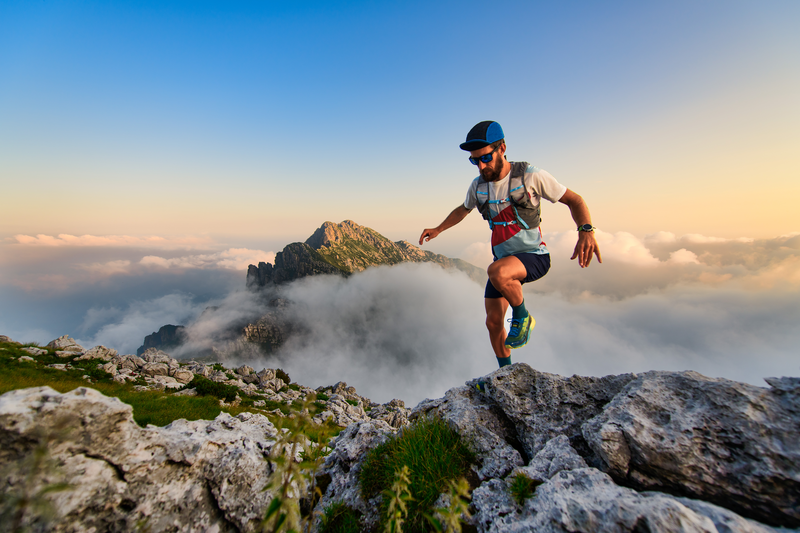
95% of researchers rate our articles as excellent or good
Learn more about the work of our research integrity team to safeguard the quality of each article we publish.
Find out more
ORIGINAL RESEARCH article
Front. Conserv. Sci. , 29 October 2024
Sec. Animal Conservation
Volume 5 - 2024 | https://doi.org/10.3389/fcosc.2024.1462664
This article is part of the Research Topic Understanding Rest-Activity Rhythms: Uncovering Factors Shaping Behavioural Rhythms in Diverse Ecological Contexts. View all 5 articles
Understanding the daily activity patterns and social dynamics of giraffes (Giraffa camelopardalis) is crucial for their management and conservation. In this study, a comprehensive 24-hour observational analysis of three female reticulated giraffes kept for three weeks at the Opel-Zoo in Kronberg, Germany, was conducted. Using infrared-sensitive cameras, the study captured behavioral data across baseline and two intervention phases involving changes in group composition. Social network analysis was performed using the Mantel test to assess changes in social interactions between day and night and across different study periods, while the MRQAP was applied to evaluate the influence of individual subtypes on the social structure. During the day, the giraffes exhibited a high level of activity, primarily engaging in walking, standing, and feeding behaviors. Diurnal resting was minimal, with sporadic lying phases. Night-time behavior was markedly different, with the giraffes spending most of the night lying down, interspersed with periods of feeding. The presence of a male giraffe during one intervention phase significantly altered diurnal activity patterns, increasing standing behaviors and decreasing feeding time. Social interactions, including nearest neighbor preferences, varied across day and night periods and changed with alterations in group composition. The study highlights the complexity of giraffe social dynamics and their adaptation to different social contexts. These findings provide valuable insights into the natural behavioral rhythms and social behaviors of giraffes, which are essential for effective zoo management and conservation strategies.
Understanding the intricate interplay between an animal’s internal rhythms and external environmental cues is fundamental to comprehending its daily life. Circadian rhythms, which are controlled by internal clocks, govern an animal’s activity and rest phases, which are significantly influenced by sunlight (Mistlberger and Rusak, 2005; Partch et al., 2014). Both the activity and rest phases fulfill vital functions for the physiological balance of an animal (Berger, 2011; Merrow et al., 2005). The temporal distribution of activity and rest varies across species, with classifications such as diurnal, nocturnal, cathemeral, or crepuscular, depending on peak activity times within the day (Bennie et al., 2014; Wu et al., 2018). Behavioral patterns in large herbivores are known to vary greatly between day and night. Most ungulates display diurnal to crepuscular cycles, being most active during the day (Bennie et al., 2014; Wu et al., 2018). While there is extensive research on the diurnal behaviors of many ungulate species, nocturnal behaviors remain less understood, especially in wild settings (Berger, 2011; Rattenborg et al., 2017). However, a recent study on captive ungulates revealed interesting insights into their nocturnal behavior and sleep rhythms (Gübert et al., 2023a). In particular, the study found differences between individuals of different ages, but no differences in terms of sex. For most species, there was a significant increase in the proportion of resting periods during the night.
Since a wide variety of factors influence animal behavior both during the day and at night (Brando and Buchanan-Smith, 2018), observations should ideally be conducted over a 24-hour period to create the most comprehensive behavioral profile possible. Understanding behavior in zoo settings over this full period remains a challenge despite its importance for animal management and welfare (Brando and Buchanan-Smith, 2018), necessitating a holistic approach to behavioral research (Favreau et al., 2009; Price and Stoinski, 2007). Behavioral observation provides insights into zoo animals’ time allocation and aids in management decisions and welfare assessments (Hill and Broom, 2009; Hutchins et al., 2019; Watters et al., 2009). In particular, analyzing activity budgets provides valuable information about an animal’s well-being and its response to internal and external stimuli (Rose, 2023). Among other factors, age plays a significant role in shaping an animal’s behavior, with activity levels often increasing and rest time decreasing with age (Cajochen et al., 2006; Lesku et al., 2008). In addition to external factors such as light, feeding routines, and enclosure conditions, the social context in which an animal lives may also influence its behavior patterns. With regard to mating tactics, for example, this has been demonstrated for over two hundred species of ungulates (Bowyer et al., 2020; Szemán et al., 2021).
