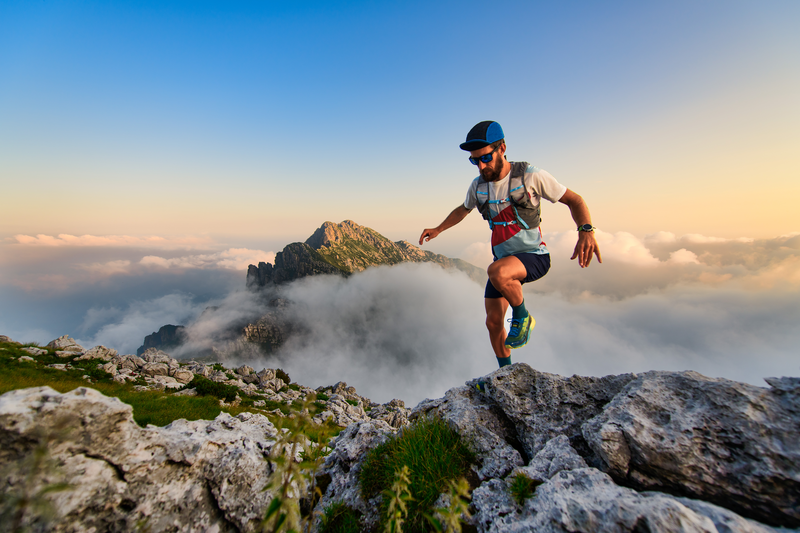
95% of researchers rate our articles as excellent or good
Learn more about the work of our research integrity team to safeguard the quality of each article we publish.
Find out more
ORIGINAL RESEARCH article
Front. Conserv. Sci. , 08 May 2023
Sec. Plant Conservation
Volume 4 - 2023 | https://doi.org/10.3389/fcosc.2023.1130138
This article is part of the Research Topic Agrobiodiversity at Different Scales for Improving Conservation Strategies View all 9 articles
Introduction: Effective monitoring of the in situ conservation status and change dynamics of landrace populations in their centers of origin ideally requires the identification of sites that are complementary in terms of the richness, uniqueness and coverage of genetic diversity.
Methods: We applied a 4-step approach to identify sites of high potato landrace diversity which will guide the set-up of a network of complementary prospective conservation observatories in Peru, the potato center of origin. A GIS mapping approach was used to determine which combination of sites would provide the most comprehensive and complementary genepool coverage. A landrace inventory was developed from 49 sources, comprising 47,272 landrace records, 97.1% with coordinates, which was used to derive landrace, cultivated species, and cultivar group richness. Data on known indicators of agrobiodiversity, including potato wild relative concurrence, cultivated area, ecogeographic diversity, and ethnolinguistic diversity were included in the spatial overlay analysis, which was used in conjunction with expert opinion data to provide further insight to hotspot selection.
Results: Thirteen hotspots with high, unique, and complementary levels of landrace diversity were identified. We recommend that robust baselines are established, documenting current diversity in these sites using semi-standardized methods and metrics for future tracking.
Discussion: Our results, while being the most robust of their kind to date, were inevitably affected by data gaps, infrastructure and hotspot biases. New documentation efforts should record landrace diversity in uncovered regions, as well as explore complementary mechanisms to track the conservation status of unique endemic landraces that occur in coldspots.
A large reservoir of crop genetic diversity continues to be conserved on-farm with the most widely utilized diversity by farmers and breeders being landrace populations (Dreisigacker et al., 2005; Veteläinen et al., 2009; Oliveira et al., 2016). These locally adapted traditional, ancestral, farmer or indigenous varieties have developed - and continue to evolve - through an intricate process of human selection and diversification in response to dynamic socioecological environments (Camacho Villa et al., 2005; Tiranti and Negri, 2007; Choudhury et al., 2013). Intraspecific diversity provides an essential means for households and rural communities to achieve yield stability, adaptive capacity and food security while retaining their cultural identity. Landraces also offer a key reservoir of characters providing farmers with the flexibility to adapt to diverse environmental or biological conditions, as well as being an essential source of traits for the development of new resilient and nutritious crop varieties (Frankel and Bennett, 1970; Esquinas-Alcazar, 1993; Gibson, 2009; Zabel et al., 2021). Importantly, landraces are also biocultural resources carrying local identity, prestige and heritage (Nazarea, 1998; Ellen and Simon Platten, 2011).
Potato is the fourth most important staple in the world in terms of production and the most important non-grain crop for human food intake (Ezekiel et al., 2013). Its domestication is characterized by a complex evolutionary history (Hawkes, 1990; Hardigan et al., 2017; Denham et al., 2020). The Andes are its center of origin where, 8,000 to 10,000 years after its initial domestication, a large pool of landraces continue to be managed and nurtured by smallholder and indigenous farmers (De Haan et al., 2010; Monteros, 2011; CIP et al., 2021). International missions to collect and safeguard diversity ex situ started in the 1930s in response to the early needs of formal breeding programs and perceived threat of diversity loss (Hawkes, 1973; Ochoa, 1975). The International Potato Centre (CIP) was established in Peru in 1971 with the mission to securely conserve potato genetic resources. Soon after its establishment, CIP scientists recognized the unique role of on-farm conservation and started researching the ongoing conservation dynamics and human ecology of the crop in its natural habitat (Jackson et al., 1980; Brush et al., 1981).
The complementary nature of integrated crop genetic resources approaches, or efforts aiming to effectively link ex situ and in situ approaches, is receiving increased recognition (Maxted et al., 1997; Dulloo, 2010; Nagel et al., 2022). In the case of potato in Peru it has resulted in multidirectional germplasm exchange and the emergence of diverse conservation approaches ranging from agrobiodiversity zones and annual biodiversity seed fairs to Payments for Agrobiodiversity Conservation Services schemes. However, processes of regular monitoring and tracking remain relatively underdeveloped within integrated conservation contexts, hindering the wider understanding of processes of varietal and genetic change. The need for such a framework is arguably more urgent than ever. The high Andes are characterized by accelerated change which is likely to affect intraspecific diversity. The climate crisis is affecting these high altitude rainfed cropping systems through increased temperatures and pest-disease pressure as well as more frequent extreme out-of-season weather events such as hails, frosts and droughts (Hijmans, 2003; Quiroz et al., 2018; Raymundo et al., 2018; Klein et al., 2019; Narouei-Khandan et al., 2020; Poveda et al., 2020). The bulk of landrace diversity is concentrated in a narrow altitudinal range with intense levels of abiotic stress (De Haan and Juarez, 2010; Arce et al., 2019). Rapidly changing socioeconomic drivers such as markets, migration and food policies also affect Andean agriculture (Remy, 2014; Antiporta et al., 2016; Bedoya-Perales et al., 2020).
Historically, it is likely that landrace populations in the Andes have been affected by numerous extreme events. These would have included the little ice age (1300-1800), the demographic collapse following the Conquista (1520-1620) and more recently from 1980 to 1995 a period of rural violence in Peru (Smith et al., 1970; Rabatel et al., 2008). The current reality for potato diversity in the Andes suggests a complex dynamic with processes of loss, enrichment and conservation occurring in parallel (De Haan et al., 2010; Monteros, 2011). Even for a relatively well-studied crop like potato, a knowledge gap persists when it comes to the conservation status of landraces in the centre of origin (Veteläinen et al., 2009). Robust country-wide inventories of landraces do not yet exist in any country (Veteläinen et al., 2009). However, a rough estimate of 3,000-4,000 landraces is often mentioned for Peru based on genebank inventories (De Haan and Rodriguez, 2016; Lüttringhaus et al., 2021; Parra-Rondinel et al., 2021). The volume of detailed baseline inventories at the subnational level continues to grow (e.g., De Haan et al, 2016b; CIP et al, 2021).
