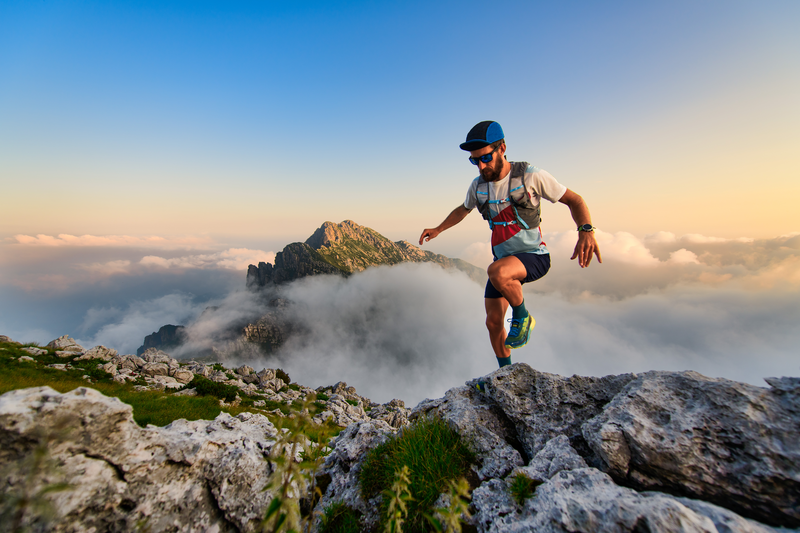
94% of researchers rate our articles as excellent or good
Learn more about the work of our research integrity team to safeguard the quality of each article we publish.
Find out more
REVIEW article
Front. Conserv. Sci. , 27 September 2024
Sec. Plant Conservation
Volume 5 - 2024 | https://doi.org/10.3389/fcosc.2024.1383370
Climate change and biodiversity conservation are two of the most urgent challenges of the twenty-first century. Current global climate models indicate that climate-related events will continue to increase in frequency and intensity, leading to severe impacts on ecosystems, particularly plant diversity. Despite the 2015 Paris Agreement, efforts to limit global warming to 1.5 degrees Celsius and secure adequate climate financing remain unsatisfactory. In addition, the National Biodiversity Strategic Action Plans (NBSAPs), a core mechanism of the Convention on Biological Diversity (CBD), have faced issues in implementation at the national level. Many NBSAPs lack clear and measurable biodiversity targets, which limits their effectiveness. This review presents a comprehensive analysis of these urgent issues, highlighting the significant challenges and deficiencies in current climate and biodiversity conservation policies. It evaluates the effectiveness of the Kew conservation strategy as a model for protecting and conserving plant diversity. Furthermore, this review underscores the pivotal role of plant tissue culture (PTC) technology in achieving plant conservation targets within the post-2020 global biodiversity framework. This review strongly supports the post-2020 global biodiversity framework and the integration of PTC into global plant conservation strategies to meet the ambitious 30-by-30 targets. This review also advocates for the establishment of the Kew-Wide Mechanism (KWM) to bolster climate resilience, reduce anthropogenic impacts on plant diversity, revitalize global conservation efforts, and accelerate ecosystem restoration in the face of ongoing climate change. Proposed as a comprehensive approach to plant conservation, the KWM offers a strategic, innovative, and scalable solution as the global economy transitions toward decarbonization.
Climate change and the loss of plant diversity are two major challenges in the modern era. Climate change is a primary factor contributing to the decline in plant diversity, along with human activities. Global climate forecasts indicate that the frequency and intensity of climatic phenomena will escalate over time. This includes an anticipated rise in heat waves, droughts, unpredictable wildfires, severe rainfall, and floods (Crowley, 2000; Alley et al., 2007; Alfonso et al., 2021; Tebaldi et al., 2021; Zandalinas et al., 2021). These climate-related occurrences are exerting an adverse influence on human society and livelihoods, as well as natural ecosystems globally (Bergholt and Lujala, 2012; Gray and Mueller, 2012; Vermeulen et al., 2012; McMichael, 2013; Fang et al., 2019; Schleuning et al., 2020).
Biodiversity plays a critical role in sustaining different life forms on Earth, including humans, and without it, humans cannot maintain the healthy ecosystems on which they rely to provide ecological services such as clean air, food, medicine, and natural aesthetics. Extinction is an inherent occurrence in the Earth’s historical timeline. Nevertheless, the present rates of extinction are projected to be at least 1000 times higher than the average rate in the past, which gives rise to apprehensions regarding the potential occurrence of a sixth mass extinction event before the conclusion of this century (Pimm et al., 2014; Proença and Pereira, 2017; Grusin, 2018; Jepson, 2019; Romshoo et al., 2020). Natural disturbances such as seasonal changes that cause plant and invertebrate populations to rise or fall, wildfires, floods, and volcanic eruptions, as well as anthropogenic disturbances and climate change, are among the leading causes of biodiversity loss (Powers and Jetz, 2019; Ritchie and Roser, 2021). Furthermore, overexploitation of wildlife for wood, food, medicine, commercial, ornamental, and cultural purposes threatens and leads to the extinction of many species (Thuiller et al., 2019; de Souza and Prevedello, 2020; Caro et al., 2022). Climate events have different effects on individual organisms and entire ecosystems. Several studies indicate a significant decrease in the population of bryophyte species in forests due to increased fire and drought occurrences. Additionally, the loss of soil microbial diversity has disrupted the crucial soil microbial structures necessary for plant growth (Dubey et al., 2019; Viles and Cutler, 2012; Rillig et al., 2019; Pereira et al., 2021).
The 2015 Paris Agreement represents a significant turning point in the worldwide endeavor to address climate change. However, there are intricate and diverse challenges that need to be overcome, such as the difficulty of restricting global warming to a maximum of 1.5 degrees Celsius above pre-industrial levels, as well as the issue of financing. These obstacles have persisted for more than eight years and multiple Conference of Parties (COP) meetings (Kuyper and Schroeder, 2018). The National Biodiversity Strategies and Action Plans (NBSAPs) of the Convention on Biological Diversity (CBD) serve as crucial tools for integrating biodiversity and executing conservation strategies on a national scale (Sarkki et al., 2016). However, implemented measures in most countries and regions have not been effective enough to halt the loss of original biological diversity. Many habitats are still degraded, and unless more effective and far-reaching measures are implemented, more species will become endangered or extinct in the near future (Auvinen et al., 2007). Furthermore, most national NBSAPs lack explicit biodiversity goals, resulting in limited contributions to biodiversity conservation (Wu et al., 2019; Tong, 2020).
Given all of these challenges, the only viable option is to employ a variety of strategies. For example, the CBD is currently working on the adoption of the post-2020 global biodiversity framework, a new plan to protect biological diversity. Annual Conference of Parties (COP) meetings, on the other hand, are held to devise countermeasures to climate change. Additionally, massive investment in research and development to create innovative green technologies that are dependable, accessible, and can be implemented regardless of geographical constraints is another option that is being pursued. However, it is impossible to predict when such game-changing technologies will be available, as well as their global accessibility, including cost and scalability.
By 2030, the post-2020 global biodiversity conservation framework mandates that protected areas and other effective area-based conservation measures must cover a minimum of 30% of the Earth's surface. This worldwide initiative, known as “30-by-30,” is a global campaign with specific targets that aims to designate 30% of Earth’s land and marine areas as protected areas by 2030 (Box 1). This review wholeheartedly endorses this bold endeavor, as it encompasses a highly ambitious strategy to preserve natural ecosystems. Additionally, it serves as a protective measure to minimize the impact of climate change and expedite the attainment of goals related to biodiversity conservation and climate before the year 2050. This review equally focuses on how, despite recent advances in plant tissue culture technology, it is potentially underutilized in plant conservation efforts, thus, advocating for its unabated implementation to achieve the 30-by-30 terrestrial biome targets for plant diversity protection and conservation (Figure 1, Box 1).
The objective of this review is to underscore the persistent difficulties faced by climate change and biodiversity conservation policies and to stress the necessity of a globally coordinated Kew-Wide Mechanism (KWM) that is executed at the country level (Figure 2). The conservation of biodiversity confronts a myriad of challenges, driven by anthropogenic activities such as unsustainable land use, deforestation, and illicit wildlife trade, exacerbated by the impacts of climate change. Without the adoption of the 30-by-30 framework, these challenges are expected to rapidly worsen, rendering traditional biodiversity conservation approaches increasingly ineffective. This rapid escalation could potentially make existing conventional conservation methods obsolete, highlighting the critical need for innovative approaches to preserving the Earth's flora, among other natural resources. Plant tissue culture technology emerges as a promising solution for plant conservation, yet its global application remains limited. This study introduces the KWM architecture, which integrates plant tissue culture technology as a pivotal conservation tool to address the plant-related objectives of the 30-by-30 post-2020 global biodiversity conservation agenda. By incorporating plant tissue culture technology, the KWM architecture represents a pioneering global plant conservation policy framework, offering a novel approach to safeguarding global plant diversity amidst 21st-century challenges and presenting a viable alternative to conventional conservation strategies.
The literature review process for this study began in 2022 and concluded in 2024. We conducted a literature review using keywords from the Google Scholar database to identify potential studies. Google Scholar is an openly accessible web search engine that catalogs the complete text of academic literature from various publishing formats and fields of study. A wider and more varied selection of publications, including published articles, preprints, theses, books, and court opinions, forms the basis of Google Scholar counts. In addition, Google Scholar offers a broader selection of resources for non-journal content, including PDF files, Word documents, technical reports, theses, and dissertations.
Google Scholar is quite similar to Google in that it automatically places AND between words, quotes, sentences, or titles and searches for alternative terms using OR, with the terms contained in parenthesis. We generated the search string using the Google Scholar filter tool, which resulted in a search outcome that reflected the diverse nature of the database compared to other platforms. On Google Scholar, we used an approach that involved running multiple searches and modifying our keywords with each search. We also employed a citation mining method, also known as ‘Pearl Growing’ or ‘Snowballing’, to conduct an opportunistic search of relevant literature. Citation mining is an efficient method of looking for relevant articles. Stage one involves identifying an article that meets the inclusion criteria. During the second stage, more pertinent articles are identified through the examination of reference lists. We achieved this by conducting a comprehensive search in both the ‘retrospective’ direction, which involves examining the papers referenced by the article of interest, and the ‘prospective’ direction, which involves identifying any papers that have cited the article of interest. We kept repeating this process until we couldn't find any more relevant articles. This approach combines reference tracking and citation tracking. In this review, we used ‘citation mining’ as a supplemental approach to further extend the literature search (Wohlin, 2016; Mourão et al., 2020; Wohlin et al., 2022). This is because reviews are more likely to be relevant to a wide range of end users if they draw from a diverse range of experiences in terms of both the subject matter and the approach used (Lasserson et al., 2019).
Given the broad theme, scope, and issues addressed in this review study, we ultimately utilized a mixed method to search the literature. In the first phase, we specified our study scope: climate change, mitigation, and problems; biodiversity, specifically plant diversity; global and regional conservation policies; and challenges encountered. We also focused on the advancements and potential applications of plant tissue culture technology. Secondly, we defined our search criteria and used both the basic and advanced filters of Google Scholar to find publications that featured these terms throughout the entire text, published within the last eight years, except for a few exceptions (Figures 3A, B). In summary, our search process combined the aforementioned concepts with appropriate synonyms. We produced 50 to 100 or more articles, depending on the search field, and then conducted a thorough screening to find the best match that aligned with the main idea and purpose of our review content. Thirdly, we limited our search to at least 85% peer-reviewed articles, with the remaining 15% coming from institutional websites, web-based searches, and non-peer-reviewed articles like conference papers, technical reports, theses, and even proceedings that were pertinent to and consistent with the main themes of this review. When necessary, we periodically conducted supplementary literature searches through online databases and relevant organizational websites to ensure a comprehensive review of the existing research (Briscoe, 2018). After screening article titles and abstracts to identify those that addressed the selected themes, we thoroughly read the chosen papers. We ultimately selected about 325 articles and works, using more than 180 of them as references in this review article.
Figure 3. (A) Flow chart illustrating the process of searching and incorporating the literature (1952–2024) into this review article. (B) The literature review includes publications from 1952 to 2024, with the majority published between 2018 and 2022. This suggests that the most recent scholarship and research in the field are being considered. It’s worth noting that many of the cited publications are from 2020, indicating a focus on relevant research during that period.
Fourth, we read the roughly 325 documents that made it past the initial screening and categorized them according to the research topics listed below: “biodiversity loss” (56 articles), “biodiversity conservation methods” (15 articles), “CITES” (41 articles), “climate denial” (8 articles), “climate-driven extinction of species” (12 articles), “climate policy” (41 articles), “conservation policy” (20 articles), “extinction” (31 articles), “global warming” (6 articles), “impacts of climate change” (33 articles), “IPCC projections” (9 articles), “Kew Gardens models” (3 articles), “limitations of current conservation approaches” (20 articles), “lower plants and threats to biodiversity” (17 articles), “plant tissue culture and quality planting materials” (27 articles), “soil microbiome” (18 articles), “post-2020 framework for biodiversity conservation” (5 articles), and “role of plant tissue culture” (25 articles) (Figure 3A). One study could be assigned to a single topic area, several subject areas, or none at all. All the studies were in English.
Climate refers to the long-term patterns and averages of weather conditions in a specific area, including temperature, humidity, precipitation, wind, and other atmospheric elements. Typically, scientists measure and average these patterns over a period of 30 years or more. Meanwhile, climate change refers to long-term shifts in temperature, precipitation, wind patterns, and other aspects of the Earth's climate system. The causes of climate change can be categorized into two primary groups: natural causes and man-made causes. The natural causes of climate change have been attributed to an increase in solar irradiance and solar cycle variabilities, and to a lesser extent volcanism, but these have played only a subsidiary role in the 20th-century warming (Crowley, 2000). The warming observed in the 20th century is unparalleled when compared to the previous 1000 years. The majority of this warming may be traced to a significant rise in emissions of greenhouse gases (GHGs) (Crowley, 2000; Alley et al., 2007). The atmospheric concentration of greenhouse gases (GHGs) such as carbon dioxide (CO2), nitrous oxide (N2O), and methane (CH4) has significantly risen since the industrial revolution. This increase is mostly attributed to the excessive use of fossil fuels and changes in land use, which contribute to the rise in CO2 levels. Additionally, agricultural practices are responsible for the higher levels of CH4 and N2O. Observations have shown that the warming has led to several long-term climate changes, including alterations in polar ice and temperatures, precipitation amounts, tropical storms, heat waves, droughts, extreme sea levels, and wind patterns (Tebaldi et al., 2021). The adverse impacts of human-induced warming have also been documented to influence plant and marine life on a worldwide level (Alfonso et al., 2021; Zandalinas et al., 2021).
Between 1901 and 2020, global temperatures rose by approximately 1.2°C (IPCC, 2021) but climate change is more than just about temperature rise, far from that; it is about the current negative impacts felt across the world (Figure 4). Even though climate change affects the entire world, its perceived impacts are uneven, different and vary across the biosphere and human society as a whole. Climate-related natural disasters occur when extreme weather events or natural hazards such as strong winds, tropical storms, heavy rainfall or even exposure to extreme temperatures affect a vulnerable population causing human casualties, significant damage or loss of property and infrastructure (Slettebak, 2012). These extreme weather events are very likely to keep increasing in frequency with the exacerbation of global warming (Nordhaus, 2010; Mendelsohn et al., 2012; Banholzer et al., 2014).
Figure 4. Some global climate change impacts (green impacts directly affecting the environment, and orange impacts directly affecting human society). We categorize the weightage of these impacts on a scale from (0) representing ‘no effect’ to (3) indicating ‘ very severe’, with (1) denoting ‘less severe’ and (2) representing ‘mildly severe’.
The impacts of climate change on different sectors of human society are interconnected but even so, these impacts are unevenly distributed across geographical regions affecting those that are least prepared (Hobbie and Grimm, 2020; Stuart Chapin and Díaz, 2020). For instance, multiple studies demonstrate a robust association between climatic change and the proliferation of diseases (Mills et al., 2010; Singh et al., 2011; Parkinson et al., 2014); floods cause damage to ecosystems, livelihoods and infrastructure (Idowu et al., 2011; Gray and Mueller, 2012); droughts cause low agricultural productivity leading to massive food shortages and severe famine in the worst case scenario (Vermeulen et al., 2012; Coulibaly et al., 2020); all of which have dire consequences on human health and well-being (McMichael, 2013; Wu et al., 2016). The effects of climate change on human cultural heritage monuments and sites have also been well documented (Sesana et al., 2021). The rise in global temperatures has led to a shift in the balance between political, economic, security and social factors; the outcome of which is an increase in the frequency of climate-related conflicts all over the world (Bergholt and Lujala, 2012; Scheffran et al., 2012; Slettebak, 2012).
A multitude of studies unequivocally affirm the concept that climate change is a substantial catalyst for the depletion of biodiversity (Alexander et al., 2018; Attorre et al., 2018; García-Valdés et al., 2018; Suggitt et al., 2018; Foden et al., 2019; Silva et al., 2019; Román-Palacios and Wiens, 2020; Weiskopf et al., 2020). Another study demonstrates that climate change significantly influences the reorganization of ecological communities and interactions between different species, often resulting in a detrimental impact on biodiversity (Schleuning et al., 2020). Species exhibit responses to climate change through modifications in their physical characteristics and behavior, as well as alterations in their seasonal patterns and geographic distribution (Box 2). Both evolutionary changes that take place over generations and plasticity, which refers to the capacity to adapt within a person’s lifetime, have an impact on these responses. The reactions of species and populations, along with the direct effects of climate change on ecosystems, result in extensive alterations in productivity, species interactions, susceptibility to biological invasion, and other new issues (Box 2). Collectively, these effects alter the advantages and provisions that natural ecosystems can offer to society (Nogués-Bravo et al., 2018; Nadeau and Urban, 2019; Weiskopf et al., 2020).
The Intergovernmental Panel on Climate Change (IPCC) was created in the 1980s by the United Nations and the World Meteorological Organization (WMO) to address growing environmental issues, specifically climate change. Subsequently, the 1992 “Earth Summit,” or United Nations Conference on Environment and Development (UNCED), took place in Rio de Janeiro. This progression continued with the formation of the United Nations Framework Convention on Climate Change (UNFCCC) in 1994 and the adoption of the Kyoto Protocol to the UNFCCC in 1997 (Clarke, 2008; Seo, 2017). Article 2 of the UNFCCC states that “the primary goal of the convention is to achieve a stable level of greenhouse gas concentrations in the atmosphere, thereby preventing any harmful human-induced influence on the climate system. An adequate degree of achievement should be reached within a certain timeframe to facilitate the natural adaptation of ecosystems to climate change, safeguard food production, and promote sustainable economic development.” (UNFCCC, 1992).
Overall, the UNFCCC Convention has experienced several changes since its establishment in 1994. Initially, it shifted from legally obligating industrialized nations to reduce emissions under the Kyoto Protocol to encouraging voluntary contributions from both developed and developing countries through Nationally Determined Contributions (NDCs) under the Paris Agreement (Figure 5). Subsequently, there has been a transition from the hierarchical Kyoto framework to a combined hierarchical and participatory Paris framework. Additionally, the scope of the Kyoto Protocol’s efforts to reduce greenhouse gas emissions has expanded to include not only mitigation but also adaptation and financial support, as outlined in the Paris Agreement (Kuyper and Schroeder, 2018). Of all the international meetings and proceedings organized to discuss and implement appropriate measures to curb climate change, the 2015 Paris Agreement is a landmark of the multilateral climate process. This is due to the unprecedented global consensus among nations and non-state actors to fundamentally transform their development trajectories and business strategies to prioritize sustainability and mitigate global warming. The objective is to limit the increase in global temperatures to well below 2°C, preferably 1.5°C, compared to pre-industrial levels (Hale, 2016). To achieve these objectives, it is necessary to decrease global carbon dioxide (CO2) emissions by 45% by 2030 compared to the levels recorded in 2010 (about 390 parts per million) and ultimately achieve a state where there are no net emissions by 2050. The latest report from the United Nations reveals that in 2020, the levels of greenhouse gases in the atmosphere reached record highs. The average concentration of carbon dioxide (CO2) globally exceeded 410 parts per million (ppm). Despite the COVID-19 pandemic, countries have made progress in taking action against climate change. There has been a greater emphasis on adapting to climate change, and developed countries (Annex I parties) have increased their financial support for climate initiatives in developing countries by 10% between 2015–2016 and 2017–2018. The annual average support during 2017–2018 was $48.7 billion (SDG Report, 2021).
Figure 5. (A, B) display the timeline of major conventions (and initiatives) related to climate change and biodiversity respectively. GPPC, Global Partnership for Plant Conservation; GSPC, Global Strategy for Plant Conservation; CBD, Convention on Biological Diversity; CITES, Convention on International Trade in Endangered Species of Wild Fauna and Flora; COP, Conference of Parties; Post-2020 GBCF, Post-2020 Global Biodiversity Conservation Framework.
Recently, the Glasgow Conference of Parties 26 (COP 26) meeting made efforts to increase resilience to climate change, reduce emissions, increase climate financing, declare zero-emission vehicles to promote green and sustainable transportation, and recognize the overexploitation of forest resources. However, an evaluation of the conference’s intended outcomes reveals that they were not met, and the overall roadmap is still uncertain (Arora and Mishra, 2021). At the Sharm el-Sheikh Climate Change Conference (COP 27) and Dubai Climate Change Conference (COP 28), the primary focus was on two crucial topics: preserving the Paris Agreement’s objective of restricting global warming to 1.5°C over pre-industrial levels and the issue of financing and gradually eliminating the use of fossil fuels.
The Intergovernmental Panel on Climate Change (IPCC) is the United Nations organization responsible for evaluating the scientific aspects of climate change. Its purpose is to furnish policymakers with periodic scientific evaluations of climate change. The organization produces comprehensive assessment reports that analyze the current state of scientific, technical, and socio-economic knowledge regarding climate change. These reports also evaluate the impacts and future hazards associated with climate change, as well as potential strategies for mitigating its rate of occurrence. The IPCC produces assessments and special reports through the collaboration of three distinct working groups, each focusing on a specific facet of climate change science: Working Group I (the Physical Science Basis), Working Group II (Impacts, Adaptation, and Vulnerability), and Working Group III (Mitigation of Climate Change). The IPCC additionally maintains a Task Force dedicated to National Greenhouse Gas Inventories, with the primary aim of formulating and enhancing a methodology for the computation and documentation of national greenhouse gas emissions and removals. The Working Groups and Task Force are responsible for overseeing the compilation of reports, including the recruitment and supervision of experts who serve as authors. The Technical Help Units (TSU) help each working group and the task force carry out their activities.
“Various scenarios involving different rates and magnitudes of climate change serve as a foundation for evaluating the likelihood of surpassing discernible thresholds in terms of both physical transformations and the effects on biological and human systems.” The Intergovernmental Panel on Climate Change (IPCC) released the initial climate change forecasts, known as IS92, in 1992. In the year 2000, the IPCC published a set of estimates known as the Special Report on Emissions Scenarios (SRES), which was the second generation of such projections. These assessments, including the Third Assessment Report (TAR) and Assessment Report Four (AR4), have served as significant benchmarks for climate science research during the past decade (Box 3).
Scenarios in climate change research are built to study the probable effects of human-caused climate change by describing plausible trajectories of various parts of the future. Scenarios encompass significant factors influencing climate change policy, such as processes, impacts (physical, ecological, and socioeconomic), and potential solutions (Box 3). They facilitate the transfer of information between different research domains, such as the transition from studying energy systems and greenhouse gas emissions to climate modeling. Additionally, they are utilized to examine the ramifications of climate change on decision-making processes. For instance, they assess the resilience of water management infrastructure development plans to various unpredictable future climate circumstances. The objective of utilizing scenarios is not to forecast the future but rather to enhance comprehension of uncertainties and alternative futures. This allows for the evaluation of the resilience of various actions or options across a broad spectrum of potential futures.
Box 1. A Revised Perspective on the 2030 Action Targets that Pioneer the Core Principles of the Kew-Wide Mechanism to Propel 30-by-30 Biodiversity Conservation Milestones by 2030 and Beyond [Reference: https://www.cbd.int/doc/c/409e/19ae/369752b245f05e88f760aeb3/wg2020-05-l-02-en.pdf]
1. Reducing threats to biodiversity
Target 1
Drawing on comprehensive, participatory, and integrated biodiversity-inclusive spatial planning and/or other effective management processes is imperative to tackle land-use and sea-use change holistically across all areas. It’s essential to prioritize the retention or minimization of the loss of intact ecosystems and areas of significant biodiversity, striving to approach zero loss, with particular attention to ecosystems that pose challenges for restoration. Moreover, efforts must be directed toward enhancing ecological integrity and connectivity while upholding ecosystem functions and services. This approach should be grounded in a commitment to respecting the rights of indigenous peoples and local communities, aligning with the principles outlined in the United Nations Declaration on the Rights of Indigenous Peoples and international human rights law.
Target 2
Strive to restore a substantial portion of degraded terrestrial, inland waters, coastal, and marine ecosystems, aiming for a range of 20–30 percent, or a minimum of 1 billion hectares, while considering their natural state as a baseline reference. This commitment to restoration not only addresses biodiversity loss and ecosystem degradation but also contributes to mitigating climate change, enhancing ecosystem services, and fostering sustainable development.
Target 3
Pledging to safeguard a minimum of 30 percent of the world’s biodiversity on a national scale, with a particular focus on critical biodiversity hotspots, imperiled ecosystems, and other significant regions. This conservation endeavor entails the establishment of meticulously managed, ecologically representative, well-connected, and justly governed networks of fully protected areas, including indigenous territories where applicable. The conservation strategies endeavor to proscribe environmentally detrimental activities and seamlessly integrate protected zones into broader terrestrial and marine landscapes while concurrently upholding the rights of indigenous peoples and local communities.