The social behavior of giraffes, one of Africa’s most iconic ungulates, has also currently being studied intensively at the zoo and in the wild. For a long time, giraffes were considered to occasionally come together in loose fission-fusion groups but do not develop social preferences or even closer social bonds with conspecifics (Muller et al., 2018b). However, recent studies show that female giraffes in particular do exhibit social preferences and dominance hierarchy (Carter et al., 2013a, b; Horová et al., 2015). In the wild, sex and age as well as spatial overlap, kinship and context may be drivers for fission-fusion dynamics and social preferences (Carter et al., 2013a, b; Muller et al., 2018b). Moreover, Van der Waal et al. (2014) found a multilevel social organization wherein individuals belonged to social cliques. Underpinning these results, Bond et al. (2021) confirmed female giraffes to form stable long-term associations. In captive settings, female giraffes were also observed to show social preferences and maintaining relationships (Bashaw et al., 2007; Grasso et al., 2022; Lewton and Rose, 2019) as well as to respond to social separation with a higher activity level during the day (Tarou et al., 2000). Furthermore, the results of Lewton and Rose (2019) show that social bonds can change over longer periods of time and that giraffes are therefore flexible in their choice of social partners in zoo-managed herds.
Understanding how giraffes allocate their time during the day and the night and identifying factors influencing their behavior is crucial for their conservation and management, especially considering their vulnerable status (Muller et al., 2018a). Despite the numerous studies on daily activity patterns, there is little research on behavior over 24 hours, especially in large herbivores such as giraffes (Baxter and Plowman, 2001; Tobler and Schwierin, 1996). In this study, activity budgets were recorded over 24-hour periods of three female reticulated giraffes (Giraffa camelopardalis reticulata), with three different group compositions evaluated during the study period: (1) three female reticulated giraffes and additionally two female Rothschild’s giraffes (Giraffa camelopardalis camelopardalis), (2) three female reticulated giraffes, and (3) three female and one male reticulated giraffe. The analysis focused on changes in activity patterns of the three female reticulated giraffes during the day and at night as well as on the social relationships and preferences of the animals in the individual phases in order to determine the influence of changing group composition on these parameters. We hypothesize that the activity budget of the three female reticulated giraffes does not change significantly due to the changing group composition. In the case of social contacts, a closer bond between reticulated giraffes and Rothschild’s giraffes is hypothesized and in the presence of the reticulated giraffe bull, changes in social bonds should be altered by interactions originating from the bull.
Data were collected from three reticulated giraffes housed at Opel-Zoo Kronberg, Germany. Video recordings were conducted over 22 consecutive days and nights from 13.03.2023 to 03.04.2023, spanning 24 hours. Daytime was defined from 07:00 to 17:00 while night spanned from 17:00 to 7:00 in order to be able to compare the nocturnal behavior results with those of recent studies (Burger et al., 2021; Gübert et al., 2023b).
During the study period, the group composition of the three reticulated giraffes changed twice. For analysis purposes, the study period was divided into three phases: Baseline, Intervention I1, and Intervention I2. The baseline (B) lasted from 13.03. to 19.03.2023. During this period, five female giraffes of two subspecies were kept at Opel Zoo: the three study animals (Reticulated giraffes F1, F2, F3) and two Rothschild giraffes (F4, F5). The first intervention (I1) took place from 20.03. - 27.03.2023, during which the two Rothschild giraffes left the group at Opel-Zoo and were transported to another zoo. Intervention 2 (I2) lasted 6 days and nights, starting on 28.03.2023 when a reticulated giraffe bull (M1) joined the group of the three reticulated giraffe females. During this period the bull was separated from the females at night. In total, 528 h of data were collected for the three reticulated females F1, F2 and F3.
Infrared-sensitive cameras (Mobotix AllRound Dual M15) covering the entire indoor enclosure were used to accurately document the behavioral patterns. The recording rate was 1 frame per second. The image quality of the night recordings was limited, so that the analysis was restricted to clearly recognizable behaviors (see ethogram in the Supplementary Materials). Since cameras could have been only installed at the giraffe’s indoor enclosure, behavioral data recording was only possible when the animals were inside.
For video analysis BORIS 2.1.5 software was used (Friard and Gamba, 2016). This software enabled continuous recording of the behavior of each individual. Continuous recording allows the exact frequency and duration of behavioral events to be determined with the recorded start and end time stamps. As sampling rule focal sampling was used (Martin and Bateson, 2007).
With focal sampling, one individual is observed for a certain period of time and all occurring behaviors are recorded. Focal sampling allows behavioral patterns to be recorded in a more detailed way than with temporally structured data recordings.
The behavioral patterns were categorized based on whether the animal exhibited active or resting behavior, following validated ethograms (Seeber et al., 2012; Burger et al., 2020b). The observed behaviors were classified into five main states: feeding, walking, standing, lying, and REM sleep (Table 1). Behaviors performed while the animal was upright on all four legs, such as feeding, walking, or standing, were classified as standing activities. When the animal was resting on the ground, it was categorized as lying down or REM (rapid eye movement) sleep posture. Feeding giraffes were observed standing while browsing, ingesting concentrates, or drinking. Standing behavior was recorded when the giraffe stood on all four legs without forward movement, contrasting with walking behavior, observed when the giraffe moved in one direction. Rumination could occur while the giraffe was walking, standing, or lying down; however, continuous observation of rumination was hindered by the video quality. Stereotypical behaviors were not included in the analyses due to similar observational limitations. A lying giraffe was observed sitting on the ground with the abdomen or flank folded under and slightly displaced to the side, with the neck and head erect or slightly bent. REM sleep posture was identified when the giraffe lay on the ground, bent its neck backward, and rested its head on the flank or ground. If an individual is outside the field of vision for more than 5 seconds and cannot be assigned to other activities, it is labelled as ‘out’. This can be the case when the giraffes are in the outdoor enclosure, because no usable videos can be recorded there.
In addition, nearest neighbor (NN) and social partner interactions were analyzed. NN was defined as the individual that was within a maximum distance of two body lengths from the observed animal for more than five seconds (Muller et al., 2018b). If two individuals were at the same distance from the observed animal, both were considered NNs. If there are two animals in this area, the individual that is closer to the observed animal is referred to as NN. The individual that engaged in a social interaction with the observed animal was referred to as the social partner.
