- 1School of Marine Sciences, University of Maine, Orono, ME, United States
- 2Marine Mammal Commission, Bethesda, MD, United States
- 3Maine Sea Grant, Orono, ME, United States
Successful conservation of pinnipeds in the northwest Atlantic has led to increasing populations of harbor seals (Phoca vitulina) and gray seals (Halichoerus grypus) in the Gulf of Maine. Within this region, habitat restoration and diadromous fish conservation in the Penobscot River have also been top priorities for the past decade. To understand the overlap between the regional recovery of pinnipeds and the aggregative response of pinnipeds to increasing forage fish, we assessed how counts, distribution, and behavior of seals in the Penobscot River Estuary have changed over time from 2012 to 2020 and determined whether those changes were related to changes in fish biomass that are occurring as the result of diadromous fish restoration. We did not see increased counts of hauled-out seals, but consistent with regional harbor seal phenology, hauled out seal counts were highest in late spring and declined throughout the summer and into the fall. The number of swimming harbor and gray seals, analyzed as a proxy for changes in behavior, showed a stronger annual trend with an increase throughout the study period. Fish biomass was negatively associated with total number of hauled out seals and swimming gray seals but positively associated with swimming harbor seals. We also documented the potential displacement of harbor seals when gray seals are present. Together, these results begin to provide insights into how regional conservation and local restoration efforts interact to affect multiple trophic levels in an ecosystem. Continued monitoring of predator-prey interactions, along with diet and movement studies, will further elucidate seal aggregative response to increasing prey species in this system and the potential impact of recovering predator populations on restored prey populations. Knowledge gained regarding pinniped response to increasing fish biomass has important implications for other systems with ongoing conservation measures that aim to improve habitat, decrease exploitation, or recover protected species. Studies like these can be critical for finding paths forward to reconcile the potentially competing objectives of marine mammal protection and fish restoration.
1 Introduction
Habitat restoration and conservation efforts have led to many successful recovery stories worldwide. These success stories often result in unintended consequences, such as increasing interaction between protected species and humans, or negative impacts to vulnerable, protected prey populations following protected predator recovery (Yodzis, 2001; Marshall et al., 2016). Ecosystem-based management has been proposed as one solution to these challenges (Okey and Wright, 2004; Wells et al., 2020), and progress towards its implementation has been made through the development of ecosystem models that account for predator-prey interactions (Townsend et al., 2019). Yet, balancing the competing needs of multiple protected species with human use is complicated by persistent gaps in knowledge surrounding food web structure (Pringle and Hutchinson, 2020), particularly in recovering systems (Vander Zanden et al., 2006). Gaining a better understanding of how predators and prey both respond to habitat restoration efforts could ultimately increase our ability to successfully and adaptively manage natural resources while promoting overall ecosystem health.
In the Northeast United States (U.S.), seals occupy the role of top or near-top predators in many coastal ecosystems that are the focus of contemporary restoration and conservation efforts (Hayes et al., 2022). Seals, which were historically hunted to near or complete local extirpation, have been generally increasing in the region since federal legislation mandating protection of all marine mammals in U.S. waters was passed in 1972 (Roman et al., 2013; Hayes et al., 2022). Harbor seals (Phoca vitulina) were the first to experience population growth (Gilbert et al., 2005), followed by immigration and rapid population growth of gray seals (Halichoerus grypus) (Wood et al., 2022). More recently, as gray seal numbers continue to grow, the harbor seal population has appeared to be steady or in decline (Sigourney et al., 2021; Hayes et al., 2022). While gray and harbor seals are often found hauled out together and exhibit site fidelity to the same locations, gray seals have also displaced harbor seals at some sites (Murray, 2008; Pace et al., 2019), which could be a factor in the recent decline of harbor seal population growth rates (Waring et al., 2015).
The growth of seal populations in the Northeast U.S. has occurred alongside numerous other conservation efforts in the region, including many focused on recovering depleted fish populations. Although the recovery of healthy prey populations can support the growth and recovery of predator populations, these efforts may at times be perceived as in conflict, with predator population growth inhibiting prey recovery. For example, gray seals are often blamed for the failed recovery of cod (Gadus morhua) in Canada (Chouinard et al., 2005). In the Pacific Northwest, pinniped consumption may negatively impact or prevent the recovery of populations of salmonids, steelhead trout (Oncorhynchus mykiss irideus), and Pacific herring (Clupea pallasii), even if these species comprise a small percentage of pinniped diet (Berejikian et al., 2016; Nelson et al., 2023; Moore et al., 2024). These interactions and their impacts are complex; the scale of impact can be dependent on the periodicity of migration for diadromous species (Falkegård et al., 2023), and the direction of impact can vary from negative to positive depending on interacting bottom-up and indirect effects of predation (Conwell et al., 2024; Trzcinski et al., 2024). It is therefore challenging to predict how recovering populations of prey will impact predators and vice versa.
The Penobscot River Estuary, the largest watershed in the state of Maine in the Northeast U.S., provides an opportunity to examine how protected predators respond to major habitat restoration and fish conservation efforts. In addition to harbor and gray seals, the Penobscot River is also home to 12 species of diadromous fish, all of which have experienced significant population declines due to dam construction, pollution, and overfishing (Saunders et al., 2006; Bernier, 2017). Along with the declines in diadromous fish populations, loss or reduction of ecological services, such as regulating and provisioning estuary and marine food webs, have also occurred (Ouellet et al., 2022). With the goal of restoring diadromous fish runs and their ecological services while balancing the need for hydropower production, major restoration efforts have included the removal of two dams in 2012 and 2013, the installation of the river’s first fish lift in 2014, and the construction of a nature-like fish bypass at a second dam in 2016 (NRCM, 2019).
Restoration and conservation efforts in the Penobscot River have resulted in increasing diadromous fish use of the river and estuary each year (Gardner et al., 2013; Scherelis et al., 2019; Stevens et al., 2023). Fish counts at the river’s southernmost dam reveal significant increases in blueback herring (Alosa aestivalis) and alewives (Alosa pseudoharengus), collectively known as river herring, since dam removal began in 2012 (MDMR, 2018, 2019). Similarly, fish biomass in the estuary, as estimated by hydroacoustic surveys, has been increasing since 2012 during a period of diadromous fish restoration, with more areas of high fish density appearing in later years (Stevens et al., 2023). The estuary biomass is a complex composed of mainly Clupeidae species which are known forage fish for seals in the Northwest Atlantic (Bowen and Harrison, 1996; Hammill et al., 2014; Sette et al., 2020). Accordingly, anecdotal reports of seals have increased as fish populations have recovered, along with concern regarding the impacts of these predators on fish species of conservation concern in the river. Some studies have reported increased observations of seal predation on diadromous fish, primarily Atlantic salmon (Kusnierz et al., 2014), while others suggest that increasing forage fish may provide protection to adult salmon against seal predation (Leach et al., 2022).
While habitat restoration and conservation efforts in rivers and estuaries have often focused on diadromous fish, the bottom-up effects of ecological restoration on fish predators in these systems remains understudied. This study therefore aims to investigate the overlap between the regional recovery of pinnipeds and the aggregative response of pinnipeds to increasing forage fish populations. Our objectives were to: 1) assess how counts, distribution, and behavior of seals in the Penobscot River Estuary have changed over time, seasonally and annually, from 2012 to 2020; and 2) determine if these changes are related to changes in fish biomass. Because the response of predators to increasing prey could include an increase in presence or a shift in behavior, we evaluated counts of hauled out seals to assess changes in relative abundance over time and evaluated the number of animals that were swimming as a proxy for behavior. Considering the divergent recovery trajectories of gray seals and harbor seals in the region, we used two years of species-specific data to evaluate species-specific interactions and relationships with fish biomass over time. Knowledge gained regarding pinniped response to increasing fish biomass could improve our understanding of current and potential future impacts of prey-focused ecological restoration on predators. Lessons learned also could be applicable to other systems with ongoing conservation measures that aim to improve habitat, decrease exploitation, or recover protected species. This insight could inform future management decisions on how to best reconcile the potentially competing objectives of marine mammal protection and fish restoration.
2 Materials and methods
2.1 Data collection
Boat-based transect surveys of the Penobscot River Estuary were conducted by the National Oceanic and Atmospheric Administration (NOAA) from 2012 through 2020 to assess fish and seal abundance and distribution. As described in Lipsky et al. (2019), hydroacoustic surveys of the Penobscot River Estuary were conducted from April through October, as weather allowed, each year (Supplementary Table S1). Surveys were scheduled weekly through mid-June, during the peaks of several diadromous fish runs, and biweekly throughout the remainder of the season. Beginning on a flood tide, surveys followed pre-determined transect lines from south to north (Figure 1). This section of the river is approximately 50 kilometers long (Lipsky et al., 2019).
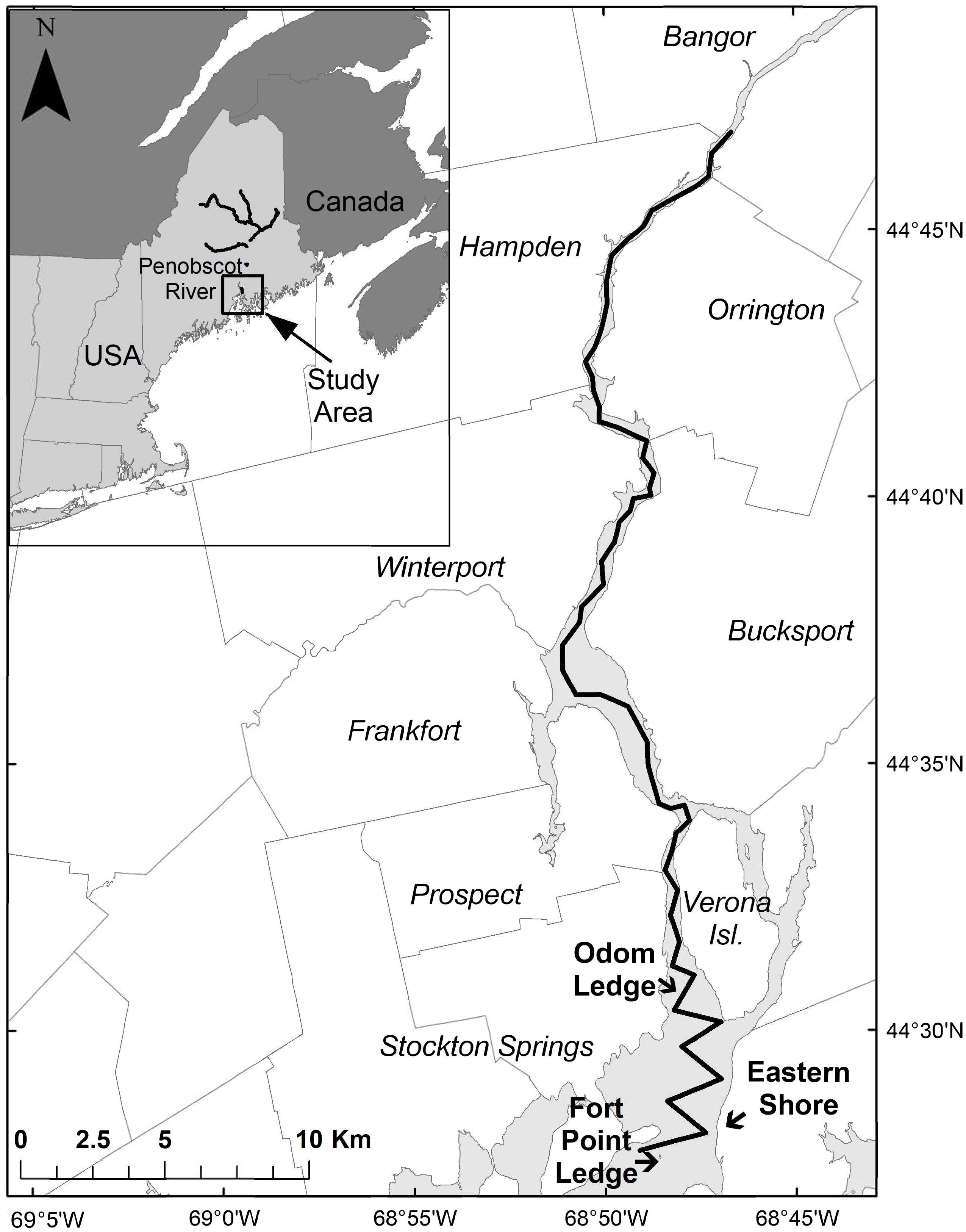
Figure 1. Map of transect survey route with major seal haul-out locations labeled in relation to the Northeast U.S.