Target 4
Address the pressing need for species recovery and conservation, particularly focusing on threatened species, with the overarching goal of averting human-induced extinctions and mitigating extinction risks. This entails a multifaceted approach encompassing the maintenance and restoration of genetic diversity within and between populations of native, wild, and domesticated species to safeguard their adaptive capacity. Implementing both in situ and ex situ conservation methods is crucial to achieve these objectives, as it diversifies and tailors conservation efforts to the specific needs of different species and ecosystems. Moreover, effective management of human-wildlife interactions is imperative to mitigate conflicts and foster harmonious coexistence between human populations and wildlife, thereby promoting sustainable conservation outcomes.
Target 5:
Establish a comprehensive framework for ensuring the sustainability, safety, and legality of activities involving the exploitation, harvesting, trading, and utilization of wild species. To monitor these activities, effective regulations and mechanisms for traceability must be implemented. Additionally, there is a clear mandate for eradicating all forms of illegal, unsustainable, or unsafe harvesting, trade, and use of wild species while also proactively preventing overexploitation and minimizing adverse impacts on non-target species and ecosystems through the application of ecosystem-based approaches. Also, the goal makes it clear how important it is to stop and get rid of biopiracy and other illegal activities that involve taking genetic resources and related traditional knowledge without permission. Importantly, it advocates for the respect for and protection of customary sustainable practices exercised by indigenous peoples and local communities, recognizing their invaluable role in biodiversity conservation efforts.
Target 6
To mitigate the adverse effects of invasive alien species on native biodiversity by employing a multifaceted approach. This involves meticulously managing the pathways facilitating their introduction, pre-emptively thwarting the establishment of priority invasive species, slashing the introduction rate of other invasive species by a minimum of 50%, and rigorously pursuing eradication or effective control measures for existing invasive species. This comprehensive strategy not only addresses the immediate threats posed by invasive species but also lays a robust foundation for safeguarding native ecosystems and promoting biodiversity conservation on a global scale.
Target 7
Efforts to mitigate pollution must encompass a holistic approach, targeting emissions and deposits of various pollutants such as light, noise, and plastics. The primary objective is to attain pollution levels that pose no threat to biodiversity, ecosystem functionality, or human well-being, with a keen consideration of cumulative impacts. Central to this endeavor is the substantial reduction of excess nutrient leakage into the environment, aiming for a minimum halving, thereby enhancing nutrient cycling and efficiency of use. Moreover, there is a pressing need to mitigate the risks associated with pesticide and highly hazardous chemical usage, aiming for a reduction of at least two-thirds while carefully balancing considerations for food security and livelihoods. Furthermore, there is a clear imperative to address the menace of plastic pollution comprehensively, encompassing measures to prevent, reduce, and ultimately eliminate plastic waste discharge, including both plastic and electronic waste.
Target 8
A resolute dedication to mitigating the detrimental effects of climate change and ocean acidification on biodiversity, striving to bolster resilience through multifaceted strategies encompassing mitigation, adaptation, and disaster risk reduction. It emphasizes the importance of embracing nature-based solutions and ecosystem-based approaches in order to fortify ecosystems against impending threats. Specifically, the target sets forth an ambitious goal to actively participate in global mitigation endeavors, aiming to curtail emissions by a substantial 10 gigatons of CO2 equivalent annually by the year 2030, rooted in the principles of common but differentiated responsibilities and respective capabilities.
2. Meeting people’s needs through sustainable use and benefit-sharing
Target 9
Promoting the sustainable management and utilization of wild species to generate tangible social, economic, and environmental advantages for diverse populations, with particular consideration given to those in vulnerable circumstances. This comprehensive approach entails advocating for the development and adoption of sustainable biodiversity-based products and services, safeguarding the longstanding customary sustainable practices of indigenous peoples and local communities, and acknowledging the role of sustainable trophy hunting within the broader framework. By emphasizing these facets, the target underscores the imperative to harmonize conservation efforts with the socio-economic needs of communities while safeguarding biodiversity for present and future generations.
Target 10
In the pursuit of sustainable development, this target emphasizes the imperative for a comprehensive approach to the sustainable management of agriculture, aquaculture, fisheries, forestry, and other productive uses, with a primary focus on promoting the sustainable utilization of biodiversity resources. This entails fostering long-term efficiency, productivity, and resilience within production systems by conserving and restoring biodiversity, upholding ecosystem services, and embracing agroecological principles alongside biodiversity-friendly practices. The strategy aims to greatly improve sustainable intensification by using new methods, such as growing crops that can handle changing climates and expanding the use of helpful biotechnology. Moreover, it underscores the necessity of eliminating and gradually phasing out trade-distorting agricultural subsidies while concurrently supporting the establishment of seed banks in developing nations. Furthermore, the initiative advocates for the formulation of sector-specific action plans grounded in agro-ecology and ecosystem approaches, fostering collaboration with smallholder farmers, indigenous food systems, and women. The initiative sets a pivotal target of managing at least 25 percent of agricultural land under agro-ecological or other biodiversity-friendly practices, highlighting the importance of integrating sustainability principles across agricultural landscapes.
Target 11
Nature’s contributions to people should be restored, maintained, and enhanced through nature-based solutions and ecosystem-based approaches. This involves safeguarding ecosystem functions and services, such as air and water regulation, climate stabilization, soil health maintenance, pollination, and protection from natural hazards and disasters. Central to this approach is a focus on “Mother Earth-centric actions,” particularly in regions critical for delivering these services, as well as the incorporation of payment for environmental services to benefit both people and nature.
Target 12
To significantly enhance the area, quality, and connectivity of urban green and blue spaces sustainably by integrating biodiversity conservation and sustainable use into urban planning. This approach promotes native biodiversity and ecological connectivity, improves human health and well-being, fosters a connection to nature, and supports inclusive and sustainable urbanization. It also emphasizes the provision of essential ecosystem functions and services, ensuring that urban development aligns with ecological sustainability and resilience.
Target 13
To foster the fair and equitable sharing of benefits stemming from the utilization of genetic resources. This entails the implementation of robust legal, policy, administrative, and capacity-building measures across all levels of governance. Key components of this approach include ensuring unfettered access to genetic resources, bolstering capacity-building initiatives and developmental efforts, fostering technical and scientific collaboration, facilitating the transfer of pertinent technologies, and upholding the rights of all stakeholders involved. Furthermore, adequate funding mechanisms are underscored as essential to effectively contributing to the dual goals of biodiversity conservation and sustainable resource utilization.
3. Tools and solutions for implementation and mainstreaming
Target 14
This target has a clear imperative for the comprehensive integration of biodiversity and its diverse values into policy frameworks, regulations, planning strategies, and developmental processes at all governmental levels and across various sectors. This integration extends to pivotal sectors such as agriculture, forestry, fisheries, aquaculture, finance, tourism, health, manufacturing, infrastructure, energy, and mining, with a specific emphasis on the inclusion of safeguards for deep-sea mining. Moreover, this target underscores the critical need to harmonize both public and private sector initiatives, alongside fiscal and financial flows, with the objectives and milestones delineated within the overarching framework. Notably, it advocates for the establishment of cross-sectoral as well as sector-specific objectives to ensure the sustainable utilization of resources, encompassing emerging sectors like insurance, geo-engineering, and biotechnology.
Target 15
To foster a paradigm shift in business practices towards biodiversity conservation. This entails a multifaceted strategy, including incentivizing and empowering businesses, especially large and transnational corporations, to prioritize biodiversity preservation as a core component of their operations. One crucial aspect involves enforcing mandatory regulations compelling companies to rigorously monitor, assess, and transparently disclose their impacts on biodiversity. Concurrently, efforts should be directed towards enhancing consumer awareness by providing them with comprehensive information to make responsible consumption choices that promote biodiversity conservation. Additionally, robust mechanisms for ensuring compliance and reporting on access and benefit-sharing must be implemented to hold companies accountable for their actions. Legal frameworks should be strengthened to hold companies liable for any infringements, impose appropriate penalties, and facilitate redress for any damage caused. Embracing a rights-based approach, encompassing not only human rights but also recognizing the intrinsic rights of Mother Earth, is essential to mitigating adverse effects on biodiversity. Furthermore, transitioning towards sustainable production patterns and fostering a circular economy aligned with international commitments and governmental regulations is paramount for long-term biodiversity conservation efforts.
Target 16
This target delineates a multifaceted approach to promoting sustainable consumption practices and reducing environmental impact. It emphasizes the importance of establishing supportive policy, legislative, or regulatory frameworks to incentivize sustainable consumption choices, alongside efforts to enhance education and access to accurate information and alternatives. Moreover, the target aims to significantly decrease the global footprint of diets or consumption per capita and halve per capita global food waste, thereby addressing key contributors to environmental degradation. Additionally, it underscores the imperative to substantially reduce waste generation and potentially eliminate overconsumption of natural resources and other materials fairly and equitably. Ultimately, this target envisions a future where all individuals can thrive in harmony with Mother Earth, reflecting a holistic and aspirational goal for sustainable development.
Target 17
The establishment and diligent implementation of science-based measures worldwide to mitigate the potential adverse effects of biotechnology, encompassing synthetic biology and other emerging genetic techniques, on both biodiversity and human health. This necessitates a thorough risk assessment and management protocol, as well as the proactive adoption of horizon scanning, monitoring, and assessment frameworks. As well as recognizing the potential benefits of biotechnological progress in helping reach the Convention’s goals and other relevant sustainable development goals, this goal stresses how important it is to include socioeconomic factors in decision-making processes.
Target 18
This target underscores a profound commitment to identifying and eradicating detrimental subsidies for biodiversity by the year 2025, with a resolute aim of curtailing them by no less than 500 billion United States dollars annually. This initiative encompasses a deliberate prioritization of phasing out or reforming subsidies within critical sectors like fisheries and agriculture while simultaneously redirecting resources towards endeavors that foster a net benefit to nature. Moreover, it is imperative to ensure that the incentives set forth are not only positive but also neutral in their impact on biodiversity, thereby aligning harmoniously with international obligations.
Target 19
This target proposes a comprehensive strategy to bolster financial resources for biodiversity conservation, with a primary goal of achieving a minimum of USD 700 billion, achieved through a reduction of harmful subsidies by USD 500 billion and an annual allocation of USD 200 billion by 2030. This commitment underscores the urgency and scale of the financial mobilization needed to address biodiversity loss effectively. Additionally, it aims to mobilize international public financial resources, setting a target of at least USD 100 billion annually until 2030, while recognizing the principle of common but differentiated responsibilities and distinguishing from climate financing. The strategy emphasizes leveraging private finance through innovative instruments such as payment for ecosystem services and blended finance. Doubling domestic resource mobilization by 2030 is a key objective, to be realized through mechanisms like mainstreaming biodiversity across sectors, introducing innovative incentive schemes, and addressing sovereign debt challenges. To facilitate these efforts, the proposal includes the establishment of a new international financing instrument—a global biodiversity fund—to be operational by 2025, designed to complement existing structures like the Global Environment Facility. Moreover, it advocates for building upon lessons from climate financing to enhance resource use effectiveness, efficiency, and transparency. The strategy also calls for promoting new ideas both at home and abroad, such as nature-based solutions, green bonds, biodiversity offsets, and debt-for-nature swaps. This will help create a wide range of ways to pay for biodiversity conservation efforts around the world.
Target 19
Robust capacity-building initiatives, technological advancement, and the facilitation of innovative practices through enhanced scientific collaboration hold paramount importance in this target. Specifically, there is a pronounced focus on bolstering the capacity of individuals and institutions, ensuring equitable access to cutting-edge technologies, and facilitating their transfer, particularly in regions grappling with developmental challenges. Fostering collaborative endeavors in technology development and research is a crucial strategy for promoting biodiversity conservation and sustainable utilization, especially in developing nations. Moreover, the framework underscores the imperative of enhancing scientific research capabilities and monitoring mechanisms to align them with the ambitious objectives and targets, thereby strengthening the foundation for effective biodiversity management and preservation efforts.
Target 20
This target places a strong emphasis on ensuring the accessibility of high-quality data, information, and knowledge, catering to the needs of decision-makers, practitioners, and the wider public. This imperative extends beyond mere accessibility to encompass the integration of traditional knowledge, innovations, practices, and technologies originating from indigenous peoples and local communities. Crucially, this integration is predicated upon the principles of free, prior, and informed consent, acknowledging and respecting the rights and sovereignty of these communities. By prioritizing the inclusion of diverse perspectives and expertise, it underscores the importance of fostering a collaborative and inclusive approach toward decision-making and problem-solving in the realm of biodiversity conservation and sustainable development.
Target 21
In this target, there’s a pronounced focus on fostering comprehensive, gender-responsive, and inclusive representation in decision-making about biodiversity, underscoring the prioritization of indigenous peoples and local communities while upholding their rights to lands, territories, resources, and traditional knowledge. Furthermore, the target recognizes the critical importance of integrating women, girls, children, youth, and individuals with disabilities into these decision-making processes, valuing their voices and perspectives. Finally, the target underscores the critical need to provide unwavering protection for environmental human rights defenders, acknowledging their pivotal role in safeguarding biodiversity and advocating for sustainable practices.
Target 22
In light of this target, prioritizing gender equality within the framework’s implementation becomes paramount. This necessitates a comprehensive gender-responsive strategy that not only acknowledges but actively ensures equal rights and access to land and natural resources for women and girls. Moreover, it advocates for their full, equitable, meaningful, and informed engagement, as well as leadership, across all facets of biodiversity-related action, policy formulation, and decision-making processes. This commitment underscores a broader imperative to address gender disparities and foster inclusive participation, essential for achieving the Convention’s objectives effectively.
Table 1 shows the IPCC’s predictions for the average global temperature, sea level, and CO2 levels in the atmosphere for the years 2030, 2050, and 2100, based on all six Assessment Reports (ARs). It only shows the best- and worst-case scenarios for each type of model used in an AR. Only absolute values are considered, and in situations where there is a lack of absolute value or the model is too complex, making it difficult to determine a value, either the trend is described or it is outright stated that the value was not explicitly specified (Table 1).
Table 1. Summary of IPCC projections for the years 2030, 2050, and 2100 using the most conservative values of global mean temperatures, sea level, and CO2 concentration featured in all the six official IPCC Assessment Reports between 1990 and 2022.
Climate action is steadily increasing among countries with some adopting more ambitious goals than others, which is significant from a micro-perspective. However, holistically, the sum of all these individual efforts is not sufficient to build-up the momentum required to address the magnitude of the current climate crisis which continues unabated. A simple solution to this conundrum would be that every country should start taking more ambitious actions given that what needs to be done is already known to all. However, in reality, this is easier said than done because the challenges are complex and multi-faceted (Figure 6).
Figure 6. Some significant challenges associated with climate change adaptation and mitigation efforts. The severity of these challenges is categorized on a scale from (0) representing ‘non-extant’ to (3) indicating ‘high’, with (1) denoting ‘low’ and (2) representing ‘medium’.
The 2015 Paris Agreement and the 2030 Agenda for Sustainable Development (SDGs) both call for the world to simultaneously tackle climate change and development. Although negotiated separately, both agreements share some common goals, most notably limiting global temperatures. However, growing national interest in adaptation and other SDG goals means that funds would be allocated in multiple directions, which inevitably creates trade-offs between goals (Kuyper and Schroeder, 2018). For instance, in a study that compared countries’ progress on SDG goals to their per-capita CO2 emission, a decomposition analysis of SDG index scores and per-capita CO2 emissions revealed that what distinguishes “low CO2” countries from “high CO2” countries are the innate abilities of each to mitigate per-capita CO2 emissions from energy-intensive sectors of their respective economies, such as the industrial, energy, and transport sectors. The same study goes forward by stipulating that, even if a country is a “low CO2” and “low SDG index score” today, the growing need for economic development, which translates to aiming to attain higher SDG index scores, would inherently cause an increase in per-capita CO2 emissions (Kobayakawa, 2021).
The Paris Agreement is legally binding solely in procedural terms and exclusively pertains to the global stocktake, which connects the execution of Nationally Determined Contributions (NDCs) with the overarching objectives of the Paris Agreement and the enhancement of climate ambitions. Geography is implicit in the notion of jurisdiction and this presents a challenge as it is difficult to establish jurisdiction under the current context. Without jurisdiction, rules, laws, and accountability for law enforcement cannot be established. Regarding international law, it should be noted that NDCs do not possess legal binding force on governments. As a result, the UNFCCC’s capacity to effectively accomplish the goals of the Paris Agreement is hindered both domestically and internationally due to the absence of a legal framework that enables the incentivization or penalization of individuals or entities based on their respective reduction or increase of greenhouse gas emissions. Hence, the Paris Agreement has so far only been an obligation for individual countries (Taebi and Safari, 2017). The UNFCCC is urged to assume a more coordinating function and investigate alternative strategies, such as publicizing and criticizing non-compliant parties, offering specialized knowledge, and taking the lead in debates during COPs, to promote voluntary initiatives and motivate compliance with NDC objectives (Falkner, 2016; Taebi and Safari, 2017).
Climate change is complex, making it difficult for most people to establish a causal relationship (Samantray and Pin, 2019; Kovaka, 2021). Most people, for instance, lack imagination and cannot see a link between the intensive use of coal-fired power plants on one side of the world and the occurrence of natural disasters or extreme weather on the other. Additionally, as individuals, people are hesitant to accept regulations to reduce greenhouse gas emissions because they know that others will not consider it important for the greater good (Wong-Parodi and Feygina, 2020). Government is the solution to collective action but there is a growing trend in government distrust and globally, both government and international skepticism has been on the rise (Torcal, 2014; Heimstädt, 2017; Marshall and Drieschova, 2018). Global climate governance involves a complex network of interconnected actors, each with varying impacts on the climate system. As a result, pinpointing the parties responsible for positive or negative outcomes can be exceedingly challenging (Kuyper and Schroeder, 2018).
Biodiversity, as defined by the Intergovernmental Science-Policy Platform on Biodiversity and Ecosystem Services (IPBES), refers to “the range of different living species found in various environments, such as land, sea, and other aquatic ecosystems, together with the interconnected ecological systems they generate. This encompasses the diversity in genetic, phenotypic, phylogenetic, and functional characteristics, along with alterations in the abundance and distribution throughout time and place within and across species, biological groups, and ecosystems” (IPBES Secretariat, 2022a). In other words, biodiversity creates an interactive ecosystem made up of multiple species of organisms that collectively contribute to and sustain major planetary processes. For instance, terrestrial and marine ecosystems play an important role in regulating the Earth’s surface temperature by sequestering large amounts of atmospheric carbon emissions every year. A diverse ecosystem is better equipped to withstand environmental stress, mitigate the impacts of extreme weather events, and provide resilience against climate change. Healthy ecosystems provide the foundation for ecological stability via the proliferation of various species of organisms that play important roles in an ecosystem such as capture and storage of energy (through the process of photosynthesis), and decomposition of waste. Healthy ecosystems also support water supply and water quality, and protect against water-related risks. Food systems worldwide are completely dependent on biodiversity and a diverse range of ecosystem services that enhance agricultural productivity, including pollination, pest management, and soil fertility (CBD, 2020). Biodiversity is also a repertoire of all the material resources for the manufacture of various products of economic value. Sanctuaries, national parks, and biosphere reserves provide recreational services and also serve as avenues for tourism. Biodiversity also plays a crucial role in many cultures around the world. For instance, some plant species, especially orchids are used as ornamentals, in traditional ceremonies, and the concoction of various medicines.
Biodiversity loss refers to “the decline of any form of biological diversity, including genetic, species, and ecosystem diversity, in a specific area due to death (including extinction), destruction, or deliberate removal. This loss can occur at various scales, ranging from global extinctions to population extinctions, ultimately leading to a decrease in overall diversity at the corresponding scale” (IPBES Secretariat, 2022b).
Extinction is a natural process of the Earth’s past, present, and future. The current understanding of species extinction is based on predictions derived from various types of modeling and extinction risk assessments (Johnson et al., 2017; Humphreys et al., 2019). Throughout history, there have been five major mass extinction events: the Ordovician, Devonian, Permian, Triassic, and Cretaceous extinctions. In each of these events, the rate of extinction significantly exceeded natural or background rates—those not influenced by human activities—which are established through analysis of the fossil record. These extinctions resulted in a catastrophic loss of at least 75% of all species within a relatively brief period of time on the geological timescale (Proença and Pereira, 2017).
Current extinction rates are projected to be at least 1,000 times higher than the historical average and approximately 100 times greater than the natural extinction rate. It is anticipated that future rates of extinction will be at least as high as the current rates and possibly 10 to 100 times higher (Pimm et al., 2014; Proença and Pereira, 2017). Given the recent surge in species extinctions, the scientific community is assessing whether the Anthropocene epoch may be experiencing a sixth mass extinction event (Ceballos et al., 2017; Turvey and Crees, 2019; Cowie et al., 2022). To accurately estimate the global extinction rate and assess biodiversity loss, it is critical to understand both the magnitude of species extinctions and the rate at which they occur (Pimm et al., 2006, 2014). In light of this, a targeted investigation compared current extinction rates with those of past mass extinctions, commonly known as "the Big Five." The study determined that although the recent decline in species has been significant and severe, leading to the extinction of entire populations, it does not qualify as a mass extinction by paleontological standards set by "the Big Five" (Barnosky et al., 2011). Indeed, the rate of species extinction has markedly accelerated over the past 500 years, though it has predominantly affected a limited number of genera (Andermann et al., 2020; Pimm et al., 2006; Ritchie and Roser, 2021; Thuiller et al., 2019). Mass extinctions are not selective in contrast to background extinctions which tend to be selective and take place at comparatively lower magnitudes (Jablonski, 1986; McCallum, 2015). Nonetheless, if the current rate of species extinction continues to accelerate, it is projected that numerous groups of species could face extinction by the end of the 21st century (McCallum, 2015).
Presently, only 2% to 4% of species that have ever lived are thought to still exist today. Over the past 500 years, human activity has led to the extinction of nearly 900 species (IUCN, 2022). Presently, there are more than 17,000 plant and animal species that are at risk of extinction. The IUCN Red List, which evaluates species’ conservation status, has only examined 6% of the estimated 2 million species that have been reported so far (IUCN, 2022). This means that there are still around 14 million species that have not been reviewed, and their existence is not officially documented. Furthermore, as stated by the International Union for Conservation of Nature (IUCN), over 40,000 species, which account for 28% of all evaluated species, are at risk of becoming extinct. As such, it is not far-fetched to surmise that the assessment of only 6% of all described species fails to accurately capture the extinction risks of the remaining bulk of unknown species. Every single land-based terrestrial biome in the past three decades has lost 20% of species due to anthropogenic activities (Martin, 2019). Moreover, the diversity of various insect species worldwide is under threat and by some estimations, insect species are going down the path of extinction which could cause the catastrophic collapse of many ecosystems globally (Montgomery et al., 2020; Wagner, 2020). The global insect population is decreasing at a rate that is eight times greater than the decline in the populations of mammals, birds, and reptiles. At the current pace, in the coming decades, around 40% of the global insect species will become extinct (Sánchez-Bayo and Wyckhuys, 2019). The decrease in the prevalence of individual species or taxonomic groups is noteworthy, but there are also alterations in insect biomass, which is a significant factor for ecological functioning (Hallmann et al., 2021). The collective biomass of insects is declining at a rapid rate of 2.5% annually, indicating that they may disappear within a span of one hundred years (UNEP, 2019). An improved procedure was employed in a research study to quantify the total biomass of insects in 63 protected areas in Germany over a span of 27 years. The study revealed a seasonal reduction of 76% and a mid-summer decline of 82% in the biomass of flying insects (Hallmann et al., 2017). The decline in insect variety and abundance is anticipated to impact insect species that fulfill both specialized and generalized functions within ecosystems. This threatens ecosystem services such as pollination and other expected cascades of effects that can disrupt the food webs, causing the majority of insect species that cannot adapt in response to go extinct (Hallmann et al., 2021).
Soil harbors almost 25% of the total species on Earth, making it a significant repository of biodiversity (Bach et al., 2020). Soil biodiversity, which includes bacteria, fungi (including mycorrhiza), protists, and invertebrates, has a crucial role in supporting life in various ecosystems. It contributes to important processes such as nutrient cycling and retention, food production, soil rejuvenation, pollution remediation, climate regulation, and reducing the potential for harmful pathogens and underground biological conflicts (Delgado-Baquerizo et al., 2020b). Soil deterioration is progressing all over the world affecting soil conditions and threatening soil biodiversity with extinction; and coincidentally, most areas threatened with soil deterioration tend to harbor high levels of soil biodiversity (Guerra et al., 2020). Without soil biodiversity, terrestrial ecosystems may collapse because there is an inextricable link between above and below-ground biodiversity.
The decline in plant biodiversity is a widespread occurrence observed in various environments and geographic regions; however, the significant alterations in plant distribution are not as commonly acknowledged. For instance, a study investigated the spatiotemporal changes in the occurrence of 2136 species of vascular plants over an area of about 350,000 km2 in Germany and discovered that over a 60-year period (1960 to 2017) more than 70% of plant species suffered severe population decline (Eichenberg et al., 2021). According to another study, the reduction of habitats by 2050 will lead to a decline in global vascular plant diversity. This decline is estimated to range from 7% to 24% compared to the diversity levels in 1995. The decline will occur after populations have adjusted to the reduced habitats. The biomes that are projected to experience the greatest loss of species include warm mixed forests, savannahs, shrublands, tropical forests, and tropical woodlands (van Vuuren et al., 2006).
