- 1Center for Evolution and Conservation Biology, Southern Marine Science and Engineering Guangdong Laboratory (Guangzhou), Guangzhou, China
- 2CAS Key Laboratory of Animal Ecology and Conservation Biology, Institute of Zoology, Chinese Academy of Sciences, Beijing, China
- 3Key Laboratory of Conservation and Application in Biodiversity of South China, School of Life Sciences, Guangzhou University, Guangzhou, Guangdong, China
- 4Key Laboratory for City Cluster Environmental Safety and Green Development of the Ministry of Education, School of Ecology, Environment and Resources, Guangdong University of Technology, Guangzhou, China
- 5College of Forestry, Jiangxi Agricultural University, Nanchang, China
Coral reefs are among the most productive and diverse ecosystems on the planet, yet have suffered sharp declines in the past few decades. To better understand how reef fish communities respond to disturbances in the South China Sea, we investigated the species diversity, trophic composition, and shoaling size of fish communities of four coral reefs in different states of degradations. We found that reef degradation was related to the dominance of herbivores and the decline of planktivores, reflecting the shift towards an algae-dominant community causing by reef degradation. Additionally, degradation reduced the shoaling size of planktivores, but herbivores and omnivores might benefit from the degradation and formed larger groups in Yongle Reef. Our results shed light on the response of the reef fish community to habitat degradation and provided new insights into the conservation of coral reefs in the South China Sea.
Introduction
Coral reefs are one of the most productive ecosystems in the ocean. As keystone species of coral reef ecosystems, reef-forming corals created complex three-dimensional landscapes that support diverse reef-dwelling fish species with a range of feeding habits, from extreme specialists to flexible generalists (Floeter et al., 2018; Borstein et al., 2019). Despite their ecological importance, coral reefs have been subject to global degradation in recent decades due to natural and anthropogenic factors, such as climate change, outbreaks of crown-of-thorns starfish (CoTS, Acanthaster spp.), overfishing, and pollution (De'ath et al., 2012; Robinson et al., 2017; Hughes et al., 2018). This degradation has led to the mass death of living corals, increasing the erosion of coral skeletons by waves and reduced the structural complexity of reef, which weakened the ecosystem services of coral reefs (Zawada et al., 2019).
Increasing studies had demonstrated that degradation of coral reefs had substantial effects on the structure of reef fish assemblages (Smale et al., 2019). Habitat degradation, manifested as loss of coral diversity and coverage, can alter the structure of fish assemblages in a bottom-up manner. When habitat degradation occurs, species that cannot adapt to the new environment are eliminated, while species that utilize broken reef substrata, such as dead corals or rubble, would take their place. For example, a loss of structural complexity can reduce the refuges of small reef fish, exposing them to greater threats such as predation and fishing, leading to population decreases (Graham and Nash, 2013; Fontoura et al., 2020). In addition, degradation of coral reefs is often accompanied by algal blooms, which boosts the population of algae-eating fish while inhibiting the reproduction of other fish (Cheal et al., 2010). Moreover, the disruption of fish community structure, especially the loss of fish involved in coral recruitment, in turn impedes the natural recovery of corals and coral reef ecosystems, damaging the resilience of the coral reef ecosystem (Robinson et al., 2019). Therefore, the recruitment of fish species can drive the community composition of degraded coral reefs (Feary et al., 2007). Understanding the turnover of coral reef fish species after destruction will not only help us understand the ecological regulation mechanism of reef fish communities, but also promote the recovery of reef fish populations and the conservation of coral reef biodiversity.
Classification of trophic groups based on the diet of fish species provides new perspectives on the ecological roles of reef fish, contributing to understanding the relationship between species recruitment and environmental factors (Beukhof et al., 2019). For example, degraded coral reefs usually shift to an algae-dominant state, leading to the increase of herbivorous fish (Pandolfi et al., 2003). Moreover, herbivores can be further divided into several sub-guilds according to the different types of algae consumed, including grazer/detritivore, scaper/excavators and browsers. Both grazer/detritivore and scaper/excavators graze turf algae but the former rarely moved the underlying substratum, while scaper/excavators usually remove some underlying carbonate substratum when feeding, and browsers exclusively feed on macroalgae (Edwards et al., 2014). Therefore, the relative proportion of trophic groups indicates the different recruitment of fish in different degrees of degraded coral reefs. Shoaling behavior is also an important functional characteristic of reef fish, reflecting the living status in post-recruitment (Pitcher, 1986). A smaller group of fish may result from poor resources in the ecosystems and/or overfishing, suggesting a decline of the ecosystem functioning of coral reefs (Guerra et al., 2022). Investigating shoaling behavior can help to understand the regulation of coral reef fish behavior under environmental stress during degradation.
Coral reefs in the South China Sea (SCS) are important parts of the Coral Triangle, the hotspot of marine biodiversity (Stuart-Smith et al., 2013; Fan et al., 2023). However, the coral reefs in the SCS are facing a sharp decline due to the increased intensity of marine heat waves and outbreaks of CoTS (Wu et al., 2019; Yan et al., 2019; Xiao et al., 2022), resulting in the changes of fish community structure (Dai et al., 2022). Conservation of coral reefs in the SCS is urgently needed, but our understanding of fish communities’ response to degradation is far from enough (Zhou et al., 2021). To investigate the influence of different degrees of coral reef degradation on fish species recruitment, we surveyed the fish diversity of four typical coral reefs in the SCS and compared the species composition, the relative abundance of different trophic groups, and the shoaling size of key fish species of reefs at the same latitude.
Materials and methods
Study location and data collection
We conducted a survey on four coral reef sites at different degrees of degradation, Meiji Reef, Yongshu Reef, Qilian Island, and Yongle Islands, in May 2022. By comparing different islands and reefs within the same latitude and small scale, we aimed to explore the influence of reef plate destruction on the community structure of coral reef fish. This approach allowed us to eliminate the mixed influence of multiple objective factors such as latitude, ocean current, marine realms, and human fishing intensity to a certain extent. The Meiji Reef and Yongshu Reef located in the Nansha Islands in the SCS and were almost at the same latitude (~ 9°N) (Figure 1A). While Meiji Reef maintains a more complex coral structure community, Yongshu Reef was suffering from the predation of CoTS, which has disturbed the coral community structure to some extent (Figure 1B Upper left and right). The Qilian Island and Yongle Islands, located in the Xisha Islands in the northern SCS at ~16°N (Figure 1A), showed a different pattern. The degradation degree of coral biodiversity in Yongle Island was more serious than that in Qilian Island (Figure 1B Lower left and right). Typically, the reef-forming corals in Yongle Island have died off in large numbers, and have shifted to a soft-coral-covered ecosystem. The newly born soft corals have a single species composition and low spatial organization complexity. Overall, Meiji Reef and Yongshu Reef, as well as Qilian Island and Yongle Island, have similar hydrological and ecological environments, due to similar latitude, ocean current, marine realms, and human fishing pressure. However, due to the marine heat wave or the outbreak of CoTS and other reasons, the reefs of Yongshu Reef showed initial signs of degradation, and Yongle Island was seriously damaged, while the corresponding Meiji Reef and Qilian Island were well preserved (Table 1). How does this change further affect the community structure of its inhabitants, especially coral reef fish?
