- 1Plant Conservation Unit, Department of Biological Sciences, University of Cape Town, Cape Town, South Africa
- 2Conservation and Research Unit, Grootbos Foundation, Grootbos Private Nature Reserve, Gansbaai, South Africa
Grootbos Nature Reserve falls within the Cape Floristic Region of South Africa and comprises fynbos and forest vegetation elements, which exist as alternate stable states and are naturally maintained by feedbacks between vegetation, fire, topography and climate. The objective of this study was to investigate changes in forest extent in the Baviaansfontein valley in Grootbos Nature Reserve in order to understand whether current forest coverage was greater or lesser prior to colonial settlement. Incorporating paleoecology has the potential to define historical ranges of landscape variability that can guide forest restoration programs that are aligned to historical forest occurrence. Not all landscapes are suited for tree planting initiatives as they harbor indigenous open vegetation, which is of conservation value. Historical and palaeoecological information is especially valuable as tree planting initiatives and reforestation programs are becoming more popular. Here we present a combined palynological (pollen, spores, charcoal) and geochemical approach (X-ray fluorescence elemental ratios, Loss-on-ignition), to evaluate the historic extent and distribution of forest and fynbos vegetation for the past ~250 years at Baviaansfontein in the Grootbos Nature Reserve. The start of the record was dominated by pollen typical of asteraceous fynbos, with evidence of forest elements also present, though in low abundance. After 200 years (c.1750 CE–1950 CE) of increased input of micro- and macrocharcoal, the subsequent decline in charcoal indicates a decrease in fire occurrence. This decline coincides with increased input of forest pollen taxa, suggesting that fire suppression most likely triggered forest expansion and perhaps initiated the beginning of a biome boundary shift. The continued decline in fire promoted the development of the present-day proteoid fynbos type around ~1980 CE and was responsible for the replacement of the previously more asteraceous-dominated fynbos type. The results show that changes in fire occurrence have driven compositional changes over time within fynbos but also contributed to the expansion of forest at the expense of fynbos. Considering the palaeoecological record and the expected warmer climate with more fires, forest expansion at Baviaansfontein is not recommended. The proteoid-dominated fynbos biome might be similarly negatively affected by warmer climate and a shift to an asteraceous fynbos community could occur in the future.
1 Introduction
The protection of the world’s remaining forests and the promotion of tree planting projects (e.g., African Forest Landscape Restoration Initiative, Bonn Challenge, New York Declaration on Forests, World Economic Forum’s One Trillion Trees Initiative) are advocated internationally as being part of a nature-based adaptation strategy to combat global warming. Forest ecosystems are among the most effective natural systems for sequestering carbon (Canadell and Raupach, 2008; Pan et al., 2011). Around the world, areas have been identified that have the potential for tree-based landscape reforestation and afforestation (World Resource Institute, 2023). However, some of these harbour ancient open landscapes are unsuitable for tree-planting due to their unique biodiversity and the ecosystem services they provide (Bond et al., 2019).
In Africa, the proposed targeted landscapes are primarily centered around the semi-arid drylands of the Sahel (The Great Green Wall: Turner et al., 2023) and the grasslands and savanna grasslands of sub-Saharan Africa (The African Forest Landscape Restoration Initiative, 2023) . These landscapes are often perceived to be degraded or to have become deforested over time (Zaloumis and Bond, 2016; Bond et al., 2019; Turner et al., 2023). However, many landscapes with lower tree cover are not always linked to landscape degradation. In some cases, such mixed tree-grass landscapes have evolved over millennia, driven by feedbacks from interacting bio-geosphere processes notably fire-vegetation feedbacks (Zaloumis and Bond, 2016; Bond et al., 2019; Turner et al., 2023). Because of this, it is important to understand past forest extent and composition to effectively guide tree-planting efforts while also conserving ancient open and mosaic ecosystems (Bond et al., 2019; Zaloumis and Bond, 2016). It further demonstrates that the long-term efficiency and appropriateness of tree planting initiatives varies depending on the history of the targeted region (compare e.g., Holl and Brancalion, 2020; Ghantous, 2021; Ricciardi et al., 2022; Song et al., 2023).
Incorporating paleoecology into forest rehabilitation questions has the potential to provide insights to assess forest distribution patterns over timescales longer than those captured by satellite imagery, human memory, or repeat photography (Gillson et al., 2023). Palaeoecological data can deliver information for restoration and conservation management strategies beyond well-adapted restoration methodologies (Barak et al., 2016; Wingard et al., 2017; Gillson et al., 2021).
Although changes in past environmental conditions are not a direct analogue to contemporaneous and future environmental changes, nevertheless they can provide valuable contextual information, and insight into thresholds of vegetation-climate feedback loops and future trajectories of range shifts (Bernhardt and Willard, 2009; Holbourn et al., 2015). Such information is needed to set achievable restoration targets and helps to minimize the risks of biodiversity loss that occurs when afforestation takes place in unsuitable landscapes.
In the Cape Floristic Region (CFR), forest patches are embedded within fynbos, a mega-diverse shrubland dominated by Proteaceae, Asteraceae, Restionaceae and Ericaceae. Vegetation from these two biomes exists as alternate stable states (Mucina and Geldenhuys, 2006). The boundaries between forest and fynbos are maintained by climate-fire-vegetation and soil feedback processes, as well as topography and local hydrology (Coetsee et al., 2011; Cramer et al., 2019; Manders and Richardson, 1992; Lu et al., 2022; McKenzie et al., 1976; Mucina and Geldenhuys, 2006) in which fynbos is fire adapted while forest is not. There is often a steep gradient from forest to fynbos vegetation with the boundary between the two being maintained by fire (Mucina and Geldenhuys, 2006; Cramer et al., 2019; Slingsby et al., 2020; Lu et al., 2022). However, under longer fire-free periods this sharp boundary becomes less well-defined and disturbance-tolerant forest precursor species can spread into the fynbos (Mucina and Geldenhuys, 2006; Poulsen and Hoffman, 2015), paving the way for forest expansion through exclusion of fire.
More information is needed to better understand the drivers that define and stabilize the fynbos-forest biome boundary. Palaeoecological research revealed that the interplay of different drivers was responsible for internal changes in fynbos composition and setting the boundaries between forest and fynbos alternate stable states over time (Gillson et al., 2020; Quick et al., 2016; MacPherson et al., 2019; Prader et al., 2023). The waning of afrotemperate forest in various areas of the CFR accelerated at times under increased aridity and fire activity (e.g., Medieval Warm Period, MWP: 900–1250 CE), as well as during increased rainfall seasonality, despite a relative higher rainfall amount (Quick et al., 2016; Prader et al., 2023). In recent decades, there are concerns that forest expansion could accelerate a loss of biodiversity within fynbos vegetation and negatively impact hydrological processes within the CFR (Poulsen and Hoffman, 2015; Slingsby et al., 2020; Moncrieff et al., 2021). Nevertheless, ongoing tree-planting initiatives aim to restore indigenous forests in the CFR with the prospect of mitigating land degradation and climate warming (Greenpop, 2023; Platbos Africa’s Southernmost Forest, 2023; South African Reforestation Trust, 2023).
While climatic-driven rainfall seasonality and aridity are non-manageable ecological drivers, fires can be managed and therefore used to manipulate the relative abundance of fynbos and forest elements. Over decades, fires have been suppressed throughout the CFR which is thought to have contributed to forest expansion on parts of the Cape Peninsula (Poulsen and Hoffman, 2015; Slingsby et al., 2020). Palaeoecological research has also revealed that since fire has been excluded, forest cover in Table Mountain National Park (Orange Kloof) (Prader et al., 2023). expanded, as visible through an increased input of pollen derived from Searsia, Olea but also through Podocarpus (Prader et al., 2023).
Nevertheless, the potential forest cover during one of the wettest periods during the Holocene (Little Ice Age; LIA: 1250–1850 CE) in the winter rainfall zone is unknown, as it coincides with intensive tree logging during European settlement (Prader et al., 2023).
Grootbos Nature Reserve is situated in the Walker Bay area on the Western edge of the Agulhas Plain and comprises fynbos and forest vegetation elements (Figure 1; Mergili and Privett, 2008). The Reserve has been actively managed for conservation since 1995 (Mergili and Privett, 2008) with a focus on (1) the restoration and maintenance of fynbos and forest vegetation and (2) the removal of alien species (Privett and Lutzeyer, 2010). Grootbos has its own reforestation program and falls within the broader Walker Bay Fynbos Conservancy (WBFC). The WBFC focuses on landscape level fynbos and forest conservation management and includes a collective reforestation strategy.
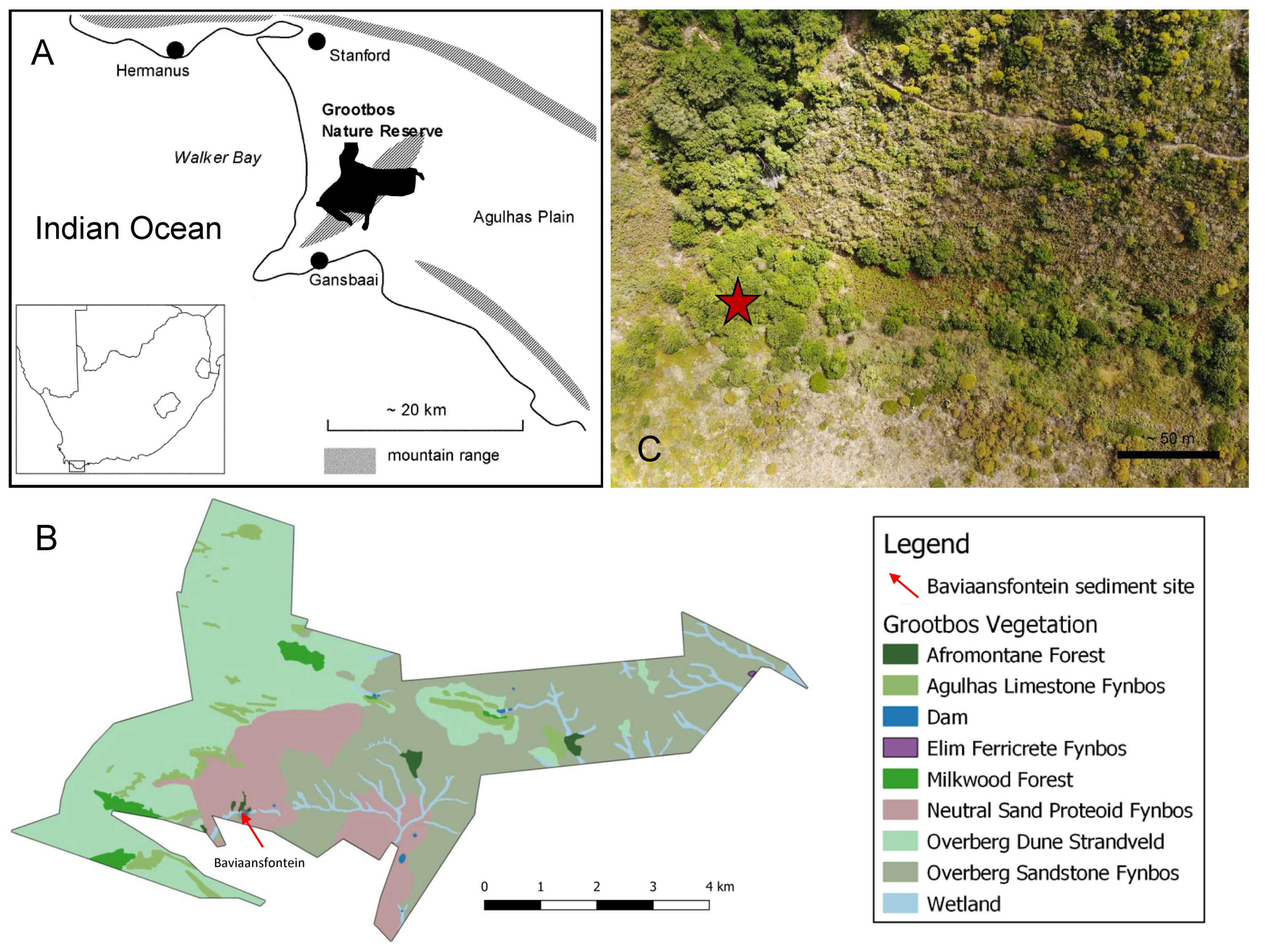
Figure 1 (A) Map showing location of Grootbos Nature Reserve along the south coast of South Africa (modified after Mergili and Privett, 2008). (B) The main vegetation communities of Grootbos Nature Reserve (Legend provides information about vegetation communities). (C) Drone image from Baviaansfontein showing Afrotemperate Forest on the left side of the image. The red star indicates the location from where the sediment core at Baviaansfontein was recovered.
Despite the current tree-planting initiatives at Grootbos, the forest restoration plan is under review to determine whether management initiatives should further focus on forest rehabilitation in certain areas or not (Grootbos Foundation, 2023a).