Along the transect, fish abundance and distribution were characterized via hydroacoustic data gathered using mobile split beam echosounders at 38kHz and 120kHz frequencies (O’Malley et al., 2017). Concurrently, both sides of the river, as well as the area in front of the boat, were continuously scanned for marine mammal sightings using 10x50 magnification binoculars, and the time, species, number of animals, and behavioral data for all sightings were recorded. In addition, the number of hauled out seals were recorded at three major seal haul-outs along the survey route: Odom Ledge, Fort Point Ledge, and Eastern Shore (Figure 1). Odom Ledge (44° 30’ 57”N 68° 48’ 03”W) and Fort Point Ledge (44° 27’ 38”N 68° 48’ 35”W) are emergent ledges at most tide heights and marked on NOAA navigational chart US5ME26M as navigational hazards. Eastern Shore (44° 28’ 11”N 68° 47’ 13”W) is an area characterized as emergent rocky habitat near low tide (also described as a navigational hazard on NOAA charts). We acknowledge that since these surveys were designed to assess relative seal abundance, these counts represent a minimum number present, not absolute abundance.
Prior to 2019, the incidental take of marine mammals during this work was authorized by Letter of Authorization #2016-22582 issued by the National Marine Fisheries Service (NMFS) and haul-outs were not directly approached closer than 500 meters for targeted assessment of seals. During 2019 and 2020, haul-outs were approached to within 100 meters and photographs were taken using a Canon 7D Mark I camera with a 100-400 millimeter lens so that counts and species identification could be verified after the survey. This pinniped-focused research was authorized by NMFS permit #21719-01.
2.2 Data filtering
Site-specific factors including time from low tide, time of day, and wind have been shown to affect the number of seals hauled out at a given time (Schneider and Payne, 1983; Yochem et al., 1987; Watts, 1996; Raposa and Dapp, 2009). Our surveys typically occurred in the morning, began at Fort Point at low tide, and could only be conducted in relatively good weather, so these potentially confounding factors were partially controlled for in the survey design. The data were filtered to further limit variation in environmental effects so that we could conservatively assess trends in counts over time.
Typically, seal surveys in our region are conducted within two hours on either side of low tide (Gilbert et al., 2005; Waring et al., 2015; Sigourney et al., 2021), as this is when the greatest number of animals tend to be hauled out on land (Watts, 1996). We therefore excluded any surveys that did not begin within two hours of low tide from the analysis. Approximate time from low tide was calculated for each survey using the “rtide” package (v0.0.5; Thorley et al., 2018) and R version 4.4.1 in RStudio (v2024.04.2 + 764) and historic tide data from a station near Fort Point.
Environmental variables, such as fog, sea state, and wind, were not recorded during the surveys, so our ability to directly account for the effects of these environmental factors is limited. The hydroacoustic survey, however, has informal requirements for sea state because high winds and rough seas are not conducive to quality acoustic data collection due to the generation of acoustic noise (Simmonds and MacLennan, 2008). Therefore, we assumed that all surveys were conducted in relatively similar weather conditions in the absence of high winds and rough seas. To verify this assumption, approximate wind speed data for each sighting were pulled from NOAA’s National Climatic Data Center’s (NCDC) recordings from a sensor at Bangor International Airport (approximately 38 kilometers from the start of the survey). An average wind speed was calculated for the time we surveyed the southern section of the estuary during each survey using the NCDC data. Most surveys occurred at wind speeds less than 5 kilometers per hour, and the highest estimated wind speed was 8.06 kilometers per hour. A negative binomial generalized linear model, which was used to account for overdispersion of the data, showed that there was not a significant relationship between wind speed and seal counts (p = 0.611) and no outliers were detected (MASS package v.7.3-61; Venables and Ripley, 2002). Therefore, we did not exclude any surveys due to wind speed.
Finally, incomplete surveys missing data from any of the three primary seal haul-outs or a biomass estimate were excluded. In total, this conservative filtering approach retained 93 out of 134 surveys for the analyses across the full time series (Supplementary Tables S2, S3). Similar filtering of the 2019 and 2020 photo-count data, which included species-level information on hauled out seals, retained 20 out of 24 surveys (Supplementary Table S4).
2.3 Data analysis
2.3.1 Temporal analysis of hauled out and swimming seals
To assess change in seal abundance and behavior in the Penobscot River Estuary over time, as well as whether those changes were related to changes in fish biomass, we analyzed the total number of hauled out seals and number of swimming gray and harbor seals per survey. Seal assessment methods typically estimate abundance using hauled out seal counts with a correction factor to account for the number of seals at sea (Gilbert et al., 2005; Waring et al., 2015; Sigourney et al., 2021), and use in-water counts to characterize distribution (Herr et al., 2009; Vincent et al., 2017) or behavior (e.g., in response to underwater sound; Ampela et al., 2021) at sea. Accordingly, we analyzed our counts of hauled out and swimming animals separately, using hauled out seal counts to represent relative abundance (without a correction factor) and counts of swimming seals to explore changes in behavior. Our surveys took place near low tide, when most seals are expected to be hauled out on the tidally emergent ledges in this system. As such, changes in the number of swimming seals during the survey could reflect changes in behaviors including foraging, socializing, and transiting. Because species-level identification can be difficult at a distance and the survey originally did not approach haul-outs for targeted assessments of seals, all analyses that include data from 2012 to 2018 focus on the total number of hauled out seals (i.e., counts of both gray and harbor seals) instead of specifying species. Swimming animals, however, were often documented closer to the survey vessel where they could be easily identified, so swimming counts for each species were analyzed separately. Each survey date was associated with a standard week and an estimated value of fish biomass present in the estuary, calculated by Stevens et al. (2023) using the hydroacoustic data collected along the survey transect line.
We used generalized linear models (GLMs) to assess the effect of year, standard week, and fish biomass on seal counts. We also evaluated the interaction between biomass and the two temporal variables, to assess if the effect of biomass on seals varied throughout the survey season or between years. The swimming seal models further assessed whether the total number of hauled out seals was related to the number of animals swimming. All covariates were centered (by subtracting the mean) and scaled (by dividing by the standard deviation) for modeling analysis, so that regression coefficients could be directly compared between covariates measured on different scales (Schielzeth, 2010). We assessed biomass to be skewed so we transformed this covariate to log10 biomass (logBiomass). We assessed our models for covariate collinearity using variance inflation factors (VIF) and used simulation-based tests for overdispersion, zero-inflation, and temporal autocorrelation (DHARMa package v.0.4.6; Hartig, 2022). When data were not overdispersed, we ran GLMs using a Poisson distribution appropriate for positive integer count data. When overdispersion was detected, GLMs were run using a negative binomial distribution (MASS package v. 7.3-61; Venables and Ripley, 2002). When zero-inflation was detected, we ran a zero-inflation GLM with a negative binomial distribution (pscl package v. 1.5.9; Jackman, 2024). From the global model for each dataset, we conducted all-subsets model selection (MuMIn package v.1.48.4; Bartoń, 2024) and ranked models based on the corrected Akaike’s Information Criterion (AICc). We report model fit as Nagelkerke’s pseudo-R2 for Poisson and negative binomial models and as R2 based on the residual variance divided by the total variance for zero-inflated models (performance package, v. 0.12.2; Lüdecke et al., 2021). The goal of our model selection process was to identify informative covariates and evaluate their effect on seal counts. Covariate importance was evaluated based on standardized effect sizes and Akaike weights (Schielzeth, 2010).
2.3.2 Species-specific analysis of hauled out seals
Using similar methods, the 2019 and 2020 photo-count data were used to explore species-specific relationships between the number of hauled out seals, week, and biomass. Counts of gray seals were also included as a covariate in the harbor seal model, and vice versa, to explore whether interspecific interactions affect counts of hauled out seals.
3 Results
Hauled out and swimming seals were observed during 96.77% and 78.49% of surveys (n = 93), respectively. Across all surveys from 2012 to 2020, on average, 31.83 (sd: 23.73; range: 0-97) seals were observed per survey on haul-outs and 4.51 (sd: 4.87; range: 0-19) seals were observed per survey swimming. Among the swimming seals, which could be identified to species, 78.28% were harbor seals and 21.72% were gray seals across the full dataset. Similarly, when hauled out seals were identified to species through photo-analysis, an average of 80.39% (sd: 21.41; range: 45.45-100%) and 81.96% (sd: 15.39; range: 50.67-100%) of the hauled out seals counted per survey in 2019 and 2020, respectively, were harbor seals.
There was large interannual variation in seal counts across the time series, but on average, the greatest number of hauled out seals was observed at Odom Ledge (average: 17.27, sd: 16.61, range: 0-60) and Fort Point (average: 12.19, sd: 13.23, range: 0-60), with fewer seals observed at Eastern Shore (average: 2.20, sd: 4.74, range: 0-23) and other sites. Hauled out seals were observed at lesser-used rocky sites, primarily in the lower estuary, on seven days across the study period, four of which occurred in 2019 and 2020. The number of seals at Odom Ledge tended to decrease from 2012 to 2020; while the minimum number of animals appeared constant across low years, the maximum number of animals observed in years with many seals consistently decreased from 2012 to 2013 and again from 2015 to 2018 (Figure 2B). In contrast, the number of seals counted at Eastern Shore tended to increase throughout the time series, particularly after 2015, with the highest mean and median values observed in 2018 and 2020 (Figure 2C).