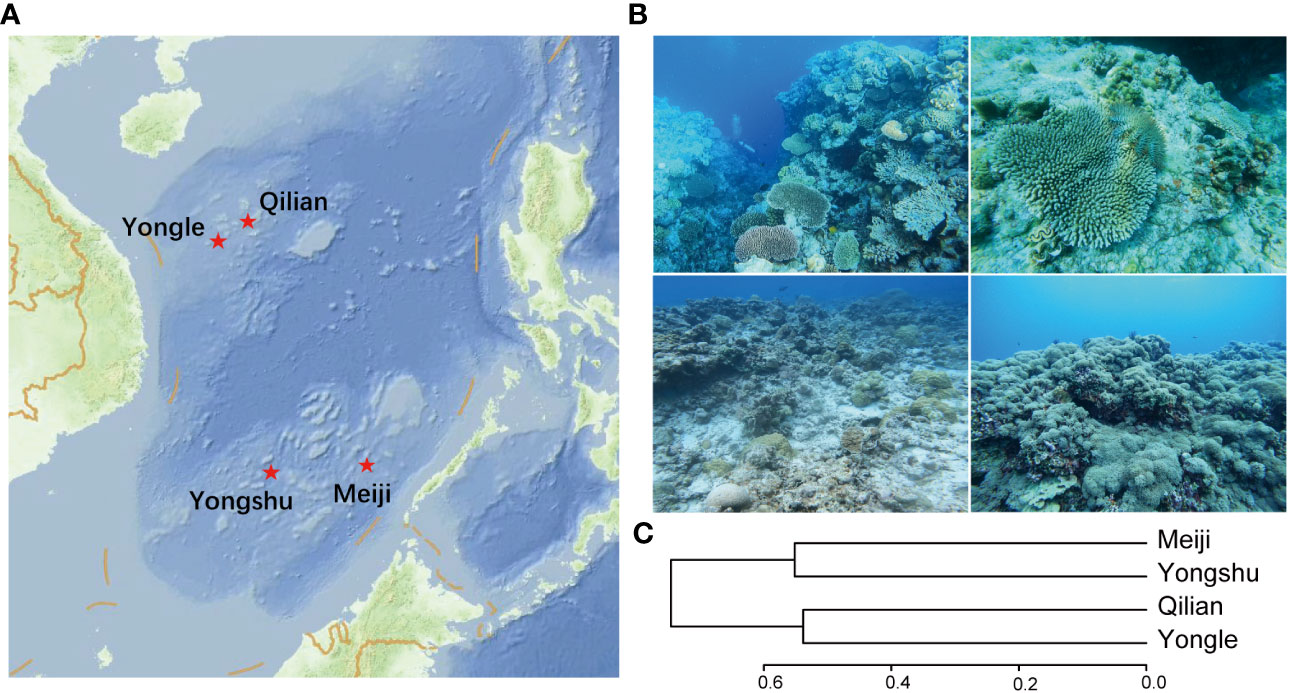
Figure 1 The location and status of coral reefs in this study. (A) Study areas in the South China Sea; (B) Photos showed the status of coral reefs across the four sites. Upper left: Meiji Reef with the highest structural complexity and complete ecosystem. Upper right: Yongshu Reef with high structural complexity but suffering the predation of CoTS. Lower left: Qilian Island with low reef coverage and structural complexity. Lower right: Yongle Islands with the shift to a soft-coral-covered community; (C) Community clustering results of the four coral reefs.
We totally set up 32 transects in Meiji Reef, 17 transects in Yongshu Reef, 9 transects in Qilian Reef, and 4 transects in Yongle Reef to conduct the underwater visual census (Table 2). The underwater visual census (UVC) was conducted by line transects by the same divers equipped with Gopro cameras at a depth of no more than 18 meters in the whole survey to avoid the survey bias. Each transect was no less than 50 meters in length or 15 minutes in dive time. We then analyzed the videos to identify fish species and abundance. Frame compliance was performed at a rate of 30 frames per second, using a 10-second sliding window. Each frame was divided into four parts, upper left, lower right, upper right and lower right to count the shoaling size of fish occurred at the same time. Any fish whose images were too fuzzy to be identified were excluded from the analysis. Additionally, the structural complexity of coral reefs in each transect was also recorded, which were crucial in understanding the relationship between fish abundance and reef structure.
Community comparison
We calculated the sample coverage and estimated species diversity of each reef fish community using the iNext function in the R inext package based on the abundance of each reef fish species (Hsieh et al., 2016). Hill numbers of different q orders were calculated. When q = 0, Hill numbers mean species richness; q = 1, Hill numbers mean Shannon diversity; q = 2, Hill numbers mean Simpson diversity. As q increases from 0 to 2, this index places less emphasis on the impact of rare species. Community clustering was conducted using the Bray-Curtis distance for species and family composition. Then we explored the species abundance patterns of each reef fish community to identify their distinct community structure.
Trophic groups
Trophic groups play a crucial role in reef fish communities’ functioning. In this research, reef fishes were categorized into five primary trophic groups: carnivores, corallivores, herbivores (including detritivores), omnivores and planktivores. To provide a more detailed understanding, carnivores were further divided into piscivores, general carnivores (feed on fish and invertebrates), invertivores, scaly fish and ectoparasite feeders, following Pratchett et al. (2011). Similarly, herbivores were subdivided into grazer/detritivores, scaper/excavators and browsers, following Edwards et al. (2014). Each species was assigned to a sub-trophic group based on their feeding habits on the fishbase (https://www.fishbase.org/). Finally, we calculated the relative abundance of each trophic group to reveal the trophic structure of each reef site.
Shoaling size
We counted the shoaling size of each fish species of each site, which was defined as the number of individuals of the same fish species occurring in the same frame in the video. Wilcoxon rank sum tests were conducted to investigate the differences between the median fish group size across the four reefs. Additionally, we investigated the group size of each reef community’s top five abundant species. All analyses were performed by R Statistical Software v4.1.0 (R Core Team, 2021).
