- 1Department of Geography, University at Buffalo, Buffalo, NY, United States
- 2Biological Sciences Department, University of Cape Town, Cape Town, South Africa
- 3School for Climate Studies, Stellenbosch University, Stellenbosch, South Africa
Mosaics of closed, fire-sensitive forests and open flammable ecosystems are common across Africa and other parts of the world. The open ecosystems have long been interpreted as products of deforestation, but diverse lines of evidence point to their origins millions of years before humans. There is widespread concern over the survival of fire-sensitive forest in a flammable matrix, leading to diverse measures, including fire suppression, to protect forests. But if both systems are ancient, how did fire-sensitive forests survive the regular fires in the flammable open ecosystems? Here we discuss recent research on this topic, with a focus on Africa, including the stability of these mosaics through time and the factors accounting for this. These include local topography, variation in grass flammability, the presence of fire-tolerant forest margin tree species, and fauna that create firebreaks along the forest edge. We go on to discuss the conditions under which mosaics are less stable, for example during extreme fires, and consider the uncertain future of mosaicked landscapes under climate change. Finally, we suggest a set of guidelines for consideration by conservation managers concerned about fire damage to forest patches.
Introduction
Mosaics of closed forest and open ecosystems (e.g. grasslands, shrublands, savannas) are widespread in the tropics and some temperate regions. Open ecosystems are often flammable, supporting regular fires from which they recover readily. This contrasts with closed forests which seldom burn and, when they do, suffer long-term damage and loss of fire-sensitive species. Forest patches within a flammable matrix have long been viewed as remnants of a once-continuous forest destroyed by felling and fire. However, there is growing support for the view that these forests are alternative stable states to the flammable open ecosystems (Hirota et al., 2011; Staver et al., 2011b). Various lines of evidence, including stable isotope analysis of soil organic matter, support the alternative stable states hypothesis (reviewed in Bond, 2019; Bond, 2021; Pausas and Bond, 2021). While there are clear examples of fire destroying forest, especially where it has been logged for human use, there are also clear examples of mosaics where forests existed for many millennia despite fires regularly burning the open flammable ecosystems.
Given that ancient mosaics of forest and flammable open ecosystems exist, their stability is puzzling and little explored. How do fire-sensitive forest patches persist? The answers could have useful applications in conservation and, indeed, in suggesting guidelines for fire protection of human settlements. In managed conservation areas, protection of forest patches from fire has led to major interventions (Figure 1) including the construction of fire breaks, their regular maintenance, suppression of fire in the flammable ecosystems adjacent to the forest, and even tree planting, often with fast-growing non-native trees, to promote a forest microclimate, suppress flammable fuels and ‘restore’ forest cover. Such activities are informed by fire suppression tactics used to protect property and people from vegetation fires. Consequently, forest patches in nature can be seen as analogues to fire-sensitive human structures. Analyses of how natural forest patches survive in a flammable matrix could prove useful not only for conserving mosaics of alternative closed and open biome states but also in sharing ideas for managing vegetation fires threatening communities in Europe, North America, Australia and elsewhere.
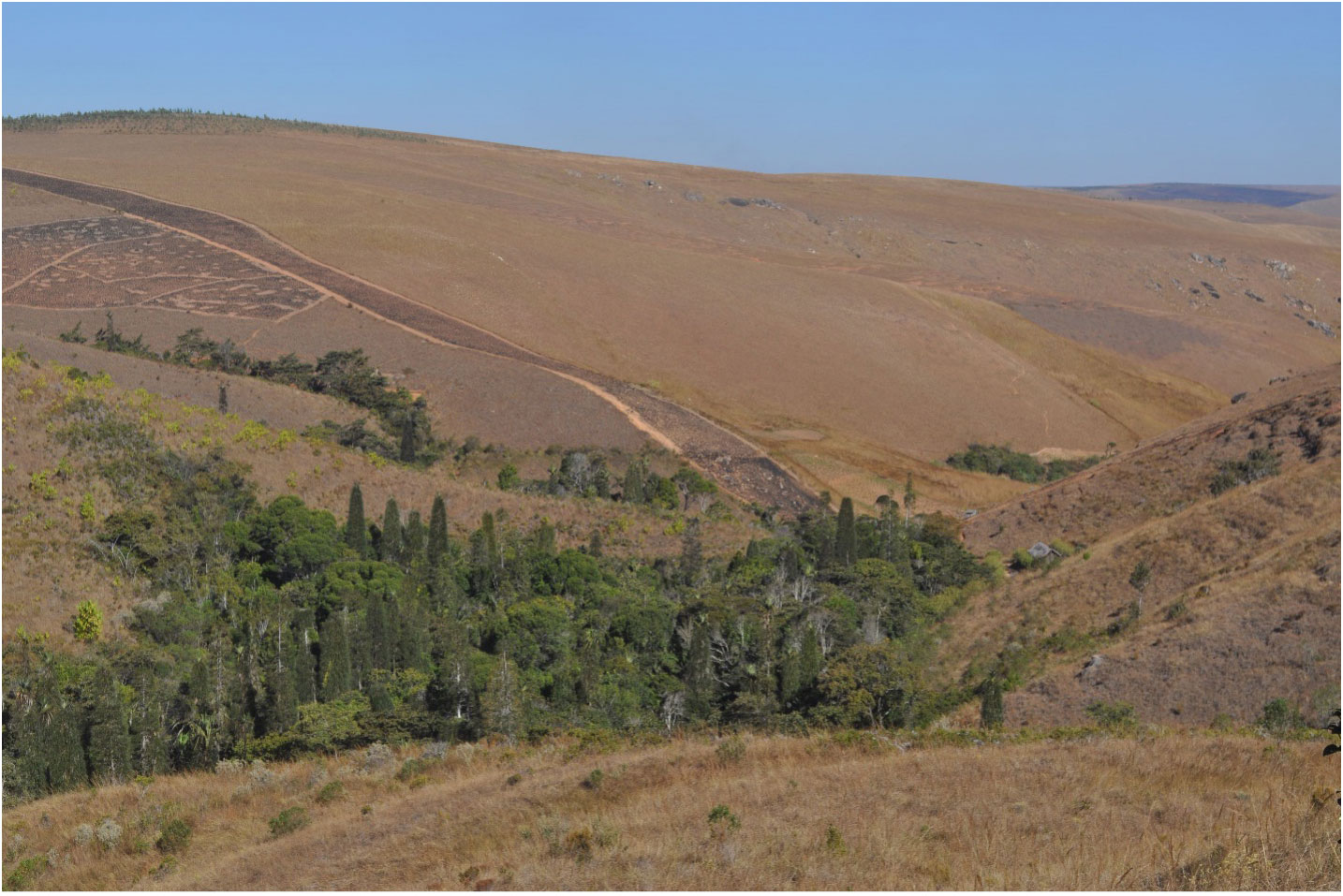
Figure 1 Active forest protection from grassland fires in Madagascar. The parallel strips have been cleared to make a firebreak. Fires are suppressed completely on the forest side of the firebreak. Australian acacias (light green) have been planted on the slope above the forest. (Photo: W. J. Bond).
Here we explore different factors contributing to fire protection of forest patches. We draw on examples familiar to us based on recent research mostly from African grassy ecosystems. Our intention is to stimulate wider study of the question of forest persistence in diverse ecological communities with diverse fire regimes and contrasting landscape features. Such studies would help provide pointers that can be applied for conserving forest/flammable mosaics where both components deserve conservation (Erdős et al., 2018). Though conservation management is typically heavily weighted toward protecting forests, there is growing recognition of the conservation importance of open ecosystems (Bond and Parr, 2010; Silveira et al., 2022). Open ecosystems can seldom be managed passively (e.g. Staver et al., 2017), and decisions have to be made on managing the key consumers that typically maintain the open state, usually fire or large vertebrate herbivores (e.g. elephants). One-sided decisions protecting only one component of the mosaic, usually forest, will lead to the loss of open ecosystem biodiversity and its services (Durigan and Ratter, 2016). Few interventions are as extreme as complete fire suppression in fire-dependent grassy ecosystems. We provide pointers on how to rank forest patches for their risk of potential fire damage and which interventions would be most useful for protecting them from fire. Pointers for recognizing old-growth versus secondary open ecosystems are discussed elsewhere (see e.g. Veldman et al., 2015; Veldman, 2016; Zaloumis and Bond, 2016; Buisson et al., 2019).
Stability of fire-intolerant forest patches within a flammable matrix
How stable are forest/open ecosystem mosaics? Are forests merely the remnants of once-continuous ecosystems destroyed by human activities? Or are they naturally restricted to soils and microclimates favoring forest with open landscapes unsuited for tree growth? Or are they an alternative stable state with each state maintaining itself by feedbacks to disturbance regime and altered growing conditions? All three options may exist in a given region. However, there is growing support for the third option – alternative stable states - as the norm for large parts of the world. As alternative stable states, forest remains forest by shading out sun-loving flammable grasses, while grassy ecosystems remain open by regular fire top-killing any saplings in the herbaceous layer (Staver et al., 2011a; Hoffmann et al., 2012a). The implication of the alternative stable state concept is that forests and adjacent flammable ecosystems can stably coexist, but with the potential for ‘sudden’ switches between states with catastrophic consequences for the transformed state including loss of its distinctive biota.