To assess whether the social network of the giraffes differed between daytime and nighttime across the three study periods, the Mantel test was employed. This test measures the correlation between two distance matrices, comparing the social association matrix of interactions observed during the day to the matrix of interactions observed at night. Interaction data were tabulated in association matrices where the rows and columns represented individuals and the cells contained the observed frequency of interactions in percentage. The Mantel statistic was calculated using Pearson’s product-moment correlation, and permutation testing with 999 randomizations was performed to assess the significance of the observed correlations. Separate Mantel tests were conducted for each pairwise comparison between the three study periods, allowing for the analysis of changes in social bonds across different periods and times of the day.
To evaluate whether subspecies played a significant role in shaping the social network structure, a Multiple Regression Quadratic Assignment Procedure (MRQAP) was employed. This method tests the relationship between a dependent matrix (social association matrix) and one or more independent matrices (predictor matrices). For this study, an association matrix representing the frequency of social interactions between giraffes was regressed against a subspecies similarity matrix, which coded pairwise relationships as 1 if two individuals belonged to the same subspecies and 0 otherwise. The MRQAP was performed using 1000 permutations to assess statistical significance. The MRQAP approach was chosen because it accounts for the inherent non-independence of network data, where each individual’s interaction is likely influenced by the interactions of others in the group.
All analyses were conducted in the R statistical environment (RStudio 2023.12.0). The Mantel test was performed using the “vegan” package, and MRQAP was implemented using the “sna” package. The significance level for all tests was set at p < 0.05 (*), p < 0.005 (**) and p < 0.001 (***).
This study analyzed the 24-hour activity budgets of three female reticulated giraffes before and during changes in group composition. Giraffes exhibited distinct diurnal and nocturnal activity levels regardless of the observation period (Figure 1). During the day, the giraffes were primarily active, with walking (8.52 ± 0.57%), standing (36.5 ± 2.16%), and feeding (30.17 ± 2.1%) being the most common behaviors (Figure 2). Diurnal lying events were rare in F1 (1.46 ± 0.87%) and F3 (2.1 ± 0.83%), while F2 regularly exhibited one to two lying phases per day (6.41 ± 1.62%). At night, activity levels decreased (from 75.19 ± 8.47% to 49.34 ± 7.23%). With the onset of darkness, all individuals began to rest. Giraffes spent most of the night lying down (48.87 ± 0.98%), beginning between 19:00-20:00 and ending in the early morning between 6:00-7:00. REM sleep behavior (1.37 ± 0.13%) only occurred during lying bouts and was of very short duration. However, lying behavior was frequently interrupted by active behaviors, especially feeding (28.43 ± 1.17%) and standing (17.47 ± 0.95%). Overall, the giraffes demonstrated a specific day-night activity rhythm, with daytime behavior being primarily active and nighttime behavior characterized by lying and short sleep events.
Figure 1. 24-hour rhythms of three female reticulated giraffes. Shown are active behaviors including standing, feeding and locomotion (blue) as well as lying with head upright (dark green) and REM sleep posture (light green). Seven days are shown for each individual (F1-3) to visualize the low variability within the three study periods (B = baseline; I1 = intervention 1; I2 = intervention 2). The gaps (without color code) correspond to the periods in which the animals are out of sight.
Figure 2. Activity budget of the three female reticulated giraffes (F1-3) for day and night. Shown are active behaviors including standing (dark blue), feeding (blue) and locomotion (light blue) as well as lying with head upright (dark green), REM sleep posture (light green) and out of sight (light grey). The activity budgets in the three study periods (B = baseline; I1 = intervention 1; I2 = intervention 2) are shown for each individual.
For each individual the activity budgets remained relatively constant at night despite changes in group composition (Figure 2; Supplementary Table S1). However, clear changes in feeding and standing behavior were observed during the day. In the baseline period (B), the three giraffes together spent 28.79 ± 3.12% of the day standing and 41.32 ± 4.44% feeding. During the first intervention (I1), standing increased to 32.45 ± 3.47% and feeding decreased to 25.07 ± 2.86%. After the bull joined the female group (I2), standing behavior increased further (48.83 ± 3.29%) and feeding decreased (24.85 ± 2.33%). At night, giraffes spent 16.82 ± 1.69% standing and 29.24 ± 1.99% feeding during baseline. During I1, standing increased to 19.48 ± 1.76% and feeding decreased to 26.94 ± 1.84% During I2, standing was 15.81 ± 1.39% and feeding was 29.34 ± 2.33%. The night values for standing and feeding did not differ distinctly across the different study periods.
A series of Mantel tests were performed to evaluate the correlation between giraffe social networks during the day and night (Table 2), as well as across different study periods (B, I1 and I2) (Table 3).
The Mantel test comparing the baseline social network structure between day and night yielded a Mantel statistic r=0.2427 (p = 0.5), suggesting a weak positive correlation with no significant difference between day and night. For I1, the Mantel r=0.918 (p = 0.333), indicating a stronger correlation between day and night, although this result was not statistically significant. However, the second intervention (I2) produced a Mantel statistic r=−0.9994 (p = 1), suggesting a strong negative correlation between the day and night social networks, but again, without significance (Table 2).
Comparing the social network structures across time points during the day, the Mantel test between the baseline and I1 yielded r=0.366 (p = 0.5), while the baseline and I2 comparison resulted in r=0.3892 (p = 0.6667). Both results show weak to moderate correlations between the time points but are statistically insignificant (Table 3). The comparison between I1 and I2 produced a Mantel r=−0.7148 (p = 1), indicating a negative correlation, though not statistically significant.
Overall, the results suggest that the social structure of giraffes exhibits variations over time and between day and night across different conditions. However, these differences are not statistically significant, implying that the changes in group composition and time of day do not strongly influence the giraffes’ social network patterns in a consistent or predictable way.
MRQAP) was conducted to assess the influence of subspecies on the giraffes’ social network structure during both day and night observations of the Base line (B). The model included subspecies as a predictor variable and was tested against the association matrices representing the social relationships between individuals (Table 4).
During the day, the regression model revealed a coefficient estimate for the intercept of 4.67 (p = 0.170) and a coefficient estimate for subspecies of 11.85 (p = 0.087). Although the results indicated a substantial positive effect of subspecies on the social bond strength, this effect was not statistically significant. However, the overall model fit was strong, with an F-statistic of 70.5 on 1 and 18 degrees of freedom and a highly significant p-value of 1.223e-07. The model explained 79.66% of the variance (R² = 0.7966), indicating a substantial influence of subspecies on social structure during the day.