The crop genetic resources community remains a late adopter of in situ monitoring approaches compared to communities of practice working with wild flora and fauna where in situ application is the norm and ex situ is only used as a backup for in situ (Maxted et al., 1997). An underlying factor relates to the comparatively complex nature of documenting landraces as compared to species and that for agrobiodiversity ex situ provides the means of supplying the germplasm to potential users. Systematic inventorying and monitoring of agrobiodiversity is the foundational approach for tracking the actual conservation status and dynamics of biodiversity (Maxted et al., 2007; Bruford et al., 2017). A range of semi-standard protocols and complementary methodologies have been developed for potato landraces (De Haan et al., 2016a). The establishment of multilevel baselines covering time- and geotagged genetic, ploidy, species, nutritional, traditional knowledge, red lists and spatial assessments is still a relatively costly effort. Yet, it is essential as in situ conservation in centers of crop origin sustains ongoing evolution and ex situ conservation gaps persist (Ramirez-Villegas et al., 2022). A resource-efficient starting point for conservation monitoring could involve baseline documentation and regular resampling in those regions with the highest complementary landrace diversity.
To maximize the efficiency, genepool coverage and comparability of monitoring efforts, one approach is to set-up a network of complementary sites or observatories. Here we report on an integrated multilevel hotspot analysis for potato landraces in Peru, the largest of its kind to date, designed to inform ongoing and future efforts to build a network of complementary sites or observatories for the systematic monitoring of the conservation status and change dynamics of intraspecific diversity. Like other monitoring efforts for flora, fauna and ecosystems (e.g., Baker et al., 2021; Rhea et al., 2023), we hypothesize that long-term and regular monitoring can provide researchers, policymakers and local communities with valuable information. Here, we provide an exhaustive analysis of possible complementary hotspots for potato landraces in Peru to guide investment in and the gradual expansion of a network and community of practice focused on systematic monitoring. This in turn will further our understanding of the actual conservation status of potato landrace diversity and inform the need to take conservation action.
A 4-step approach was followed to identify complementary conservation sites for systematic potato landrace diversity monitoring (Figure 1). Step 1: inventory all potato landrace collection and occurrence data we could access. Step 2: weighted overlay analysis involving different spatially explicit layers that together combine the best possible conditions for landrace diversity to thrive and evolve. Step 3: complementary reserve analysis to assure the highest level of complementary diversity is captured between conservation sites. Step 4: final prioritization combining steps 1, 2 and 3, adding the results of a key expert survey.
Figure 1 A 4-step approach to prioritize complementary conservation sites for systematic landrace diversity monitoring.
All accessible occurrence data for Peruvian potato landraces were compiled and documented into a single inventory. Collection mission reports, genebank passport data, in depth location-specific catalogues, university theses, varietal adoption impact assessments, participatory mapping baseline assessments and grey literature were included (Table 1). In total 47,722 landrace occurrences from 49 sources were assimilated (see: S1 Supplementary Materials). The following variables (where present) were compiled: (i) landrace name and synonym (ii) location data (community, district, province, and department), (iii) geographic coordinates, (iv) cultivated species, (v) main cultivar group, and (vi) farmer information (converted to unique anonymized IDs). Where possible, missing variables were derived and added. This mostly involved geographic coordinates based on location data or for taxonomic equivalents (Table 2). Non-recoverable variables were simply maintained as missing data.
Due to the oral nature of indigenous Andean languages, there were different spellings of the same landrace across different sources. To avoid synonyms being classed as different landraces, all spellings were standardized with uniform homologs assigned for a total of 1305 terms, predominantly following the panandean alphabet (DIGEIBIR, 2013). The determination and sorting of homologs involved standardizing written spelling in Aymara, Quechua and Spanish based on the principles of indigenous biosystematics (De Haan et al., 2007). Homologs were used in the distinct landrace counts with the original names also being left in the dataset for reference. Apostrophes, numbers, and any other punctuation were removed, as well as landrace records that only had codes and records of modern varieties.
Analysis was done using both coordinates and district (ADM3) locations. Many sources had coordinates along with community names. To define coordinates where they were not provided with the source data, community, district, and province names were matched with those in the Gazeteer (INEI, 2012). Spatial data was converted to UTM Zone 18S projection. After data cleaning, georeferencing and standardization a useable database with 45,884 records remained for analysis. The resulting database covers a 1913 - 2018 timeframe and contains 10,426 distinct landrace names (Table 1; Supplementary Material).
Species data and cultivar group data was determined for 9,550 and 25,806 records respectively. These are key variables to assure taxonomic groups are adequately covered. Several taxonomies exist for the cultivated potato and there is no uniform system among the genebank community (Hawkes, 1990; Ochoa, 1990; Ochoa, 1999; Huamán and Spooner, 2002; Spooner et al., 2007; Ovchinnikova et al., 2011). We used the taxonomy of (Ochoa, 1990; Ochoa, 1999) which is also used by the CIP genebank and reclassified records accordingly where possible. The cultivar group is a taxon in between the landrace and species level, recognizing three basic taxa: (i) floury landraces, (ii) bitter landraces, (iii) modern varieties. The later was not included in this study as we only considered landraces.
Multiple complementary spatially explicit data sources and key indicators were used as an input for analysis to determine landrace hotspots. A key layer involves the large national dataset of potato landrace collection and occurrence data, including species richness and cultivar groups derived from Step 1. Additional layers used for the spatially explicit weighted overlay analysis included data on: (i) crop wild relative concurrence, (ii) area cultivated with landraces, (iii) ethnolinguistic diversity, and (iv) ecogeographical diversity. The 6 key variables, each treated as a layer, were combined as rasters in Arcmap 10.7 and classified using the Reclassify tool to be combined in the weighted overlay analysis (Figure 1). The relative weight given to each indicator is also shown in Figure 1.
Areas of high landrace richness were evaluated at the district (ADM 3) and raster (25km cell) level. The district is a subunit of the province level, which in turn is a subunit of a department or region. Most landrace registers were originally collected at the community or village (district) level. A total of 591 Andean districts where potato landraces are grown were included in the assessment. The complete dataset for this study can be acceded using the following link: https://doi.org/10.21223/68RQFJ. Sampling intensity was identified using the point to raster tool in ArcMap 10.7 via the count function. Landrace richness was determined using the Richness point to grid tool in DIVA GIS version 7.5 (Hijmans et al., 2012). A simple point to grid procedure was used and data was exported as an ASCII file to be processed in ArcMap 10.7.
Based on the taxonomy of Ochoa (1990, 1999) a range of 8 species were examined, involving: (i) Solanum goniocalyx, (ii) S. stenotomum, (iii) S. phureja, (iv) S. ajanhuiri, (v) S. chaucha, (vi) S. juzepczukii, (vii) S. tuberosum subsp. andigena, and (viii) S. curtilobum (Table 2). All these species have landraces populations in Peru, including diploids (2n=2x=24), triploids (2n=3x=36), tetraploids (2n=4x=48) and pentaploids (2n=5x=60). The subspecies S. tuberosum subsp. tuberosum was not included as true landrace populations are restricted to central and southern Chile.
Crop wild relatives (CWR) are defined in Maxted et al. (2006) as wild plant taxa that have an indirect use derived from their relatively close genetic relationship to a crop. Geneflow between the potato CWR and landraces has been well documented and can potentially contribute to ongoing evolution (Johns and Keen, 1986; Jackson and Hanneman, 1999; Scurrah et al., 2008). Location data for the closest wild relatives of the potato - sorted for primary, secondary and tertiary gene pool status - were taken from Castañeda-Álvarez et al. (2015) and Särkinen et al., (2015) (available at: https://figshare.com/articles/dataset/Gap_analysis_potatoes_occurrences/1284187a ). This compiled database contains location data from multiple repositories, including EURISCO, GRIN and CIP. Concurrent distribution of these species is essential as an enabler of geneflow and possible evolutionary processes. The analysis included 3, 32 and 13 species of the primary, secondary and tertiary genepools respectively which have known occurrence in Peru (De Haan and Rodriguez, 2016). In total, 43 out of the 48 species are endemic to Peru (Spooner et al., 2014).