Open ecosystems have long been considered transient successional states to climax forest. However palaeoecological evidence from pollen, carbon isotopes, and phytoliths has shown that mosaics of open and closed ecosystems can persist for hundreds to thousands of years (Aleman et al., 2018). Remote sensing, including aerial photos, since the mid-20th century, combined with historical archives, provide additional evidence for the persistence of open ecosystems where the climate can support forests [e.g. (Fairhead and Leach, 1996)]. Sun-loving plants and animals are also key indicators of ancient open ecosystems contrasting with secondary systems created by deforestation (Veldman, 2016; Zaloumis and Bond, 2016). Nevertheless, despite diverse lines of evidence, the stability of forest/grassy ecosystem mosaics and their potential for change continue to be contentious and controversial (e.g. Staal et al., 2018; Veenendaal et al., 2018; Joseph and Seymour, 2021; Bond et al., 2022). An important tenet of alternative stable state theory is that each state is stable but also that each state can replace the other. Again, palaeoecological evidence has proved very useful in this respect with evidence for stability and regime shifts on the scale of centuries to millennia. Long term experiments, necessarily maintained for many decades to trace changes in tree populations, have also proved invaluable for assessing both the rate and potential for regime shifts, in particular climate and soil conditions. The accumulation of such studies has shown that single factor explanations are seldom adequate for explaining alternative biome states. Thus, soils supposedly hostile to forest tree growth prove suitable for forests after long fire suppression, and the effects of increasing CO2 vary depending on browsing pressure, fire, and drought (Ripley et al., 2022).
Changing atmospheric CO2 is often overlooked and underappreciated as a major driver of global change, especially in open ecosystems. Both models (e.g. Higgins and Scheiter, 2012) and experimental studies (e.g. Kgope et al., 2009) have shown that tree growth rates vary with changing atmospheric CO2 (and tree species). Low CO2 during glacial periods would have greatly reduced woody plant growth and facilitated C4 grassland expansion (Cerling et al., 1997). Low woody plant growth rates prolong sapling exposure to fire, browsing and drought. The interactions would tend to reduce tree populations by reducing seedling recruitment. Increasing atmospheric CO2 over the last few decades would have promoted tree growth at the expense of grasses, increasing recruitment rates and speeding up recovery of burnt forest edges – threatening grassy ecosystems. The interaction between climate, CO2, and fire has been explored at global scales using physiologically based dynamic vegetation models coupled with fire simulations (Bond et al., 2003; Higgins and Scheiter, 2012; Sato et al., 2021). Sato et al. (2021) simulated changes in the size and distribution of Amazon forests from the last glacial maximum (LGM) to pre-industrial times using climate change only, CO2 change only and both physical drivers with or without fire. Adding fire to the LGM simulation had the greatest effect, with the Amazon forest splitting in two, separated by a corridor of savanna. Sato et al. (2021) cite new paleoecological evidence supporting these simulation results.
Topographical influences
Mosaics of closed forest and open ecosystems are common in areas with complex topography. If the configuration of these mosaics was edaphically driven, one could expect a stable mosaic with vegetation types congregating on their preferred substrate, and unable to establish in other areas (Bowman, 2000). In some instances, however, the vegetation types in these mosaics show little or no association with specific substrates, rather they are dynamic over time (Beckett, 2018). In analyses of correlates of savannas versus forests in the southern continents, the role of topographic complexity was not statistically significant (Archibald et al., 2009; Lehmann et al., 2011) contrasting with smaller scales of investigation where topography is a major predictor of forest occurrence (Beckett and Bond, 2019). The effect of topography on fire behavior is thought to play a large role in facilitating the growth and persistence of closed forest ecosystems in fire refugia (Geldenhuys, 1994; Clarke, 2002).
In southern Africa, landscape scale patterns of forest distribution were long considered to be anthropogenic in origin (Philiips, 1931; Acocks, 1953; Philips, 1963; Feeley, 1980; Granger, 1984) or related to edaphic limitation (Rutherford and Westfall, 1994; Mills et al., 2013). However, Geldenhuys (1994) suggested the distribution of forests in fynbos-forest mosaics in the Southern Cape region of South Africa was determined by their position relative to the strong winds that drive extreme fires. Refugia within this ‘firescape’ occur where topography hampers fire spread, creating areas where the fire return interval is longer, the probability of burning is lower (Mermoz et al., 2005; Penman et al., 2007) and fire intensity and severity are lower (Kushla & Ripple, 1997; Alexander et al., 2006; Holden et al., 2009; Bradstock et al., 2010). The safety of a fire refuge can vary depending on wind direction (Figure 2). Fire suppression and management strategies can potentially alter the timing of fires in these systems from later in the dry season, as would have happened before human interference, to earlier, when the fires are easier to control (Archibald et al., 2017). This would have altered the direction and intensity of burns in mosaics, especially if wind direction changes seasonally, likely leading to fires approaching forest edges that had not experienced fire before. By simulating fire behavior under the prevailing wind direction during the fire season one can identify where these fire refugia are most likely to occur (Beckett, 2018) and the most appropriate conditions under which to set fires.
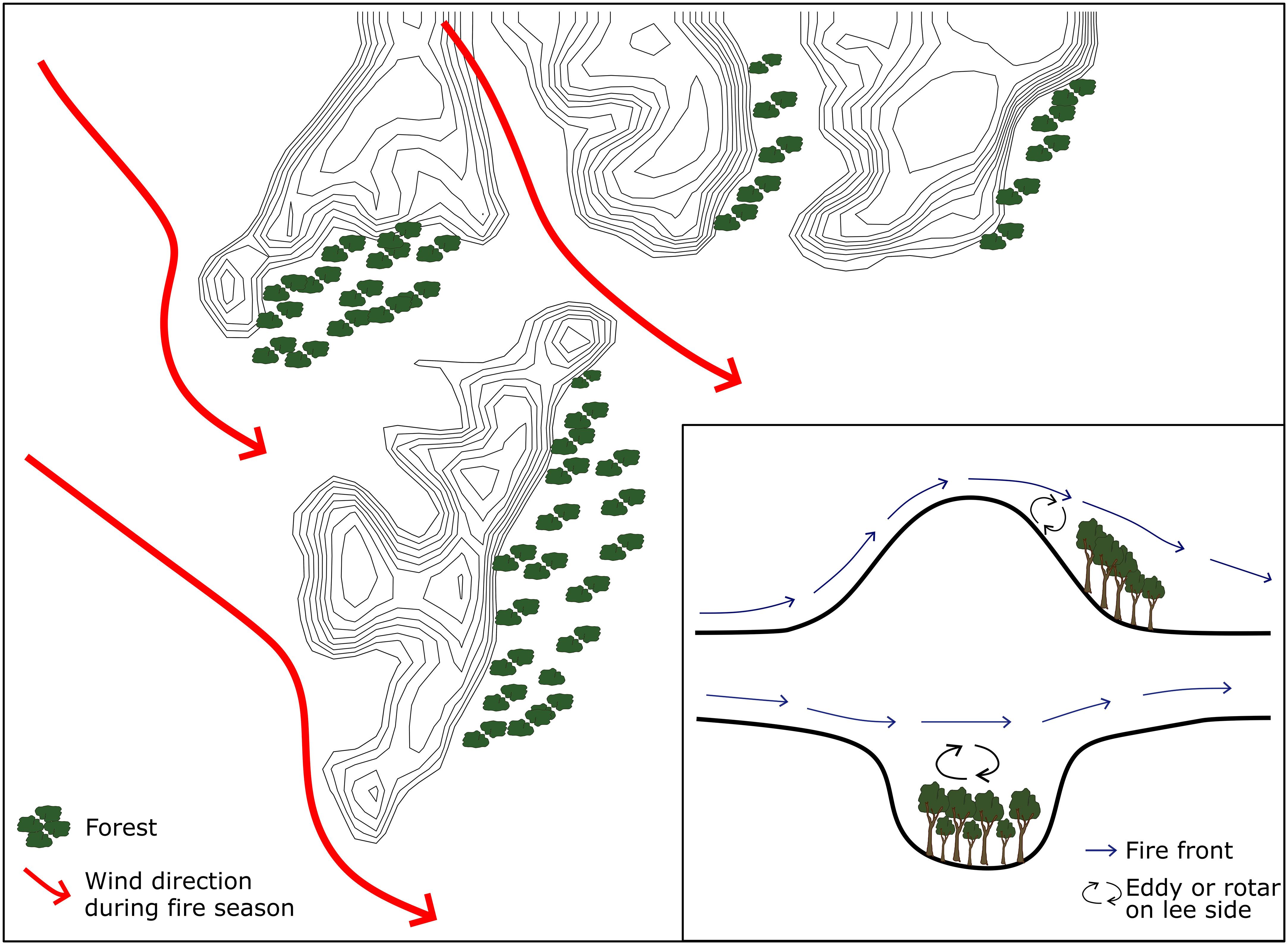
Figure 2 Hypothetical wind flow patterns, fire spread direction, and the location of fire refugia forests in relation to topography and dominant wind direction during the fire season. Inset showing a schematic of air flow across topographic barriers indicating the development of leeward eddies and the probable locations of forests in wind shadows. Adapted from Geldenhuys (1994).
Fuel and flammability
Grasses commonly fuel fire in mosaicked landscapes as their seasonally cured biomass can carpet open parts of the landscape in a continuous flammable understory (Bond and van Wilgen, 2012). Flammability, however, can vary significantly between grass species (Simpson et al., 2016), and the forest edge casts enough shade to induce a change in grass species composition, from faster to slower curing C4 species (Wills, 2015; Cardoso et al., 2018), or from flammable C4 to less flammable C3 species (Charles-Dominique et al., 2018). This predictable change in grass community near forest edges reduces the intensity of approaching fire fronts well before the flames reach the tree line (Cardoso et al., 2018) (Figure 3). Consequently, fires reach “healthy” forest edges (e.g. those that are not invaded by alien grasses or trees and not degraded by logging) at vastly slower speeds, and are easily extinguished by the discontinuity in fuel introduced by the forest’s understory and/or the lower flammability of the available ground fuels (Biddulph and Kellman, 1998; Hennenberg et al., 2006; Hoffmann et al., 2012a; Hoffmann et al., 2012b).