During the night, the model showed an intercept estimate of 15.09 (p = 0.105) and a subspecies estimate of 6.24 (p = 0.150). Similar to the day, the model suggested a positive relationship between subspecies and social bonds, but the effect did not reach statistical significance. The F-statistic was 3.542 on 1 and 18 degrees of freedom, with a p-value of 0.076. The R² value of 0.1644 indicated that subspecies explained approximately 16.44% of the variance in the social structure during the night.
While subspecies showed a stronger effect on social bonds during the day compared to the night, neither model reached statistical significance in predicting the role of subspecies on social structure. However, the day model explained a notably higher proportion of variance in the giraffes’ social relationships, suggesting that subspecies might play a more prominent role in daytime social dynamics. Nonetheless, further studies with additional data may be needed to confirm the role of subspecies on the giraffes’ social networks across different times of day.
To analyze whether social preferences remained constant over time and with changing group compositions, the nearest neighbor (NN) and social partner were recorded for each female (F1, F2, F3) over 22 consecutive days (Figure 3; Table 5). During the day, when the two Rothschild giraffes (F4, F5) were part of the group (B), the reticulated females spent more time close to each other than to the Rothschild giraffes, with minimal interaction between the species. All reticulated females had low NN time and nearly no social interaction with F4 and F5. At night, NN compositions and interaction rates among the reticulated giraffes were similar, but they spent more time as NN of the Rothschild females compared to the day, with still minimal social interaction.
Figure 3. Context-dependent networks of social affiliation in the giraffes studied. Each network shows social preferences between individuals in the three study periods (B = baseline; I1 = intervention 1; I2 = intervention 2) during the day (left column) and at night (right column). F1-F3: female reticulated giraffes; M1: male reticulated giraffes; F4 and F5: female Rothschild’s giraffes. The line thickness symbolizes the strength of the affiliation. The dashed line at I2 at night symbolizes the separation of the bull. The affiliation between F4 and F5 was not recorded as the focus was on the female reticulated giraffes (F1-F3) in the study.
After the Rothschild females left the group (I1), changes in NN choice and social interaction were observed among the reticulated females. F1 spent equal time with F2 and F3 both during the day and night, with a slightly higher interaction rate with F2. F2 showed different preferences during the day and night; during the day, F1 and F3 were equally its NN, with more interaction with F3. At night, F2 preferred F3 as NN and interacted slightly more with it than with F1. F3 was equally NN to F1 and F2 during the day, with more social interaction with F1. At night, F3 spent more time close to F1, although interaction time was low with both F1 and F2.
High changes occurred when the bull (M1) joined the group (I2). During the day, F1 spent equal time as NN with F2 and F3. At night, the time F1 spent close to F2 and F3 increased compared to during B or I1. Despite the high NN time at night, no interaction was observed among the females. Although F1 showed the second-highest interaction rate with the bull during the day, it had the least interaction at night. F2 was most frequently NN to the bull and interacted the most with it during the day. At night, F2 and F3 showed similar interaction rates with the bull.
The findings of this study highlight a clear day-night activity rhythm in giraffes, characterized by a high level of active behavior during the day and predominantly resting behavior at night. The distinct diurnal and nocturnal activity levels observed underscore the importance of understanding giraffe behavior in both timeframes to fully comprehend their daily routines and social interactions.
During the daytime, the giraffes exhibited predominantly active behaviors, with distinct time allocated to walking, standing, and feeding (Figure 2). The observation of rare lying events during the day for F1 (1.46%) and F3 (2.1%), and more frequent lying phases for F2 (6.41%), underscores the variation in individual activity budgets among the giraffes, possibly influenced by factors such as age (Burger et al., 2020b; 2021), health (Sicks, 2012), or social rank (Horová et al., 2015). As many other ungulates, giraffes become more active and sleep less with increasing age (Burger et al., 2020b, 2021). Poor health conditions may also lead to a deviating activity budget. This change is often reflected in sleep behavior, with animals either sleeping very little or very much (Sicks, 2012). Horová et al. (2015) described dominance hierarchy in captive female giraffes as another potential driver for an altering activity budget. The three reticulated giraffes studied were all healthy and adult, so it can be assumed that the social component is the most likely cause of deviating activity budgets.
With the onset of darkness, the giraffes exhibited a marked decrease in activity levels, with the onset of rest characterized by lying down. They spent a substantial portion of the night lying, typically beginning their rest between 19:00-20:00 and ending in the early morning hours between 6:00-7:00. Sleep behavior occurred during these lying bouts, though it was brief. The observed decreases in activity levels and the increase in lying behavior reflect a natural rest period typically seen in diurnal ungulates (Bennie et al., 2014; Wu et al., 2018). Confirming former study results on the sleep behavior and fragmented sleep structure of giraffes (Burger et al., 2020b; Tobler and Schwierin, 1996; Veasey et al., 1996), short sleep events during lying phases were also observed in this study. These results suggest that giraffes are vigilant against predators even when resting - a behavior that is still present in the safe environment of zoos. The presence or absence of predators can impact sleep patterns, nocturnal social group formation, and vigilance behaviors (Voirin et al., 2014; Beauchamp, 2015). The required duration of sleep is, often correlated with factors such as age, body size, and ecological elements like environment, diet, and the safety of the animal’s resting site (Ohayon et al., 2004; Siegel, 2005). Tobler and Schwierin (1996) noted that the specific body position during sleep would likely make giraffes more vulnerable to predation. Additionally, the short sleep events may help giraffes avoid this vulnerability. However, the frequent interruptions of sleep by active behaviors, especially feeding, suggest that giraffes also need to feed at times during the night when they are awake. In the wild, these phases are probably also used to react to their environment, possibly to watch out for predators (Lima et al., 2005). In the wild, the animals in a group alternate between these active phases (Burger et al., 2020a), a behavior that is also largely preserved in zoos (compare Figure 1). Overall, this study underpins the giraffes’ nocturnal rhythm as biphasic (Burger et al., 2020a, b; Hart et al., 2020). This rhythm is consistent with the natural behavior of many large herbivores, who balance feeding and activity during the day with resting and minimal activity at night to conserve energy and remain vigilant (Bennie et al., 2014; Gübert et al., 2023a).
