- 1Albert Katz International School for Desert Studies, Jacob Blaustein Institutes for Desert Research, Ben-Gurion University of the Negev, Midreshet Ben-Gurion, Israel
- 2Mitrani Department of Desert Ecology, Swiss Institute of Dryland, Environmental and Energy Research, Jacob Blaustein Institutes for Desert Research, Ben-Gurion University of the Negev, Midreshet Ben-Gurion, Israel
- 3Southern Arava R&D, Eilot Regional Council, D.N., Hevel Eilot, Israel
- 4The Arava Institute for Environmental Studies, D.N., Hevel Eilot, Ketura, Israel
Insectivorous bats are an important group of natural enemies that use echolocation for communication, navigation, and foraging. While foraging, bats often eavesdrop on calls from other foraging individuals and are attracted to them as these indicate prey abundance. In addition, they use hedgerows such as tree lines in agroecosystems for commuting and foraging. In the present study, we employed two approaches to enhance the activity of desert-dwelling bats in fields of melon, pumpkin and onion in the hyper-arid Arava desert, Israel. We predicted that both approaches will enhance the activity of the bats in these agricultural fields. We broadcasted playbacks of echolocation calls of two common desert bats, Hypsugo ariel and Eptesicus bottae over the crop fields as a direct means and used gaps in windbreak lines made of planted trees Tamarix spp. as indirect means. Playback manipulation had differing results between the two species. The activity of H. ariel was initially higher in the control plot, but as broadcasting continued, the manipulated plot exhibited significantly higher activity over onion fields. In contrast, the activity of E. bottae declined over Cucurbit fields in the manipulated plots. These differences may reflect differences between the species in the level of sociality while foraging. The testing of the indirect approach showed that total bat activity and feeding buzzes ratio were significantly higher in small windbreak gaps compared to plots with continues tree line. Our results indicate the potential and the limitations of enhancing bat activity by broadcasting echolocation calls in open crop fields and the importance of landscape features which increase the heterogeneity of the crop fields to attract natural enemies in Conservation Biological Control.
1 Introduction
One of the main goals of sustainable agriculture is the reduction of pesticides usage while maintaining high crop production (Reganold et al., 1990; Velten et al., 2015). A promising approach for achieving this goal is Conservation Biological Control (CBC), in which the activity of the natural enemies of the pest, such as insectivorous bats, is enhanced (Barbosa, 1998; Kunz et al., 2011). Insectivorous bats, which account for 70% of all bat species (over 1400 species, Simmons and Cirranello, 2024) may have a major impact on arthropods, as they can locate and exploit bursts of insects populations (Aizpurua et al., 2018; Cohen et al., 2020). This foraging behavior may in turn provide efficient pest control in various agricultural systems and crops (Russo et al., 2018), and their contribution to pest control has been demonstrated to be economically significant (Cleveland et al., 2006; Taylor et al., 2018; Rodríguez-San Pedro et al., 2020). For example, Boyles et al. (2011) estimated that insectivorous bats may provide $22.9 billion/year worth of pest suppression for the agriculture industry in the United States alone.
Currently, much of the research on bats in agroecosystems is focused on understanding the practices and management schemes that can enhance bat activity, species richness and their functionality in pest control (Tuneu-Corral et al., 2023). Enhancement of bat activity can be obtained indirectly by methods that are generally used to support biodiversity and ecosystem services at the landscape scale. These may include diversification of habitats, land sparing, where natural habitat patches are preserved within the agricultural landscape (Lindell et al., 2018) and the preservation and management of hedgerows at the edge of the field crop (Froidevaux et al., 2019). Within the cultivated areas, organic farming (Wickramasinghe et al., 2003) and crop diversification (Olimpi and Philpott, 2018) were also found to be key factors in promoting bat activity. However, while indirect methods may increase bat abundance and activity, they may simultaneously affect the activity of pests as well (Shields et al., 2019), so that the net effect of these indirect methods on pest abundance may be positive, neutral, or even negative (Olimpi and Philpott, 2018). In addition to these general practices, more direct methods that specifically enhance bat activity, species richness and foraging have been developed. For example, installing bat houses around rice paddies in Spain led to an increase in bat population size and activity, accompanied by a significant decrease in infested rice stems (Puig-Montserrat et al., 2015). Similarly, placing artificial pools in date plantations increased the activity of desert-dwelling bats (Arzi et al., 2023). In our research, we tested both indirect and direct methods for enhancing bat activity in a hyper-arid agroecosystem. As a direct approach, we used a species-specific broadcast to attract local insectivorous bats to cultivated fields.
Bats use various vocal signals to communicate and to perceive their environment (Fenton, 2003). One type of those vocal signals is that of echolocation calls, which are used for navigation and prey capture (Griffin et al., 1960; Kalko, 1995). By eavesdropping on echolocation calls of foraging individuals, neighboring individuals may obtain useful information on the presence, identity and activity of the other foragers (Gillam, 2007; Kazial et al., 2008). Moreover, echolocations can also be used as a proxy for prey availability; especially when the calls include feeding buzzes (Griffin et al., 1960). Hence, eavesdropping potentially leads to the aggregation of foraging bats (Prat and Yovel, 2020); a behavioral response that can be utilized in various experimental manipulations and as a management tool to enhance bat activity for pest control purposes. Previous studies have shown that playbacks of different calls of bats such as social calls (Hill and Greenaway, 2005; Braun De Torrez et al., 2017), distress calls (Quackenbush et al., 2016), and foraging echolocation calls (Gillam, 2007; Übernickel et al., 2013; Aylen et al., 2022) led to higher activity and/or higher capture rates of bats (bat luring). However, the use of playbacks in agroecology systems to enhance bat activity for pest control has not been tested yet. In addition to the direct approach, we tested the effect of small gaps in planted tree lines along field margins (i.e. windbreaks) on the foraging behavior of bats.
Windbreaks, typically made of natural elements such as trees and shrubs, play an important economic and ecological role in agriculture (Dix and Leatherman, 1988; Brandle et al., 1992). Mechanically, tree lines alter wind velocity, which lead to a changing microclimate, especially on the leeward side (Brandle and Finch, 1991; Brandle et al., 2004). This alteration of microclimate may benefit the crop yields in various agroecosystems (Cleugh, 1998; Nuberg, 1998). In addition to their physical effect, windbreaks influence the local fauna and their movement ecology. Windbreaks provide sheltered zone for various insects, including potential pests and natural enemies (Pasek, 1988; Dix et al., 1995), which tend to accumulate particularly on the leeward side (Lewis and Dibley, 1970; Pasek, 1988). Bat activity may also be affected by windbreaks. Naturally, bats track the insect abundance and benefit from the accumulation of insect near the windbreak (Downs and Racey, 2006; Foxley et al., 2023). However, bats have been shown to use tree lines for commuting, regardless of wind or insect abundance (Verboom and Spoelstra, 1999). The accumulation of bat activity along tree lines at field boundaries is a well described phenomena (Verboom and Huitema, 2010; Wolcott and Vulinec, 2012; Harms et al., 2020). Yet, the drivers and promoters of this phenomenon still need further investigation. For example, Foxley et al. (2023) have shown how different characteristics of the field boundaries contributed to bat activity, as they suggested that small gaps in tree lines had benefited the activity of Pipistrellus pipistrellus.
In light of the above, we combined observational (our indirect approach) and manipulation-based (our direct approach) experiments to address two research questions regarding bat activity in relation to agricultural pest services. We expect that the results of these two approaches will give a better insight into the potential of echolocation broadcasting (bat luring) as a bat activity enhancer in an agricultural context and show the importance of small gaps within windbreaks for foraging bats in an arid agriculture environment. We hypothesized that bats would respond to foraging echolocation calls of other bats and predicted that they will especially respond to conspecific calls while heterospecific activity will remain unchanged. For the indirect approach, we predicted that small gaps (5-15 meters) at the windbreak barrier of singular tree line will positively affect bat activity and foraging along it. Both approaches were tested in open vegetable agricultural fields in the hyper-desert area of the Arava Valley, Israel.
2 Materials and methods
2.1 Study area
The study was carried out in an agricultural landscape located in the southern Arava Valley, Israel (Figure 1). The Arava Valley is a long and narrow valley (165 km long and 5-15 km wide), located in a hot and hyper-arid desert (Goldreich and Karni, 2001). Mean annual temperature is 25.4 °C (January: 15.8°C - July: 33.8°C). Average annual precipitation, which is mostly confined to a few rainy days in the winter (October-May), is 27.6 mm, with extreme interannual variation1. Most agricultural production in that region is completely dependent on irrigation to grow seasonal vegetables (during Spring and Autumn). Sixteen species of insectivorous bats were observed within the agricultural ecosystems in the study area, and some were found to provide biocontrol services in the regional date plantations (Hackett et al., 2013; Schäckermann et al., 2022; Arzi et al., 2023). Most of the agriculture fields, in which we conducted our experiment and monitoring, are surrounded by planted windbreak barriers (singular tree line, genus Tamarix).