This indicator is identified in Brown and Brubaker (2001) as an indicator for sustainable management of plant genetic resources. The greater the coverage of landraces, the more likely that endogenous management practices and evolutionary processes are in place. Data was taken from the 2012 Agricultural census (INEI, 2012) and filtered for native and bitter potatoes. This resulted in 295,829 data points for native potato cultivation area: 266,268 and 29,561 for floury and bitter landraces respectively. Districts cultivating less than 1ha of potatoes were discarded due to the possibility of outliers.
The potato in Peru is grown in a diverse array of mountain environments and cropping systems (Zimmerer, 1991a). Crops subject to more varying environmental factors potentially have a greater adaptive genetic diversity (Brown and Brubaker, 2001). The SelecVar tool (Parra-Quijano, 2020) and literature search were used to determine the following bioclimatic variables suitable for potato for ecogeographic land characterization: (i) mean annual temperature, (ii) maximum temperature of hottest month, (iii) minimum temperature of coldest month, (iv) elevation, (v) solar radiation, (vi) longitude. Ecogeographic data was obtained from WorldClim (Fick and Hijmans, 2017). Ecogeographic zones were identified using the ELCMapas tool at 5km cells (Parra-Quijano et al., 2012). These cells were aggregated to 25km cell using the point to grid richness tool in DIVA GIS 7.5 (Hijmans et al., 2012) to identify cells of high ecogeographic diversity.
Ethnolinguistic diversity is a solid proxy of biocultural diversity associated with landrace conservation (Benz et al., 2007; Naino Jika et al., 2017). Traditional potato farmers in the Peruvian Andes speak Quechua, Aymara, Jaqaru and/or Spanish. Biocultural diversity in turn has great predictive power for landrace richness and is therefore a valuable variable to consider when evaluating potential hotspots (Perales et al., 2005; Ureta et al., 2013), with higher proportions of indigenous farmers being associated with greater landrace diversity (Spirito et al., 2022). Geospatial data on the proportion of indigenous languages spoken was taken from agricultural census data and applied exclusively to farmers cultivating potato landraces (INEI, 2012). This was aggregatred to the 25km cells by calculating the percentage of farmers speaking an indigenous language compared to solely Spanish.
A complementary reserve analysis was conducted in grid cells at 25 km². The number of unique landraces per cell was identified using the Reserve Selection tool in DIVA GIS described by Rebelo (Rebelo, 1994). A key feature of the procedure is the use of the principle of complementarity. It ensures that areas chosen for inclusion in a network of monitoring sites that complement each other based on their unique added value (Justus and Sarkar, 2002; Kati et al., 2004). In our in-depth analysis, the principle of complementarity specifically involved identifying those hotspots or complementary conservation sites with the highest proportion of unique landraces not covered in other sites. Standardized vernacular nomenclature was used as a proxy for unique landrace presence based on our current knowledge of the indigenous biosystematics of potato landraces (De Haan et al., 2007). A total of 100 cells covered 89.1% of unique names captured. We used the threshold of 20 cells covering more than 50% of unique landrace names.
An online expert survey was conducted using Microsoft Forms. Confirmation by 14 Peruvian experts in potato landrace diversity without prior knowledge of the results of steps 1, 2 and 3 was used as an additional criterion prior to the final selection of sites. These were senior experts from public genetic resources conservation programs and civil society organizations with cumulative experience of over 400 years. The experts were asked to identify hotspots with the highest unique potato landrace diversity (community to province level).
A final prioritization was done combining the previous steps. The hotspots selected were required to meet two out of three criteria. First, correspond to a cell with a mean overlay score value of 5.6 or higher. Second, be scored in a cell corresponding to the top 20 complementary reserve sites. Third, be ranked as a hotspot by key experts.
The total potato cropping area covers 367,692 ha with 142,429 ha and 9,254 ha dedicated to floury and bitter landraces respectively (Figure 2). The regions with the largest cultivated areas of landraces include Puno, Cusco and Huánuco (Figure 3C). Floury landraces occur in the whole of the country’s highland region from 16.6˚ south in Puno to 4.3˚ degrees south latitude in Piura covering altitudes from 3,200 to 4,300 m (86% of the records fall in this range). In most of Peru, floury landraces concur with modern varieties. Bitter landraces on the other hand have a more restricted distribution range compared to floury landraces, occurring from the central to southern Andes between 10.2˚ and 4.3˚ degrees south latitude with the bulk of the cropping area concentrated in the regions of Cusco and Puno. Bitter landraces occur in a restricted altitudinal belt between 4,000 and 4,300 m (80% of the records fall in this range) with limited concurrence with modern varieties.
Figure 2 Distribution of modern and landrace varieties of potatoes based on agricultural census data 2012.
Figure 3 Combined set of maps used in the study for the overlay analysis considering: (A) potato landrace richness, (B) landrace species richness, (C) potato landrace cultivated area in Ha (including floury and bitter varieties), (D) wild potatoes species richness, (E) percentage of native languages spoken by farmers (different than Spanish), and (F) eco geographic diversity.
Figure 3 shows the results of six different spatial layers considered in the weighted overlay analysis. Clearly the areas richest in potato landraces and cultivated species are concentrated in central and southern Peru (Figures 3A, B). Central Cusco represents a large continuous region rich in landrace diversity. Other regions with large, connected areas with comparatively high levels of landrace richness include Apurimac, Ayacucho, Huancavelica, Junín, Pasco and Huánuco. Areas containing 6 to 7 out of the 8 potato species are not uncommon but spatially scattered. Regions with patchy concentrations of landrace richness are Puno, Lima, Ancash, La Libertad, and Cajamarca.
The wild relatives of the potato are widely distributed throughout the country with many having a restricted distributed range. Certain regions stand out for their CWR species richness (Figure 3D). Notably central Cusco, the highlands of Lima, Ancash, Huánuco and La Libertad where the concurrence of multiple wild relatives with potato landraces can facilitate geneflow and evolutionary processes. The regions with the highest concentration of indigenous languages and associated culture are distributed and concentrated from the region of Huancavelica in central Peru up to Puno bordering with Bolivia and Chile (Figure 3E). The largest cropping area of potato landraces overlaps with regions where indigenous languages are spoken. It is in these regions that the indigenous knowledge system is most intrinsically linked to landrace diversity. On the other hand, the ecogeographic diversity is highest towards the eastern slopes of the Andes where the highlands transition towards the Amazon (Figure 3F). The maximum variation present per grid cell is three ecogeographic zones, with most cells having only one type of zone.
All combined layers were assessed using a scoring system with relative weight factors. All cells with a value of 5.6 (1 standard deviation) or higher are considered key landrace hotspots (Figure 4A). All cells with a value between 3.6 and 5.5 are considered of intermediate interest while cells with values of 3.5 and lower are currently considered of low interest. A total of 94 cells classify as hotspots. These cover a total of 15 single cells and 11 clusters covering 13 highland regions. Clearly, hotspots are scattered throughout the Peruvian Andes with a notable absence in the most northern (Piura, Amazonas) and southwestern (Arequipa, Moquegua, Tacna) regions of Peru. This absence may in part be attributable to sampling gaps, though it is well-known that landrace richness declines towards northern Peru.
Figure 4 (A) Weighted overlay analysis, (B) Complementary reserve analysis produced a grid cell network of 20 areas conserving 52.1% of potato landraces diversity and (C) Expert survey.