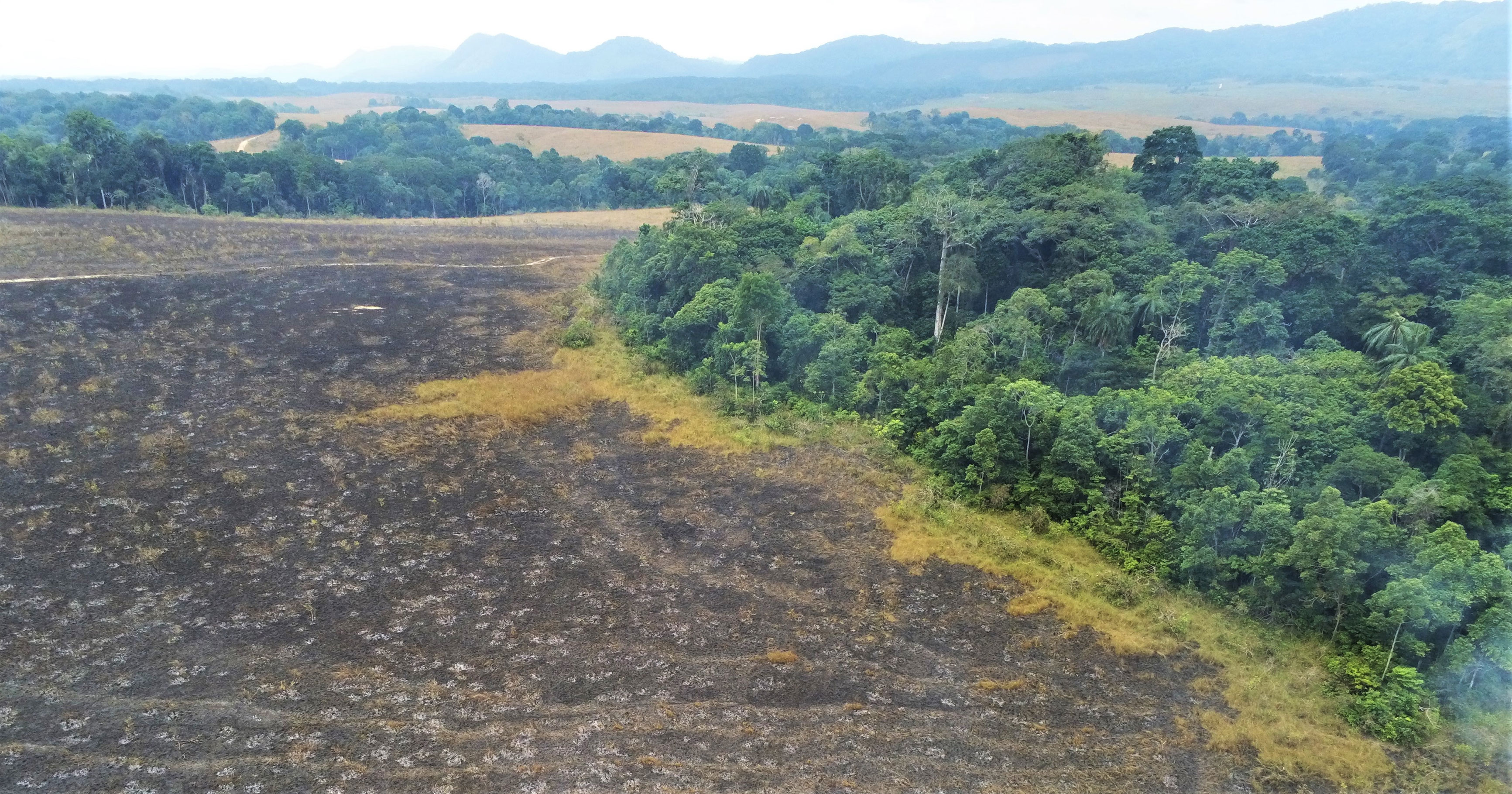
Figure 3 A forest edge in Lopé National Park, Gabon, shortly after the adjacent savanna burnt. The fire was extinguished by the buffer of slow curing, low flammability grasses before reaching the forest edge. (Photo: A. W. Cardoso).
The existence of low flammability “fire buffers” surrounding healthy forest patches and the intrinsic fire retardant nature of forests suggests that the colonial practice of fire suppression in open landscapes is not necessary to prevent forest patches burning (Bloesch, 1999). In fact, fire suppression accomplishes the opposite: without fire to consume biomass, flammable fuels accumulate in open vegetation and cause abnormally high fire intensities when they do eventually burn (Moreira et al., 2020). These intense burns cause rampant destruction of fire sensitive forest species when they overcome the fire suppressing effects of healthy forest edges (Kraaij et al., 2018; Beckett et al., 2022). Consequently, healthy forest edges can become degraded as a result of repeated high intensity burns, leading to a more open canopy that promotes repeated burning by facilitating the establishment of shade-sensitive flammable grasses (Balch et al., 2009; Silvério et al., 2013; Flores et al., 2016; Flores and Holmgren, 2021; Beckett et al., 2022). This is the process by which forests are often irreversibly lost after logging (Cochrane and Laurance, 2002).
Forest edge tree communities
Fire incursion into forests is prevented not only by lowered fire intensities near the forest edge but also by the existence of a fire-resistant edge-specialist tree community. This community is characterized by early successional species with thicker and faster growing bark that can withstand regular burning in a way that forest interior species cannot (Barlow et al., 2003; Hoffmann et al., 2009; Brando et al., 2012; Staver et al., 2019; Cardoso et al., 2021). Combined with its fire-resistance, the edge community also has a dense canopy that creates a light-blocking “wall of leaves” that effectively eliminates grassy fuels from the forest understory (Charles-Dominique et al., 2018; Cardoso et al., 2021). Forest edge trees thus ensure that the core forest remains protected from burning, even if the surrounding matrix goes up in flames (Adie et al., 2017; Beckett and Bond, 2019; Abiem et al., 2020).
To ensure the forest edge community fulfils its core-protecting function, its trees must be given time between burns to recover. A recovered community is one where the canopy has regrown from scorching and formed a new “wall of leaves”, and when an acceptable proportion of the trees have crossed the “fire resistance threshold”, above which their bark is thick enough to prevent them from being top-killed during burning (Hoffmann et al., 2012a). Interestingly, because the threshold bark thickness needed to prevent top-kill is lower for less intense fires, the forest edge tree community can recover quicker after burning when surrounded by an intensity-reducing grass buffer. For example, in higher rainfall forest-savanna mosaics in Gabon in Central Africa, recovery is so fast that edges can burn twice a year and still show no evidence that fire is threatening the forest core, with forest edges even expanding into the grassland in some cases (Jeffery et al., 2014).
A thriving edge community is essential to protect forest patches from burning, but the same community threatens neighboring flammable ecosystems under conditions of fire suppression. During periods of infrequent burning, the forest edge community – comprised of forest pioneer species (Cardoso et al., 2021) – will transgress the forest edge and become established in the flammable matrix (Favier et al., 2004). In some high rainfall areas in Africa, this is already happening and has prompted management to limit prescribed burning to the driest, hottest times of day in an effort to increase the intensity of the fires (Jeffery et al., 2014; Cardoso et al., 2018).
Firebreaks and fauna
Across ecosystems, a widely applied fire management tool is the construction of firebreaks. Firebreaks are discontinuities in the flammable fuel layer that aim to stop fire spread. Stopping fire spreading into forest patches is unlikely to be a management concern when the forest edge is healthy, since the edge performs this function well without assistance. However, if the forest edge is degraded and threatened by fire incursion, management may be tempted to install fire breaks along the forest edge (Frangiço and Birkinshaw, 2021). Anthropogenic firebreaks, such as defoliated strips of land or installed roads, are often expensive, labor intensive, ecologically destructive, and may not even be that effective (Price et al., 2007). Consequently, there are other ways to manage mosaicked landscapes that may help protect forest patches with degraded edges.
One strategy to manage fire regimes is rewilding (Johnson et al., 2018), or “reintroducing missing animals” (Monbiot, 2013) into an ecosystem. There is evidence that grazer extinctions in Africa lead to increased fire activity (Karp et al., 2021), and therefore reintroducing native grazers may help protect forest patches by decreasing fire frequency and intensity, since grazers compete with fire to consume the same grassy fuel (Hobbs, 1996; O’Connor et al., 2011; Kimuyu et al., 2014; Archibald and Hempson, 2016). Similarly, some animals favor forest edges for foraging, creating trails that can act as effective firebreaks under non-extreme burning conditions (Wills, 2015; Cardoso et al., 2020). Although ‘footpath firebreaks’ are more commonly created by large mammals such as elephant (Cardoso et al., 2020) (Figure 4), they have also been observed in animals as small as ants (Carvalho et al., 2012).