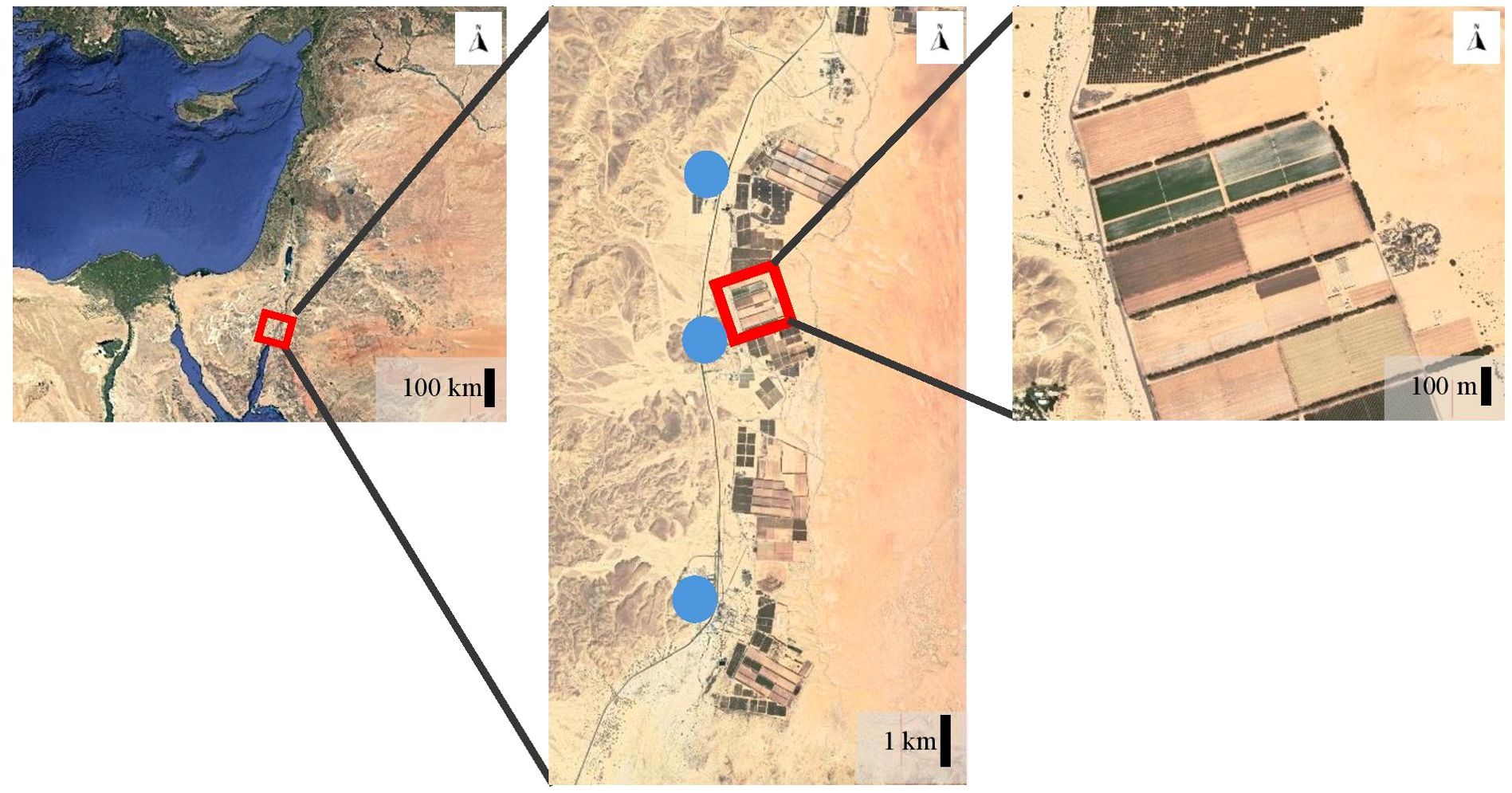
Figure 1. Map of the study area in the Arava Valley, Israel. Left: Regional map, the study area is marked with a red square. Center: Agriculture fields that were used for both the broadcast experiment and the gap assessment. Experiment sites were scattered along this presented area, blue circles mark the villages of: Ktura, Grofit, Yotvata. Right: An example of the study fields bordered by a planted singular tree line. (Maps extracted from Google Earth, https://earth.google.com/web/).
2.2 Experimental design
We conducted a field experiment and a field assessment in which we recorded bat activity above crop fields in relation to the broadcasting of calls or to windbreak hedgerows formation. As Bat activity, in general, varies considerably in both space (Kunz, 1973) and time (Hayes, 1997), we a) restricted our experiment to specific locations and temporal windows, and b) paired experimental plots with control plots, both in space and in time. First, as bat activity might be affected by moon phase (Appel et al., 2017), we limited the experiment to nights with less than 50% moonlight. Second, bat activity was always monitored simultaneously in paired plots (treatment and control). The plots were paired so that they exhibited similar structure in terms of vegetation (crop type and crop stage) and field spatial orientation (direction of the field and field edge structure) (Heim et al., 2018; Rodríguez-San Pedro et al., 2018).
2.3 Broadcasting experiment
The experiment was performed between October and November 2021 at 16 different sites that at the time of the experiment were planted with either cultivated pumpkin, Cucurbita pepo (3 sites), melon, Cucumis melo (6 sites), or onion, Allium cepa (7 sites). Pumpkin and Melon belong to the same family (Cucurbits) and share very similar pests in the study region (Dobrinin, 2020). Thus, we treated them as a single crop type (Cucurbits) in the experimental design and for any further analysis. In total, we conducted the manipulation experiment for 20 detector nights in onion fields, and 20 detector nights in Cucurbits fields. During each night of the experiment, we conducted the experiment in two sites that were at least 400 meters away from one another to minimize the possible influence of one set on the other. Within each site, we placed paired plots (a Broadcasting manipulation plot and a Control plot) in two corners of the same field that were separated by 200-300 meters (Supplementary Figure S1). In the broadcasting manipulation plot, three ultrasonic speakers were positioned, mounted on tripods 1.5 meters above ground, angled 30° upward from the horizon and facing different directions. A bat detector was placed one meter behind the speakers and mounted on a tripod one meter above the ground, pointing 45° upward from the horizon (Supplementary Figure S1). This setting of the bat detectors and speakers was designed to minimize the pseudo-recordings of the playback by the detector. The control plot included three tripods (without speakers) and a bat detector in the same setting as in the manipulation treatment.
On each experimental night, we monitored bat activity from sunset until 45 minutes before dawn. However, the broadcast started 100 minutes after sunset. This time was chosen according to the observed activity time of the two common tested bat species (see next section on calls recordings and broadcasting) in the study area (Schäckermann et al., 2022). Thus, each experimental night was divided distinctively into three phases: a pre-broadcasting phase of 100 minutes, a broadcasting phase of 160 minutes, and a post-broadcasting phase of 400 minutes (Supplementary Figure S2).
2.4 Call recordings and broadcasting
We created a playlist consisting of full-echolocation call sequences of the two most active bat species in the study site (Hypsugo ariel and Eptesicus bottae, Schäckermann et al., 2022). The broadcasted echolocation sequences had been recorded at the study site with a Wildlife Acoustic Song Meter SM4, including an external ultrasonic microphone SMM-U1 (Wildlife acoustics, Inc., USA) at a sample rate of 96 kHz. Echolocation sequences which matched the clearness criteria (no sound distortion, overlapping of bat calls and loud background noises on the file) were edited with SASLAB PRO (Avisoft Bioacoustics, version 5.2.15, Germany) into short duration sequences (2.5-4 seconds), and background noises were removed by using high pass filtering (cutoffs: 40khz for H. ariel and 28khz for E. bottae). In total, the playlist consisted of 15 echolocation sequences of H. ariel and 9 sequences of E. bottae.
As mentioned above, the broadcasting phase in each experimental night lasted for 160 minutes. These 160 minutes were composed of 8 sections of 20 minutes. Each of these sections was composed of 10 minutes in which a unique combination of call sequence was broadcasted (‘broadcasting temporal unit’) and 10 minutes of silence (‘silence temporal unit’), which served as the local baseline (natural) bat activity (Supplementary Figure S2). These playlists were loaded on two computers: Toshiba Satellite Pro R50-B with a Realtek high-definition audio soundcard (version: 6.0.1.7521) and Dell Latitude E5410 with an IDT high-definition audio CODEC soundcard (version: 6.10.0.6292). Both computers broadcasted the playlist via the ultrasonic loudspeaker AVISOFT USG player BL Pro 2 (Avisoft Bioacoustics, Germany) with a 16-bit resolution and a 96 kHz sampling rate, that was connected to two dynamic ultrasonic “vifa” speakers (Avisoft Bioacoustics, Germany). The speakers were powered by 3 external 12V, 7.2A acid batteries (ROSTEC Advanced Technologies Ltd, RC12-7S, Israel). The volume was adjusted manually each night to ensure maximum broadcast volume while avoiding sound distortions, as indicated by a clipping alert bulb on the speaker.
2.5 Windbreak gap assessment
We monitored bat activity throughout the entire night across 12 different sites in the Arava Valley, sampling two sites each night. All sites were located at the margin of open fields used for growing seasonal vegetables and bordered by windbreaks. At the time of the monitoring, only 6 of these sites were cultivated with pumpkin, melon or onion. The windbreaks consisted of a single row of Tamarix spp. trees, approximately 10 meters tall. These linear single tree lines occasionally had gaps of 5-15 meters where agricultural dirt road intersected them. We placed a bat detector 10 meters away from the gap, facing the gap to monitor the activity within this area (“gap treatment”). A control detector was positioned 200 meters away along the same tree line as the gap, in a continues section without gaps (Supplementary Figure S3). Both detectors were posited on the leeward side of the windbreak.