Results
Species diversity
Our results showed that the sample coverage of each reef community was > 99%, indicating a strong and nearly equal sample effort for each site, despite their different number of transects and reef areas (Figure 2), indicating that our data was sufficiently representative of the local reef fish biodiversity. Our analysis of diversity indexes from different perspectives revealed that Meiji Reef (225 species) harbors the richest biodiversity, followed by Yongshu Reef (182 species) and Qilian Island (114 species), and Yongle Islands (72 species) had the poorest. The rank of the structure complexity of the four reef sites was: Meiji Reef > Yongshu Reef > Qilian Island > Yongle Island, which was consistent with their species diversity rank, suggesting the relevance between species diversity and reef structure complexity. The community clustering results showed that Meiji Reef and Yongshu Reef, as well as Qilian Island and Yongle Islands, formed a sister clade respectively, which was consistent with their geographical distance (Figure 1C). These results provide valuable insight into the distribution of reef fish biodiversity in the studied area.
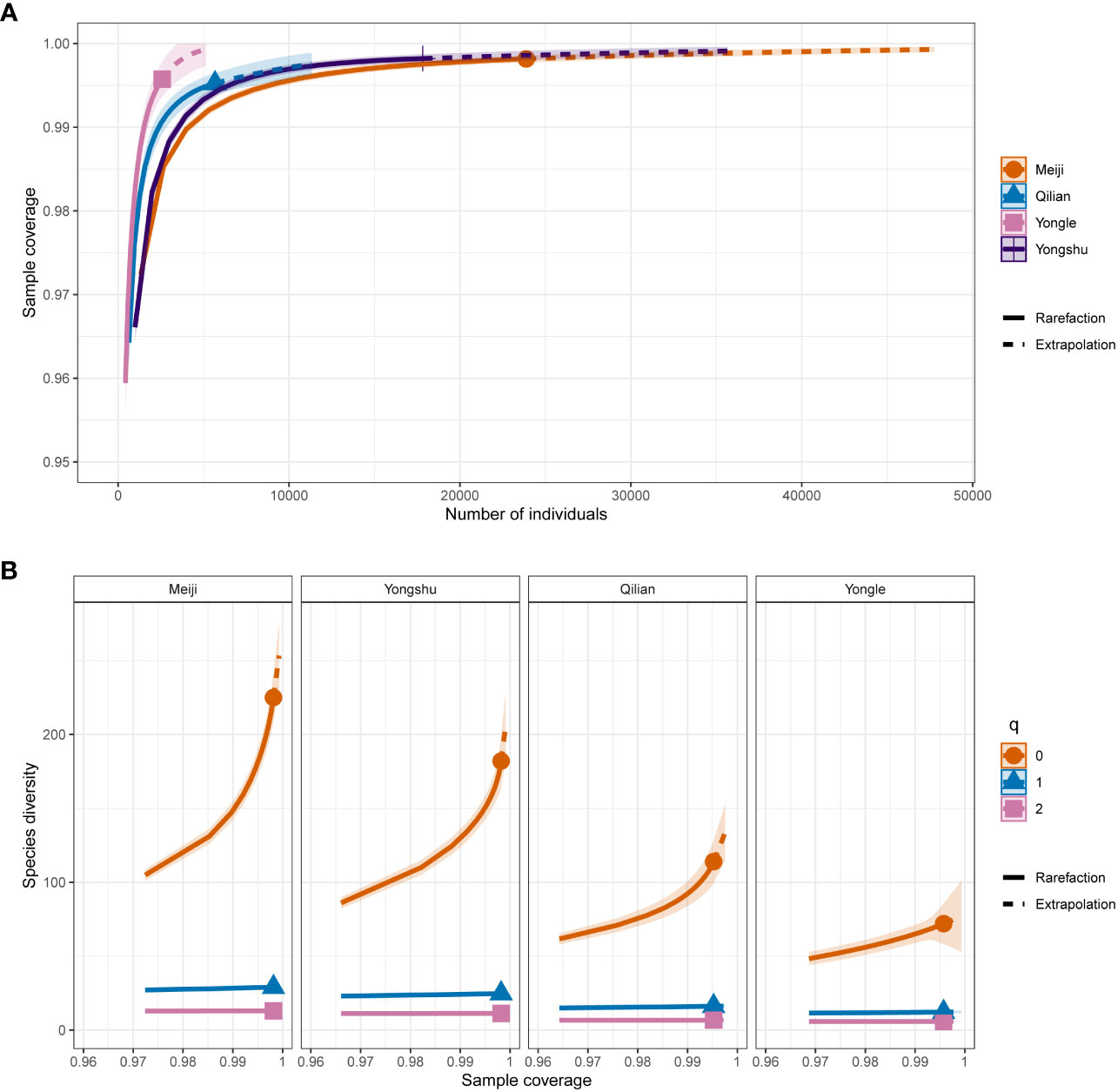
Figure 2 Sample coverage and species diversity estimation of the four reefs. (A) Sample coverage estimation of the four reefs. The solid shape marks the observed sample coverage. (B) Species diversity estimation of the different q orders. When q = 0: Species richness; q = 1: Shannon diversity; q = 2: Simpson diversity. The solid shape marks the observed diversity values.
As typical reef fishes, Labridae, Pomacentridae, and Chaetodontidae species were the most dominant fish in all four reef communities (Figure 3). Specifically, in Nansha study areas, Meiji Reef harbored more coral-reef-associated fish species than Yongshu Reef which might be due to the ongoing outbreak of CoTS in Yongshu Reef. Meiji Reef had more abundant Pomacentridae (43 species vs 32 species), Chaetodontidae (20 species vs 12 species), Scaridae (17 species vs 11 species), and Serranidae (17 species vs 8 species) species than Yongshu Reef, while Yongshu Reef had more Labridae species than Meiji Reef (40 species vs 35 species). Regarding the dominant species of communities, Chromis margaritifer and Thalassoma amblycephalum were among the most abundant species in Meiji Reef, while Odonus niger was dominant in Yongshu Reef, accounting for 19.7% of the fish population. This pattern also observed in the Xisha coral reef ecosystem. Notably, the diversity of reef fish in Yongle Reef, where reef-building corals were severely degraded, was significantly lower than that in Qilian Island. In addition, Chromis margaritifer was the second richest species (18.4%) in Qilian Island, while in Yongle Islands Chlorurus spilurus took its place in the community (20.0%) (Figure 3). Our results indicated a discrepancy between the community composition of different reefs at the same latitude, which might reflect the influence of local environmental pressure caused by reef plate degradation.