This study suggests that the activity budget of female reticulated giraffe changes distinctly during the day in response to changes in group composition, while their nocturnal activity budgets remained relatively constant (Figures 1, 2). The giraffes’ daytime behavior showed substantial changes in both standing and feeding activities across different group compositions. After the two Rothschild’s giraffe have left the reticulated females (I1), an increase in standing and a notable decrease in feeding has been observed. The introduction of the bull (I2) further amplified these changes. Standing behavior at daytime increased dramatically, while feeding remained low (Figure 2). The presence of the bull likely introduced new dynamics, such as increased vigilance or social interactions related to mating or dominance, leading to reduced feeding time and increased standing. Young and Isbell (1991) found sex differences in wild giraffe feeding ecology and habitat use. Furthermore, Stone et al. (2017) analyzed that each sex-age class of giraffes adjusts vigilance levels based on socio-sexual factors, influenced by their reproductive status and social rank. Thus, the presence of adult males reduced the food intake of immature males, while juveniles fed more in the presence of adult females. This would be a possible explanation that could explain the changes in the presence of the bull in this study. Furthermore, the type of food composition (Duggan et al., 2016; Sasson-Yenor and Powell, 2019), the presentation of food (Fernandez et al., 2008; Gussek et al., 2017) and the integration of feeding programs (Orban et al., 2016) also have an influence on the activity budget. However, these factors were not investigated in this study, as the focus was on the influence of changes in the social group composition.
Early studies of the social structure of giraffes described them as having little structure (Leuthold, 1979). However, this picture is increasingly changing due to more recent work on the social dynamics of giraffes.
The Mantel test results reveal that social network structures in giraffes appear to be relatively stable throughout the day in the baseline period, with a Mantel r value of 0.2427 and a p-value of 0.5, indicating no significant temporal variation in social interactions. This suggests that the baseline network is consistent across day and night (Figure 3). In contrast, during Period I1, the high Mantel r value of 0.918 (p = 0.333) suggests a high degree of stability in social networks between day and night, though the statistical significance was not achieved. However, in Period I2, the Mantel r value of -0.9994 with a p-value of 1 is particularly striking and suggests a deviation in network structure between day and night. This unexpected result may point to underlying changes in social dynamics, potentially due to the introduction of new individuals or other environmental factors (Figure 3).
When comparing different study periods, the Mantel tests indicate moderate similarities between the baseline and Period I1, with a Mantel r value of 0.366 (p = 0.5), and between the baseline and Period I2, with a Mantel r value of 0.3892 (p = 0.667). These results imply that while there are some changes in social structures between periods, they are not statistically significant. The significant negative correlation between Period I1 and Period I2 (Mantel r = -0.7148, p = 1) suggests substantial shifts in social networks, further highlighting the substantial changes in social structure with the male’s introduction. However, this result’s significance is questionable, likely due to the small sample sizes or other factors.
The MRQAP analysis during daytime observations revealed that subspecies explained a substantial portion of the variance in social bonds (R² = 0.7966), with a relatively large coefficient estimate for subspecies (11.85), although the p-value (p = 0.087) falls just short of a statistically significant effect. This suggests that subspecies might have a notable, though not conclusive, impact on social relationships during daylight hours.
Conversely, the results from nighttime observations showed a weaker overall influence of subspecies on the social network, as indicated by a lower R² value (0.1644) and a smaller coefficient for subspecies (6.24), with the effect again failing to reach statistical significance (p = 0.150). This could imply that social bonds during the night are less influenced by subspecies and may be driven by other factors (Muller et al., 2018b), such as environmental or behavioral differences at night.
Overall, the results suggest that giraffe social networks show some stability over time (Van der Waal et al., 2014), as indicated by the high Mantel r values, but the lack of statistical significance (high p-values) suggests caution in drawing strong conclusions. Additionally, subspecies may contribute to the structure of giraffe social networks (Carter et al., 2013a; Muller et al., 2018b; Burger et al., 2021), particularly during the day, but this effect was not statistically significant, warranting further investigation. Future research should explore additional factors that could influence social structure and consider larger sample sizes to clarify the role of subspecies in shaping giraffe social interactions.
Previous studies have shown that giraffes are organized into multilevel social structures, exhibiting a range of socially complex behaviors (Horová et al., 2015; Van der Waal et al., 2014). Social preferences or avoidance in giraffes are influenced by factors such as genetic relatedness, home range overlap, and individual preferences (Carter et al., 2013a). Additionally, gender, age, and environmental conditions play a significant role in shaping social interactions (Bercovitch et al., 2006; Bercovitch and Berry, 2015; Gloneková et al., 2017).
Our results align with these findings, as the reticulated giraffes in our study spent more time in close proximity to each other during the day, showing minimal interaction with the Rothschild giraffes. This species-specific social preference supports the idea that conspecific relationships are central to giraffe social dynamics (Carter et al., 2013a; Burger et al., 2021). The changes observed in individual F2, particularly after the introduction of a male, may reflect potential mating interests or shifts in the social hierarchy, as documented in other species where social and reproductive dynamics are influenced by group composition (Cameron and Du Toit, 2005; Muller et al., 2018b). These patterns highlight the nuanced social behaviors of giraffes and the factors that shape them.
Since the limited image quality and recording rate, especially in the night-time recordings, the present study is limited to clearly visible behaviors and social contacts. In addition, the giraffes spent a lot of time out of sight. Rumination for example, can be a key indicator of welfare in ruminants (Paudyal et al., 2018), an additional determination of this behavior would be desirable for follow-up studies. This also applies to negative welfare indicators, which include oral and locomotor stereotypies in giraffes (Bashaw et al., 2007), which were also not clearly identifiable at night in this study. The small sample size may reduce the statistical power and limit the generalizability of the findings. Additionally, mainly female giraffes and a limited number of subspecies were included, which may not fully represent the broader giraffe population or account for variations in social dynamics influenced by gender or other subspecies. Finally, the non-significant results highlight the need for further exploration, particularly with larger sample sizes and more refined predictors.