2.6 Acoustic bat monitoring data processing and analysis
In both experiments, we monitored bat activity from sunset until 45 minutes before dawn. We sampled bat foraging activity using an acoustic bat detector (AnaBat SD2, Titley Electronics, Australia). Bat call analysis software (AnalookW, version 4.1z, www.hoarybat.com) was used to manually analyze the recorded calls, counting bat passes and feeding buzzes (FB). Calls were classified into species through visual examination of the sonogram based on species-specific acoustic characteristics known for the bats in the research area (Hackett et al., 2017). A sequence of calls was counted as a single bat pass if it met the two following criteria: a) it included at least two consecutive clear calls from the same species (Gillam, 2007), b) the gap between two consecutive calls in the sequence was shorter than half of the total length of the whole sequence (Song et al., 2019). Otherwise, the sequence was split and counted as separate bat passes. To eliminate the possibility of counting pseudo-recordings (i.e. recording of the broadcasted calls) as bat passes, we excluded from the analysis any recordings that had repeated bat sequences patterns that are typical of the composed playlists.
2.7 Statistical analysis
2.7.1 Broadcast experiment
To test the factors that affect bat activity, we ran Generalized Linear Mixed Models (GLMM). All models were initiated from the same complete model structure, which included the following fixed variables: crop type, plot type, temporal unit (broadcast/silent), night phase and their interactions of interest. Environmental covariates included average nightly temperature, average nightly wind speed taken from Yotvata station of the Israel Meteorological Service1 approximately 1-4 km from the agricultural fields. Site (site ID), detector and computer were defined as random variables. Bat passes were modeled using a negative binominal error distribution with a log-link function. To test the effect of the manipulation under different time scales, we generated different GLMM models for each time scale: (1) night phase - testing the effect before, during, and after the broadcasting. (2) sum of broadcast vs. silent - comparing the sum of temporal units within the broadcasting phase (80 minutes of broadcast vs. 80 minutes of silent). (3) Broadcast vs. silent temporal units – comparing the 10 minutes of silent vs. the following 10 minutes of broadcast. Due to the different durations of the night phases, bat activity for the comparison of night phases was normalized to “bat passes per 60 minutes”. Cases of empty blocks, meaning no activity in both compared units, were excluded from the analysis. GLMM models were ran by using Jamovi software (version 2.3.28, The Jamovi project, 2021).
For each analysis, we employed a model selection procedure in which we maintained the fixed variables that are inherent to the specific hypotheses that we tested, and we allowed the removal of other variables (e.g. covariates) whenever they did not contribute to the model fit, as indicated by a reduction of 2 points or more from the Akaike’s Information Criteria (AIC, Arnold, 2010).
2.7.2 Wind gap assessment
We applied GLMM models for both response variables: bat passes and FB ratio. The FB ratio was calculated by dividing the total count of feeding buzzes by the total count of bat passes for the whole night. All analyses were initiated with the same full model and variables that didn’t contribute to the model fit were removed sequentially according to a model selection procedure, as described for the broadcasting experiment. The initial models included the fixed variable of tree line treatment and the environmental factor of average nightly temperature. Site was defined as a random variable. The response variable of ‘FB ratio’ was modeled using a Gamma error distribution with a log-link function, while ‘Bat passes’ were modeled using a negative binominal error distribution with a log-link function.
3 Results
3.1 Broadcasting experiment
Throughout the broadcasting experiment, we recorded and identified 18,332 bat passes, with 98% identified to the species level. On average, 235.05 ± 25.08 bat passes were recorded each night. A total of 12 species were found active within the field crops, with four dominant species accounting for 86% of the total bat passes: Hypsugo ariel (46%), Rhinopoma cystops (24%), Tadarida teniotis (9%), and Eptesicus bottae (8%). Two of these species were mainly active either before (Rhinopoma cystops) or after (Tadarida teniotis) the manipulation phase of the experiment. Therefore, we didn’t analyze their activity in response to the manipulation. One species, Rhinolophus hipposideros, was extremely rare, with only two identified passes throughout the entire experiment (Supplementary Table S1).
We found that bat activity (for all species combined) significantly decreased throughout the night (Table 1). Additionally, there was a significant positive effect of average night temperature on bat activity (Table 1). Species-specific analysis for H. ariel and E. bottae revealed a significant effect of crop type on the activity of E. bottae (GLMM, X² df=1 = 11.72, P < 0.001). Eptesicus bottae exhibited a higher rate of bat passes in the cucurbit fields compared to the onion fields (5.95 ± 1.12, 2.24 ± 0.68, respectively per 60 minutes of activity, Figure 2). In contrast, H. ariel did not show a significant difference in activity between the two crops (15.4 ± 2.64, 13.5 ± 2.11 respectively, Figure 2).
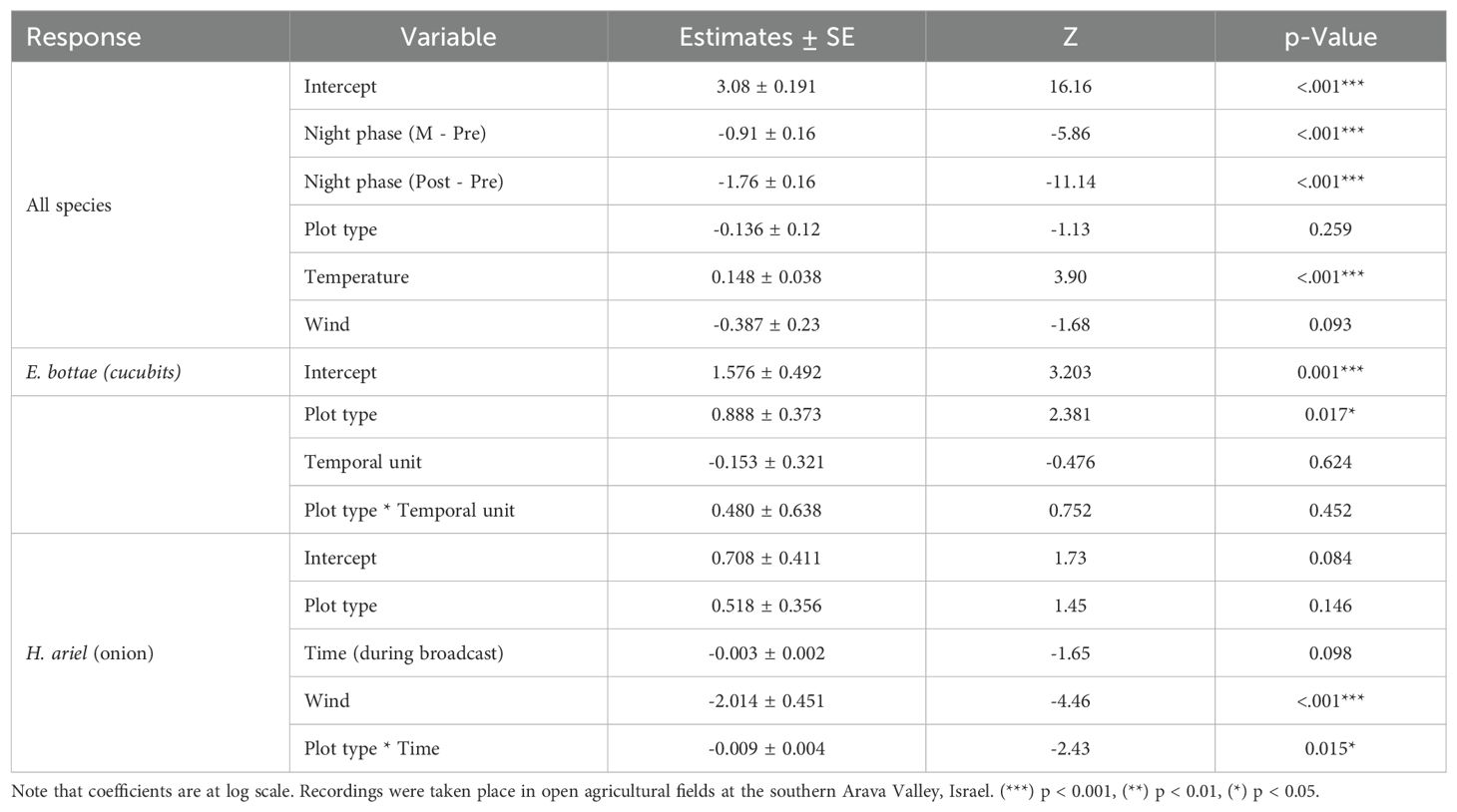
Table 1. Results of the selected GLMM models testing the effect of broadcast manipulation composed of playback of echolocation calls and silence units at three scales: all species together, Eptesicus bottae and Hypsugo ariel.