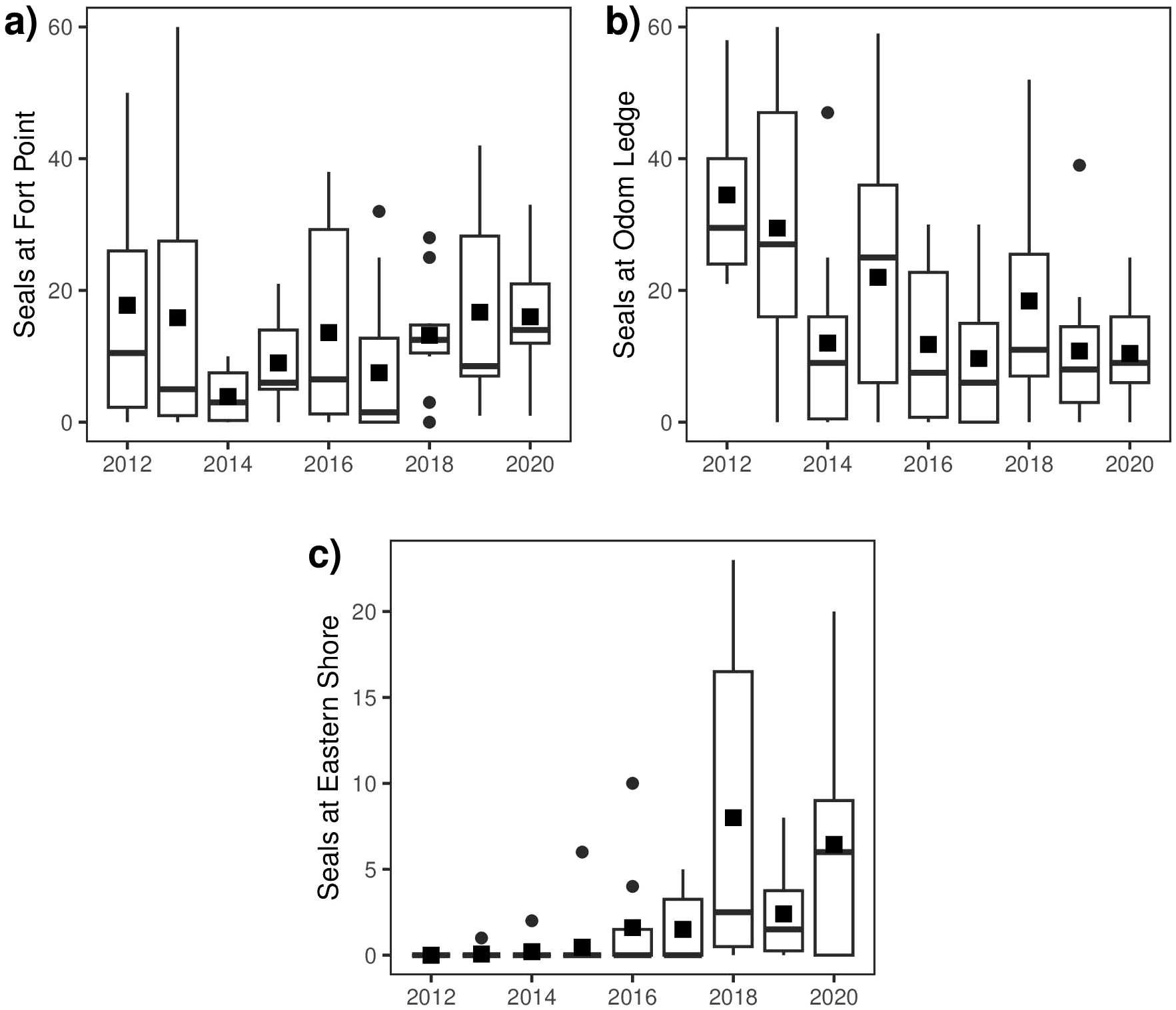
Figure 2. Number of seals (gray and harbor) per survey counted at three haul-out sites [(A) Fort Point, (B) Odom Ledge, and (C) Eastern Shore] in the Penobscot River Estuary from 2012-2020. Box plots show the median and lower and upper quartiles; black squares indicate the mean; whiskers extend to 1.5x the interquartile range; and individual dots show values falling beyond this range.
3.1 Temporal analysis of hauled out and swimming seals
To determine whether the number of seals in the Penobscot River Estuary changed over time and whether those changes were related to increasing fish biomass, within the context of known seasonal dynamics (e.g., breeding and molting periods), generalized linear models were used to explore the effects of year, fish biomass, and week on the total number of hauled out seals and the number of swimming gray and harbor seals counted during each survey. The number of hauled out seals was modeled using a zero-inflated negative binomial model to account for overdispersion detected in the initial Poisson model (ratio observed:expected variance = 9.91, p < 1x10-15) and evidence of zero-inflation detected after applying a negative binomial family (ratio observed:predicted zeros = 5.56, p = 0.03). There was no evidence of temporal autocorrelation within years in the negative binomial GLM that would warrant addition of an autoregressive term (Durbin-Watson test, all p > 0.05). Furthermore, there no evidence of an influence of covariate collinearity in the final global model (all VIF < 3.75). The interaction terms between logBiomass and the temporal covariates were not significant in the global model, so they were removed prior to nested model selection. Subsequent model ranking revealed two top models with strong evidence based on Akaike weights (Tables 1, 2). The top model included only standard week, while the second top model included both standard week and logBiomass. Standard week had a negative effect, reflecting that the number of hauled out seals was highest earlier in the year and decreased throughout the surveys each year (Figure 3A). LogBiomass also had a negative effect, reflecting that fewer seals were hauled out when fish biomass was greater, but this effect was smaller and there was more variation around the relationship (Figure 3A).
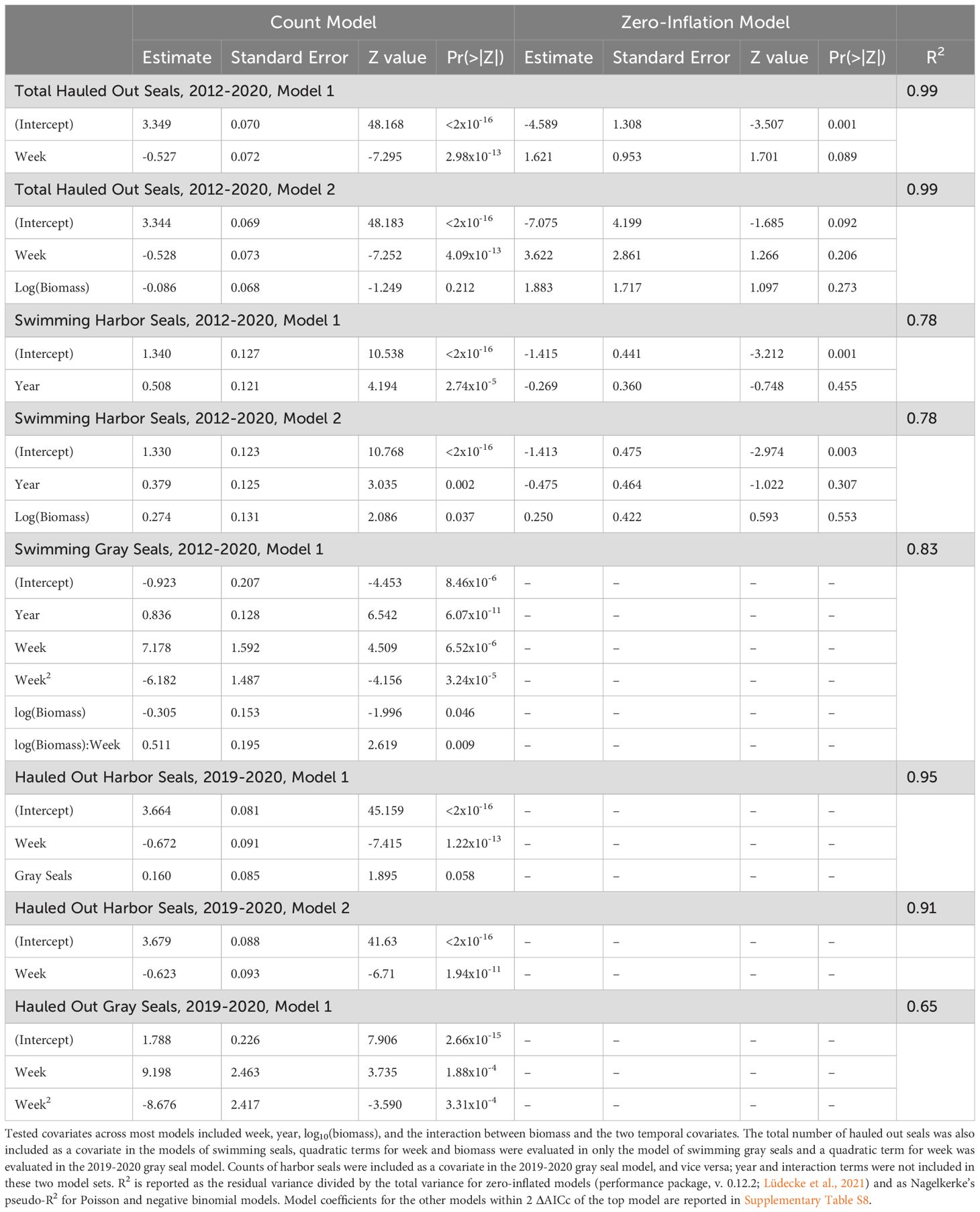
Table 1. Model coefficients for the top models exploring drivers of the number of hauled out seals, swimming harbor seals, and swimming gray seals during surveys of the Penobscot River Estuary from 2012-2020, and the numbers of hauled out harbor and gray seals in 2019-2020.

Table 2. Model ranking of generalized linear models evaluating the effects of log10(biomass), standard week, and year on the number of hauled out seals per survey in the Penobscot River Estuary, 2012-2020.