Biodiversity decline may stem from seasonal fluctuations affecting plant and invertebrate populations, including insects. Additionally, natural ecological disruptions such as wildfires, floods, and volcanic eruptions can contribute to this decline by eradicating local populations of specific species and reshaping entire biological communities. Ecosystems are believed to have the ability to adapt to these fluctuations and disruptions, despite their prevalence and transient nature. Conversely, when humans produce disruptions, the resulting biodiversity losses tend to lead to more severe, long-lasting, and irreversible ecological changes or damages in ecosystems, landscapes, and the global biosphere. The majority of the Earth’s land-based ecosystems underwent a change from their natural state to being under the influence of human activity between the 18th and the beginning of the 21st century (Ellis et al., 2010). Currently, pasture land and arable land make up a growing portion of the Earth’s surface area. Approximately half of the Earth’s inhabitable area is dedicated to agriculture, with 77 percent utilized for cattle grazing (Ritchie and Roser, 2013). Multiple studies indicate that the intensification of current land-use changes will significantly contribute to the decline of biodiversity, especially within agricultural landscapes (Ellis et al., 2010; Powers and Jetz, 2019; Lambertini, 2020; Benton et al., 2021). Additionally, a 2019 report by the Intergovernmental Science-Policy Platform on Biodiversity and Ecosystem Services found that anthropogenic activity puts as many as one million species of flora and animals at risk of extinction. The activities of deforestation, wetland reclamation, waterway diversions, and construction of infrastructure such as roads and buildings have significant and noticeable effects on the ecological development of landscapes.
The primary anthropogenic factors contributing to the decline in biodiversity, as commonly described in scientific literature, include land-use changes encompassing agriculture, habitat loss and degradation, invasive species, overexploitation, and pollution (Figure 7). Furthermore, climate change is acknowledged as another significant driver that can work in concert with anthropogenic factors to intensify the impacts. (Box 2) (Pereira et al., 2010). The agricultural sector is the primary driver of biodiversity decline, and its consequences are increasing as a result of shifting consumption patterns and population growth. Agriculture diminishes biodiversity through the transformation of natural environments into highly controlled systems, resulting in the emission of pollutants, including greenhouse gases (Dudley and Alexander, 2017; Lanz et al., 2018; Geisen et al., 2019; Sánchez-Bayo and Wyckhuys, 2019). The process of thinning, fragmentation, or destruction of a preexisting natural habitat leads to a reduction or complete elimination of the available food resources and living space for the majority of species. Species that cannot migrate or rapidly adjust to environmental changes sometimes face extinction (Pereira et al., 2010). Excessive logging and wood harvesting, overfishing, unsustainable hunting of fauna for food and sports, and overharvesting of wild flora for food, medicinal, and cultural purposes lead to overexploitation. This causes certain species to be depleted to very low numbers and others to become extinct, as their populations are unable to recover from the losses (de Souza and Prevedello, 2020; Oldfield et al., 2019; Caro et al., 2022). Alien species have the potential to substantially alter or disturb the ecosystems they invade, and they can outcompete indigenous species for resources and living space. This phenomenon elicits reductions in the population of indigenous species. Nonetheless, invasive species can colonize unfamiliar regions either through natural dispersal or deliberate human intervention (Sánchez-Bayo and Wyckhuys, 2019; Frick et al., 2020). Biodiversity loss occurs when the addition of any material or energy to the environment exceeds the rate at which it can be distributed, diluted, decomposed, recycled, or stored harmlessly. This leads to the accumulation of substances across different trophic levels in the ecosystem, resulting in biodiversity loss. Exposure in certain instances can result in lethal doses or reproductive issues that jeopardize the survival of the species (Brei et al., 2016; Lee et al., 2017; Sánchez-Bayo and Wyckhuys, 2019). While anthropogenic climate change is associated with a decline in biodiversity, it is not currently considered the primary driver of biodiversity loss (Thomas et al., 2004; Caro et al., 2022). The continuous growth of the human population and extensive use of natural resources have a significant impact on biodiversity loss drivers. Furthermore, interactions among multiple factors can accelerate the rate at which biodiversity declines.
Figure 7. Various underlying synergistic drivers of plant diversity decline. The severity of these impacts is categorized on a scale from (0) representing ‘non-extant’ to (3) indicating ‘high’, with (1) denoting ‘low’ and (2) representing ‘medium’.
Box 2. Impacts of Climate Change on Lower Plants and Soil Microbial Communities
(i) Cryptogams and Climate Change
Cryptogams, encompassing algae, fungi, slime molds, lichens, and bryophytes (including mosses, liverworts, and hornworts), are a diverse group of non-vascular plants that reproduce via spores. These organisms are integral to various ecosystems worldwide, performing essential ecological functions (Hao and Chu, 2021; Singh et al., 2018). Bryophytes, for example, are critical to ecosystem regulation because they act as a buffer system for neighboring plants, providing essential water and nutrients that support plant growth. At high latitudes and altitudes, bryophytes play a significant role in biomass production, carbon and nutrient cycling, and overall ecosystem dynamics (Alatalo et al., 2015). They are especially valuable in areas where mining or other disturbances have led to the loss of vascular vegetation, demonstrating resilience and ecological recovery potential (Ren et al., 2021).
Despite their ecological importance and evolutionary precedence over vascular plants, bryophytes receive less attention in the context of climate change compared to vascular species (He et al., 2016; Küttim et al., 2019; Vanneste et al., 2017). Unlike vascular plants, bryophytes are characterized by their small size and poikilohydric nature, meaning their water and nutrient uptake are entirely dependent on their immediate environment. This unique adaptation makes them highly sensitive to environmental changes, with survival and reproductive success closely tied to fluctuations in their surroundings (Cacciatori et al., 2022; Désamoré et al., 2012). As such, understanding the impact of climate change on bryophytes is crucial for assessing their role in ecosystem health and resilience.
The impact of climate change on biodiversity is evident through complex interactions of habitat degradation and shifts in geographical distribution (Alatalo et al., 2014; Dai et al., 2022). Bryophytes play a crucial role in climate studies because of their unique ecological roles and sensitivities. They significantly influence global carbon cycles, particularly through carbon sequestration in peatlands, with Sphagnum mosses exhibiting the highest carbon storage among plant genera. Unlike angiosperms, bryophytes lack roots and therefore do not directly draw water from the soil but rely on atmospheric precipitation, making them highly dependent on rainfall for hydration.
Bryophytes, especially those in temperate regions, exhibit narrower optimal temperature ranges and lower heat tolerance compared to angiosperms. These distinctive ecophysiological traits position bryophytes as effective indicators for monitoring the effects of climate change on biodiversity (Ruklani et al., 2021; Zanatta et al., 2020). Climate projections indicate an increasing trend in the frequency and severity of extreme weather events, such as heat waves, droughts, unpredictable wildfires, intense rainfall, and flooding (Alatalo et al., 2014). These phenomena affect ecosystems at multiple levels, including bryophytes (Viles and Cutler, 2012). Heat waves have been particularly detrimental, leading to the mortality of peat mosses within the Sphagnum genus, which includes about 380 species. More frequent fires and droughts are increasingly threatening bryophytes, the second-most diverse group of terrestrial plants after angiosperms, leading to substantial declines in species diversity and the extinction of some forest-dwelling bryophytes (Pereira et al., 2021). These changes emphasize the importance of bryophytes as indicators of climate change, as well as the urgent need to monitor and address their conservation.
(ii) Soil Microbial Community Diversity and Climate Change
Soil is the loose, uppermost layer of the Earth's crust that covers much of the land surface (Gupta et al., 2020). It serves as a critical medium for various ecological functions and is teeming with a diverse array of microorganisms. These soil-resident microbes, including bacteria and fungi, are essential for soil formation and maintenance. These microbial communities are integral to several critical soil processes, including the decomposition of organic matter, the mineralization of complex organic substances, nutrient recycling, plant growth promotion, and carbon storage and sequestration (Chu et al., 2020).
The functionality of the soil ecosystem is heavily reliant on the dynamic interactions between microorganisms and their environment. These interactions facilitate the transfer of chemical signals, carbon, and nutrients across different levels of the food chain. Through their roles in regulating biogeochemical cycles and ecosystem processes, soil microbes help to maintain ecological balance and support overall ecosystem resilience. Thus, soil microbiota preservation is critical for sustaining soil health and ecosystem stability (Singh and Gupta, 2018; Wang et al., 2018; Yang et al., 2021).
Soil organisms engage in complex interactions with each other and with plants, significantly influencing and maintaining ecosystem characteristics. These interactions can affect the distribution and traits of both plant and animal species within a given area (Classen et al., 2015). Climate change is altering the geographical ranges of species and reshaping the relationships between organisms. Ecological communities are intricate, comprising species with varied life histories, temperature tolerances, and dispersal capabilities. Climate change disrupts species interactions, which has detrimental effects on biodiversity and terrestrial ecosystems (Lladó et al., 2017; Yang et al., 2021). Research consistently shows a strong connection between microbial diversity and soil health and stability (Dubey et al., 2019; Razanamalala et al., 2018; Rillig et al., 2019; Tardy et al., 2014). Ongoing global changes, such as increased frequency of extreme weather events, nitrogen loading, and biotic exploitation, could exacerbate biodiversity loss, further compromising ecosystem stability and resilience.
A conservation policy aims to save or restore a declining species, community, ecosystem, or natural or semi-natural site (Meinard, 2017). The International Union for the Conservation of Nature (IUCN) and the Convention on Biodiversity (CBD) are the primary global institutions that work at the highest levels to inform global and national policies that promote biodiversity conservation, restoration, and sustainable management (Box 4). Both institutions have successfully marshaled global nature conservation policy over the past decades, but the sheer magnitude of the objectives and targets still hampers their efforts (Box 4).
Box 3. IPCC Assessment Report Scenarios (IS92 – SSP)
IS92: The IS92 scenarios, developed in the 1992 Supplementary Report to the IPCC Assessment, offer six alternative trajectories (IS92a to f) for future greenhouse gas emissions. These scenarios reflect a variety of assumptions about how emissions may evolve in the absence of new climate policies beyond those already in place at the time. Each scenario envisions a different global context, taking economic, social, and environmental factors into account, resulting in a wide range of potential outcomes for greenhouse gas emissions—ranging up to tenfold. The scenarios were grounded in data from widely respected international organizations and expert analyses, providing a diverse and comprehensive outlook on potential futures shaped by varying levels of economic growth, technological development, and policy responses.
SRES: The "Special Report on Emissions Scenarios" (SRES) outlines six key scenarios of greenhouse gas emissions, each accompanied by socio-economic narratives. These scenarios are designed to facilitate comparative analysis across various studies on climate change mitigation, adaptation, and impacts. The scenarios are grouped into four families, with one scenario each from the A2, B1, and B2 families, and three scenarios from the A1 family. The A1 family scenarios reflect different energy technology trajectories: A1FI represents a fossil-intensive trajectory, A1T focuses on predominantly non-fossil energy sources, and A1B provides a balanced mix of energy sources. Together, these scenarios capture a broad spectrum of possible pathways for energy development and their implications for global climate change.
RCP: Radiative forcing (RF) describes the imbalance between incoming and outgoing radiation energy at a planetary scale. Positive radiative forcing occurs when external factors cause incoming energy to exceed outgoing energy, leading to an increase in the planet’s temperature. On the other hand, negative radiative forcing occurs when outgoing energy exceeds incoming energy, resulting in a decrease in the planet's temperature.
Representative Concentration Pathways (RCPs) are scenarios that project radiative forcing (RF) levels by the year 2100 relative to pre-industrial levels in 1750. The IPCC's fifth assessment report analyzed four RCP scenarios to evaluate potential climate changes from the present day through 2100 and beyond. These scenarios are designed to account for the inherent uncertainties in future emissions due to variables such as population growth and economic development. Consequently, the RCPs cover a wide range of possible futures.
For instance, scenarios such as RCP6.0 and RCP8.5 represent pathways with minimal or no mitigation efforts, leading to higher levels of global warming. Conversely, RCP2.6 depicts a scenario where stringent mitigation measures successfully limit global warming to a maximum of 2 °C. Each RCP defines a specific level of radiative forcing, which climate models use to project the corresponding changes in the Earth's climate system. Each RCP is associated with a specific radiative forcing value, reflecting the expected increase in energy trapped in the Earth's atmosphere that influences future climate conditions.
SSP: The Shared Socio-Economic Pathways (SSPs) provide researchers with a framework to analyze the impacts of different emission scenarios by incorporating a range of socio-economic variables, such as population growth, GDP, and urbanization. This approach represents an advancement over the earlier Special Report on Emission Scenarios (SRES), which generally aligned future emissions with a single socio-economic trajectory, except for the A1 scenario family. The A1 scenarios were divided into three distinct sub-scenarios (A1FI, A1T, and A1B), each reflecting different socio-economic assumptions under the overarching "high-growth" narrative. These sub-scenarios illustrated the critical role of socio-economic factors in shaping emissions outcomes.
The SSPs provide a more detailed perspective by simulating various socio-economic pathways developed by multiple independent organizations. These pathways encompass a range of assumptions regarding GDP, population, urbanization, and development at both national and regional levels. The resulting quantifications of these scenarios are critical for understanding the potential socio-economic impacts on emissions and form the core components of the SSP framework.
CITES (the Convention on International Trade in Endangered Species of Wild Fauna and Flora) was signed by representatives from 80 countries in Washington, DC, on 3 March 1973, and entered into force on 1 July 1975 (Norton, 2018). There are currently 183 parties to the Convention. The Convention is an international agreement between governments established as a response to growing concerns that the overexploitation of wildlife through international trade contributed to the rapid decline of many species of plants and animals worldwide (Morton et al., 2021; Scheffers et al., 2019). Today, it accords varying degrees of protection to more than 35,000 species of animals and plants, whether they are traded as live specimens, fur coats or dried herbs (Robinson and Sinovas, 2018) (Box 5).
Wildlife trade across most sectors has increased since monitoring began, for example, between 1996 and 2018 the global fish market rose from $40 billion to $180 billion, wood from $65 billion to $137 billion and reptile leather for fashion trade from $140 million to $600 million. In concert, the annual number of trades legally traded through CITES has also grown, from under 5000 transactions in 1977 to peaking at over 1.3 million in 2015, with shipment size increasing in parallel and seizures of illegally traded species showing similar trends (Harfoot et al., 2018).
Balancing the needs of people for livelihood generation, especially with access and benefit-sharing rights, with the impact on species survival remains difficult (Table 2). Issues like the role of trophy and sports hunting within conservation remain a topic of debate in the conservation community. Identifying strategies that ensure long-term species survival, promote equity, and sustain livelihoods is an ongoing challenge (‘t Sas-Rolfes et al., 2019).
Box 4. Background on IUCN and CBD
(i) International Union for the Conservation of Nature (IUCN)
Modern conservation history began with the establishment of the International Union for the Conservation of Nature (IUCN) on October 5, 1948, as the world’s first global environmental organization. Since then, IUCN has become the global authority on the state of nature and the measures needed to protect it by providing scientific knowledge and essential tools to guide conservation action. IUCN also aims to support, encourage international cooperation, and assist societies around the world to preserve the integrity and diversity of nature; and to ensure that all uses of natural resources are fair and environmentally sustainable (MacDonald, 2003).
To achieve its goals, over the years, IUCN has played an important role in the creation and development of international treaties, conventions, and agreements on the environment such as the Ramsar Convention, the Convention on International Trade in Endangered Species (CITES), the World Heritage Convention, and the Convention on Biological Diversity (CBD) and has encouraged States to adhere to these agreements. IUCN is also one of the pioneer organizations that guided the creation of the Rio Conventions on Biodiversity (CBD), the United Nations Framework Convention on Climate Change (UNFCCC), the United Nations Convention to Combat Desertification (UNCCD) and even more recently the Paris Agreement (Christoffersen, 1997).
Considered the premier association of conservation agencies worldwide, including states, government agencies and NGOs, IUCN is uniquely positioned to reflect, lead and promote the priorities of the global conservation community (Christoffersen, 1997). As a result, due to increasing global environmental problems, IUCN became the only environmental organization to have observer status at the United Nations General Assembly in 1999, enabling it to deliver the policy perspectives of its members at the highest international level of diplomacy. Today, with its wide reach and expertise, IUCN is by far the largest and most diverse environmental network in the world. It is at the forefront promoting nature-based solutions as key to the implementing international agreements.
(ii) Convention on Biological Diversity (CBD)
In 1992, the Rio Earth Summit established the Convention on Biological Diversity (CBD) as a comprehensive global framework to halt and reverse the ongoing loss of biodiversity. The Convention has three goals: the conservation of biodiversity, the sustainable use of its components and the fair and equitable sharing of the benefits arising from the use of genetic resources. It attempts to reconcile conservation with economic development. More broadly speaking, the Convention has one simple overriding goal: to maintain the biological foundation on which all human societies depend (Zedan, 2005). The CBD’s governing body is the Conference of the Parties (COP) and they meet every two years to review progress, set priorities and commit to work plans. The Convention provides a framework for action. Over the years, the Parties have translated its provisions into programmes of work covering all major types of ecosystems and on cross-cutting issues such as traditional knowledge, access to genetic resources and benefit-sharing, biodiversity and tourism, and incentive measures.
The International Union for Conservation of Nature (IUCN) has played a crucial role in shaping the Convention on Biological Diversity (CBD) from its inception, contributing to the development and implementation of its objectives. IUCN consistently contributes to the decisions of the Conference of the Parties (COP) through its global, regional, and national policy efforts, ensuring alignment with the CBD's overarching objectives. The IUCN's work with the CBD spans multiple areas, including but not limited to:
1. Supporting the implementation of the Strategic Plan for Biodiversity 2011–2020 and its Aichi Biodiversity Targets;
2. Facilitating the execution of the Nagoya Protocol on Access and Benefit Sharing;
3. Parties receive assistance in revising, updating, and formulating National Biodiversity Strategies and Action Plans (NBSAPs);
4. Shaping CBD negotiations by advocating for conservation-focused policies through the development and promotion of strategic policy papers.
The Convention has made significant progress, but its implementation is hindered by critical factors such as inadequate cooperation and technology transfer, insufficient political will and resources, and a pervasive underestimation of biodiversity's true value for socioeconomic development and poverty alleviation (Zedan, 2005). As a result, conservation efforts have mainly focused on reducing damage rather than addressing the root causes of environmental degradation. Despite the successes in laying the groundwork for future species and ecosystem recovery and demonstrating effective conservation strategies, the underlying drivers of unsustainable environmental use remain inadequately addressed (Johnson et al., 2017). It is urgent to tackle these underlying issues to improve the effectiveness of conservation efforts and ensure long-term sustainability.
Despite scientific evidence that biodiversity loss is a prominent, serious, and pervasive global issue, humanity’s response has fallen short (Evans, 2021; Rands et al., 2010). Conservation efforts have advanced significantly over time, beginning with the enactment of the first species-protection laws in the nineteenth century. This progression continued with the establishment of the first national park in the mid-twentieth century, followed by the implementation of the United Nations Convention on Biological Diversity and the development of national biodiversity strategies (Ibisch, 2005). The national biodiversity strategies and action plans (NBSAPs) under the Convention on Biological Diversity serve as key mechanisms for integrating biodiversity considerations into national policies (Sarkki et al., 2016). Overall, the action plan has facilitated global discussions on the importance of biodiversity protection, leading to varying degrees of positive attitudes toward nature conservation. However, in many countries and regions, the measures implemented have been insufficient or ineffective in curbing the continued loss of biological diversity (Figure 8). Numerous habitats remain significantly degraded, and without more robust and extensive interventions, additional species are at risk of becoming endangered or facing extinction in the near future (Auvinen et al., 2007).
Figure 8. A vivid illustration of the intricate network of interconnected challenges between global climate and conservation policy.
The establishment of protected areas is the most common in-situ conservation strategy in most countries around the world, followed by the implementation of various ex-situ conservation and management strategies for the conservation of wild species, such as nurseries, botanical gardens, zoos, germplasm banks, aquariums, species reproduction and rehabilitation centres (Attuaquayefio and Folib, 2005; Khuroo et al., 2020; Le Saout et al., 2013; Mestanza-Ramón et al., 2020; Oseni et al., 2018; Zhu et al., 2021). However, conflicts between conservation efforts and local livelihoods, as well as between broader and more localized interests, are an inherent aspect of many conservation initiatives globally (Chhatre and Saberwal, 2005; Verma and Mohammad, 2020) (Boxes 6, 7).
Most NBSAPs at the national level lack explicit biodiversity goals, resulting in limited contributions to biodiversity conservation (Wu et al., 2019; Tong, 2020). In some cases, ineffective regulatory policies stifle international collaboration (Mason et al., 2020). Moreover, PAs are frequently understaffed, underfunded and poorly managed (Mestanza-Ramón et al., 2020; Selig et al., 2014). Issues with responsibility allocation create a divide between environmental management and other critical policy sectors (Martin et al., 2013; Meng and Li, 2022; Young et al., 2014). In conservation planning, there is often inadequate integration of biodiversity and ecosystem services, coupled with ineffective monitoring that hampers the adaptive governance of environmental programmes. Additionally, there is a lack of stakeholder inclusion and insufficient mainstreaming of biodiversity (Sarkar et al., 2006; Strayer and Dudgeon, 2010; Tabarelli et al., 2005). Many conservation strategies also incorrectly assume that different taxonomic groups share congruent geographical patterns of diversity. Given that biodiversity is unevenly distributed, prioritization is crucial to minimize loss, yet this remains a significant challenge at the national level due to limited technical capabilities (Bolpagni et al., 2019; Brooks et al., 2006; Grenyer et al., 2006; Velasco et al., 2015).
Box 5. CITES—Aim and Operating Mechanism.
International trade in wildlife species is estimated to be worth billions of dollars annually (Morton et al., 2021). It comprises the legal trade in commercial fishing ($180 billion), timber ($227 billion), fashion goods ($2.5 billion), and illegal trade in meat, medicine, ornamental use, pets, and ivory (Hughes, 2021). Wildlife trade includes the trade of any organism, including fungi, plants, and animals, sourced from the wild. The global wildlife trade is a significant contributor to species decline, with many animal and plant species experiencing high levels of exploitation. This intense commercial pressure threatens biodiversity by reducing population sizes, disrupting ecosystems, and accelerating the extinction risk for numerous species. The trade-in, together with other factors, such as habitat loss, is capable of heavily depleting their populations and even bringing some species close to extinction (Heinrich et al., 2022; Kideghesho, 2016; Liew et al., 2021; Ribeiro et al., 2019; Scheffers et al., 2019). CITES (the Convention on International Trade in Endangered Species of Wild Fauna and Flora) aims to ensure that international trade of wild animal and plant species does not threaten their survival.
CITES is an international agreement to which States and regional economic integration organizations adhere voluntarily. States that have agreed to be bound by the Convention (‘joined’ CITES) are known as Parties. Although CITES is legally binding on the Parties – in other words, they have to implement the Convention – it does not take the place of national laws. Instead, it provides a framework to be respected by each Party, which has to adopt its domestic legislation to ensure that CITES is implemented at the national level.
Parties regulate the international trade of CITES species through a system of permits and certificates that are required before specimens listed in their appendices are imported, exported, or introduced from the sea. Each party is required to adopt national legislation and to designate two national authorities, namely, a Management Authority responsible for issuing permits and certificates under the control of a Scientific Authority (Foster et al., 2016). These two national authorities also assist with CITES enforcement through cooperation with customs, police, and other appropriate agencies. The Parties have an obligation to provide an annual report on all trade in species of flora and fauna listed in the Appendices to the Convention and to provide a biennial report on legislative, regulatory, and administrative measures taken. Each Party must maintain records of trade in species covering: names and addresses of exporters and importers; numbers and types of permits and certificates granted; and states with which trade has occurred.
The operational bodies of CITES include the Standing Committee (SC) and two scientific committees: The Plants Committee (PC) and the Animals Committee (AC). The Standing Committee provides policy guidance to the Secretariat concerning the implementation of the Convention and oversees the management of the Secretariat’s budget. Beyond these key roles, it coordinates and oversees, where required, the work of other committees and working groups; carries out tasks given to it by the Conference of the Parties; and drafts resolutions for consideration by the Conference of the Parties (CITES, 2022d). The scientific committee (Animals and Plants Committees) of experts was established at the sixth meeting of the Conference of the Parties (CITES COP6, 1989) to fill gaps in biological and other specialized knowledge regarding species of animals and plants that are (or might become) subject to CITES trade controls. Their role is to provide technical support to decision-making about these species. These two Committees have similar terms of reference, detailed in Resolution Conf. 18.2, Annex 2, which includes inter alia, providing scientific advice and guidance to the Conference of the Parties, the other committees, working groups and the Secretariat; dealing with nomenclatural issues; undertaking periodic reviews of species, in order to ensure appropriate categorization in the CITES Appendices; advising when certain species are subject to unsustainable trade and recommending remedial action (through a process known as the ‘Review of Significant Trade’); and drafting resolutions on animal and plant matters for consideration by the Conference of the Parties (CITES, 2022d).
Appendices I, II, and III of the Convention outline different levels of protection for species to prevent overexploitation (CITES, 2022a.)
Appendix I includes species that are the most endangered among those listed by CITES. These species face a high risk of extinction, and international trade in their specimens is generally prohibited. Exceptions to this prohibition are made only for non-commercial purposes, such as scientific research. In these cases, trade may occur if both an import permit and an export permit (or re-export certificate) are granted. Article VII of the Convention details specific exemptions to this general prohibition.
Appendix II contains species that are not currently at risk of extinction, but could become so if trade is not strictly regulated. This appendix also encompasses "look-alike species," species whose specimens bear a striking resemblance to those of Appendix I species, potentially leading to confusion in trade. For Appendix-II species, international trade can be authorized through the issuance of an export permit or re-export certificate. While CITES does not require an import permit for these species, some countries have implemented stricter measures. Authorities only issue permits or certificates if they are confident that trade will not negatively impact the species' survival in the wild.
Appendix III lists species that are included at the request of a party that already regulates their trade and seeks international cooperation to prevent unsustainable or illegal exploitation. Trade in specimens of Appendix-III species is permitted only when accompanied by the appropriate permits or certificates (CITES, 2022b).
Species may be added to or removed from Appendix I and II, or moved between them, only by the Conference of the Parties, either at its regular meetings or by postal procedures. But species may be added to or removed from Appendix III at any time and by any Party unilaterally (although the Conference of the Parties has recommended that changes be timed to coincide with amendments to Appendices I and II).
While the terrestrial protected area status of only two countries (Cameroon and India) is explicitly examined here concerning the 30-by-30 targets and the challenges they face, this situation mirrors the broader global context. Approximately 80% of countries are similarly falling short of these targets, with only about 20% meeting them by default (Figure 10) (World Bank Group, 2021). As a result, there is a pressing need for a new global mechanism to combat plant diversity loss and ensure effective implementation and attainment of the 30-by-30 targets before the 2050 deadline (Figure 2 and Box 1).