The complementary reserve analysis shows that the first 20 cells (25 x 25 km²) conserve 52.1% of the total nomenclatural diversity (see: S2 Supplementary Materials). The number in the cell indicates the sequence in which order cells were selected (Figure 4B). Combined they cover 11 spatially distanced areas: 3 clusters of connected cells and 8 single cells. Together, these areas conserve the maximum diversity of unique landraces within the least space based on unique nomenclature. They cover eight regions: Puno, Cusco, Apurimac, Huancavelica, Junín, Huánuco, Pasco, and La Libertad. 100 cells conserve 89.1% of total diversity of landraces based on nomenclature. The matrix in S2 Supplementary Materials provides an overview of each of the reserves or cells, the unique potato landrace contained within and the combined cumulative of potato landrace diversity that is not present in any of the previously selected reserves.
The expert survey shows a consistent high level of agreement with the weighted overlay and complementary reserve analysis (Figure 4C). Results point to 87 districts (41 connected clusters) from 48 provinces and 14 regions as priority landrace hotspots. Importantly, the expert survey highlights some specific sites within the regions of Arequipa and Amazonas that were not captured in the other analyses. These departments and sites are characterized by known sampling gaps, suggesting they may indeed be underappreciated hotspots. Four districts were noted as hotspots of by three different experts. These were the three districts of Paucará, Yauli and Lircay in the region of Huancavelica and the single district of Comas in the region of Junín.
Based on the weighted overlay analysis and the complementary analyses a total of 13 hotpots emerged (Figure 5). These represent complementary conservation sites with the highest value for systematic in situ diversity conservation and monitoring. Each of the hotspots met at least two of the following criteria: (i) a mean overlay score >5.6, (ii) scored in top 20 of complementarity, (iii) ranked in the expert survey. The sites cover a total of 107 district, 34 provinces and 11 regions (S3 Supplementary Materials). Except for hotspot 9, all others coincide with at least 2 out of 3 of Peru’s CWR from the primary genepool present in their territories: S. candolleanum, S. acaule and/or S. brevicaule. The southernmost hotspots - 1, 5, 6, 7 and 8 - have all 3 species from the potato’s primary crop wild relative genepool present in their territory.
Figure 5 Selected hotspots of potato landrace biodiversity based on the overlay analysis, the complementarity analysis, and the expert survey.
This is the largest continuous complementary conservation site and covers a total of 10,115 km², 7 provinces and 32 districts. This vast landscape contains landrace richness levels of 2,622, and most farmers are primarily Quechua speaking. Well-known landrace groups include maqtillu, boli, kusi, qompis, pitikiña. Historically, it has been one of the best documented sites because of early collection missions, international research, and national conservation efforts. Farmer communities between 3,700-4,300 m of altitude tend to maintain unique and high levels of floury and bitter landrace diversity with certain communities belonging to districts like Lares, Pisac, Colquepata and Paucartambo having a reputation as potato diversity stewards.
This complementary conservation site covers a large area of 2,989.1 km² across the border of two regions with 5 provinces and 25 districts. The site is characterized by highly commercial potato production on flat land combined with diverse landraces being grown in the upper belts in so-called chaqru mixtures in high-altitude fallowing systems. Landrace richness levels in the hotspot are 484. Well-known landrace groups include huayro, amarilla, camotillo, winku, duraznillo. Farmers in the site are both Quechua and Spanish speaking. Specific communities belonging to districts like Comas, Quilcas Pasos and Huasahuasi have a reputation as potato diversity stewards.
This relatively isolated complementary conservation site is characterized by small-scale mixed subsistence and market-oriented potato cropping systems. The site covers 1,730 km², 4 provinces, 8 districts and includes the ethically unique Chopcca group. Landrace richness is recorded at 525 in the hotspot. Potato farmers primarily speak Quechua and well-known landrace groups include puqya, suytu, manua, gaspar and pasña.
This is the second largest complementary site covering 4,606.6 km², 6 provinces and 21 districts. This is the heartland of diploid yellow potato landraces belonging to S. goniocalyx and the unique ancestral practice of anaerobic fermentation of tubers into tocosh. Landrace richness in the hotspot is 1,051. Well-known landrace groups, often grown in so-called wachuy mixtures, include walas, tumbay, pishgosh, warmi, coleto. Farmer speak a distinct Quechua dialect with most of the population being bilingual. Wild potatoes from the primary genepool with very large tubers occur in this region (S. ambosinum according to Ochoa, 1999, reclassified as S. candolleanum by Spooner et al., 2014).
These two sites are each comparatively small and show striking differences. The North Apurimac site cover 683 km², 2 provinces and 2 districts. It represents a well-connected commercial potato production site where both landraces and modern varieties are produced. The Southern Apurimac site is largely subsistence oriented with land dedicated to landraces. It covers 676 km², 1 province and 2 districts that have recently been affected by mining. Potato farmers in both sites are primarily Quechua speaking. Between the two sites, landrace richness is 306 for hotspot 5 and 157 in hotspot 6. Well-known landrace groups from the North and South site include qeqorani, putis, huancaina, millco and wallata, susu, linli, tikachi, isakaña respectively. The so-called waña group is common in both sites and used for freeze-drying chuño.
These two sites are the most southern hotspots and unique as they are in what is frequently considered the birthplaceof potato domestication. Also, both sites are characterized by considerable diversity of bitter landraces belonging to the species S. ajanhuiri, S. juzepczukii and S. curtilobum. The North Titicaca Lake site covers 120.3 km² (1 province, 2 district) while the South Titicaca Lake site covers 239.8 km² (1 province, 5 districts). Aymara is the primary language of farmers and cross border seed exchange with Bolivia is common. Between the two sites, landrace richness is 210 for hotspot 7 and 168 in hotspot 8. Well-known landrace groups include imilla, piñaza, keta, ruki, locka.
These two sites are the most northern hotspots with a relatively small surface area of 515.9 and 338.2 km² each. They are relatively isolated – particularly the Southeast Libertad site – and cover 1 district in a separate province. This secluded location may in part have contributed to the relatively high levels of diversity grown in mixtures known as papa embrosada. Farmers in these sites do not speak an indigenous Andean language and this is reflected in the predominantly Spanish varietal nomenclature. Landrace richness is 134 for hotspot 9 and 156 in hotspot 10. Well-known landrace groups from the Central and Southeast site include lampina, huevo de indio, ñata, bretaña and jovera, escalera, kaywa, tarantina respectively.
This intermediate size hotspot of 2,878.3 km² comprises the most northern point where the sporadically distributed bitter landraces are still grown. The site covers four districts, each belonging to a different province, with a regional reputation as tuber seed sources for landraces. Farmers are predominantly Spanish speaking. Landrace richness is 430. Well-known landrace groups from this complementary site include chaulina, galleta, camotillo, huayro.
This complementary site is relatively isolated and well-known in the Ayacucho region for its high levels of potato landrace diversity. The site covers 310.0 km² with only the district of Tambo in the province of La Mar qualifying as a hotspot in our analysis. Potato farmers are predominantly Quechua speaking. Landrace richness is 153. Well-known landrace groups from this complementary site include wira pasña, llumchuy waqachi, millqus, sisa, waña.
This is the most western hotspot and in a part of the Lima highlands where small-scale terrace agriculture persists. The site covers 532.3 km². Farmers in the site are monolingual Spanish speaking though pockets of the Andean Quechua and Jaqaru languages persist in the wider Yauyos province. Districts of Laraos and Carania are qualified as a hotspot in our analysis, but the districts of Miraflores and Huancachi are also well-known for their landrace diversity. Landrace richness is 59. Common landrace groups include bauchi, cuchi ismay, cachito, huayro, piña.