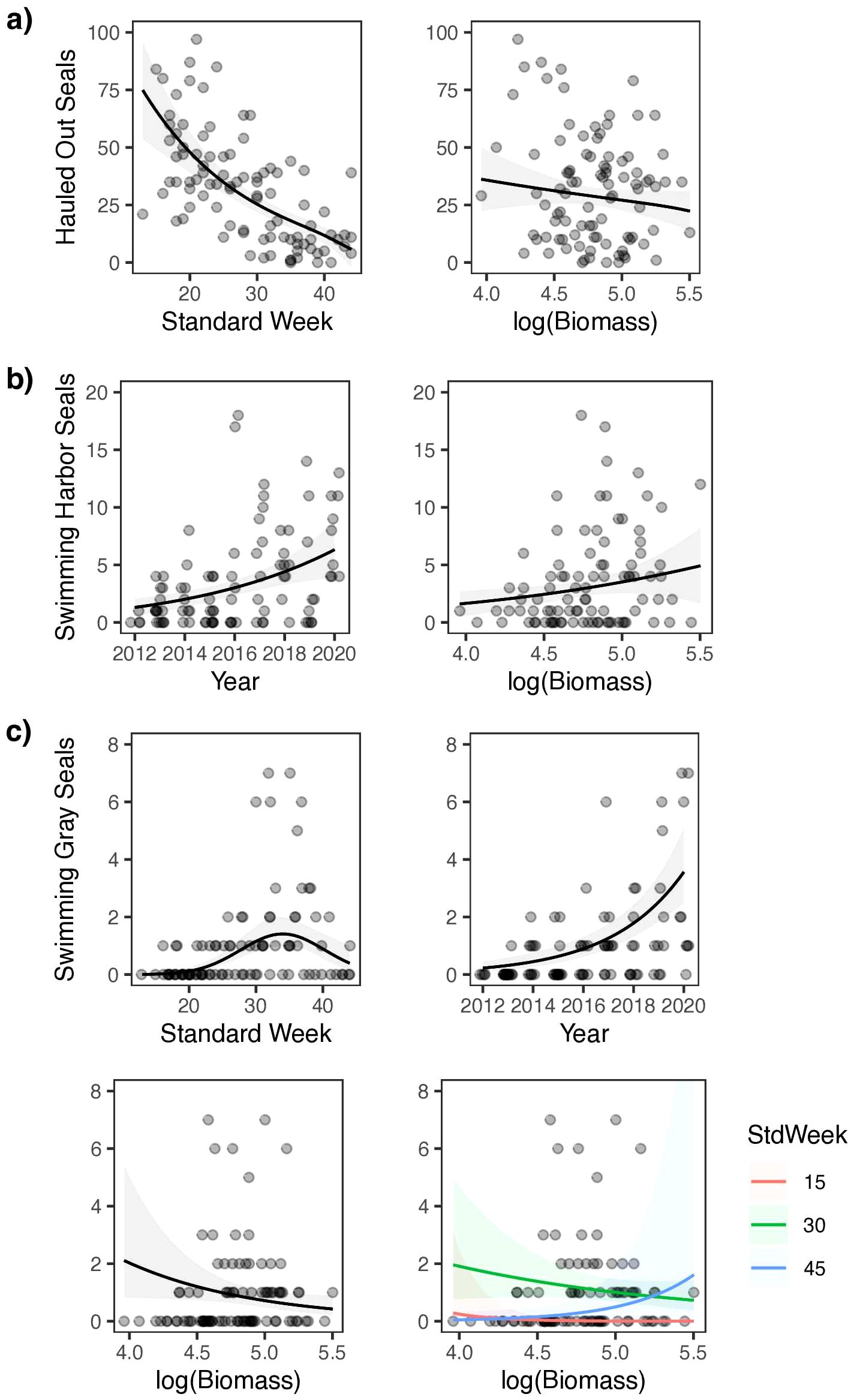
Figure 3. Partial effect plots showing the effects of year, log10(biomass), standard week, and the interaction of log10(biomass) with the temporal covariates on the (A) total number of hauled out seals, (B) number of swimming harbor seals and (C) number of swimming gray seals, counted during surveys of the Penobscot River Estuary, 2012 to 2020. Biomass refers to fish biomass in the estuary, as estimated by hydroacoustic surveys by Stevens et al. (2023). Only covariates found in the top two models are plotted. Trend lines with 95% confidence intervals depict the predicted relationships from models 2 for the total number of hauled out seals and the number of swimming harbor seals, and from model 1 for the number of swimming gray seals (see Tables 1–3 for additional model information). Standard weeks correspond to seasons as follows: spring (March-June) includes weeks 17-26; summer (July-August) includes weeks 27-35; and fall (September-October) includes weeks 36-44.
The number of swimming harbor seals was also modeled using a zero-inflated negative binomial model to account for overdispersion detected in the initial Poisson model (ratio observed:expected variance = 3.53, p < 1x10-15) and evidence of possible zero-inflation detected after applying a negative binomial family (ratio observed:predicted zeros = 1.16, p=0.35). There was no evidence of temporal autocorrelation within years after a Bonferroni correction (Durbin-Watson test: 2017 p=0.03, 2019 p=0.03, all other years p > 0.05). Furthermore, there was no evidence of an influence of covariate collinearity in the final global model (all VIF < 2.2). The interaction terms between logBiomass and the temporal covariates were not significant, so they were removed prior to nested model selection. Subsequent model ranking again revealed two top models with very similar support based on Akaike weights (Tables 1, 3). The top model included only year, while the second top model included both year and logBiomass. Year had a positive effect, reflecting that the number of swimming harbor seals increased over time throughout our study (Figure 3B). LogBiomass also had a positive effect, reflecting that there were more swimming harbor seals when fish biomass was greater (Figure 3B).
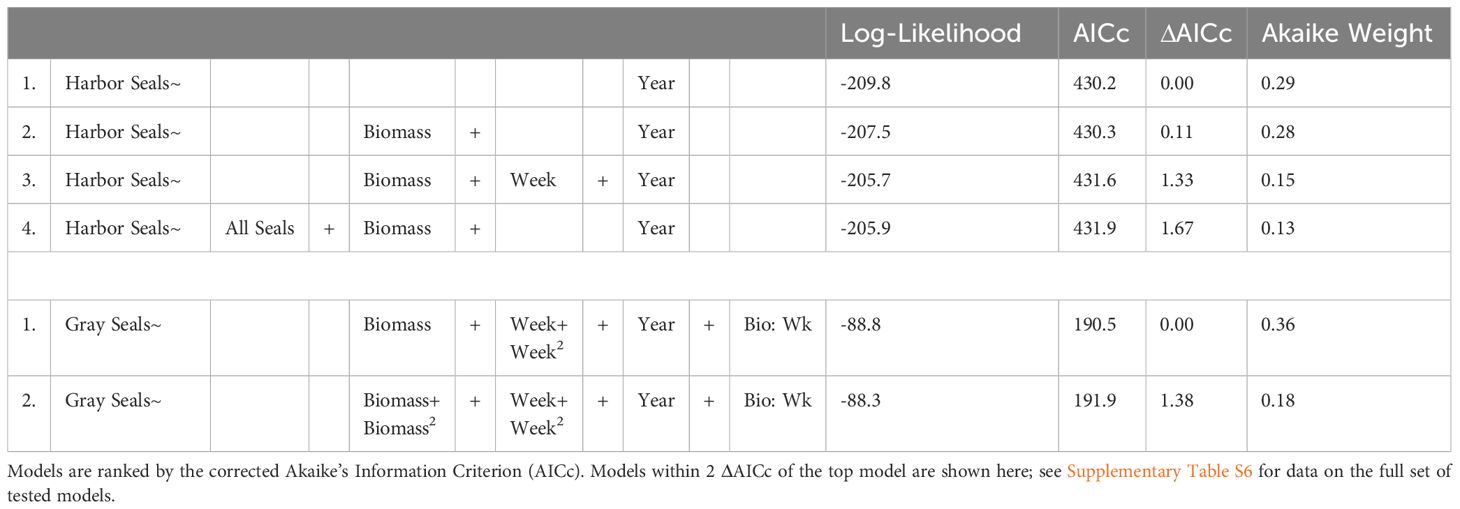
Table 3. Model ranking of generalized linear models evaluating the effects of the number of hauled out seals, log10(biomass), standard week, and year on the numbers of swimming harbor and gray seals per survey in the Penobscot River Estuary, 2012-2020.
The number of swimming gray seals was modeled using a Poisson GLM as the initial model showed no evidence of overdispersion (ratio observed:expected variance = 1.21, p = 0.36), zero-inflation (ratio observed:predicted zeros = 0.98, p = 0.85), or temporal autocorrelation within years (Durbin-Watson test, all p >0.05). Furthermore, there was no evidence of an influence of covariate collinearity (all VIF < 2.0). The interaction term between logBiomass and year was not significant, so it was excluded prior to nested model selection. Subsequent model ranking revealed that the top model contained logBiomass, standard week, year, and the interaction term between logBiomass and week. Upon inspection of the effect plots, however, it appeared that the relationship of swimming gray seal counts with standard week and logBiomass might be nonlinear. Following a reviewer recommendation, we therefore added quadratic terms for both covariates and re-ran the model selection process, which revealed two models within 2 ΔAICc (Tables 1, 3). The top model contained the linear and quadratic terms for standard week, year, biomass, and the interaction between biomass and week. The second top model also included the quadratic term for logBiomass, but based on its effect size and Akaike weight, this quadratic term was considered less likely to be informative. The number of swimming gray seals increased across years and appeared to peak between weeks 30 and 35 (Figure 3C). The observed relationship between counts of swimming gray seals and logBiomass appeared to be negative (Figure 3C).
3.2 Species-specific analysis of hauled out seals
To determine whether the changes in relative abundance were driven by species-specific patterns, GLMs were used to explore the effects of week, biomass, and presence of the other seal species on counts of hauled out harbor and gray seals during 2019 and 2020, when photographs of haul-outs enabled species identification. The harbor seal data were modeled using a negative binomial GLM to account for overdispersion detected in an initial Poisson model (ratio observed:expected variance = 6.86, p < 1x10-15). There were no zero-counts, evidence of temporal autocorrelation in the dataset (Durbin-Watson test, all p>0.05), or evidence of an influence of covariate collinearity (all VIF < 1.4). Model ranking revealed two top models within 2 ΔAICc with strong evidence based on Akaike weights (Tables 1, 4). Both of the top models included a negative effect of standard week, reflecting that harbor seal counts decreased throughout the survey season. One of the top models also included a slightly positive effect of the number of gray seals present, though this trend appeared driven by a few surveys with a large number of gray seals (Figure 4A).
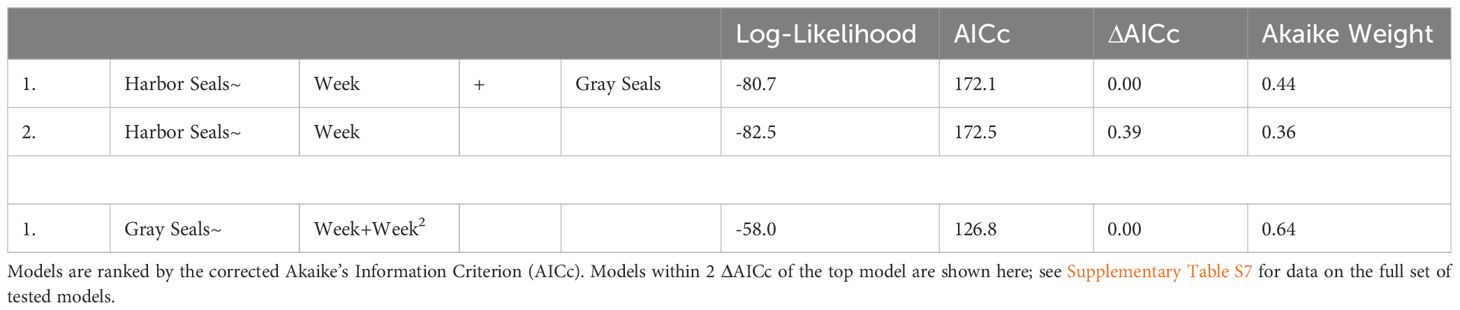
Table 4. Model ranking of generalized linear models evaluating the effects of log10(biomass), standard week, and the number of gray and harbor seals on the numbers of hauled out harbor and gray seals per survey in the Penobscot River Estuary, 2019-2020.