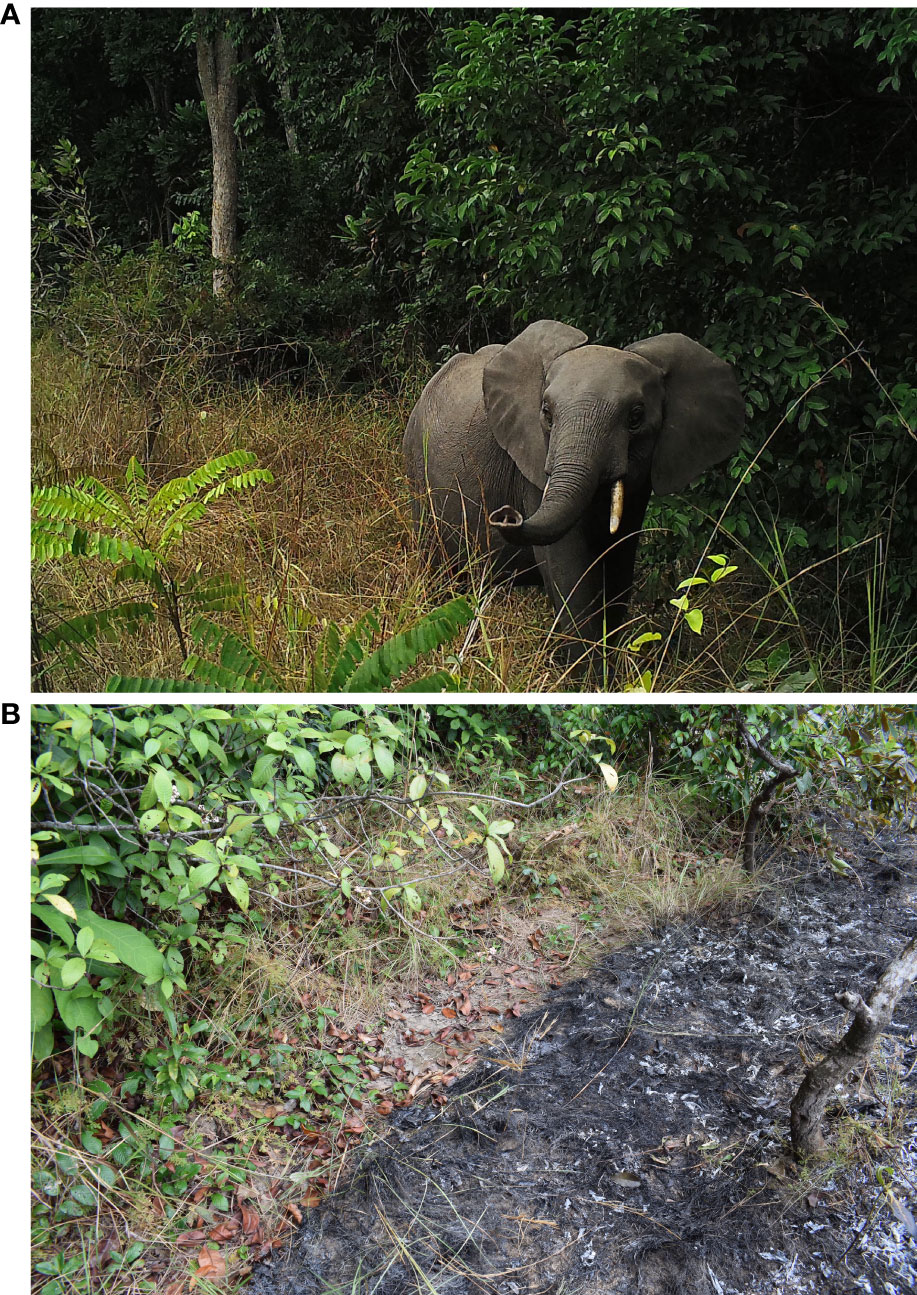
Figure 4 Showing how repeated travel along a path at the forest edge by forest elephants (Loxodonta cyclotis) (A) in Lopé National Park, Gabon, leads to the creation of a firebreak (B) that prevents savanna fires from burning into the forest interior. (Photos: A. W. Cardoso).
Managers can also employ “green” fire breaks, or strips of low flammability vegetation, that can help stop the spread of fire (Curran et al., 2017). In cases where forest edges are degraded, edge-specialist tree species could be planted along forest edges. In a central African forest savanna mosaic an edge community that was on average only 10m wide was able to efficiently stop 95% of fires reaching the forest core (Cardoso et al., 2021), suggesting that the effort required to restore a degraded forest edge may be similar to, but longer lasting than, creating firebreaks along the forest edge. Protecting and restoring forest edges may also involve seeding slow curing, low flammability grass species near the forest edge. An experimental burning study showed that, under regular (i.e. non-extreme) burning conditions, once a threshold mean fuel moisture content is reached in a part of the landscape, grass-fuelled fires are unable to spread even if sufficient fuel biomass is still available (Cardoso et al., 2022). Using this information, management can assess the likelihood that any section of grass is likely to act as a “green firebreak” based on its mean fuel moisture, mean biomass, and the expected fire weather (Cardoso et al., 2022).
Extreme fires and fire weather
As noted above, in African systems, fires from flammable open ecosystems rarely cross healthy boundaries into closed forest ecosystems. This can be due to landscape configuration and topographic refugia, changes in vegetation structure, composition, and flammability, or the existence of natural firebreaks (Figure 5). These buffers to fire transgressions create stability in mosaic ecosystems, whether it be decadal or millennial scale stability. There are however rare extreme events, “firestorms”, where these buffers no longer offer resistance and fires are able to penetrate deeper into forest patches (Beckett et al., 2022), providing a destabilizing mechanism which could trigger a ‘catastrophic regime shift’ and flip the system from a closed to an open canopy (Scheffer and Carpenter, 2003). Once such a shift takes place, it is very difficult, if not impossible, to return the systems to its original state without another extreme disturbance in the opposite direction (for example, total fire suppression for decades).
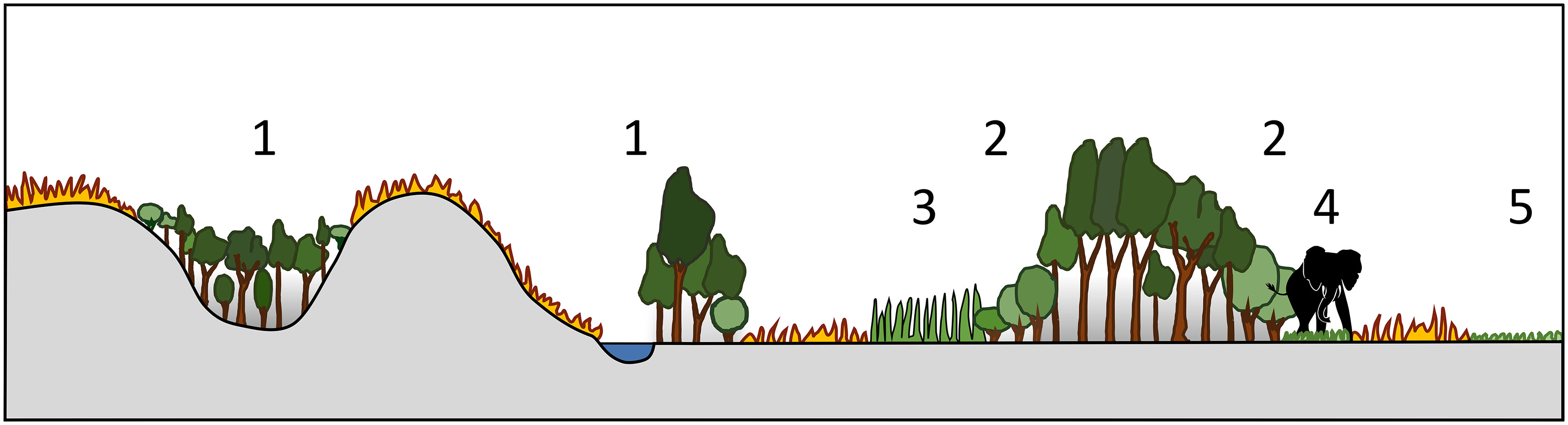
Figure 5 Natural protection of forest patches from fires in open, flammable landscapes. 1. Topographic refuge and barriers to fire spread 2. Fire-tolerant protective woody margins 3. Low flammability grass margins 4. Footpath firebreak margins 5. Low flammability grazing lawns.
The extent and severity of fires are controlled by a number of biotic and abiotic factors that range in both temporal and spatial scale (Bond and Keeley, 2005), which leads to a degree of variability even within single fire events. A ‘typical’ fire is constrained by its fire environment (wind speed, fuel availability and moisture content, topography). High-intensity fires, on the other hand, are powerful enough to influence and alter the fire environment. These are extreme natural phenomena which occur when a fire achieves sufficient intensity to begin generating its own wind system, a convective column caused by warm rising air, which draws in oxygen and fuels a particularly intense fire (Archibald et al., 2017; Beckett et al., 2022). An extreme fire occurred in a forest/savanna mosaic in Hluhluwe iMfolozi Park (HiP), South Africa, which helped to identify the conditions under which firestorms are thought to develop. Bradstock et al. (2009) usefully described these as a set of ‘switches’ that need to be ‘flipped on’ for a firestorm to develop (Bradstock et al., 2009). In the savanna/forest mosaic, key switches were sufficient biomass of dry grass fuel, air temperature above 30°C, relative humidity below 30% and wind speeds gusting to 30 km/h or more (Browne and Bond, 2011). Under these conditions the fire jumped from savannas, over the forest edge, and penetrated several hundred meters into closed forest.
These extreme fires are potentially useful tools for combatting bush encroachment and forest expansion in mosaics (Twidwell et al., 2016; Scholtz et al., 2022). Following firestorms in HiP, encroached, closed ‘thicket’ vegetation formations that were burnt were opened up, a regular fire regime was established followed by an increase in flammable grasses and the colonization of species associated with open vegetation types (Beckett et al., 2022). There are concerns, however, over the use of extreme fires as a management tool as they are very difficult to manage and apply safely (Smit et al., 2016). An attempt in Kruger National Park to create a firestorm in 2010 (Smit et al., 2016) was successful to the point that it burnt out of control, killing one rhino and causing a public outcry. Herein lies the issue, once the ‘switches are flipped on’, it is very difficult to turn a firestorm off. The uncertainty of the future of firestorms and how this will affect forests is an important area of research. Extreme events, especially wildfires, are becoming more common globally due to greater variability in rainfall and increased temperatures (Jolly et al., 2015). Understanding the effects of firestorms on vegetation dynamics is becoming increasingly relevant in the context of climatic change, particularly so in mosaics where landscape heterogeneity leads to a high diversity of specialized fauna and flora (Scholtz et al., 2022).
The future of mosaics
The future of mosaics of alternative biome states is difficult to forecast. The closed forest state is coupled to climate, but the open state is not – it would likely be forest if vegetation was directly climate-controlled. Extreme fires are much in the news and a major threat to people and to conservation. Major fires in conifer forests are transforming ecosystems in North America (Coop et al., 2020; Keeley and Syphard, 2021), while eucalypt forests in Australia have suffered unprecedented fires (Nolan et al., 2020; Bowman et al., 2021). These fires are thought to be linked to climate change, triggered by prolonged droughts and episodes of extreme fire weather with high temperatures, low humidity, and high wind speeds. These conditions dry out the woody fuels promoting high intensity fires in woody vegetation such as conifer and eucalypt forests. But the same conditions can have the opposite effect in the more arid grassy ecosystems. Prolonged drought and extreme weather can reduce fire activity in drier flammable grassy vegetation, where fire activity is often fuel limited. This is because adequate rain is essential for producing enough grass to fuel fire spread. Unlike woody fuels, bunch grasses, especially tropical C4 grasses, dry out rapidly in the usual annual dry seasons. Consequently extended droughts would be expected to reduce the probability of high intensity burns by reducing grassy fuels while good rains would tend to increase burnt area. Thus the climate changes that trigger big, dangerous fires have opposite effects depending on the fuel type (Krawchuk et al., 2009; Bradstock, 2010; Krawchuk and Moritz, 2011; Moritz et al., 2012; Pausas and Paula, 2012; Archibald et al., 2013; Alvarado et al., 2020).
