxonomic richness, (B) Functional richness, (C) Taxonomic diversity, and (D) Functional diversity. Each dot represents the median parameter estimate, and the thick horizontal bars represent the 95% credible intervals. Distributions intersecting zero indicate uncertainty or negligible differences, while shifts to the right of zero (dotted line) suggest higher values for the parameter on the left-hand side, and shifts to the left indicate lower values for the parameter on the right.
Generally, uninhabited, rural, and suburban areas showed higher functional richness than cities. The difference was most discernible between Uninhabited and City areas (median difference ± SD: 17.819 ± 31.512 [95% CI: -7.582, 118.312], PP = 0.924, ER01 = 10.158, Table 2A); all pairs except ‘Uninhabited – City’ have their 95% credible interval intersecting the zero mark (Figure 4B).
On diversity, our results indicated that rural, uninhabited, and suburban areas clearly had higher taxonomic diversity (highlighted in light blue in Figure 4C) compared to cities (Rural – City: 0.325 ± 0.066 [95% CI: 0.198, 0.459]; Suburban – City: 0.269 ± 0.068 [95% CI: 0.132, 0.397]; Uninhabited – City: 0.245 ± 0.052 [95% CI: 0.140, 0.345]; PP = 1, ER01 = Inf; Table 2C). We found no substantial differences between Uninhabited – Rural, Uninhabited–Suburban and Suburban - Rural, as the evidence was weak (PP< 0.85, ER< 5, Table 2C), with the distribution intersecting with zero effect mark. Conversely, there was strong evidence for greater functional diversity in cities compared to rural, suburban, and uninhabited areas (Rural – City: -0.277 ± 0.067 [95% CI: -0.417, -0.147]; Suburban – City: -0.282 ± 0.067 [95% CI: -0.421, -0.156]; Uninhabited – City: -0.337 ± 0.055 [95% CI: -0.448, -0.233]; PP =1, ER01 = Inf; Table 2D) as highlighted in light blue in Figure 4D. Comparisons between Suburban – Rural, Uninhabited – Rural, and Uninhabited – Suburban displayed negligible differences, with distributions closely intersecting the zero-effect line, indicating limited evidence for variation in functional diversity outside cities.
The following section presents the patterns in the relationship; a more detailed model summary can be found in the Supplementary Tables S1 and S2. Generally, the models demonstrated a good fit, as indicated by Rhat values close to 1, High Effective Sample Size (ESS), and proper mixing of the Markov chains.
Figure 5 illustrates the relationships between HMI and taxonomic and functional richness and diversity. Overall we found a predominantly negative relationship in taxonomic richness and diversity and functional richness across different levels of human modification. Areas with higher HMI tended to support fewer species and a reduced variety of functions that species perform (Figures 5A–C). However, the relationship between functional diversity and the HMI was complex and nonlinear (Figure 5D). Initially, functional diversity decreased, but reversed to increasing trend at higher levels of HMI. Despite this variability, the overall findings suggest that human activities exerted varying effects on functional diversity.
Figure 5. Effect of human modification on taxonomic and functional richness and diversity. (A) Taxonomic richness, (B) Functional richness, (C) Taxonomic diversity, and (D) Functional diversity. Each plot shows the 95% credible intervals for the respective metric.
Finally, we investigated the impact of urban expansion on the taxonomic and functional richness and diversity (detailed model estimates are provided in the Supplementary Table S2). Taxonomic richness (Figure 6A), functional richness (Figure 6B), and taxonomic diversity (Figure 6C) as a function of urban expansion showed similar patterns: an initial decline, followed by a slight rise as the probability of urban expansion increases, with a pronounced decrease observed at higher probabilities (around 75% and above, Figures 6A–C) up to 100% probability of urban expansion. In contrast, there was a slight and steady increase in functional diversity with increasing urban expansion (Figure 6D), indicating that some functional traits may persist or even become more prevalent in urbanized environments. Overall, our findings indicate that urbanization has a complex but predominantly negative impact on the richness and diversity of African bird communities.
Figure 6. Effect of urban expansion on taxonomic and functional richness and diversity. (A) Taxonomic richness, (B) Functional richness, (C) Taxonomic diversity, and (D) Functional diversity. Each plot shows the 95% credible intervals for the respective metric.
Urbanization, as a form of global change, has been shown to significantly alter the physical environment (Kaye et al., 2006), creating new environments and opportunities for species. Consequently, urbanization often leads to variations in bird community metrics across different gradients (Sol et al., 2020; Petersen et al., 2022). Our results indicate that taxonomic richness and diversity were notably higher in Mostly Uninhabited Areas (MUAs) compared to cities. MUAs, characterized by more natural or “pristine” vegetation, offer a variety of habitats conducive to diverse bird communities. The availability of suitable habitats is critical for species distribution and with urbanization natural or near-natural environments are transformed for housing, industrial, or commercial purposes, leading to habitat loss for bird communities (Donnelly and Marzluff, 2006; Sandström et al., 2006; Croci et al., 2008). This change shape communities by filtering out some species while allowing others to replace them. In some cases, certain bird species may remain if fragments of near-natural habitats persist (Haire et al., 2000).