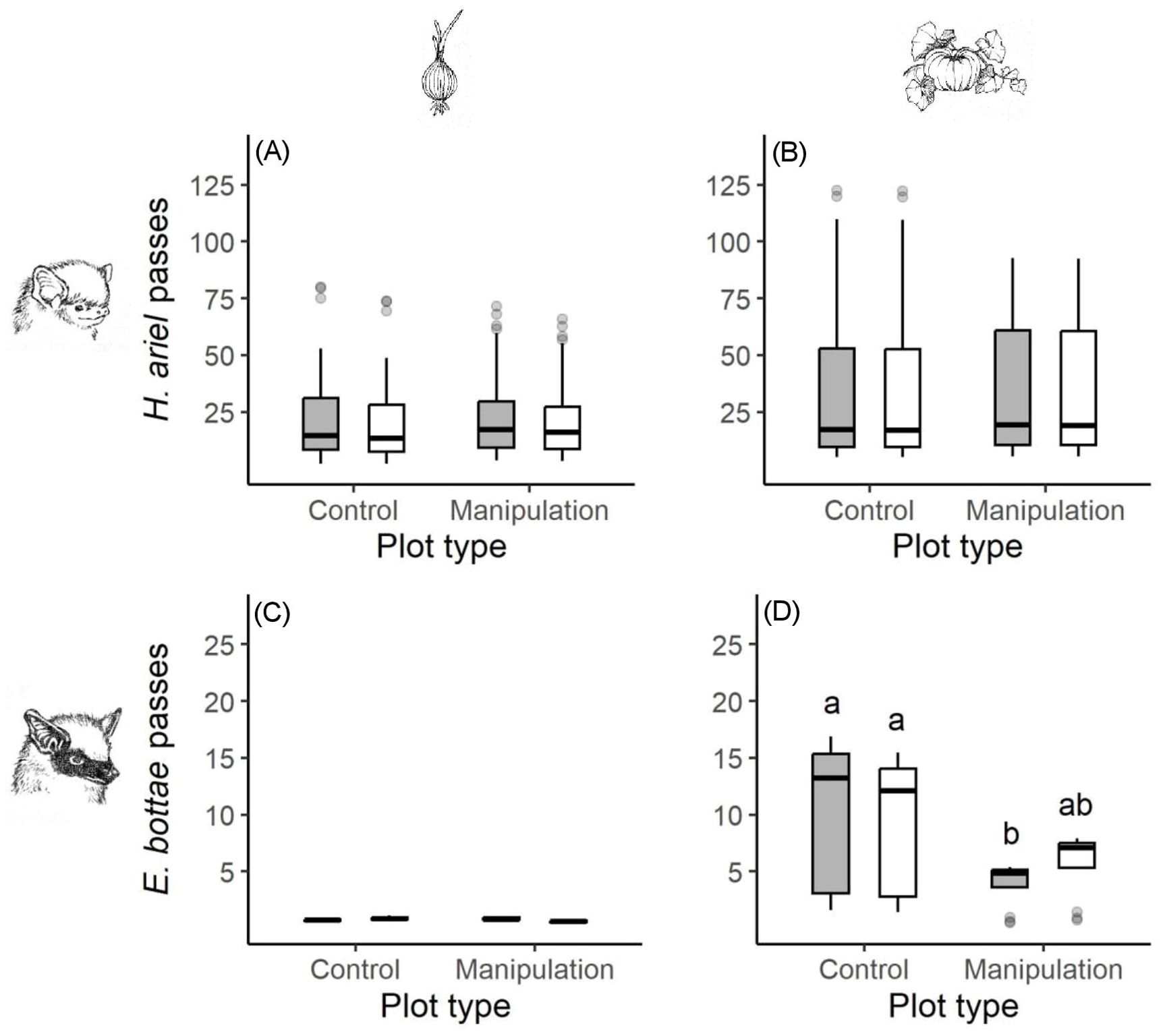
Figure 2. Average passes in 60 minutes for Hypsugo ariel in onion (A) and cucurbits (B) and average bat passes of Eptesicus bottae in onion (C) and cucurbits (D). The average passes for each temporal unit within the plot types are represented in grey for the broadcast unit, and white for the silent unit. Broadcast unit is phase when echolocations calls were played, and silence unit is the phase between each broadcasting units. Different alphabetic letters represent significant difference between the plot types and temporal units. Recordings were taken place in open agricultural fields at the southern Arava Valley, Israel. (Illustration of bats and crops, © Yuval Siboni).
Analysis of H. ariel activity across both cucurbit and onion fields revealed no significant difference in activity between the broadcasting manipulation and the control plots nor between the temporal units (broadcast and silent units, Figure 2). Analysis for the onion fields alone showed a significant interaction between time and plot type (Table 1). At the beginning of broadcasting phase, H. ariel activity was higher in the control plot (the plot without actual broadcasting), but as the broadcasting continued, the manipulated plot exhibited higher activity than the control (Figure 3). A similar pattern was observed also in the cucurbit fields analysis, though the trend was not significant. Additionally, there was a significant negative effects of wind speed on the activity of H. ariel (Table 1).
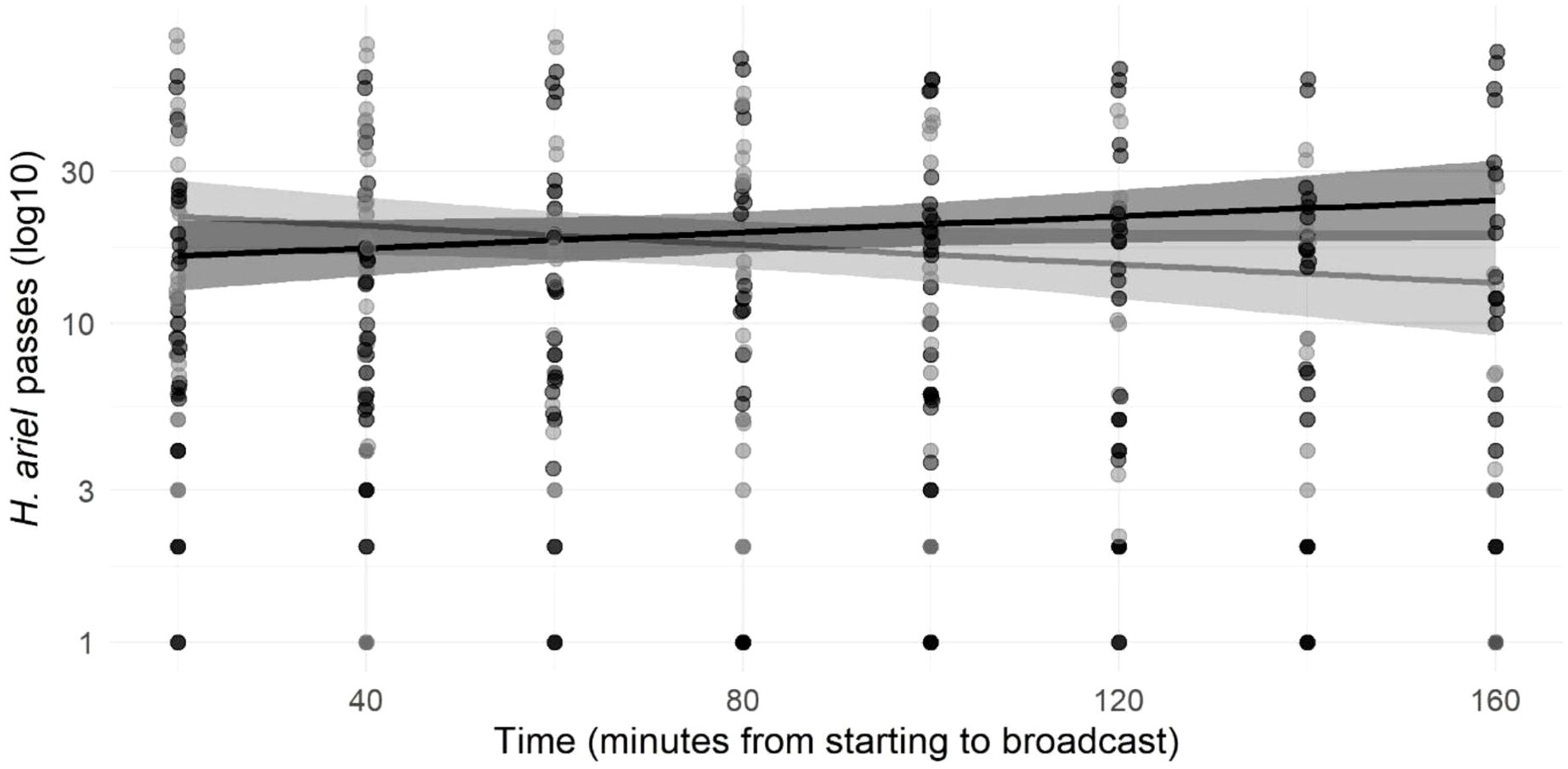
Figure 3. GLMM model comparing the activity of Hypsugo ariel during the broadcasting phase between control and manipulation plots. The darker line represents activity in the manipulated plot, while the lighter line indicates activity in the control plot. Data points are colored accordingly. Note that the Y-axis is log-transformed. Recordings were conducted in open agricultural fields in the southern Arava Valley, Israel.
Analysis of E. bottae activity in the cucurbit fields during the broadcasting phase revealed a significant decrease in activity in the manipulated plot compared to the control plot (Table 1, Figure 2). However, within each plot type, the activity level of E. bottae did not differ between the broadcasting and the silence temporal units (Table 1, Figure 2).
3.2 Windbreak gap assessment
Throughout the Windbreak gap assessment, we recorded and identified 10,216 bat passes, belonging to 12 species (the same species assembly as in the broadcasting experiment). Additionally, 554 FB were recorded. Bat activity was significantly affected by the gap, with higher rates of bat passes and higher FB ratio at the gap compared to the continues tree line (Table 2). On average, 477.25 ± 84.08 passes per night with a FB ratio of 0.07 ± 0.02 were recorded at the gap, compared to 374.08 ± 62.75 passes with a FB ratio of 0.02 ± 0.01 at the control (Figure 4).
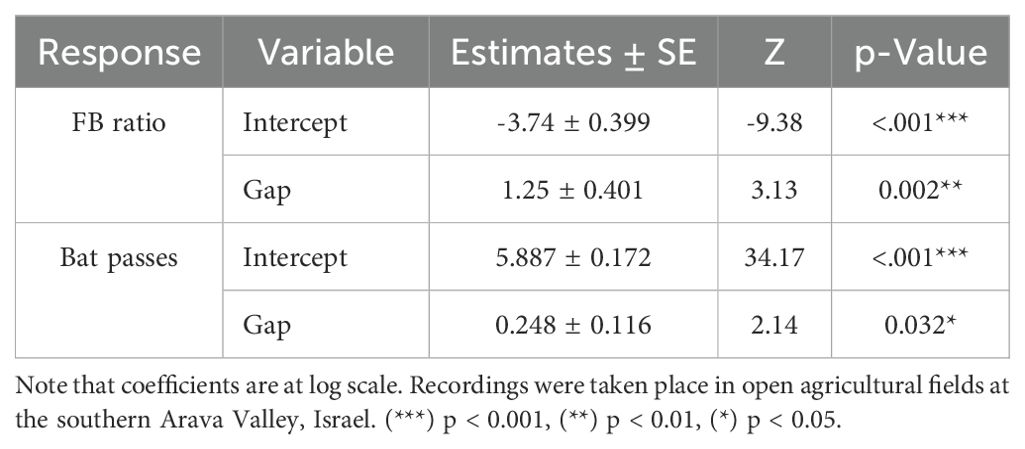
Table 2. Results of the top GLMM models testing the effect of bat activity in gaps compared to continues treeline bordering agricultural fields.