Box 6. Case study I: The scenario in Cameroon
Cameroon is ranked as the fifth-most diverse country in Africa in terms of fauna and the fourth-most diverse in terms of flora (Fokunang et al., 2011; Agbor, 2022). Cameroon, situated in central Africa, is recognized as one of the global hubs for biodiversity. Cameroon is called “Africa in miniature” due to its wide range of landforms, such as coastal regions, highlands, savannas, and rainforests. It also boasts a variety of ecosystems and related biodiversity, as well as a rich tapestry of cultures and traditions (Bobo et al., 2015; Muh et al., 2018). The tropical forests of Cameroon, which cover an area of 19.6 million hectares, play a crucial role in the ecosystem of the Congo Basin forest (Topa et al., 2009). The Congo Basin is believed to harbor some 10,000 species of tropical plants, with 30% of them being exclusive to this particular location. The country is home to over 8,300 plant species (NBSAP II, 2012). A total of 630 species in Cameroon are classified as threatened according to the International Union for Conservation of Nature’s (IUCN) Red List. Among these, 183 species are categorized as endangered, while 115 are classified as critically endangered (DowntoEarth, 2021).
Cameroon now has approximately 54 protected areas (PAs), which account for approximately 10.98% of the total land area, equivalent to 51,538 square kilometers. Out of these PAs, around 30 are subjected to management efficiency evaluations, covering approximately 8.13% of the land area, equivalent to 38,177 square kilometers (Figure 9) (UNEP-WCMC, 2022). The 42 terrestrial protected areas are distributed in the following manner: The country has a total of 1 Flora Sanctuary, 27 National Parks, 2 Strict Nature Reserves, 6 Wildlife Reserves, 6 Wildlife Sanctuaries, 3 UNESCO-MAB Biosphere Reserves (such as the Benoué, Waza, and Dja Reserves), 2 World Heritage Sites (either natural or mixed), and 7 Ramsar Sites, which are Wetlands of International Importance (Ajonina, 2022).
By 2035, Cameroon's biodiversity vision aims to establish a sustainable relationship with its biodiversity. This vision seeks to harness and share the benefits of biodiversity to meet developmental needs and enhance human well-being while maintaining ecosystem balance. Achieving this vision involves sectoral and decentralized integration, with active participation from all stakeholders, including local communities.
The implementation strategy revolves around four strategic objectives: (i) Addressing the causes of biodiversity degradation by mitigating both direct and indirect pressures on biodiversity; (ii) Maintaining and enhancing biodiversity status through the protection of ecosystems, habitats, species, and genetic diversity, and addressing the adverse effects of human and natural pressures such as landscape changes and habitat fragmentation, which undermine ecosystem resilience and stability; (iii) Promoting the sustainable use of biodiversity to create wealth and alleviate poverty, thereby addressing the human impacts of biodiversity loss, including poverty, disease, conflict, food insecurity, loss of income, and unemployment; and (iv) Integrating biodiversity considerations into sectoral and local planning and development processes (NBSAPII, 2012).
Pursuing these ambitious objectives faces significant challenges, particularly given the dependence of many Cameroonians on biological resources for their livelihoods within an economy largely reliant on agriculture. The increasing demand for biological resources and the global wildlife trade have intensified threats to numerous species, with many facing extinction.
Moreover, both climate change and human activities pose severe risks to Cameroon's plant biodiversity, further exacerbating these challenges (Kemeuze et al., 2015). Several significant anthropogenic factors contribute to the decline of biodiversity in Cameroon, including the escalating rates of wildlife habitat conversion for commercial agriculture, fuel wood consumption, air and land pollution, natural disasters, bushfires, overgrazing, unsustainable logging practices, road construction, offshore and onshore oil exploitation, as well as extractive and mining industries (Beckline et al., 2022; Gosline et al., 2022). The nation’s socio-cultural context poses notable obstacles in terms of natural resource utilization and administration. Traditional knowledge is not adequately recognized or employed, and there is a dearth of focused initiatives to raise awareness about biodiversity. Ignorance and negative behavioral patterns resulting from these factors have had indirect detrimental effects on ecosystems and biodiversity. The botanical significance of specific plant species, as well as the species that are of utmost value for conservation, are sometimes not well understood (Zekeng et al., 2021).
Over the past ten years, there have been major changes in the national policies and laws aimed at protecting biodiversity. However, these changes have had a limited effect in terms of reversing the decline in biodiversity. Although there have been substantial endeavors in formulating policies and passing appropriate laws, the execution and enforcement of these measures are ineffective due to insufficient adherence to international environmental agreements at the national level, inadequate integration of different sectors, and a lack of consistency and clashes among important legislative instruments. The insufficient allocation of funds for biodiversity initiatives poses a significant obstacle and necessitates immediate intervention from all major stakeholders and collaboration with partners to change the current trend of neglecting biodiversity (MINEPDED, 2012).
Moreover, there is significant apprehension regarding the execution of the CBD post-2020 30-by-30 objectives, as there has been a prior occurrence of exacerbating infringements on the land rights of indigenous groups in the nation. Therefore, to ensure the successful implementation of the post-2020 30-by-30 targets outlined by the Convention on Biological Diversity (CBD) in Cameroon, the state needs to adopt a conservation strategy that respects the land rights of indigenous and traditional communities. This necessitates a framework firmly grounded in human rights principles, emphasizing the meaningful involvement of indigenous groups in decision-making processes that directly affect their lands and livelihoods (Hoyte, 2021; Agbor, 2022).
Some critically endangered and endangered plant species in Cameroon - Consensus of both IUCN Red List (https://www.iucnredlist.org/search) and Earth’s Endangered Creatures database (http://www.earthsendangered.com/search-regions3.asp) (Supplementary Excel Sheet file 1):
Afrothismia pachyantha; Afrothismia winkleri; Allexis obanensis; Angraecum sanfordii; Autranella congolensis; Begonia bonus-henricus; Begonia furfuracea; Begonia pelargoniiflora; Begonia pseudoviola; Brachystephanus kupeensis; Bulbophyllum filiforme; Bulbophyllum jaapii; Bulbophyllum modicum; Cassipourea acuminata; Chassalia laikomensis; Crotalaria mentiens; Crotonogyne impedita; Cyathula fernando-poensis; Cyperus microcristatus; Disperis kamerunensis; Disperis mildbraedii; Drypetes magnistipula; Eugenia gilgie; Eugenia kameruniana; Habenaria maitlandii; Impatiens etindensis; Impatiens letouzeyi; Kupea martinetugei; Lecaniodiscus punctatus; Ledermanniella keayi; Ledermanniella letouzeyi; Ledermanniella onanae; Ledermanniella thalloidea; Liparis goodyeroides; Macropodiella pellucida; Magnistipula conrauana; Magnistipula cuneatifolia; Millettia conraui; Millettia laurentii; Newtonia camerunensis; Ormocarpum klainei; Oxyanthus okuensis; Oxygyne triandra; Pararistolochia preussii; Pavetta brachycalyx; Pavetta muiriana; Pavetta rubentifolia; Placodiscus caudatus; Plectranthus dissitiflorus; Polystachya cooperi; Polystachya farinose; Polystachya victoriae; Psychotria bimbiensis; Psychotria densinervia; Psychotria minimicalyx; Psychotria moliwensis; Psychotria moseskemei; Pyrenacantha cordicula; Rinorea fausteana; Sabicea xanthotricha; Sarcophrynium villosum; Saxicolella marginalis; Scleria afroreflexa; Sclerochiton preussii; Stelechantha arcuate; Tieghemella Africana; Tricalysia lejolyana; Vangueriella zenkeri and Vepris heterophylla.
Despite significant efforts in conservation, the 2010 Aichi targets have not been fully achieved. One of the primary obstacles is the inadequate formulation of national targets by many countries. This is compounded by inadequate investments, limited knowledge, and insufficient accountability in biodiversity conservation efforts (Xu et al., 2021). The interconnectedness of nature transcends geopolitical borders, underscoring the universal vulnerability to ecological crises and emphasizing the necessity for collective action. The 15th Conference of the Parties to the Convention on Biological Diversity (CBD COP15) was held in Kunming to foster communication and consensus-building among participating nations. The objective was to devise a comprehensive Post-2020 Global Biodiversity Framework (Post-2020 GBF). This framework aims to guide biodiversity conservation efforts in the next decade and beyond, emphasizing collaborative measures to reverse the alarming trend of global biodiversity loss (Wei, 2021).
The post-2020 global biodiversity framework represents a crucial evolution from the preceding Strategic Plan for Biodiversity 2011–2020. It delineates an ambitious strategy aiming to instigate widespread transformative action, fostering a profound shift in humanity’s relationship with biodiversity. The overarching objective is to realize the shared vision of coexisting harmoniously with nature by 2050. This framework seeks to mobilize urgent and transformative efforts from governments, inclusive of indigenous peoples, local communities, civil society, and businesses. By articulating its vision, mission, goals, and targets, the framework aspires to contribute substantially to the overarching objectives of the Convention on Biological Diversity, its Protocols, and other pertinent biodiversity-focused multilateral agreements, processes, and instruments. The primary focus of implementation is at the national level, with supportive actions at the subnational, regional, and global tiers. The framework offers a comprehensive, outcome-oriented structure for formulating national and, where applicable, regional goals and targets. It emphasizes the need for periodic updates to national biodiversity strategies and action plans, facilitating ongoing monitoring and global-level progress assessment. Furthermore, the framework is designed to foster synergies and coordination across the Convention on Biological Diversity, its Protocols, and other pertinent processes, ensuring a unified and integrated approach towards global biodiversity conservation.
The proposed framework is a significant contribution to effectively realizing the 2030 Agenda for Sustainable Development. The Sustainable Development Goals play a crucial role in establishing the necessary conditions for the framework’s successful implementation. The framework outlines four overarching long-term goals for 2050, aligning with the envisioned biodiversity vision for 2050. These goals are accompanied by ten milestones to be assessed by 2030, serving as critical indicators of progress. The framework comprises 21 action-oriented targets for immediate attention over the coming decade, outlining specific actions within each target that must be promptly initiated and conclusively accomplished by 2030. This concerted effort is anticipated to culminate in the achievement of the 2030 milestones and the realization of outcome-oriented goals for 2050, as articulated by the Convention on Biological Diversity (CBD, 2021). Table 3 provides a comprehensive overview of plant-related actions that hold the potential to significantly contribute to the attainment of the Global Biodiversity Framework 2030 targets.
Plant tissue culture is a well-established technique that has evolved through various stages, progressing from basic applications to more sophisticated methodologies, much like many other technological advancements (Abdin et al., 2017; Mosoh et al., 2024a, 2024b). Originally, plant tissue culture was employed as a research instrument to nurture and examine the growth of small, isolated portions of plant tissues or individual cells. By the mid-twentieth century, there was a widespread acceptance of the idea that plants could be regenerated or reproduced through callus or organ culture. This led to practical applications in the plant propagation sector (Idowu et al., 2009; Wojtania and Mieszczakowska-Frąc, 2021). Consequently, numerous commercial laboratories were built globally to facilitate the large-scale reproduction of horticultural plants through cloning (Mosoh et al., 2024b). Plant tissue culture applications have broadened significantly beyond traditional clonal propagation and micropropagation (Figure 1). Common techniques now include somatic embryogenesis, somatic hybridization, synthetic seed technology, cryopreservation, protoplast fusion, plant medicine, and using bioreactors for large-scale mass clonal propagation. The value of these technologies goes beyond their application in large-scale cloning to encompass advanced molecular biology (genetic engineering, metabolic engineering, synthetic biology, advanced imaging technologies), plant improvement, bioprocessing, and, notably, conservation and the restoration of endangered plant species (Lynch, 1999; Chandana et al., 2018; Loyola-Vargas and Ochoa-Alejo, 2018; Khandel et al., 2022).
The decline in plant diversity is primarily caused by the combined effects of climate change and human activities, which result in rapid and substantial alterations in plant communities. The key drivers of this decline are changes in temperature, rainfall patterns, and evaporation rates, as well as an alarming increase in extreme weather events. However, the stochastic permutations and combinations of these events introduce uncertainty, resulting in outcomes that range from potential benefits, such as tropical expansion that could enhance diversity, to worst-case scenarios that could lead to further erosion of plant diversity (Ivetić and Devetaković, 2016; Velásquez et al., 2018).
An often-overlooked aspect of climate change is its impact on plant diseases and pests, which adversely affects the availability and quality of planting materials in both natural ecosystems and agricultural settings (Beckford and Norman, 2016; Delgado-Baquerizo et al., 2020a; Velásquez et al., 2018). Historical evidence indicates that favorable climatic conditions can lead to significant crop losses due to disease development. This observation can be similarly applied to plants in their natural habitats. Extensive literature explores how climate change may impact the prevalence and severity of plant diseases, the frequency of epidemics, and their geographical distribution in agriculture (Donatelli et al., 2017; Zhou et al., 2020). Natural ecosystems, with their diverse species and intricate interactions, contrast markedly with the relatively simplistic structure and species composition of most agricultural environments. This complexity creates a multifaceted network of interactions between wild plants and pathogens, making it challenging to fully comprehend the implications for natural ecosystems and communities. The consequences could be more severe and troubling, potentially leading to cascading effects such as local extinction events caused by declines in host health, which can subsequently impact the composition and stability of entire plant communities. Moreover, humans exert significant influence over the scale and impact of pathogen populations in agriculture through practices such as selective breeding, agronomic techniques, nutrient and moisture management, and chemical treatments. However, in natural plant ecosystems, the application of these methods is neither feasible nor environmentally acceptable. As a result, our ability to mitigate alterations in these natural systems is significantly more constrained compared to agricultural environments (Burdon and Zhan, 2020; Malhi et al., 2021). Plant tissue culture technology, with its diverse applications, can complement traditional agriculture practices and control systems that are often unsuitable for wild plants in their natural ecosystems. Plant tissue culture technology offers more targeted strategies and effective solutions for conserving endangered plant species in their natural habitats (Mosoh et al., 2023).
In many countries, the current cultivation areas for fruits, vegetables, and ornamental plants are likely to become insufficient to meet the demands of rapidly growing populations. Plant tissue culture technology, which is still underutilized in numerous regions, holds considerable promise for significantly enhancing productivity. Beyond agriculture and horticulture, plant tissue culture applications extend to diverse fields such as orchidology, forestry, medicinal plant production, and derived products, offering a wide range of benefits (Loyola-Vargas and Ochoa-Alejo, 2018). Commercially valuable plants are at significant risk of extinction due to overexploitation and the destruction of their natural habitats. Plant tissue culture applications have the potential to be highly effective in alleviating pressure on natural populations of medicinal and commercially important plants, thereby promoting their sustainable utilization (Yadav et al., 2012; Pant, 2013; Chandana et al., 2018). For instance, integrating traditional knowledge with modern plant tissue culture techniques offers a systematic and reliable approach to discovering new medicines and ensuring their sustainable production. This synergy enables cost-effective production through well-designed in vitro systems, such as bioreactors, reducing the need for unsustainable wild collection practices. Furthermore, developing countries are currently implementing climate change mitigation programmes in the forestry sector, including REDD+ (Reducing Emissions from Deforestation and Forest Degradation). The “+” symbolizes the incorporation of conservation, sustainable forest management, and the enhancement of forest carbon stocks. However, the primary obstacle faced is the insufficient availability of high-quality planting stocks (Rawat et al., 2020).
The main obstacle encountered by afforestation projects and programmes globally is the scarcity of high-quality planting stocks. Access to superior planting materials can significantly enhance forest regeneration and restoration efforts. Therefore, ensuring the availability of high-quality planting stocks is crucial for boosting forest productivity. Plant tissue culture technology stands out as the most reliable method for addressing this challenge (Sudhersan et al., 2003; Tegen and Mohammed, 2016; Mulugo et al., 2020).
Box 8 offers a comprehensive overview of plant tissue culture (PTC), highlighting its crucial role in plant conservation. It highlights the advantages of PTC, such as the ability to produce large quantities of plants with desirable traits, rapid propagation, and genetic diversity preservation. However, it also acknowledges the associated challenges, including high costs, technical expertise requirements, genetic instability risks, and contamination potential.
Box 7. Case study II: The Pachmarhi Biosphere Reserve (PBR) in India
Among the world’s 196 countries, India is one of 17 designated as mega biodiversity centres (mega-diverse countries have the highest biodiversity indices, including a large number of endemic species; and collectively, they contain at least 70% of the planet’s terrestrial biological diversity). The Pachmarhi Biosphere Reserve (PBR) is located in Central India among the lofty hills and plateaus of the Satpura Ranges (Chandra, 2009).
The Pachmarhi Biosphere Reserve (PBR) is situated within the geographical coordinates of 20° 10’ to 22° 50’ north latitude and 77° 45’ to 78° 56’ east longitude. It is one of the 18 biosphere reserves in India. It spans three districts in Madhya Pradesh: Hoshangabad, Betul, and Chhindwara. The overall land area of PBR is 4926.28 square kilometers, with 524.37 square kilometers designated as the core zone and the rest, 4462.93 square kilometers, allocated to the buffer zone. Panna Biosphere Reserve (PBR) comprises three distinct animal conservation areas: Satpura National Park, spanning an area of 524.37 square kilometers; Bori Animal Sanctuary, covering 518.00 square kilometers; and Pachmarhi Sanctuary, including 461.37 square kilometers (Chandra, 2009; Patil, 2018). The core zone of PBR is Satpura National Park, while the buffer zone includes the Bori and Pachmarhi sanctuaries. In general, temperatures in this area range from 11 to 42°C (Kala, 2013).
Biosphere reserves are areas that provide economic and social benefits to indigenous peoples while also demonstrating sustainable development and conservation in the larger biogeographical region. These are the defined geographical zones exclusively intended for the long-term preservation of the diverse range of plant and animal species that inhabit them, as well as to provide sustainable livelihoods (Aggarwal et al., 2020). PBR is known for its diverse plant diversity (both lower and higher plants) as well as its cultural diversity, as it is home to a variety of tribal and non-tribal communities, with the Gond tribe being the most dominant indigenous community (Nath et al., 2012; Sahay and Gupta, 2015; Kala, 2015a). In terms of the cryptogamic flora of PBR, researchers have been studying algae, lichens, bryophytes, and pteriodophytes since the late twentieth century (Pande and Bhardwaj, 1952; Gupta and Asthana, 2016).
Climate change, industrialization, and the use of synthetic hazardous substances have led to significant environmental degradation, posing severe threats to natural ecosystems (Kala, 2015b). Concurrently, the growing demand for ethnobotanicals and evolving market trends have compelled indigenous communities to engage in unsustainable extraction practices, diverging from their traditional methods. Historically, these communities practiced careful harvesting, but modern pressures have led to overexploitation, causing a decline in the availability of valuable forest resources. For instance, after intensive harvesting, the yield of certain ethnobotanicals has diminished significantly, making it increasingly difficult to obtain these resources in subsequent years.
As a result, many indigenous plant species are now endangered. Notably, eight out of fourteen traded species are listed in various categories on the IUCN Red List (Kala, 2013). Additionally, overharvesting, fire, overgrazing, tourism-related activities, and the exploitation of high-value medicinal plants by local tribes and traders have further reduced the availability of these plants, leading to the extinction of several valuable species (Shadangi and Nath, 2008).
If the current constraints, particularly those driven by human activities, continue to hinder the regrowth of many plant species, stakeholders may face severe ecological and societal repercussions similar to an ecological catastrophe. Conservation efforts have been largely inadequate. Proposed solutions, such as monitoring the status of the Pachmarhi Biosphere Reserve (PBR), training local communities, developing tourist infrastructure, conducting agro-ecological studies, and documenting indigenous knowledge, have failed to achieve substantial impact (Kala, 2015a; Patil, 2018). Furthermore, only about 7.52% of India’s total land area is designated as protected, falling short of the 30% target set by the new 30-by-30 goal of the post-2020 biodiversity conservation framework (Figure 10).
Figure 10. Terrestrial protected areas (% of total land area) in relation to the 30-by-30 target (WorldBank Group, 2021).
Some critically endangered and endangered plant species in India—Consensus of the IUCN Red List (https://www.iucnredlist.org/search) and the Council of Scientific and Inductrial Research—National Botanical Research Institute (CSIR-NBRI) list of threaten plants (https://nbri.res.in/threatened-plants-conservation/) (Supplementary Excel sheet 2 and 3 files):
Abutilon ranadei Woodrow &Stapf; Aconitum chasmanthum Stapf ex Holmes; Aconitum heterophyllum var. bracteatum Stapf; Adelocaryum malabaricum (C.B.Clarke) Brand; Adinandra griffithii Dyer; Agasthiyamalaia pauciflora (Bedd.) S.Rajkumar &Janarth; Aglaia malabarica Sasidh; Aldrovanda vesiculosa L.; Amentotaxus assamica D.K.Ferguson; Anacolosa densiflora Bedd.; Angelica glauca Edgew.; Aponogeton satarensis Sundararagh., A.R.Kulk. &S.R.Yadav; Aporosa bourdillonii Stapf; Aquilaria khasiana Hallier f.; Aquilaria malaccensis Lam.; Ardisia blatteri Gamble; Atropa acuminata Royle ex Lindl.; Atuna indica (Bedd.) Kosterm.; Atuna travancorica (Bedd.) Kosterm.; Bentinckia nicobarica (Kurz) Becc.; Biermannia jainiana S.N.Hegde &A.N.Rao; Buchanania barberi Gamble; Byrsophyllum tetrandrum (Bedd.) Hook.f.; Capparis pachyphylla M.Jacobs; Ceropegia huberi Ansari; Ceropegia jainii Ansari &B.G.P.Kulk.; Ceropegia odorata Nimmo; Chlorophytum borivilianum Santapau &R.R.Fern.; Cinnamomum perrottetii Meisn.; Cinnamomum riparium Gamble; Cinnamomum travancoricum Gamble; Cinnamomum walaiwarense Kosterm.; Cinnamomum wightii Meisn.; Cleistanthus travancorensis Jabl.; Coelogyne hajrae Phukan; Commiphora wightii (Arn.) Bhandari; Coptis teeta Wall.; Curcuma bhatii (R.M.Sm.) Škorničk. &M.Sabu; Cycas beddomei Dyer; Cycas circinalis L.; Cycas sphaerica Roxb.; Cycas zeylanica (J.Schust.) A.Lindstr. &K.D.Hill; Cynometra beddomei Prain; Cynometra bourdillonii Gamble; Cynometra travancorica Bedd.; Cypripedium elegans Rchb.f.; Cypripedium himalaicum Rolfe; Dactylorhiza hatagirea (D.Don) Soó; Dalbergia congesta Wight &Arn.; Dalbergia gardneriana Benth.; Decalepis hamiltonii Wight &Arn.; Dialium travancoricum Bourd.; Dimeria hohenackeri Hochst. ex Miq.; Dimeria hohenackeri Hochst. ex Miq.; Dipterocarpus bourdillonii Brandis; Dipterocarpus indicus Bedd.; Drimia razii Ansari; Drypetes andamanica (Kurz) Pax &K.Hoffm.; Drypetes porteri (Gamble) Pax &K.Hoffm.; Dysoxylum malabaricum Bedd. ex C.DC.; Elaeocarpus blascoi Weibel; Elaeocarpus gaussenii Weibel; Eriocaulon rouxianum Steud.; Eugenia argentea Bedd.; Eugenia discifera Gamble.; Eugenia floccosa Bedd.; Eugenia singampattiana Bedd.; Eulophia obtusa (Lindl.) Hook.f.; Euonymus serratifolius Bedd.; Euphorbia epiphylloides Kurz; Euphorbia sebastinei Binojk. &N.P.Balakr.; Euphorbia vajravelui Binojk. &N.P.Balakr.n; Ficus andamanica Corner; Garcinia cadelliana King; Garcinia imbertii Bourd.; Garcinia rubroechinata Kosterm.; Garcinia travancorica Bedd.; Gentiana kurroo Royle; Goniothalamus simonsii Hook.f. &Thomson; Gymnema khandalense Santapau; Gymnocladus Assamicus P.C.Kanjilal; Heritiera fomes Buch.-Ham.; Hildegardia populifolia Schott &Endl.; Hopea canarensis Hole; Hopea erosa (Bedd.) Slooten; Hopea jacobi C.E.C.Fisch.; Hopea racophloea Dyer; Humboldtia bourdillonii Prain; Humboldtia vahliana Wight; Hydrocotyle conferta Wight; Ilex gardneriana Wight; Ilex khasiana Purkay.; Ilex venulosa Hook.f.; Illicium griffithii Hook.f. &Thomson; Iphigenia stellata Blatt.; Isonandra stocksii C.B.Clarke; Isonandra villosa Wight; Ixora johnsonii Hook.f.; Ixora lawsonii Gamble; Ixora lawsonii Gamble; Lamprachaenium microcephalum (Dalzell) C.B.Clarke; Lilium polyphyllum D.Don; Limnopoa meeboldii (C.E.C.Fisch.) C.E.Hubb.; Madhuca bourdillonii (Gamble) H.J.Lam; Madhuca diplostemon (C.B.Clarke) P.Royen; Madhuca insignis (Radlk.) H.J.Lam; Magnolia gustavii King; Magnolia pleiocarpa (Dandy) Figlar &Noot.; Meizotropis pellita (Prain) Sanjappa; Melicope indica Wight; Memecylon flavescens Gamble; Memecylon sisparense Gamble; Meteoromyrtus wynaadensis (Bedd.) Gamble; Myristica magnifica Bedd.; Nardostachys jatamansi (D.Don) DC.; Nepenthes khasiana Hook.f.; Orophea thomsonii Bedd.; Paphiopedilum charlesworthii (Rolfe) Pfitzer; Paphiopedilum druryi (Bedd.) Stein; Paphiopedilum fairrieanum (Lindl.) Stein; Paphiopedilum insigne (Wall. ex Lindl.) Pfitz.; Paphiopedilum venustum (Wall. ex Sims.) Pfitz.; Pecteilis triflora (D.Don) Tang &F.T.Wang; Picrorhiza kurroa Royle ex Benth.; Pimpinella tirupatiensis N.P.Balakr. &Subram.; Piper barberi Gamble; Pittosporum eriocarpum Royle; Popowia beddomeana Hook.f. &Thomson; Psychotria globicephala Gamble; Psydrax ficiformis (Hook.f.) Bridson; Pterocarpus indicus Willd.; Pterocarpus santalinus L.f.; Rapanea striata Mez; Rotala ritchiei Koehne; Salacia brunoniana Wight &Arn.; Saussurea costus (Falc.) Lipsch.; Shorea tumbuggaia Roxb.; Sonneratia griffithii Kurz; Symplocos oligandra Bedd.; Syzygium alternifolium (Wight) Walp.; Syzygium andamanicum (King) N.P.Balakr.; Syzygium beddomei (Duthie) Chithra; Syzygium bourdillonii (Gamble) Rathakr. &N.C.Nair; Syzygium courtallense (Gamble) Alston; Syzygium manii (King) N.P.Balakr.; Syzygium microphyllum Gamble; Syzygium palghatense Gamble; Syzygium periyarensis Augustine &Sasidh.; Syzygium stocksii (Duthie) Gamble; Syzygium travancoricum Gamble; Tamarix kutchensis B.V.Shetty &R.P.Pandey; Tarenna agumbensis Sundararagh.; Taxus wallichiana var. chinensis (Pilg.) Florin; Tecomella undulata (Sm.) Seem.; Tephrosia jamnagarensis Santapau; Tribulus rajasthanensis Bhandari &V.S. Sharma; Utleria salicifolia Bedd. ex Hook.f.; Utricularia cecilii P. Taylor; Valeriana leschenaultii DC; Vateria macrocarpa K.M.Gupta; Wendlandia andamanica Cowan and Wendlandia angustifolia Wight ex Hook.f.