In light of this, along with the ongoing climate change, and the expected drier hydroclimatic conditions, as well as increasing fire activity in the winter rainfall region (Mbokodo et al., 2020; Jain et al., 2022), this study aims to elucidate the site-specific historical extent of forest and the dynamics of fynbos and forest in Grootbos Nature Reserve over the last ~250 yr.
Here we present a combined palynological and geochemical approach, which examines the historical distribution of forest and fynbos vegetation at Baviaansfontein in the Grootbos Nature Reserve. The results are placed in context with the ongoing reforestation efforts at Grootbos Nature Reserve and the broader region.
1.1 Study region: environmental and ecological setting of Grootbos Nature Reserve with special emphasis on the site at Baviaansfontein
Grootbos Nature Reserve (Grootbos = Big Forest in Afrikaans) stretches over 3,500 hectares along the Western edge of the Agulhas Plain (Figures 1A–C; Mergili and Privett, 2008). Moving eastwards a along the Agulhas Plain, the rainfall regime shifts from a pronounced winter rainfall zone (WRZ) into a year-round rainfall zone (YRZ; Carr et al., 2006). Areas in the WRZ receive > 66% of rain during the austral winter months between May and August, while the rainfall pattern in the YRZ is evenly distributed (Tyson and Preston-Whyte, 2000). Modern climatological records (Diechmann and Eklundh, 1991) indicate that the WRZ on the Agulhas Plain extends west and north of De Kelders (Carr et al., 2006). According to this climatological axis, Grootbos Nature Reserves can be described as being located at the boundary of the YRZ and the pronounced WRZ (Carr et al., 2006), and obtains a mean annual precipitation of ~ 730 mm (Mergili and Privett, 2008). The climate of Grootbos is thus often described as an attenuated Mediterranean climate (Mergili and Privett, 2008).
Various vegetation types of fynbos, forests, and wetland vegetation are found within the reserve which are subdivided into (1) Overberg Dune Strandveld, (2) Agulhas Limestone Fynbos, (3) Overberg Sandstone Fynbos, (4) Elim Ferricrete Fynbos, (5) Afrotemperate Forest, (6) Milkwood Forest, and (7) wetland (Figure 1B; Privett and Lutzeyer, 2010; Grootbos Foundation, 2023b). The complexity and uniqueness of vegetation types is based primarily on geology and resultant soil formations (Mergili and Privett, 2008; Privett and Lutzeyer, 2010).
The Baviaansfontein site where the sediment core was collected is situated at an intersection of several vegetation types. The north-facing warmer, drier slopes of the valley are characterised by Overberg Sandstone Proteoid Fynbos. This is characterized by a proteoid layer comprising several Proteaceae genera (Privett and Lutzeyer, 2010). At Baviaansfontein Leucadendron, Protea, Aulax and Mimetes can be found (Privett and Lutzeyer, 2010). The more mesic, south-facing slopes are characterized by Neutral Sand Proteoid Fynbos. This vegetation type, together with Dune Asteraceous Fynbos and Protea repens Proteoid Fynbos vegetation types form the Alkaline Sand Fynbos Complex in Grootbos. This complex is found on soils of various nutrient statuses, pH, and depths (Mergili and Privett, 2008). In wetter areas, the Neutral Sand Proteoid Fynbos becomes intermixed with species within the Restionaceae (Privett and Lutzeyer, 2010).
There are five small Afrotemperate Forest patches extending to the south of Baviaansfontein. The south-facing slopes form steep ravines in which the Afrotemperate Forest is protected from fire (Privett and Lutzeyer, 2010). In Grootbos these forests are characterized by species such as Rapanea melanophloeos (Primulaceae), Kiggelaria africana (Achariaceae), Celtis africana (Cannabaceae), and Sideroxylon inerme (Sapotaceae), while no Podocarpus trees grow in the Reserve (Mergili and Privett, 2008). The east-facing slopes are covered by Neutral Sand Proteoid Fynbos with Leucadendron coniferum a dominant species. These slopes also include Erica magnisylvae, which is a very localised member of the Ericaceae and a Grootbos endemic.
In the valley bottom, there is a small ephemeral wetland fed by ephemeral streams and dominated by Cyperaceae, Restionaceae, and Psoralea. The Wetlands in Grootbos consists often of three vegetation strata of which the tallest consists of species like Psoralea arborea (Fabaceae), Kiggelaria africana (Achariaceae), Searsia laevigata (Anancardiaceae) and Salvia africana-lutea (Lamiaceae). The vegetation stratum in the middle can be composed of Pteris dentata (Pteridaceae), or Artemisia afra (Asteraceae), and Leonotis leonurus (Lamiaceae). Species characteristic of the ground vegetation stratum include Cliffortia ferruginea, Helicrysum cymosum, and Hippia frutescens (Asteraceae) as well as Zantedeschia aethiopica (Araceae; Privett and Lutzeyer, 2010).
1.2 Precolonial and early colonial land history of the Agulhas Plain
The Agulhas Plain has been used by hominins for millennia (Compton, 2016). Hunter-gatherer communities hunted animals and used fire to promote the growth of fire-adapted food plants such as Watsonia angusta (Iridaceae; Smith, 1986; Deacon, 1992; Sadr, 2003, 2008; Pooley, 2014). Khoekhoen pastoralists arrived at the Cape around 2000 yr ago (50 CE; Deacon, 1992; Sadr, 2003, 2008) and depended on the changing spatiotemporal distribution of plants and animals in the CFR for their livelihoods. They actively modified and managed the natural habitats of the Cape by using fire stick farming to enhance the grazing grounds for their livestock (Smith, 1986). However, because of the relatively low number of people in the landscape, their impact on the natural habitats of the Agulhas Plain is thought to have been relatively limited (Deacon, 1992). However, the pattern of human pressure on the landscape changed significantly after the arrival of European settlers in 1652 CE (Pooley, 2018). Parts of the CFR landscape were transformed through intensified tree logging and the introduction of pines and other alien species such as oaks and Australian acacias, as well as increased burning and agricultural activities (Pooley, 2018). Also, between 1920 CE and 1968 CE, fire was rigorously suppressed as it was considered to be harmful to fynbos, but after the 1970s, the use of fire slowly became an integral part of the CFR’s conservation policy (Pooley, 2018).
2 Materials and methods
2.1 Fieldwork, sediment core inspection, and sampling
In July 2021 one sediment core at the Baviaansfontein site (S −34.54353 E 19.4379; Figure 1) was retrieved using a vibracorer and stored in the core repository of the Plant Conservation Unit at the University of Cape Town. The core had a total length of 96 cm and was subsampled at intervals of 2 cm.
2.2 Lithology of the Baviaansfontein site core
A lithological description of the Baviaansfontein site core involved a visual assessment of the sediment color determined by the Munsell Color Company (1954). Average grain size and sediment structure were determined with the Troels-Smith Classification System (Troels-Smith, 1955).
2.3 Age–depth model
For the establishment of the age–depth relationship, three organic samples were sent to Beta Analytic Inc. (Miami, FL, USA) for radiocarbon dating by AMS (accelerator mass spectrometry; Table 1). Radiocarbon dates were converted to calendar years based on the SHCal20 calibration curve (Hogg et al., 2020) using the rbacon package v. 2.5.3 (Blaauw and Christen, 2011) in R (R Core Team, 2021). The geology of the area consists of quarzitic Table Mountain Sandstone and Bredasdorp Limestone (Mergili and Privett, 2008). The samples were furthermore treated with a weak acidic solution to remove carbonates.
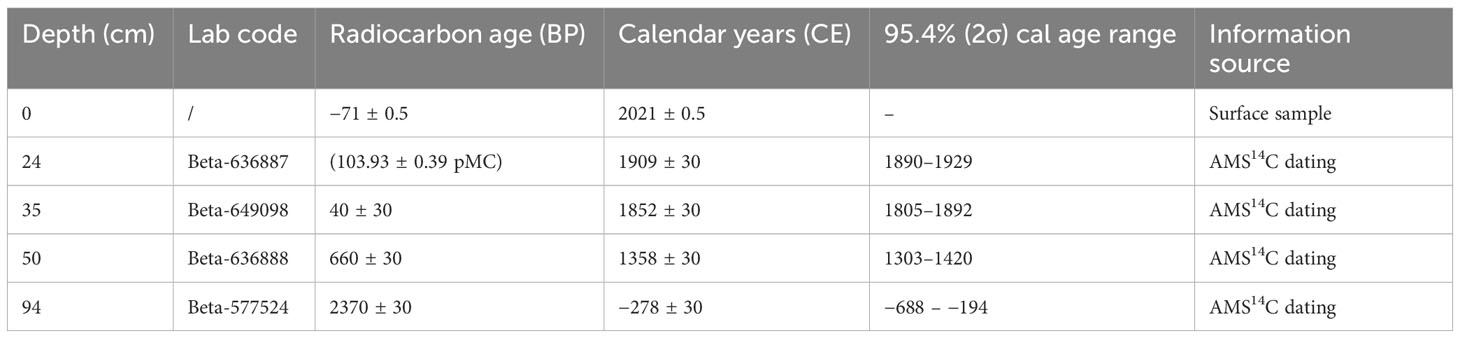
Table 1 The results of accelerator mass spectrometry (AMS) 14C dating of organic sediments at Baviaansfontein.
2.4 Paleoenvironmental proxies investigated at Baviaansfontein
Terrestrial palynomorphs: Standard palynological preparation techniques (Bennett and Willis, 2001) were applied at the Laboratories of the Environmental and Geographical Sciences, at the University of Cape Town (UCT). The recovered sediment core was subsampled over the entire length of 96 cm. From 92 cm to 44 cm processed levels contained no palynomorphs, and this was also the case for levels taken at 28 cm and 24 cm. The absence of palynomorphs is relatively common in sediment cores recovered from semi-arid terrestrial environments such as from Grootbos Nature Reserve. Sediment cores from these areas often only preserve pollen to a limited extent, which can be attributed to higher oxidation processes due to the temporary drying out of the wetland and to changes in the sediment composition (Traverse, 2008). Both processes led to a differential preservation of palynomorphs in the analyzed sediment core. Processed subsamples from the upper 40 cm of the sediment core contained moderately preserved palynomorphs which enabled counting and palynomorph identification. The upper 40 cm was subsampled at a 2 cm resolution. A total of 23 levels were sub-sampled. For each level, 2 cm³ of sediment was treated with warm HCl and NaOH to remove carbonates and humic acids, respectively. Hydrofluoric acid (HF; 60%) was used to remove silicates.
Because of the fragility of preserved palynomorphs, acetolysis treatment was not undertaken. Samples were sieved through a mesh size of 150 μm to separate macrofossils (e.g., macrocharcoal) from microfossils (e.g., pollen, spores, and microcharcoal) and sub-samples were spiked with exotic Lycopodium spores (L. clavatum; Batch no. 100320201) to estimate absolute pollen concentration (Stockmarr, 1971).
To establish a robust palaeoecological reconstruction, between 260 and 310 pollen grains were counted per level (e.g., Scott and Woodborne, 2007). Aquatic taxa and Cyperaceae were excluded from the pollen sum as they would reflect the composition of the wetland rather than the surrounding terrestrial vegetation. They were treated as non-pollen-palynomorphs (NPP: e.g., fungal and fern spores, algae). NPPs were counted until the minimum count of pollen grains was reached per level. The applicability of using NPP as an independent proxy for possible past disturbance regimes, such as from those created by herbivores, (obligate coprophilous fungi like Sordaria, Arnium) is well established (e.g., van Geel, 2001; van Geel and Aptroot, 2006; Shumilovskikh and van Geel, 2020).
Charcoal: To determine the fire signal at Baviaansfontein, micro- (<150µm) and macrocharcoal (>150µm) particles were counted (Whitlock and Larsen, 2001; Conedera et al., 2009; Power et al., 2010). Microcharcoal particles were counted using the same palynological slides as used to generate palynomorph counts. Only particles with sharp uneven edges, which appeared opaque and completely black were identified as microcharcoal particles. At least 300 such objects were counted per level together with Lycopodium spores (Tinner and Hu, 2003; Finsinger and Tinner, 2005). Macrocharcoal particles were identified and counted using the residues collected during sample processing by sieving at 150 μm. Residues were bleached using a weak 6% hydrogen peroxide solution to facilitate the counting of macrocharcoal particles (Stevenson and Haberle, 2005).
Major sediment elements (X-ray fluorescence elemental ratios, XRF): To assess changes in abiotic environmental conditions and changes in sediment deposition, geochemical proxies such as major basic compounds (e.g., SiO2, TiO2) in the sediments can be used. For this purpose, of each level 1 cm3 were finely ground and sent to the Central Analytical Facilities at Stellenbosch University for measurement. Major elements were measured using an XRF spectrometry on a Panalytical Axios Wavelength Dispersive spectrometer which is fitted with a Rh tube at 3kWatt.