Over time, urbanization results in the replacement of specialist species by generalists (Oliviera et al., 2017; MacLean et al., 2018), as specialists are more susceptible to environmental changes. As the specialists are filtered out of the urban areas (Evans et al., 2011; Sol et al., 2014; Fischer et al., 2015), the unique functional traits they contribute to ecosystem functioning are lost, potentially leading to lower functional richness in the urban centers with crucial conservation implications (Fischer et al., 2015; Sol et al., 2014). This corroborates findings from other studies indicating that urbanization drives species decline (McKinney, 2006; Ibáñez-Álamo et al., 2017). On the other hand, functional diversity increased with the urbanization gradient, with cities exhibiting a higher functional diversity than MUAs. However, a continuous increase in HMI revealed a possible decline, indicating that while cities may initially support a diverse range of functional traits, excessive human modification eventually reduces this diversity after reaching a tipping point. This pattern suggests a transitional phase in bird communities, particularly in newer cities, where species are still adapting. Over time, as seen in established cities like Nairobi, only a few adaptable species may persist, leading to shifts in community composition, such as the disappearance of Hooded Vultures (Necrosyrtes monachus), which were once common in Kenya (Odino et al., 2014), and the proliferation of Marabou Storks (Leptoptilos crumeniferus). This “undulating” relationship might reflect the dynamic nature of species adaptation in response to urbanization. Additionally, while functional diversity is expected to decline at some point with urbanization (Fischer et al., 2015; Sol et al., 2020), our findings agree with Lee et al. (2021), who reported slightly higher functional diversity in urbanized areas as compared to non-urbanized areas. One explanation for this could be because functional diversity is an abundance-weighted metric that is easily influenced by the proliferation of generalist and disturbance tolerant species. Urbanization could also result in humans providing other sources of food through human modification of habitats. For example, the gardens, refuse dumps and ornamental plants could provide additional sources of food in urban areas (Pauw and Louw, 2012).
Our findings reveal variability in the relationship between the urbanization measures and bird community metrics, thus highlighting the importance of employing various metrics in evaluating the effects of urbanization on bird communities (Matuoka et al., 2020; Sol et al., 2020; Lee et al., 2021; Petersen et al., 2022; Suárez-Castro et al., 2022). These findings are critical for managing landscapes especially in the face of growing urbanization. HMI negatively influenced taxonomic diversity, taxonomic richness and functional richness but had an unstable relationship with functional diversity. Functional diversity initially declined with the human modification index, then followed by a subsequent increase in the functional diversity as the human modification increased. Our result suggests that though species richness declines, there may be no clear change in abundance along the urbanization gradient and in some cases certain species may inflate the total abundance of individuals (Chamberlain et al., 2017). One possible reason for this is that the total abundance may limit species richness such that where there are more individuals, the resources available may not be able to support more species, thus some species will have small populations, leading to their extirpation in such environments (Gaston, 2000). For this reason, species richness could be lower in cities (with higher human modification index), while functional diversity is either maintained (Lee et al., 2021; Callaghan et al., 2023) or higher as we have found. As more habitats are modified with increasing urbanization, there will be fewer habitats available to meet the niche requirement of bird communities (Grimm et al., 2008; Pautasso et al., 2011; Oliviera et al., 2017). For example, there is a decline in food availability along an urbanization gradient (Shochat et al., 2006), which thus limits the species found within the cities. However, cities may provide more feeding opportunities for raptors as well as reduced predation on them (Chace and Walsh, 2006). Thus, settlement-types with some natural habitats will provide more habitats to meet the natural requirements of more bird species, thus leading to an increase in taxonomic and functional richness.
Both the Human Modification Index (HMI) and the likelihood of urban expansion negatively influenced taxonomic diversity, taxonomic richness, and functional richness but positively influenced functional diversity. Our results suggest that although species richness declines, the overall abundance of individuals may not change clearly along the urbanization gradient. In some cases, certain species may disproportionately contribute to the total abundance (Chamberlain et al., 2017). One possible reason for this is that total abundance may limit species richness such that in environments with more individuals, available resources may not support additional species, leading to smaller populations and eventual extinction of some species (Gaston, 2000). Consequently, species richness could be lower in cities (with higher Human Modification Index), while functional diversity is either maintained (Lee et al., 2021; Callaghan et al., 2023) or higher, as we have found. As more habitats are modified with increasing urbanization, there will be fewer habitats available to meet the niche requirements of bird communities. For example, food availability declines along an urbanization gradient (Shochat et al., 2006), limiting the species found within cities.
Habitats are lost due to urbanization and specialists are replaced by generalists (De Coster et al., 2015), resulting in high species turnover rates. This could result in some niches not being utilized, thus providing opportunities for invasive species to explore (Jonason et al., 2017). Such opportunities could also lead to high populations of specific species, which could increase functional diversity in cities. For example, some studies have shown urbanized areas to have more individuals of fewer species (Chace and Walsh, 2006; Chamberlain et al., 2017). This is a probable reason why species richness declined while functional diversity increased with the urbanization gradient.