This study highlights the specific day-night activity rhythms of female reticulated giraffes, and the complex interplay between activity budgets and social dynamics. The findings emphasize the importance of considering both diurnal and nocturnal behaviors to fully understand giraffe ecology and social structure. The behavioral changes in response to group composition alterations underscore the sensitivity of giraffes to social environment changes, which could have implications for their management and conservation in both wild and captive settings. Future research should continue to explore the individual differences in activity patterns and the long-term effects of group composition changes to develop more comprehensive giraffe behavioral models. Understanding how giraffes respond behaviorally to social changes can inform strategies aimed at enhancing their welfare and promoting successful breeding programs.
The raw data supporting the conclusions of this article will be made available by the authors, without undue reservation.
Ethical review and approval was not required for the animal study because the study is a non-invasive study and not an animal experiment. All procedures performed in our studies with zoo animals met the ethical standards of the facility and were in accordance with all applicable national and/or institutional guidelines. The video recordings were not associated with changes in management routines and thus did not result in a change in normal behavior as a result of the measurement. Written informed consent was obtained from the owners for the participation of their animals in this study.
HM: Conceptualization, Data curation, Formal analysis, Investigation, Methodology, Writing – original draft, Writing – review & editing. SS: Formal analysis, Methodology, Validation, Writing-review & editing. PD: Conceptualization, Formal analysis, Funding acquisition, Project administration, Supervision, Visualization, Writing – original draft, Writing – review & editing. AB-S: Conceptualization, Formal analysis, Investigation, Methodology, Supervision, Writing – original draft, Writing – review & editing.
The author(s) declare that financial support was received for the research, authorship, and/or publication of this article. This study was supported by Opel-Zoo Foundation Professorship in Zoo Biology from the “von Opel Hessische Zoostiftung”.
The study was greatly supported by animal keepers of the Opel-Zoo Kronberg.
The authors declare that the research was conducted in the absence of any commercial or financial relationships that could be construed as a potential conflict of interest.
All claims expressed in this article are solely those of the authors and do not necessarily represent those of their affiliated organizations, or those of the publisher, the editors and the reviewers. Any product that may be evaluated in this article, or claim that may be made by its manufacturer, is not guaranteed or endorsed by the publisher.
The Supplementary Material for this article can be found online at: https://www.frontiersin.org/articles/10.3389/fcosc.2024.1462664/full#supplementary-material
Supplementary Table S1 | Percentage of individual behaviors in the three observation phases.
Bashaw M. J., Bloomsmith M. A., Maple T. L., Bercovitch F. B. (2007). The structure of social relationships among captive female giraffe (Giraffa camelopardalis). J. Comp. Psychol. 121, 46–53. doi: 10.1037/0735-7036.121.1.46
Baxter E., Plowman A. B. (2001). The effect of increasing dietary fibre on feeding, rumination and oral stereotypies in captive giraffes (Giraffa camelopardalis). Anim. Welfare. 10, 281–290. doi: 10.1017/S0962728600024052
Bennie J. J., Duffy J. P., Inger R., Gaston K. J. (2014). Biogeography of time partitioning in mammals. Proc. Natl. Acad. Sci. U.S.A. 111, 13727–13732. doi: 10.1073/pnas.1216063110
Bercovitch F. B., Bashaw M. J., del Castillo S. M. (2006). Sociosexual behavior, male mating tactics, and the reproductive cycle of giraffe. Giraffa camelopardalis. Horm. Behav. 50, 314–321. doi: 10.1016/j.yhbeh.2006.04.004
Bercovitch F. B., Berry P. S. M. (2015). The composition and function of all-male herds of Thornicroft's giraffe, Giraffa camelopardalis thornicrofti, in Zambia. Afric. J. Ecol. 53, 167–174. doi: 10.1111/aje.12169
Berger A. (2011). Activity patterns, chronobiology and the assessment of stress and welfare in zoo and wild animals. Int. Zoo Yearb. 45, 80–90. doi: 10.1111/j.1748-1090.2010.00121.x
Bond M. L., Lee D. E., Farine D. R., Ozgul A., König B. (2021). Sociability increases survival of adult female giraffes. Proc. R. Soc Lond. B Biol. Sci. 288, 20202770. doi: 10.1098/rspb.2020.2770
Bowyer R. T., McCullough D. R., Rachlow J. L., Ciuti S., Whiting J. C. (2020). Evolution of ungulate mating systems: Integrating social and environmental factors. Ecol. Evol. 10, 5160–5178. doi: 10.1002/ece3.6246
Brando S., Buchanan-Smith H. M. (2018). The 24/7 approach to promoting optimal welfare for captive wild animals. Behav. Process. 156, 83–95. doi: 10.1016/j.beproc.2017.09.010
Burger A. L., Fennessy J., Fennessy S., Dierkes P. W. (2020a). Nightly selection of resting sites and group behavior reveal antipredator strategies in giraffe. Ecol. Evol. 10, 2917–2927. doi: 10.1002/ece3.6106