Global change can also alter mosaics where rates of post-burn recovery are sensitive to climate change and to changing atmospheric CO2. Increased productivity, linked to climate change and increasing atmospheric CO2, would favor tree growth and the rate at which saplings or resprouts grow to fire-resistant sizes (Bond and Midgley, 2012; Calvo and Prentice, 2015). Forest margin trees benefitting from CO2 fertilization would be expected to recover more rapidly after fire damage. Thus a major effect of global change could be increased woody plant growth, manifested as expanding forest margins or woody thickening within the open savannas, and an associated decrease in grassy ecosystem function (Parr et al., 2012). If fire regimes were unchanged, or fire activity declined, we would then expect forest expansion at the expense of open ecosystems as a major challenge for conservation management (e.g. Wigley et al., 2010; Jeffery et al., 2014). Most studies in grassy ecosystems and a few in forest savanna mosaics have reported increased woody cover as a major trend over the last few decades (Archer et al., 2017; Ondei et al., 2017; Stevens et al., 2017; Venter, 2018). So is this the end of open grassy ecosystems? Not if conditions favoring high intensity fires were to increase threatening forest patches. An added complication is the spread of invasive species. Invasive flammable grasses are altering fire regimes and threatening forests in some regions (Rossi et al., 2014; Kerns et al., 2020). Elsewhere invasive shrubs, such as Lantana, reduce grassy fuels, suppressing fires, so that the open grassy ecosystems are threatened (Foxcroft et al., 2010; Mungi et al., 2020). In both cases, invasive plants change the fire regime which changes the configuration of landscape mosaics.
Conservation managers responsible for landscape mosaics have double the trouble of those managing landscapes with just a single biome. In addition to the uncertainties of climate-related changes to fire regimes, or CO2 driven changes in plant productivity and its effects on growth form mix, there are the added uncertainties created by alien invasive plants which might promote or inhibit fires. The presence or absence of native fauna with significant impacts on fire spread also need consideration. And to add to these ecological problems there are the cultural challenges restricting fire management: public aversion to burning and a fetish for forests common especially in developed countries (Silveira et al., 2022; Tölgyesi et al., 2022). Above all we have a pattern of changing land use by humans, fragmenting the landscape, transforming nature, and changing the probability of fires spreading across landscapes adding to a toxic mix for the future (Stevens et al., 2022). While in Africa climate change has thus far been more widely reported to be impacting grassy ecosystems over closed canopy forests, there is an incredible amount of uncertainty in the future and all scenarios are possible – from the expansion of forests in wetter climates under higher CO2 to the expansion of flammable grassy systems if fire intensity and frequency increases.
Applications
We suggest a set of guidelines for consideration by conservation managers in Africa concerned about fire damage to forest patches. We first suggest exploring the location of forest patches in the wider landscape to gauge intrinsic patch vulnerability to fire. The forest patches themselves should then be explored to determine whether there are fire-resistant forest margin communities. Fuel properties often change near forest patches with plant species switching to less flammable fuels. Margins may also have some intrinsic protection from footpath firebreaks. This evaluation of intrinsic forest patch protection will help indicate the necessity for any interventions.
1) Forest patch evaluation:
o In hilly country, evaluate topographic fire protection of each forest patch. Fires move slowly downslope and rapidly upslope so that, for example, forests at the bottom of a steep valley should be well protected. In landscapes of subdued relief, water bodies such as lakes, rivers, seasonal wetlands, or the sea protect forest patches. Quantitative tools are available for evaluating topographic protection.
o Forests with protective woody margins will not require special measures to avoid fire damage. They can be identified by differing in species composition from the forest interior and having functional traits resistant to fire and/or adapted to higher light conditions. These include thicker bark, protected buds, capacity to resprout after being burnt, and canopies casting more shade than savanna species. Foliage profiles (Bond et al., 1980) are a simple, integrated, rapid measure of contrasting architectures and light environments.
o Risks of forest fires can be greater if alien and/or invasive species have been planted next to native forest patches. Exotic conifers, eucalypts, and other intrinsically flammable shrubs increase the risk of spreading fires into the forest interior.
o Forests with protective grassy margins are less likely to sustain fire damage. These margins may differ in grass species composition from matrix grasses, have tall, thick culms but few leaves, and/or remain green much longer into the dry fire season.
o Forests with animal trails at or near the edges, or adjacent to heavily grazed patches are less likely to sustain fire damage. These animal trails can be a positive benefit of rewilding.
o Having evaluated forest patch vulnerability to fire, interventions to reduce risk can be considered. Prescribed burning is important both for protecting forest from extreme fires and for maintaining open ecosystem health.
2) Management interventions:
o If you are concerned about fire intruding into forest patches, burn under low intensity conditions using time of day, time of year, and optimal wind season and direction. Repeated low intensity fires can compromise the flammable ecosystem and negatively affect its biota, but total fire suppression is the most damaging while also increasing the risks from fuel build-up on forest margins.
o Where forest patches are protected in topographic refugia, fire direction should be set to the likely historical condition when fires would be expected to burn most intensely at the most fire-resistant margins.
o Fires initiated in flammable ecosystems are most likely to jump into closed forests under extreme weather conditions. Weather forecasting has vastly improved and should be used for early warning of extreme fire weather requiring extreme vigilance to prevent ignitions or to suppress fire starts very rapidly. The science and technology of predicting and controlling fire storms under extreme weather is far from being understood or applied effectively.
o Restoration of protective margins can reduce fire damage to the forest interior. Indigenous species should be used wherever possible. Exotic species such as highly flammable conifers and eucalypts should be removed.
o Avoid practices that open up forests allowing the understory to thicken up and providing fuel for fire spread. Logging or, for example, harvesting for charcoal has to be done with great care where forest patches are at risk of burning because of proximity to flammable ecosystems.
o It is natural for forest margins to be scorched where forests abut flammable open ecosystems. Scorched margins typically recover rapidly. The problems come when fires burn beyond the margins, penetrating into the forest interior killing poorly protected trees. Concentrated action to prevent follow-up fires will be needed until a forest canopy capable of shading out understorey plants has developed.
Conclusions
Closed/open mosaics are generally stable and only sometimes the result of recent human deforestation. However, they are simultaneously unstable, since they have the potential for regime shifts between states. An abrupt regime shift from forest to savanna, driven by major fires, is a common concern, often predicted for the Amazon forests. But the reverse transition, with open ecosystems being swallowed up by forests, is also a concern, especially in Africa, though of less concern since it is occurring at a slower pace than the havoc of abrupt fire events. Nevertheless, that pace is within the life span of a single tree and should not be overlooked. Changes in tree growth driven by a warmer world, with 50% more CO2 than preindustrial times, coupled with changing land use and reduced fire and large mammal herbivory poses widespread threats to many open ecosystems. Conservation of both systems requires a difficult balancing act and awareness of changes in both systems. The seemingly contradictory threats to closed and open ecosystems can seem impossible to manage concurrently in a mosaicked landscape. We hope the above guidelines for consideration can assist in a transition in theory and in practice to management of protected areas in Africa and elsewhere that reduce threats to both open and closed ecosystems thereby maintaining the rich landscape diversity from both shade-and sun-loving biotas and those species that make use of the edge habitats.
Data availability statement
The original contributions presented in the study are included in the article/supplementary material. Further inquiries can be directed to the corresponding author.
Author contributions
WB suggested the framework for this paper. AC prepared the final draft. All authors contributed to the article and approved the submitted version.
Funding
AC was supported by NASA Grant #80NSSC21K0086.
Acknowledgments
WB thanks the Association for Fire Ecology for their invitation to speak at the Fire Ecology Conference in Florence in October 2022, which helped crystallize the content of this contribution.
Conflict of interest
The authors declare that the research was conducted in the absence of any commercial or financial relationships that could be construed as a potential conflict of interest.
Publisher’s note
All claims expressed in this article are solely those of the authors and do not necessarily represent those of their affiliated organizations, or those of the publisher, the editors and the reviewers. Any product that may be evaluated in this article, or claim that may be made by its manufacturer, is not guaranteed or endorsed by the publisher.
References
Abiem I., Arellano G., Kenfack D., Chapman H. (2020). Afromontane forest diversity and the role of grassland-forest transition in tree species distribution. Diversity 12 (1), 30. doi: 10.3390/d12010030
Adie H., Kotze D. J., Lawes M. J. (2017). Small fire refugia in the grassy matrix and the persistence of afrotemperate forest in the Drakensberg mountains. Sci. Rep. 7 (1), 6549. doi: 10.1038/s41598-017-06747-2
Aleman J. C., Jarzyna M. A., Staver A. C. (2018). Forest extent and deforestation in tropical Africa since 1900. Nat. Ecol. Evol. 2 (1), 26–33. doi: 10.1038/s41559-017-0406-1
Alexander J. D., Seavy N. E., Ralph C. J., Hogoboom B. (2006). Vegetation and topographical correlates of fire severity from two fires in the klamath-siskiyou region of Oregon and California. Int. J. Wildland Fire 15 (2), 237. doi: 10.1071/WF05053
Alvarado S. T., Andela N., Silva T. S. F., Archibald S. (2020). Thresholds of fire response to moisture and fuel load differ between tropical savannas and grasslands across continents. Global Ecol. Biogeography 29 (2), 331–344. doi: 10.1111/geb.13034
Archer S. R., Andersen E. M., Predick K. I., Schwinning S., Steidl R. J., Woods S. R. (2017). “Woody plant encroachment: causes and consequences,” in Rangeland systems. Ed. Briske D. D. (Cham: Springer International Publishing), 25–84. doi: 10.1007/978-3-319-46709-2_2
Archibald S., Beckett H., Bond W. J., Coetsee C., Druce D. J., Staver A. C. (2017). “Interactions between fire and ecosystem processes,” in Conserving africa’s mega-diversity in the anthropocene: the hluhluwe-iMfolozi park story, 233.