Furthermore, functional richness is determined by the presence or absence of specific traits in the niche space, while species-abundance-based diversity and differences among species influence functional diversity (RaoQ index) (Botta Dukát, 2005). This further highlights the effect that the number of individuals has on functional diversity. As a result, functional diversity may decrease if species richness increases. As species with unique traits are filtered out, it increases the average dissimilarity among species. On the other hand, both intermediary levels of urbanization and the MUAs had higher species richness due to the availability of suitable habitat, which allows the occurrence of both specialists and generalists. Additionally, if species occupying the same functional space replace each other, it does not influence functional trait diversity, thus masking species loss and changes in species composition when only functional diversity is considered (De Coster et al., 2015). For example, habitat loss had little or no influence on functional diversity but resulted in species loss and changes in species composition in forest bird communities (Coetzee and Chown, 2016; Matuoka et al., 2020). This highlights the need to incorporate more metrics in understanding the influence of urbanization on bird communities since functional diversity alone may not provide a comprehensive understanding of changes in bird communities.
The continuous growth in population will cause more people to live in cities by 2050. This highlights the need for a functioning ecosystem as it is critical for human well-being (Cardinale et al., 2012). While our results add to the already existing body of knowledge on the effects of urbanization on bird communities, it also gives insight into the future. Our results provide evidence that urbanized areas will allow functional diversity to increase while functional richness, taxonomic richness and diversity decline. A hundred percent probability of urban expansion leads to decrease in taxonomic richness, functional richness and taxonomic diversity but at the same time results in a corresponding increase in functional diversity. This result is critical for landscape management and urban planning. We should be cautious to draw conclusions that functional diversity increasing with urbanization is positive as this may not necessarily benefit bird conservation, ecosystem functioning and human wellbeing. As generalists replace specialists, they are able to provide a wide range of functional traits within urban centers. With more generalists, there is high niche overlap which eventually causes functional redundancy. While increased functional diversity in urban environments may mitigate the impact of species loss on ecosystem function to some extent, the loss of specific species within the ecosystem results in the disappearance of their unique traits. This can create gaps within the ecosystem, particularly, in communities with lower functional redundancy (Winfree et al., 2015). The replacement of specialists by generalists can also lead to functional redundancy as some niches are left unoccupied. For instance, if insectivorous birds that control insect population (Whelan et al., 2015) are filtered out of the cities, it could lead to pest outbreaks. Also, an increase in green areas will result in a corresponding increase in habitat diversity within cities, thus creating more niches that meet the ecological needs of bird communities (Oliviera et al., 2017). We therefore suggest that urban planners should incorporate natural vegetation within urban centers to maintain other community and functional indices within urban centers.
The original contributions presented in the study are included in the article/Supplementary Material. Further inquiries can be directed to the corresponding authors.
BD: Conceptualization, Data curation, Formal analysis, Investigation, Methodology, Software, Validation, Visualization, Writing – original draft, Writing – review & editing. PN: Conceptualization, Data curation, Formal analysis, Investigation, Methodology, Software, Validation, Visualization, Writing – original draft, Writing – review & editing. II: Conceptualization, Data curation, Formal analysis, Investigation, Methodology, Software, Validation, Visualization, Writing – original draft, Writing – review & editing. AK: Conceptualization, Funding acquisition, Investigation, Methodology, Project administration, Resources, Supervision, Validation, Writing – original draft, Writing – review & editing. TT: Conceptualization, Data curation, Funding acquisition, Project administration, Resources, Supervision, Writing – original draft, Writing – review & editing. SN: Data curation, Funding acquisition, Project administration, Resources, Validation, Writing – original draft, Writing – review & editing. PN: Data curation, Resources, Supervision, Validation, Writing – original draft, Writing – review & editing. CJ: Conceptualization, Supervision, Writing – original draft, Writing – review & editing. BO: Project administration, Resources, Writing – original draft, Writing – review & editing. UO: Conceptualization, Funding acquisition, Project administration, Resources, Supervision, Writing – original draft, Writing – review & editing. AC: Resources, Supervision, Writing – original draft, Writing – review & editing. RT: Conceptualization, Funding acquisition, Project administration, Resources, Supervision, Writing – original draft, Writing – review & editing, Methodology.
The author(s) declare financial support was received for the research, authorship, and/or publication of this article. The training workshops on analyzing citizen science data and communicating results, which contributed to this research, were funded by a UK Government Darwin Initiative grant (Grant #DARCC020) awarded to the Tropical Biology Association.
This work would not have been possible without the support of the Tropical Biology Association, in collaboration with the Kenya Bird Map and the Nigerian Bird Atlas Project, and the A.P. Leventis Ornithological Research Institute (APLORI). The training workshops on analyzing citizen science data and communicating results that contributed to this research were funded by a UK Government Darwin Initiative grant awarded to the Tropical Biology Association. We would also like to acknowledge the dedication of the citizen scientists whose tireless efforts in data collection have been invaluable in informing decisions that are shaping our planet today.
The authors declare that the research was conducted in the absence of any commercial or financial relationships that could be construed as a potential conflict of interest.
The author(s) declare that no Generative AI was used in the creation of this manuscript.
All claims expressed in this article are solely those of the authors and do not necessarily represent those of their affiliated organizations, or those of the publisher, the editors and the reviewers. Any product that may be evaluated in this article, or claim that may be made by its manufacturer, is not guaranteed or endorsed by the publisher.