The history of Kew Gardens dates back to the nineteenth century when conservation was not a matter of great concern as it is now in modern times. At the time, the primary motive for gathering rare plants was not conservation but rather trade and displaying a collection of rare and extinct species of plants in the wild (Prance, 2010). Over time, successive administrations at Kew Gardens expanded the collection, increasing its significance and introducing the concept of preserving its value through careful management and curation. Nowadays, many of the earlier collections of species are recorded as endangered by the IUCN. When environmental concerns began brewing up in the middle of the twentieth century, the focus of Kew Gardens started shifting towards conservation (Figure 9). This evolution culminated in the creation of the Millennium Seed Bank, the world's largest collection of wild plant seeds. Over the years, Kew Gardens has increasingly embraced both ex-situ and in-situ conservation efforts, evolving into a hub not only for conservation but also for developing a sustainable economic model around these activities. This transformation has been supported by a robust research programme encompassing plant tissue culture technology, advanced laboratories, systematics, molecular genetics, a comprehensive herbarium, and an extensive library. Now, organizations such as the Threatened Plants Unit of IUCN and Botanic Gardens Conservation International (BGCI) have their roots in Kew Gardens as well as other conservation initiatives namely; the wild trade monitoring network TRAFFIC (Trade Records Analysis of Flora and Fauna in Commerce), CITES and Convention on Biodiversity (CBD).
The Global Strategy for Plant Conservation (GSPC) is an initiative of the Convention on Biological Diversity (CBD). Kew Gardens is an active signatory to this programme (Cowell et al., 2022). At the XVI International Botanical Congress in St Louis, Missouri, U.S.A., in August, 1999, attended by over 5,000 botanists from all parts of the world, the recognition that two-thirds of plants are at risk of extinction by the end of the 21st century, called for plant conservation to be recognized as an outstanding global priority in biodiversity conservation. In response to the Congress resolution, an ad hoc group drawn from major international and national organizations, institutions and other bodies involved in biodiversity conservation from 14 countries came together in Gran Canaria, Spain on 3–4 April, 2000 to consider the need for a global initiative for plant conservation. The group recognized the pressing need to create a Global Strategy for Plant Conservation (GSPC) and its implementation programme within the framework of the United Nations Convention on Biological Diversity. This strategic initiative aims to support and promote effective plant conservation efforts at all levels, as well as address the ongoing and unacceptable loss of plant diversity (Crane, 2000). At CBD COP-5 in 2000, a proposal was made to establish a Global Strategy for Plant Conservation (GSPC) at COP-6. In 2002, during COP-6, the international community adopted the GSPC, marking the first instance of setting targets for biodiversity conservation. To support the national implementation of the Global Strategy for Plant Conservation (GSPC), the COP-7 in 2004 established the Global Partnership for Plant Conservation (GPPC). Today, the GPPC comprises over 50 institutions, organizations, and networks that run national, regional, and international plant conservation programmes. In 2010, GSPC targets were updated for 2020, taking into account progress that had already been made and adopted at COP-10, with a decision that implementation of the GSPC should be pursued as part of the broader framework of the Strategic Plan for Biodiversity 2011–2020 (Table 4).
The GSPC highlights the importance of plants and the ecosystem services they provide for all life on Earth, and aims to ensure their conservation The vision of the Global Strategy for Plant Conservation (GSPC) is encapsulated in the statement, “Without plants, there is no life. The functioning of the planet and our survival depend on plants. The Strategy seeks to halt the continuing loss of plant diversity.” The GSPC aims to halt the ongoing loss of plant diversity. Five main objectives guide the GSPC, which encompasses 16 specific targets for plant conservation, all aimed for achievement by 2020 and beyond (Dhanda et al., 2019) (Table 4).
The global loss of plant diversity is a pressing issue that threatens the stability of ecosystems and the well-being of human populations. This loss not only disrupts ecological balance but also undermines the myriad benefits that plants provide, including medicinal resources, agricultural productivity, and climate regulation. We propose the Kew-Wide Mechanism (KWM) as an innovative and comprehensive approach to biodiversity conservation in response to this critical threat (Figure 2). It aims to halt the decline of plant diversity and promote sustainable ecosystems through global collaboration, scientific research, and local community engagement. By addressing the root causes of plant diversity loss and implementing targeted conservation strategies, the KWM aims to preserve plant species for future generations and enhance global ecosystem health.
The mission of the KWM is to safeguard global plant diversity by implementing innovative, inclusive, and sustainable conservation practices. This initiative is dedicated to halting the decline of endangered plant species and ensuring the long-term health of ecosystems. By leveraging cutting-edge scientific research, community engagement, and strategic partnerships, the KWM aims to create a resilient framework for plant conservation that can adapt to emerging challenges. The KWM envisions a future where plants not only survive but thrive, fostering flourishing ecosystems that offer significant environmental, cultural, and economic benefits to human societies. KWM aims to create a world in which the intricate web of plant life supports and enhances the well-being of all living organisms, ensuring sustainable and harmonious coexistence.
The KWM sets forth ambitious strategic objectives to address the urgent challenge of plant diversity loss. Central to these objectives is the goal of achieving the 30-by-30 biodiversity conservation milestones by 2030. This target serves as a critical benchmark for the initiative's immediate efforts, ensuring that significant strides are made in the short term. Simultaneously, the initiative pledges to achieve wider conservation objectives by 2050 (Box 1). This long-term vision focuses on the sustained preservation of plant diversity, promoting ecological resilience and stability for future generations.
A cornerstone of the KWM is leveraging its global reach to develop a comprehensive and robust inventory of endangered plant species. This inventory will be pivotal in guiding conservation efforts, providing a detailed understanding of the species most at risk and the ecosystems they inhabit. By systematically documenting and analyzing the status of plant species worldwide, the KWM aims to prioritize actions, allocate resources efficiently, and implement targeted conservation strategies. This data-driven approach ensures that efforts are both effective and sustainable, addressing the most critical needs in plant conservation and fostering a coordinated global response to biodiversity loss.
The KWM encompasses a broad spectrum of stakeholders, each playing a crucial role in its implementation and success. The Convention on Biological Diversity (CBD) spearheads the initiative globally through a special task force that provides overarching guidance and coordination. Kew Gardens, with its extensive botanical expertise and resources, collaborates closely to integrate scientific knowledge and technical support. Member states contribute by aligning national policies with the initiative’s objectives and providing essential political and financial backing.
On the national level, the KWM engages governments and parliamentary bodies, which are instrumental in shaping and enforcing conservation policies. National governments are responsible for executing the initiative’s strategies within their jurisdictions, while parliamentary bodies ensure legislative support and oversight. Local conservation agencies work directly on the ground to implement conservation measures, monitor progress, and adapt strategies to regional needs.
Expert groups, including the Satellite Expert Group plus Kew Representatives (SEGK), bring specialized knowledge and technical expertise to the initiative. These groups, along with other international organizations, play a pivotal role in providing scientific research, data analysis, and practical solutions. Their involvement guarantees that cutting-edge science and best practices inform the KWM.
Local communities, including Indigenous groups and community organizations, are integral to the success of the KWM. Their engagement is essential for the grassroots implementation of conservation strategies and the integration of traditional knowledge with modern practices. By fostering strong partnerships with these local stakeholders, the initiative promotes community ownership and participation, which is crucial for sustainable and effective conservation outcomes.
CBD Special Task Force: At the global level, the CBD Special Task Force is pivotal in supporting and steering the KWM. Its responsibilities include coordinating with the Satellite Expert Group plus Kew Representatives (SEGK) and other relevant partners to ensure cohesive and effective action. A key initiative spearheaded by the CBD Special Task Force is the implementation of the Plant Diversity Fund. This fund aims to furnish crucial financial resources and credit to bolster SEGK operations, empowering them to embark on crucial conservation projects and research. Additionally, the CBD Special Task Force oversees the development and maintenance of an effective communication system. Global, national, and local stakeholders can coordinate seamlessly with this system, which ensures smooth information flow and regular monitoring and reporting of conservation milestone progress. The communication system also includes the posting of progress reports, which are crucial for transparency and accountability. By ensuring that all parties are informed and engaged, the CBD Special Task Force helps to maintain momentum and oversee progress toward achieving conservation milestones, ensuring that global efforts align with the initiative's strategic goals.
Kew Gardens: Kew Gardens plays a crucial role by offering specialized technical expertise and resources essential for the success of the KWM. This includes facilitating SEGK activities, providing advanced scientific knowledge, and supporting the development and implementation of conservation strategies. Kew Gardens' involvement ensures that conservation efforts are grounded in cutting-edge research and best practices.
National governments are responsible for effective governance and the implementation of conservation strategies within their jurisdictions. They secure funding, uphold international agreements, and enforce a robust regulatory framework. This includes conducting Environmental Impact Assessments (EIA) for infrastructure projects, implementing taxation and penalties, and making necessary subsidy reforms to prevent activities that threaten plant diversity. Additionally, they must protect indigenous rights and collaborate with Kew Gardens and other partners to enhance conservation efforts.
Parliamentary Bodies: In the KWM framework, parliamentary bodies play a critical oversight role. They are responsible for confirming membership within the Satellite Expert Group (SEG) and approving budgetary allocations for the initiative. A special parliamentary committee monitors the actions and outcomes of the SEGK, ensuring accountability and effective use of resources. This oversight is vital for maintaining transparency and ensuring that the KWM's objectives are met.
The SEGK (Satellite Expert Group plus Kew Representatives) is integral to addressing localized conservation issues. It provides leadership, technical expertise, and coordination to facilitate information sharing and support national implementation efforts. The SEGK guides the achievement of conservation targets and ensures the tailoring of strategies to local needs and conditions.
Local Communities: Local communities are essential to the success of the KWM. They actively participate in conservation efforts, contribute valuable traditional knowledge, and advocate for sustainable practices. Their engagement ensures that conservation strategies are culturally relevant and effectively implemented, fostering a collaborative approach to preserving plant diversity.
The KWM is designed to function seamlessly across both global and national levels to maximize its impact on plant conservation. It serves as a central hub for the exchange of critical information, strategic insights, and intelligence among Satellite Expert Groups and Kew Representatives (SEGK) at the global level. This centralized approach ensures that conservation efforts are well-coordinated and effective, facilitating the alignment of strategies and resources across diverse geographic regions and expertise areas. The global framework supports the integration of data and best practices, enabling a unified response to the challenges of plant diversity loss.
On the national level, the KWM adopts a more localized approach, focusing on specific regional needs and priorities. This includes the identification and designation of Important Plant Areas (IPAs), which are crucial for preserving key habitats and species. National efforts also involve conducting ethnobotanical surveys to assess the conservation status of plant species and understand their cultural significance. By prioritizing high-impact species and tailoring conservation strategies to regional contexts, the KWM ensures that interventions are both relevant and effective. This dual-layered operational framework not only enhances global coordination but also empowers national and local actors to address plant conservation challenges in a targeted and impactful manner.
Public-private partnerships are crucial for advancing plant conservation efforts. By establishing reliable collaborations between governmental bodies and private sector entities, the KWM can secure the necessary funding and operational support for plant tissue culture (PTC) laboratories and polyhouses. These facilities are critical for scalable conservation initiatives, allowing for large-scale propagation and management of endangered plant species.
International organizations play a significant role in augmenting both global and national conservation strategies. By partnering with these entities, the KWM can leverage their technical expertise and resource mobilization capabilities. This collaboration enhances the effectiveness of conservation efforts through shared knowledge, advanced technologies, and coordinated actions that address plant diversity loss on a worldwide scale.
Collaboration with research institutions and non-governmental organizations (NGOs) is also vital. Engaging with local and international research institutions ensures the generation of meaningful scientific data and fosters innovative solutions to conservation challenges. NGOs contribute practical conservation solutions and field expertise, which are essential for implementing effective strategies and achieving the KWM’s conservation goals. Together, these partnerships and collaborations create a robust network of support, driving significant advancements in plant conservation and promoting a sustainable future for global plant diversity.
Community engagement is a cornerstone of the KWM, ensuring that conservation efforts are deeply rooted in the participation and empowerment of local populations. Educational programmes are a key component, designed to implement targeted initiatives and curricula aimed at communities and institutions, with a particular focus on youth. These programmes aim to foster a culture of conservation and facilitate the exchange of knowledge, instilling a sense of stewardship and responsibility towards biodiversity from an early age. Complementing these educational efforts are awareness campaigns that promote conservation practices by integrating traditional knowledge with modern techniques. This approach bridges the gap between local cultural practices and scientific advancements, creating a holistic and inclusive strategy for plant conservation. Additionally, capacity-building initiatives are vital, providing comprehensive training for students, local farmers, communities, and relevant institutions in sustainable practices and resource management. These training programmes equip participants with the skills and knowledge needed to implement and sustain effective conservation measures, ensuring that local communities are not only beneficiaries but active contributors to the KWM's goals. Through these multifaceted community engagement strategies, the KWM aims to create resilient and self-sustaining local conservation efforts that are integral to the global mission of halting plant diversity loss and promoting sustainable ecosystems.
Effective funding and resource management are critical to the success of the KWM’s global conservation efforts. The initiative employs a blended finance model, which combines funding from international donors, government allocations, and private-sector investments. This diversified approach not only ensures the sustainability of conservation efforts but also enhances their scalability, allowing the initiative to expand and adapt as needed. In addition to traditional funding sources (primarily government funding), the KWM actively develops alternative streams of income by providing services where applicable. These services might include consulting, training programmes, and partnerships that generate revenue while furthering conservation goals.
Strategic and efficient resource allocation is paramount to the initiative's success. Resources are directed to high-priority areas and projects based on rigorous assessment and prioritization processes. This ensures that the most critical regions and species receive the necessary support to achieve maximum impact. The allocation process is continually monitored and evaluated to adapt to changing circumstances and optimize outcomes. The KWM employs these comprehensive financial strategies to establish a robust and adaptable framework for global plant conservation, which ensures the effective and sustainable use of resources to halt biodiversity loss and promote the health of ecosystems worldwide.
Monitoring and evaluation are critical components of the KWM, ensuring that conservation efforts are effective, transparent, and adaptive. Key Performance Indicators (KPIs) serve as essential tools for tracking progress, utilizing metrics such as the number of species protected, improvements in conservation status, and levels of community engagement. These indicators provide a quantitative basis for assessing the initiative's impact and identifying areas needing further attention or adjustment.
Regular reporting is another crucial aspect, involving the provision of transparent and detailed progress reports. These reports include independent audits to maintain high standards of accountability and credibility. They offer stakeholders a clear view of the initiative’s achievements and challenges, fostering trust and support.
Furthermore, the KWM employs an adaptive management approach, which involves continuously revising and refining strategies based on ongoing monitoring data and evaluation results. This dynamic process ensures that conservation practices remain relevant and effective in the face of changing environmental conditions and emerging challenges.
By integrating these rigorous monitoring and evaluation practices, the KWM not only demonstrates its commitment to achieving its conservation goals but also enhances its capacity to respond proactively to new developments, ultimately ensuring the long-term success and sustainability of plant diversity conservation efforts.
The KWM is designed to achieve transformative outcomes and long-lasting impacts by 2030 and beyond. One of the primary goals is to significantly reduce the global loss of plant diversity, thereby preventing the extinction of numerous plant species and preserving the intricate web of life that depends on them. By enhancing ecosystem resilience, the initiative aims to bolster the ability of natural systems to withstand and recover from environmental stresses such as climate change, pollution, and habitat destruction. This, in turn, supports the sustainable use of plant resources, ensuring that these vital assets are available for future generations without compromising their ecological integrity.
Moreover, the KWM is committed to improving the well-being of human societies. Healthy ecosystems provide a myriad of benefits, including clean air and water, fertile soil for agriculture, and resources for medicines and other essential products. By safeguarding plant diversity, the initiative helps secure these ecosystem services that are fundamental to human health, livelihoods, and quality of life.
The initiative also aims to empower communities, fostering a sense of ownership and active participation in conservation efforts. Through educational programmes, awareness campaigns, and capacity-building initiatives, the KWM ensures that local populations are well-equipped to manage and protect their natural resources effectively. This empowerment is crucial for creating resilient communities that can adapt to environmental challenges and contribute to the global conservation mission.
In summary, the KWM's expected outcomes include healthier and more resilient ecosystems, sustained biodiversity, and empowered communities engaged in proactive conservation. These efforts collectively pave the way for a sustainable future where both nature and human societies can thrive in harmony.
Box 8. Plant Tissue Culture Technology: Background, Significance, Challenges and Opportunities
(a) Basics of plant tissue culture (PTC)
Plant tissue culture (PTC) is a specialized technique involving the meticulous cultivation of plant cells, tissues, or organs in a controlled, in vitro environment, typically in a laboratory setting. This method utilizes nutrient-rich growth media and precise conditions to nurture seeds or explants, facilitating applications in plant propagation, genetic engineering, and the conservation of rare or endangered species. While PTC offers significant advantages, including the ability to regenerate plant material, conserve cultures year-round, and provide a steady supply of uniform biomass, it also presents several challenges. These include high operational costs, the need for technical expertise, genetic instability, and contamination risks. Unlike natural field growth conditions, PTC relies on nutritional inputs. Issues such as delayed callus formation, the potential for undesirable traits, and susceptibility to water loss and hyperhydricity further complicate its application. Despite these challenges, PTC plays a crucial role in micropropagation, producing medicinal and commercial compounds, improving crops, and cultivating high-quality disease-free plants. It also supports genetic, morphological, and pathological research on individual cells and aids in developing crops with enhanced traits through beneficial mutations and transgenic modifications. Effective PTC operations require well-maintained laboratories equipped for sterilization, media preparation, aseptic transfer, and environmental control, along with skilled personnel to ensure successful outcomes.
(b) The significance of plant tissue culture (PTC) technology relative to ecological restoration and plant conservation.
Examining the relevance of plant tissue culture (PTC) technology vis-à-vis ecological restoration and plant conservation necessitates a nuanced analysis of their distinct roles in conservation efforts. Both fields synergistically contribute to tackling the multifaceted challenges posed by biodiversity loss and ecosystem degradation. However, while both ecological restoration and plant conservation stand as essential tenets of conservation science, aimed at ameliorating biodiversity decline and revitalizing ecosystems, they diverge significantly in their core objectives, methodologies, and extents of impact.
Ecological restoration aims to restore degraded ecosystems by endeavoring to return them to their natural states or functional equivalents while prioritizing ecosystem health, resilience, and biodiversity enhancement. Achieving this requires proactive measures such as reintroducing native species, managing invasive species, and restoring habitat integrity through techniques like reforestation and wetland rehabilitation. The scale of restoration projects varies widely, ranging from local-scale initiatives to expansive landscape-level interventions. Given the complexity of ecosystems and the extent of degradation, these projects often span decades or even centuries to achieve the desired outcomes. Crucially, successful ecological restoration relies on collaborative efforts among a diverse array of stakeholders, including scientists, land managers, government agencies, and local communities, underscoring the necessity of collective responsibility and a multidisciplinary approach to achieving sustainable restoration outcomes.
Conversely, plant conservation is a multifaceted discipline that aims to protect plant species, populations, and habitats, thereby preventing extinction and preserving genetic diversity. Through a combination of in situ and ex situ conservation methods, such as protecting plants within their natural habitats and preserving them outside their native environments in botanical gardens or seed banks, plant conservationists deploy a diverse array of strategies. These strategies encompass habitat protection, species reintroductions, captive breeding, and genetic management, tailored to meet the unique needs of individual species or ecosystems. Plant conservation efforts span a spectrum, ranging from targeted conservation of specific species to broader initiatives aimed at conserving entire ecosystems and their associated plant communities. Conservation actions encompass both short-term interventions to address immediate threats and long-term strategies aimed at ensuring the sustained viability of plant populations over time. Collaboration among a wide range of stakeholders, including botanists, conservation biologists, government agencies, NGOs, botanical gardens, and local communities, is paramount to driving successful plant conservation initiatives. This collaborative approach enables the effective prioritization of species and habitats, as well as the implementation of comprehensive conservation strategies to safeguard plant diversity.
Overall, plant tissue culture technology is a cornerstone in plant conservation efforts, offering invaluable tools and methodologies for the preservation, propagation, and genetic enhancement of endangered and threatened plant species. Through tissue culture techniques, conservationists can efficiently multiply limited plant material, safeguarding genetic diversity and bolstering the resilience of at-risk populations. Moreover, tissue culture facilitates the rescue and propagation of rare and endangered plant species that are challenging to propagate using conventional methods. By enabling the rapid multiplication of plant material under controlled conditions, tissue culture technology significantly enhances the ex situ conservation of endangered plants in botanical gardens, seed banks, and other conservation repositories. Furthermore, tissue culture enables the genetic improvement of plant species through methods like somatic embryogenesis and genetic transformation, providing ways to improve traits such as resistance to disease and the ability to adapt to new environments. Ultimately, the integration of plant tissue culture technology into conservation strategies contributes decisively to the long-term survival and conservation of endangered and threatened plant species, safeguarding biodiversity for future generations.
Mitigating and adapting to climate change entails substantial financial commitments for numerous countries. Governments worldwide face numerous political, sociocultural, and economic challenges, and as a result, trade-offs are frequently made at the expense of climate mitigation and adaptation measures (Figure 8). There is no global harmonized framework for reporting climate-related risk, which allows for significant arbitrage, particularly in the private sector. There is a lack of ambition in the Nationally Determined Contributions (NDCs), and even when certain parties display ambition in their NDCs, the execution often falls below expectations at the national level. Climate funding remains inadequate and fails to meet the requirements for driving substantial transformation.
Renewables (such as solar, geothermal, and wind) make economic sense for only about 10 to 15% of the world’s countries. Climate projections for the twenty-first century are highly consistent across models and iterations of the IPCC assessment reports. However, because feedback loops are not discounted in these projections, current models should be regarded as conservative and lenient, because even if zero-emissions were hypothetically achievable immediately, it would take time for the feedback loops to subside before the true gains of a zero-emissions economy could be obtained. Given all of these challenges, it makes more sense to invest in cutting-edge green technologies that are scalable, accessible, and not geographically constrained.
Current conservation measures are marginally effective, but it appears to be a battle of attrition, given that drivers of plant diversity loss, such as climate change and unsustainable anthropogenic activities, are becoming more intense as the human population grows rapidly (Figure 8). The mobilization of funds for conservation action is a major factor that can tip the balance in the right direction. The challenge, however, is that financing is still a major issue that will require a four-fold increase by 2050 (i.e. 133 billion USD annually based on 2020 but needs to be at least 536 billion USD annually by 2050). The insufficient allocation of funds to deter illegal trade in endangered wildlife species hinders the effectiveness of CITES, and the transition into and out of the blacklisting system is slow (Table 2). The national biodiversity strategic action plan is poorly implemented in most countries, and it is generally not a priority due to the many competing interests for which the national budget is allocated. Unsustainable land-use practices continue to be a major source of concern around the world, owing primarily to rapid population growth and the extension of agricultural land into forests.
Given all the available information, it can be concluded that technology is the sole solution to the current situation. However, given the current baseline, it will require a massive leap in technological innovation to “green the entire global economy.” Furthermore, even if we decide to be optimistic and invest heavily in research and development for better green technologies, it is difficult to predict how long it will take to achieve the desired outcome. Thus, it is prudent to invest in R&D while also exploring other options, as highlighted at the recent UN Biodiversity Conference (CBD COP15 (Part I), 2021) in Kunming, China, where parties adopted the non-binding Kunming Declaration. The incorporation of the “Post-2020 Global Biodiversity Framework” is a component of this novel strategy aimed at preserving life on Earth. This framework will delineate the specific actions that countries need to undertake, both alone and collaboratively, in the coming decade and beyond, to steer humanity towards the CBD’s overarching goal of “living in harmony with nature” by 2050. An essential aspect of this new framework is the stipulation that protected areas and other effective area-based conservation measures must encompass a minimum of 30% of the Earth’s surface by 2030. This criterion also acknowledges and respects the rights and contributions of indigenous peoples and local communities. It is essential to incorporate all significant areas for biodiversity, such as key biodiversity areas (KBAs), within the 30% target. Measures should be implemented to guarantee the connection of habitats (Post-2020 Global Biodiversity Framework, 2022).