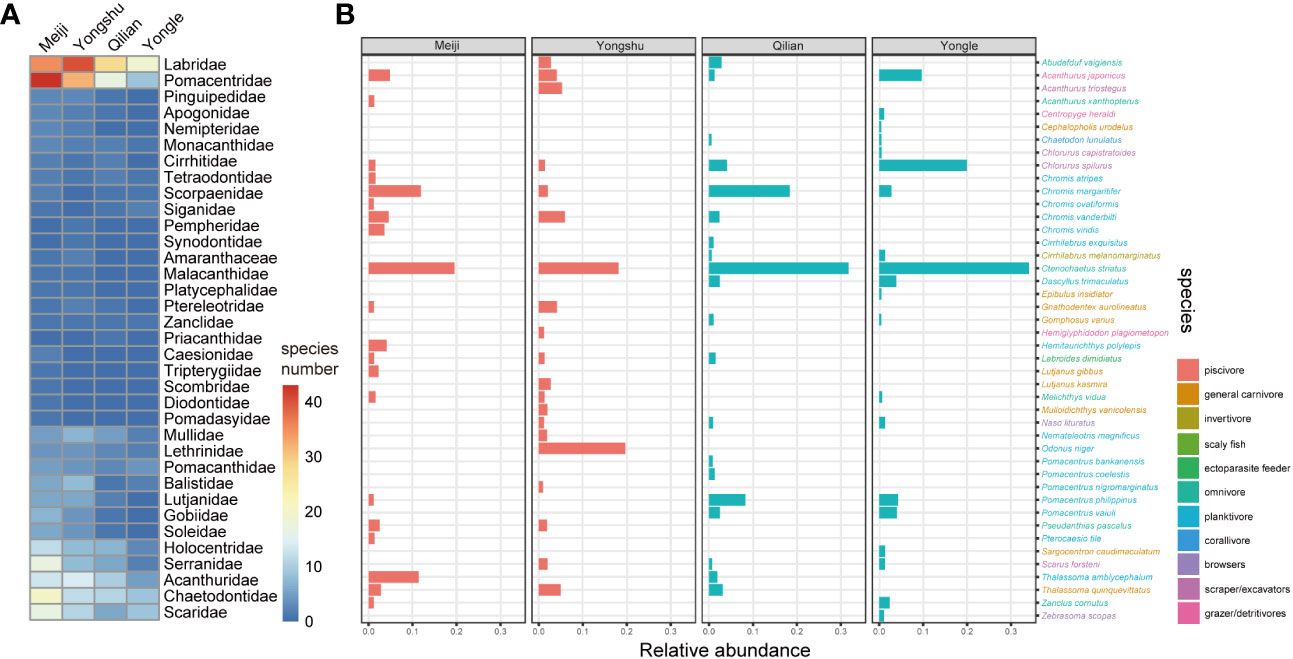
Figure 3 Species composition across four reefs. (A) The species number of each family of the four reefs. (B) The top 20 most abundant species of each reef. Color of species name indicates their trophic group.
Trophic composition
Analysis of the feeding function of coral reef fishes suggested that the species trophic composition of coral reef fishes was similar in different coral reef ecosystems. The trophic groups with a relatively high number of species were general carnivores, omnivores, invertivores, planktivores, scaper/excavators and grazer/detritivores for all reef sites (Figure 4A). In addition, by comparing the composition of reef fish trophic groups at the same latitude, we found that different degrees of coral reef damage had an impact on the species’ trophic composition of coral reef fish community. The most typical one was the Yongle Islands, whose coral reefs had been severely degraded and transformed into an ecosystem dominated by soft corals and algae (Figure 1B right). This transformation disrupted the stability of the existing coral reef fish composition, resulting in a larger proportion of scraper/excavators (22.68%) and a smaller proportion of planktivores species (9.72%) than the neighboring Qilian Island (5.17% accounted for scraper/excavators and 17.54% accounted for planktivores) (Figure 4A). Moreover, the similar trophic structure of Meiji Reef and Yongshu Reef suggested that the relative species number of trophic groups could keep relatively stable at mild degradation or in the process of being degraded (Figure 4A).
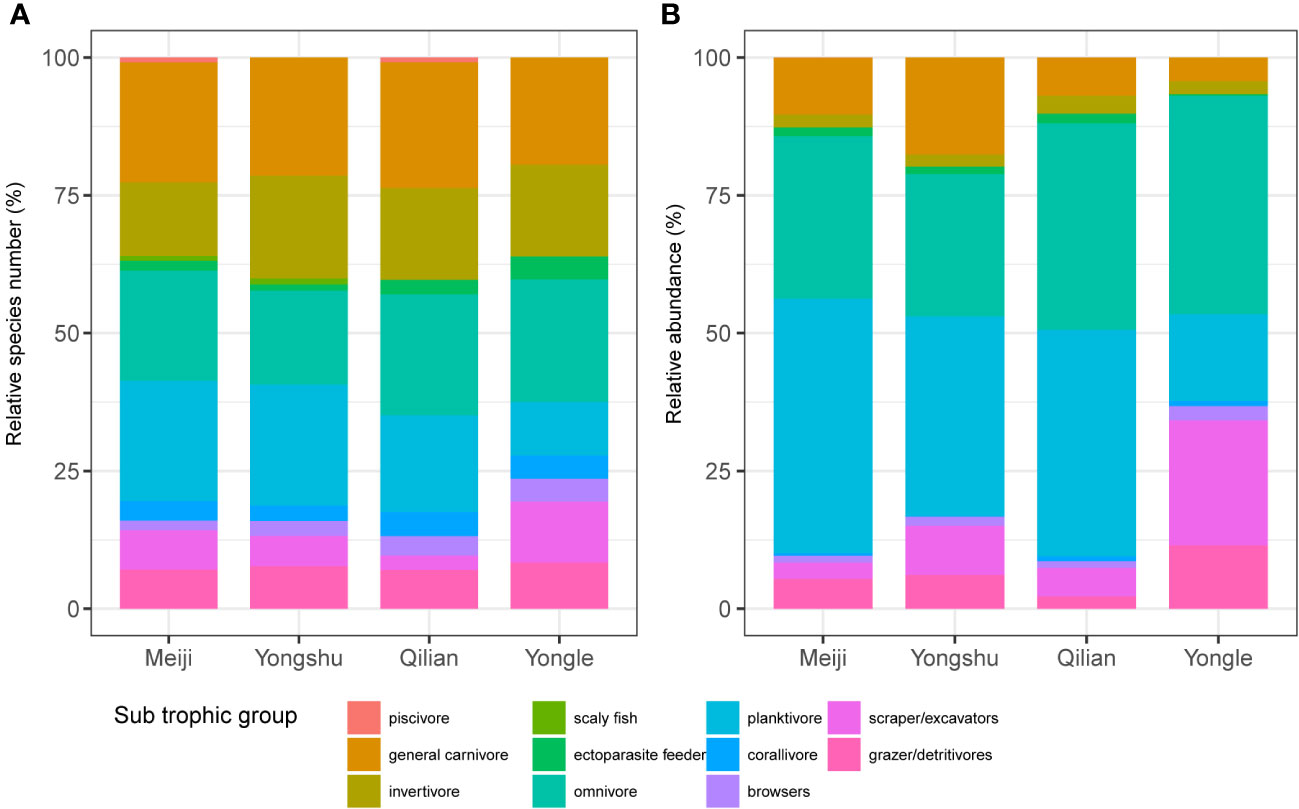
Figure 4 The composition of trophic groups of the four reefs. (A) Species composition. (B) Abundance composition.