The Supplementary Material for this article can be found online at: https://www.frontiersin.org/articles/10.3389/fcosc.2024.1503408/full#supplementary-material
Awoyemi A. G., Barshep Y., Manu S., Ibáñez-Álamo J. D. (2024). Associations between urbanization and avian communities in the Afrotropics: Evidence from taxonomic, functional and phylogenetic diversity. Global Ecol. Conserv. 54, e03108. doi: 10.1016/J.GECCO.2024.E03108
Awoyemi A. G., Ibáñez-Álamo J. D. (2023). Status of urban ecology in Africa: A systematic review’. Landscape Urban Plann. 233, 104707. doi: 10.1016/J.LANDURBPLAN.2023.104707
Baker P. J., Molony Susie E., Stone E., Cuthill I. C., Harris S. (2008). Cats about town: is predation by free-ranging pet cats Felis catus likely to affect urban bird populations? Ibis 150, 86–99. doi: 10.1111/J.1474-919X.2008.00836.X
Batáry P., Kurucz K., Suarez‐Rubio M., Chamberlain D. E. (2018). Non-linearities in bird responses across urbanization gradients: A meta-analysis. Global Change Biol. 24, 1046–1054. doi: 10.1111/gcb.13964
BirdLife International. (2024). ‘IUCN Red List for birds’. Available online at: https://datazone.birdlife.org/species/searchon10/11/2024 (Accessed November 10, 2024).
Blair R. B. (1996). Land use and avian species diversity along an urban gradient. Ecol. Appl. 6, 506–519. doi: 10.2307/2269387
Botta Dukát Z. (2005). Rao’s quadratic entropy as a measure of functional diversity based on multiple traits. J. Vegetation Sci. 16, 533–540. doi: 10.1111/J.1654-1103.2005.TB02393.X
Bregman T. P., Sekercioglu C. H., Tobias J. A. (2014). Global patterns and predictors of bird species responses to forest fragmentation: Implications for ecosystem function and conservation. Biol. Conserv. 169, 372–383. doi: 10.1016/J.BIOCON.2013.11.024
Bürkner P.-C. (2021). Advanced bayesian multilevel modeling with the R package brms. R J. 10, 395–411. doi: 10.48550/arXiv.1705.11123
Callaghan C. T., Chase J. M., McGlinn D. J. (2023). Anthropogenic habitat modification causes nonlinear multiscale bird diversity declines. Ecography. e06759. doi: 10.1111/ecog.06759
Cardinale B. J., Duffy J. E., Gonzalez A., Hooper D.U., Perrings C., Venail P., et al. (2012). Biodiversity loss and its impact on humanity. Nature 486, 59–67. doi: 10.1038/nature11148
Carpenter B., Gelman A., Hoffman M. D., Lee D., Goodrich B. Betancourt M., et al. (2017). Stan: A probabilistic programming language. J. Stat. Software 76, 1–32. doi: 10.18637/JSS.V076.I01
Carvajal-Castro J. D., Ospina-L, A. M., Toro-López Y., Pulido-G, A., Cabrera-Casas L. X., Guerrero-Peláez S., et al, et al. (2019). Birds vs bricks: Patterns of species diversity in response to urbanization in a Neotropical Andean city. PloS One 14, e0218775. doi: 10.1371/JOURNAL.PONE.0218775
Cazzolla Gatti R., Castaldi S., Lindsell J. A., Coomes D. A., Marchetti M., Maesano M., et al. (2015). The impact of selective logging and clearcutting on forest structure, tree diversity and above-ground biomass of African tropical forests. Ecol. Res. 30, 119–132. doi: 10.1007/S11284-014-1217-3/METRICS
Chace J. F., Walsh J. J. (2006). Urban effects on native avifauna: a review. Landscape Urban Plann. 74, 46–69. doi: 10.1016/J.LANDURBPLAN.2004.08.007
Chamberlain D., Kibuule M., Skeen R., Pomeroy D. (2017). Trends in bird species richness, abundance and biomass along a tropical urbanization gradient. Urban Ecosyst. 20, 629–638. doi: 10.1007/S11252-016-0621-6/METRICS
Chan K. M. A., Balvanera P., Benessaiah K., Chapman M., Díaz S., Gómez-Baggethun E., et al. (2016). Why protect nature? Rethinking values and the environment. PNAS 113, 1462–1465. doi: 10.13140/RG.2.1.5146.0560
Child M. F., Cumming G. S., Amano T. (2009). Assessing the broad-scale impact of agriculturally transformed and protected area landscapes on avian taxonomic and functional richness. Biol. Conserv. 142, 2593–2601. doi: 10.1016/J.BIOCON.2009.06.007
Coetzee B. W. T., Chown S. L. (2016). Land-use change promotes avian diversity at the expense of species with unique traits. Ecol. Evol. 6, 7610–7622. doi: 10.1002/ece3.2389
Cresswell W., Kazeh N. W., Patchett R. (2020). Local human population increase in the non-breeding areas of long-distance migrant bird species is only weakly associated with their declines, even for synanthropic species. Diversity Distributions 26, 340–351. doi: 10.1111/ddi.13006
Croci S., Butet A., Clergeau P. (2008). [amp]]lsquo;Does urbanization filter birds on the basis of their biological traits. Condor 110, 223–240. doi: 10.1525/COND.2008.8409
De Coster G., Banks-Leite C., Metzger J. P. (2015). Atlantic forest bird communities provide different but not fewer functions after habitat loss. Proc. R. Soc. B: Biol. Sci. 282. doi: 10.1098/rspb.2014.2844