Archibald S., Hempson G. P. (2016). Competing consumers: contrasting the patterns and impacts of fire and mammalian herbivory in Africa. Philos. Trans. R. Soc. B: Biol. Sci. 371 (1703), 20150309. doi: 10.1098/rstb.2015.0309
Archibald S., Lehmann C. E. R., Gómez-Dans J. L., Bradstock R. A. (2013). Defining pyromes and global syndromes of fire regimes. Proc. Natl. Acad. Sci. 110 (16), 6442–6447. doi: 10.1073/pnas.1211466110
Archibald S., Roy D. P., van Wilgen B. W., Scholes R. J. (2009). What limits fire? an examination of drivers of burnt area in southern Africa. Global Change Biol. 15 (3), 613–630. doi: 10.1111/j.1365-2486.2008.01754.x
Balch J. K., Nepstad D. C., Curran L. M. (2009). “Pattern and process: fire-initiated grass invasion at Amazon transitional forest edges,” in Tropical fire (Berlin: Springer), 481–502.
Barlow J., Lagan B. O., Peres C. A. (2003). Morphological correlates of fire-induced tree mortality in a central Amazonian forest. J. Trop. Ecol. 19 (3), 291–299. doi: 10.1017/S0266467403003328
Beckett H. (2018). Firestorms in a mesic savanna-forest mosaic (Cape Town: University of Cape Town).
Beckett H., Bond W. J. (2019). Fire refugia facilitate forest and savanna co-existence as alternative stable states. J. Biogeography 46 (12), 2800–2810. doi: 10.1111/jbi.13707
Beckett H., Staver A. C., Charles-Dominique T., Bond W. J. (2022). Pathways of savannization in a mesic African savanna–forest mosaic following an extreme fire. J. Ecol. 110 (4), 902–915. doi: 10.1111/1365-2745.13851
Biddulph J., Kellman M. (1998). Fuels and fire at savanna-gallery forest boundaries in southeastern Venezuela. J. Trop. Ecol. 14 (4), 445–461. doi: 10.1017/S0266467498000339
Bloesch U. (1999). Fire as a tool in the management of a savanna/dry forest reserve in Madagascar. Appl. Vegetation Sci. 2 (1), 117–124. doi: 10.2307/1478888
Bond W. J. (2019). Open ecosystems: ecology and evolution beyond the forest edge (Oxford University Press).
Bond W. J. (2021). Out of the shadows: ecology of open ecosystems. Plant Ecol. Diversity 14 (5–6), 202–222. doi: 10.1080/17550874.2022.2034065
Bond W. J., Ferguson M., Forsyth G. (1980). Small mammals and habitat structure along altitudinal gradients in the southern cape mountains. South Afr. J. Zoology 15 (1), 34–43. doi: 10.1080/02541858.1980.11447681
Bond W., Keeley J. (2005). Fire as a global ‘herbivore’: the ecology and evolution of flammable ecosystems. Trends Ecol. Evol. 20 (7), 387–394. doi: 10.1016/j.tree.2005.04.025
Bond W. J., Midgley G. F. (2012). Carbon dioxide and the uneasy interactions of trees and savannah grasses. Philos. Trans. R. Soc. B: Biol. Sci. 367 (1588), 601–612. doi: 10.1098/rstb.2011.0182
Bond W. J., Midgley G. F., Woodward F. I. (2003). The importance of low atmospheric CO 2 and fire in promoting the spread of grasslands and savannas: FIRE, LOW CO 2 and TREES. Global Change Biol. 9 (7), 973–982. doi: 10.1046/j.1365-2486.2003.00577.x
Bond W. J., Parr C. L. (2010). Beyond the forest edge: ecology, diversity and conservation of the grassy biomes. Biol. Conserv. 143 (10), 2395–2404. doi: 10.1016/j.biocon.2009.12.012
Bond W. J., Silander J. A., Ratsirarson J. (2022). Madagascar’s grassy biomes are ancient and there is much to learn about their ecology and evolution. J. Biogeography 14494. doi: 10.1111/jbi.14494
Bond W. J., van Wilgen B. W. (2012). Fire and plants (Netherlands: Springer Science & Business Media).
Bowman D. M. J. S., Williamson G. J., Gibson R. K., Bradstock R. A., Keenan R. J. (2021). The severity and extent of the Australia 2019–20 eucalyptus forest fires are not the legacy of forest management. Nat. Ecol. Evol. 5 (7), 1003–1010. doi: 10.1038/s41559-021-01464-6
Bowman D. M. J. S. (2000). Australian rainforests: islands of green in a land of fire. Cambridge, UK ; New York, NY, USA: Cambridge University Press.
Bradstock R. A. (2010). A biogeographic model of fire regimes in Australia: current and future implications: a biogeographic model of fire in Australia. Global Ecol. Biogeography 19 (2), 145–158. doi: 10.1111/j.1466-8238.2009.00512.x
Bradstock R. A., Cohn J. S., Gill A. M., Bedward M., Lucas C. (2009). Prediction of the probability of large fires in the Sydney region of south-eastern Australia using fire weather. Int. J. Wildland Fire 18 (8), 932. doi: 10.1071/WF08133
Bradstock R. A., Hammill K. A., Collins L., Price O. (2010). Effects of weather, fuel and terrain on fire severity in topographically diverse landscapes of south-eastern Australia. Landscape Ecol. 25 (4), 607–619. doi: 10.1007/s10980-009-9443-8
Brando P. M., Nepstad D. C., Balch J. K., Bolker B., Christman M. C., Coe M., et al. (2012). Fire-induced tree mortality in a neotropical forest: the roles of bark traits, tree size, wood density and fire behavior. Global Change Biol. 18 (2), 630–641. doi: 10.1111/j.1365-2486.2011.02533.x
Browne C., Bond W. J. (2011). Firestorms in savanna and forest ecosytems: curse or cure? Veld Flora 97 (2), 62–63.
Buisson E., Le Stradic S., Silveira F. A. O., Durigan G., Overbeck G. E., Fidelis A., et al. (2019). Resilience and restoration of tropical and subtropical grasslands, savannas, and grassy woodlands: tropical grassland resilience and restoration. Biol. Rev. 94 (2), 590–609. doi: 10.1111/brv.12470
Calvo M. M., Prentice I. C. (2015). Effects of fire and CO2 on biogeography and primary production in glacial and modern climates. New Phytol 208 (3), 987–994. doi: 10.1111/nph.13485
Cardoso A. W., Archibald S., Bond W. J., Coetsee C., Forrest M., Govender N., et al. (2022). Quantifying the environmental limits to fire spread in grassy ecosystems. Proc. Natl. Acad. Sci. 119 (26), e2110364119. doi: 10.1073/pnas.2110364119
Cardoso A. W., Malhi Y., Oliveras I., Lehmann D., Ndong J. E., Dimoto E., et al. (2020). The role of forest elephants in shaping tropical forest–savanna coexistence. Ecosystems 23 (3), 602–616. doi: 10.1007/s10021-019-00424-3
Cardoso A. W., Oliveras I., Abernethy K. A., Jeffery K. J., Glover S., Lehmann D., et al. (2021). A distinct ecotonal tree community exists at central African forest–savanna transitions. J. Ecol. 109 (3), 1170–1183. doi: 10.1111/1365-2745.13549
Cardoso A. W., Oliveras I., Abernethy K. A., Jeffery K. J., Lehmann D., Edzang Ndong J., et al. (2018). Grass species flammability, not biomass, drives changes in fire behavior at tropical forest-savanna transitions. Front. Forests Global Change 1. doi: 10.3389/ffgc.2018.00006
Carvalho K. S., Alencar A., Balch J., Moutinho P. (2012). Leafcutter ant nests inhibit low-intensity fire spread in the understory of transitional forests at the amazon’s forest-savanna boundary. Psyche: A J. Entomology 2012, 1–7. doi: 10.1155/2012/780713
Cerling T. E., Harris J. M., MacFadden B. J., Leakey M. G., Quade J., Eisenmann V., et al. (1997). Global vegetation change through the Miocene/Pliocene boundary. Nature 389 (6647), 153–158. doi: 10.1038/38229
Charles-Dominique T., Midgley G. F., Tomlinson K. W., Bond W. J. (2018). Steal the light: shade vs fire adapted vegetation in forest-savanna mosaics. New Phytol. 218 (4), 1419–1429. doi: 10.1111/nph.15117
Clarke P. J. (2002). Habitat islands in fire-prone vegetation: do landscape features influence community composition? J. Biogeography 29 (5–6), 677–684. doi: 10.1046/j.1365-2699.2002.00716.x
Cochrane M. A., Laurance W. F. (2002). Fire as a large-scale edge effect in Amazonian forests. J. Trop. Ecol. 18 (3), 311–325. doi: 10.1017/S0266467402002237
Coop J. D., Parks S. A., Stevens-Rumann C. S., Crausbay S. D., Higuera P. E., Hurteau M. D., et al. (2020). Wildfire-driven forest conversion in Western north American landscapes. BioScience 70 (8), 659–673. doi: 10.1093/biosci/biaa061
Curran T., Perry G., Wyse S., Alam M. (2017). Managing fire and biodiversity in the wildland-urban interface: a role for green firebreaks. Fire 1 (1), 3. doi: 10.3390/fire1010003
Durigan G., Ratter J. A. (2016). The need for a consistent fire policy for cerrado conservation. J. Appl. Ecol. 53 (1), 11–15. doi: 10.1111/1365-2664.12559
Erdős L., György K.-D., Zoltán B., Bence K., Csaba N., János K. P., et al. (2018). Habitat heterogeneity as a key to high conservation value in forest-grassland mosaics. Biol. Conserv. 226, 72–80. doi: 10.1016/j.biocon.2018.07.029
Fairhead J., Leach M. (1996). Misreading the African landscape: society and ecology in a forest-savanna mosaic (Cambridge University Press).