Globally, less than 15% of the land is designated as a protected area, approximately 17% of global forests are protected, and less than 50% of important sites for terrestrial biodiversity are protected (Ritchie and Roser, 2021). Plant tissue culture has been overlooked in global conservation policy for far too long, despite the enormous evolution it has undergone over the last few decades. Perhaps this is why, despite increased funding, current conservation efforts have lagged in many ways. To successfully implement the 30-by-30 post-2020 global biodiversity framework, it is crucial to secure substantial financial resources from all parties involved. Additionally, it is essential to align climate and biodiversity policies at the regional level (Box 1). Moreover, it is of utmost importance to establish an advanced plant tissue culture laboratory and its complementary elements and units to support protected area, such as national parks, biosphere reserves, and wildlife sanctuaries. This advanced technology will greatly assist in the protection, restoration, and conservation of plant diversity (Figure 1, Boxes 6, 7). The market size of the global plant tissue culture industry was approximately $383 million in 2020. It is projected to expand to over $900 million by 2030, with a compound annual growth rate (CAGR) of 8.5% from 2021 to 2030 (Allied Market Research, 2022). These figures provide a clear indication of the sector’s growth by 2030 and strongly suggest the need to scale up and increase public-private partnerships in the coming years. Plant tissue culture companies already have the technical capabilities to be potential partners in plant conservation, and these capabilities are expected to grow even more. Governments must fund the establishment of plant tissue culture facilities to support each protected area. Tourism must evolve into a more reliable conservation ally by integrating biodiversity into its core practices and strategies. Conserving soil biodiversity is critical for sustaining the multitude of ecosystem functions that soils provide for both natural environments and human societies. Kew Gardens is a United Kingdom public organization sponsored by the Department for Environment. Its main objective is to halt the extinction problem and actively contribute to the establishment of a world where nature is safeguarded, appreciated, and sustainably governed. So far, the Kew Gardens model for ex situ and in situ conservation is the best in the world and should serve as a model for other governments worldwide. The Kew model, however, should not be limited to Kew. While the Kew model has demonstrated efficacy on a bilateral level, its application on a multilateral scale, particularly within the proposed Kew-Wide Mechanism (KWM) for global adoption as a solution to climate and plant diversity loss, remains untested. This study establishes the framework for a global initiative, necessitating validation through a series of pilot programmes (Figure 2). The Kew-Wide Mechanism is the most comprehensive plan, taking into account the climate and plant diversity emergencies and emphasizing the need for global and national coordination in order to achieve the 30-by-30 terrestrial biome target under the new global biodiversity conservation framework by 2050 and beyond.
This review underscores the intricate relationship between climate change and biodiversity loss, elucidating both the individual and correlated driving forces and the multifaceted policy challenges hindering progress towards ambitious goals such as achieving a net-zero emissions economy by 2050 and the overarching post-2020 biodiversity framework goals. Despite the commitment laid out in the Paris Agreement nearly a decade ago, challenges persist, including a lack of solidarity at the national level, amplified global conflicts resulting in a shift in national priorities towards defense spending. Moreover, the challenge is compounded by the scarcity of capital, influenced by macroeconomic factors such as rising interest rates and demographic shifts worldwide, particularly in affluent nations. For instance, as people retire, they often tap into their retirement savings or pensions to sustain their post-retirement lifestyle, resulting in a reduction of available capital for investment in sectors like renewable energy. Furthermore, retirees tend to favor conservative investments with predictable returns over renewable energy projects, which necessitate substantial upfront investment and boast longer payback periods. Additionally, retirees may liquidate assets such as stocks or bonds to finance retirement, thereby further depleting available capital. Lastly, as the workforce diminishes due to retirements, contributions to investment vehicles decline, further constricting capital availability for renewable energy and other critical sectors.
In addressing these challenges, decarbonization of energy emerges as a pivotal solution. However, its realization remains uncertain due to insufficient R&D financing and associated financial risks. Additionally, the practicality and scalability of carbon capture and storage technology pose significant obstacles for most countries. Furthermore, the transportation sector ranks as the second-largest contributor to greenhouse gas emissions, following the energy industry. Mitigating its environmental impact is also a paramount concern for governments, industry stakeholders, and researchers worldwide. Battery technologies, although increasingly popular in electric vehicles (EVs), continue to encounter persistent challenges that hinder widespread acceptance. Limitations such as restricted driving range, high maintenance costs, battery-related issues (including recycling challenges), and inadequate charging infrastructure remain prevalent. Moreover, concerns regarding the initial high purchase cost and the broader acceptance of EV technology remain significant barriers. Despite their promise, physicochemical limitations constrain the advancement of lithium-ion batteries, and prolonged charging times deter potential consumers. Addressing these challenges necessitates a focus on innovative battery technologies capable of overcoming existing limitations and driving EV adoption. These innovative solutions should aim to enhance battery lifespan, bolster safety features, and facilitate faster charging capabilities, thereby addressing concerns regarding maintenance costs and extended charging durations. By fostering innovation in battery technology, the industry can pave the way for more accessible and widely accepted electric vehicles, ultimately mitigating the obstacles currently impeding widespread EV adoption.
Addressing the dual challenges of climate change and biodiversity loss necessitates innovative financial mechanisms, advanced technological development, and enhanced collaboration among diverse stakeholders. Therefore, effective solutions must be pursued through concerted efforts at both global and national levels. Moving forward, policymakers must prioritize investments in R&D for revolutionary energy technologies and foster international cooperation to overcome financial barriers. Moreover, promoting renewable energy projects and incentivizing investor participation are critical steps towards achieving decarbonization goals. Addressing the challenges associated with carbon capture and storage technology requires increased research efforts and strategic investments to enhance scalability and affordability. Ultimately, achieving a net zero emissions economy and biodiversity conservation goals requires a coordinated, multidimensional approach that integrates climate action, biodiversity conservation, and sustainable development. By addressing the identified challenges head-on and implementing targeted policy interventions, nations can pave the way for a more resilient and sustainable future.
The pivotal role of plant biomes as the highest carbon sink underscores the urgent need for their conservation. Unlike many climate mitigation and adaptation measures that pose financial and logistical challenges for most countries, plant conservation measures offer a more accessible pathway to address climate change impacts. Given that greenhouse gases are the primary cause of climate change, which in turn drives the decline in plant diversity, addressing anthropogenic drivers is crucial (Figure 7). By promoting sustainable practices and restoring plant ecosystems, a positive feedback loop can be established, enhancing the capacity of plants to sequester carbon emissions. Conventional methods for plant production and conservation, however, are inadequate to address the magnitude of current challenges. Fortunately, advancements in plant tissue culture technology offer a promising solution. Over the past decades, this technology has evolved significantly, enabling mass production and conservation of plants on a scale previously unattainable. Furthermore, plant tissue culture facilitates the production of high-quality plant stock and elite cultivars tailored to thrive in diverse environmental conditions (Figure 1, Box 8). To capitalize on the potential of plant tissue culture technology, policymakers must prioritize its integration into conservation strategies and allocate resources accordingly. By leveraging this cutting-edge technology, policymakers and conservationists can establish robust plant conservation programmes capable of mitigating climate change impacts and safeguarding biodiversity.
The existing conservation strategies exhibit a concerning degree of passivity, relying heavily on the goodwill of national governments. While this approach is commendable, it falls short of effectively addressing the complex conservation challenges encountered nowadays (Figure 8). Moreover, protected areas, often hailed as bastions of conservation, are not impervious to unsustainable practices such as resource overexploitation for economic and domestic purposes. Furthermore, certain protected areas are disproportionately impacted by climate change due to their geographical location, exacerbating the decline in plant diversity within these regions (Figure 4). The Convention on International Trade in Endangered Species of Wild Fauna and Flora (CITES) faces significant challenges in its current form (Table 2). Financially outmatched by illegal trade, CITES struggles with issues of traceability, listing inadequacies, and inadequate multilateral coordination. These shortcomings undermine the efficacy of CITES in combating the illegal wildlife trade and protecting endangered plant species. Addressing these policy challenges requires proactive measures to enhance conservation efforts and strengthen regulatory frameworks. Policymakers must prioritize the development and implementation of comprehensive regulatory frameworks that not only curb illegal trade but also promote sustainable practices within protected areas. Strengthening international cooperation and coordination mechanisms is paramount to enhance the effectiveness of initiatives like CITES and ensure their relevance in addressing contemporary conservation challenges. This may involve streamlining listing procedures, improving funding mechanisms, and fostering greater collaboration among member states. Furthermore, investing in innovative technologies and data-driven approaches can bolster conservation efforts by improving monitoring, enforcement, and traceability. Embracing digital solutions and harnessing the power of big data can enhance our understanding of biodiversity trends and inform targeted interventions.
By funding and establishing KWM as a central entity tasked with overseeing plant conservation goals, including the 30-by-30 targets outlined in the post-2020 biodiversity conservation framework, nations can bridge the gap between global and national priorities. At the heart of KWM lies the SEGK, comprising technocrats confirmed by parliament or any other representative government body rather than political figures. These experts act as the crucial link between government officials and international partners, facilitating the coordination and effective execution of conservation objectives. This composition ensures that technical expertise guides objective decision-making, free from unwarranted political influence. The implementation of KWM at the national level offers several benefits. Firstly, it aligns national conservation efforts with global agendas, promoting coherence and collaboration in achieving shared goals. Secondly, by leveraging technocratic expertise, KWM ensures that decisions are informed by scientific evidence and best practices, enhancing the efficacy of conservation strategies. Additionally, government funding provides the necessary resources to support KWM’s initiatives, ensuring their sustainability and long-term impact. Moreover, KWM serves as a platform for fostering partnerships and knowledge exchange among stakeholders at all levels. By engaging with diverse actors, including government agencies, research institutions, civil society organizations, and local communities, KWM promotes inclusivity and ownership of conservation initiatives. This participatory approach enhances the legitimacy and effectiveness of plant conservation efforts, contributing to the broader objectives of biodiversity conservation. In conclusion, the implementation of KWM at the national level represents a strategic approach to addressing the challenges of plant conservation while advancing global solidarity. By harnessing technocratic expertise, government funding, and inclusive governance mechanisms, KWM offers a pathway towards achieving ambitious conservation targets and safeguarding plant diversity for future generations.
The successful implementation of KWM initiative necessitates the establishment of a sufficient number of state-of-the-art plant tissue culture laboratories and complementary infrastructure strategically located across national territories or within designated protected areas. These facilities are essential for meeting the demands and imperatives associated with mass plant production and the conservation of endangered species. By strategically positioning these laboratories, logistical challenges can be minimized, thereby enhancing the efficiency and effectiveness of conservation efforts. Furthermore, plant tissue culture facilities have the potential to serve multiple purposes beyond conservation. In addition to their primary role in mass plant production, these facilities can also cater to commercial interests, particularly during periods of minimal demand for the propagation of endangered plant species. By diversifying their services, plant tissue culture laboratories can generate revenue streams that support their ongoing operation and maintenance, ensuring their long-term sustainability. Moreover, these facilities can serve as hubs for vocational training and entrepreneurship development, providing valuable opportunities for skill development and capacity building. Through structured training programmes, participants can acquire specialized skills in plant tissue culture techniques, entrepreneurship, and research methodologies, fostering the growth of a highly skilled workforce in the field of plant biotechnology and conservation. Furthermore, the affiliation of plant tissue culture laboratories with higher education institutions offers additional benefits. By collaborating with academic institutions, these facilities can contribute to the advancement of scientific knowledge through research and innovation. Additionally, partnerships with universities provide opportunities for student engagement and mentorship, nurturing the next generation of conservation scientists and biotechnologists.
The implementation of the KWM initiative presents a unique opportunity to engage the public, raise awareness, and foster behavioral changes regarding climate change and biodiversity conservation. By leveraging its platform and resources, KWM can serve as a catalyst for public education and sensitization efforts, empowering individuals to take meaningful action to address pressing environmental challenges. One of the key strengths of KWM lies in its ability to champion inclusiveness and collaboration among all stakeholders in addressing current environmental challenges. By bringing together diverse groups, including government agencies, conservation organizations, local communities, and the private sector, KWM promotes a holistic approach to conservation that integrates various perspectives and expertise. This inclusive approach not only enhances the effectiveness of conservation efforts but also fosters a sense of ownership and responsibility among all stakeholders. Furthermore, KWM can play a pivotal role in promoting behavioral changes that support climate change mitigation and biodiversity conservation. Through targeted outreach campaigns, educational programmes, and community engagement initiatives, KWM can raise awareness about the importance of preserving natural ecosystems, reducing carbon emissions, and adopting sustainable practices. By empowering individuals to make informed choices and take proactive steps to protect the environment, KWM contributes to a culture of environmental stewardship and collective action. By championing inclusivity, fostering collaboration, and empowering individuals and communities, KWM can catalyze a collective response to environmental challenges, leading to more resilient ecosystems, healthier communities, and a more sustainable future for all.
The 21st century is an unprecedented era in human history that demands resolute action to counteract the decline in plant diversity driven by climate change and anthropogenic influences. Although commendable progress has been made in mitigating climate change, adapting to its effects, and conserving biodiversity, these achievements represent only the first steps towards our overarching objectives. Given the scale of the challenge, they can be viewed as initial steps towards our desired targets, which include reducing greenhouse gas emissions and transitioning to a net-zero economy by 2050. Moreover, the milestone goals outlined in the 30-by-30 targets under the post-2020 biodiversity framework must be met by 2030, paving the way for broader objectives by 2050 (Box 1). These intertwined goals underscore the symbiotic relationship between forests, encompassing biodiversity as a whole, and their critical role as the “lungs” of the Earth, serving as vital carbon sinks. To achieve these ambitious yet realistic objectives, the global community needs to work together and address the weaknesses and limitations that have impeded progress (Figures 6–8). Moreover, it is imperative to acknowledge that alternative approaches, which may have yet to be initially considered, may serve as the linchpin for achieving the desired targets. This recognition underscores the importance of flexibility, adaptability, and innovation in navigating challenges and optimizing outcomes.
In light of this perspective, our review article proposes the implementation of a novel global policy framework termed the Kew-Wide Mechanism (KWM). Drawing inspiration from the successful Kew Gardens model for plant conservation, the KWM holds promise for making a profound positive impact on a global scale. By bridging the gap between global and national priorities for plant conservation, the KWM endeavors to advance humanity’s collective endeavor of safeguarding plant diversity, thereby contributing to a sustainable future for all (Figure 2).
The KWM is driven by two primary forces. Firstly, the SEGK stands as the foundational driving force within the KWM, comprising predominantly national experts in plant conservation and related fields. Equipped with advanced technical expertise and executive authority, these experts collaborate closely with external advisors from prestigious institutions like Kew Gardens and other key global partners, providing invaluable monitoring and advisory support. Serving as the bridge between governments and critical international partners such as IUCN and CBD, the SEGK ensures stakeholder accountability by facilitating seamless coordination and collaboration to achieve collective targets. Secondly, the integration of advanced plant tissue culture laboratories (PTCs) within the KWM underscores its innovative and practical approach, marking the first global-scale utilization of this cutting-edge technology for plant conservation. This strategic incorporation of PTCs represents a pioneering effort to confront the urgent challenges of plant diversity decline in the contemporary global landscape.
To effectively preserve biodiversity and mitigate climate change, a comprehensive approach involving policy reforms and collaborative efforts is imperative. CITES requires restructuring to enhance its effectiveness in biodiversity conservation, necessitating policy modifications, increased funding, strengthened enforcement mechanisms, and enhanced international cooperation. The tourism industry must adopt sustainable practices to align with conservation goals and promote responsible tourism. Public-private partnerships must be scaled up to advance conservation efforts and enhance ecological resilience. While the Kew-Wide Mechanism (KWM) shows promise as a coordination tool for plant diversity preservation, its efficacy requires validation through dedicated financial resources for pilot studies. These investigations will assess feasibility, identify challenges, and refine implementation strategies to ensure global effectiveness. The KWM represents a comprehensive strategy addressing policy gaps, fostering collaboration, and fostering innovation necessary for enhancing conservation efforts and securing biodiversity for future generations (Figure 11).
DM: Conceptualization, Data curation, Formal analysis, Funding acquisition, Investigation, Methodology, Project administration, Resources, Software, Supervision, Validation, Visualization, Writing – original draft, Writing – review & editing. OP: Investigation, Supervision, Validation, Writing – review & editing. AK: Investigation, Writing – review & editing. WV: Investigation, Supervision, Validation, Writing – review & editing.
The author(s) declare that no financial support was received for the research, authorship, and/or publication of this article.
The corresponding author extends heartfelt gratitude to Chief MOSOH Paul Tandong and Chieftess Ateyim Espe MOSOH Ostensia Nkeng of PINYIN (Santa, North-West Region, Cameroon) for their tremendous support. The corresponding author extends heartfelt gratitude to Mr. Tetu Acha Samuel (MD, USA) for his prompt and invaluable assistance with procurement appropriation. We express our sincere gratitude to the USDA National Institute of Food and Agriculture for their invaluable support through the Hatch project 7001563. Additionally, we express our sincere appreciation to the reviewer(s) for their diligent review of our work, and for their insightful comments and suggestions, which have significantly enriched the quality of our manuscript.
The authors declare that the research was conducted in the absence of any commercial or financial relationships that could be construed as a potential conflict of interest.
All claims expressed in this article are solely those of the authors and do not necessarily represent those of their affiliated organizations, or those of the publisher, the editors and the reviewers. Any product that may be evaluated in this article, or claim that may be made by its manufacturer, is not guaranteed or endorsed by the publisher.
The Supplementary Material for this article can be found online at: https://www.frontiersin.org/articles/10.3389/fcosc.2024.1383370/full#supplementary-material
Abdin M. Z., Kiran U., Kamaluddin, Ali A. (Eds.). (2017). Plant Biotechnology: Principles and Applications (Singapore: Springer), 1–392. doi: 10.1007/978-981-10-2961-5
Agbor D. T. (2022). Convention on biological diversity post 2020 target: A critical analyses of the threat posed by 30 X 30 target, devoid of a human rights approach towards conservation to land rights in developing countries: case study Cameroon. Am. J. Environ. Climate 1, 92–99. doi: 10.54536/ajec.v1i2
Aggarwal K., Puri K., Joshi R., Garkoti S. C. (2020). Biosphere reserves: A solution reconciling biodiversity conservation with its sustainable use. ESSENCE – International Journal for Environmental Rehabilitation and Conservation.
Ajonina G. N. (2022). “Cameroon Mangroves: Current Status, Uses, Challenges, and Management Perspectives,” in Mangroves: Biodiversity, Livelihoods and Conservation. Eds. Das, Pullaiah S. C., Ashton E. C. (Singapore: Springer Nature), 565–609. doi: 10.1007/978-981-19-0519-3_21
Alatalo J. M., Jägerbrand A. K., Molau U. (2014). Climate change and climatic events: Community-, functional- and species-level responses of bryophytes and lichens to constant, stepwise, and pulse experimental warming in an alpine tundra. Alpine Bot. 124, 81–91. doi: 10.1007/s00035-014-0133-z
Alatalo J. M., Jägerbrand A. K., Molau U. (2015). Testing reliability of short-term responses to predict longer-term responses of bryophytes and lichens to environmental change. Ecol. Indic. 58, 77–85. doi: 10.1016/j.ecolind.2015.05.050
Alexander J. M., Chalmandrier L., Lenoir J., Burgess T. I., Essl F., Haider S., et al. (2018). Lags in the response of mountain plant communities to climate change. Global Change Biol. 24, 563–579. doi: 10.1111/gcb.13976
Alfonso S., Gesto M., Sadoul B. (2021). Temperature increase and its effects on fish stress physiology in the context of global warming. J. Fish Biol. 98, 1496–1508. doi: 10.1111/jfb.14599
Alley R., Berntsen T., Bindoff N. L., Chen Z., Chidthaisong A., Friedlingstein P., et al. (2007). Corrections made as of February 5th 2007, Vol. 19.
Allied Market Research. (2022). Plant tissue culture market statistics | Industry forecast—2030 (Allied Market Research). Available online at: https://www.alliedmarketresearch.com/plant-tissue-culture-market-A14265.
Andermann T., Faurby S., Turvey S. T., Antonelli A., Silvestro D. (2020). The past and future human impact on mammalian diversity. Sci. Adv. 6, eabb2313. doi: 10.1126/sciadv.abb2313
Arora N. K., Mishra I. (2021). COP26: More challenges than achievements. Environ. Sustainability 4, 585–588. doi: 10.1007/s42398-021-00212-7
Attorre F., Abeli T., Bacchetta G., Farcomeni A., Fenu G., De Sanctis M., et al. (2018). How to include the impact of climate change in the extinction risk assessment of policy plant species? J. Nat. Conserv. 44, 43–49. doi: 10.1016/j.jnc.2018.06.004
Attuaquayefio D., Folib J. (2005). An overview of biodiversity conservation in ghana: Challenges and prospects. West Afr. J. Appl. Ecol. 7 (1).
Auvinen A.-P., Hildén M., Toivonen H., Primmer E., Niemelä J., Aapala K., et al. (2007). Evaluation of the Finnish national biodiversity action plan 1997-2005. Finnish Environment Institute. Available online at: https://helda.helsinki.fi/items/e4783c46-96ad-4112-b2a1-e32a9066539d (Accessed on July 31, 2024).
Bach E. M., Ramirez K. S., Fraser T. D., Wall D. H. (2020). Soil biodiversity integrates solutions for a sustainable future. Sustainability 12, 2662. doi: 10.3390/su12072662
Banholzer S., Kossin J., Donner S. (2014). The Impact of Climate Change on Natural Disasters. Reducing Disaster: Early Warning Systems For Climate Change. (Dordrecht: Springer), 21–49. doi: 10.1007/978-94-017-8598-3_2
Barnosky A. D., Matzke N., Tomiya S., Wogan G. O. U., Swartz B., Quental T. B., et al. (2011). Has the Earth’s sixth mass extinction already arrived? Nature 471, 51–57. doi: 10.1038/nature09678
Beckford C. L., Norman A. (2016). “Climate change and quality of planting materials for domestic food production: Tissue culture and protected agriculture,” in Globalization, Agriculture and Food in the Caribbean (Springer), 189–215. doi: 10.1057/978-1-137-53837-6
Beckline M., Manan A., Dominic N., Mukete N., Hu Y. (2022). Patterns and challenges of forest resources conservation in Cameroon. Open Access Library J. 9, Article 5. doi: 10.4236/oalib.1108683
Benton T. G., Bieg C., Harwatt H., Pudasaini R., Wellesley L. (2021). Food system impacts on biodiversity loss. Three levers for food system transformation in support of nature. (London: Chatham House), pp 1–75.
Bergholt D., Lujala P. (2012). Climate-related natural disasters, economic growth, and armed civil conflict. J. Peace Res. 49, 147–162. doi: 10.1177/0022343311426167
Bobo K. S., Aghomo F. F. M., Ntumwel B. C. (2015). Wildlife use and the role of taboos in the conservation of wildlife around the Nkwende Hills Forest Reserve; South-west Cameroon. J. Ethnobiol. Ethnomed. 11, 1–24. doi: 10.1186/1746-4269-11-2
Bolpagni R., Poikane S., Laini A., Bagella S., Bartoli M., Cantonati M. (2019). Ecological and conservation value of small standing-water ecosystems: A systematic review of current knowledge and future challenges. Water 11 (3), 402. doi: 10.3390/w11030402
Brei M., Pérez-Barahona A., Strobl E. (2016). Environmental pollution and biodiversity: Light pollution and sea turtles in the Caribbean. J. Environ. Economics Manage. 77, 95–116. doi: 10.1016/j.jeem.2016.02.003
Briscoe S. (2018). A review of the reporting of web searching to identify studies for Cochrane systematic reviews. Res. Synthesis Methods 9, 89–99. doi: 10.1002/jrsm.1275
Brooks T. M., Mittermeier R. A., da Fonseca G. A. B., Gerlach J., Hoffmann M., Lamoreux J. F., et al. (2006). Global biodiversity conservation priorities. Science 313 (5783), 58–61. doi: 10.1126/science.1127609
Burdon J. J., Zhan J. (2020). Climate change and disease in plant communities. PloS Biol. 18, e3000949. doi: 10.1371/journal.pbio.3000949
Cacciatori C., Czerepko J., Lech P. (2022). Long-term changes in bryophyte diversity of central European managed forests depending on site environmental features. Biodiversity Conserv. 31, 2657–2681. doi: 10.1007/s10531-022-02449-y
Cameroon NBSAPII. (2012). National biodiversity strategy and action plan version II (NBSAP II). Available online at: https://www.cbd.int/doc/world/cm/cm-nbsap-v2-en.pdf.
Caro T., Rowe Z., Berger J., Wholey P., Dobson A. (2022). An inconvenient misconception: Climate change is not the principal driver of biodiversity loss. Conserv. Lett. 15 (3), e12868. doi: 10.1111/conl.12868
CBD. (2020). Secretariat of the Convention on Biological Diversity, (2020) Global Biodiversity Outlook 5 – Summary for Policy Makers (Montréal).
CBD COP15. (Part I). (2021). Kunming declaration (CBD). Available online at: https://www.cbd.int/doc/c/c2db/972a/fb32e0a277bf1ccfff742be5/cop-15-05-add1-en.pdf.
CBD. (2021). First draft of the post-2020 global biodiversity framework. Available online at: https://www.cbd.int/doc/c/abb5/591f/2e46096d3f0330b08ce87a45/wg2020-03-03-en.pdf (Accessed July 31, 2024).
CBD. (2022). A strategy for plant conservation as part of the post 2020 global biodiversity framework. Available online at: https://www.bgci.org/wp/wp-content/uploads/2022/09/Paper-4.2.-GSPC_Briefing.pdf (Accessed July 31, 2024).