DeGraaf R. M., Wentworth J. M. (1986). Avian guild structure and habitat associations in suburban bird communities. Urban Ecol. 9, 399–412. doi: 10.1016/0304-4009(86)90012-4
Díaz S., Lavorel S., de Bello F., Quétier F., Grigulis K., Robson T. M. (2007). Incorporating plant functional diversity effects in ecosystem service assessments. Proc Natl Acad Sci U. S. A. 104(52), 20684–20689. doi: 10.1073/PNAS.0704716104
Donnelly R., Marzluff J. M. (2006). Relative importance of habitat quantity, structure, and spatial pattern to birds in urbanizing environments. Urban Ecosyst. 9, 99–117. doi: 10.1007/S11252-006-7904-2/METRICS
Evans K. L., Chamberlain D. E., Hatchwell B. J., Gregory R. D., Gaston K. J. (2011). What makes an urban bird? Global Change Biol. 17, 32–44. doi: 10.1111/J.1365-2486.2010.02247.X
Ferenc M., Sedláček O., Fuchs R., Dinetti M., Fraissinet M., Storch D. (2014). Are cities different? Patterns of species richness and beta diversity of urban bird communities and regional species assemblages in Europe. Global Ecol. Biogeography 23, 479–489. doi: 10.1111/geb.12130
Filloy J., Zurita G. A., Bellocq M. I. (2019). Bird diversity in urban ecosystems: the role of the biome and land use along urbanization gradients. Ecosystems 22, 213–227. doi: 10.1007/S10021-018-0264-Y/METRICS
Fischer J. D., Cleeton S. H., Lyons T. P., Miller J. R. (2012). Urbanization and the predation paradox: the role of trophic dynamics in structuring vertebrate communities. BioScience 62, 809–818. doi: 10.1525/bio.2012.62.9.6
Fischer J. D., et al. (2015). Categorizing wildlife responses to urbanization and conservation implications of terminology Author. Conserv. Biol. 29, 1246–1248. doi: 10.1111/cobi
Fraixedas S., Lindén A., Piha M., Cabeza M., Gregory R., Lehikoinen A. (2020). A state-of-the-art review on birds as indicators of biodiversity: Advances, challenges, and future directions. Ecol. Indic. 118, 106728. doi: 10.1016/J.ECOLIND.2020.106728
García-Jiménez R., Morales-Reyes Z., Pérez-García J. M., Margalida A. (2021). Economic valuation of non-material contributions to people provided by avian scavengers: Harmonizing conservation and wildlife-based tourism. Ecol. Economics 187, 107088. doi: 10.1016/J.ECOLECON.2021.107088
García-Jiménez R., Pérez-García J. M., Margalida A., Morales-Reyes Z. (2022). Avian scavengers’ contributions to people: The cultural dimension of wildlife-based tourism. Sci. Total Environ. 806, 150419. doi: 10.1016/J.SCITOTENV.2021.150419
Garden J. G., Mcalpine C. A., Possingham H. P., Jones D. N. (2007). Habitat structure is more important than vegetation composition for local-level management of native terrestrial reptile and small mammal species living in urban remnants: A case study from Brisbane, Australia. Austral Ecol. 32, 669–685. doi: 10.1111/J.1442-9993.2007.01750.X
Gaston K. J. (2000). Global patterns in biodiversity. Nature 405, 220–227. Available at: www.nature.com.
Grenié M., Gruson H. (2023). fundiversity: a modular R package to compute functional diversity indices. Ecography 2023, e06585. doi: 10.1111/ecog.06585
Grimm N. B., Foster D., Groffman P., Grove J. M., Hopkinson C. S., Nadelhoffer K. J., et al. (2008). The changing landscape: ecosystem responses to urbanization and pollution across climatic and societal gradients. Front. Ecol. Environ. 6, 264–272. doi: 10.1890/070147
Haire S. L., Bock C. E., Cade B. S., Bennett B. C. (2000). The role of landscape and habitat characteristics in limiting abundance of grassland nesting songbirds in an urban open space. Landscape Urban Plann. 48, 65–82. doi: 10.1016/S0169-2046(00)00044-X
Ibáñez-Álamo J. D., Rubio E., Benedetti Y., Morelli F. (2017). Global loss of avian evolutionary uniqueness in urban areas. Global Change Biol. 23, 2990–2998. doi: 10.1111/GCB.13567
Isaksson C. (2018). Impact of urbanization on birds. Bird species 235, 257. doi: 10.1007/978-3-319-91689-7_13
Jonason D., Ekroos J., Öckinger E., Helenius J., Kuussaari M., Tiainen J., et al. (2017). Weak functional response to agricultural landscape homogenisation among plants, butterflies and birds. Ecography 40, 1221–1230. doi: 10.1111/ECOG.02268
Kaye J. P., Groffman P. M., Grimm N. B., Baker L. A., Pouyat R. V. (2006). A distinct urban biogeochemistry? Trends Ecol. Evol. 21, 192–199. doi: 10.1016/j.tree.2005.12.006
Kennedy C. M., Oakleaf J. R., Theobald D. M., Baruch‐Mordo S., Kiesecker J. (2019). Managing the middle: A shift in conservation priorities based on the global human modification gradient. Global Change Biol. 25, 811–826. doi: 10.1111/gcb.14549
Kennedy C. M., Oakleaf J. R., Theobald D. M., Baruch-Mordo S., Kiesecker J. (2020). Global human modification of terrestrial systems. (Palisades, NY: NASA Socioeconomic Data and Applications Center (SEDAC)). doi: 10.7927/edbc-3z60