Burger A. L., Hartig J., Dierkes P. W. (2020b). Shedding light into the dark: age and light shape nocturnal activity and sleep behaviour of giraffe. Appl. Anim. Behav. Sci. 229, 1–10. doi: 10.1016/j.applanim.2020.105012
Burger A. L., Hartig J., Dierkes P. W. (2021). Biological and environmental factors as sources of variation in nocturnal behavior of giraffe. Zoo Biol. 40, 171–181. doi: 10.1002/zoo.21596
Cajochen C., Münch M., Knoblauch V., Blatter K., Wirz-Justice A. (2006). Age-related changes in the circadian and 417 homeostatic regulation of human sleep. Chronobiol. Int. 23, 461–474. doi: 10.1080/07420520500545813
Cameron E. Z., Du Toit J. T. (2005). Social influences on vigilance behaviour in giraffes, Giraffa camelopardalis. Anim. Behav. 69, 1337–1344. doi: 10.1016/j.anbehav.2004.08.015
Carter K. D., Brand R., Varter J. K., Shorrocks B., Goldizen A. W. (2013b). Social networks, long-term associations and age-related sociability of wild giraffe. Anim. Behav. 86, 901–910. doi: 10.1016/j.anbehav.2013.08.002
Carter K. D., Seddon J. M., Frere C. H., Carter J. K., Goldizen A. W. (2013a). Fission-fusion dynamics in wild giraffe may be driven by kinship, spatial overlap and individual social preferences. Anim. Behav. 85, 385–394. doi: 10.1016/j.anbehav.2012.11.011
Duggan G., Burn C. C., Clauss M. (2016). Nocturnal behavior in captive giraffe (Giraffa camelopardalis)-A pilot study. Zoo Biol. 35, 14–18. doi: 10.1002/zoo.21248
Favreau A., Richard-Yris M.-A., Bertin A., Houdelier C., Lumineau S. (2009). Social influences on circadian behavioural rhythms in vertebrates. Anim. Behav. 77, 983–898. doi: 10.1016/j.anbehav.2009.01.004
Fernandez L. T., Bashaw M. J., Sartor R. L., Bouwens N. R., Maki T. S. (2008). Tongue twisters: feeding enrichment to reduce oral stereotypy in giraffe. Zoo Biol. 27, 200–212. doi: 10.1002/zoo.20180
Friard O., Gamba M. (2016). BORIS: A free, versatile open-source event-logging software for video/audio coding and live observations. Meth. Ecol. Evol. 7, 1325–1330. doi: 10.1111/2041-210X.12584
Gloneková M., Vymyslická P. J., Žáčková M., Brandlová K. (2017). Giraffe nursing behaviour reflects environmental conditions. Behaviour 154, 115–129. doi: 10.1163/1568539X-00003413
Grasso C., Poso G., Lenzi C. (2022). Social network-proximity association: Preliminary evaluation of giraffe sociality in a zoo-housed group. Anim. Behav. Cogn. 9, 80–88. doi: 10.26451/abc.09.01.07.2022
Gübert J., Hahn-Klimroth M., Schneider G., Dierkes P. W. (2023a). Nocturnal behavioral patterns of African ungulates in zoos. Ecol. Evol. 13, e10777. doi: 10.1002/ece3.10777
Gübert J., Hahn-Klimroth M., Dierkes P. W. (2023b). A large-scale study on the nocturnal behavior of African ungulates in zoos and its influencing factors. Front. Ethol. 2. doi: 10.3389/fetho.2023.1219977
Gussek I., Hirsch S., Hartmann M., Südekum K.-H., Hummel J. (2017). Feeding practices for captive giraffes (Giraffa camelopardalis) in Europe: A survey in EEP zoos. JZAR 5, 62–70. doi: 10.19227/jzar.v5i1.152
Hart E. E., Fennessy J., Hauenstein S., Ciuti S. (2020). Intensity of giraffe locomotor activity is shaped by solar and lunar zeitgebers. Behav. Process. 178, 104178. doi: 10.1016/j.beproc.2020.104178
Hill S. P., Broom D. M. (2009). Measuring zoo animal welfare: theory and practice. Zoo Biol. 28, 531–544. doi: 10.1002/zoo.20276
Horová E., Brandlová K., Gloneková M. (2015). The first description of dominance hierarchy in captive giraffe: not loose and egalitarian, but clear and linear. PLoS One 10, e0124570. doi: 10.1371/journal.pone.0124570
Hutchins M., Wiese R. J., Smith B. (2019). “Introduction: research in zoos and aquariums: purpose, justification, utility and welfare,” in Scientific Foundations of Zoos and Aquariums. Their Role in Conservation and Research. Eds. Kaufman A. B., Bashaw M. J., Maple T. L. (Cambridge University Press, Cambridge).
Lesku J. A., Roth T. C., Rattenborg N. C., Amlaner C. J., Lima S. L. (2008). Phylogenetics and the correlates of mammalian sleep: a reappraisal. Sleep Med. Rev. 12, 229–244. doi: 10.1016/j.smrv.2007.10.003
Leuthold B. M. (1979). Social organization and behaviour of giraffe in Tsavo East National Park. Afric. J. Ecol. 17, 19–34. doi: 10.1111/j.1365-2028.1979.tb00453.x
Lewton J., Rose P. E. (2019). Evaluating the social structure of captive Rothschild’s giraffes (Giraffa camelopardalis rothschildi): Relevance to animal management and animal welfare. J. Appl. Anim. Welf. Sci. 23, 178–192. doi: 10.1080/10888705.2019.1573682
Lima S., Rattenborg N., Lesku J., Amlaner C. (2005). Sleeping under the risk of predation. Anim. Behav. 70, 723–736. doi: 10.1016/j.anbehav.2005.01.008
Martin P., Bateson P. P. G. (2007). Measuring behaviour: An introductory guide (Cambridge: Cambridge University Press, 3rd edition).
Merrow M., Spoelstra K., Roenneberg T. (2005). The circadian cycle: Daily rhythms from behaviour to genes. EMBO Rep. 6, 930–935. doi: 10.1038/sj.embor.7400541
Mistlberger R. E., Rusak B. (2005). “Circadian rhythms in mammals: Formal properties and environmental influences,” in Principles and practice of sleep medicine, 4th Edition. Eds. Kryger M., Roth T., Dement W. C. (Amsterdam, Netherlands: Elsevier), 321–334.