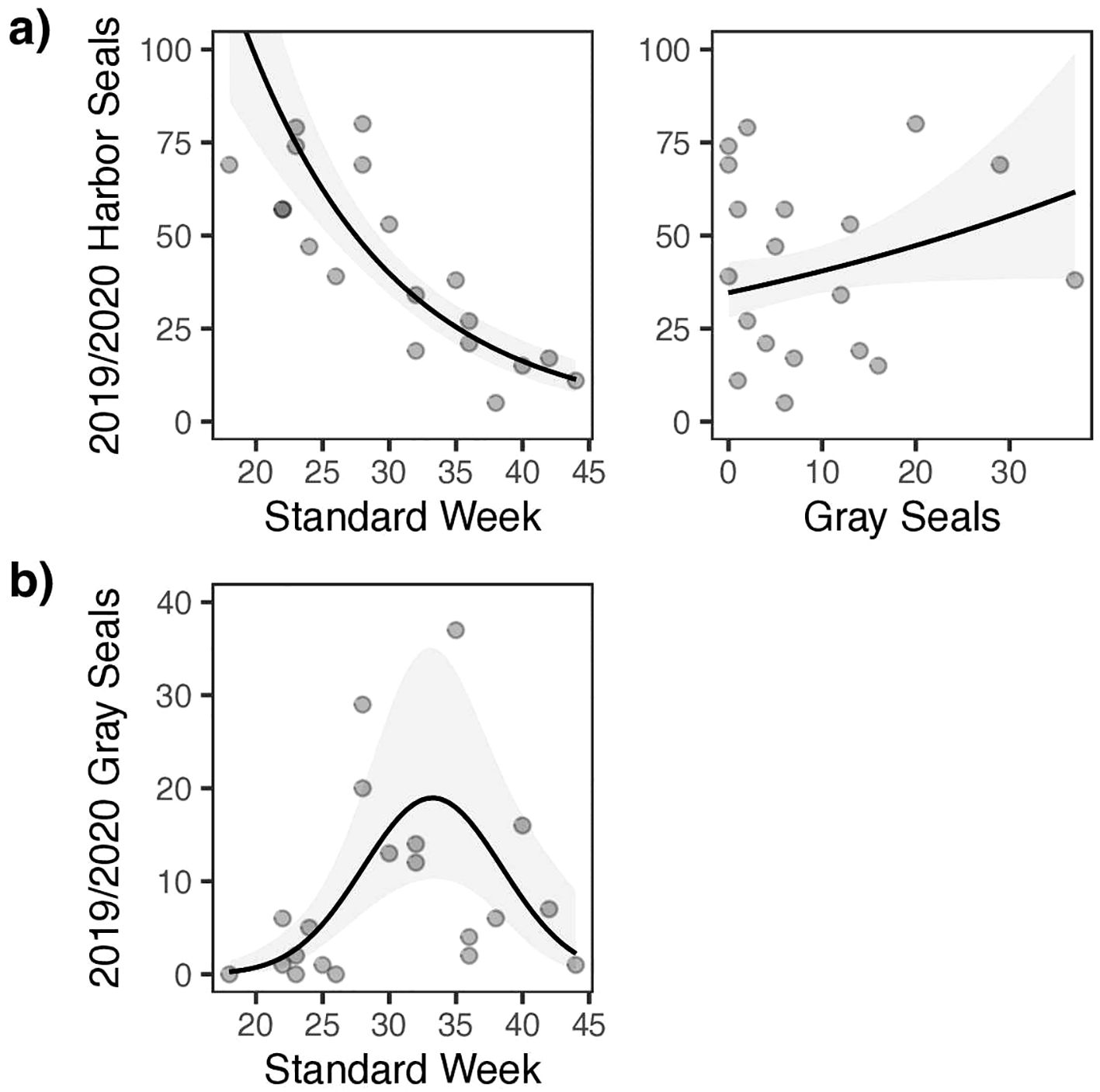
Figure 4. Partial effect plots showing the effects of standard week and the other seal species on the number of hauled out (A) harbor seals and (B) gray seals counted using photographs of haul-outs during surveys of the Penobscot River Estuary, 2019 to 2020. Only covariates found in the top model are plotted. Trend lines with 95% confidence intervals depict the predicted relationships from model 1 for both species (see Tables 1, 4 for additional model information). Standard weeks correspond to seasons as follows: spring (March-June) includes weeks 17-26; summer (July-August) includes weeks 27-35; and fall (September-October) includes weeks 36-44.
The gray seal data were also modeled using a negative binomial GLM to account for overdispersion detected in an initial Poisson model (ratio observed:expected variance = 6.40, p < 1x10-15). The model showed no evidence of zero-inflation (ratio observed:predicted zeros = 1.01, p = 1), temporal autocorrelation (Durbin-Watson test, all p>0.05), or influence of covariate collinearity (all VIF < 2.7). Because our swimming gray seal model provided evidence for a nonlinear relationship with standard week, we included a quadratic term for standard week in this model as well. The only top model within 2 ΔAICc included the linear and quadratic terms for standard week (Tables 1, 4). Gray seal counts appeared to increase throughout the survey season starting around week 20, peaking between weeks 30 and 35, and declining for the rest of the season (Figure 4B).
To further explore the relationship between the numbers of gray seals and harbor seals, we considered the spatial distribution of the two species when hauled out in the estuary in 2019 and 2020 (Figure 5). Both gray and harbor seals utilized Fort Point and Odom Ledge, but only harbor seals were observed at Eastern Shore. Harbor seals were observed at Eastern Shore in the late summer and early fall, with the greatest increases in numbers typically observed during times when gray seal counts increased at the other haulouts.
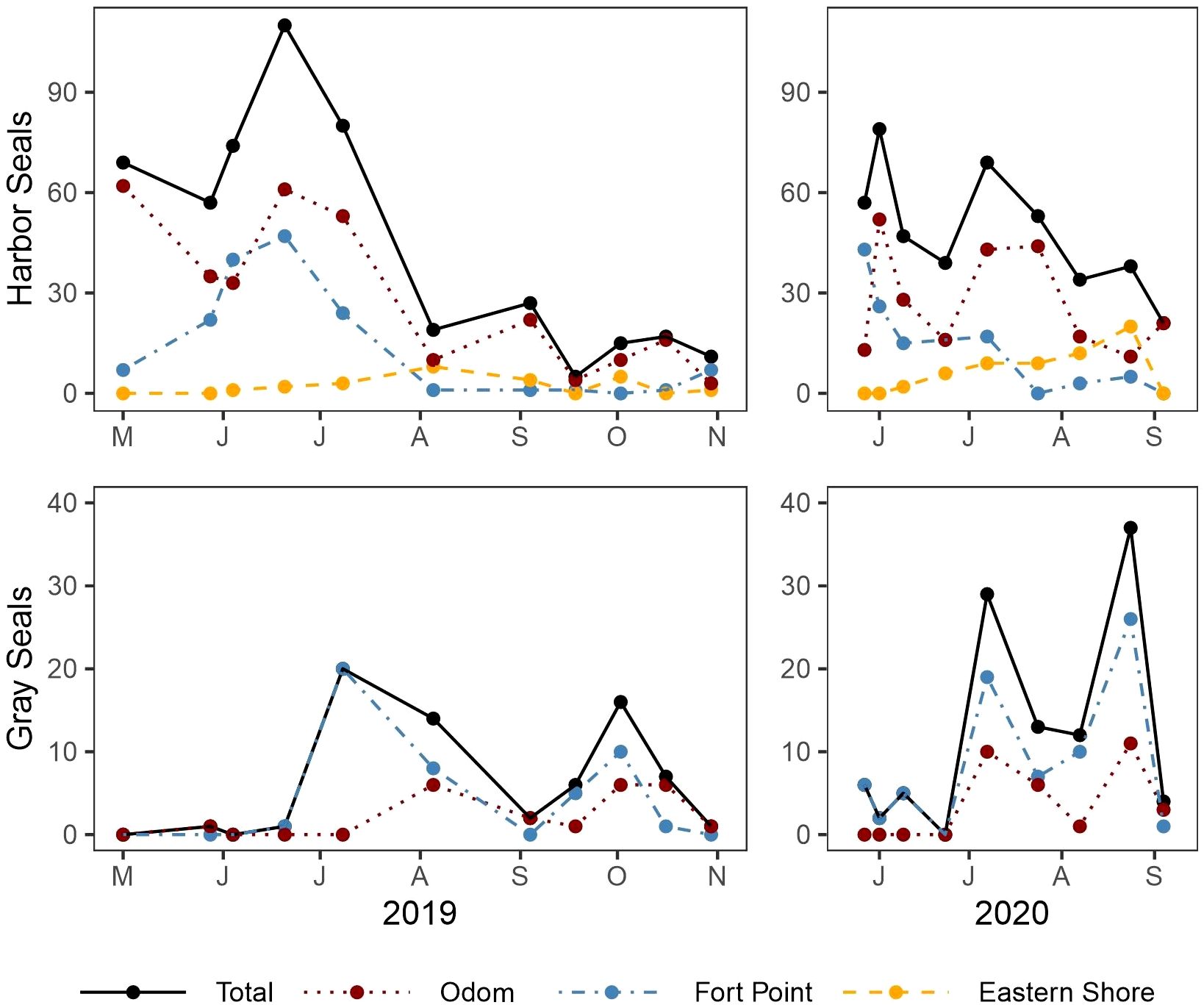
Figure 5. Number of harbor and gray seals counted via photographs taken at three haul-out sites (Fort Point, Odom Ledge, and Eastern Shore) in the Penobscot River Estuary from May to November, 2019 and June to September, 2020.
4 Discussion
The Penobscot River Estuary survey has provided a unique opportunity to study long-term trends in rebounding seal populations in a system that is recovering a forage base of diadromous fish. Separate conservation efforts targeted at predators and prey in this region have both reported individual success (Hayes et al., 2022; Stevens et al., 2023), but the interaction of the two programs had not previously been studied. By considering these putative predator-prey relationships, as well as potential interactions between the two seal species that are recovering in this system, we contribute to the ongoing conversation about complex, sometimes unintended consequences, of marine mammal recovery (Cammen et al., 2019).
In our analysis, we utilized complementary data collection of predators using visual counts and putative prey through hydroacoustic measurements. While neither effort is meant to estimate absolute population size, our ability to track the relative abundance change is powerful due to the repeatability of the survey that follows the same route, at relatively the same point in the tide cycle, in similar weather conditions, allowing observations of changes over time that provide ecological insights.
Throughout our study, hauled out seal counts, assessed both as total counts of seals for the full time series and at the species-level for harbor and gray seals during the two most recent years, did not demonstrate increases over the time period but were most closely related to seasonal phenology. Appropriately since the majority of hauled out seals were harbor seals, we found total seal counts generally followed patterns consistent with harbor seal biological and life-cycle milestones. Seal counts peaked early in our survey season, which corresponds with harbor seal pupping season in May and June, when harbor seals move into the Gulf of Maine and tend to spend more time on the rocks (Brown and Mate, 1983). Seal counts were lowest in fall, consistent with dispersal to southern New England and mid-Atlantic waters (Hayes et al., 2022). For gray seals in 2019 and 2020, counts appeared to increase starting in mid-May (around week 20), peaked in late July and August (between weeks 30 and 35), and then declined. Gray seals in the U.S. and Canada experience a spring molting season between mid-April and June (Lesage and Hammill, 2001; Pace et al., 2019). Gray seals appear to move into our study area after dispersing at the end of their molting season and before they congregate at pupping colonies for their winter pupping season (Lesage and Hammill, 2001). Year was not included in any of our top models, however, it should be noted that in 2012, only 4 surveys were retained for this analysis, most of which occurred early in the season. Similarly, 9 of the 16 surveys conducted in 2013 occurred early in the season. It is possible that the wide variation and high means seen from those years in Figure 2 are contributing to the lack of detectable trend across years.