As for the abundance of trophic groups, Meiji Reef and Yongshu Reef harbored more abundant carnivorous fish than Qilian and Yongle Islands (Figure 4B). Furthermore, the difference between Meiji Reef and Yongshu Reef was mainly in general carnivores, planktivores, and herbivores fauna. Meiji Reef had more abundant planktivores (Meiji vs Yongshu: 46.2% vs 36.2%), while in Yongshu Reef general carnivores and scraper/excavators were more common (Meiji vs Yongshu: 10.3% vs 17.6%; 3% vs 8.9%) (Figure 4B). The higher percentage of general carnivores in Yongshu Reef contrast to Meiji Reef was mainly contributed by the increase of four species (Gnathodentex aurolineatus, Lutjanus Kasmira, Mulloidichthys vanicolensis and Thalassoma quinquevittatus) (Figure 3B). Besides, the composition of herbivores in Yongle Islands differed from that in Qilian Island, reflected by the dominance of scraper/excavators (Yongle vs Qilian: 22.7% vs 5.2%) and grazer/detritivores (Yongle vs Qilian: 11.5% vs 2.2%), suggesting the transition to the soft corals- and algae-dominant ecosystem in Yongle Islands (Figure 4B).
Shoaling size
We compared the shoaling size of the same fish species between coral reefs using wilcoxon rank test. We totally found 16 species with significant difference among coral reefs, among which shoaling size of all planktivores and one omnivores species in Meiji Reef was higher than that in Yongshu Reef (Table 3). However, two general carnivores, one scraper/excavators and two omnivore species had larger shoaling size in Yongshu Reef than Meiji Reef. This result indicated that the shoaling behavior of coral reef fish might change due to environmental factors such as the outbreak of CoTS. As for Qilian and Yongle Island, three herbivores and two omnivores and one planktivores species gather a larger shoaling size in Yongle Island, except one planktivores, Chromis margaritifer, had larger group in Qilian Island. Therefore, most degraded Yongle Island fed larger herbivores and omnivores group, which might be due to the shift to algae-dominant ecosystem. In conclusion, degradation reduced the shoaling size of planktivores, but supported larger herbivores and omnivores group.
We further investigated the difference in the group size of the top five most abundant species of each site and totally focus on 11 species (Figure 5), which could be affected by external factors such as food and overfishing. Results showed that seven species in Meiji Reef had higher group-size ceilings than Yongshu Reef, and the diet of these species was diverse, including carnivore, herbivore, planktivore, and omnivore (Figure 5), indicating Meiji Reef could support larger fish group than Yongshu Reef in general. The median group size of Chromis margaritifier (Wilcoxon rank sum test, p < 0.001) and Ctenochaetus striatus (Wilcoxon rank sum test, p < 0.001) in Meiji Reef was also higher than Yongshu Reef. However, the upper bound of the group size of Acanthurus triostegus and Pomacentrus vaiuli in Yongshu Reef were higher than in Meiji Reef. It was also interesting to note that large aggregations of A. triostegus and Odonus niger have been found on Yongshu Reef, whereas no aggregations have been found on other reefs, especially on Meiji Reef, which is very close to the Yongshu reef (Figure 5). In addition, the group-size ceilings and median values of three species in Yongle Islands, Acanthurus japonicus (Wilcoxon rank sum test, p < 0.001), Chlorurus spilurus (Wilcoxon rank sum test, p < 0.001), and Ctenochaetus striatus (Wilcoxon rank sum test, p < 0.05), were higher than that in Qilian Island, suggesting that Yongle Islands held a larger herbivore group.
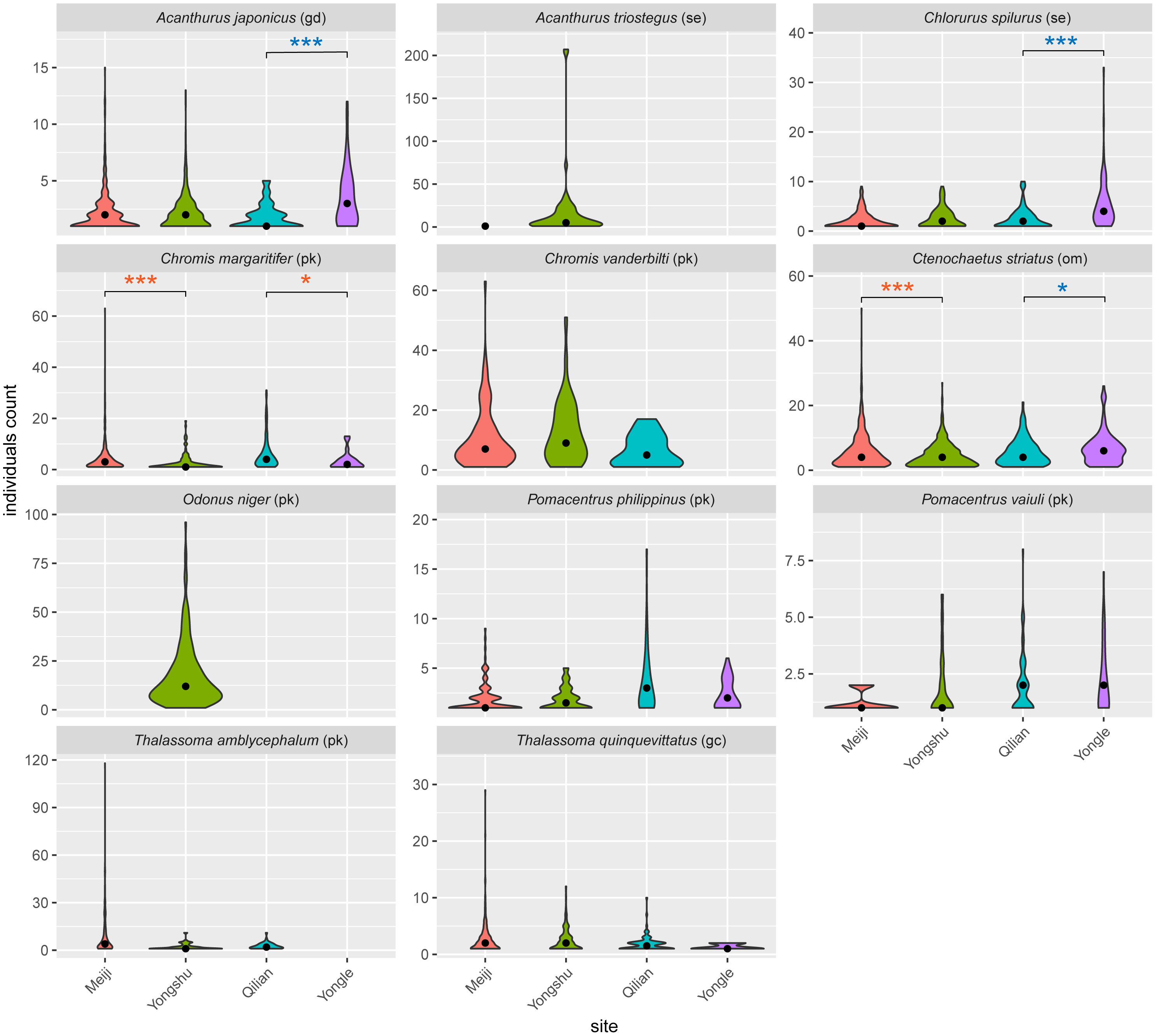
Figure 5 The group size of the top five most abundant species of each reef. gd, grazer/detritivore; se, scraper/excavator; pk, planktivore; om, omnivore; gc, general carnivore. Black points indicated the median group size values. Significant results of Wilcoxon tests were marked, “***”: p < 0.001; “*”: p < 0.05. The orange color of “*” meant the median value of the left side was higher than the right one in the pairs of comparison, while the blue color was the opposite.