Favier C., De Namur C., Dubois M.-A. (2004). Forest progression modes in littoral Congo, central Atlantic Africa: forest progression modes in Congo. J. Biogeography 31 (9), 1445–1461. doi: 10.1111/j.1365-2699.2004.01094.x
Feeley J. M. (1980). Did iron age man have a role in the history of zululand’s wilderness landscapes? South Afr. J. Sci. 76 (4), 150–152.
Flores B. M., Fagoaga R., Nelson B. W., Holmgren M. (2016). Repeated fires trap Amazonian blackwater floodplains in an open vegetation state. J. Appl. Ecol. 53 (5), 1597–1603. doi: 10.1111/1365-2664.12687
Flores B. M., Holmgren M. (2021). White-sand savannas expand at the core of the Amazon after forest wildfires. Ecosystems 24 (7), 1624–1637. doi: 10.1007/s10021-021-00607-x
Foxcroft L. C., Richardson D. M., Rejmánek M., Pyšek P. (2010). Alien plant invasions in tropical and sub-tropical savannas: patterns, processes and prospects. Biol. Invasions 12 (12), 3913–3933. doi: 10.1007/s10530-010-9823-7
Frangiço J. M., Birkinshaw C. (2021). Status of three threatened lemur species At the analavelona scared forest, south-Western Madagascar, Vol. 4. Available at: http://mobot.mg/conservation/wp-content/uploads/2022/06/STATUS-OF-THREE-THREATENED-LEMUR-SPECIES-AT-THE-ANALAVELONA-SCARED-FOREST.pdf.
Geldenhuys C. J. (1994). Bergwind fires and the location pattern of forest patches in the southern cape landscape, south Africa. J. Biogeography 21 (1), 49. doi: 10.2307/2845603
Granger J. E. (1984). “Fire in forest. in ecological effects of fire in south African ecosystems,” in Ecological effects of fire in south African ecosystems (Berlin: Springer), 177–197.
Hennenberg K. J., Fischer F., Kouadio K., Goetze D., Orthmann B., Linsenmair K. E., et al. (2006). Phytomass and fire occurrence along forest–savanna transects in the comoé national park, ivory coast. J. Trop. Ecol. 22 (03), 303–311. doi: 10.1017/S0266467405003007
Higgins S. I., Scheiter S. (2012). Atmospheric CO2 forces abrupt vegetation shifts locally, but not globally. Nature 488 (7410), 209–212. doi: 10.1038/nature11238
Hirota M., Holmgren M., Van Nes E. H., Scheffer M. (2011). Global resilience of tropical forest and savanna to critical transitions. Science 334 (6053), 232–235. doi: 10.1126/science.1210657
Hobbs N. T. (1996). Modification of ecosystems by ungulates. J. Wildlife Manage. 60 (4), 695. doi: 10.2307/3802368
Hoffmann W. A., Adasme R., Haridasan M., T. de Carvalho M., Geiger E. L., Pereira M. A. B., et al. (2009). Tree topkill, not mortality, governs the dynamics of savanna–forest boundaries under frequent fire in central Brazil. Ecology 90 (5), 1326–1337. doi: 10.1890/08-0741.1
Hoffmann W. A., Geiger E. L., Gotsch S. G., Rossatto D. R., Silva L. C. R., Lau O. L., et al. (2012a). Ecological thresholds at the savanna-forest boundary: how plant traits, resources and fire govern the distribution of tropical biomes. Ecol. Lett. 15 (7), 759–768. doi: 10.1111/j.1461-0248.2012.01789.x
Hoffmann W. A., Jaconis S. Y., Mckinley K. L., Geiger E. L., Gotsch S. G., Franco A. C. (2012b). Fuels or microclimate? understanding the drivers of fire feedbacks at savanna-forest boundaries: FLAMMABILITY OF SAVANNA-FOREST BOUNDARY. Austral Ecol. 37 (6), 634–643. doi: 10.1111/j.1442-9993.2011.02324.x
Holden Z. A., Morgan P., Evans J. S. (2009). A predictive model of burn severity based on 20-year satellite-inferred burn severity data in a large southwestern US wilderness area. For. Ecol. Manage. 258 (11), 2399–2406. doi: 10.1016/j.foreco.2009.08.017
Jeffery K. J., Korte L., Palla F., Walters G., White L. J. T., Abernethy K. A. (2014). Fire management in a changing landscape: a case study from lopé national park, Gabon. PARKS 20 (1), 39–52. doi: 10.2305/IUCN.CH.2014.PARKS-20-1.KJJ.en
Johnson C. N., Prior L. D., Archibald S., Poulos H. M., Barton A. M., Williamson G. J., et al. (2018). Can trophic rewilding reduce the impact of fire in a more flammable world? Philos. Trans. R. Soc. B: Biol. Sci. 373 (1761), 20170443. doi: 10.1098/rstb.2017.0443
Jolly W. M., Cochrane M. A., Freeborn P. H., Holden Z. A., Brown T. J., Williamson G. J., et al. (2015). Climate-induced variations in global wildfire danger from 1979 to 2013. Nat. Commun. 6 (1), 7537. doi: 10.1038/ncomms8537
Joseph G. S., Seymour C. L. (2021). The unlikely a’ntiquity of madagascar’s grasslands’: disproportionately forest-limited endemic fauna support anthropogenic transformation from woodland. J. Biogeography 48 (8), 2111–2115. doi: 10.1111/jbi.14132
Karp A. T., Faith J. T., Marlon J. R., Staver A. C. (2021). Global response of fire activity to late quaternary grazer extinctions. Science 374 (6571), 1145–1148. doi: 10.1126/science.abj1580
Keeley J. E., Syphard A. D. (2021). Large California Wildfires: 2020 fires in historical context. Fire Ecol. 17 (1), 22. doi: 10.1186/s42408-021-00110-7
Kerns B. K., Tortorelli C., Day M. A., Nietupski T., Barros A. M. G., Kim J. B., et al. (2020). Invasive grasses: a new perfect storm for forested ecosystems? For. Ecol. Manage. 463, 117985. doi: 10.1016/j.foreco.2020.117985
Kgope B. S., Bond W. J., Midgley G. F. (2009). Growth responses of African savanna trees implicate atmospheric [CO2] as a driver of past and current changes in savanna tree cover: RESPONSE OF AFRICAN SAVANNA TREES TO CO2. Austral Ecol. 35 (4), 451–463. doi: 10.1111/j.1442-9993.2009.02046.x
Kimuyu D. M., Sensenig R. L., Riginos C., Veblen K. E., Young T. P. (2014). Native and domestic browsers and grazers reduce fuels, fire temperatures, and acacia ant mortality in an African savanna. Ecol. Appl. 24 (4), 741–749. doi: 10.1890/13-1135.1
Kraaij T., Baard J. A., Arndt J., Vhengani L., van Wilgen B. W. (2018). An assessment of climate, weather, and fuel factors influencing a large, destructive wildfire in the knysna region, south Africa. Fire Ecol. 14 (2), 4. doi: 10.1186/s42408-018-0001-0
Krawchuk M. A., Moritz M. A. (2011). Constraints on global fire activity vary across a resource gradient. Ecology 92 (1), 121–132. doi: 10.1890/09-1843.1
Krawchuk M. A., Moritz M. A., Parisien M.-A., Van Dorn J., Hayhoe K. (2009). Global pyrogeography: the current and future distribution of wildfire. PLoS One 4 (4), e5102. doi: 10.1371/journal.pone.0005102
Kushla J. D., Ripple W. J. (1997). The role of terrain in a fire mosaic of a temperate coniferous forest. For. Ecol. Manage. 95 (2), 97–107. doi: 10.1016/S0378-1127(97)82929-5
Lehmann C. E. R., Archibald S. A., Hoffmann W. A., Bond W. J. (2011). Deciphering the distribution of the savanna biome. New Phytol. 191 (1), 197–209. doi: 10.1111/j.1469-8137.2011.03689.x
Mermoz M., Kitzberger T., Veblen T. T. (2005). LANDSCAPE INFLUENCES ON OCCURRENCE AND SPREAD OF WILDFIRES IN PATAGONIAN FORESTS AND SHRUBLANDS. Ecology 86 (10), 2705–2715. doi: 10.1890/04-1850
Mills A. J., Milewski A. V., Fey M. V., Gröngröft A., Petersen A., Sirami C. (2013). Constraint on woody cover in relation to nutrient content of soils in western southern Africa. Oikos 122 (1), 136–148. doi: 10.1111/j.1600-0706.2012.20417.x
Monbiot G. (2013). “A Manifesto for Rewilding the World,” Available at: https://www.monbiot.com/2013/05/27/a-manifesto-for-rewilding-the-world/. (Accessed Aug. 19, 2022).