Loss-on-ignition (LOI): The organic and inorganic carbon content through the sediment facies was determined using loss of mass on ignition at different temperatures. Samples were dried at 110°C to eliminate water and hazardous volatiles and then combusted at 950°C (Heiri et al., 2001; Santisteban et al., 2004). The procedure was conducted using the same samples as for the XRF analysis and was performed at the Central Analytical Facilities at Stellenbosch University.
2.5 Statistical analysis and visualization of data
Coniss Cluster Analysis based on the Bray-Curtis Dissimilarity Index and a broken-stick model, to identify the number of pollen zones was calculated using Rioja package v.1.0-6 (Juggins, 2022). Two diversity indices were applied to the palynological assemblage. (1) The Shannon Wiener Index (H(s)) was applied to quantify the diversity of the analyzed levels (Spellerberg and Fedor, 2003), while the (2) Rarefaction Analysis was applied to assess the species richness of the analyzed levels with variable count size (Birks et al., 2016). A Detrended Correspondence Analysis (DCA) was chosen to address the relationship between environmental variables and palynological taxa under the assumption that the relationship is nonlinear. The dataset was Hellinger transformed. The diversity indices and the DCA were performed using Vegan package v.2.5.6 (Oksanen et al., 2022). Correlation analyses were performed using Performance Analytics package 2.0.4 (Peterson and Carl, 2020). Graphs were plotted with ggplot2 package v3.3.2 (Wickham, 2016) and C2 (Juggins, 2007).
3 Results
3.1 Chronology
Results of AMS 14C dating for Site Baviaansfontein are listed in Table 1. The 14C-based age-depth model is shown in Figure 2 and gives a basal age of 278 BCE at 94 cm.
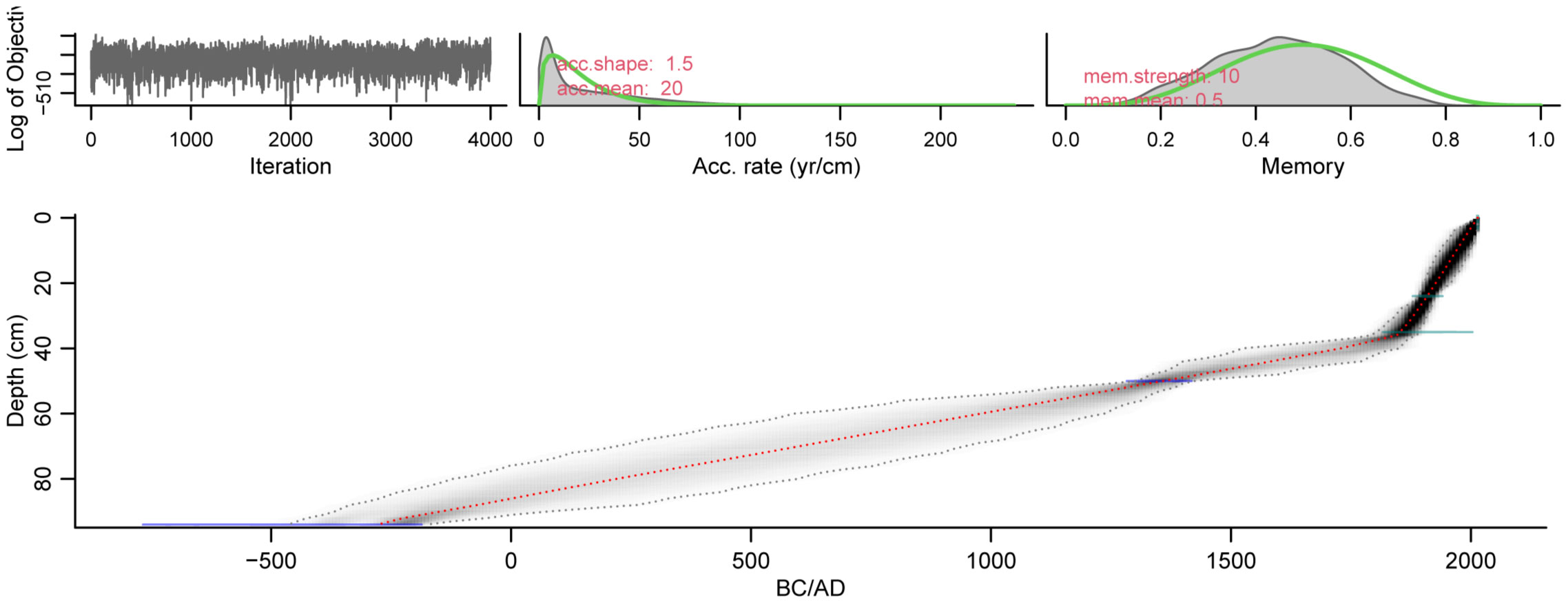
Figure 2 Age depth model of Baviaansfontein sediment core using Bacon package V. 2.5.3 (Blaauw and Christen, 2011) based on the SHCal20 calibration curve (Hogg et al., 2020). The weighted mean age is represented by the red line. Dotted grey lines and greyscale depict the 95% confidence interval.
3.2 Lithology of Baviaansfontein sediment core
The sediment core of Baviaansfontein was 96 cm long and consisted of six visually distinct lithological units. While the coarser-grained sands were mainly part of the lowermost core section, the very fine organic components occurred through the entire sediment core and increased in the uppermost core section (Supplementary Figure 1). Clay minerals also increased towards the topmost section of the core.
The bottom-most lithological Unit I (96–80 cm; Supplementary Figure 1), was composed of coarser-grained sands with mineral particles up to 2 mm and very low content of clayey minerals intermixed with fine-grained organic material. Lithological Unit II (80–70 cm; Supplementary Figure 1A) had a similar composition to that of lithological Unit I, although it differed in the color of the sediment. The color of the sediment in lithological Unit I was dark reddish grey (3/1) while the sediment layers of lithological Unit II and all overlaying lithological Units were reddish black (2.5/1). The amount of gravel increased in lithological Unit III (70–67 cm). The organic component in these facies was fine-grained and was intermixed with a low amount of clayey minerals. The amount of clayey minerals increased in the overlying lithological Unit IV (67–24 cm; Supplementary Figure 1B) and was accompanied by a reduction of the sand fraction. Lithological Unit V (24–6 cm; Supplementary Figure 1C) consisted of clayey layers intermixed with a low amount of organic material. The uppermost facies of lithological Unit VI (6–0 cm) consisted of an organic-rich herbaceous layer with a high amount of clay and a decrease of coarser-grained sand.
3.3 Palynomorph assemblage of Baviaansfontein
Pollen taxa identified in the Baviaansfontein core were derived mainly from fynbos vegetation (Supplementary Table 1). Their relative abundances fluctuated through the investigated time frame (Figures 3, 4) but showed a decline toward younger levels (~1970 CE, 11 cm). The relative abundance of forest taxa reflected an opposing trend (Figure 4). Introduced plants were more common in younger intervals with Pinus dominating the recent assemblage (Figure 3). Taxa belonging to coprophilous fungi clearly increased through the analysed time interval (Figures 3, 4). Detrended Correspondence Analysis divided the palynological assemblage and the corresponding environmental variables into two distinctive phases (Figure 5). Shannon Wiener Index (H(s)) values varied between 1.7 and 2.3 for fynbos and 0.7 and 2.1 for forest (between levels: Supplementary Figure 4). Rarefaction analysis revealed fluctuations in the fynbos assemblage from 19 to 28 and for the forest from 3 and 11 (between levels: Supplementary Figure 4).

Figure 3 Relative Abundances (%) of most important fynbos- and forest pollen taxa, coprophilous fungal spores and alien plant pollen versus age (CE) and depth (cm) of Baviaansfontein. Zones I–IV according to a Coniss Cluster Analysis with Bray-Curtis Dissimilarity Index using R package rioja v.1.0-6 (Juggins, 2022). The border of Zones is indicated by black horizontal lines and determined by the broken-stick model (Juggins, 2022). The light blue color reflects the climatic event of the Little Ice Age (LIA: which is a climatic event lasting from 1250–1850 CE; sensu PAGES 2k Consortium, 2013).
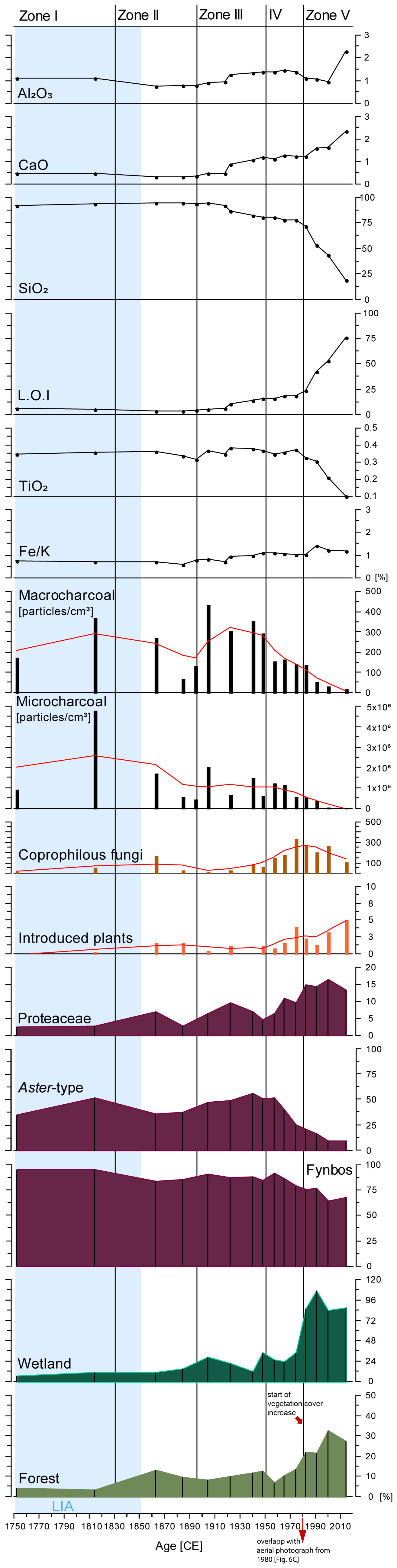
Figure 4 Sum of the relative abundances (%) of fynbos, forest, and introduced plants versus age (CE) and depth (cm) at Baviaansfontein. Micro- and macrocharcoal (particles per 1 cm3), Fe/K ratios, and changes of minerogenic sediment composition (TiO2, LOI, SIO2, CaO, Al2O3) are also shown. Zones I–IV are based on Coniss Cluster Analysis with a Bray-Curtis Dissimilarity Index using R package rioja v.1.0-6 (Juggins, 2022). The border of Zones is indicated with black horizontal lines. The light blue shading reflects the Little Ice Age (LIA: 1250–1850 CE; sensu PAGES 2k Consortium, 2013).
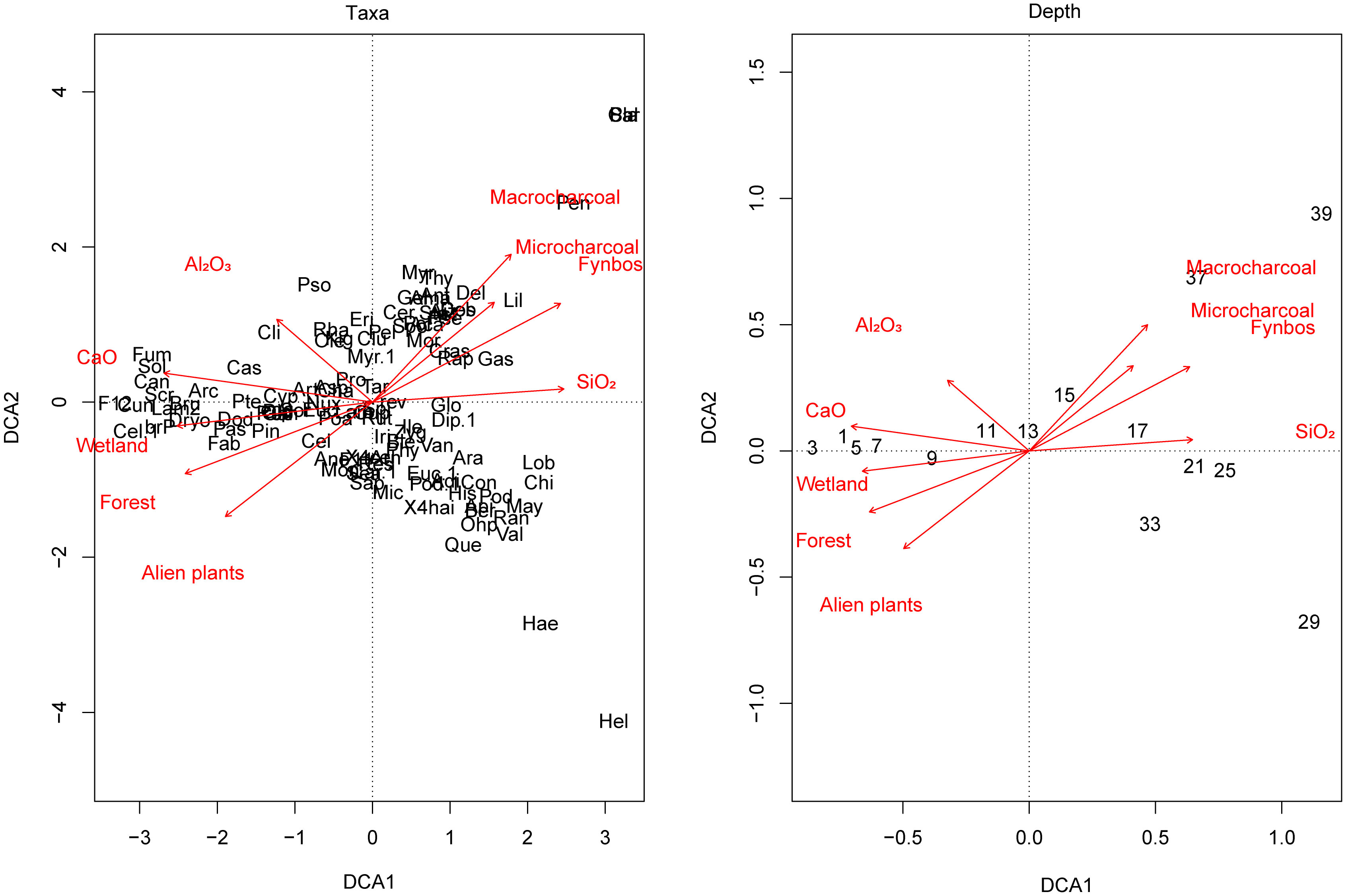
Figure 5 Detrended Correspondence Analysis (DCA) plot, showing the influence of the main environmental factors on vegetation composition of Baviaansfontein. DCA plot on the left shows the relationship between taxa and environmental variables, while the relationship between depths (sample depth) and environmental variables are given on the right. The analysis was carried out on all identified palynological taxa (compare Supplementary Table 1) and was performed using vegan package v.2.5.6 (Oksanen et al., 2019).