Discussion
Differences of reef fish species diversity
Nansha Islands situated closer to the Coral Triangle and equator than Xisha Islands. Therefore, the superior environment and the dispersion from the Coral Triangle resulted in the richer biodiversity of Nansha Islands, manifested as the higher fish diversity of Meiji Reef and Yongshu Reef in our results. Anthropogenic interference might also play an important role, given that Xisha Islands had a larger human population (Ruppert et al., 2018). However, our results also revealed some discrepancies in biodiversity within the Nansha Islands (Meiji Reef and Yongshu Reef), and Xisha Islands (Qilian Islands and Yongle Islands), which might be due to the varying degrees of reef degradation. Various unfavorable factors contributed to the lower diversity of Yongshu Reef compared with Meiji Reef. For example, the overall complexity of Yongshu Reef is lower than that of Meiji Reef, and most of it is relatively shallow reef flat, which is easily affected by marine heat waves. And its structural complexity was also lower than Meiji Reef. Furthermore, the coral reef at Yongle Islands had undergone severe damage and transitioned to a soft coral community, rendering it the poorest in terms of biodiversity.
Trophic structure as an indicator of coral reef status
The trophic structure of reefs could also reflect the status of ecosystem. At the top of food chain, carnivores depend on the prey abundance, making them an important indicator of reef health (Mumby et al., 2012). Herbivores, on the other hand, mediate the competition between corals and algae, and can indicate whether a reef is coral- or algae-dominant (Poore et al., 2012; Vergés et al., 2014). Typically, higher herbivory usually suggests an algae-dominant reef. Among the four sites in this study, Yongle Islands had the highest herbivory, followed by Qilian Islands, Yongshu Reef, and Meiji Reef. Carnivory, however, was the opposite, except for Yongshu Reef. This indicates a shift towards an algae-dominant reef in Yongle Islands, which is consistent with the degree of degradation observed. The unusually high relative abundance of general carnivores in Yongshu Reef might indicate an imbalance of the trophic cascade causing by degradation due to the outbreak of CoTS. In other words, when low-trophic fish are heavily preyed upon without individual replenishing, there is a brief spike in the relative abundance of general carnivores. However, the total fish stocks would eventually decrease because of the exhaustion of prey and subsequent collapse of the carnivore population resulting from the scarcity of prey. Our survey showed that Yongshu Reef (~ 18000 fish individuals) didn’t has greater fish stocks than Meiji Reef (~ 24000 fish individuals) when at the same sample coverage (= ~ 0.9982) (Figure 2).
Reef fish could also pose an impact on the coral reef ecosystems in a top-down manner. Herbivores were known to play a crucial role in preventing the settlement of macroalgae on coral reef surfaces. This, in turn, helps to maintain a suitable microhabitat for coral recruitment and growth, ultimately promoting the dominance of hard corals (Green and Bellwood, 2009). Therefore, herbivores could resist the phase shift from hard-coral-dominant to macroalgae-dominant ecosystems after coral mortality in the degraded coral reefs(Cheal et al., 2013). In our results, the high proportion of herbivores observed in Yongle Islands could be considered as an indicator of reef resilience (Heenan and Williams, 2013). However, subsequent monitoring was needed to assess whether herbivores could effectively reverse the coral-macroalgal phase shifts in Yongle Islands.
Factors causing the outbreak of CoTS
The outbreaks of the CoTS had cause great damage to coral reefs, which could be attributed to the abundant zooplankton supply for larvae, free from predator, and suitable water temperature and salinity (Brodie, 1992; Kroon et al., 2021). The outbreak of the CoTS was observed in Yongshu Reef, where the planktivores accounted for a lower percentage of fish assemblage than Meiji Reef and Qilian Island. A reasonable explanation is that the rapid growth of phytoplankton, due to the absence of planktivores, provided sufficient food for the larvae of CoTS, leading to the local outbreaks of CoTS (Mos et al., 2023). Rich coralline algae and their associated microbial communities might also induce the larval settlement of CoTS (Doll et al., 2023). Future studies were required to investigate the driving factors and consequences of the outbreak of CoTS in Yongshu Reef.
Degradation affecting the fish shoaling size
Living in groups provides many benefits but also incurs costs. The group size can be restricted by the availability of resources, such as food, shelter, and living space (Krause and Ruxton, 2008; Griesser et al., 2011). Our result demonstrated that the degradation of coral reefs could affected the shoaling behavior of reef fish, regulating the shoaling size. Our results showed that Meiji reef harbored the largest group size, while Yongle Islands had the smallest, consistent with the rank of the degree of damage of reef. However, some herbivores might benefit from the shift to algae-dominant ecosystems and formed a larger group size in Yongshu and Yongle Reef. In addition, comparing to degraded habitats, undamaged reefs such as Meiji Reef and Qilian Reef were a suitable habitat for planktivores, which could gather larger groups.