The periodicity of migration for diadromous species to and from the ocean creates a critical overlap in space and time for predators and their prey, which may limit the recovery of depressed populations despite restoration measures (Falkegård et al., 2023). In the Penobscot River Estuary, the peak in seal counts overlaps with the timing of the diadromous fish migrations. Adult Atlantic salmon migrating from the ocean into the river typically peak between mid-May and early July (weeks 20-27), adult river herring migration peaks between mid-May and mid-June (weeks 20-25), and American shad migration peaks between late May through late June (weeks 22-26) (Bruchs et al., 2018). Downstream migration of juvenile Atlantic salmon smolts also occurs in spring (McCormick et al., 1998; Saunders et al., 2006; Stich et al., 2015). This overlap in presence occurs at a time of high energetic demand for female harbor seals that must alter their behavior, including foraging, to support lactation and their own metabolism (Boness et al., 1994; Bowen et al., 2001; Schwarz et al., 2018).
To indirectly explore changes in seal behavioral trends in the Penobscot River Estuary throughout and following restoration, we analyzed counts of swimming harbor and gray seals across the time series. For both species, we observed increasing numbers of swimming seals from 2012 to 2020. For gray seals, we found that counts of swimming animals peaked in late July and August (between weeks 30 to 35), similar to the counts of hauled out gray seals in 2019 and 2020. For this species, it is possible that the increasing number of swimming seals reflects the increasing size of the population in the northwest Atlantic (Hayes et al., 2022). The fact that standard week and the total number of hauled out seals, which is consistent with regional harbor seal trends, were not strongly related to counts of swimming harbor seals is a promising indication that these counts represent ecologically-relevant behavior, not just population size in the river. The increase in swimming harbor seals, however, is difficult to interpret using our data because a swimming seal may be engaging in a variety of behaviors, including foraging, transiting, or socializing. To complicate matters, we would expect seals to spend varying amounts of time hauled out or in the water throughout the season. For example, we might expect more seals to be hauled out during the pupping and molting seasons (Stobo and Fowler, 1994), more males to spend time in the water during the mating season (Hayes et al., 2006), and the presence of gray seals could alter harbor seal haul-out patterns and increase their susceptibility to flushing from haul-out sites when disturbed (Murray, 2008; Russell et al., 2015). Additionally, because our study system is not closed, it is also possible that the increase in swimming harbor seals is the result of seals coming into the survey area from nearby regions. There are several other harbor seal haul-outs in the upper part of Penobscot Bay within 10 to 25 kilometers of our study site, a distance easily traveled by harbor seals during foraging trips (Lowry et al., 2001; Cunningham et al., 2008; Sharples et al., 2012). Expanding the geographic scope of this study in the future, for example, by placing tags on seals from nearby haul-outs, could help reveal whether the estuary has become a more desirable foraging location for seals located elsewhere in this part of the Gulf of Maine. Furthermore, diet studies will also be important to confirm the predator-prey interactions that we are assuming in our interpretation of the data and to more clearly understand the potential impact of seal predation on continued fish recovery efforts.
In addition to describing how seals in the Penobscot River have changed over time, our study set out to test if these changes are related to changes in fish biomass that have occurred as a result of river restoration. LogBiomass was found among the top models for total hauled out seals and swimming seals of both species; however, its effect size was typically smaller than that reported for the temporal covariates, reflecting more noise around the predicted relationships. In the models that included logBiomass, its effect was slightly negative for total hauled out seals and swimming gray seals but slightly positive for harbor seals. Here and elsewhere, the complex relationships between seals and their prey complicate efforts to understand the impacts of fish abundance on pinniped populations (Li et al., 2010). Studies in the Pacific Northwest have shown that the response of seals to prey aggregations, for example, during herring spawning, can vary depending on the size of the aggregation, prey energy density, and the availability of alternative prey (Thomas et al., 2011; Lance et al., 2012). Other studies have not found a strong relationship between forage fish abundance and predator productivity, especially for highly mobile, generalist predators, such as seals (Hilborn et al., 2017; Free et al., 2021). However, seals also are central place foragers, and increases in local prey abundance near breeding sites could lead to benefits through reduced foraging effort (Free et al., 2021). For example, declines in pup production of Northern fur seals (Callorhinus ursinus) in Alaska have been attributed in part to fisheries-depletion of important prey (Short et al., 2021). As a result of the depletion of local prey resources, lactating females must expend increased foraging effort during longer foraging trips, likely contributing to reduced pup growth and survival at St. Paul Island (Short et al., 2021; McHuron et al., 2023). In the Penobscot River Estuary, where fish trends are reversed as a result of river restoration efforts, it is thus possible that increasing local prey abundance could lead to reduced foraging effort and subsequent increasing pinniped productivity and pup survival. Our data do not allow us to test this hypothesis and instead reflect a weak relationship between relative abundance and fish biomass. This may be due to the mismatch between the spring peak in seal counts and summer peak in biomass, so it is possible that seal counts could begin to increase more significantly over time.
In addition to predator-prey interactions, we considered the impact of ecological restoration on interactions between predator species. Though there have been a limited number of reported instances of direct antagonistic interactions between gray and harbor seals (van Neer et al., 2015; Westphal et al., 2023), we assume most ecological interactions between the two species occur as a result of indirect competition for food and/or haul-out space. During our surveys in 2019 and 2020, the first sighting of gray seals at Fort Point and Odom Ledge coincided with a decline in harbor seals at Fort Point Ledge, which reversed when gray seals left near the end of the season. Coincident with the first sighting of gray seals on Fort Point Ledge, we also observed the first sightings of harbor seals at Eastern Shore, located across the river from Fort Point Ledge, suggesting harbor seals may move to this haul-out when gray seals are present. The potential displacement of harbor seals by gray seals when they first arrive at haul-out sites in the estuary is consistent with inter-specific interactions observed between gray and harbor seals elsewhere (Murray, 2008; Pace et al., 2019; Sette et al., 2020). There are also some indications in our dataset that this displacement began earlier than we documented it in 2019; the number of seals observed at Eastern Shore began to increase around 2016 (Figure 2C), suggesting that gray seals may have started increasing in the estuary around that time.
During this study, individual gray seals were documented on haul-outs only four times from 2012 to 2018, though as we have mentioned, species level identification in those years may not have been accurate as haul-outs were not specifically approached as in 2019 and 2020. The presence of multiple gray seals at the haul-out sites was first documented on our July 8, 2019 survey. Throughout the rest of the 2019 season, we observed gray seals hauled out on three major haul-outs in the survey area, with as many as 26 gray seals seen at one haul-out site in one day. While this influx of gray seals appears to be new for the Penobscot River Estuary, it reflects similar changes that have been documented throughout the Gulf of Maine (Gilbert et al., 2005; Pace et al., 2019).
We recognize that hauled out and swimming pinnipeds can be difficult to detect and count from a distance and expect that imperfect detection due to availability bias and perception bias during our surveys may have led to some of the observed variation in seal counts during our study. For example, variation in seal behavior, dive duration, environmental conditions, and observer experience can affect the probability that a seal will be detected during a survey, meaning that seal presence may not have been accurately and consistently captured. Additionally, the number of seals present in an area, as well as the proportion hauled out at a certain time are highly variable (Pace et al., 2019), which could also affect our results. It is also possible that disturbance of seals affected our counts and the potential observed patterns over time. Odom Ledge is adjacent to the main boating channel, so seals on that ledge are regularly exposed to vessel traffic in the estuary. Based on our observations during the survey, the seals appeared more likely to flush earlier in the season before presumably habituating to vessel presence as the season went on. Seals were also more likely to be disturbed by the closer vessel approaches during the survey in 2019 and 2020 compared to the previous years. Finally, distribution patterns may have also influenced counts in the post-dam removal period (2014-2020) due to the expanded access that pinnipeds had outside the survey area to an additional 14 kilometers of free-flowing river. Despite those caveats, we report expected seasonal patterns and local abundance trends that mirror regional trends for hauled out gray and harbor seals. Within that context, changes in fish biomass that have occurred during the study period appear to have little effect on the relative abundance of seals in the Penobscot River Estuary. Several ecological models of predator-prey dynamics predict that the predator population will lag slightly behind that of the prey (Gause, 1935). It is therefore possible that more time is needed before pinnipeds exhibit a stronger response to the growth in fish populations in the Penobscot River, especially considering the different life histories and reproductive strategies of seals compared to river herring. Continued assessment of pinnipeds in this system therefore remains important, and we recommend a particular focus on targeted assessments of seal behavior, which this study suggested have significantly changed over time during this period of shifting prey base.
Continued work to understand seal diet and response to fish restoration efforts in the Penobscot River Estuary, and ultimately the subsequent impact seals have on those fish populations, is not only important for understanding predator-prey dynamics in systems focusing on habitat restoration, fish conservation, or recovery from human exploitation, but could also inform future efforts to conserve or recover other predator species. For example, pinniped aggregative response to increasing forage fish has major implications regarding if and when increasing prey provides population-level benefits to seals and should be considered when developing recovery plans for prey species of conservation concern (Hill et al., 2020). Although seals in the Penobscot are not threatened or endangered, efforts to recover other opportunistic predators could also use this work to evaluate the potential for habitat restoration and increasing forage fish to help achieve their recovery goals.
Data availability statement
The raw data supporting the conclusions of this article will be made available by the authors, without undue reservation.
Ethics statement
Ethical review and approval was not required for the study on animals in accordance with the local legislation and institutional requirements.
Author contributions
LL: Data curation, Formal analysis, Funding acquisition, Investigation, Writing – original draft, Writing - review & editing. JS: Data curation, Investigation, Methodology, Project administration, Writing – review & editing. KC: Conceptualization, Formal analysis, Funding acquisition, Methodology, Visualization, Writing – review & editing.
Funding
The author(s) declare financial support was received for the research, authorship, and/or publication of this article. This work was funded by the Maine Outdoor Heritage Fund (Project 181-03-01), Ruth Hiebert Memorial Fellowship, and the University of Maine’s Graduate Student Government. The survey was supported, in part, by NOAA Fisheries through the Cooperative Institute for the North Atlantic Region (CINAR) under Cooperative Agreement NA14OAR4320158. Data collection was funded by NOAA Fisheries in partnership with Maine Sea Grant. Funding for manuscript publication was provided by the Marine Mammal Commission.
Acknowledgments
We thank Paul Music, Rory Saunders, Tim Sheehan, and Christine Lipsky from NOAA Fisheries for their contribution to the conceptual design of the estuary survey and the overall Penobscot River monitoring efforts. We thank the various staff and interns at the NOAA Northeast Fisheries Science Center Atlantic Salmon Ecosystems Research Team for support in conducting acoustic surveys. We especially thank John Kocik for continued support and guidance of this research program. Special thanks to Gayle Zydlewski and Stephanie Wood for their comments on previous versions of this manuscript, as well as to Lisa Sette and Stephanie Wood for helping to develop our seal survey method. Finally, we thank two reviewers for their input, particularly in regards to shaping the statistical analyses presented in this final manuscript.