Coniss Cluster analysis using the broken-stick model (Supplementary Figure 3) divided the palynological assemblage into four zones (Figure 4; Supplementary Figure 2). The main component in Zone I (~1760–1820 CE, 39–37 cm) of counted pollen grains was derived from fynbos vegetation, of which the main abundances are from Aster pollen type (max: 52.4% at ~1810 CE; Figure 3) followed by Pentzia pollen type (max: 32.0% at ~1760 CE) Stoebe/Elytropappus pollen type, and Crassulaceae (both 5.3% at ~1820 CE). Relative abundances of e.g., Morella or Passerina remained minor but stable components in Zone I (Figure 3). Minor fynbos elements in this interval were Cyperaceae, Ericaceae and Cliffortia pollen as well as forest pollen taxa (Figures 3, 4). The coprophilous fungal spore Sporormiella (13.8% at ~1820 CE) is found (Figure 3).
Zone II (~1860–1950 CE/33–15 cm). The main changes were reflected within fynbos vegetation. Relative percentages of Proteaceae increased >9.6% at ~1920 CE (Figure 3). Cyperaceae pollen also increase continuously (24.1% at ~1950 CE), while Pentzia pollen showed a drastic reduction (Figure 3). Forest taxa such as Euclea, Searsia or Rapanea pollen increased slightly toward the end of this Zone with maxima values of 5.7% for Euclea and 1.3% for Searsia and Rapanea at ~1940 CE (Figure 3). Celastraceae were also represented. Sordaria spores also had considerable increased values (Figure 3). At the end of Zone II Pinus became abundant and was continuously recorded in the following Zones (Figure 3).
In Zone III (~1950–1980 CE, 13–9 cm) forest pollen taxa were remarkably abundant. This change was accompanied by a decline in the overall relative abundances of fynbos pollen (Figure 4). Despite this overall decline, some important fynbos pollen taxa increased during this interval, (Figure 3) including Proteaceae (min 6.6% at ~1960 CE; max: 11.1% at ~1970 CE), Cyperaceae (min: 11.9% at ~1960 CE; max: 17.9% at ~1980 CE), and Ericaceae (min: 12% at ~1960 CE; max: 4.8% at ~1970 CE). There was a notable decrease in the relative abundances of Aster- type pollen (min: 25.8 at ~1980 CE; Figure 3). Forest taxa such as Euclea (min: 3.5% at ~1960 CE; max: 6.9% at ~1980 CE) or Olea (min: 0.0% at ~1980 CE; max: 1.3% at ~1960 CE) are important in this Zone (Figure 3). A sharp shift in the palynomorph composition is reflected in the relative percentages of fungal spores in which Sordaria spores reached maximum values of 320.3% at ~1980 CE Pinus pollen increased toward the end of this Zone (Figure 3).
Zone IV (~1990–2020 CE, 7–0 cm) is characterized by the lowest pollen count derived from the fynbos vegetation. The relative abundances reached values of 80.9% at ~2010 CE (Figure 4). Within this vegetation type dominant taxa were Cyperaceae (max: 67.6% at ~1990 CE), Proteaceae (max: 16.6% at ~2010 CE), or Cliffortia (max: 8.1% at ~2020 CE), whereas Aster-type pollen were at their lowest (min: 10.0% at ~2010 CE; Figure 3).
Pollen derived from afrotemperate forest taxa reached abundances of 32.8% and 27.3% at ~2010 CE and ~2020 CE respectively (Figure 4). Dominant forest taxa were Euclea (max: 19.5% at 3 cm/~2010 CE), Olea (max: 6.2% at ~2010 CE), Searsia (max: 2.3% at 7 cm/~1990 CE), or Kiggelaria (max: 4.3% at ~2020 CE; Figure 3). Pinus reached its highest relative abundances within this Zone (max: 4.3% at ~2020 CE). Relative counts of coprophilous fungi such as Delitschia, Sporormiella, Sordaria and Arnium were high but showed an overall decline relative to the youngest analysed core sediment level (Figure 3).
3.4 Micro- and macrocharcoal
Micro- and macrocharcoal counts reflected a general declining trend toward the youngest interval. Statistical analysis reflects a strong correlation of micro- and macrocharcoal occurrence: R2 0.466; P<0.05 (Supplementary Figure 5). The highest charcoal concentration was recorded in Zone I (~1820 CE/37 cm), which remained relatively high throughout Zone II.
Starting with Zone III (~1960–1980 CE/13–9 cm) micro- and macrocharcoal reflected a downward trend which continued into Zone IV (Figure 4). In Zone IV (~1990–2020 CE/7–0 cm) micro- and macrocharcoal concentrations were the lowest throughout the analysed time interval reaching minima concentrations of 55542.92 particles/cm3 at ~2020 CE for microcharcoal and 22 particles/cm3 ~2020 CE for macrocharcoal particles respectively.
3.5 Minerogenic sediment composition
The mineral SiO2 (Quartz) dominated the sediment core reaching the highest value of 94.6% around ~1870 CE and remained constantly high through Zone I to II (Figure 4). Its value steadily decreased towards the youngest analysed interval (Zone IV Figure 4). Similar fluctuating patterns in the minerogenic record is reflected for TiO2 and K2O, and Fe2O3 values which reached their lowest percentages values at ~2020 CE. The percentages of CaO showed an opposing temporal variation to that of SiO2 (Figure 4).
Fe/K ratios varied between 0.6% and 1.2% and only showed minor and weak fluctuations throughout the investigated time. This trend of increasing values toward the youngest analysed level is further reflected in CaO values (Figure 4).
Inorganic carbon content exhibited through Loss-on-Ignition (LOI) ranged between 3.9% (~1880 CE) and 76.2% (~2020 CE). Values remained relatively stable between ~1610 CE and ~1920 CE varying from 5.5% and 5.3% respectively (Figure 4). After ~1920 CE values continuously increased, exhibiting the highest values of 76.2% in the uppermost level (Figure 4).
4 Discussion: paleoecology of Baviaansfontein
4.1 The asteraceous dominated fynbos biome (~1760–1830 CE; Zone I)
Despite the very low resolution of this interval, relative pollen abundances of Baviaansfontein reflect a landscape dominated by fynbos into which forest islands were probably only present in areas that were protected from fire and with higher moisture availability (Figure 4). The high input of pollen derived from Aster- and Pentzia- pollen-type is perhaps indicative of an open, asteraceous fynbos biome that prevailed during the last extension of the Little Ice Age (LIA; 1250–1850 CE; sensu PAGES 2k Consortium, 2013; Figures 3, 4). This type of fynbos community is predominantly composed of non-ericaceous ericoids, succulents and fleshy-leaved shrubs forming a low fynbos vegetation cover (Campbell, 1986). Pollen derived from such structural representatives (e.g., Stoebe/Elytropappus-, Aster-type, Aizoaceae, Crassulaceae) are found in relatively higher percentages in this interval, which argues for an asteraceous-dominated fynbos biome type at Baviaansfontein (Figure 3).
When comparing Baviaansfontein to other palaeoecological records spanning the same period (~1750 CE to ~1850 CE) a heterogenous picture is reflected along the southern Cape Coast. Palaeoecological data from Bo Langvlei (du Plessis et al., 2020) and Platbos (along Garden Route; MacPherson et al., 2019) depict a similar palynological signal pointing towards a more open landscape with reduced forest cover. However, an opposing picture emerges from e.g., Eilandvlei (Quick et al., 2018). At this site, extensive forests have been reconstructed.
This heterogeneity is further documented in the palaeoclimatological signals. At Baviaansfontein, local low Fe/K values suggest reduced chemical weathering during increased aridity (Govin et al., 2012; Simon et al., 2015) and accord well with the results from Bo Langvlei (du Plessis et al., 2020) and Swartvlei (Kirsten, 2014), depicting locally drier conditions around ~1750 CE. Opposing this trend, wetter local conditions are reflected in palaeoclimatological studies from Eilandsvlei (Quick et al., 2018) and Seweweekspoort (Chase et al., 2013; 2017), which further overlap with signals of wetter and cooler conditions depicted from the WRZ (e.g., Stager et al., 2012; Weldeab et al., 2013; Granger et al., 2018; Strobel et al., 2019; Perren et al., 2020). The local climate signal from Groenvlei transitions from drier to more humid/modern conditions between ~1700 and ~1900 CE (Wündsch et al., 2016). These differences in climate perhaps could have been responsible for the development of forested areas, although feedback loops between vegetation-rainfall seasonality and vegetation-fire feedbacks are important.
While at Bo Langvlei (du Plessis et al., 2020) and Baviaansfontein, fynbos was more asteraceaeous-dominated, the fynbos at Platbos is interpreted to have been herbaceous-grassy (MacPherson et al., 2019). Both fynbos types can develop during times of reduced water availability and/or increased seasonality accompanied by increased fire (MacPherson et al., 2019; Prader et al., 2023). While at Platbos increased rainfall seasonality (strong summer drought) and fire were the main drivers (MacPherson et al., 2019), at Baviaansfontein, drier conditions are coupled with increased fire (Figure 4). Both feedback mechanisms (dry climatic conditions with fire versus wet climatic conditions with high precipitation seasonality and fire) reinforce the development of open landscapes and amplify the reduction of forested areas (Figure 4; MacPherson et al., 2019; du Plessis et al., 2020).
The presence of a low amount of coprophilous fungi (Sporomiella, Delitschia) suggests that herbivores have been present in the area for a considerable time (Figures 3, 4). The fire signal and input of coprophilous fungi might have been modified to some degree by anthropogenic activity, as this period overlaps with the times of Khoekhoen pastoralists (Pooley, 2014). Khoekhoen pastoralists used fire to improve their pastures for livestock (Pooley, 2009, 2014). Nevertheless, the input of coprophilous fungi in this interval remains lower compared to later intervals indicating fewer herbivores during the precolonial period (Figures 3, 4).
It appears that the fynbos-forest biome boundary at Baviaansfontein was stable for nearly 100 years at the time of first colonial settlement (~1760 CE – ~1830 CE). As the palynological data reflects only minor anthropogenic activity, the forest-fynbos ecotone was possibly stabilized through feedbacks between fire and vegetation. But because of the low temporal resolution of this interval, it remains unclear as to how stable this interval really was, as some biome shifts might have gone undetected.
4.2 The transition phase (~1830 CE–~1950 CE; Zone II)
The asteraceous fynbos taxa continue to dominate in this phase until ~1980 CE (Zone III). However, the slight decline in Asteraceae perhaps suggests a still persistent but weakening feedback loop between asteraceous fynbos and fire (Figures 3, 4). This perhaps allowed various fynbos taxa such as Proteaceae, Lamiaceae or Cyperaceae to become more dominant in the landscape as the relative abundance of pollen of these taxa increased around ~1900 CE (Figure 3).