The landrace inventory developed is substantial and the most comprehensive available for potatoes in their Andean center of origin. However, it is not an infallible source for determining complementary conservation sites. Our assessment and results are inevitably affected by data gaps and other limitations. Not all landrace growing areas have adequate occurrence data. This includes some remote and inaccessible regions, but also the source of occurrence data directly affects representativeness. Typically, genebank collection missions have followed road systems and aimed to cover comparatively large distances in the least amount of time. This infrastructure bias is strong even for wild species (Hijmans et al., 2000). Therefore, genebank passport data are often only representative of landrace distribution pattern at the subspecies and ploidy levels, rather than fine-grained ecogeography of intraspecific or landrace level diversity (e.g., Hawkes, 1990; Spooner et al., 2010). To avoid redundancy, genebanks routinely remove accessions of so-called duplicates with the same genetic identity but from different locations if they are already conserved. Consequently, spatially and temporally explicit occurrence data for the same landrace is typically absent in genebank databases. In situ catalogues and multi-household assessment surveys on the other hand are often much more representative of the varietal diversity in a particular locality.
The hotspot bias involves the disproportionate sampling of landraces in perceived hotspots relative to the total distribution area in which intraspecific diversity occurs. This has inevitably affected our results and is evidenced by the fact that 27.7% of all occurrence data corresponds to the Cusco region. The fact that some of Peru’s well-known potato experts like Cesar Vargas and Carlos Ochoa were from Cusco, in combination with the region’s numerous attractions, will likely have contributed to the bias. While potato is undoubtedly among Peru’s most intensely researched crop genetic resources, there are still many localities that are under-represented in terms of their intraspecific landrace diversity. Both the infrastructure and hotspot bias may make identification of hotspots more opaque. Importantly, it also poses the question, how the conservation status of unique landraces in coldspots can be systemically monitored. Crop diversity is more than just the number of landraces in a region, and like wild flora and fauna (Marchese, 2015; Thompson et al., 2021), it is doubtful that a holistic in situ conservation strategy can be exclusively based on the number of taxa present in a particular geography. Therefore, we included a range of additional criteria in our hotspot assessment to provide greater insight to the true level of potato diversity. Given the current shortage of systematic monitoring efforts for in situ landrace diversity in centers of crop origin, it makes sense to initially prioritize complementary hotspots. Monitoring unique and highly endemic varietal diversity outside of hotspots will need complementary approaches in the future.
Another limitation relates to the use of vernacular nomenclature or landrace names as a proxy for a unique landrace. We assume that areas with high levels of recorded landraces indicate higher levels of richness (Brown and Brubaker, 2001; Maxted et al., 2009; Porfiri et al., 2009; Pacicco et al., 2018). This is partially supported by research suggesting that the folk taxonomy of the potato is consistent and robust (Hawkes, 1947; Brush, 1980), and as concluded by Maxted et al. (2009) this is a common practical assumption applied through necessity. Yet, only cosmopolitan and commercial landraces receive names that are consistent at geographical scales beyond the region. And rare or scarce landraces that are of particular interest for conservation tend to receive numerous names (De Haan et al., 2007). Using vernacular names can lead to either under- and over-representation of diversity (Quiros et al., 1990; Monteros-Altamirano et al., 2017). Despite extensive written standardization of landrace names, our inventory consists of over 10,000 different names, when there are estimated to be only 3,000 to 4,000 genetically distinct landraces in Peru (De Haan and Rodriguez, 2016). However, trust in folk taxonomy is our most practical option to initially assess landrace richness at the national and hotspot scale. Over time this assumption can be tested with more time-consuming and costly tools such as genetic fingerprinting, ploidy counts and morphological descriptions.
Despite limitations, our integrated multi-level hotspot analysis, and the resulting identification of thirteen complementary conservation sites for systematic landrace diversity monitoring in Peru, provides a key step forward to anchor in situ conservation and monitoring efforts in locations that jointly provide robust socioecological coverage. Specifically, it will facilitate the targeting of the most representative genepool coverage possible in terms of richness and complementarity of cultivated species and landraces, as well as biocultural drivers that enable evolution. The in situ management of landraces is by definition dynamic, individual landraces will go extinct and new landraces form over time (Veteläinen et al., 2009). Yet a knowledge gap exists concerning the conservation dynamics of farmer-managed landrace diversity, particularly in centers of origin, which affects our ability to generate timeline monitoring series and evidence underlying genetic erosion, cultivar loss and/or incorporation of new cultivars (Veteläinen et al., 2009; FAO, 2019; Khoury et al., 2021). Systematic diversity monitoring requires science-based site selection and prioritization to establish long-term population monitoring, in combination with the application of semi-standardized tools to establish multilevel baselines and assess natural and deleterious population changes.
A range of semi-standard tools currently exists to establish multilevel baselines from the gene to the landscape level. These include (i) genetic fingerprinting, (ii) rapid agrobiodiversity assessments, (iii) farmer field trials and morphological description, (iv) photographic documentation, (v) quantitative red listing, (vi) qualitative red listing, (vii) cataloging, (viii) threat assessment and (ix) participatory cartography (Veteläinen et al., 2009; De Haan et al., 2016a; Dulloo et al., 2017; Poets et al., 2020). Furthermore, participatory approaches have been developed that involve teachers, students and parents from local communities in the documentation of the indigenous knowledge associated with varietal diversity (e.g., Hilares, 2021). Many of these tools can be applied separately yet, their combined use helps to build a holistic assessment of the genetic, varietal, spatial and cultural conservation status of crop diversity.
In addition to sites and shared monitoring tools, an active community of practice is needed to systematically measure and track the landrace diversity in each site. Network approaches, institutional and technological innovations are needed to build an agile knowledge base. Many of the location- specific in situ baseline catalogues used in our analysis are examples of diversity assessments for a single point in time (e.g., Cosio Cuentas et al, 2006; Egúsquiza Bayona et al., 2018; CIP et al, 2021). While these inventories with detailed morphological, genetic and ethnobotanical details offer a solid register, they often lack temporal occurrence data per landrace. To increase the number of observations in space and time, citizen science and crowdsourcing approaches hold great promise to underpin long-term diversity monitoring (Johnston et al., 2022; Suter et al., 2023). An example of such a pilot-level effort is the WikiPapa Platform (www.wikipapa.org). Both action research approaches and participation incentive systems for custodian farmer organizations, civil society organizations, schools, students, teachers, and parents can contribute to intergenerational conservation knowledge exchange and raise awareness of the true value of agrobiodiversity conservation and use.
The datasets used in this study can be found in the following online repositories: https://doi.org/10.21223/68RQFJ and https://doi.org/10.21223/1RBTIV.
SH designed the study. SH, TD and HJ performed collections of landrace occurrence and observation data. SH, TD and HJ cleaned the dataset. TD and HJ performed the spatial analysis. SH, TD, NM and HJ performed analyses and interpreted results. SH and NM supervised the study and acquired funding. SH, TD, HJ and NM wrote the manuscript collaboratively. All authors approved the final manuscript.
This work was financially supported by the CGIAR Research Program on Roots, Tubers and Bananas and the CGIAR Initiative on Nature-Positive Solutions (Nature+).
We thank Alejandra Arce, Maria Fernanda Diaz Guevara and Franklin Plasencia Amaya for their support digitizing part of the information. We also want to acknowledge the essential contribution of different potato experts in Peru for sharing with us their knowledge and expertise.
The authors declare that the research was conducted in the absence of any commercial or financial relationships that could be construed as a potential conflict of interest.
All claims expressed in this article are solely those of the authors and do not necessarily represent those of their affiliated organizations, or those of the publisher, the editors and the reviewers. Any product that may be evaluated in this article, or claim that may be made by its manufacturer, is not guaranteed or endorsed by the publisher.
The Supplementary Material for this article can be found online at: https://www.frontiersin.org/articles/10.3389/fcosc.2023.1130138/full#supplementary-material
Antiporta D. A., Smeeth L., Gilman R. H., Miranda J. J. (2016). Length of urban residence and obesity among within-country rural-to-urban Andean migrants. Public Health Nutr. 19, 1270–1278.