Muller Z., Bercovitch F., Brand R., Brown D., Brown M., Bolger D., et al. (2018a). Giraffa camelopardalis (amended version of 2016 assessment). The IUCN Red List of Threatened Species 2018. doi: 10.2305/IUCN.UK.2016-3.RLTS.T9194A136266699.en
Muller Z., Cantor M., Cuthill I. C., Harris S. (2018b). Giraffe social preferences are context dependent. Anim. Behav. 146, 37–49. doi: 10.1016/j.anbehav.2018.10.006
Ohayon M. M., Carskadon M. A., Guilleminault C., Vitiello M. V. (2004). Meta-analysis of quantitative sleep parameters from childhood to old age in healthy individuals: developing normative sleep values across the human lifespan. Sleep 27, 1255–1273. doi: 10.1093/sleep/27.7.1255
Orban D. A., Siegford J. M., Snider R. J. (2016). Effects of guest feeding programs on captive giraffe behavior. Zoo Biol. 35, 157–166. doi: 10.1002/zoo.21275
Paudyal S., Maunsell F. P., Richeson J. T., Risco C. A., Donovan D. A., Pinedo P. J, et al. (2018). Rumination time and monitoring of health disorders during early lactation. Animal 12, 1484–1492. doi: 10.1017/S1751731117002932
Partch C. L., Green C. B., Takahashi J. S. (2014). Molecular architecture of the mammalian circadian clock. Trends Cell Biol. 24, 90–99. doi: 10.1016/j.tcb.2013.07.002
Price E. P., Stoinski T. S. (2007). Group size: Determinants in the wild and implications for the captive housing of wild mammals in zoos. Appl. Anim. Behav. Sci. 103, 255–264. doi: 10.1016/j.applanim.2006.05.021
Rattenborg N., La Iglesia H., Kempenaers B., Lesku J., Meerlo P., Scriba M. (2017). Sleep research goes wild: new methods and approaches to investigate the ecology, evolution and functions of sleep. Philos. Trans. R. Soc B 372, 20160251. doi: 10.1098/rstb.2016.0251
Rose P. (2023). Identifying essential elements of good giraffe welfare - Can we use knowledge of a species’ fundamental needs to develop welfare-focussed husbandry? J. Zool. Bot. Gard 4, 549–566. doi: 10.3390/jzbg4030039
Sasson-Yenor J., Powell D. M. (2019). Assessment of contrafreeloading preferences in giraffe (Giraffa camelopardalis). Zoo Biol. 38, 414–423. doi: 10.1002/zoo.21513
Seeber P. A., Ciofolo I., Ganswindt A. (2012). Behavioural inventory of the giraffe (Giraffa camelopardalis). BMC Res. Notes. 5, 650. doi: 10.1186/1756-0500-5-650
Sicks F. (2012). Paradoxer Schlaf als Parameter zur Messung der Stressbelastung bei Giraffen (Giraffa camelopardalis). Goethe University Frankfurt, Germany. Available at: https://d-nb.info/1044276150/34.
Siegel J. (2005). Clues to the functions of mammalian sleep. Nature 437, 1264–1271. doi: 10.1038/nature04285
Stone D. B., Cherry M. J., Martin J. A., Cohen B. S., Miller K. V. (2017). Breeding chronology and social interactions affect ungulate foraging behavior at a concentrated food resource. PLoS One 12, e0178477. doi: 10.1371/journal.pone.0178477
Szemán K., Liker A., Székely T. (2021). Social organization in ungulates: Revisiting Jarman’s hypotheses. J. Evol. Biol. 34, 604–613. doi: 10.1111/jeb.13782
Tarou L. R., Bashaw J. B., Maple T. L. (2000). Social attachment in giraffe: Response to social separation. Zoo Biol. 19, 41–51. doi: 10.1002/(SICI)1098-2361(2000)19:1%3C41::AID-ZOO5%3E3.0.CO;2-J
Tobler I., Schwierin B. (1996). Behavioural sleep in the giraffe (Giraffa camelopardalis) in a zoological garden. J. Sleep Res. 5, 21–32. doi: 10.1046/j.1365-2869.1996.00010.x
Van der Waal K., Wang H., McCowan B., Fushing H., Isbell L. A. (2014). Multilevel social organization and space use in reticulated giraffe (Giraffa camelopardalis). Behav. Ecol. 25, 17–26. doi: 10.1093/beheco/art061
Veasey J. S., Waran N. K., Young R. J. (1996). On comparing the behaviour of zoo housed animals with wild conspecifics as a welfare indicator, using the giraffe (Giraffa camelopardalis) as a model. Anim. Welf. 5, 139–153. doi: 10.1017/S0962728600018571
Voirin B., Scriba M., Martinez-Gonzalez D., Vyssotski A., Wikelski M., Rattenborg N. (2014). Ecology and neurophysiology of sleep in two wild sloth species. Sleep 37, 753–761. doi: 10.5665/sleep.3584
Watters J. V., Margulis S. W., Atsalis S. (2009). Behavioral monitoring in zoos and aquariums: a tool for guiding husbandry and directing research. Zoo Biol. 28, 35–48. doi: 10.1002/zoo.20207
Wu Y., Wang H., Wang H., Feng J. (2018). Arms race of temporal partitioning between carnivorous and herbivorous mammals. Sci. Rep. 8, 1713. doi: 10.1038/s41598-018-20098-6
Keywords: 24/7 activity budget, Giraffa camelopardalis, social behavior, diurnal and nocturnal behavior, behavioral rhythms
Citation: Mebus H, Schneider S, Dierkes PW and Burger-Schulz AL (2024) Around the clock: unveiling giraffe rest-activity rhythms and social dynamics. Front. Conserv. Sci. 5:1462664. doi: 10.3389/fcosc.2024.1462664
Received: 10 July 2024; Accepted: 07 October 2024;
Published: 29 October 2024.
Edited by:
Oded Berger-Tal, Ben-Gurion University of the Negev, IsraelReviewed by:
Paul Rose, University of Exeter, United KingdomCopyright © 2024 Mebus, Schneider, Dierkes and Burger-Schulz. This is an open-access article distributed under the terms of the Creative Commons Attribution License (CC BY). The use, distribution or reproduction in other forums is permitted, provided the original author(s) and the copyright owner(s) are credited and that the original publication in this journal is cited, in accordance with accepted academic practice. No use, distribution or reproduction is permitted which does not comply with these terms.
*Correspondence: Hanna Mebus, TWVidXNAZW0udW5pLWZyYW5rZnVydC5kZQ==
Disclaimer: All claims expressed in this article are solely those of the authors and do not necessarily represent those of their affiliated organizations, or those of the publisher, the editors and the reviewers. Any product that may be evaluated in this article or claim that may be made by its manufacturer is not guaranteed or endorsed by the publisher.
Research integrity at Frontiers
Learn more about the work of our research integrity team to safeguard the quality of each article we publish.