Based on Fe/K ratios the local water availability continues to be relatively stable (Figure 4; Govin et al., 2012). Starting from the 1900s this stability is supported by precipitation records from Stanford, about 10 km from Grootbos, which underlines the depicted rainfall pattern of the Fe/K ratios (compare Figure 4 and Supplementary Figure 6). While the micro- and macrocharcoal input between ~1830 CE and ~1950 CE tends to be relatively more variable, complex interactions occur between changes in fire and the vegetation at Baviaansfontein (Figure 4). Numerous studies have demonstrated that variable fire cycles and fire intensities create environments that enhance the co-existence of different fynbos taxa (Esler and Cowling, 1990; Thuiller et al., 2007). This signal of increasing diversity stimulated through fire alternations is weakly represented through the Rarefaction and the Shannon Wiener Index (H(s)) (Supplementary Figure 4).
Changes in the fire record appear to have also affected the abundance and composition of afrotemperate forest taxa. The forest’s composition indicates a close relation between perturbation and expansion (Figure 3). The Baviaansfontein forest is primarily composed of taxa, such as Rapanea, Euclea and Searsia which are able to withstand elevated disturbance (Figure 3; Campbell and Moll, 1977; Masson and Moll, 1987; Giddey et al., 2022). These taxa represent forest precursor species (Campbell and Moll, 1977; Masson and Moll, 1987; Giddey et al., 2022) and an increase in their abundance in the palynological record might illustrate a slight increase in forested areas (Figure 4). However, the relatively constant input of both forest and fynbos pollen over this interval points to a generally stable fynbos-forest biome boundary (Figure 4).
Reasons for the change in fire patterns, and the degree to which humans were involved in these changes, remain unclear. The temporal resolution of the 19th century is again quite sparse but overlaps with the first permanent settlers at Grootbos (1840 CE; Mouton, 2008). European settlers adopted the use of fire from the Koekhoen pastoralists but used fire more extensively to increase pasture yields (Wicht, 1945; Pooley, 2014).
The reduced input of micro- and macrocharcoal into the sediment core during the 19th century seems thus to be contrary to this view (Figure 4; Wicht, 1945; Pooley, 2014). The micro- and macrocharcoal signal during this time period suggests that fires were perhaps not that extensive in the area in the 19th century. Later, however, around ~1900 CE, the increasing input of micro- and macrocharcoal, would align with the documented intensive fire history of that time (Figure 4; Pooley, 2009; van Wilgen, 2009; van Wilgen et al., 2016). As with the fire signal, relative abundances of coprophilous fungal spores started to increase around ~ 1920 CE (Figures 3, 4). Although Grootbos was used for cattle farming and hunting (Mouton, 2008), the input of coprophilous fungal spores through the 19th century remains low (Figures 3, 4). This might be related to the rugged topography and relatively remote setting of Baviaansfontein within Grootbos. Baviaansfontein might only have been used for livestock grazing as anthropogenic pressure at Grootbos increased over time. An increase in human disturbance is further reflected by the occurrence of pollen from non-native plants such as Pinus and Quercus and aligns well with other investigations from the wider fynbos region (Figure 3; Neumann et al., 2011; Forbes et al., 2018; MacPherson et al., 2019; du Plessis et al., 2020; Prader et al., 2023);. Although the 1850s marked the beginning of intensive, human-related landscape transformation on the Agulhas Plain (Heydenrych, 1999), at Baviaansfontein, the human influence remained relatively moderate perhaps because of its remote setting.
4.3 The decline of the asteraceous component of fynbos vegetation (Zone III: ~1950–1980 CE)
Between the period 1950–1980 CE, Proteaceae became more prevalent in the Baviaansfontein landscape, as shown by the increase in the relative abundance of Proteaceae pollen (Figure 3). This increase and the simultaneous decline of Aster-type pollen resulted in a shift from a more asteraceous-dominated fynbos to a more proteoid-dominated fynbos around ~1980 CE, which characterizes today’s landscape at Baviaansfontein (Figure 3).
The continuing and constant decline of fire was perhaps the trigger that resulted in the compositional shift within this neutral-sand fynbos community (Figure 4). A similar negative association between elevated Proteaceae pollen abundances and declining charcoal particles is reported from the Cedarberg Mountains (~15.000 cal. yr BP; Valsecchi et al., 2013) and at Platbos (~1550 cal. yr BP; MacPherson et al., 2019). Both records explain this trend to have been stimulated by declining fire frequency and fire intensity at times of increased aridity or humidity during cooler climates (Valsecchi et al., 2013; MacPherson et al., 2019). At Baviaansfontein, the internal reorganization of fynbos from asteraceous to proteoid dominance can be considered as a by-product of land use changes and reduced human-induced, burning activities of that time.
The conservation efforts of the ~1950s on the Agulhas Plain focused on reducing forest losses, as a result of too frequent burning, similar to other fynbos areas such as the Cape Peninsula (Pooley, 2009; van Wilgen, 2009; van Wilgen et al., 2016). Subsequently, fire was suppressed on the Agulhas Plain and Walker Bay area (Heydenrych, 1999). The changed burning practice allowed Proteaceae to be dominant which is consistent with observations that too frequent burning (i.e., less than 5-year intervals) is detrimental to Proteaceace and favors other fynbos elements (Kraaij et al., 2013; van Wilgen, 1981).
The declining micro- and macrocharcoal signal evident in the sediment core of Baviaansfontein reflects a steady decline in burning, which accords well with the fire exclusion policy, with witness reports and with observations from aerial photographs from 1961–1998 (Figures 4, 6). These all suggest that Baviaansfontein and the area immediately around it remained relatively fire-free for almost 30 yr (1970–2006 CE). However, in the wider Grootbos area over this time eye-witness reports and evidence from aerial photographs suggest that burning remained a feature of the landscape (Figure 6). The increasing input of coprophilous fungi over this interval coincides with sheep farming at Baviaansfontein, which continued until the early mid-1990s CE (Figures 3, 4; S.D.J. Privett, Grootbos Foundation, pers. com.). The decline in fire from 1950 led to an overall expansion of afrotemperate forest cover at Baviaansfontein, marking the onset of the shift in the forest-fynbos biome boundary (Figure 4). Forest expansion is evident through increasing input of forest pollen (Figure 3; Campbell and Moll, 1977; Masson and Moll, 1987; Giddey et al., 2022). Although forests at Grootbos are often dominated by taxa such as Rapanea and Kiggelaria (Mergili and Privett, 2008; Privett and Lutzeyer, 2010) a similar pollen composition signal during the early stages of forest expansion is reported from e.g., Orange Kloof (Cape Peninsula; Prader et al., 2023). It seems plausible that the feedback between fynbos and fire became increasingly disrupted by the suppression of fire which resulted in an increase of forest cover. Elsewhere, the suppression of fire as part of a forest conservation plan accounts for the steady increase in forest cover (Marloth, 1924; Campbell and Moll, 1977; Luger and Moll, 1993; Pooley, 2009; van Wilgen, 2009; van Wilgen et al., 2016).
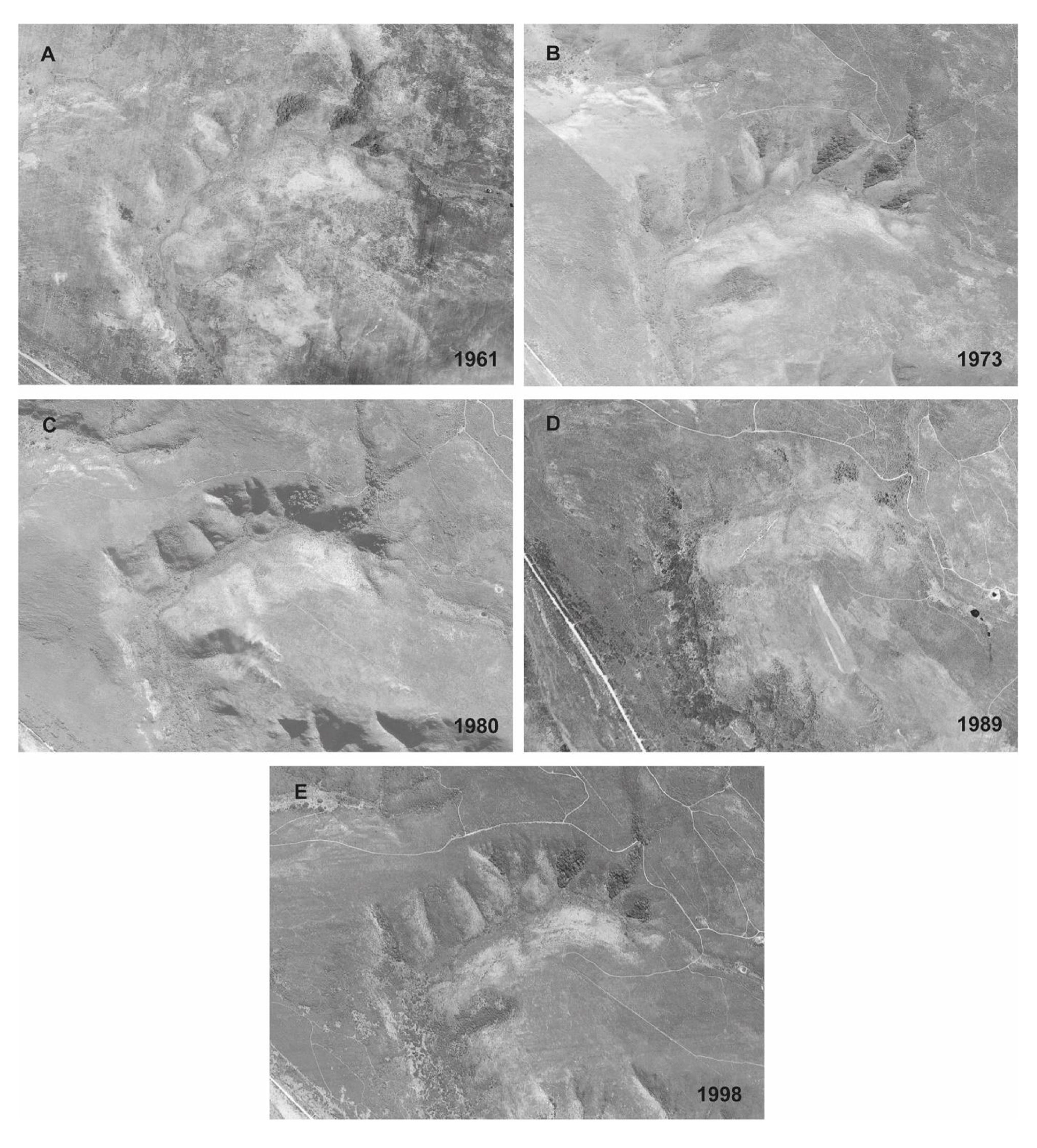
Figure 6 Aerial photographs showing changes in fire activity and vegetation cover in the wider area of Baviaansfontein over 37 years. From 1961 to 1980 (A–C) fire activity and intensive farming were common, as indicated by the relatively open landscape and the diffuse and irregular boundaries of the vegetation. From 1980 (C), there was a decline in fire activity, leading to a uniform increase in vegetation cover (C–E). (Aerial photographs: Department of Agriculture, Land Reform, and Rural Development in Mowbray, Cape Town: National Geospatial Information: (A) NGI job no. 461-11-08595; (B) NGI job no. 719-30-01778; (C) NGI job no. 498-148-01-01275; (D) NGI job no. 931-07-02107; (E) NGI job no. 1004-24-03373).
The results suggest that humans have created new interventions between vegetation and fire through land use changes or fire management strategies (Campbell and Moll, 1977; Luger and Moll, 1993). Both are paramount in reshaping the landscapes and could inform future conservation approaches based on a return to landscapes that better reflect vegetation states that dominated before intensive fire management.
The continuing and overall increasing anthropogenic pressure on the landscape is obvious and further inferred from the increasing input of pollen derived from introduced plants such as Pinus (Figures 3, 4). While Grootbos has never been used for pine plantations, a few scattered pines have established in the reserve, having colonized from more distant plantation areas. The increased input of pine pollen could reflect a regional, rather than a local signal, as bisaccate pollen often have a more distant area of origin (e.g., Hooghiemstra et al., 1988). The increase in pine pollen most likely corresponds to a signal of an increase in pine as well as other introduced species in the region. Pines are among the many invasive species that have largely been eradicated from Grootbos but are still abundant in the broader Agulhas Plain region, where their uncontrolled spread is a widespread problem (Gaertner et al., 2011; Boxriker et al., 2022).
4.4 The establishment of today’s landscape (Zone IV)
The pollen assemblage in the top 10 cm of the core is rich in afrotemperate forest- and wetland taxa. This is in contrast to the pollen influx of fynbos taxa which is relatively low (Figure 4). This shift in palynological composition suggests that the progressive expansion of forest elements has resulted in a switch to an alternate stable state at Baviaansfontein between forest and fynbos at around ~1985 CE (Figure 3). The continued reduction in micro- and macrocharcoal coincides with the forest-fynbos shift and is co-incident with forest expansion, while hydroclimatic conditions (yearly precipitation records Stanford for the period 1925–1995 CE) appear to be stable (Figure 4; Supplementary Figure 7).