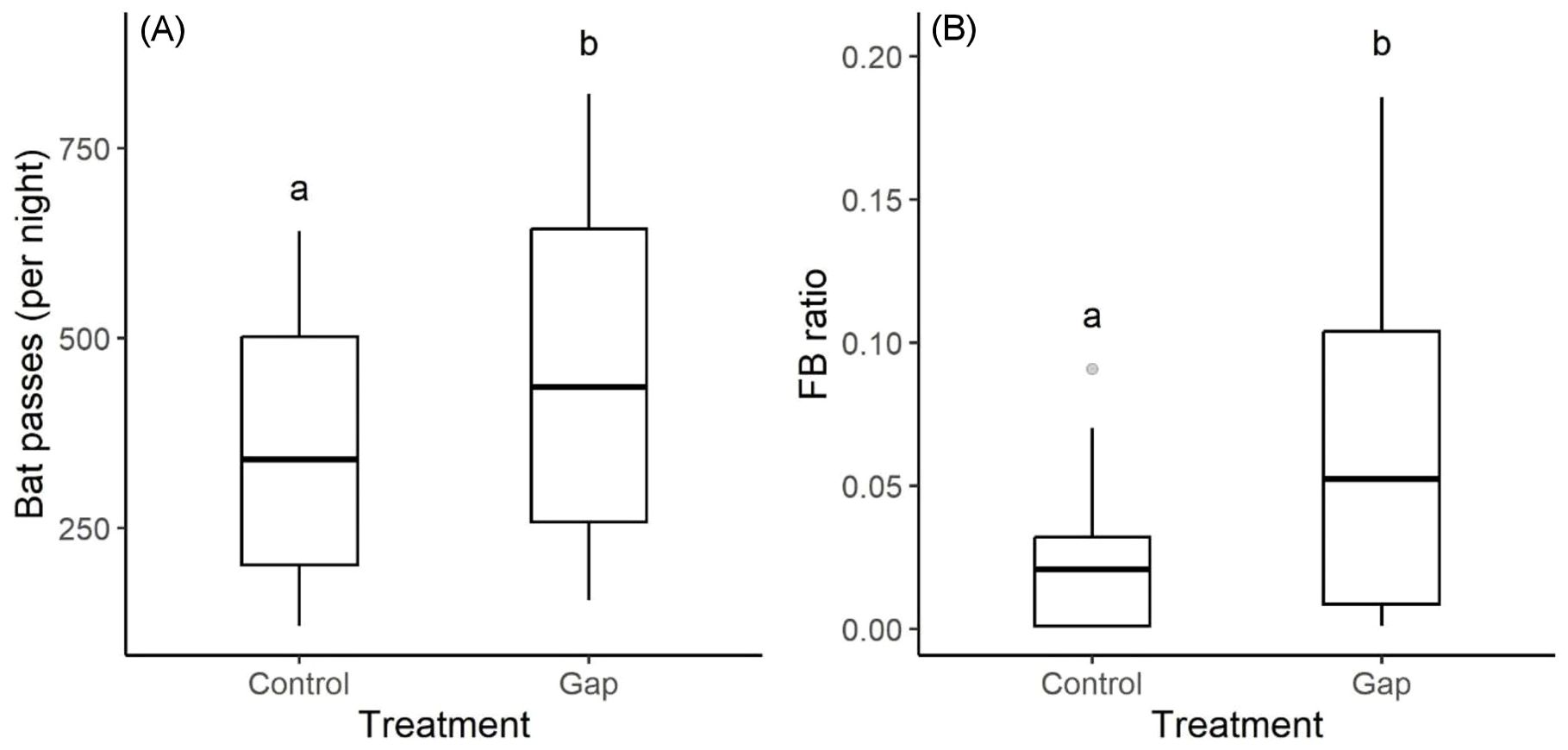
Figure 4. Results of the windbreak gap assessment, in which bat activity was compared between the gap and the continues tree line. Average count of all species passes per night (A) and average FB ratio (FB/total bat passes) per night (B). Different alphabetic letters represent significant differences between the gaps and the tree lines. Recordings were taken place in open agricultural fields at the southern Arava Valley, Israel.
4 Discussion
Our results suggest that both the direct approach (broadcast manipulation) and the indirect approach (windbreak) may enhance bat activity. In addition, our study also highlights the limitations of implementing the proposed practices as part of CBC in a hyper-arid desert environment. Despite the scarcity of water in arid environments (Noy-Meir, 1974), agriculture practices have become common, successful and economically-feasible in arid lands due to the development and implementation of efficient irrigation practices (Fernández-Cirelli et al., 2009). The irrigated land often supports large insect populations that thrive due to the high availability of nutrients, water, and increased humidity (Chown and Terblanche, 2006). Consequently, these large populations are including pests that may serve as a primary food source for several species of desert-dwelling bats (Schäckermann et al., 2022). Our finding indicates that 12 of the 16 bat species that are known from the region (Yom-Tov and Kadmon, 1998) were foraging at these studied crops. The monitored bat activity levels in this study during summer and early autumn were relatively high (≈250 bat passes per night) comparable to activity levels that were measured in other systems in the southern Arava. For example, approximately 400 bat passes per night were recorded during summer in nearby date plantations (Schäckermann et al., 2022; Arzi et al., 2023), while at a natural habitats of singular Acacia trees at the norther part of the Arava Valley, a much lower activity (≈40 bat passes) was documented (Hackett et al., 2013). Thus, reducing the current application of pesticides on crops in this area by adopting CBC practices could support and benefit both farmers and local bat populations.
4.1 Broadcast manipulation
The application of broadcast manipulation on bats is known as a bat luring technique that increases capture rates at bat surveys (Hill and Greenaway, 2005; Loeb and Britzke, 2010; Braun De Torrez et al., 2017). In our study, we employed this method as a CBC strategy with the aim of directly enhancing the activity of desert dwelling insectivorous bat species, which prey on a variety of pests in the Arava Valley (Schäckermann et al., 2022). To our knowledge, this is the first time that a broadcasting manipulation (i.e. bat luring) method was tested in the context of pest control services (Tuneu-Corral et al., 2023). We found that the activity level of Eptesicus bottae was reduced in response to the broadcast (Figure 2), while Hypsugo ariel showed no response (Figure 2). The difference in the responses between the two species can be attributed to their different sociality levels and competition tolerance. Eptesicus bottae is known to roost and forge either solitary or in small groups of few individuals (Benda et al., 2006; Shehab et al., 2007; Korine, 2020). Further, studies on species from the same genus have demonstrated a deterring effect when using broadcasted social calls for luring (Quackenbush et al., 2016). Our results, shows a deterring effect at higher conspecifics activity levels (cucurbit fields) and no apparent response at lower activity levels (onion fields), therefore it is likely that E. bottae avoids relatively high bat densities. Alternatively, it is possible that the observed repellent response is due to ambient noise intolerance (von Frenckell and Barclay, 1987). As passing individuals may not perceive the broadcast as calls of a foraging bats but rather as ultra-sonics interfering sounds (i.e. masking) that can deter foraging bats (Masters and Raver, 1996; Gilmour et al., 2021). In contrast to E. bottae response, the activity of H. ariel was not affected by the broadcast, despite exhibiting higher conspecific baseline activity compared to E. bottae. The social structure of H. ariel has not been formally studied, but observations of over 200 individuals emerging together from a roost (Yom-Tov et al., 1992) and their tendency to forage in large groups over water (Kounitsky et al., 2015) suggest that this species, like others in its genus, is social. Socially foraging bats are more susceptible to bat luring (Quackenbush et al., 2016) and more prone to adopt eavesdropping behavior to facilitate foraging (Barclay, 1982), especially when food is scarce (Prat and Yovel, 2020). However, their response to broadcast manipulation have been shown to be influenced by both bat density and prey densities (Hügel et al., 2017; Lewanzik et al., 2019). For example, Pipistrellus spp. was attracted to broadcasted feeding buzzes when baseline conspecific activity was low, but was deterred by broadcasting as conspecific activity increased (Lewanzik et al., 2019). Racey and Swift (1985) observed that P. pipistrellus exhibited a shift in behavior, leaving the area or becoming aggressive towards conspecifics as the availability of prey relative to conspecific density decreases. In our study we did not find strong evidence that H. ariel was attracted to the broadcast. However, the significant interaction observed between plot type and time during the broadcasting phase suggests a change in response (Table 1, Figure 3). Our broadcast rate of activity (bat passes per minute of broadcasting) remained constant during the broadcasting phase. However, bat activity and food availability naturally fluctuate throughout the night (Hayes, 1997; Kuenzi and Morrison, 2003). If H. ariel exhibits a similar behavioral shift in response to conspecific density as described above for P. pipistrellus, then the impact of broadcasting foraging calls may vary depending on the local density of conspecifics. Notably, in our study, we broadcasted a mixed playlist of foraging calls of E. bottae and H. ariel. These two species belong to the same foraging guild (Korine and Pinshow, 2004) and share a similar diet (Feldman et al., 2000). Razgour et al. (2011) showed that interspecific competition among desert dwelling bats over water, in the Negev desert, affects bat community structure as well as foraging time. Therefore, it is impossible to determine whether the observed responses of each species were mainly due to a response to conspecific calls, heterospecific calls or any the combination of these. Interestingly, the activity of E. bottae was significantly lower in the onion fields compared to the cucurbit fields (Figure 2). This may be due to the more homogenized structure of onion plants versus the complex structure of cucurbit plants, which could affect insect diversity. However, further research is needed to understand the drivers underlying this observation.