Korányi D., Gallé R., Donkó B., Chamberlain D. E., Batáry P. (2021). Urbanization does not affect green space bird species richness in a mid-sized city. Urban Ecosyst. 24, 789–800. doi: 10.1007/S11252-020-01083-2/FIGURES/4
Lee A. T. K., Altwegg R., Barnard P. (2017). Estimating conservation metrics from atlas data: The case of southern African endemic birds. Bird Conserv. Int. 27, 323–336. doi: 10.1017/S0959270916000307
Lee A. T. K., Ottosson U., Jackson C., Shema S., Reynolds C. (2021). Urban areas have lower species richness, but maintain functional diversity: insights from the African Bird Atlas Project. Ostrich 92, 1–15. doi: 10.2989/00306525.2021.1902876
Lim H. C., Sodhi N. S. (2004). Responses of avian guilds to urbanization in a tropical city. Landscape Urban Plann. 66, 199–215. doi: 10.1016/S0169-2046(03)00111-7
Lundberg J., Moberg F. (2003). Mobile link organisms and ecosystem functioning: Implications for ecosystem resilience and management. Ecosystems 6, 87–98. doi: 10.1007/S10021-002-0150-4/METRICS
MacGregor-Fors I., Vázquez L., Vega-Rivera J. H., Schondube J. E. (2009). Non-exotic invasion of great-tailed grackles quiscalus mexicanus in a tropical dry forest reserve. Ardea, 97 (3), 367–369. doi: 10.5253/078.097.0312
MacLean S. A., Rios Dominguez A. F., de Valpine P., Beissinger S. R. (2018). A century of climate and land-use change cause species turnover without loss of beta diversity in California’s Central Valley. Global Change Biol. 24, 5882–5894. doi: 10.1111/GCB.14458
Magle S. B., Hunt V. M., Vernon M., Crooks K. R. (2012). Urban wildlife research: Past, present, and future. Biol. Conserv. 155, 23–32. doi: 10.1016/J.BIOCON.2012.06.018
Marzluff J. M. (2001). “Worldwide urbanization and its effects on birds,” in An urbanizing world. Eds. Marzluff J. M., Bowman R., Donnelly R. (Kluwer Academy, Boston), 331–364.
Marzluff J. M. (2016). A decadal review of urban ornithology and a prospectus for the future. Ibis 159, 1–13. doi: 10.1111/IBI.12430
Marzluff J. M., Bowman R., Donnelly R. (2001). “A historical perspective on urban bird research: trends, terms, and approaches,” in Avian Ecology and Conservation in an Urbanizing World (Springer, Boston, MA), 1–17. doi: 10.1007/978-1-4615-1531-9_1
Matuoka M. A., Benchimol M., de Almeida-Rocha J. M., Morante-Filho J. C. (2020). Effects of anthropogenic disturbances on bird functional diversity: A global meta-analysis. Ecol. Indic. 116, 106471. doi: 10.1016/J.ECOLIND.2020.106471
McKinney M. L. (2002). Urbanization, Biodiversity and Conservation: the impacts of urbanization on native species are poorly studied, but educating a highly urbanized human population about these impacts can greatly improve species conservation in all ecosystems. BioScience 52, 883–890. doi: 10.1641/0006-3568(2002)052[0883:UBAC]2.0.CO;2
McKinney M. L. (2006). Urbanization as a major cause of biotic homogenization. Biol. Conserv. 127, 247–260. doi: 10.1016/J.BIOCON.2005.09.005
Mckinney M. L. (2008). Effects of urbanization on species richness: A review of plants and animals. Urban Ecosyst. 11, 161–176. doi: 10.1007/s11252-007-0045-4
Melchiorri M. (2022). The global human settlement layer sets a new standard for global urban data reporting with the urban centre database. Front. Environ. Sci. 10. doi: 10.3389/fenvs.2022.1003862
Newbold T., Butchart S. H., Şekercioğlu Ç. H., Purves D. W., Scharlemann J. P. (2012). Mapping functional traits: comparing abundance and presence-absence estimates at large spatial scales. PloS One 7, 1–11. doi: 10.1371/journal.pone.0044019
Newbold T., Hudson L. N., Hill S. L., Contu S., Lysenko I., Senior R. A., et al. (2015). Global effects of land use on local terrestrial biodiversity. Nature 520, 45–50. doi: 10.1038/nature14324
Newbold T., Hudson L. N., Contu S., Hill S. L., Beck J., Liu Y., et al. (2018). Widespread winners and narrow-ranged losers: Land use homogenizes biodiversity in local assemblages worldwide. PloS Biol. 16, e2006841. doi: 10.1371/JOURNAL.PBIO.2006841
Njoroge J. B., NdaNg’ang’a P. K., Natuhara Y. (2014). The pattern of distribution and diversity of avifauna over an urbanizing tropical landscape. Urban Ecosyst. 17, 61–75. doi: 10.1007/S11252-013-0296-1/METRICS
Odino M., Imboma T., Ogada D. L. (2014). Assessment of the occurrence and threats to Hooded Vultures Necrosyrtes monachus in western Kenyan towns. Vulture News 67, 3–20. doi: 10.4314/vulnew.v67i2.1
Oksanen J., Simpson G., Szöcs E., Solymos P., Lahti L., Hannigan G., et al (2018). Vegan: community ecology package. Available online at: https://github.com/vegandevs/vegan (Accessed January 23, 2024).