The fires during this interval occurred mostly in responses to the conservation strategy of implementing management burns in the mid-1990s in order to maintain fynbos vegetation on Grootbos (Privett and Lutzeyer, 2010). Owing to the rugged nature of the landscape in and around the Baviaansfontein site, controlled management burns have not been feasible, while potential wildfires that may have burnt the site have been suppressed by management authorities (with the exception of the extensive 2006 wildfire). As a result, the micro- and macrocharcoal input has remained relatively low (Figure 4) and perhaps explains why afrotemperate forest has continued to grow. Controlled burning has not occurred near forest edges or biome boundaries, allowing forest precursor species to spread. As a result, forest cover remains more extensive than at the start of the record (Figure 4).
The longer fire frequency as a result of fire suppression and the inability to implement management burns ensures that proteoid fynbos is dominant at the Baviaansfontein site and therefore explains the high proportion of Proteaceae pollen in this interval (Figure 3; Privett and Lutzeyer, 2010).
The shift from fynbos to an alternate stable forest state also coincides with the expansion of characteristic wetland vegetation at the bottom of the valley. The pollen assemblage in the top layer is rich in Passerina, Cliffortia, and Cyperaceae (Figure 3), which are typical wetland taxa and often grow together with Leonotis leonurus (Lamiaceae) and Pteris dentata (Pteridaceae). The increase in pollen of these species suggests the expansion over this time period of the wetland complex at Grootbos (Figures 3, 4; compare Mergili and Privett, 2008). It appears that the wetland development and the establishment of the afrotemperate forest in its present form coincide with changes in the minerogenic composition (Figures 4, 5). The enrichment of soils with CaO and Al2O3 corresponds to the enhanced deposition of clay minerals, which was most likely driven by an increased mineral dust input, as the parent rock (quarzitic sandstone: Table Mountain Group) is generally poor in these elements (Thwaites and Cowling, 1988; Soderberg and Compton, 2007). This interpretation accords well with lithological Unit 6, which contains a higher amount of clay minerals than, for example, lithological Unit I (compare 3.2). Additionally, there is a simultaneous drop in SiO2, which has occurred perhaps in response to a decline in gravel and coarser-grained sand in this interval, and most likely originated from the underlining bedrock (Figures 4, 5; compare 3.2; Thwaites and Cowling, 1988; Soderberg and Compton, 2007; Mackenzie et al., 2017). As precipitation remained relatively constant throughout the time period the transition leading to the wetland expansion was perhaps initiated and controlled through changes in the minerogenic composition. The progressive aggregation of clay layers in the bottom of the valley created a strong positive feedback loop between wetland expansion and soil as clayey soils have a higher capacity to retain water (Karaborni et al., 1996; Sposito et al., 1999). While an increased aggregation of clay minerals through forest expansion can be ruled out, as there are no remarkable differences between the soil composition of fynbos and forest (Cramer et al., 2019). The decreasing input of coprophilous fungi most likely indicates the termination of extensive livestock farming in the Grootbos area (Figure 3; P. Strauss, Grootbos Foundation, pers. comm.). However, neither the clearance of alien species nor the charcoal signals of the 2006 fire are recorded in the palynomorph assemblage (Figures 3, 4).
This interval shows that the expansion of the afrotemperate forest and wetland is a contemporaneous phenomenon in which the wetland expansion in its modern form is possibly related to the increased clay deposition, while the forest expansion is related to decreasing fire. The current long interval between fires at Baviaansfontein allows forest and proteoid fynbos to coexist and indicates that the manipulation of fire regimes can affect vegetation structure and composition.
4.5 How to move forward: conservation strategies for a sustainable future
The palaeoecological approach outlined in this paper suggests that the spread of the afrotemperate forest at Baviaansfontein is a modern phenomenon of the past few decades and does not correspond to a more extensive historical forest expansion within the last 250 yr. This is similar to the Cape Peninsula, where areas which were once covered with fynbos vegetation have become increasingly dominated by afrotemperate forests (Poulsen and Hoffman, 2015; Slingsby et al., 2020; Prader et al., 2023). Altered and reduced fire frequencies are the main driver for modern forest expansion (Poulsen and Hoffman, 2015; Slingsby et al., 2020; Prader et al., 2023). However, at Baviaansfontein there remains a tension between preventing forest expansion through more frequent burning and maintaining proteoid-dominated fynbos vegetation that cannot withstand frequent fires (Kraaij et al., 2013; Valsecchi et al., 2013; MacPherson et al., 2019; van Wilgen, 1981). Under the expected warmer future climates predicted for the winter rainfall region (Mbokodo et al., 2020; Jain et al., 2022), forests at Baviaansfontein will most likely retreat and again become restricted, as likely was the case during the LIA (~1750–1850 CE). Furthermore, forest composition through the last 250 years has remained largely unchanged apart from an increase in Euclea and Searsia (Figure 3). There is no clear palynological evidence that Podocarpus grew at Baviaansfontein in the last 250 years. Because of this, Grootbos is not planting Podocarpus trees in their tree planting initiatives nor do they plan to incorporate this species in the future. It remains a matter of interest to know whether this species did historically occur in the Afrotemperate forests at Grootbos as there are Podocarpus populations as close as 17km NE of the Baviaansfontein area. Based on the palynological analysis presented here, forest expansion would not be recommended at Baviaansfontein. However, comparison with other sites in the area will be required in order to develop a more general policy concerned with reforestation in the area.
As with the predicted warmer climate, more frequent and more intense fires are also expected (Mbokodo et al., 2020; Jain et al., 2022). If this does occur then our results suggest that, in the absence of active management, proteoid fynbos will most likely decline and become dominated by more asteraceous fynbos taxa, which can withstand hotter fires and shorter return intervals. It is the intention of management at Grootbos to allow wildfires to move through the landscape within natural fire frequencies, but, in the absence of wildfires, to attempt to implement management burns across the site (Anonymous, 2023). As fire is one factor which can be manipulated, the proteoid fynbos at Baviaansfontein could persist, or potentially be transitioned back to a more asteraceous fynbos landscape through active management. Grootbos Reserve management does conduct management burns across the Reserve with the aim of creating a mosaic of vegetation patches with different post-fire ages where feasible. However, the Baviaansfontein kloof is steep and relatively inaccessible and it would be difficult for managers of the reserve to carry out a safe, controlled burn.
This study adds to a growing body of high-resolution palaeoecological studies that can help inform management and restoration of fynbos and associated vegetation, for example by informing appropriate levels of fire and grazing (e.g. Forbes et al., 2018) or in flagging possible changes in Fynbos composition in warmer drier climates (e.g. Prader et al., 2023; MacPherson et al., 2018; 2019).
4.6 Conclusion
Throughout 250 years the fynbos biome dominated the Baviaansfontein landscape and patches of afrotemperate forest existed in fire-sheltered areas. The pollen-based reconstruction revealed that between ~1760 and ~1850 CE a more open asteraceous fynbos type dominated the landscape as the relative percentage of Aster-type pollen is high. This fynbos type was possibly maintained by an increase of fire and continued to dominate until ~1980 CE. Fire remained a common element until about ~1950 CE. At this time, a slight increase of forest taxa coincides with an overall reduction of fires and perhaps indicates the beginning of forest expansion. Anthropogenic activity increases continuously towards the modern period. This is indicated by the influx of pollen from non-native plants, such as Pinus. While the observed increased input of fungal spores is possibly associated with livestock, it cannot unequivocally be assigned to increased anthropogenic activity. At around ~1980 CE asteraceous dominated fynbos type shifted into a proteoid fynbos biome which is prevalent at Baviaansfontein until today. In the following decades, the establishment of the afrotemperate forest and wetland developed into its present form.
This palynological investigation reveals that recent expansion of forest around Baviaansfontein is a modern phenomenon and does not mirror a resurgence of a more widespread forest in the past; at least not over the past 270 yr. The relatively recent expansion was most likely driven by fire suppression, whose effects overwhelmed the capacity of fynbos to resist the encroachment of forest taxa through internal reorganization. Based on this study and the predicted warming and increase in fire in the CFR a further expansion of forest vegetation is unlikely, while for the fynbos vegetation a return to a more open, asteraceous-fynbos type could be possible.
The study is a further illustration of the relevance of palaeoecology in informing restoration targets and ecosystem management in a changing climate.
Data availability statement
The datasets of this research will be available at UCT’s Ziva Hub (zivahub.uct.ac.za) and on the Neotoma Paleoecology Database. The raw data supporting the conclusions of this article will be made available by the authors, without undue reservation.
Ethics Statement
Ethical approval was not required for the study involving humans in accordance with the local legislation and institutional requirements. Written informed consent to participate in this study was not required from the participants or the participants' legal guardians/next of kin in accordance with the national legislation and the institutional requirements.
Author contributions
SP: Data curation, Formal analysis, Investigation, Visualization, Writing – original draft. LG: Conceptualization, Funding acquisition, Writing – review & editing. PS: Resources, Writing – review & editing. SP: Conceptualization, Writing – review & editing. MH: Conceptualization, Funding acquisition, Writing – review & editing.
Funding
The author(s) declare financial support was received for the research, authorship, and/or publication of this article. This research was funded by the National Research Foundation of South Africa/Global Change Grand Challenge/SASSCAL (Grant number 118589), the NRF Competitive Programme for Rated Researchers (Grant Number 118538), and the Plant Conservation Unit University of Cape Town.
Acknowledgments
We thank Manzano, S., Strahlendorf, JD. and Grootbos staff who assisted with retrieving the sediment cores. We thank Rossouw, R., and Grobbelaar-Moolman, M., from the Central Analytical Facilities at Stellenbosch University for conducting geochemical sample processing and Hess, S. for permission to use the HF-Laboratory at EGS. Dames R. is acknowledged for the contribution of the Grootbos map.
Conflict of interest
The authors declare that the research was conducted in the absence of any commercial or financial relationships that could be construed as a potential conflict of interest.
The author(s) declared that they were an editorial board member of Frontiers, at the time of submission. This had no impact on the peer review process and the final decision.
Publisher’s note
All claims expressed in this article are solely those of the authors and do not necessarily represent those of their affiliated organizations, or those of the publisher, the editors and the reviewers. Any product that may be evaluated in this article, or claim that may be made by its manufacturer, is not guaranteed or endorsed by the publisher.
Supplementary material
The Supplementary Material for this article can be found online at: https://www.frontiersin.org/articles/10.3389/fcosc.2024.1357711/full#supplementary-material
References
Anonymous. (2023). Conservation management plan - grootbos nature reserve” (Grootbos Nature Reserve: Grootbos Foundation Conservation and Research Unit). Unpublished document.
Barak R. S., Hipp A. L., Cavender-Bares J., Pearse W. D., Hotchkiss S. C., Lynch E. A., et al. (2016). Taking the long view: integrating recorded, archeological, paleoecological, and evolutionary data into ecological restoration. Int. J. Plant Sci. 177, 90–102. doi: 10.1086/683394
Bennett K. D., Willis K. J. (2001). “Pollen,” in Tracking environmental change using lake sediments. Eds. Smol J. P., Birks H. J. B., Last W. M., Bradley R. S., Alverson K. (Springer Netherlands, Dordrecht), 5–32.
Bernhardt C. E., Willard D. A. (2009). Response of the Everglades ridge and slough landscape to climate variability and 20th-century water management. Ecol. Appl. 19, 1723–1738. doi: 10.1890/08-0779.1
Birks H. J. B., Felde V. A., Bjune A. E., Grytnes J.-A., Seppä H., Giesecke T. (2016). Does pollen-assemblage richness reflect floristic richness? A review of recent developments and future challenges. Rev. Palaeobotany Palynology 228, 1–25. doi: 10.1016/j.revpalbo.2015.12.011
Blaauw M., Christen J. A. (2011). Flexible paleoclimate age-depth models using an autoregressive gamma process. Bayesian Anal. 6, 457–474, 418. doi: 10.1214/11-BA618
Bond W. J., Stevens N., Midgley G. F., Lehmann C. E. R. (2019). The trouble with trees: afforestation plans for Africa. Trends Ecol. Evol. 34, 963–965. doi: 10.1016/j.tree.2019.08.003
Boxriker M., Privett S. D. J., Richardson D. M., Gaertner M. (2022). Active restoration in South African fynbos – A long-term perspective from the Agulhas Plain. Trans. R. Soc. South Afr. 77, 133–143. doi: 10.1080/0035919X.2022.2087116
Campbell B. M. (1986). Montane plant communities in the fynbos biome. Vegetatio 66, 3–16. doi: 10.1007/BF00044078
Campbell B. M., Moll E. J. (1977). The forest communities of Table Mountain, South Africa. Vegetatio 34, 105–115. doi: 10.1007/BF00054478
Canadell J. G., Raupach M. R. (2008). Managing forests for climate change mitigation. Science 320, 1456–1457. doi: 10.1126/science.1155458
Carr A. S., Thomas D. S. G., Bateman M. D. (2006). Climatic and sea level controls on Late Quaternary eolian activity on the Agulhas Plain, South Africa. Quaternary Res. 65, 252–263. doi: 10.1016/j.yqres.2005.10.001
Chase B. M., Boom A., Carr A. S., Meadows M. E., Reimer P. J. (2013). Holocene climate change in southernmost South Africa: rock hyrax middens record shifts in the southern westerlies. Quaternary Sci. Rev. 82, 199–205. doi: 10.1016/j.quascirev.2013.10.018
Chase B. M., Chevalier M., Boom A., Carr A. S. (2017). The dynamic relationship between temperate and tropical circulation systems across South Africa since the last glacial maximum. Quaternary Sci. Rev. 174, 54–62. doi: 10.1016/j.quascirev.2017.08.011
Coetsee C., Stock W. D., Craine J. M. (2011). Do grazers alter nitrogen dynamics on grazing lawns in a South African savannah? Afr. J. Ecol. 49, 62–69. doi: 10.1111/j.1365-2028.2010.01236.x
Compton J. H. (2016). Human origins: how diet, climate and landscape shaped us (Cape Town: Earthspun Books).