4.2 Windbreaks gaps
Our results indicate that bats utilize small gaps in windbreaks bordering crop fields for foraging. We recorded higher activity levels and more frequent prey capture attempts (FB) at the windbreak gap compared to continues windbreak sections (Figure 4). These gaps, created by an agricultural road that intersects the windbreaks, seem to enhance bat activity and foraging. Bats typically avoid crossing both major and smaller roads, especially when these roads interrupt commuting routs, such as tree lines (Berthinussen and Altringham, 2012; Bennett and Zurcher, 2013; Medinas et al., 2019). However, our findings align with Foxley et al. (2023) which noted that agricultural roads might positively affect bat activity in agricultural landscape. We propose two explanations for this preference in foraging habitat. Firstly, the gap adds structural complexity to the windbreak, which facilitates the search and capture of potential prey by bats. In addition, the gaps may allow bats to better exploit and instantaneously track pest abundance at the two sides of the windbreak. Secondly, the wind flow patterns around windbreaks may play a role in the flight of the insects. Flying insects, particularly those considered ‘poor flyers,’ are often carried by winds and turbulence created around windbreak (Lewis and Dibley, 1970; Pasek, 1988). Further, gaps in windbreaks can act as wind funnels, concentrating wind flow (Bitog et al., 2012). Consequently, numerous flying insects may accumulate around the gap, potentially leading to higher prey density that bats can exploit. Additionally, the gap in the windbreak may compel insects to fly between tree canopies from one side of the gap to the other, exposing them to an open area where they might be more vulnerable to predation (Schroeder et al., 2009; Morris et al., 2010). It is important to note that we did not directly assess the abundance of flying insects (i.e., potential prey) in this study. Therefore, determining which explanation underlies the favorable foraging behavior at the gap requires further investigation.
4.3 Potential of the two proposed methods as CBC practices
The results from the direct CBC approach (broadcast manipulation) suggest that enhancing bat activity by broadcast manipulation may be feasible in specific scenarios. Our findings highlight the importance of the social behavior of the broadcasted bat species, while the inter-specific and intra-specific interactions may pose challenges to the method’s application for pest suppression. Additionally, echolocation calls are known to attenuate rapidly in the air (Lawrence and Simmons, 1982), making them audible to other bats only within short distances of 35-160 meters (Dechmann et al., 2009; Cvikel et al., 2015). Dechmann et al. (2009) observed a response to broadcast manipulation only within a few meters, whereas Loeb and Britzke (2010) reported higher bat capture rates but no increase in activity due to the broadcast. This also raises concerns regarding the effectiveness of using echolocation call broadcasts as a CBC practice. However, we suggest that a better fine tuning between the rate of the broadcasted echolocation calls with actual on-site bat activity may improve the outcomes. Furthermore, we suggest that adding an insect lurer (e.g. pheromone trap; Korine et al., 2022) at the broadcasting site may facilitate the enhancement of broadcast manipulations. Additionally, we recommend that future field experiments in broadcasting will broadcast the calls of a single species at a time.
The indirect approach (windbreak gap) demonstrated potential for enhancing bat activity and foraging. Our data suggest that small gaps within windbreaks provide a more favorable foraging habitat for bats in semi-arid agriculture environments. Which for some of the threatened desert bat species might be crucially important. These findings align with other studies that found a positive effect of landscape heterogeneity on biodiversity, insect abundance and bat activity (Tuneu-Corral et al., 2023). Further, Foxley et al. (2023) also reported improved bat activity near small roads, especially when at both sides of it there were trees or hedges. Therefore, we suggest that creating more frequent small gaps within windbreaks (which by themselves promotes bat activity) may facilitate foraging activity and improve pest control. Moreover, this method is relatively inexpensive and simple to implement before or after the windbreaks had been established. However, it is important to note that during the experiment, wind speed was relatively low (3.54 ± 0.57 m/s), and stronger winds may lead bats to prefer foraging at windbreak sheltered zone and avoid gaps (Verboom and Huitema, 2010). Our study did not account for crop damage from wind, nor did it consider pest and natural enemies’ abundances and dynamics. Therefore, further research on the long-term cumulative effects of small gaps at windbreaks, along with monitoring their impact on crop yield, bat activity and pest’s abundance is needed. Nevertheless, reducing pesticides use in the Arava area by adopting CBC practices could support and benefit local bat populations and control the pest populations.
Data availability statement
The raw data supporting the conclusions of this article will be made available by the authors, without undue reservation.
Author contributions
AE: Conceptualization, Formal analysis, Methodology, Writing – review & editing, Writing – original draft. IG: Conceptualization, Formal analysis, Methodology, Writing – review & editing. JS: Conceptualization, Methodology, Writing – review & editing. CK: Conceptualization, Formal analysis, Funding acquisition, Methodology, Project administration, Writing – review & editing.
Funding
The author(s) declare financial support was received for the research, authorship, and/or publication of this article. This study was supported by the Israel Ministry of Agriculture and Rural Development, # 16-38-0032 (CK), Israel.
Acknowledgments
We thank the farmers from Kibutz Yotvata, Grofit and Ktura for allowing us to conduct this research in their fields and the team of the Southern Arava R&D for their great hospitality and assistance. This study was supported by the Israel Ministry of Agriculture and Rural Development, # 16-38-0032 (CK), Israel. We thank Svetlana Dobrinin for her useful information regarding pests and pesticide use in the study area. We thank Rabbi Michael Cohen for English editing.
Conflict of interest
The authors declare that the research was conducted in the absence of any commercial or financial relationships that could be construed as a potential conflict of interest.
Publisher’s note
All claims expressed in this article are solely those of the authors and do not necessarily represent those of their affiliated organizations, or those of the publisher, the editors and the reviewers. Any product that may be evaluated in this article, or claim that may be made by its manufacturer, is not guaranteed or endorsed by the publisher.
Supplementary material
The Supplementary Material for this article can be found online at: https://www.frontiersin.org/articles/10.3389/fcosc.2024.1491713/full#supplementary-material
Footnotes
References
Aizpurua O., Budinski I., Georgiakakis P., Gopalakrishnan S., Ibañez C., Mata V., et al. (2018). Agriculture shapes the trophic niche of a bat preying on multiple pest arthropods across Europe: Evidence from DNA metabarcoding. Mol. Ecol. 27, 815–825. doi: 10.1111/mec.14474
Appel G., López-Baucells A., Magnusson W. E., Bobrowiec P. E. D. (2017). Aerial insectivorous bat activity in relation to moonlight intensity. Mamm. Biol. 85, 37–46. doi: 10.1016/j.mambio.2016.11.005
Arnold T. W. (2010). Uninformative parameters and model selection using Akaike’s information criterion. J. Wildl. Manage. 74, 1175–1178. doi: 10.1111/j.1937-2817.2010.tb01236.x
Arzi Y., Segoli M., Schäckermann J., Korine C. (2023). Providing water sources to insectivorous bats for conservation biological control in arid date plantations. Biol. Control 187, 105374. doi: 10.1016/j.biocontrol.2023.105374
Aylen O., Bishop P. J., bin Haji Abd Wahab R., Grafe T. U. (2022). Effectiveness of acoustic lures for increasing tropical forest understory bat captures. Ecol. Evol. 12 (4), e8775. doi: 10.1002/ece3.8775
Barclay R. M. R. (1982). Interindividual use of echolocation calls: Eavesdropping by bats. Behav. Ecol. Sociobiol. 10, 271–275. doi: 10.1007/BF00302816
Benda P., Andreas M., Kock D., Lučan R., Munclinger P., Nová P., et al. (2006). Bats (Mammalia: Chiroptera) of the Eastern Mediterranean. Part 4. Bat fauna of Syria: distribution, systematics, ecology. Acta Societatis Zool. Bohemicae. 70, 1–329.
Bennett V. J., Zurcher A. A. (2013). When corridors collide: Road-related disturbance in commuting bats. J. Wildl. Manage. 77, 93–101. doi: 10.1002/jwmg.467
Berthinussen A., Altringham J. (2012). The effect of a major road on bat activity and diversity. J. Appl. Ecol. 49, 82–89. doi: 10.1111/j.1365-2664.2011.02068.x
Bitog J. P., Lee I.-B., Hwang H.-S., Shin M.-H., Hong S.-W., Seo I.-H., et al. (2012). Numerical simulation study of a tree windbreak. Biosyst. Eng. 111, 40–48. doi: 10.1016/j.biosystemseng.2011.10.006
Boyles J. G., Cryan P. M., McCracken G. F., Kunz T. H. (2011). Economic importance of bats in agriculture. Science 332(6025), 41–42. doi: 10.1126/science.1201366
Brandle J., Finch S. (1991). “How Windbreaks Work,” in Papers in Natural Resources (Lincoln: University of Nebraska). Available at: https://digitalcommons.unl.edu/natrespapers/121.