Ceballos G., Ehrlich P. R., Dirzo R. (2017). Biological annihilation via the ongoing sixth mass extinction signaled by vertebrate population losses and declines. Proceedings of the national academy of sciences, 114 (30), E6089–E6096. doi: 10.1073/pnas.1704949114
Challender D. W., Hinsley A., Milner-Gulland E. (2019). Inadequacies in establishing CITES trade bans. Front. Ecol. Environ. 17 (4), 199–200. doi: 10.1002/fee.2034
Chandana B., Nagaveni H., Lakshmana D., Kolakar S. S., Heena M. (2018). Role of plant tissue culture in micropropagation, secondary metabolites production and conservation of some endangered medicinal crops. J. Pharmacognosy Phytochem. 7, 246–251.
Chhatre A., Saberwal V. (2005). Political incentives for biodiversity conservation. Conserv. Biol. 19 (2), 310–317. doi: 10.1111/j.1523-1739.2005.00012.x
Christoffersen L. E. (1997). IUCN: A bridge-builder for nature conservation. Green globe Yearbook, 59–70.
Chu H., Gao G.-F., Ma Y., Fan K., Delgado-Baquerizo M. (2020). Soil microbial biogeography in a changing world: recent advances and future perspectives. MSystems 5, e00803–e00819. doi: 10.1128/mSystems.00803-19
CITES. (2022a). Appendices | CITES. Available online at: https://cites.org/eng/app/appendices.php (Accessed July 31, 2024).
CITES. (2022b). Convention on international trade in endangered species of wild fauna and flora | CITES. Available online at: https://cites.org/eng/disc/text.php (Accessed July 31, 2024).
CITES. (2022c). Financial budget 2020–2022. Available online at: https://cites.org/sites/default/files/eng/cop/18/Com_II/CoP18-Com-II-18-AppA.pdf (Accessed July 31, 2024).
CITES. (2022d). The structure of CITES | CITES. Available online at: https://cites.org/eng/disc/org.php (Accessed July 31, 2024).
CITES COP6. (1989). Proceedings of the sixth meeting of the Conference of the Parties. Convention on International Trade in Endangered Species of Wild Fauna and Flora, Ottawa, Canada, 12–24 July 1987. Secretariat of the Convention. Available at: https://portals.iucn.org/library/node/7157 [Accessed on August 20, 20].
Clarke M. (2008). “Venues for Contestation” within the United Nations framework convention on climate change: subsidiary bodies and working groups. Politics 5, 18–35.
Classen A. T., Sundqvist M. K., Henning J. A., Newman G. S., Moore J. A. M., Cregger M. A., et al. (2015). Direct and indirect effects of climate change on soil microbial and soil microbial-plant interactions: What lies ahead? Ecosphere 6, art130. doi: 10.1890/ES15-00217.1
Cooney R., Challender D. W., Broad S., Roe D., Natusch D. J. (2021). Think before you act: Improving the conservation outcomes of CITES listing decisions. Front. Ecol. Evol. 9. doi: 10.3389/fevo.2021.631556
Coulibaly T., Islam M., Managi S. (2020). The impacts of climate change and natural disasters on agriculture in African countries. Economics Disasters Climate Change 4, 347–364. doi: 10.1007/s41885-019-00057-9
Cowell C. R., Bullough L. A., Dhanda S., Harrison Neves V., Ikin E., Moore J., et al. (2022). Fortuitous alignment: The royal botanic gardens, kew and the sustainable development goals. Sustainability 14 (4), 2366. doi: 10.3390/su14042366
Cowie R. H., Bouchet P., Fontaine B. (2022). The Sixth Mass Extinction: Fact, fiction or speculation? Biol. Rev. 97, 640–663. doi: 10.1111/brv.12816
Crane P. R. (2000). The gran canaria declaration calling for a global program for plant conservation. Available online at: https://www.cbd.int/doc/meetings/cop/cop-05/information/cop-05-inf-32-en.pdf (Accessed July 31, 2024).
Crowley T. J. (2000). Causes of climate change over the past 1000 years. Science 289, 270–277. doi: 10.1126/science.289.5477.270
Dai Z., Zhang J., Wang J. (2022). Ability of bryophytes to track areas of suitable climate depends on their habitat preferences. J. Systematics Evol. 61 (1), 227. doi: 10.1111/jse.12832
David F. (2002). Overview of CITES | animal legal & historical center. Available online at: https://www.animallaw.info/article/overview-cites (Accessed July 31, 2024).
Delgado-Baquerizo M., Guerra C. A., Cano-Díaz C., Egidi E., Wang J.-T., Eisenhauer N., et al. (2020a). The proportion of soil-borne pathogens increases with warming at the global scale. Nat. Climate Change 10, 550–554. doi: 10.1038/s41558-020-0759-3
Delgado-Baquerizo M., Reich P. B., Trivedi C., Eldridge D. J., Abades S., Alfaro F. D., et al. (2020b). Multiple elements of soil biodiversity drive ecosystem functions across biomes. Nat. Ecol. Evol. 4, 210–220. doi: 10.1038/s41559-019-1084-y
Désamoré A., Laenen B., Stech M., Papp B., Hedenäs L., Mateo R. G., et al. (2012). How do temperate bryophytes face the challenge of a changing environment? Lessons from the past and predictions for the future. Global Change Biol. 18, 2915–2924. doi: 10.1111/j.1365-2486.2012.02752.x
de Souza A. C., Prevedello J. A. (2020). The importance of protected areas for overexploited plants: Evidence from a biodiversity hotspot. Biol. Conserv. 243, 108482. doi: 10.1016/j.biocon.2020.108482
Dhanda S., Williams C., Cowell C. (2019). Kew and the global strategy for plant conservation. R. Botanic Gardens Kew 76.
Donatelli M., Magarey R. D., Bregaglio S., Willocquet L., Whish J. P. M., Savary S. (2017). Modelling the impacts of pests and diseases on agricultural systems. Agric. Syst. 155, 213–224. doi: 10.1016/j.agsy.2017.01.019
DowntoEarth. (2021). World wildlife day: Cameroon’s rich biodiversity face innumerable threats. Available online at: https://www.cbd.int/kb/record/newsHeadlines/127443?Subject=ENDS.
Dubey A., Malla M. A., Khan F., Chowdhary K., Yadav S., Kumar A., et al. (2019). Soil microbiome: A key player for conservation of soil health under changing climate. Biodiversity Conserv. 28, 2405–2429. doi: 10.1007/s10531-019-01760-5
Dudley N., Alexander S. (2017). Agriculture and biodiversity: A review. Biodiversity 18, 45–49. doi: 10.1080/14888386.2017.1351892
Dumenu W. K. (2019). Assessing the impact of felling/export ban and CITES designation on exploitation of african rosewood (Pterocarpus erinaceus). Biol. Conserv. 236, 124–133. doi: 10.1016/j.biocon.2019.05.044
Eichenberg D., Bowler D. E., Bonn A., Bruelheide H., Grescho V., Harter D., et al. (2021). Widespread decline in Central European plant diversity across six decades. Global Change Biol. 27, 1097–1110. doi: 10.1111/gcb.15447
Ellis E. C., Klein Goldewijk K., Siebert S., Lightman D., Ramankutty N. (2010). Anthropogenic transformation of the biomes 1700 to 2000: Anthropogenic transformation of the biomes. Global Ecol. Biogeogr. 19 (5), 589–606. doi: 10.1111/j.1466-8238.2010.00540.x
Evans M. C. (2021). Re-conceptualizing the role(s) of science in biodiversity conservation. Environ. Conserv. 48 (3), 151–160. doi: 10.1017/S0376892921000114
Falkner R. (2016). The Paris Agreement and the new logic of international climate politics. Int. Affairs 92, 1107–1125. doi: 10.1111/1468-2346.12708
Fang J., Lau C. K. M., Lu Z., Wu W., Zhu L. (2019). Natural disasters, climate change, and their impact on inclusive wealth in G20 countries. Environ. Sci. pollut. Res. 26, 1455–1463. doi: 10.1007/s11356-018-3634-2
Foden W. B., Young B. E., Akçakaya H. R., Garcia R. A., Hoffmann A. A., Stein B. A., et al. (2019). Climate change vulnerability assessment of species. WIREs Climate Change 10, e551. doi: 10.1002/wcc.551
Fokunang C., Ndikum V., Tabi O., Jiofack R., Ngameni B., Guedje N., et al. (2011). Traditional medicine: Past, present and future research and development prospects and integration in the National Health System of Cameroon. Afr. J. Traditional Complementary Altern. Medicines 8, 284–295. doi: 10.4314/ajtcam.v8i3.65276
Foster S., Wiswedel S., Vincent A. (2016). Opportunities and challenges for analysis of wildlife trade using CITES data - seahorses as a case study: ANALYSIS OF WILDLIFE TRADE USING CITES DATA. Aquat. Conservation: Mar. Freshw. Ecosyst. 26 (1), 154–172. doi: 10.1002/aqc.2493
Frick W. F., Kingston T., Flanders J. (2020). A review of the major threats and challenges to global bat conservation. Ann. New York Acad. Sci. 1469, 5–25. doi: 10.1111/nyas.14045
Fukushima C. S., Tricorache P., Toomes A., Stringham O. C., Rivera-Téllez E., Ripple W. J., et al. (2021). Challenges and perspectives on tackling illegal or unsustainable wildlife trade. Biol. Conserv. 263, 109342. doi: 10.1016/j.biocon.2021.109342
García-Valdés R., Bugmann H., Morin X. (2018). Climate change-driven extinctions of tree species affect forest functioning more than random extinctions. Diversity and Distributions 24 (7), 906–918. doi: 10.1111/ddi.12744
Geisen S., Wall D. H., van der Putten W. H. (2019). Challenges and opportunities for soil biodiversity in the anthropocene. Curr. Biol. 29, R1036–R1044. doi: 10.1016/j.cub.2019.08.007
Gosline G., Cheek M., Onana J. M., Tchatchouang E. N., Burgt X. M., van der, MacKinnon L., et al. (2022). Uvariopsis dicaprio (Annonaceae) a new tree species with notes on its pollination biology, and the Critically Endangered narrowly endemic plant species of the Ebo Forest, Cameroon. PeerJ 10, e12614. doi: 10.7717/peerj.12614
Gray C. L., Mueller V. (2012). Natural disasters and population mobility in Bangladesh. Proc. Natl. Acad. Sci. 109, 6000–6005. doi: 10.1073/pnas.1115944109
Grenyer R., Orme C. D. L., Jackson S. F., Thomas G. H., Davies R. G., Davies T. J., et al. (2006). Global distribution and conservation of rare and threatened vertebrates. Nature 444 (7115), 93–96. doi: 10.1038/nature05237
Guerra C. A., Rosa I. M. D., Valentini E., Wolf F., Filipponi F., Karger D. N., et al. (2020). Global vulnerability of soil ecosystems to erosion. Landscape Ecol. 35, 823–842. doi: 10.1007/s10980-020-00984-z
Gupta R., Asthana A. K. (2016). Diversity and distribution of liverworts across habitats and altitudinal gradient at Pachmarhi Biosphere Reserve (India). Plant Sci. Today 3, 354–359. doi: 10.14719/pst.2016.3.4
Gupta V., Roper M., Thompson J., Pratley J., Kirkegaard J. (2020). Harnessing the benefits of soil biology in conservation agriculture. Australian Agriculture, 237–253.
Hale T. (2016). All hands on deck”: the paris agreement and nonstate climate action. Global Environ. Politics 16, 12–22. doi: 10.1162/GLEP_a_00362
Hallmann C. A., Sorg M., Jongejans E., Siepel H., Hofland N., Schwan H., et al. (2017). More than 75 percent decline over 27 years in total flying insect biomass in protected areas. PloS One 12, e0185809. doi: 10.1371/journal.pone.0185809
Hallmann C. A., Ssymank A., Sorg M., de Kroon H., Jongejans E. (2021). Insect biomass decline scaled to species diversity: General patterns derived from a hoverfly community. Proc. Natl. Acad. Sci. 118, e2002554117. doi: 10.1073/pnas.2002554117
Hao J., Chu L. M. (2021). Short-term detrimental impacts of increasing temperature and photosynthetically active radiation on the ecophysiology of selected bryophytes in Hong Kong, Southern China. Global Ecol. Conserv. 31, e01868. doi: 10.1016/j.gecco.2021.e01868
Harfoot M., Glaser S. A. M., Tittensor D. P., Britten G. L., McLardy C., Malsch K., et al. (2018). Unveiling the patterns and trends in 40 years of global trade in CITES-listed wildlife. Biol. Conserv. 223, 47–57. doi: 10.1016/j.biocon.2018.04.017
He X., He K. S., Hyvönen J. (2016). Will bryophytes survive in a warming world? Perspect. Plant Ecol. Evol. Systematics 19, 49–60. doi: 10.1016/j.ppees.2016.02.005
Heimstädt M. (2017). Openwashing: A decoupling perspective on organizational transparency. Technological Forecasting Soc. Change 125, 77–86. doi: 10.1016/j.techfore.2017.03.037
Heinrich S., Toomes A., Shepherd C. R., Stringham O. C., Swan M., Cassey P. (2022). Strengthening protection of endemic wildlife threatened by the international pet trade: The case of the australian shingleback lizard. Anim. Conserv. 25 (1), 91–100. doi: 10.1111/acv.12721
Hobbie S. E., Grimm N. B. (2020). Nature-based approaches to managing climate change impacts in cities. Philos. Trans. R. Soc. B: Biol. Sci. 375, 20190124. doi: 10.1098/rstb.2019.0124
Hoyte S. (2021). “Co-designing extreme citizen science projects in Cameroon: Biodiversity conservation led by local values and indigenous knowledge,” in Geographic Citizen Science Design: No One Left Behind (UCL Press, London). doi: 10.2307/j.ctv15d8174
Hughes A. C. (2021). Wildlife trade. Curr. Biol. 31 (19), R1218–R1224. doi: 10.1016/j.cub.2021.08.056
Humphreys A. M., Govaerts R., Ficinski S. Z., Nic Lughadha E., Vorontsova M. S. (2019). Global dataset shows geography and life form predict modern plant extinction and rediscovery. Nat. Ecol. Evol. 3, 1043–1047. doi: 10.1038/s41559-019-0906-2
Ibisch P. L. (2005). Biodiversity conservation in bolivia: History, trends and challenges. Environ. Issues Latin America Caribbean, 55–71.
Idowu A. A., Ayoola S. O., Opele A. I., Ikenweiwe N. B. (2011). Impact of climate change in Nigeria. Iranica J. Energy Environ. 2, 145–152. Available online at: https://ir.unilag.edu.ng/handle/123456789/6309.
Idowu P., Ibitoye D., Ademoyegun O. (2009). Tissue culture as a plant production technique for horticultural crops. Afr. J. Biotechnol. 8, 3782–3788.
IPBES Secretariat. (2022a). Glossary | IPBES secretariat. ‘Glossary: Biodiversity’. Available online at: https://ipbes.net/glossary/biodiversity (Accessed 12 March. 2022).
IPBES Secretariat. (2022b). Glossary | IPBES secretariat 2. ‘Glossary: Biodiversity Loss’. Available online at: https://ipbes.net/glossary/biodiversityloss (Accessed 12 March. 2022).
IPCC. (2021). Climate Change 2021: The Physical Science Basis. Contribution of Working Group I to the Sixth Assessment Report of the Intergovernmental Panel on Climate Change [Masson-Delmotte V., Zhai P., Pirani A., Connors S. L., Péan C., Berger S., et al (eds.)]. Cambridge University Press, Cambridge, United Kingdom and New York, NY, USA, In press, doi: 10.1017/9781009157896
IUCN. (2022). The IUCN red list of threatened species. Available online at: https://www.iucnredlist.org/en.
Ivetić V., Devetaković J. (2016). Reforestation challenges in Southeast Europe facing climate change. Reforesta 1, 178–220. doi: 10.21750/10.21750/REFOR.1.10.10
Jablonski D. (1986). Background and mass exnctions: the alternation of macroevolutionary regimes. Science 231, 129–133. doi: 10.1126/science.231.4734.129
Jepson P. (2019). Recoverable Earth: A twenty-first century environmental narrative. Ambio 48, 123–130. doi: 10.1007/s13280-018-1065-4
Johnson C. N., Balmford A., Brook B. W., Buettel J. C., Galetti M., Guangchun L., et al. (2017). Biodiversity losses and conservation responses in the Anthropocene. Science 356, 270–275. doi: 10.1126/science.aam9317
Kala C. P. (2013). Harvesting and supply chain analysis of ethnobotanical species in the pachmarhi biosphere reserve of India. Am. J. Environ. Prot. 1, 20–27. doi: 10.12691/env-1-2-2
Kala C. P. (2015a). Traditional farming system of Gond and other communities in the Pachmarhi Biosphere Reserve of India. Sci. Educ. 3, 140–145.
Kala C. P. (2015b). Forest structure and anthropogenic pressures in the Pachmarhi biosphere reserve of India. J. Forestry Res. 26, 867–874. doi: 10.1007/s11676-015-0083-3
Kemeuze V., Mapongmetsem P., Sonwa D., Fongnzossie E., Nkongmeneck B. (2015). Plant diversity and carbon stock in sacred groves of semi-arid areas of Cameroon: Case study of Mandara mountains. Int. J. Environ. 4, 308–318. doi: 10.3126/ije.v4i2.12659
Khandel A. K., Gantait S., Verma S. K. (2022). Optimization of growing conditions, substrate-types and their concentrations for acclimatization and post-acclimatization growth of in vitro-raised flame lily (Gloriosa superba L.) plantlets. Vegetos 35, 228–236. doi: 10.1007/s42535-021-00297-9
Khuroo A. A., Mehraj G., Muzafar I., Rashid I., Dar G. H. (2020). “Biodiversity conservation in jammu and kashmir state: Current status and future challenges,” in Biodiversity of the himalaya: Jammu and kashmir state. topics in biodiversity and conservation, vol. 18 . Eds. Dar G., Khuroo A. (Singapore: Springer). doi: 10.1007/978-981-32-9174-4_41
Kideghesho J. R. (2016). The elephant poaching crisis in tanzania: A need to reverse the trend and the way forward. Trop. Conserv. Sci. 9 (1), 369–388. doi: 10.1177/194008291600900120
Kobayakawa T. (2021). Country diagnostics for low carbon development: Can developing countries pursue simultaneous implementation of the Sustainable Development Goals and the Paris Agreement? BUSINESS STRATEGY Dev. 4, 294–312. doi: 10.1002/bsd2.159
Korwin S., Denier L., Lieberman S., Reeve R. (2019). Verification of legal acquisition under the CITES convention: The need for guidance on the scope of legality. J. Int. Wildlife Law Policy 22 (3), 274–304. doi: 10.1080/13880292.2019.1695718
Kovaka K. (2021). Climate change denial and beliefs about science. Synthese 198, 2355–2374. doi: 10.1007/s11229-019-02210-z
Küttim M., Laine A. M., Küttim L., Ilomets M., Robroek B. J. M. (2019). Winter climate change increases physiological stress in calcareous fen bryophytes. Sci. Total Environ. 695, 133867. doi: 10.1016/j.scitotenv.2019.133867
Kuyper J., Schroeder H. (2018). The Evolution of the UNFCCC. Annual Review of Environment and Resources 43 (1), 343–368. doi: 10.1146/annurev-environ-102017-030119
Lambertini M. (2020). Living planet report 2020: bending the curve of biodiversity loss. Available online at: http://www.deslibris.ca/ID/10104983.
Lanz B., Dietz S., Swanson T. (2018). The expansion of modern agriculture and global biodiversity decline: an integrated assessment. Ecol. Economics 144, 260–277. doi: 10.1016/j.ecolecon.2017.07.018
Lasserson T. J., Thomas J., Higgins J. P. (2019). “Starting a review,” in Cochrane handbook for systematic reviews of interventions. Eds. Higgins J. P. T., Thomas J., Chandler J., Cumpston M., Li T., M.J., Welch V. A. (John Wiley & Sons, Ltd), 1–12. doi: 10.1002/9781119536604.ch1
Lavelle K. C. (2020). “The challenges of multilateralism,” in The challenges of multilateralism (USA: Yale University Press). doi: 10.12987/9780300252323
Lavorgna A., Rutherford C., Vaglica V., Smith M. J., Sajeva M. (2018). CITES, wild plants, and opportunities for crime. Eur. J. Criminal Policy Res. 24 (3), 269–288. doi: 10.1007/s10610-017-9354-1
Le Saout S., Hoffmann M., Shi Y., Hughes A., Bernard C., Brooks T. M., et al. (2013). Protected areas and effective biodiversity conservation. Science 342 (6160), 803–805. doi: 10.1126/science.1239268
Lee B. P. Y.-H., Davies Z. G., Struebig M. J. (2017). Smoke pollution disrupted biodiversity during the 2015 El Niño fires in Southeast Asia. Environ. Res. Lett. 12, 094022. doi: 10.1088/1748-9326/aa87ed
Liew J. H., Kho Z. Y., Lim R. B. H., Dingle C., Bonebrake T. C., Sung Y. H., et al. (2021). International socioeconomic inequality drives trade patterns in the global wildlife market. Sci. Adv. 7 (19), eabf7679. doi: 10.1126/sciadv.abf7679
Lladó S., López-Mondéjar R., Baldrian P. (2017). Forest soil bacteria: diversity, involvement in ecosystem processes, and response to global change. Microbiol. Mol. Biol. Rev. 81, e00063–e00016. doi: 10.1128/MMBR.00063-16
Loyola-Vargas V. M., Ochoa-Alejo N. (2018). “An Introduction to Plant Tissue Culture: Advances and Perspectives,” in Plant Cell Culture Protocols, vol. 1815 . Eds. Loyola-Vargas V. M., Ochoa-Alejo N. (Springer, New York), 3–13. doi: 10.1007/978-1-4939-8594-4_1
Lynch P. T. (1999). “Tissue culture techniques in in vitro plant conservation,” in Plant conservation biotechnology (UK: CRC Press), 67–88.
Mak G. J. K., Song W. (2018). Transnational norms and governing illegal wildlife trade in china and japan: Elephant ivory and related products under CITES. Cambridge Rev. Int. Affairs 31 (5), 373–391. doi: 10.1080/09557571.2018.1530636
Malhi G. S., Kaur M., Kaushik P. (2021). Impact of climate change on agriculture and its mitigation strategies: A review. Sustainability 13, 1318. doi: 10.3390/su13031318
Marshall H., Drieschova A. (2018). Post-truth politics in the UK’s brexit referendum. New Perspect. 26, 89–105. doi: 10.1177/2336825X1802600305
Martin. (2019). UN report: nature’s dangerous decline “Unprecedented”; species extinction rates “Accelerating.” (United Nations Sustainable Development). Available online at: https://www.un.org/sustainabledevelopment/blog/2019/05/nature-decline-unprecedented-report/.
Martin A., McGuire S., Sullivan S. (2013). Global environmental justice and biodiversity conservation: Global environmental justice and biodiversity conservation. Geographical J. 179 (2), 122–131. doi: 10.1111/geoj.12018
Mason N., Ward M., Watson J. E. M., Venter O., Runting R. K. (2020). Global opportunities and challenges for transboundary conservation. Nat. Ecol. Evol. 4 (5), 694–701. doi: 10.1038/s41559-020-1160-3
Mathur A. (2009). CITES and livelihood: Converting words into action. J. Environ. Dev. 18 (3), 291–305. doi: 10.1177/1070496509337788
McCallum M. L. (2015). Vertebrate biodiversity losses point to a sixth mass extinction. Biodiversity Conserv. 24, 2497–2519. doi: 10.1007/s10531-015-0940-6
McMichael A. J. (2013). Globalization, climate change, and human health. New Engl. J. Med. 368, 1335–1343. doi: 10.1056/NEJMra1109341
Meinard Y. (2017). What is a legitimate conservation policy? Biol. Conserv. 213, 115–123. doi: 10.1016/j.biocon.2017.06.042
Mendelsohn R., Emanuel K., Chonabayashi S., Bakkensen L. (2012). The impact of climate change on global tropical cyclone damage. Nat. Climate Change 2, 205–209. doi: 10.1038/nclimate1357
Meng S. L. Y.-P. Z., Li C. J. (2022). Current situation, challenges and recommendation of biodiversity conservation standard system in china. Biodiversity Sci., 22117.
Mestanza-Ramón C., Henkanaththegedara S. M., Vásconez Duchicela P., Vargas Tierras Y., Sánchez Capa M., Constante Mejía D., et al. (2020). In-situ and ex-situ biodiversity conservation in ecuador: A review of policies, actions and challenges. Diversity 12 (8), 315. doi: 10.3390/d12080315
Mills J. N., Gage K. L., Khan A. S. (2010). Potential influence of climate change on vector-borne and zoonotic diseases: A review and proposed research plan. Environ. Health Perspect. 118, 1507–1514. doi: 10.1289/ehp.0901389
MINEPDED (2012). Cameroon Fourth National Report to the CBD. Republic of Cameroon 2012, NBSAP II – MINEPDED.