Conclusion
Our study suggested that the degradation of coral reefs had deeply affected the biodiversity, community trophic structure, and the group size of dominant species, resulting in the dominance of herbivores and the decline of planktivores, and the reduction of the mean and upper bound of the group size of fish across different trophic groups. Our findings promoted the understanding of the consequences of reef damage and can enhance the conservation of coral reefs in the SCS. For example, Meiji Reef still holds a healthy coral reef ecosystem, and requires continuous monitoring. Urgent actions should be taken to recover the ecosystem functioning of Yongle Islands, such as the curb of overfishing and pollution and establishment of protection areas.
Data availability statement
The datasets and code of this study can be found in online repositories (https://github.com/Huangmp1996/fish-coummnity-in-the-SCS).
Ethics statement
The animal study was approved by Committee for Animal Experiments of the Southern Marine Science and Engineering Guangdong Laboratory (Guangzhou). The study was conducted in accordance with the local legislation and institutional requirements.
Author contributions
FW and WZ conceived and managed the project. WZ, SW, MH and ZP conducted the filed survey. MH, QL, KG and YC performed the analysis. All authors contributed to the article and approved the submitted version.
Funding
This work was supported by the Ministry of Science and Technology of China (2021YFF0502800), and the Science and Technology Department of Guangdong Province (2021QN02H103), and the Guangdong Forestry Administration (SLYJ2023B4004), and the PI Project of Southern Marine Science and Engineering Guangdong Laboratory (Guangzhou) (GML2020GD0804, GML2022GD0804).
Acknowledgments
We are grateful to Weijian Guo, Xin Huang, Yisi Hu, and the divers for their kind assistance during the field work in the 2022 South China Sea voyage.
Conflict of interest
The authors declare that the research was conducted in the absence of any commercial or financial relationships that could be construed as a potential conflict of interest.
Publisher’s note
All claims expressed in this article are solely those of the authors and do not necessarily represent those of their affiliated organizations, or those of the publisher, the editors and the reviewers. Any product that may be evaluated in this article, or claim that may be made by its manufacturer, is not guaranteed or endorsed by the publisher.
Supplementary material
The Supplementary Material for this article can be found online at: https://www.frontiersin.org/articles/10.3389/fcosc.2023.1229513/full#supplementary-material
References
Beukhof E., Dencker T. S., Pecuchet L., Lindegren M. (2019). Spatio-temporal variation in marine fish traits reveals community-wide responses to environmental change. Mar. Ecol. Prog. Ser. 610, 205–222. doi: 10.3354/meps12826
Borstein S. R., Fordyce J. A., O'Meara B. C., Wainwright P. C., McGee M. D. (2019). Reef fish functional traits evolve fastest at trophic extremes. Nat. Ecol. Evol. 3, 191–199. doi: 10.1038/s41559-018-0725-x
Brodie J. E. (1992). Enhancement of larval and juvenile survival and recruitment in Acanthatser planci from the effects of terrestrial runoff: a review. Mar. Freshw. Res. 43, 539. doi: 10.1071/MF9920539
Cheal A. J., Emslie M., Aaron M. M., Miller I., Sweatman H. (2013). Spatial variation in the functional characteristics of herbivorous fish communities and the resilience of coral reefs. Ecol. Appl. 23, 174–188. doi: 10.1890/11-2253.1
Cheal A. J., MacNeil M. A., Cripps E., Emslie M. J., Jonker M., Schaffelke B., et al. (2010). Coral–macroalgal phase shifts or reef resilience: links with diversity and functional roles of herbivorous fishes on the Great Barrier Reef. Coral. Reefs. 29, 1005–1015. doi: 10.1007/s00338-010-0661-y
Dai X., Li Y., Cai Y., Gong Y., Zhang J., Chen Z. (2022). Variations in Fish Community Structure at the Lagoon of Yongshu Reef, South China Sea between 1999 and 2016–2019. Diversity 14, 763. doi: 10.3390/d14090763
De'ath G., Fabricius K. E., Sweatman H., Puotinen M. (2012). The 27-year decline of coral cover on the Great Barrier Reef and its causes. Proc. Natl. Acad. Sci. U. S. A. 109, 17995–17999. doi: 10.1073/pnas.1208909109
Doll P. C., Uthicke S., Caballes C. F., Diaz-Pulido G., Abdul Wahab M. A., Lang B. J., et al. (2023). Settlement cue selectivity by larvae of the destructive crown-of-thorns starfish. Biol. Lett. 19, 20220399. doi: 10.1098/rsbl.2022.0399
Edwards C. B., Friedlander A. M., Green A. G., Hardt M. J., Sala E., Sweatman H. P., et al. (2014). Global assessment of the status of coral reef herbivorous fishes: evidence for fishing effects. Proc. Biol. Sci. 281, 20131835. doi: 10.1098/rspb.2013.1835
Fan H., Huang M., Chen Y., Zhou W., Hu Y., Wei F. (2023). Conservation priorities for global marine biodiversity across multiple dimensions. Natl. Sci. Rev. 10 (6), nwac241. doi: 10.1093/nsr/nwac241
Feary D. A., Almany G. R., Jones G. P., McCormick M. I. (2007). Coral degradation and the structure of tropical reef fish communities. Mar. Ecol. Prog. Ser. 333, 243–248. doi: 10.3354/meps333243
Floeter S. R., Bender M. G., Siqueira A. C., Cowman P. F. (2018). Phylogenetic perspectives on reef fish functional traits. Biol. Rev. 93, 131–151. doi: 10.1111/brv.12336
Fontoura L., Zawada K. J. A., D'agata S., Álvarez-Noriega M., Baird A. H., Boutros N., et al. (2020). Climate-driven shift in coral morphological structure predicts decline of juvenile reef fishes. Glob. Change Biol. 26, 557–567. doi: 10.1111/gcb.14911
Graham N. A. J., Nash K. L. (2013). The importance of structural complexity in coral reef ecosystems. Coral. Reefs. 32, 315–326. doi: 10.1007/s00338-012-0984-y
Green A. L., Bellwood D. R. (2009). Monitoring functional groups of herbivorous reef fishes as indicators of coral reef resilience: a practical guide for coral reef managers in the Asia Pacific region (Gland, Switzerland: IUCN).