Brandle J. R., Hodges L., Zhou X. H. (2004). “Windbreaks in North American agricultural systems,” in New Vistas in Agroforestry: A Compendium for 1st World Congress of Agroforestry. Eds. Nair P. K. R., Rao M. R., Buck L. E. (Springer Netherlands, Dordrecht), 65–78. doi: 10.1007/978-94-017-2424-1_5
Brandle J. R., Johnson B. B., Akeson T. (1992). Field windbreaks: are they economical? J. Product. Agric. 5, 393–398. doi: 10.2134/jpa1992.0393
Braun De Torrez E. C., Samoray S. T., Silas K. A., Wallrichs M. A., Gumbert M. W., Ober H. K., et al. (2017). Acoustic lure allows for capture of a high-flying, endangered bat. Wildl. Soc. Bull. 41, 322–328. doi: 10.1002/wsb.778
Chown S. L., Terblanche J. S. (2006). Physiological diversity in insects: ecological and evolutionary contexts. Adv. Insect Physiol. 33, 50–152. doi: 10.1016/S0065-2806(06)33002-0
Cleugh H. A. (1998). Effects of windbreaks on airflow, microclimates and crop yields. Agroforestry Syst. 41, 55–84. doi: 10.1023/A:1006019805109
Cleveland C. J., Betke M., Federico P., Frank J. D., Hallam T. G., Horn J., et al. (2006). Economic value of the pest control service provided by Brazilian free-tailed bats in south-central Texas. Front. Ecol. Environ. 4, 238–243. doi: 10.1890/1540-9295(2006)004[0238:EVOTPC]2.0.CO;2
Cohen Y., Bar-David S., Nielsen M., Bohmann K., Korine C. (2020). An appetite for pests: Synanthropic insectivorous bats exploit cotton pest irruptions and consume various deleterious arthropods. Mol. Ecol. 29, 1185–1198. doi: 10.1111/mec.15393
Cvikel N., Egert Berg K., Levin E., Hurme E., Borissov I., Boonman A., et al. (2015). Bats aggregate to improve prey search but might be impaired when their density becomes too high. Curr. Biol. 25, 206–211. doi: 10.1016/j.cub.2014.11.010
Dechmann D. K. N., Heucke S. L., Giuggioli L., Safi K., Voigt C. C., Wikelski M. (2009). Experimental evidence for group hunting via eavesdropping in echolocating bats. Proc. R. Soc. B: Biol. Sci. 276, 2721–2728. doi: 10.1098/rspb.2009.0473
Dix M. E., Johnson R. J., Harrell M. O., Case R. M., Wright R. J., Hodges L., et al. (1995). Influences of trees on abundance of natural enemies of insect pests: a review. Agroforest Syst. 29, 303–311. doi: 10.1007/BF00704876
Dix M. E., Leatherman D. (1988). Insect management in windbreaks. Agricult. Ecosyst. Environ. 22–23, 513–537. doi: 10.1016/0167-8809(88)90043-6
Dobrinin S. (2020). Pest control recommendation for melons and onions. In Hebrew, Israel Ministry of Agriculture and Rural Development
Downs N. C., Racey P. A. (2006). The use by bats of habitat features in mixed farmland in Scotland. Acta Chiropterol. 8, 169–185. doi: 10.3161/150811006777070893
Feldman R., Whitaker J. O., Yom-Tov Y. (2000). Dietary composition and habitat use in a desert insectivorous bat community in Israel. Acta Chiropterol. 2 (1), 15–22. Available at: https://www.infona.pl//resource/bwmeta1.element.agro-dfe2aeaf-b164-429a-a1f0-202b37fb1c9a.
Fenton M. B. (2003). Eavesdropping on the echolocation and social calls of bats. Mammal Rev. 33, 193–204. doi: 10.1046/j.1365-2907.2003.00019.x
Fernández-Cirelli A., Arumí J. L., Rivera D., Boochs P. W. (2009). Environmental effects of irrigation in arid and semi-arid regions. Chil. J. Agr. Res. 69, 27–40. doi: 10.4067/S0718-58392009000500004
Foxley T., Lintott P., Stone E. (2023). What drives bat activity at field boundaries? J. Environ. Manage. 329, 117029. doi: 10.1016/j.jenvman.2022.117029
Froidevaux J. S. P., Boughey K. L., Hawkins C. L., Broyles M., Jones G. (2019). Managing hedgerows for nocturnal wildlife: Do bats and their insect prey benefit from targeted agri-environment schemes? J. Appl. Ecol. 56, 1610–1623. doi: 10.1111/1365-2664.13412
Gillam E. H. (2007). Eavesdropping by bats on the feeding buzzes of conspecifics. Candian J. Zool. 85, 795–801. doi: 10.1139/Z07-060
Gilmour L. R. V., Holderied M. W., Pickering S. P. C., Jones G. (2021). Acoustic deterrents influence foraging activity, flight and echolocation behaviour of free-flying bats. J. Exp. Biol. 224, jeb242715. doi: 10.1242/jeb.242715
Goldreich Y., Karni O. (2001). Climate and precipitation regime in the Arava Valley, Israel. Israel J. Earth Sci. 50, 53–59. doi: 10.1092/1V61-FPGF-Y5VK-ADAG
Griffin D. R., Webster F. A., Michael C. R. (1960). The echolocation of flying insects by bats. Anim. Behav. 8, 141–154. doi: 10.1016/0003-3472(60)90022-1
Hackett T. D., Holderied M. W., Korine C. (2017). Echolocation call description of 15 species of Middle-Eastern desert dwelling insectivorous bats. Bioacoustics 26, 217–235. doi: 10.1080/09524622.2016.1247386
Hackett T. D., Korine C., Holderied M. W. (2013). The importance of Acacia trees for insectivorous bats and arthropods in the Arava desert. PloS One 8, e52999. doi: 10.1371/journal.pone.0052999
Harms K., Omondi E., Mukherjee A. (2020). Investigating bat activity in various agricultural landscapes in Northeastern United States. Sustainability 12, 1959. doi: 10.3390/su12051959
Hayes J. P. (1997). Temporal variation in activity of bats and the design of echolocation-monitoring studies. J. Mammal. 78, 514–524. doi: 10.2307/1382902
Heim O., Lenski J., Schulze J., Jung K., Kramer-SChadt S., Eccard J. A., et al. (2018). The relevance of vegetation structures and small water bodies for bats foraging above farmland. Basic Appl. Ecol. 27, 9–19. doi: 10.1016/j.baae.2017.12.001
Hill D. A., Greenaway F. (2005). Effectiveness of an acoustic lure for surveying bats in British woodlands. Mammal Rev. 35, 116–122. doi: 10.1111/j.1365-2907.2005.00058.x
Hügel T., van Meir V., Muñoz-Meneses A., Clarin B.-M., Siemers B. M., Goerlitz H. R. (2017). Does similarity in call structure or foraging ecology explain interspecific information transfer in wild Myotis bats? Behav. Ecol. Sociobiol. 71, 168. doi: 10.1007/s00265-017-2398-x
Kalko E. K. V. (1995). Insect pursuit, prey capture and echolocation in pipestirelle bats (Microchiroptera). Anim. Behav. 50, 861–880. doi: 10.1016/0003-3472(95)80090-5
Kazial K. A., Kenny T. L., Burnett S. C. (2008). Little brown bats (Myotis lucifugus) recognize individual identity of conspecifics using sonar calls. Ethology 114, 469–478. doi: 10.1111/j.1439-0310.2008.01483.x
Korine C. (2020). “Botta’s Serotine Eptesicus bottae (Peters 1869),” in Handbook of the Mammals of Europe, vol. 1–9 . Eds. Hackländer K., Zachos F. E. (Springer International Publishing, Cham). doi: 10.1007/978-3-319-65038-8_46-1
Korine C., Cohen Y., Kahnonitch I. (2022). Insect pest pheromone lures may enhance the activity of insectivorous bats in mediterranean vineyards and apple orchards. Sustainability 14, 16566. doi: 10.3390/su142416566
Korine C., Pinshow B. (2004). Guild structure, foraging space use, and distribution in a community of insectivorous bats in the Negev Desert. J. Zool. 262, 187–196. doi: 10.1017/S0952836903004539
Kounitsky P., Rydell J., Amichai E., Boonman A., Eitan O., Weiss A. J., et al. (2015). Bats adjust their mouth gape to zoom their biosonar field of view. Proceed. Natl. Acad. Sci. U.S.A. 112, 6724–6729. doi: 10.1073/pnas.1422843112
Kuenzi A. J., Morrison M. L. (2003). Temporal patterns of bat activity in Southern Arizona. J. Wildl. Manage. 67, 52–64. doi: 10.2307/3803061
Kunz T. H. (1973). Resource utilization: temporal and spatial components of bat activity in central Iowa. J. Mammal. 54 (1), 14–32. doi: 10.2307/1378869
Kunz T. H., Braun de Torrez E., Bauer D., Lobova T., Fleming T. H. (2011). Ecosystem services provided by bats. Ann. New York Acad. Sci. 1223, 1–38. doi: 10.1111/j.1749-6632.2011.06004.x
Lawrence B. D., Simmons J. A. (1982). Measurements of atmospheric attenuation at ultrasonic frequencies and the significance for echolocation by bats. J. Acoustic. Soc. America 71, 585–590. doi: 10.1121/1.387529
Lewanzik D., Sundaramurthy A. K., Goerlitz H. R. (2019). Insectivorous bats integrate social information about species identity, conspecific activity and prey abundance to estimate cost–benefit ratio of interactions. J. Anim. Ecol. 88, 1462–1473. doi: 10.1111/1365-2656.12989
Lewis T., Dibley G. C. (1970). Air movement near windbreaks and a hypothesis of the mechanism of the accumulation of airborne insects. Ann. Appl. Biol. 66, 477–484. doi: 10.1111/j.1744-7348.1970.tb04627.x