Oliveira E. H., Hagen O., Ibáñez-Álamo J. D., Petchey O. L., Evans K. L. (2017). Impacts of urban areas and their characteristics on avian functional diversity. Front. Ecol. Evol. 5. doi: 10.3389/FEVO.2017.00084
Palacio F. X., Ibañez L. M., Maragliano R. E., Montalti D.. (2018). Urbanization as a driver of taxonomic, functional, and phylogenetic diversity losses in bird communities. Can. J. Zoology 96, 1114–1121. doi: 10.1139/CJZ-2018-0008
Pautasso M., Böhning‐Gaese K., Clergeau P., Cueto V. R., Dinetti M., Fernández‐Juricic E., et al. (2011). Global macroecology of bird assemblages in urbanized and semi-natural ecosystems. Global Ecol. Biogeography 20, 426–436. doi: 10.1111/J.1466-8238.2010.00616.X
Pauw A., Louw K. (2012). Urbanization drives a reduction in functional diversity in a guild of nectar-feeding birds. Ecol. Soc. 17. doi: 10.5751/ES-04758-170227
Petchey O. L., Gaston K. J. (2006). Functional diversity: back to basics and looking forward. Ecol. Lett. 9, 741–758. doi: 10.1111/j.1461-0248.2006.00924.x
Petersen T. K., Speed J. D., Grøtan V., Frøyen Y. K., Austrheim G. (2022). Urbanization and land-cover change affect functional, but not compositional turnover of bird communities. Urban Ecosyst. 25, 1679–1698. doi: 10.1007/s11252-022-01258-z
Pigot A. L., Bregman T., Sheard C., Daly B., Etienne R. S., Tobias J. A. (2016). Quantifying species contributions to ecosystem processes: A global assessment of functional trait and phylogenetic metrics across avian seed-dispersal networks. Proc. R. Soc. B: Biol. Sci. 283. doi: 10.1098/rspb.2016.1597
Pigot A. L., Sheard C., Miller E. T., Bregman T. P., Freeman B. G., Roll U., et al. (2020). Macroevolutionary convergence connects morphological form to ecological function in birds. Nat. Ecol. Evol. 4, 230–239. doi: 10.1038/s41559-019-1070-4
Proppe D. S., Sturdy C. B., St. Clair C. C. (2013). Anthropogenic noise decreases urban songbird diversity and may contribute to homogenization. Global Change Biol. 19, 1075–1084. doi: 10.1111/GCB.12098
Rao C. R. (1982). Diversity and dissimilarity coefficients: a unified approach. Theor. population Biol. 21, 24–43. doi: 10.1016/0040-5809(82)90004-1
R Core Team (2023). R: A language and environment for statistical computing (Vienna, Austria: R Foundation for Statistical Computing). Available at: https://www.R-project.org/.
Reis E., López-Iborra G. M., Pinheiro R. T. (2012). Changes in bird species richness through different levels of urbanization: Implications for biodiversity conservation and garden design in Central Brazil. Landscape Urban Plann. 107, 31–42. doi: 10.1016/J.LANDURBPLAN.2012.04.009
Ricotta C., de Bello F., Moretti M., Caccianiga M., Cerabolini B. E., Pavoine S. (2016). Measuring the functional redundancy of biological communities: a quantitative guide. Methods Ecol. Evol. 7, 1386–1395. doi: 10.1111/2041-210X.12604
S̜ekercioğlu C., Wenny D. G., Whelan C. J. (2016). Why birds matter: avian ecological function and ecosystem services. (Chicago, USA: University of Chicago Press).
Sandström U. G., Angelstam P., Mikusiński G. (2006). Ecological diversity of birds in relation to the structure of urban green space. Landscape Urban Plann. 77, 39–53. doi: 10.1016/J.LANDURBPLAN.2005.01.004
Sekercioglu C. H. (2006). Increasing awareness of avian ecological function. Trends Ecol. Evol. 21, 464–471. doi: 10.1016/j.tree.2006.05.007
Seto K. C., Fragkias M., Güneralp B., Reilly M. K. (2011). A meta-analysis of global urban land expansion. PloS One 6, 23777. doi: 10.1371/journal.pone.0023777
Seto K. C., Güneralp B., Hutyra L. R. (2012). Global forecasts of urban expansion to 2030 and direct impacts on biodiversity and carbon pools. Proc. Natl. Acad. Sci. United States America 109, 16083–16088. doi: 10.1073/PNAS.1211658109/SUPPL_FILE/PNAS.201211658SI.PDF
Shannon C. E., Weaver W. (1949). The Mathematical Theory of Communication (Champaign, IL, USA: University of Illinois Press).