Conflict of interest
The authors declare that the research was conducted in the absence of any commercial or financial relationships that could be construed as a potential conflict of interest.
Publisher’s note
All claims expressed in this article are solely those of the authors and do not necessarily represent those of their affiliated organizations, or those of the publisher, the editors and the reviewers. Any product that may be evaluated in this article, or claim that may be made by its manufacturer, is not guaranteed or endorsed by the publisher.
Supplementary material
The Supplementary Material for this article can be found online at: https://www.frontiersin.org/articles/10.3389/fcosc.2024.1408982/full#supplementary-material
References
Ampela K., Jefferson T. A., Smultea M. A. (2021). Estimation of in-water density and abundance of harbor seals. J. Wildlife Manage. 85, 706–712. doi: 10.1002/jwmg.22019
Bartoń K. (2024). MuMIn: Multi-Model Inference. R package version 1.48.4. Available at: https://CRAN.R-project.org/package=MuMIn.
Berejikian B. A., Moore M. E., Jeffries S. J. (2016). Predator-prey interactions between harbor seals and migrating steelhead trout smolts revealed by acoustic telemetry. Mar. Ecol. Prog. Ser. 543, 21–35. doi: 10.3354/meps11579
Bernier M. (2017). Penobscot River Habitat Focus Area 2016 Annual Report. Marine Sea Grant Publications. 145. Available at: https://digitalcommons.library.umaine.edu/seagrant_pub/145.
Boness D. J., Bowen W. D., Oftedall O. T. (1994). Evidence of a maternal foraging cycle resembling that of otariid seals in a small phocid, the harbor seal. Behav. Ecol. Sociobiology 34, 95–104. doi: 10.1007/BF00164180
Bowen W. D., Harrison G. D. (1996). Comparison of harbour seal diets in two inshore habitats of Atlantic Canada. Can. J. Zoology 74, 125–135. doi: 10.1139/z96-017
Bowen W. D., Iverson S. J., Boness D. J., Oftedal O. T. (2001). Foraging effort, food intake and lactation performance depend on maternal mass in a small phocid seal. Funct. Ecol. 15, 325–334. doi: 10.1046/j.1365-2435.2001.00530.x
Brown R. F., Mate B. R. (1983). Abundance, movements, and feeding habits of harbor seals, Phoca vitulina, at Netarts and Tillamook Bays, Oregon. Fishery Bull. 81, 291–301.
Bruchs C., Simpson M., Valliere J. (2018). Atlantic Salmon Trap Operating and Fish-Handling Protocols. Bangor, Maine: Maine Department of Marine Resources Policy Number A-99-15.
Cammen K. M., Rasher D. B., Steneck R. S. (2019). Predator recovery, shifting baselines, and the management challenges they create. Ecosphere 10, e02579. doi: 10.1002/ecs2.2579
Chouinard G. A., Swain D. P., Hammil M. O., Poirier G. A. (2005). “Covariation between grey seal (Halichoerus grypus) abundance and natural mortality of cod (Gadus morhua) in the southern Gulf of St. Lawrence,” in Canadian Journal of Fisheries and Aquatic Sciences, vol. 62. (NRC Research Press, Ottawa, Canada), 1991–2000. doi: 10.1139/f05-107
Conwell H. C., Lewis Z. K., Thomas A., Acevedo-Gutierrez A., Schwarz D. (2024). Sex-specific diet differences in harbor seals (Phoca vitulina) via spatial assortment. Ecol. Evol. 14, e11417. doi: 10.1002/ece3.11417
Cunningham L., Baxter J. M., Boyd I. L., Duck C. D., Lonergan M., Moss S. E., et al. (2008). Harbour seal movements and haul-out patterns: implications for monitoring and management. Aquat. Conservation: Mar. Freshw. Ecosyst. 19, 398–407. doi: 10.1002/aqc.983
Falkegård M., Lennox R. J., Thorstad E. B., Einum S., Fiske P., Garmo O. A., et al. (2023). Predation of Atlantic salmon across ontogenetic stages and impacts on populations. Can. J. Fisheries Aquat. Sci. 80, 1696–1713. doi: 10.1139/cjfas-2023-0029
Free C. M., Jensen O. P., Hilborn R. (2021). Evaluating impacts of forage fish abundance on marine predators. Conserv. Biol. 35, 1540–1551. doi: 10.1111/cobi.13709
Gardner C., Coghlan S. M. Jr., Zydlewki J., Saunders R. (2013). Distribution and abundance of stream fishes in relation to barriers: Implications for monitoring stream recovery after barrier removal. River Res. Appl. 29, 65–78. doi: 10.1002/rra.1572
Gause G. F. (1935). Experimental demonstration of Volterra’s periodic oscillations in the numbers of animals. J. Exp. Biol. 12, 44–48. doi: 10.1242/jeb.12.1.44
Gilbert J. R., Waring G. T., Wynne K. M., Guldager N. (2005). Changes in abundance of harbor seals in Maine 1981-2001. Mar. Mammal Sci. 21, 519–535. doi: 10.1111/j.1748-7692.2005.tb01246.x
Hammill M. O., Stenson G. B., Swain D. P., Benoît H. P. (2014). Feeding by grey seals on endangered stocks of Atlantic cod and white hake. ICES J. Mar. Sci. 71, 1332–1341. doi: 10.1093/icesjms/fsu123
Hartig F. (2022). DHARMa: Residual Diagnostics for Hierarchical (Multi-Level / Mixed) Regression Models. R package version 0.4. Available at: http://florianhartig.github.io/DHARMa/.
Hayes S. A., Costa D. P., Harvey J. T., le Boeuf B. J. (2006). Aquatic mating strategies of the male Pacific harbor seal (Phoca vitulina richardii): Are males defending the hotspot? Mar. Mammal Sci. 20, 639–656. doi: 10.1111/j.1748-7692.2004.tb01184.x
Hayes S. A., Josephson E., Maze-Foley K., Rosel P. E., Wallace J., Brossard A., et al. (2022). “US Atlantic and Gulf of Mexico marine mammal stock assessments 2021,” in NOAA Technical Memorandum NMFS-NE-288, Woods Hole, Massachusetts.
Herr H., Scheidat M., Lehnert K., Siebert U. (2009). Seals at sea: Modelling seal distribution in the German bight based on aerial survey data. Mar. Biol. 156, 811–820. doi: 10.1007/s00227-008-1105-x
Hilborn R., Amoroso R. O., Bogazzi E., Jensen O. P., Parma A. M., Szuwalski C., et al. (2017). When does fishing forage species affect their predators. Fisheries Res. 191, 211–221. doi: 10.1016/j.fishres.2017.01.008
Hill S. L., Hinke J., Bertrand S., Fritz L., Furness R. W., Ianelli J. N., et al. (2020). Reference points for predators will progress ecosystem-based management of fisheries. Fish Fisheries 21, 368–278. doi: 10.1111/faf.12434
Jackman S. (2024). pscl: Classes and Methods for R Developed in the Political Science Computational Laboratory (Sydney, Australia: University of Sydney). Available at: https://github.com/atahk/pscl/. R package version 1.5.9.
Kusnierz P. C., Trial J. G., Cox O. N., Saunders R. (2014). Seal-induced injuries on adult Atlantic salmon returning to Maine. Mar. Coast. Fisheries: Dynamics Management Ecosystem Sci. 6, 119–126. doi: 10.1080/19425120.2014.893466
Lance M. M., Chang W.-Y., Jeffires S. J., Pearson S. F., Acevedo-Gutierrez A. (2012). Harbor seal diet in northern Puget Sound: Implications for the recovery of depressed fish stocks. Mar. Ecol. Prog. Ser. 464, 257–271. doi: 10.3354/meps09880
Leach L., Simpson M., Stevens J. R., Cammen K. (2022). Examining the impacts of pinnipeds on Atlantic salmon: The effects of river restoration on predator-prey interactions. Aquat. Conservation: Mar. Freshw. Ecosyst. 32, 645–657. doi: 10.1002/aqc.3783
Lesage V., Hammill M. O. (2001). The status of the grey seal, Halichoerus grypus, in the Northwest Atlantic. Can. Field Nat. 115, 653–662. doi: 10.5962/p.363863
Li L., Ainsworth C., Pitcher T. (2010). Presence of harbour seals (Phoca vitulina) may increase exploitable fish biomass in the Strait of Georgia. Prog. Oceanography 87, 235–241. doi: 10.1016/j.pocean.2010.09.006
Lipsky C. A., Saunders R., Stevens J. (2019). Developing Sampling Strategies to Assess the Penobscot River Estuary, (2010-2013) (Orono, Maine: Northeast Fisheries Science Center Reference Document 19-02).
Lowry L. F., Frost K. J., Ver Hoep J. M., Delong R. A. (2001). Movements of satellite-tagged subadult and adult harbor seals in Prince William Sound, Alaska. Marine Mammal Sci. 17, 835–861. doi: 10.1111/j.1748-7692.2001.tb01301.x
Lüdecke D., Ben-Shachar M., Patil I., Waggoner P., Makowski D. (2021). performance: an R package for assessment, comparison and testing of statistical models. J. Open Source Software 6, 3139. doi: 10.21105/joss.03139
Marshall K. N., Stier A. C., Samhouri J. F., Kelly R. P., Ward E. J. (2016). Conservation challenges of predator recovery. Conserv. Lett. 9, 70–78. doi: 10.1111/conl.12186
McCormick S. D., Hansen L. P., Quinn T. P., Saunders R. L. (1998). Movement, migration, and smolting of Atlantic salmon (Salmo salar). Can J Fish Aquat Sci. 55, 77–92. doi: 10.1139/d98-011
McHuron E. A., Sterling J. T., Mangel M. (2023). The influence of prey availability on behavioral decisions and reproductive success of a central-place forager during lactation. J. Theor. Biol. 560, 111293. doi: 10.1016/j.jtbi.2022.111392
MDMR. (2018). Historical Trap Counts. Available online at: https://www.maine.gov/dmr/sites/maine.gov.dmr/files/docs/trapcounts2021.pdf (Accessed 12 April 2020).
MDMR. (2019). Sea-Run Fish - River Trap Counts. Available online at: https://www.maine.gov/dmr/science-research/searun/programs/trapcounts.html (Accessed 12 April 2020).
Moore M. E., Malick M. J., Thomas A. C., Klungle M. M., Berejikian B. A. (2024). Harbor seal predation on migrating steelhead smolts entering marine waters. Mar. Ecol. Prog. Ser. 743, 139–157. doi: 10.3354/meps14639
Murray M. (2008). Behavioral interactions between harbor seals (Phoca vitulina) and gray seals (Halichoerus grypus) on Cape Cod, Massachusetts (Boston, Massachusetts: Northeastern University Digital Repository Service). doi: 10.17760/d10016624
Nelson B. W., McAllister M. K., Trites A. W., Thomas A. C., Walters C. J. (2023). Quantifying impacts of harbor seal Phoca vitulina predation on juvenile Coho Salmon in the Strait of Georgia, British Columbia. Mar. Coast. Fisheries: Dynamics Management Ecosystem Sci. 16, e10271. doi: 10.1002/mcf2.10271
NRCM. (2019). Penobscot River Restoration Project: Preserving Maine’s Waterways. Available online at: https://www.nrcm.org/projects/waters/penobscot-river-restoration-project/ (Accessed 19 October 2019).