Lindell C., Eaton R. A., Howard P. H., Roels S. M., Shave M. E. (2018). Enhancing agricultural landscapes to increase crop pest reduction by vertebrates. Agricult. Ecosyst. Environ. 257, 1–11. doi: 10.1016/j.agee.2018.01.028
Loeb S. C., Britzke E. R. (2010). Intra- and interspecific responses to Rafinesque’s big-eared bat (Corynorhinus rafinesquii) social calls. Acta Chiropterol. 12, 329–336. doi: 10.3161/150811010X537909
Masters W. M., Raver K. A. (1996). The degradation of distance discrimination in big brown bats (Eptesicus fuscus) caused by different interference signals. J. Comp. Physiol. A 179, 703–713. doi: 10.1007/BF00216134
Medinas D., Ribeiro V., Marques J. T., Silva B., Barbosa A. M., Rebelo H., et al. (2019). Road effects on bat activity depend on surrounding habitat type. Sci. Total Environ. 660, 340–347. doi: 10.1016/j.scitotenv.2019.01.032
Morris A. D., Miller D. A., Kalcounis-Rueppell M. C. (2010). Use of forest edges by bats in a managed pine forest landscape. J. Wildl. Manage. 74, 26–34. doi: 10.2193/2008-471
Noy-Meir I. (1974). Desert ecosystems: higher trophic levels. Annu. Rev. Ecol. System. 5, 195–214. doi: 10.1146/annurev.es.05.110174.001211
Nuberg I. K. (1998). Effect of shelter on temperate crops: a review to define research for Australian conditions. Agroforestry Syst. 41, 3–34. doi: 10.1023/A:1006071821948
Olimpi E. M., Philpott S. M. (2018). Agroecological farming practices promote bats. Agricult. Ecosyst. Environ. 265, 282–291. doi: 10.1016/j.agee.2018.06.008
Pasek J. E. (1988). Influence of wind and windbreaks on local dispersal of insects. Agricult. Ecosyst. Environ. 22–23, 539–554. doi: 10.1016/0167-8809(88)90044-8
Prat Y., Yovel Y. (2020). Decision making in foraging bats. Curr. Opin. Neurobiol. 60, 169–175. doi: 10.1016/j.conb.2019.12.006
Puig-Montserrat X., Torre I., López-Baucells A., Guerrieri E., Monti M. M., Ràfols-García R., et al. (2015). Pest control service provided by bats in Mediterranean rice paddies: linking agroecosystems structure to ecological functions. Mamm. Biol. 80, 237–245. doi: 10.1016/j.mambio.2015.03.008
Quackenbush H., D’Acunto L. E., Flaherty E. A., Zollner P. A. (2016). Testing the efficacy of an acoustic lure on bat mist-netting success in North American central hardwood forests. J. Mammal. 97, 1617–1622. doi: 10.1093/jmammal/gyw125
Racey P. A., Swift S. M. (1985). Feeding ecology of Pipistrellus pipistrellus (Chiroptera: Vespertilionidae) during pregnancy and lactation. I. Foraging behaviour. J. Anim. Ecol. 54, 205–215. doi: 10.2307/4631
Razgour O., Korine C., Saltz D. (2011). Does interspecific competition drive patterns of habitat use in desert bat communities? Oecologia 167, 493–502. doi: 10.1007/s00442-011-1995-z
Reganold J. P., Papendick R. I., Parr J. F. (1990). Sustainable agriculture. Sci. Am. 262, 112–121. doi: 10.1038/scientificamerican0690-112
Rodríguez-San Pedro A., Allendes J. L., Beltrán C. A., Chaperon P. N., Saldarriaga-Córdoba M. M., Silva A. X., et al. (2020). Quantifying ecological and economic value of pest control services provided by bats in a vineyard landscape of central Chile. Agricult. Ecosyst. Environ. 302, 107063. doi: 10.1016/j.agee.2020.107063
Rodríguez-San Pedro A., Chaperon P. N., Beltrán C. A., Allendes J. L., Ávila F. I., Grez A. A. (2018). Influence of agricultural management on bat activity and species richness in vineyards of central Chile. J. Mammal. 99, 1495–1502. doi: 10.1093/jmammal/gyy121
Russo D., Bosso L., Ancillotto L. (2018). Novel perspectives on bat insectivory highlight the value of this ecosystem service in farmland: Research frontiers and management implications. Agricult. Ecosyst. Environ. 266, 31–38. doi: 10.1016/j.agee.2018.07.024
Schäckermann J., Morris E. J., Alberdi A., Razgour O., Korine C. (2022). The contribution of Desert-dwelling bats to pest control in hyper-arid date agriculture. Diversity 14, 1034. doi: 10.3390/d14121034
Schroeder B., Buddle C. M., Saint-Germain M. (2009). Activity of flying beetles (Coleoptera) at two heights in canopy gaps and intact forests in a hardwood forest in Quebec. Can. Entomol. 141, 515–520. doi: 10.4039/n09-022
Shehab A., Karataş A., Amr Z., Mamkhair I., Sözen M. (2007). The distribution of bats (Mammalia: chiroptera) in Syria. Vertebr. Zool. 57, 103–132. doi: 10.3897/vz.57.e30888
Shields M. W., Johnson A. C., Pandey S., Cullen R., González- Chang M., Wratten S. D., et al. (2019). History, current situation and challenges for conservation biological control. Biol. Control 131, 25–35. doi: 10.1016/j.biocontrol.2018.12.010
Simmons N. B., Cirranello A. L. (2024). Bat species of the world: a taxonomic and geographic database (1.5). Available online at: https://batnames.org.
Song S., Lin A., Jiang T., Zhao X., Metzner W., Feng J. (2019). Bats adjust temporal parameters of echolocation pulses but not those of communication calls in response to traffic noise. Integr. Zool. 14, 576–588. doi: 10.1111/1749-4877.12387
Taylor P. J., Grass I., Alberts A. J., Joubert E., Tscharntke T. (2018). Economic value of bat predation services – A review and new estimates from macadamia orchards. Ecosyst. Serv. 30, 372–381. doi: 10.1016/j.ecoser.2017.11.015
Tuneu-Corral C., Puig-Montserrat X., Riba-Bertolín D., Russo D., Rebelo H., Cabeza M., et al. (2023). Pest suppression by bats and management strategies to favour it: a global review. Biol. Rev. 98, 1564–1582. doi: 10.1111/brv.12967
Übernickel K., Tschapka M., Kalko E. K. V. (2013). Selective eavesdropping behaviour in three Neotropical bat species. Ethology 119, 66–76. doi: 10.1111/eth.12038
Velten S., Leventon J., Jager N., Newig J. (2015). What is sustainable agriculture? A systematic review. Sustainability 7, 7833–7865. doi: 10.3390/su7067833
Verboom B., Huitema H. H. (2010). The influence of treeline structure and wind protection on commuting and foraging common pipistrelles (Pipistrellus pipistrellus). Lutra 53, 63–80. doi: 10.1007/BF02698211
Verboom B., Spoelstra K. (1999). Effects of food abundance and wind on the use of tree lines by an insectivorous bat, Pipistrellus pipistrellus. Can. J. Zool. 77, 9. doi: 10.1139/cjz-77-9-1393
von Frenckell B., Barclay R. M. R. (1987). Bat activity over calm and turbulent water. Canadian J. Zool. 65, 219–222. doi: 10.1139/z87-035
Wickramasinghe L. P., Harris S., Jones G., Vaughan N. (2003). Bat activity and species richness on organic and conventional farms: impact of agricultural intensification. J. Appl. Ecol. 40, 984–993. doi: 10.1111/j.1365-2664.2003.00856.x
Wolcott K. A., Vulinec K. (2012). Bat activity at woodland/farmland interfaces in Central Delaware. Northeastern Nat. 19, 87–98. doi: 10.1656/045.019.0107
Yom-Tov Y., Yom-Tov Y., Makin D., Shalmon B. (1992). The biology of Pipistrellus bodenheimeri (Microchiroptera) in the Dead Sea area of Israel. Z. für Säugetierkunde : im Auftrage der Deutschen Gesellschaft für Säugetierkunde e.V 57, 65–69.
Keywords: insectivorous bats, bat lure, agricultural fields, pest control, sustainable agriculture, hyper-arid, ecosystem services
Citation: Einav A, Giladi I, Schäckermann J and Korine C (2024) Enhancing insectivorous bat activity through sound manipulation and assessing the contribution of small gaps in windbreaks in the hyper-arid Arava desert. Front. Conserv. Sci. 5:1491713. doi: 10.3389/fcosc.2024.1491713
Received: 05 September 2024; Accepted: 11 November 2024;
Published: 11 December 2024.
Edited by:
Sara Mae Kross, University of Canterbury, New ZealandReviewed by:
Luis P. Da Silva, Centro de Investigacao em Biodiversidade e Recursos Geneticos (CIBIO-InBIO), PortugalStevie Florent, University of Canterbury, New Zealand
Copyright © 2024 Einav, Giladi, Schäckermann and Korine. This is an open-access article distributed under the terms of the Creative Commons Attribution License (CC BY). The use, distribution or reproduction in other forums is permitted, provided the original author(s) and the copyright owner(s) are credited and that the original publication in this journal is cited, in accordance with accepted academic practice. No use, distribution or reproduction is permitted which does not comply with these terms.
*Correspondence: Itamar Giladi, aXR1c2hnaUBiZ3UuYWMuaWw=