Shochat E., Warren P. S., Faeth S. H., McIntyre N. E., Hope D. (2006). From patterns to emerging processes in mechanistic urban ecology. Trends Ecol. Evol. 21, 186–191. doi: 10.1016/j.tree.2005.11.019
Sol D., González‐Lagos C., Moreira D., Maspons J., Lapiedra O. (2014). Urbanization tolerance and the loss of avian diversity. Ecol. Lett. 17, 942–950. doi: 10.1111/ELE.12297
Sol D., Bartomeus I., González‐Lagos C., Pavoine S. (2017). Urbanization and the loss of phylogenetic diversity in birds. Ecol. Lett. 20, 721–729. doi: 10.1111/ELE.12769
Sol D., Trisos C., Múrria C., Jeliazkov A., González‐Lagos C., Pigot A. L., et al. (2020). The worldwide impact of urbanization on avian functional diversity. Ecol. Lett. 23, 962–972. doi: 10.1111/ele.13495
Suárez-Castro A. F., Maron M., Mitchell M. G., Rhodes J. R. (2022). Disentangling direct and indirect effects of landscape structure on urban bird richness and functional diversity. Ecol. Appl. 32, e2713. doi: 10.1002/EAP.2713
Tende T., Iniunam I. A., Ivande S. T., Awoyemi A. G., Danmallam B. A., Ringim A. S., et al. (2024). Citizen science mitigates the lack of distributional data on Nigerian birds. Ecol. Evol. 14. doi: 10.1002/ECE3.11280
Tilman D. (2001). “Functional Diversity”, in Encyclopedia of Biodiversity. Academic Press, Cambridge, 109–120.
Tobias J. A., Pigot A. L. (2019). Integrating behaviour and ecology into global biodiversity conservation strategies. Philos. Trans. R. Soc. B: Biol. Sci. 374. doi: 10.1098/rstb.2019.0012
UN (2012). World urbanization prospects: the 2011 revision. (Department of Economics and Social Affairs).
Underhill L. G. (2016). The fundamentals of the SABAP2 protocol. Biodiversity Observations 7, 1–12. Available online at: http://bo.adu.org.za/.
United Nations, Department of Economic and Social Affairs, Population Division. (2019). World Urbanization Prospects: The 2018 Revision (ST/ESA/SER.A/420). New York: United Nations.
United Nations Department of Economic and Social Affairs (2019). World urbanization prospects: the 2018 revision (ST/ESA/SER.A/420). (New York: Department of Economics and Social Affairs).
van Rensburg B. J., Peacock D. S., Robertson M. P. (2009). Biotic homogenization and alien bird species along an urban gradient in South Africa. Landscape Urban Plann. 92, 233–241. doi: 10.1016/J.LANDURBPLAN.2009.05.002
Villéger S., Mason N. W., Mouillot D. (2008). New multidimensional functional diversity indices for a multifaceted framework in functional ecology. Ecology 89, 2290–2301. doi: 10.1890/07-1206.1
Whelan C. J., Şekercioğlu Ç.H., Wenny D. G. (2015). Why birds matter: from economic ornithology to ecosystem services. J. Ornithology 156, 227–238. doi: 10.1007/S10336-015-1229-Y/METRICS
Winfree R., Fox J. W., Williams N. M., Reilly J. R., Cariveau D. P. (2015). Abundance of common species, not species richness, drives delivery of a real-world ecosystem service. Ecol. Lett. 18 (7). doi: 10.1111/ele.12424
Keywords: Afrotropical bird communities, citizen science, conservation strategies, functional diversity, urbanization
Citation: Danmallam BA, Ngila PM, Iniunam IA, Kuria A, Tende T, Ngugi S, Njoroge P, Jackson C, Okoth B, Ottosson U, Chaskda AA and Trevelyan R (2024) Ecological consequences of urbanization in Afrotropical bird communities: present and future prospects. Front. Conserv. Sci. 5:1503408. doi: 10.3389/fcosc.2024.1503408
Received: 28 September 2024; Accepted: 13 November 2024;
Published: 10 December 2024.
Edited by:
Monte Neate-Clegg, University of California, Los Angeles, United StatesReviewed by:
Timothy C. Haas, University of Wisconsin–Milwaukee, United StatesCopyright © 2024 Danmallam, Ngila, Iniunam, Kuria, Tende, Ngugi, Njoroge, Jackson, Okoth, Ottosson, Chaskda and Trevelyan. This is an open-access article distributed under the terms of the Creative Commons Attribution License (CC BY). The use, distribution or reproduction in other forums is permitted, provided the original author(s) and the copyright owner(s) are credited and that the original publication in this journal is cited, in accordance with accepted academic practice. No use, distribution or reproduction is permitted which does not comply with these terms.
*Correspondence: Bello A. Danmallam, YWRhbXViZWxsbzAwMUBnbWFpbC5jb20=; Peggy M. Ngila, bmdpbGFwZWdneUBnbWFpbC5jb20=; Iniunam A. Iniunam, aS5pbml1bmFtMkBnbWFpbC5jb20=
†These authors have contributed equally to this work and share first authorship
Disclaimer: All claims expressed in this article are solely those of the authors and do not necessarily represent those of their affiliated organizations, or those of the publisher, the editors and the reviewers. Any product that may be evaluated in this article or claim that may be made by its manufacturer is not guaranteed or endorsed by the publisher.
Research integrity at Frontiers
Learn more about the work of our research integrity team to safeguard the quality of each article we publish.