Arce A., de Haan S., Juarez H., Burra D. D., Plasencia F., Ccanto R., et al. (2019). The spatial-temporal dynamics of potato agrobiodiversity in the highlands of central Peru: A case study of smallholder management across farming landscapes. Land. 8, 169. doi: 10.3390/land8110169.
Baker T. R., Vicuña E., Banda-R, Del Castillo D., Farfan-Rios W., Lawson I. T., et al. (2021). From plots to policy: How to ensure long-term forest plot data supports environmental management in intact tropical forest landscapes. Plants People Planet. 3, 229–237.
Bedoya-Perales N. S., Pumi G., Talaminia E., Domingos A. (2020). The quinoa boom in Peru: Will land competition threaten sustainability in one of the cradles of agriculture? Land Use Policy. 79, 475–480.
Benz B., Perales H., Brush S. (2007). Tzeltal and Tzotzil farmer knowledge and maize diversity in Chiapas, Mexico. Curr. Anthropology. 48, 289–300.
Brown A. H. D., Brubaker C. L. (2001). “Indicators for sustainable management of plant genetic resources: How well are we doing?,” in Managing plant genetic diversity. Eds. Engels J., Brown A. H. D., Jackson M. T., Rao V. R. (Wallingford, UK: CABI), 249–262.
Bruford M. W., Davies N., Dulloo M. E., Faith D. P., Walters M. (2017). “Monitoring changes in genetic diversity,”. Eds. Walters M., Scholes R. J. The GEO Handbook on Biodiversity Observation Networks (Cham, Switzerland: The GEO Handbook on Biodiversity Observation Networks: Springer), 107–128.
Brush S. B. (1980). “Potato taxonomies in Andean agriculture,” in Indigenous knowledge systems and development, vol. 37–48. Eds. Brokensha D. W., Warren D. M., Werne. O. (Lanham, Maryland: University Press of America).
Brush S. B., Carney H. J., Huaman Z. (1981). Dynamics of Andean potato agriculture. Economic Bot. 35, 70–88.
Camacho Villa T. C., Maxted N., Scholten M. A., Ford-Lloyd B. V. (2005). Defining and identifying crop landraces. Plant Genet. Resources: Characterization Utilization. 3, 373–384.
Castañeda-Álvarez N., de Haan S., Juarez H., Khoury C. K., Achicanoy H. A., Sosa C. C., et al. (2015). Ex situ conservation priorities for the wild relatives of potato (Solanum l. section Petota). PloS One 10, 6. doi: 10.1371/journal.pone.0129873
Centrum voor Genetische Bronnen Nederland (2020) GCN complete passport data. Available at: https://cgn.websites.wur.nl/Website/downloads/DownloadCnr41.htm (Accessed 20/05/2020).
Choudhury B., Khan M. L., Dayanandan S. (2013). Genetic structure and diversity of indigenous rice (*Oryza sativa*) varieties in the Eastern Himalayan region of northeast India. SpringerPlus. 2, 228. doi: 10.1186/2193-1801-2-228
CIP, Lima: Centro Internacional de la Papa (CIP), Grupo Yanapai, Gobierno Regional de Huancavelica (2021). Catálogo línea de base de la diversidad de papa nativa del microcentro Yauli - Paucará, Huancavelica, Perú (Lima, Perú: Asociación de Guardianes de Papa Nativa del Centro del Perú (AGUAPAN).
Cook O. F. (1925). Peru As a center of domestication. tracing the origin of civilization through the domesticated plants (Continued from the February issue). J. Heredity 16, 95–110.
Cosio Cuentas P., Álvarez Mozo S., Ponce Rosaso J., Camargo Chávez J. (2006). Variabilidad de papas nativas en seis comunidades de Calca y Urubamba - Cusco catalgoue (Cusco: Asociación ARARIWA).
De Haan S., Bonierbale M., Ghislain M., Núñez J., Trujillo G. (2007). Indigenous biosystematics of Andean potatoes: Folk taxonomy, descriptors, and nomenclature. Acta Horticulturae. 745, 89–134.
De Haan S., Juarez H. (2010). Land use and potato genetic resources in Huancavelica, central Peru. J. Land Use Sci. 5, 3. doi: 10.1080/1747423X.2010.500681
De Haan S., Núñez J., Bonierbale M., Ghislain M. (2010). Multilevel agrobiodiversity and conservation of Andean potatoes in central Peru. Mountain Res. Dev. 30, 222–231.
De Haan S., Polreich S., Rodriguez F., Juarez H., Ccanto R., Alvarez C., et al. (2016). “A long-term systematic monitoring framework for on-farm conserved potato landrace diversity,” in Enhancing crop genepool use: Capturing wild relative and landrace diversity for crop improvement. Eds. Maxted N., Dulloo E., Ford-Lloyd B. V. (Wallingford, UK: CABI International), 289–296.
De Haan S., Rodriguez F. (2016). “Chapter 1 - potato origin and production” in advances in potato chemistry and technology. 2nd ed. (Oxford, UK: Elsevier Inc), 1–32.
Denham T., Barton H., Castillo C., Crowther A., Dotte-Sarout E., Florin S. A., et al. (2020). The domestication syndrome in vegetatively propagated field crops. Ann. Botany. 125, 4. doi: 10.1093/aob/mcz212
DIGEIBIR (2013). Documento nacional de lenguas originarias del Perú (Lima: Dirección General de Educación Intercultural Bilingüe y Rural (DIGEIBIR).
Dreisigacker S., Zhang P., Warburton M. L., Skovmand B., Hoisington D., Melchinger A. E. (2005). Genetic diversity among and within CIMMYT wheat landrace accessions investigated with SSRs and implications for plant genetic resources management. Crop Sci. 45, 653–661.
Dulloo E. (2010). Ex situ and in situ conservation of agricultural biodiversity: major advances and research needs. Notulae Botanicae Horti Agrobotanici Cluj-Napoca 38, 114–122.
Dulloo M. E., Rege J. E. O., Ramirez M., Drucker A. G., Padulosi S., Maxted N., et al. (2017). “Conserving agricultural biodiversity for use in sustainable food systems,” in Mainstreaming agrobiodiversity in sustainable food systems: scientific foundations for an agrobiodiversity index (Rome: Bioversity International), 103–140.
Egúsquiza Bayona R., Amézquita Amézquita W., Alcalde Ludeña S. (2018). Nativas cultivads en Chicla y San Mateo, Huarochirí - Lima (Lima: Universidad Nacional Agraria La Molina & Empresa Minera Los Quenuales).
Ellen R., Simon Platten S. (2011). The social life of seeds: the role of networks of relationships in the dispersal and cultural selection of plant germplasm. J. R. Anthropological Institute 17, 563–584.
Esquinas-Alcazar J. T. (1993). “Plant genetic resources,” in Plant breeding: Principles and prospects. Eds. Hayward M. D., Bosemark Romagosa N. O. L. (London, UK: Chapman & Hall), 33–51.
Ezekiel R., Singh N., Sharma S., Kaur A. (2013). Beneficial phytochemicals in potato — a review. Food Res. Int. 502. doi: 10.1016/j.foodres.2011.04.025
FAO (2019). Voluntary guidelines for the conservation and sustainable use of farmers’ Varieties/Landraces (Rome: Food and Agriculture Organization of the United Nations (FAO).
Fick S. E., Hijmans R. J. (2017). WorldClim 2: new 1km spatial resolution climate surfaces for global land areas. Int. J. Climatology 37, 4302–4315.
Frankel O. H., Bennett E. (1970). Genetic resources in plants – their exploration and conservation (Oxford, UK: Blackwell).
Gibson R. W. (2009). A review of perceptual distinctiveness in landraces including an analysis of how its roles have been overlooked in plant breeding for low-input farming systems. Economic Bot. 63, 242–255.
Genesys (2021) Available at: https://www.genesys-pgr.org/ (Accessed 3rd March 2021).
Germplasm Resources Information Network (GRIN) (2020) “solanum tuberosum germplasm" provided by Marty Reisinger. (Accessed 17/7/2020).