O’Malley M. B., Saunders R., Stevens J. R., Jech J. M., Sheehan T. F. (2017). Using hydroacoustics to describe pelagic fish distribution in the Penobscot Estuary, Maine. Trans. Am. Fisheries Soc. 146, 817–833. doi: 10.1080/00028487.2017.1308883
Okey T. B., Wright B. A. (2004). Toward ecosystem-based extraction policy for Prince William Sound, Alaska: Integrating conflicting objectives and rebuilding pinnipeds. Bull. Mar. Sci. 74, 727–747.
Ouellet V., Collins M. J., Kocik J. F., Saunders R., Sheehan T. F., Ogburn M. B., et al. (2022). The diadromous watersheds-ocean continuum: Managing diadromous fish as a community for ecosystem resilience. Front. Ecol. Evol. 10. doi: 10.3389/fevo.2022.1007599
Pace R. M., Josephson E., Wood S. A., Murray K., Waring G. (2019). Trends and Patterns of Seal Abundance at Haul-out Sites in a Gray Seal Recolonization Zone (Woods Hole, Massachusetts: NOAA Technical Memorandum NMFS-NE-251).
Pringle R. M., Hutchinson M. C. (2020). Resolving food-web structure. Annu. Rev. Ecology Evolution Systematics 51, 55–80. doi: 10.1146/annurev-ecolsys-110218-024908
Raposa K. B., Dapp R. M. (2009). “A protocol for long-term monitoring of harbor seals (Phoca vitulina concolor) in Narragansett Bay, Rhode Island,”. Narragansett Bay Research Reserve Technical Report Series 2009, vol. 2. doi: 10.13140/RG.2.1.3193.8003
Roman J., Altman I., Dunphy-Daly M. M., Campbell C., Jasny M., Read A. J. (2013). The Marine Mammal Protection Act at 40: Status, recovery, and future of U.S. marine mammals. Ann. New York Acad. Sci. 1286, 29–49. doi: 10.1111/nyas.12040
Russell D. J. F., McClintock B. T., Matthiopoulos J., Thompson P. M., Thompson D., Hammond P. S., et al. (2015). Intrinsic and extrinsic drivers of activity budgets in sympatric grey and harbour seals. Oikos 124, 1462–1472. doi: 10.1111/oik.01810
Saunders R., Hachey M. A., Fay C. W. (2006). Maine’s diadromous fish community: Past, present, and implications for Atlantic salmon recovery. Fisheries 31, 537–547. doi: 10.1577/1548-8446(2006)31[537:MDFC]2.0.CO;2
Scherelis C., Zydlewski G. B., Brady D. C. (2019). Using hydroacoustics to relate fluctuations in fish abundance to river restoration efforts and environmental conditions in the Penobscot River, Maine. River Res Appl. 36 (2), 234–246. doi: 10.1002/rra.3560
Schielzeth H. (2010). Simple means to improve the interpretability of regression coefficients. Methods Ecol. Evol. 1, 103–113. doi: 10.1111/j.2041-210X.2010.00012.x
Schneider D. C., Payne P. M. (1983). Factors affecting haul-out of harbor seals at a site in southeastern Massachusetts. J. Mammalogy 64, 518–520. doi: 10.2307/1380370
Schwarz D., Spitzer S. M., Thomas A. C., Kohnert C. M., Keates T. R., Acevedo-Gutierrez A. (2018). Large-scale molecular diet analysis in a generalist marine mammal reveals male preference for prey of conservation concern. Ecol. Evol. 8, 9889–9905. doi: 10.1002/ece3.4474
Sette L., Accardo C. M., McKenna K., Patchett K., Rose K., Sharp B. W., et al. (2020). The seasonal diet, distribution, and counts of harbor seals (Phoca vitulina vitulina) and gray seals (Halichoerus grypus atlantica) in Pleasant Bay and Chatham Harbor, Cape Cod, Massachusetts. Northeastern Nat. 27, 98–113. doi: 10.1656/045.027.s1011
Sharples R. J., Moss S. E., Patterson T. A., Hammond P. S. (2012). Spatial variation in foraging behaviour of a marine top predator (Phoca vitulina) determined by a large-scale satellite tagging program. PloS One 7, 1–14. doi: 10.1371/journal.pone.0037216
Short J. W., Geiger H. J., Fritz L. W., Warrenchuk J. J. (2021). First-year survival of Northern fur seals (Callorhinus ursinus) can be explained by pollock (Gadus chalcogrammus) catches in the Eastern Bering Sea. J. Mar. Sci. Eng. 9, 975. doi: 10.3390/jmse9090975
Sigourney D. B., Murray K. T., Gilbert J. R., Ver Hoef J. M., Josephson E., DiGiovanni J. R.A. (2021). Application of a Bayesian hierarchical model to estimate trends in Atlantic harbor seal (Phoca vitulina vitulina) abundance in Maine, U.S.A. 1993-2018. Mar. Mammal Sci. 38, 500–516. doi: 10.1111/mms.12873
Stevens J. R., Jech J. M., Zydlewski G. B., Brady D. C. (2023). Response of estuarine fish biomass to restoration in the Penobscot River, Maine. Estuaries Coasts 47, 535–550. doi: 10.1007/s12237-023-01292-w
Stich D. S., Zydlewski G. B., Kocik J. F., Zydlewski J. D. (2015). Linking behavior, physiology, and survival of Atlantic Salmon smolts during estuary migration. Mar Coast Fish. 7, 68–86. doi: 10.1080/19425120.2015.1007185
Stobo W. T., Fowler G. M. (1994). “Aerial surveys of seals in the Bay of Fundy and off Southwest Nova Scotia,” in Canadian Technical Report of Fisheries and Aquatic Sciences No. 1943 (Dartmouth, Nova Scotia: Department of Fisheries and Oceans Biological Sciences Branch).
Thomas A. C., Lance M. M., Jeffries S. J., Miner B. G., Acevedo-Gutierrez A. (2011). Harbor seal foraging response to a seasonal resource pulse, spawning Pacific herring. Mar. Ecol. Prog. Ser. 441, 225–239. doi: 10.3354/meps09370
Thorley J., Miller L., Fleishman A. (2018). rtide: Tide Heights. R package version 0.0.5. Available online at: https://github.com/poissonconsulting/rtide (Accessed June 19, 2019).
Townsend H., Harvey C. J., deReynier Y., Davis D., Zador S. G., Gaichas S., et al. (2019). Progress on implementing ecosystem-based fisheries management in the United States through the use of ecosystem models and analysis. Front. Mar. Sci. 6. doi: 10.3389/fmars.2019.00641
Trzcinski M. K., Majewski S., Nordstrom C. A., Schulze A. D., Miller K. M., Tucker S. (2024). DNA analysis of scats reveals spatial and temporal structure in the diversity of harbour seal diet from local haulouts to oceanographic bioregions. Mar. Ecol. Prog. Ser. 743, 113–138. doi: 10.3354/meps14655
Vander Zanden M. J., Olden J. D., Gratton C. (2006). “Food-web approaches in restoration ecology,” in Foundations of Restoration Ecology. Eds. Falk D. A., Palmer M., Zedler J. (Island Press, Washington, DC, USA), 165–189. doi: 10.1139/cjfas-2023-0029
van Neer A., Jensen L. F., Siebert U. (2015). Grey seal (Halichoerus grypus) predation on harbour seals (Phoca vitulina) on the island of Helgoland, Germany. J. Sea Res. 97, 1–4. doi: 10.1016/j.seares.2014.11.006
Venables W. N., Ripley B. D. (2002). Modern Applied Statistics with S. 4th ed. (New York: Springer). Available at: https://www.stats.ox.ac.uk/pub/MASS4/, ISBN: ISBN 0-387-95457-0.
Vincent C., Huon M., Caurant F., Dabin W., Deniau A., Dixneuf S., et al. (2017). Grey and harbour seals in France: Distribution at sea, connectivity and trends in abundance at haulout sites. Deep Sea Res. Part II: Topical Stud. Oceanography 141, 294–305. doi: 10.1016/j.dsr2.2017.04.004
Waring G. T., DiGiovanni R. A. Jr., Josephson E., Wood S., Gilbert J. R. (2015). 2012 Population Estimate for the Harbor Seal (Phoca vitulina concolor) in New England Waters (Woods Hole, Massachusetts: NOAA Technical Memorandum NMFS-NE-235). doi: 10.7289/V5ZC80VT
Watts P. (1996). The diel hauling-out cycle of harbour seals in an open marine environment : correlates and constraints. Zoological Soc. London 240, 175–200. doi: 10.1111/j.1469-7998.1996.tb05494.x
Wells B. K., Huff D. D., Burke B. J., Brodeur R. D., Santora J. A., Field J. C., et al. (2020). Implementing ecosystem-based management principles in the design of a salmon ocean ecology program. Front. Mar. Sci. 7. doi: 10.3389/fmars.2020.00342
Westphal L., Klemens L., Reif F., van Neer A., Dähne M. (2023). First evidence of grey seal predation on marine mammals in the German Baltic Sea. J. Sea Res. 192, 102350. doi: 10.1016/j.seares.2023.102350
Wood S. A., Josephson E., Precoda K., Murray K. T. (2022). Gray seal (Halichoerus grypus) pupping trends and 2021 population estimate in U.S. waters (Woods Hole, Massachusetts: Northeast Fisheries Science Center Reference Document 22-14).
Yochem P. K., Stewart B. S., DeLong R. L., DeMaster D. P. (1987). Diel haul-out patterns and site fidelity of harbor seals (Phoca vitulina richardsi) on San Miguel Island, California in autumn. Mar. Mammal Sci. 3, 323–332. doi: 10.1111/j.1748-7692.1987.tb00319.x
Keywords: fish biomass, gray seal, harbor seal, predator-prey interactions, protected species, recovery, river restoration
Citation: Leach L, Stevens JR and Cammen K (2024) Pinniped response to diadromous fish restoration in the Penobscot River Estuary. Front. Conserv. Sci. 5:1408982. doi: 10.3389/fcosc.2024.1408982
Received: 29 March 2024; Accepted: 29 November 2024;
Published: 18 December 2024.
Edited by:
Megan Feddern, NOAA Fisheries, United StatesReviewed by:
Alejandro Acevedo-Gutierrez, Western Washington University, United StatesElizabeth Allyn, Makah Tribal Council, United States
Brusa Jamie, Integrated Statistics, United States
Copyright © 2024 Leach, Stevens and Cammen. This is an open-access article distributed under the terms of the Creative Commons Attribution License (CC BY). The use, distribution or reproduction in other forums is permitted, provided the original author(s) and the copyright owner(s) are credited and that the original publication in this journal is cited, in accordance with accepted academic practice. No use, distribution or reproduction is permitted which does not comply with these terms.
*Correspondence: Lauri Leach, bGF1cmlsZWFjaEBnbWFpbC5jb20=