Griesser M., Ma Q., Webber S., Bowgen K., Sumpter D. J. T. (2011). Understanding animal group-size distributions. PloS One 6, e23438. doi: 10.1371/journal.pone.0023438
Guerra A. S., McCauley D. J., Lecchini D., Caselle J. E. (2022). Shoaling behavior of coral reef fishes varies between two islands with different predator abundance. Mar. Ecol. Prog. Ser. 690, 133–145. doi: 10.3354/meps14050
Heenan A., Williams I. D. (2013). Monitoring herbivorous fishes as indicators of coral reef resilience in American Samoa. PloS One 8, e79604. doi: 10.1371/journal.pone.0079604
Hsieh T. C., Ma K. H., Chao A. (2016). iNEXT: an R package for rarefaction and extrapolation of species diversity (Hill numbers). Methods Ecol. Evol. 7, 1451–1456. doi: 10.1111/2041-210X.12613
Hughes T. P., Anderson K. D., Connolly S. R., Heron S. F., Kerry J. T., Lough J. M., et al. (2018). Spatial and temporal patterns of mass bleaching of corals in the Anthropocene. Science 359, 80–83. doi: 10.1126/science.aan8048
Kroon F. J., Barneche D. R., Emslie M. J. (2021). Fish predators control outbreaks of Crown-of-Thorns Starfish. Nat. Commun. 12, 6986. doi: 10.1038/s41467-021-26786-8
Mos B., Mesic N., Dworjanyn S. A. (2023). Variable food alters responses of larval crown-of-thorns starfish to ocean warming but not acidification. Commun. Biol. 6, 639. doi: 10.1038/s42003-023-05028-1
Mumby P. J., Steneck R. S., Edwards A. J., Ferrari R., Coleman R., Harborne A. R., et al. (2012). Fishing down a Caribbean food web relaxes trophic cascades. Mar. Ecol. Prog. Ser. 445, 13–24. doi: 10.3354/MEPS09450
Pandolfi J. M., Bradbury R. H., Sala E., Hughes T. P., Bjorndal K. A., Cooke R. G., et al. (2003). Global trajectories of the long-term decline of coral reef ecosystems. Science 301, 955–958. doi: 10.1126/science.1085706
Pitcher T. J. (1986). “Functions of shoaling behaviour in teleosts,” in The behaviour of teleost fishes. Ed. Pitcher T. J. (Boston, MA: Springer US), 294–337.
Poore A. G. B., Campbell A. H., Coleman R. A., Edgar G. J., Jormalainen V., Reynolds P. L., et al. (2012). Global patterns in the impact of marine herbivores on benthic primary producers. Ecol. Lett. 15, 912–922. doi: 10.1111/j.1461-0248.2012.01804.x
Pratchett M. S., Hoey A. S., Wilson S. K., Messmer V., Graham N. A. (2011). Changes in biodiversity and functioning of reef fish assemblages following coral bleaching and coral loss. Diversity 3, 424–452. doi: 10.3390/d3030424
R Core Team (2021). R: A language and environment for statistical computing (Austria: Vienna). Available at: https://www.R-project.org/.
Robinson J. P. W., Williams I. D., Edwards A. M., McPherson J., Yeager L., Vigliola L., et al. (2017). Fishing degrades size structure of coral reef fish communities. Glob. Change Biol. 23, 1009–1022. doi: 10.1111/gcb.13482
Robinson J. P. W., Wilson S. K., Jennings S., Graham N. A. J. (2019). Thermal stress induces persistently altered coral reef fish assemblages. Glob. Change Biol. 25, 2739–2750. doi: 10.1111/gcb.14704
Ruppert J. L. W., Vigliola L., Kulbicki M., Labrosse P., Fortin M. J., Meekan M. G. (2018). Human activities as a driver of spatial variation in the trophic structure of fish communities on Pacific coral reefs. Glob. Change Biol. 24, e67–e79. doi: 10.1111/gcb.13882
Smale D. A., Wernberg T., Oliver E. C. J., Thomsen M., Harvey B. P., Straub S. C., et al. (2019). Marine heatwaves threaten global biodiversity and the provision of ecosystem services. Nat. Clim. Change 9, 306–312. doi: 10.1038/s41558-019-0412-1
Stuart-Smith R. D., Bates A. E., Lefcheck J. S., Duffy J. E., Baker S. C., Thomson R. J., et al. (2013). Integrating abundance and functional traits reveals new global hotspots of fish diversity. Nature 501, 539–542. doi: 10.1038/nature12529
Vergés A., Steinberg P. D., Hay M. E., Poore A. G. B., Campbell A. H., Ballesteros E., et al. (2014). The tropicalization of temperate marine ecosystems: climate-mediated changes in herbivory and community phase shifts. Proc. Biol. Sci. 281, 20140846. doi: 10.1098/rspb.2014.0846
Wu Z., Li Y., Liang J., Zhao J., Chen S. (2019). Analysis on the outbreak period and cause of Acanthaster planci in Xisha Islands in recent 15 years. Chin. Sci. Bull. 64, 1–7. doi: 10.1360/tb-2019-0152
Xiao J., Wang W., Wang X., Tian P., Niu W. (2022). Recent deterioration of coral reefs in the South China Sea due to multiple disturbances. PeerJ 10, e13634. doi: 10.7717/peerj.13634
Yan H., Shi Q., Yu K., Tao S., Yang H., Liu Y., et al. (2019). Regional coral growth responses to seawater warming in the South China Sea. Sci. Total. Environ. 670, 595–605. doi: 10.1016/j.scitotenv.2019.03.135
Zawada K. J. A., Madin J. S., Baird A. H., Bridge T. C. L., Dornelas M. (2019). Morphological traits can track coral reef responses to the Anthropocene. Funct. Ecol. 33, 962–975. doi: 10.1111/1365-2435.13358
Keywords: marine conservation, reef fish, species recruitment, South China Sea, herbivores
Citation: Huang M, Wei S, Li Q, Gao K, Peng Z, Chen Y, Zhou W and Wei F (2023) Degradation of coral reefs altered the community trophic structure and reduced the shoaling size of fish. Front. Conserv. Sci. 4:1229513. doi: 10.3389/fcosc.2023.1229513
Received: 26 May 2023; Accepted: 25 August 2023;
Published: 08 September 2023.
Edited by:
Zuofu Xiang, Central South University Forestry and Technology, ChinaReviewed by:
Dandan Duan, Hainan Normal University, ChinaJun Zhang, Chinese Academy of Fishery Sciences (CAFS), China
Wei Wei, China West Normal University, China
Copyright © 2023 Huang, Wei, Li, Gao, Peng, Chen, Zhou and Wei. This is an open-access article distributed under the terms of the Creative Commons Attribution License (CC BY). The use, distribution or reproduction in other forums is permitted, provided the original author(s) and the copyright owner(s) are credited and that the original publication in this journal is cited, in accordance with accepted academic practice. No use, distribution or reproduction is permitted which does not comply with these terms.
*Correspondence: Wenliang Zhou, emhvdXdsQGdtbGFiLmFjLmNu