Hardigan M. A., Parker F., Laimbeer E., Newton L., Crisovan E., Hamilton J. P., et al. (2017). Genome diversity of tuber-bearing solanum uncovers complex evolutionary history and targets of domestication in the cultivated potato. Proc. Natl. Acad. Sci. United States America .114, 46. doi: 10.1073/pnas.1714380114
Hawkes J. G. (1944). “Potato collecting expeditions in Mexico and South America II,” in Systematic classification of the collections (Cambridge , UK: Imperial Bureau of Plant Breeding and Genetics).
Hawkes J. G. (1947). On the origin and meaning of South American Indian potato names. Bot. J. Linn. Soc LIII 50, 205–250.
Hawkes J. G. (1973). Potato genetic erosion – preliminary report (Lima, Peru: International Potato Center).
Hawkes J. G. (1990). The potato: Evolution, biodiversity & genetic resources (London: Belhaven Press).
Hawkes J. G. (1997). Ecogeographic dataset for solanum taxa (Birmingham , UK: University of Birmingham).
Hijmans R. (2003). The effect of climate change on global potato production. Am. J. potato Res. 80, 271–279.
Hijmans R. J., Garrett K. A., Huaman Z., Zhang D. P., Schreuder M., Bonierbale M. (2000). Assessing the geographic representativeness of genebank collections: The case of Bolivian wild potatoes. Conserv. Biol. 14, 6. doi: 10.1046/j.1523-1739.2000.98543.x.
Hijmans R. J., Guarino L., Mathur P. (2012). DIVA-GIS version 7.5 manual (Lima: International Potato Center).
Hilares J. O. (2021). Guía para la formulación de aprendizajes basados en proyectos: experiencia educativa “Las papitas de mi comunidad” (Cusco, Peru: Cusco: Asociación Civil Fundación HoPe Holanda).
Huamán Z., Spooner D. M. (2002). Reclassification of landrace populations of cultivated potatoes (Solanum sect. petota). Am. J. Bot. 86, 947–965.
INEI (2012) IV censo nacional agropecuario. Available at: http://censos.inei.gob.pe/Cenagro/redatam/.
International Potato Center (CIP) (2021) Cultivated potato germplasm collection. Available at: https://cipotato.org/genebankcip/potato-cultivated/ (Accessed 08/04/2021).
Jackson S. A., Hanneman R. E. Jr (1999). Crossability between cultivated and wild tuber-and non tuber-bearing solanums. Euphytica. 109, 51–67.
Jackson M. T., Hawkes J. G., Rowe P. R. (1980). An ethnobotanical field study of primitive potato varieties of Peru. Euphytica. 29, 107–113.
Johns T., Keen S. (1986). Ongoing evolution of the potato on the altiplano of western Bolivia. Econ Bot. 40, 409–424.
Johnston A., Matechou E., Dennis E. B. (2022). Outstanding challenges and future directions for biodiversity monitoring using citizen science data. Methods Ecol. Evol. 14, 1. doi: 10.1111/2041-210X.13834
Juarez H., Plasencia F., Polreich S., Scurrah M., Ccanto R., De Haan S. (2017a). Data from: Participatory mapping for long-term monitoring and red listing of cultivated potato populations in Junin (Lima, Peru: Dataverse). doi: 10.21223/P3/DNQ4ED
Juarez H., Plasencia F., Polreich S., Arce A., De Haan S. (2017b). Data from: Participatory mapping for long-term monitoring and red listing of cultivated potato populations in Pasco (Peru: Dataverse). doi: 10.21223/P3/VRAXFD
Juarez H., Plasencia F., Polreich S., de Haan S. (2017c). Data from: Participatory mapping for long-term monitoring and red listing of cultivated potato populations in Cusco (Peru: Dataverse). doi: 10.21223/P3/4LWCG4
Juarez H., Plasencia F., Polreich S., Begazo D., De Haan S. (2017d). Data from: Participatory mapping for long-term monitoring and red listing of cultivated potato populations in Apurimac (Lima, Peru: Peru Dataverse). doi: 10.21223/P3/OFZMO9
Juarez H., Plasencia F., Polreich S., Scurrah M., De Haan S. (2017e). Dataset from: Participatory mapping for long-term monitoring and red listing of cultivated potato populations in Huancavelica (Lima, Peru: Dataverse). doi: 10.21223/P3/CVRYSO
Justus J., Sarkar S. (2002). The principle of complementarity in the design of reserve networks to conserve biodiversity: A preliminary history. J. Biosci. 27, 421–435.
Kati V., Devillers P., Dufrênec M., Legakis A., Vokou D., Lebrun P. (2004). Hotspots, complementarity or representativeness? Designing optimal small-scale reserves for biodiversity conservation. Conserv. Biol. 120, 471–480.
Khoury C. K., Brush S. B., Costich D. E., Curry H. A., De Haan S., Engels J. M. M., et al. (2021). Crop genetic erosion: understanding and responding to loss of crop diversity. New Phytologist. 233, 84–118.
Klein J. A., Tucker C. M., Nolin A. W., Hopping K. A., Reid R. S., Steger C., et al. (2019). Catalyzing transformations to sustainability in the world’s mountains. Earth’s Future 7, 547–557.
Lüttringhaus S., Pradel W., Suarez V., Manrique Carpintero N. C., Anglin N. L., Ellis D., et al. (2021). Dynamic guardianship of potato landraces by Andean communities and the genebank of the International Potato Center. CABI Agric. Bioscience 2, 45. doi: 10.1186/s43170-021-00065-4
Marchese C. (2015). Biodiversity hotspots: a shortcut for a more complicated concept. Global Ecol. Conserv. 3, 297–309
Maxted N., Ford-Lloyd B. V., Hawkes J. G. (1997). “Complementary conservation strategies,” in Plant genetic conservation: the in situ approach. Eds. Maxted N., Ford-Lloyd B. V., Hawkes J. G. (London, UK: Chapman & Hall).
Maxted N., Ford-Lloyd B., Jury S., Kell S., Scholten M. (2006). Towards a definition of a crop wild relative. Biodiversity conservation 15, 2673–2685.
Maxted N., Scholten M. A., Stegelitz, Germany, compilers (2007)Methodologies for the creation of national / European inventories. In: Report of a task force on on-farm conservation and management, second meeting (Rome, Italy: Bioversity International) (Accessed 19-20 June 2006).
Maxted N., Veteläinen M., Negri V., Bioversity International (2009). “Landrace inventories: Needs and methodologies,” in European Landraces: On-farm conservation, management and use. Eds. Veteläinen M., Negri V., Maxted N. (Rome, Italy: Bioversity Technical Bulletin15), 45–52.
Meza Valasco C. J. (2011). “Informe de actividades n° 04,” in Internal report from the international potato center CIP (Lima, Peru;: Universidad Nacional de Huancavelica (UNH)).
Monteros A. R. (2011). Potato landraces: description and dynamics in three areas of Ecuador. [PhD thesis.] (Wageningen (Netherlands: Wageningen University).
Monteros-Altamirano A., Buitrón-Bustamante J., Orbe-Vergara K., Cuesta X. (2017). Ecuadorian Potato landraces: Traditional names and genetic identity (Revista Fitotecnia Mexicana), 40. doi: 10.35196/rfm.2017.4.481-489.
Nagel M., Dulloo M. E., Bissessur P., Gavrilenko T., Bamberg J., Ellis D., et al. (2022). Global strategy for the conservation of potato (Bonn, Peru: Global Crop Diversity Trust).
Naino Jika A. K., Dussert Y., Raimond C., Garine E., Luxereau A., Takvorian N., et al. (2017). Unexpected pattern of pearl millet genetic diversity among ethno-linguistic groups in the Lake Chad Basin. Heredity 118, 491–502.