Moreira F., Ascoli D., Safford H., Adams M. A., Moreno J. M., Pereira J. M. C., et al. (2020). Wildfire management in Mediterranean-type regions: paradigm change needed. Environ. Res. Lett. 15 (1), 011001. doi: 10.1088/1748-9326/ab541e
Moritz M. A., Parisien M.-A., Batllori E., Krawchuk M. A., Van Dorn J., Ganz D. J., et al. (2012). Climate change and disruptions to global fire activity. Ecosphere 3 (6), art49. doi: 10.1890/ES11-00345.1
Mungi N. A., Qureshi Q., Jhala Y. V. (2020). Expanding niche and degrading forests: key to the successful global invasion of lantana camara (sensu lato). Global Ecol. Conserv. 23, e01080. doi: 10.1016/j.gecco.2020.e01080
Nolan R. H., Boer M. M., Collins L., Resco de Dios V., Clarke H., Jenkins M., et al. (2020). Causes and consequences of eastern australia’s 2019–20 season of mega-fires. Global Change Biol. 26 (3), 1039–1041. doi: 10.1111/gcb.14987
O’Connor T. G., Mulqueeny C. M., Goodman P. S. (2011). Determinants of spatial variation in fire return period in a semiarid African savanna. Int. J. Wildland Fire 20 (4), 540. doi: 10.1071/WF08142
Ondei S., Prior L. D., Vigilante T., Bowman D. M. J. S. (2017). Fire and cattle disturbance affects vegetation structure and rain forest expansion into savanna in the Australian monsoon tropics. J. Biogeography 44 (10), 2331–2342. doi: 10.1111/jbi.13039
Parr C. L., Gray E. F., Bond W. J. (2012). Cascading biodiversity and functional consequences of a global change-induced biome switch: savanna biome switch. Diversity Distributions 18 (5), 493–503. doi: 10.1111/j.1472-4642.2012.00882.x
Pausas J. G., Bond W. J. (2021). Alternative biome states challenge the modelling of species’ niche shifts under climate change. J. Ecol. 109 (12), 3962–3971. doi: 10.1111/1365-2745.13781
Pausas J. G., Paula S. (2012). Fuel shapes the fire-climate relationship: evidence from Mediterranean ecosystems: fuel shapes the fire-climate relationship. Global Ecol. Biogeography 21 (11), 1074–1082. doi: 10.1111/j.1466-8238.2012.00769.x
Penman T. D., Kavanagh R. P., Binns D. L., Melick D. R. (2007). Patchiness of prescribed burns in dry sclerophyll eucalypt forests in south-eastern Australia. For. Ecol. Manage. 252 (1–3), 24–32. doi: 10.1016/j.foreco.2007.06.004
Philiips J. (1931). Forest-succession and ecology in the knysna region (Vol. 14) (South Africa: Government Printer).
Philips J. (1963). The forests of George, knysna and the zitzikama: a brief history of their management: 1778-1939 (South Africa: Department of Forestry).
Price O., Edwards A., Russell-Smith J. (2007). Efficacy of permanent firebreaks and aerial prescribed burning in western arnhem land, northern territory, Australia. Int. J. Wildland Fire - Int. J. WILDLAND FIRE 16. doi: 10.1071/WF06039
Ripley B. S., Raubenheimer S. L., Perumal L., Anderson M., Mostert E., Kgope B. S., et al. (2022). CO 2 -fertilisation enhances resilience to browsing in the recruitment phase of an encroaching savanna tree. Funct. Ecol. 36 (12), 3223–3233. doi: 10.1111/1365-2435.14215
Rossi R. D., Martins C. R., Viana P. L., Rodrigues E. L., Figueira J. E. C. (2014). Impact of invasion by molasses grass (Melinis minutifloraP. beauv.) on native species and on fires in areas of campo-cerrado in Brazil. Acta Botanica Brasilica 28 (4), 631–637. doi: 10.1590/0102-33062014abb3390
Rutherford M. C., Westfall R. H. (1994). Biomes of southern Africa: an objective categorization (South Africa: National Botanical Institute).
Sato H., Kelley D. I., Mayor S. J., Martin Calvo M., Cowling S. A., Prentice I. C. (2021). Dry corridors opened by fire and low CO2 in Amazonian rainforest during the last glacial maximum. Nat. Geosci. 14 (8), 578–585. doi: 10.1038/s41561-021-00777-2
Scheffer M., Carpenter S. R. (2003). Catastrophic regime shifts in ecosystems: linking theory to observation. Trends Ecol. Evol. 18 (12), 648–656. doi: 10.1016/j.tree.2003.09.002
Scholtz R., Donovan V. M., Strydom T., Wonkka C., Kreuter U. P., Rogers W. E., et al. (2022). High-intensity fire experiments to manage shrub encroachment: lessons learned in south Africa and the united states. Afr. J. Range Forage Sci. 39 (1), 148–159. doi: 10.2989/10220119.2021.2008004
Silveira F. A. O., Ordóñez-Parra C. A., Moura L. C., Schmidt I. B., Andersen A. N., Bond W., et al. (2022). Biome awareness disparity is BAD for tropical ecosystem conservation and restoration. J. Appl. Ecol. 59 (8), 1967–1975. doi: 10.1111/1365-2664.14060
Silvério D. V., Brando P. M., Balch J. K., Putz F. E., Nepstad D. C., Oliveira-Santos C., et al. (2013). Testing the Amazon savannization hypothesis: fire effects on invasion of a neotropical forest by native cerrado and exotic pasture grasses. Philos. Trans. R. Soc. B: Biol. Sci. 368 (1619), 20120427. doi: 10.1098/rstb.2012.0427
Simpson K. J., Ripley B. S., Christin P., Belcher C. M., Lehmann C. E. R., Thomas G. H., et al. (2016). Determinants of flammability in savanna grass species. J. Ecol. 104 (1), 138–148. doi: 10.1111/1365-2745.12503
Smit I. P. J., Asner G. P., Govender N., Vaughn N. R., van Wilgen B. W. (2016). An examination of the potential efficacy of high-intensity fires for reversing woody encroachment in savannas. J. Appl. Ecol. 53 (5), 1623–1633. doi: 10.1111/1365-2664.12738
Staal A., Nes E. H., Hantson S., Holmgren M., Dekker S. C., Pueyo S., et al. (2018). Resilience of tropical tree cover: the roles of climate, fire, and herbivory. Global Change Biol. 24 (11), 5096–5109. doi: 10.1111/gcb.14408
Staver A. C., Archibald S., Levin S. (2011a). Tree cover in sub-Saharan Africa: rainfall and fire constrain forest and savanna as alternative stable states. Ecology 92 (5), 1063–1072. doi: 10.1890/10-1684.1
Staver A. C., Archibald S., Levin S. A. (2011b). The global extent and determinants of savanna and forest as alternative biome states. Science 334 (6053), 230–232. doi: 10.1126/science.1210465
Staver A. C., Barlow J., Morton C., Paine C. E. T., Malhi Y., Murakami A., et al. (2019). Thinner bark increases sensitivity of wetter Amazonian tropical forests to fire. Ecol Lett 8, 1–8.
Staver A. C., Botha J., Hedin L. (2017). Soils and fire jointly determine vegetation structure in an African savanna. New Phytol. 10. doi: 10.1111/nph.14738
Stevens N., Bond W., Feurdean A., Lehmann C. E. R. (2022). Grassy ecosystems in the anthropocene. Annu. Rev. Environ. Resour. 47 (1), 261–289. doi: 10.1146/annurev-environ-112420-015211
Stevens N., Lehmann C. E. R., Murphy B. P., Durigan G. (2017). Savanna woody encroachment is widespread across three continents. Global Change Biol. 23 (1), 235–244. doi: 10.1111/gcb.13409
Tölgyesi C., Buisson E., Helm A., Temperton V. M., Török P. (2022). Urgent need for updating the slogan of global climate actions from “tree planting” to “restore native vegetation”. Restor. Ecol. 30 (3). doi: 10.1111/rec.13594
Twidwell D., Rogers W. E., Wonkka C. L., Taylor C. A., Kreuter U. P. (2016). Extreme prescribed fire during drought reduces survival and density of woody resprouters. J. Appl. Ecol. 53 (5), 1585–1596. doi: 10.1111/1365-2664.12674
Veenendaal E. M., Torello-Raventos M., Miranda H. S., Sato N. M., Oliveras I., van Langevelde F., et al. (2018). On the relationship between fire regime and vegetation structure in the tropics. New Phytol. 218 (1), 153–166. doi: 10.1111/nph.14940
Veldman J. W. (2016). Clarifying the confusion: old-growth savannahs and tropical ecosystem degradation. Philos. Trans. R. Soc. B: Biol. Sci. 371 (1703), 20150306. doi: 10.1098/rstb.2015.0306
Veldman J. W., Buisson E., Durigan G., Fernandes G. W., Le Stradic S., Mahy G., et al. (2015). Toward an old-growth concept for grasslands, savannas, and woodlands. Front. Ecol. Environ. 13 (3), 154–162. doi: 10.1890/140270
Venter Z. S. (2018). Drivers of woody plant encroachment over Africa. Nat. Commun. 7. doi: 10.1038/s41467-018-04616-8
Wigley B. J., Bond W. J., Hoffman M. T. (2010). Thicket expansion in a south African savanna under divergent land use: local vs. global drivers? Global Change Biol. 16 (3), 964–976. doi: 10.1111/j.1365-2486.2009.02030.x
Wills C. (2015). The differences in grass species composition and the effects on fire behaviour in an African mesic savanna (Cape Town: MSc, University of Cape Town). Available at: https://open.uct.ac.za/bitstream/handle/11427/20014/thesis_sci_2015_wills_cameron.pdf?sequence=1.
Keywords: fire, mosaic, forest, landscape management, open ecosystem, grassland, savanna, alternative stable states
Citation: Cardoso AW, Beckett H and Bond WJ (2023) How forests survive alongside flammable open ecosystems: conservation implications for Africa. Front. Conserv. Sci. 4:1150516. doi: 10.3389/fcosc.2023.1150516
Received: 24 January 2023; Accepted: 11 April 2023;
Published: 28 April 2023.
Edited by:
Orsolya Valkó, Hungarian Academy of Sciences, HungaryReviewed by:
Swanni Alvarado, State University of Maranhão, BrazilCsaba Tölgyesi, University of Szeged, Hungary
Copyright © 2023 Cardoso, Beckett and Bond. This is an open-access article distributed under the terms of the Creative Commons Attribution License (CC BY). The use, distribution or reproduction in other forums is permitted, provided the original author(s) and the copyright owner(s) are credited and that the original publication in this journal is cited, in accordance with accepted academic practice. No use, distribution or reproduction is permitted which does not comply with these terms.
*Correspondence: Anabelle Williamson Cardoso, YW5hYmVsbGVjYXJkb3NvQGdtYWlsLmNvbQ==
†ORCID: Fang Zhou, orcid.org/0000-0002-4327-7259