Conedera M., Tinner W., Neff C., Meurer M., Dickens A. F., Krebs P. (2009). Reconstructing past fire regimes: methods, applications, and relevance to fire management and conservation. Quaternary Sci. Rev. 28, 555–576. doi: 10.1016/j.quascirev.2008.11.005
Cramer M. D., Power S. C., Belev A., Gillson L., Bond W. J., Hoffman M. T., et al. (2019). Are forest-shrubland mosaics of the Cape Floristic Region an example of alternate stable states? Ecography 42, 717–729. doi: 10.1111/ecog.03860
Deacon H. J. (1992). “Human settlement,” in The ecology of Fynbos: Nutrients, fire and diversity. Ed. Cowling R. M. (Oxford University Press, Cape Town), 260–270.
Diechmann U., Eklundh L. (1991). Global digital data sets for land degradation studies: a GIS approach. GRID Case Study Series No. 4 (Nairobi, Kenya: UNEP/GEMS and GRID).
du Plessis N., Chase B. M., Quick L. J., Haberzettl T., Kasper T., Meadows M. E. (2020). Vegetation and climate change during the Medieval Climate Anomaly and the Little Ice Age on the southern Cape coast of South Africa: Pollen evidence from Bo Langvlei. Holocene 30, 1716–1727. doi: 10.1177/0959683620950444
Esler K. J., Cowling R. M. (1990). Effects of density on the reproductive output of Protea lepidocarpodendron. South Afr. J. Bot. 56, 29–33. doi: 10.1016/S0254-6299(16)31107-3
Finsinger W., Tinner W. (2005). Minimum count sums for charcoal concentration estimates in pollen slides: accuracy and potential errors. Holocene 15, 293–297. doi: 10.1191/0959683605hl808rr
Forbes C. J., Gillson L., Hoffman M. T. (2018). Shifting baselines in a changing world: Identifying management targets in endangered heathlands of the Cape Floristic Region, South Africa. Anthropocene 22, 81–93. doi: 10.1016/j.ancene.2018.05.001
Gaertner M., Richardson D. M., Privett S. D. J. (2011). Effects of alien plants on ecosystem structure and functioning and implications for restoration: insights from three degraded sites in South African fynbos. Environ. Manage. 48, 57–69. doi: 10.1007/s00267-011-9675-7
Ghantous G. (2021). Saudi Arabia sees fields of green with major tree-planting drive (Dubai: Reuters).
Giddey B. L., Baard J. A., Kraaij T. (2022). Fire severity and tree size affect post-fire survival of Afrotemperate forest trees. Fire Ecol. 18, 5. doi: 10.1186/s42408-022-00128-5
Gillson L., Dirk C., Gell P. (2021). Using long-term data to inform a decision pathway for restoration of ecosystem resilience. Anthropocene 36, 100315. doi: 10.1016/j.ancene.2021.100315
Gillson L., Hoffman M. T., Gell P. A., Ekblom A., Bond W. J. (2023). Trees, carbon, and the psychology of landscapes. Trends Ecol. Evol. doi: 10.1016/j.tree.2023.11.008
Gillson L., MacPherson A. J., Hoffman M. T. (2020). Contrasting mechanisms of resilience at mesic and semi-arid boundaries of fynbos, a mega-diverse heathland of south africa. Ecol. Complexity 42, 100827.
Govin A., Holzwarth U., Heslop D., Ford Keeling L., Zabel M., Mulitza S., et al. (2012). Distribution of major elements in Atlantic surface sediments (36°N–49°S): Imprint of terrigenous input and continental weathering. Geochemistry Geophysics Geosystems 13, 1–23. doi: 10.1029/2011GC003785
Granger R., Meadows M. E., Hahn A., Zabel M., Stuut J.-B. W., Herrmann N., et al. (2018). Late-Holocene dynamics of sea-surface temperature and terrestrial hydrology in southwestern Africa. Holocene 28, 695–705. doi: 10.1177/0959683617744259
Greenpop (2023) Forest restoration. Available online at: https://greenpop.org/forest-restoration/ (Accessed September 2023).
Grootbos Foundation (2023a) Future trees. Available online at: https://grootbosfoundation.org/conservation-research-unit/ (Accessed September 2023).
Grootbos Foundation (2023b) Elim Ferricrete fynbos. Available online at: https://www.grootbosfoundation.org/conservation/2021/10/13/grootbos-foundation-elim-ferricrete-fynbos/ (Accessed 04 December 2023).
Heiri O., Lotter A. F., Lemcke G. (2001). Loss on ignition as a method for estimating organic and carbonate content in sediments: reproducibility and comparability of results. J. Paleolimnology 25, 101–110. doi: 10.1023/A:1008119611481
Heydenrych B. J. (1999). An investigation of land-use practices on the Agulhas Plain (South Africa), with emphasis on socio-economic and conservation issues. [master’s thesis]. University of Cape Town.
Hogg A. G., Heaton T. J., Hua Q., Palmer J. G., Turney C. S. M., Southon J., et al. (2020). SHCal20 southern hemisphere calibration, 0–55,000 years cal BP. Radiocarbon 62, 759–778. doi: 10.1017/RDC.2020.59
Holbourn A., Kuhnt W., Kochhann K. G. D., Andersen N., Sebastian Meier K. J. (2015). Global perturbation of the carbon cycle at the onset of the Miocene Climatic Optimum. Geology 43, 123–126. doi: 10.1130/G36317.1
Holl K. D., Brancalion P. H. S. (2020). Tree planting is not a simple solution. Science 368, 580–581. doi: 10.1126/science.aba8232
Hooghiemstra H., Maizels J., Ward R. G. W., Shackleton N. J., West R. G., Bowen D. Q. (1988). Palynological records from northwest African marine sediments: A general outline of the interpretation of the pollen signal. Philos. Trans. R. Soc. London. B Biol. Sciences 318, 431–449. doi: 10.1098/rstb.1988.0018
Jain P., Castellanos-Acuna D., Coogan S. C. P., Abatzoglou J. T., Flannigan M. D. (2022). Observed increases in extreme fire weather driven by atmospheric humidity and temperature. Nat. Climate Change 12, 63–70. doi: 10.1038/s41558-021-01224-1
Juggins S. (2007). C2 Version 1.5: Software for ecological and palaeoecological data analysis and visualisation. Newcastle upon Tyne.
Juggins S. (2022) rioja: Analysis of Quaternary Science Data, R package version (1.0-5). Available online at: https://cran.r-project.org/package=rioja.
Karaborni S., Smit B., Heidug W., Urai J., van Oort E. (1996). The swelling of clays: molecular simulations of the hydration of montmorillonite. Science 271, 1102–1104. doi: 10.1126/science.271.5252.1102
Kirsten K. (2014). Late Holocene diatom community responses to climate variability along the southern Cape coastal plain, South Africa. [dissertation thesis]. University of Cape Town.
Kraaij T., Cowling R. M., van Wilgen B. W., Schutte-Vlok A. (2013). Proteaceae juvenile periods and post-fire recruitment as indicators of minimum fire return interval in eastern coastal fynbos. Appl. Vegetation Sci. 16, 84–94. doi: 10.1111/j.1654-109X.2012.01209.x
Lu M., Bond W. J., Sheffer E., Cramer M. D., West A. G., Allsopp N., et al. (2022). Biome boundary maintained by intense belowground resource competition in world’s thinnest-rooted plant community. Proc. Natl. Acad. Sci. 119, e2117514119. doi: 10.1073/pnas.2117514119
Luger A. D., Moll E. J. (1993). Fire protection and afromontane forest expansion in cape fynbos. Biol. Conserv. 64, 51–56. doi: 10.1016/0006-3207(93)90382-B
Mackenzie L., Heijnis H., Gadd P., Moss P., Shulmeister J. (2017). Geochemical investigation of the South Wellesley Island wetlands: Insight into wetland development during the Holocene in tropical northern Australia. Holocene 27, 566–578. doi: 10.1177/0959683616670219
MacPherson A. J., Gillson L., Hoffman M. T. (2019). Between- and within-biome resistance and resilience at the fynbos-forest ecotone, South Africa. Holocene 29, 1801–1816. doi: 10.1177/0959683619862046
MacPherson A., Gillson L., Hoffman M. T. (2018). Climatic buffering and anthropogenic degradation of a mediterranean-type shrubland refugium at its semi-arid boundary, south africa. Holocene 28 (4), 651–666. doi: 10.1177/0959683617735582
Manders P. T., Richardson D. M. (1992). Colonization of Cape fynbos communities by forest species. For. Ecol. Manage. 48, 277–293. doi: 10.1016/0378-1127(92)90150-8
Masson P. H., Moll E. J. (1987). The factors affecting forest colonisation of fynbos in the absence of recurrent fire at Orange Kloof, Cape Province, South Africa. South Afr. Forestry J. 143, 5–10. doi: 10.1080/00382167.1987.9630294
Mbokodo I., Bopape M.-J., Chikoore H., Engelbrecht F., Nethengwe N. (2020). Heatwaves in the future warmer climate of South Africa. Atmosphere 11, 712. doi: 10.3390/atmos11070712
McKenzie B., Moll E. J., Campbell B. M. (1976). A suggested management plan for the indigenous vegetation of Orange Kloof, Table Mountain, based on a phytosociological survey. South African Forestry Journal 99(1), 1–6. doi: 10.1080/00382167.1976.9630102
Mergili M., Privett S. (2008). Vegetation and vegetation-environment relationships at Grootbos Nature Reserve, Western Cape, South Africa. Bothalia 38, 89–102. doi: 10.4102/abc.v38i1.273
Moncrieff G. R., Slingsby J. A., Le Maitre D. C. (2021). Propagating uncertainty from catchment experiments to estimates of streamflow reduction by invasive alien plants in southwestern South Africa. Hydrological Processes 35, e14161. doi: 10.1002/hyp.14161
Mucina L., Geldenhuys C. J. (2006). “Temperate, subtropical and azonal forests,” in The vegetation of South Africa, Lesotho and swaziland. Eds. Mucina L., Rutherford M. C. (South African National Biodiversity Institute, Pretoria, South Africa), 585–615.
Neumann F. H., Scott L., Bamford M. K. (2011). Climate change and human disturbance of fynbos vegetation during the late Holocene at Princess Vlei, Western Cape, South Africa. Holocene 21, 1137–1149. doi: 10.1177/0959683611400461
Oksanen J., Simpson G., Blanchet F., Kindt R., Legendre P., Minchin P., et al. (2022). vegan: Community Ecology Package_. R package version 2. 6–4.
Oksanen J., Blanchet F. G., Friendly M., et al. (2019). Vegan: Community ecology package (Vienna: R Core Team).
PAGES 2k Consortium (2013). Continental-scale temperature variability during the past two millennia. Nat. Geosciences 6, 339–346. doi: 10.1038/ngeo1797
Pan Y., Birdsey R. A., Fang J., Houghton R., Kauppi P. E., Kurz W. A., et al. (2011). A large and persistent carbon sink in the world’s forests. Science 333, 988–993. doi: 10.1126/science.1201609
Perren B. B., Hodgson D. A., Roberts S. J., Sime L., Van Nieuwenhuyze W., Verleyen E., et al. (2020). Southward migration of the Southern Hemisphere westerly winds corresponds with warming climate over centennial timescales. Commun. Earth Environ. 1, 58. doi: 10.1038/s43247-020-00059-6
Peterson B. G., Carl P. (2020). PerformanceAnalytics: Econometric Tools for Performance and Risk Analysis. R package version 2.0.4.
Platbos Africa’s Southernmost Forest. (2023). Available online at: https://platbos.co.za/ (Accessed September 2023).