Montgomery G. A., Dunn R. R., Fox R., Jongejans E., Leather S. R., Saunders M. E., et al. (2020). Is the insect apocalypse upon us? How to find out. Biol. Conserv. 241, 108327. doi: 10.1016/j.biocon.2019.108327
Morton O., Scheffers B. R., Haugaasen T., Edwards D. P. (2021). Impacts of wildlife trade on terrestrial biodiversity. Nat. Ecol. Evol. 5 (4), 540–548. doi: 10.1038/s41559-021-01399-y
Mosoh D. A., Khandel A. K., Verma S. K., Vendrame W. A. (2023). Effects of sterilization methods and plant growth regulators on in vitro regeneration and tuberization in Gloriosa superba (L.). In Vitro Cell. Dev. Biol. - Plant 59, 792–807. doi: 10.1007/s11627-023-10387-9
Mosoh D. A., Khandel A. K., Verma S. K., Vendrame W. A. (2024a). Phytochemical analysis and enhanced production of alkaloids in non-dormant corm-derived callus of Gloriosa superba (L.) using plant growth regulators and abiotic elicitors. Plant Cell Tissue Organ Culture (PCTOC) 156, 89. doi: 10.1007/s11240-023-02674-5
Mosoh D. A., Khandel A. K., Verma S. K., Vendrame W. A. (2024b). Optimizing callus induction and indirect organogenesis in nondormant corm explants of Gloriosa superba (L.) via media priming. Front. Horticulture 3. doi: 10.3389/fhort.2024.1378098
Mourão E., Pimentel J. F., Murta L., Kalinowski M., Mendes E., Wohlin C. (2020). On the performance of hybrid search strategies for systematic literature reviews in software engineering. Inf. Software Technol. 123, 106294. doi: 10.1016/j.infsof.2020.106294
Muh E., Amara S., Tabet F. (2018). Sustainable energy policies in Cameroon: A holistic overview. Renewable Sustain. Energy Rev. 82, 3420–3429. doi: 10.1016/j.rser.2017.10.049
Mulugo L., Kyazze F. B., Kibwika P., Kikulwe E., Omondi A. B., Ajambo S. (2020). Unravelling technology-acceptance factors influencing farmer use of banana tissue culture planting materials in Central Uganda. Afr. J. Science Technol. Innovation Dev. 12, 453–465. doi: 10.1080/20421338.2019.1634900
Nadeau C. P., Urban M. C. (2019). Eco-evolution on the edge during climate change. Ecography 42 (7), 1280–1297. doi: 10.1111/ecog.04404
Nath V., Pande N., Asthana A. K., Gupta R. (2012). Epiphytic moss flora of pachmarhi biosphere reserve (M.P.): an important aspect of bryophyte diversity. Natl. Acad. Sci. Lett. 35, 195–200. doi: 10.1007/s40009-012-0015-0
Nogués-Bravo D., Rodríguez-Sánchez F., Orsini L., de Boer E., Jansson R., Morlon H., et al. (2018). Cracking the code of biodiversity responses to past climate change. Trends Ecol. Evol. 33, 765–776. doi: 10.1016/j.tree.2018.07.005
Nordhaus W. D. (2010). The economics of hurricanes and implications of global warming. Climate Change Economics 1, 1–20. doi: 10.1142/S2010007810000054
Norton S. (2018). “The convention on international trade in endangered species of wild fauna and flora (CITES): an appraisal,” in Around the world in 80 species(Routledge, UK), 151–169.
Oldfield T., Compton J., Broad S. (2019). CITES CoP18: Facing the challenges of the post-2020 global biodiversity framework. TRAFFIC Bulletin 31 (2), 48–58.
Oseni O. M., Pande V., Nailwal T. K. (2018). A review on plant tissue culture, a technique for propagation and conservation of endangered plant species. Int. J. Curr. Microbiol. Appl. Sci. 7 (07), 3778–3786. doi: 10.20546/ijcmas.2018.707.438
Pande S. K., Bhardwaj D. (1952). The present position of Indian Hepaticology with a note on the Hepatic Vegetation of the country. J. Palaeosciences 1, 368–381. doi: 10.54991/jop.1952.396
Pant B. (2013). Medicinal orchids and their uses: Tissue culture a potential alternative for conservation. Afr. J. Plant Sci. 7, 448–467. doi: 10.5897/AJPS2013.1031
Parkinson A. J., Evengard B., Semenza J. C., Ogden N., Børresen M. L., Berner J., et al. (2014). Climate change and infectious diseases in the Arctic: Establishment of a circumpolar working group. Int. J. Circumpolar Health 73, 25163. doi: 10.3402/ijch.v73.25163
Patil P. (2018). Study of rare and endangered plants of Pachmarhi Biosphere Reserve, India. The Maldives National Journal of Research 6 (1), 73–79.
Pereira H. M., Leadley P. W., Proença V., Alkemade R., Scharlemann J. P. W., Fernandez-Manjarrés J. F., et al. (2010). Scenarios for global biodiversity in the 21st century. Science 330, 1496–1501. doi: 10.1126/science.1196624
Pereira M. R., Sierra A. M., Maia J. M. F., de Holanda A. S. S. (2021). A comparative study of temporal variation of two epiphytic bryophytes in a central Amazonian white-sand forest, Brazil. Hoehnea 48, e1352020. doi: 10.1590/2236-8906-135/2020
Pimm S. L., Jenkins C. N., Abell R., Brooks T. M., Gittleman J. L., Joppa L. N., et al. (2014). The biodiversity of species and their rates of extinction, distribution, and protection. Science 344, 1246752. doi: 10.1126/science.1246752
Pimm S., Raven P., Peterson A., Şekercioğlu Ç.H., Ehrlich P. R. (2006). Human impacts on the rates of recent, present, and future bird extinctions. Proc. Natl. Acad. Sci. 103, 10941–10946. doi: 10.1073/pnas.0604181103
Post-2020 global biodiversity framework. (2022). (IUCN). Available online at: https://www.iucn.org/resources/issues-brief/post-2020-global-biodiversity-framework.
Powers R. P., Jetz W. (2019). Global habitat loss and extinction risk of terrestrial vertebrates under future land-use-change scenarios. Nat. Climate Change 9, 323–329. doi: 10.1038/s41558-019-0406-z
Prance G. T. (2010). A brief history of conservation at the royal botanic gardens, kew. Kew Bull. 65 (4), 501–508. doi: 10.1007/s12225-010-9231-2
Proença V., Pereira H. M. (2017). “Comparing Extinction Rates: Past, Present, and Future,” in Reference Module in Life Sciences (Elsevier), B9780128096338020000. doi: 10.1016/B978-0-12-809633-8.02128-2
Rands M. R. W., Adams W. M., Bennun L., Butchart S. H. M., Clements A., Coomes D., et al. (2010). Biodiversity conservation: Challenges beyond 2010. Science 329 (5997), 1298–1303. doi: 10.1126/science.1189138
Rawat R. S., Arora G., Gautam S., Shaktan T. (2020). Opportunities and challenges for the implementation of REDD+ Activities in India. Curr. Sci. 119, 749. doi: 10.18520/cs/v119/i5/749-756
Razanamalala K., Razafimbelo T., Maron P.-A., Ranjard L., Chemidlin N., Lelièvre M., et al. (2018). Soil microbial diversity drives the priming effect along climate gradients: A case study in Madagascar. ISME J. 12, 451–462. doi: 10.1038/ismej.2017.178
Ren J., Liu F., Luo Y., Zhu J., Luo X., Liu R. (2021). The pioneering role of bryophytes in ecological restoration of manganese waste residue areas, Southwestern China. J. Chem. 2021, 1–19. doi: 10.1155/2021/9969253
Ribeiro J., Reino L., Schindler S., Strubbe D., Vall-llosera M., Araújo M. B., et al. (2019). Trends in legal and illegal trade of wild birds: A global assessment based on expert knowledge. Biodiversity Conserv. 28 (12), 3343–3369. doi: 10.1007/s10531-019-01825-5
Rillig M. C., Ryo M., Lehmann A., Aguilar-Trigueros C. A., Buchert S., Wulf A., et al. (2019). The role of multiple global change factors in driving soil functions and microbial biodiversity. Science 366 (6467), 886–890. doi: 10.1126/science.aay2832
Ritchie H., Roser M. (2013). Land use. Our world in data. Available online at: https://ourworldindata.org/land-use.
Ritchie H., Roser M. (2021). Biodiversity. Our world in data. Available online at: https://ourworldindata.org/extinctions.
Rivera S. N., Knight A., McCulloch S. P. (2021). Surviving the wildlife trade in southeast asia: reforming the ‘disposal ‘of confiscated live animals under CITES. Animals 11 (2), 439. doi: 10.3390/ani11020439
Robinson J. E., Sinovas P. (2018). Challenges of analyzing the global trade in CITES-listed wildlife: CITES data. Conserv. Biol. 32 (5), 1203–1206. doi: 10.1111/cobi.13095
Román-Palacios C., Wiens J. J. (2020). Recent responses to climate change reveal the drivers of species extinction and survival. Proc. Natl. Acad. Sci. 117, 4211–4217. doi: 10.1073/pnas.1913007117
Romshoo S. A., Bashir J., Rashid I. (2020). Twenty-first century-end climate scenario of Jammu and Kashmir Himalaya, India, using ensemble climate models. Climatic Change 162, 1473–1491. doi: 10.1007/s10584-020-02787-2
Ruklani S., Rubasinghe S. C. K., Jayasuriya G. (2021). A review of frameworks for using bryophytes as indicators of climate change with special emphasis on Sri Lankan bryoflora. Environ. Sci. pollut. Res. 28, 60425–60437. doi: 10.1007/s11356-021-16588-2
Sahay M., Gupta S. D. (2015). Continuity and change: A case study in two gond villages of pachmarhi biosphere reserve. J. Anthropological Survey India 64, 95–115. doi: 10.1177/2277436X20150109
Sallie Chia-Wei Y., Lin-Heng L. (2018). “Wildlife trade in china and vietnam: A comparative study of the implementation of the convention on international trade in endangered species of wild fauna and flora (Cites),” in Sustainability matters: Environmental management in the anthropocene, 25–57. doi: 10.1142/9789813230620_0002
Samantray A., Pin P. (2019). Credibility of climate change denial in social media. Palgrave Commun. 5, 127. doi: 10.1057/s41599-019-0344-4
Sánchez-Bayo F., Wyckhuys K. A. G. (2019). Worldwide decline of the entomofauna: A review of its drivers. Biol. Conserv. 232, 8–27. doi: 10.1016/j.biocon.2019.01.020
Sarkar S., Pressey R. L., Faith D. P., Margules C. R., Fuller T., Stoms D. M., et al. (2006). Biodiversity conservation planning tools: Present status and challenges for the future. Annu. Rev. Environ. Resour. 31 (1), 123–159. doi: 10.1146/annurev.energy.31.042606.085844
Sarkki S., Niemelä J., Tinch R., Jäppinen J.-P., Nummelin M., Toivonen H., et al. (2016). Are national biodiversity strategies and action plans appropriate for building responsibilities for mainstreaming biodiversity across policy sectors? The case of Finland. J. Environ. Plann. Manage. 59, 1377–1396. doi: 10.1080/09640568.2015.1076384
Scheffers B. R., Oliveira B. F., Lamb I., Edwards D. P. (2019). Global wildlife trade across the tree of life. Science 366 (6461), 71–76. doi: 10.1126/science.aav5327
Scheffran J., Brzoska M., Kominek J., Link P. M., Schilling J. (2012). Climate Change Violent Conflict. Science 336 (6083), 869–871. doi: 10.1126/science.1221339
Schleuning M., Neuschulz E. L., Albrecht J., Bender I. M. A., Bowler D. E., Dehling D. M., et al. (2020). Trait-based assessments of climate-change impacts on interacting species. Trends Ecol. Evol. 35, 319–328. doi: 10.1016/j.tree.2019.12.010
SDG Report. (2021). The Sustainable Development Goals Report. Available online at: https://unstats.un.org/sdgs/report/2021/The-Sustainable-Development-Goals-Report-2021.pdf.
Selig E. R., Turner W. R., Troëng S., Wallace B. P., Halpern B. S., Kaschner K., et al. (2014). Global priorities for marine biodiversity conservation. PloS One 9 (1), e82898. doi: 10.1371/journal.pone.0082898
Seo S. N. (2017). Beyond the Paris Agreement: Climate change policy negotiations and future directions: Beyond the Paris Agreement. Regional Sci. Policy Pract. 9, 121–140. doi: 10.1111/rsp3.12090
Sesana E., Gagnon A. S., Ciantelli C., Cassar J., Hughes J. J. (2021). Climate change impacts on cultural heritage: A literature review. WIREs Climate Change 12, e710. doi: 10.1002/wcc.710
Silva J. M. C., Rapini A., Barbosa L. C. F., Torres R. R. (2019). Extinction risk of narrowly distributed species of seed plants in Brazil due to habitat loss and climate change. PeerJ 7, e7333. doi: 10.7717/peerj.7333
Singh J. S., Gupta V. K. (2018). Soil microbial biomass: A key soil driver in management of ecosystem functioning. Sci. Total Environ. 634, 497–500. doi: 10.1016/j.scitotenv.2018.03.373
Singh B. B., Sharma R. N., Gill J. P. S., Aulakh R. S., Bangah S. (2011). Climate change, zoonoses and India: -EN- -FR- Le changement climatique et les zoonoses en Inde -ES- Cambio climático y zoonosis en la India. Rev. Scientifique Technique l’OIE 30, 779–788. doi: 10.20506/rst.issue.30.3.48
Singh J., Singh R. P., Khare R. (2018). Influence of climate change on Antarctic flora. Polar Sci. 18, 94–101. doi: 10.1016/j.polar.2018.05.006
Slettebak R. T. (2012). Don’t blame the weather! Climate-related natural disasters and civil conflict. J. Peace Res. 49, 163–176. doi: 10.1177/0022343311425693
Strayer D. L., Dudgeon D. (2010). Freshwater biodiversity conservation: Recent progress and future challenges. J. North Am. Benthological Soc. 29 (1), 344–358. doi: 10.1899/08-171.1
Stuart Chapin F., Díaz S. (2020). Interactions between changing climate and biodiversity: Shaping humanity’s future. Proc. Natl. Acad. Sci. United States America 117, 6295–6296. doi: 10.1073/pnas.2001686117
Sudhersan C., AboEl-Nil M., Hussain J. (2003). Tissue culture technology for the conservation and propagation of certain native plants. J. Arid Environments 54, 133–147. doi: 10.1006/jare.2001.0884
Suggitt A. J., Wilson R. J., Isaac N. J. B., Beale C. M., Auffret A. G., August T., et al. (2018). Extinction risk from climate change is reduced by microclimatic buffering. Nat. Climate Change 8, 713–717. doi: 10.1038/s41558-018-0231-9
Sultan F. A., Routroy S., Thakur M. (2020). Introducing traceability in the indian surimi supply chain. Materials Today: Proc. 28, 964–969. doi: 10.1016/j.matpr.2019.12.333
‘t Sas-Rolfes M., Challender D. W. S., Hinsley A., Veríssimo D., Milner-Gulland E. J. (2019). Illegal wildlife trade: Scale, processes, and governance. Annu. Rev. Environ. Resour. 44 (1), 201–228. doi: 10.1146/annurev-environ-101718-033253
Tabarelli M., Pinto L. P., Silva J. M. C., Hirota M., Bede L. (2005). Challenges and opportunities for biodiversity conservation in the brazilian atlantic forest. Conserv. Biol. 19 (3), 695–700. doi: 10.1111/j.1523-1739.2005.00694.x
Taebi B., Safari A. (2017). On effectiveness and legitimacy of ‘Shaming’ as a strategy for combatting climate change. Sci. Eng. Ethics 23, 1289–1306. doi: 10.1007/s11948-017-9909-z
Tardy V., Mathieu O., Lévêque J., Terrat S., Chabbi A., Lemanceau P., et al. (2014). Stability of soil microbial structure and activity depends on microbial diversity: Linking microbial diversity and stability. Environ. Microbiol. Rep. 6, 173–183. doi: 10.1111/1758-2229.12126
Tebaldi C., Ranasinghe R., Vousdoukas M., Rasmussen D. J., Vega-Westhoff B., Kirezci E., et al. (2021). Extreme sea levels at different global warming levels. Nat. Climate Change 11, 746–751. doi: 10.1038/s41558-021-01127-1
Tegen H., Mohammed W. (2016). The role of plant tissue culture to supply disease free planting materials of major horticultural crops in Ethiopia. Journal of Biology, Agriculture and Healthcare 6, 122–129.
Thomas C. D., Cameron A., Green R. E., Bakkenes M., Beaumont L. J., Collingham Y. C., et al. (2004). Extinction risk from climate change. Nature 427, 145–148. doi: 10.1038/nature02121
Thuiller W., Guéguen M., Renaud J., Karger D. N., Zimmermann N. E. (2019). Uncertainty in ensembles of global biodiversity scenarios. Nat. Commun. 10, 1446. doi: 10.1038/s41467-019-09519-w
Tong P. S. (2020). More policies and laws, is it better for biodiversity conservation in Malaysia? Conserv. Sci. Pract. 2, e235. doi: 10.1111/csp2.235
Topa G., Karsenty A., Megevand C., Debroux L. (2009). The Rain Forests of CAMEROON: Experience and Evidence from a Decade of Reform (World Bank Publications). doi: 10.1596/978-0-8213-7878-6
Torcal M. (2014). The decline of political trust in Spain and Portugal: economic performance or political responsiveness? Am. Behav. Scientist 58, 1542–1567. doi: 10.1177/0002764214534662
Turvey S. T., Crees J. J. (2019). Extinction in the anthropocene. Curr. Biol. 29, R982–R986. doi: 10.1016/j.cub.2019.07.040
UNEP. (2019). Insect declines are a stark warning to humanity (UNEP). Available online at: http://www.unep.org/news-and-stories/story/insect-declines-are-stark-warning-humanity.
UNEP-WCMC. (2022). Protected planet | Cameroon. Protected area profile for Cameroon from the world database on protected areas. Available online at: https://www.protectedplanet.net/country/CMR.
UNFCCC. (1992). United nations framework convention on climate change (UNFCCC), 1992. United Nations (UN). 1992 (New York: UN: United Nations Framework Convention on Climate Change). Available online at: https://unfccc.int/resource/docs/convkp/conveng.pdf.
Vanneste T., Michelsen O., Graae B. J., Kyrkjeeide M. O., Holien H., Hassel K., et al. (2017). Impact of climate change on alpine vegetation of mountain summits in Norway. Ecol. Res. 32, 579–593. doi: 10.1007/s11284-017-1472-1
van Vuuren D. P., Sala O. E., Pereira H. M. (2006). The future of vascular plant diversity under four global scenarios. Ecol. Soc. 11, art25. doi: 10.5751/ES-01818-110225
Velasco D., García-Llorente M., Alonso B., Dolera A., Palomo I., Iniesta-Arandia I., et al. (2015). Biodiversity conservation research challenges in the 21st century: A review of publishing trends in 2000 and 2011. Environ. Sci. Policy 54, 90–96. doi: 10.1016/j.envsci.2015.06.008
Velásquez A. C., Castroverde C. D. M., He S. Y. (2018). Plant–pathogen warfare under changing climate conditions. Curr. Biol. 28, R619–R634. doi: 10.1016/j.cub.2018.03.054
Verma N., Mohammad A. (2020). Challenges and strategy for achieving nationally determined contribution commitment of india. Int. J. Ecol. Environ. Sci. 2 (4), 380–385.
Vermeulen S. J., Campbell B. M., Ingram J. S. I. (2012). Climate change and food systems. Annu. Rev. Environ. Resour. 37, 195–222. doi: 10.1146/annurev-environ-020411-130608
Viles H. A., Cutler N. A. (2012). Global environmental change and the biology of heritage structures. Global Change Biol. 18, 2406–2418. doi: 10.1111/j.1365-2486.2012.02713.x
Wagner D. L. (2020). Insect declines in the anthropocene. Annu. Rev. Entomol. 65, 457–480. doi: 10.1146/annurev-ento-011019-025151
Wang C., Liu D., Bai E. (2018). Decreasing soil microbial diversity is associated with decreasing microbial biomass under nitrogen addition. Soil Biol. Biochem. 120, 126–133. doi: 10.1016/j.soilbio.2018.02.003
Wei F. (2021). Toward post-2020 global biodiversity conservation: Footprint and direction in china. Innovation 2 (4). doi: 10.1016/j.xinn.2021.100175
Weiskopf S. R., Rubenstein M. A., Crozier L. G., Gaichas S., Griffis R., Halofsky J. E., et al. (2020). Climate change effects on biodiversity, ecosystems, ecosystem services, and natural resource management in the United States. Sci. Total Environ. 733, 137782. doi: 10.1016/j.scitotenv.2020.137782
White A., Chapple B. (2021). ABOUT EIA. Available online at: https://eia-international.org/wp-content/uploads/2021-Pangolin-CITES-Briefing-SINGLE-PAGES.pdf (Accessed July 31, 2024).
Wohlin C. (2016). Second-generation systematic literature studies using snowballing. In Proceedings of the 20th International Conference on Evaluation and Assessment in Software Engineering (EASE '16). Association for Computing Machinery, New York, NY, USA, Article 15, 1–6. doi: 10.1145/2915970.2916006
Wohlin C., Kalinowski M., Romero Felizardo K., Mendes E. (2022). Successful combination of database search and snowballing for identification of primary studies in systematic literature studies. Inf. Software Technol. 147, 106908. doi: 10.1016/j.infsof.2022.106908
Wojtania A., Mieszczakowska-Frąc M. (2021). In vitro propagation method for production of phenolic-rich planting material of culinary rhubarb ‘Malinowy.’. Plants 10, 1768. doi: 10.3390/plants10091768
Wong-Parodi G., Feygina I. (2020). Understanding and countering the motivated roots of climate change denial. Curr. Opin. Environ. Sustainability 42, 60–64. doi: 10.1016/j.cosust.2019.11.008
WorldBank Group. (2021). Terrestrial protected areas (% of total land area). Available online at: https://data.worldbank.org/indicator/ER.LND.PTLD.ZS?view=map.
Wu X., Lu Y., Zhou S., Chen L., Xu B. (2016). Impact of climate change on human infectious diseases: Empirical evidence and human adaptation. Environ. Int. 86, 14–23. doi: 10.1016/j.envint.2015.09.007
Wu R., Possingham H. P., Yu G., Jin T., Wang J., Yang F., et al. (2019). Strengthening China’s national biodiversity strategy to attain an ecological civilization. Conserv. Lett. 12, e12660. doi: 10.1111/conl.12660
Xu H., Cao Y., Yu D., Cao M., He Y., Gill M., et al. (2021). Ensuring effective implementation of the post-2020 global biodiversity targets. Nat. Ecol. Evol. 5 (4), 411–418. doi: 10.1038/s41559-020-01375-y
Yadav K., Singh N., Verma S. (2012). Plant tissue culture: A biotechnological tool for solving the problem of propagation of multipurpose endangered medicinal plants in India. Journal of Agricultural Technology 8, 305–318.
Yang Y., Li T., Wang Y., Cheng H., Chang S. X., Liang C., et al. (2021). Negative effects of multiple global change factors on soil microbial diversity. Soil Biol. Biochem. 156, 108229. doi: 10.1016/j.soilbio.2021.108229
Young J. C., Waylen K. A., Sarkki S., Albon S., Bainbridge I., Balian E., et al. (2014). Improving the science-policy dialogue to meet the challenges of biodiversity conservation: Having conversations rather than talking at one-another. Biodiversity Conserv. 23 (2), 387–404. doi: 10.1007/s10531-013-0607-0
Zain S. (2020). Corrupting trade: An overview of corruption issues in illicit wildlife trade. Available online at: https://files.worldwildlife.org/wwfcmsprod/files/Publication/file/3m48phx0ap_Introductory_Overview_Corrupting_trade_An_overview_of_corruption_issues_in_illicit_wildlife_trade.pdf (Accessed July 31, 2024).
Zanatta F., Engler R., Collart F., Broennimann O., Mateo R. G., Papp B., et al. (2020). Bryophytes are predicted to lag behind future climate change despite their high dispersal capacities. Nat. Commun. 11, 5601. doi: 10.1038/s41467-020-19410-8
Zandalinas S. I., Fritschi F. B., Mittler R. (2021). Global warming, climate change, and environmental pollution: recipe for a multifactorial stress combination disaster. Trends Plant Sci. 26, 588–599. doi: 10.1016/j.tplants.2021.02.011
Zedan H. (2005). The role of the convention on biological diversity and its protocol on biosafety in fostering the conservation and sustainable use of the world’s biological wealth for socio-economic and sustainable development. J. Ind. Microbiol. Biotechnol. 32 (11–12), 496–501. doi: 10.1007/s10295-005-0237-y
Zekeng J. C., Fobane J. L., Nganyo Heya M., Sebego R., Mphinyane W. N., Onana J. M., et al. (2021). Plant diversity and conservation concerns in a semi-deciduous rainforest in Cameroon: Implications for sustainable forest management. Folia Geobotanica 56, 81–95. doi: 10.1007/s12224-021-09393-9
Zhou Z., Wang C., Luo Y. (2020). Meta-analysis of the impacts of global change factors on soil microbial diversity and functionality. Nat. Commun. 11, 3072. doi: 10.1038/s41467-020-16881-7
Keywords: 30-by-30 targets – terrestrial biomes, climate change, Kew-Wide Mechanism (KWM), global climate and plant conservation policies, biodiversity loss & plant diversity decline, plant tissue culture technology, post-2020 global biodiversity conservation framework, sixth extinction
Citation: Mosoh DA, Prakash O, Khandel AK and Vendrame WA (2024) Preserving earth’s flora in the 21st century: climate, biodiversity, and global change factors since the mid-1940s. Front. Conserv. Sci. 5:1383370. doi: 10.3389/fcosc.2024.1383370
Received: 08 February 2024; Accepted: 15 July 2024;
Published: 27 September 2024.
Edited by:
Chong Jiang, Guangdong Academy of Science (CAS), ChinaReviewed by:
Matthew E. Aiello-Lammens, Pace University, United StatesCopyright © 2024 Mosoh, Prakash, Khandel and Vendrame. This is an open-access article distributed under the terms of the Creative Commons Attribution License (CC BY). The use, distribution or reproduction in other forums is permitted, provided the original author(s) and the copyright owner(s) are credited and that the original publication in this journal is cited, in accordance with accepted academic practice. No use, distribution or reproduction is permitted which does not comply with these terms.
*Correspondence: Dexter Achu Mosoh, bW9zb2hkZXh0ZXJAaG90bWFpbC5jb20=
†ORCID: Dexter Achu Mosoh, orcid.org/0000-0001-9721-0263
Om Prakash, orcid.org/0000-0002-6568-0567
Wagner A. Vendrame, orcid.org/0000-0001-6391-7623
Ashok Kumar Khandel, orcid.org/0000-0002-0725-8165
Disclaimer: All claims expressed in this article are solely those of the authors and do not necessarily represent those of their affiliated organizations, or those of the publisher, the editors and the reviewers. Any product that may be evaluated in this article or claim that may be made by its manufacturer is not guaranteed or endorsed by the publisher.
Research integrity at Frontiers
Learn more about the work of our research integrity team to safeguard the quality of each article we publish.