Pooley S. (2009). Jan van Riebeeck as Pioneering Explorer and Conservator of Natural Resources at the Cape of Good Hope, (1652-62). Environ. History 15, 3–33. doi: 10.3197/096734009X404644
Pooley S. (2014). Burning Table Mountain: An environmental history of fire on the Cape Peninsula (Cape Town: South Africa: UCT Press). doi: 10.1057/9781137415448
Pooley S. (2018). “The long and entangled history of humans and invasive introduced plants on South Africa’s cape peninsula,” in Histories of bioinvasions in the mediterranean. Eds. Queiroz A. I., Pooley. S. (Springer International Publishing, Cham), 219–251.
Poulsen Z. C., Hoffman M. T. (2015). Changes in the distribution of indigenous forest in Table Mountain National Park during the 20th Century. South Afr. J. Bot. 101, 49–56. doi: 10.1016/j.sajb.2015.05.002
Power M. J., Marlon J. R., Bartlein P. J., Harrison S. P. (2010). Fire history and the Global Charcoal Database: A new tool for hypothesis testing and data exploration. Palaeogeography Palaeoclimatology Palaeoecol. 291, 52–59. doi: 10.1016/j.palaeo.2009.09.014
Prader S., Gillson L., Chase B. M., Hoffman M. T. (2023). Late-Holocene fynbos-forest dynamics in Orange Kloof, Table Mountain National Park, South Africa. Holocene 33, 592–604. doi: 10.1177/09596836231151823
Privett S., Lutzeyer H. (2010). Field guide to the flora of grootbos nature reserve and the walker bay region (Grootbos Foundation: Singapore, Grootbos Foundation, Craft Print International Ltd).
Quick L. J., Chase B. M., Wündsch M., Kirsten K. L., Chevalier M., Mäusbacher R., et al. (2018). A high-resolution record of Holocene climate and vegetation dynamics from the southern Cape coast of South Africa: pollen and microcharcoal evidence from Eilandvlei. J. Quaternary Sci. 33, 487–500. doi: 10.1002/jqs.3028
Quick L. J., Meadows M. E., Bateman M. D., Kirsten K. L., Mäusbacher R., Haberzettl T., et al. (2016). Vegetation and climate dynamics during the last glacial period in the fynbos-afrotemperate forest ecotone, southern Cape, South Africa. Quaternary Int. 404, 136–149. doi: 10.1016/j.quaint.2015.08.027
R Core Team (2021). R: A language and environment for statistical computing (Vienna: R Foundation for Statistical Computing). Available at: https://www.R-project.org.
Ricciardi L., D’Odorico P., Galli N., Chiarelli D. D., Rulli M. C. (2022). Hydrological implications of large-scale afforestation in tropical biomes for climate change mitigation. Philos. Trans. R. Soc. B: Biol. Sci. 377, 20210391. doi: 10.1098/rstb.2021.0391
Sadr K. (2003). The neolithic of Southern Africa. J. Afr. History 44, 195–209. doi: 10.1017/S0021853702008393
Sadr K. (2008). Invisible herders? The archaeology of Khoekhoe pastoralists. South. Afr. Humanities 20, 179–203. doi: 10.10520/EJC84795
Santisteban J. I., Mediavilla R., López-Pamo E., Dabrio C. J., Zapata M. B. R., García M. J. G., et al. (2004). Loss on ignition: a qualitative or quantitative method for organic matter and carbonate mineral content in sediments? J. Paleolimnology 32, 287–299. doi: 10.1023/B:JOPL.0000042999.30131.5b
Scott L., Woodborne S. (2007). Vegetation history inferred from pollen in Late Quaternary faecal deposits (hyraceum) in the Cape winter-rain region and its bearing on past climates in South Africa. Quaternary Sci. Rev. 26, 941–953. doi: 10.1016/j.quascirev.2006.12.012
Shumilovskikh L. S., van Geel B. (2020). “Non-pollen Palynomorphs”, in Handbook for the analysis of micro-particles in archaeological samples, ed. Henry A. H. (Springer, Berlin), 65–94.
Simon M. H., Ziegler M., Bosmans J., Barker S., Reason C. J. C., Hall I. R. (2015). Eastern South African hydroclimate over the past 270,000 years. Sci. Rep. 5, 18153. doi: 10.1038/srep18153
Slingsby J. A., Moncrieff G. R., Rogers A. J., February E. C. (2020). Altered ignition catchments threaten a hyperdiverse fire-dependent ecosystem. Global Change Biol. 26, 616–628. doi: 10.1111/gcb.14861
Smith A. B. (1986). Competition, conflict and clientship: khoi and san relationships in the Western Cape. Goodwin Ser. 5, 36–41. doi: 10.2307/3858144
Soderberg K., Compton J. S. (2007). Dust as a nutrient source for fynbos ecosystems, South Africa. Ecosystems 10, 550–561. doi: 10.1007/s10021-007-9032-0
Song S., Ding Y., Li W., Meng Y., Zhou J., Gou R., et al. (2023). Mangrove reforestation provides greater blue carbon benefit than afforestation for mitigating global climate change. Nat. Commun. 14, 756. doi: 10.1038/s41467-023-36477-1
South African Reforestation Trust. (2023). Available online at: https://saforesttrust.com/ (Accessed September 2023).
Spellerberg I. F., Fedor P. J. (2003). A tribute to claude shannon, (1916–2001) and a plea for more rigorous use of species richness, species diversity and the ‘Shannon–Wiener’ Index. Global Ecol. Biogeography 12, 177–179. doi: 10.1046/j.1466-822X.2003.00015.x
Sposito G., Skipper N. T., Sutton R., Park S.-H., Soper A. K., Greathouse J. A. (1999). Surface geochemistry of the clay minerals. Proc. Natl. Acad. Sci. 96 (7), 3358–3364. doi: 10.1073/pnas.96.7.3358
Stager J. C., Mayewski P. A., White J., Chase B. M., Neumann F. H., Meadows M. E., et al. (2012). Precipitation variability in the winter rainfall zone of South Africa during the last 1400 yr linked to the austral westerlies. Climate the. Past 8, 877–887. doi: 10.5194/cp-8-877-2012
Stevenson J., Haberle S. G. (2005). Macro charcoal analysis: A modified technique used by the Department of Archaeology and Natural History. (PalaeoWorks Technical Report 5) (Canberra: Australian National University).
Stockmarr J. (1971). Tablets with spores used in absolute pollen analysis. Pollen Spores 13, 615–621.
Strobel P., Kasper T., Frenzel P., Schittek K., Quick L. J., Meadows M. E., et al. (2019). Late Quaternary palaeoenvironmental change in the year-round rainfall zone of South Africa derived from peat sediments from Vankervelsvlei. Quaternary Sci. Rev. 218, 200–214. doi: 10.1016/j.quascirev.2019.06.014
The African Forest Landscape Restoration Initiative (2023) Projects. Available online at: https://afr100.org/projects (Accessed September 2023).
Thuiller W., Slingsby J. A., Privett S. D., Cowling R. M. (2007). Stochastic species turnover and stable coexistence in a species-rich, fire-prone plant community. PloS One 2, e938. doi: 10.1371/journal.pone.0000938
Thwaites R. N., Cowling R. M. (1988). Soil-vegetation relationships on the agulhas plain, South Africa. CATENA 15, 333–345. doi: 10.1016/0341-8162(88)90055-0
Tinner W., Hu F. S. (2003). Size parameters, size-class distribution and area-number relationship of microscopic charcoal: relevance for fire reconstruction. Holocene 13, 499–505. doi: 10.1191/0959683603hl615rp
Traverse A. (2008). “Paleopalynology,” in Topics of geobiology series, 2nd ed., vol. 28 . Eds. Landman N. H., Jones D. S. (Springer Netherlands, Dordrecht), 1–813.
Troels-Smith J. (1955). Characterization of unconsolidated sediments. Geological Survey Denmark 3, 39–73.
Turner M. D., Davis D. K., Yeh E. T., Hiernaux P., Loizeaux E. R., Fornof E. M., et al. (2023). Great green walls: hype, myth, and science. Annu. Rev. Environ. Resour. 48, 263–287. doi: 10.1146/annurev-environ-112321-111102
Tyson P. D., Preston-Whyte R. A. (2000). “The weather and climate of Southern Africa” (Cape Town: Oxford University Press).
Valsecchi V., Chase B. M., Slingsby J. A., Carr A. S., Quick L. J., Meadows M. E., et al. (2013). A high resolution 15,600-year pollen and microcharcoal record from the Cederberg Mountains, South Africa. Palaeogeography Palaeoclimatology Palaeoecol. 387, 6–16. doi: 10.1016/j.palaeo.2013.07.009
van Geel B. (2001). “Non-pollen palynomorphs,” in Tracking environmental change using lake sediments: terrestrial, algal, and siliceous indicators. Eds. Smol J. P., Birks H. J. B., Last W. M., Bradley R. S., Alverson K. (The Netherlands: Springer Netherlands, Dordrecht), 99–119.
van Geel B., Aptroot A. (2006). Fossil ascomycetes in Quaternary deposits. Nova Hedwigia 82, 313–329.
van Wilgen B. W. (2009). The evolution of fire and invasive alien plant management practices in fynbos. South Afr. J. Sci. 105, 335–342. Available at: https://hdl.handle.net/10520/EJC96966.
van Wilgen B. W., Carruthers J., Cowling R. M., Esler K. J., Forsyth A. T., Gaertner M., et al. (2016). Ecological research and conservation management in the Cape Floristic Region between 1945 and 2015: History, current understanding and future challenges. Trans. R. Soc. South Afr. 71, 207–303. doi: 10.1080/0035919X.2016.1225607
van Wilgen B. W. (1981). Some effects of fire frequency on fynbos plant community composition and structure at jonkershoek, stellenbosch. South Afr. Forestry J. 118 (1), 42–55. doi: 10.1080/00382167.1981.9630524
Weldeab S., Stuut J. B. W., Schneider R. R., Siebel W. (2013). Holocene climate variability in the winter rainfall zone of South Africa. Clim. Past 9, 2347–2364. doi: 10.5194/cp-9-2347-2013
Whitlock C., Larsen C. (2001). “Charcoal as a fire proxy,” in Tracking environmental change using lake sediments: terrestrial, algal, and siliceous indicators. Eds. Smol J. P., Birks H. J. B., Last W. M., Bradley R. S., Alverson K. (Springer Netherlands, Dordrecht), 75–97.
Wicht C. L. (1945). “Report of the committee on the preservation of the vegetation of the Southwestern Cape” (Cape Town, South Africa: Special Publication of the Royal Society of South Africa).
Wickham H. (2016). “ggplot2: elegant graphics for data analysis” (New York: Springer-Verlag). Available at: https://ggplot2.tidyverse.org, ISBN: ISBN 978-3-319-24277-4.
Wingard G. L., Bernhardt C. E., Wachnicka A. H. (2017). The role of paleoecology in restoration and resource management—The past as a guide to future decision-making: review and example from the greater everglades ecosystem, U.S.A. Front. Ecol. Evol. 5. doi: 10.3389/fevo.2017.00011
World Resource Institute (2023) Global forest watch. Available online at: https://www.globalforestwatch.org/ (Accessed September 2023).
Wündsch M., Haberzettl T., Kirsten K. L., Kasper T., Zabel M., Dietze E., et al. (2016). Sea level and climate change at the southern Cape coast, South Africa, during the past 4.2kyr. Palaeogeography Palaeoclimatology Palaeoecol. 446, 295–307. doi: 10.1016/j.palaeo.2016.01.027
Keywords: forest-fynbos boundaries, palynology, palaeoecology, compositional change, ecosystem conservation, fynbos, reforestation, restoration
Citation: Prader S, Gillson L, Strauss P, Privett SDJ and Hoffman MT (2024) A paleoecological context for forest distribution and restoration in Grootbos Nature Reserve, Agulhas Plain, South Africa. Front. Conserv. Sci. 5:1357711. doi: 10.3389/fcosc.2024.1357711
Received: 18 December 2023; Accepted: 16 February 2024;
Published: 14 March 2024.
Edited by:
José M. Rey Benayas, University of Alcalá, SpainReviewed by:
Simon Connor, Australian National University, AustraliaCésar Morales-Molino, University of Alcalá, Spain
José Carrión, University of Murcia, Spain
Copyright © 2024 Prader, Gillson, Strauss, Privett and Hoffman. This is an open-access article distributed under the terms of the Creative Commons Attribution License (CC BY). The use, distribution or reproduction in other forums is permitted, provided the original author(s) and the copyright owner(s) are credited and that the original publication in this journal is cited, in accordance with accepted academic practice. No use, distribution or reproduction is permitted which does not comply with these terms.
*Correspondence: Sabine Prader, sabineprader@gmail.com
†ORCID: Sabine Prader, orcid.org/0000-0003-0013-6154
Lindsey Gillson, orcid.org/0000-0001-9607-6760