Narouei-Khandan H. A., Shakya S. K., Garrett K. A., Goss E. M., Dufault N. S., Andrade-Piedra J. L., et al. (2020). BLIGHTSIM: A new potato late blight model simulating the response of phytophthora infestans to diurnal temperature and humidity fluctuations in relation to climate change. Pathogens 9, 659. doi: 10.3390/pathogens9080659
Ochoa C. M. (1975). “Potato collecting expeditions in Chile, Bolivia and Peru, and the genetic erosion of indigenous cultivars,” in Crop genetic resources for today and tomorrow. Eds. Frankel O. H., Hawkes J. G. (Cambridge: Cambridge University Press), 167–174.
Oliveira H. R., Tomás D., Silva M., Lopes S., Viegas W., Veloso M. M. (2016). Genetic diversity and population structure in *Vicia faba* l. landraces and wild related species assessed by nuclear SSRs. PloS One 11, 5. doi: 10.1371/journal.pone.0154801
Ovchinnikova A., Krylova E., Gavrilenko T., Smekalova T., Zhuk M., Knapp S., et al. (2011). Taxonomy of cultivated potatoes (Solanum section petota: Solanaceae). Botanical J. Linn. Soc. 165, 107–155.
Pacheco M. A., Gutierrez L. R., Salcedo O. F. (2023). Paucartambo - Cusco: Centro de Origen, Domesticación y Variabilidad de las Papas Cultivadas del Perú. Municipalidad Provincial del Cusco, Cusco.
Pacicco L., Bodesmo M., Torricelli R., Negri V. (2018). A methodological approach to identify agro-biodiversity hotspots for priority in situ conservation of plant genetic resources. PloS One 13, 6. doi: 10.1371/journal.pone.0197709
Parra-Quijano M. (2020) CAPFITOGEN 3: A toolbox for the conservation and promotion of the use of agricultural biodiversity (Bogotá, Colombia). Available at: http://www.capfitogen.net/ (Accessed 23rd August 2021).
Parra-Quijano M., Iriondo J. M., Torres M. E. (2012). Ecogeographical land characterization maps as a tool for assessing plant adaptation and their implications in agrobiodiversity studies. Genet. Resour. Crop Evol. 59, 2. doi: 10.1007/s10722-011-9676-7
Parra-Rondinel F., Casas A., Begazo D., Paco A., Márquez E., Cruz A., et al. (2021). Natural and cultural processes influencing gene flow among wild (atoq papa), weedy (araq papa and k’ipa papa), and crop potatoes in the Andean region of southern Peru. Front. Ecol. Evol. 9. doi: 10.3389/fevo.2021.617969
Perales H., Benz B. F., Brush S. B. (2005). Maize diversity and ethnolinguistic diversity in Chiapas, Mexico. Proc. Natl. Acad. Sci. United States America 102, 949–954.
Pérez Baca L. (1996). Crianza de la papa en Paucartambo -Qosqo (Cusco: Centro de Servicios Agropecuarios (CESA).
Poets A., Silverstein K., Pardey P., Hearne S., Stevenson J. (2020). “DNA Fingerprinting for crop varietal identification: Fit-for-Purpose protocols, their costs and analytical implications,” in International maize and wheat improvement center (CIMMYT), standing panel on impact assessment (SPIA)(Rome: CIMMYT and SPIA).
Polreich S., Juarez H., Plasencia F., Suarez V., Lüttringhaus S., Pradel W., et al. (2018). Data from: Analysis of socioeconomics, geospatial and agroecological indicators for potato diversity management at family level of the in-situ monitoring hotspot of Huancavelica, Peru) in 2013 and 2017 (Lima, Peru: International Potato Center (CIP). doi: 10.21223/P3/A93MJV
Porfiri O., Costanza M., Negri V. (2009). “Landrace inventories in Italy and the Lazio region case study,” in European Landraces on-farm conservation, management and use. Eds. Veteläinen M., Negri V., Maxted N. (Rome, Italy: Biodiversity International).
Poveda G., Espinoza J. C., Zuluaga. M. D., Solman S. A., Garreaud R., Van Oevelen P. J. (2020). High impact weather events in the Andes. Front. Earth Sci. 8. doi: 10.3389/feart.2020.00162
Pradel W., Hareau G., Quintanilla L., Suarez V. (2018). Data from: Adoption and diffusion of potato varieties in Peru (Lima, Peru: International Potato Center (CIP)). doi: 10.21223/P3/MDNLI6
Quiros C. F., Brush S. B., Douches D. S., Zimmerer K. S., Huestis G. (1990). ‘Biochemical and folk assessment of variability of Andean cultivated potatoes’. Econ. Bot. 44, 254–266.
Quiroz R., Ramírez D. A., Kroschel J., Andrade-Piedra J., Barreda C., Condori B., et al. (2018). Impact of climate change on the potato crop and biodiversity in its center of origin. Open Agric. 3, 273–283.
Rabatel A., Francou B., Jomelli V., Naveau P., Grancher D. (2008). A chronology of the little ice age in the tropical Andes of Bolivia (16°S) and its implications for climate reconstruction. Quaternary Res. 70, 198–212.
Ramirez-Villegas J., Khoury C. K., Achicanoy H., Diaz M. V., Mendez A., Sosa C. C., et al. (2022). State of ex situ conservation of landrace groups of twenty-five major crops. Nat. Plants 8, 491–499.
Raymundo R., Asseng, Robertson R., Petsakos A., Hoogenboom G., Quiroz R., et al. (2018). Climate change impact on global potato production. Eur. J. Agron. 100, 87–98.
Rebelo A. G. (1994). “Iterative selection procedures: centres of endemism and optimal placement of reserves,” in Botanical diversity in southern Africa. Ed. Huntley B. J. (Pretoria: National Botanic Institute), 231–257.
Rhea S., Gubbins N., DelVecchia A. G., Ross M. R. V., Bernhardt E. S. (2023). User-focused evaluation of national ecological observatory network streamflow estimates. Sci. Data 10, 89. doi: 10.1038/s41597-023-01983-w
Pacheco M.A., Gutierrez L.R., Salcedo O.F. (2023) Paucartambo - Cusco: Centro de Origen, Domesticación y Variabilidad de las Papas Cultivadas del Perú. (Cusco: Municipalidad Provincial del Cusco).
Särkinen T., Baden M., Gonzáles P., Cueva M., Giacomin L., Spooner D. M., et al. (2015). Listado anotado de solanum l. (Solanaceae) en el Perú. Revista Peruana Biología,; 22, 1. doi: 10.15381/rpb.v22i1.11121
Scurrah M., Celis Gamboa C., Chumbiauca S., Salas A., Visser R. G. F. (2008). Hybridization between wild and cultivated potato species in the Peruvian Andes and biosafety implications for deployment of GM potatoes. Euphytica. 164, 881–892.
Smith C. T., Bushnell G. H. S., Dobyns H. F., McCorkle T., Murra J. V. (1970). Depopulation of the central Andes in the 16th century. Curr. Anthropology 11, 453–464.
Spirito F., Vieli L., Montalba R. (2022). Advancing towards an understanding of the relationship between culture and agrobiodiversity. A Case study Mapuche territory South. Chile. NJAS: Impact Agric. Life Sci. 94, 1. doi: 10.1080/27685241.2022.2083987
Spooner D. M., Gavrilenko T., Jansky S. H., Ovchinnikova A., Krylova E., Knapp S., et al. (2010). Ecogeography of ploidy variation in cultivated potato (Solanum sect. petota). Am. J. Bot. 97, 2049–2060.
Spooner D. M., Ghislain M., Simon R., Jansky S. H., Gavrilenko T. (2014). Systematics, diversity, genetics, and evolution of wild and cultivated potatoes. Botanical Rev. 80, 283–383.
Spooner D. M., Núñez J., Trujillo G., Del Rosario Herrera M., Guzmán F., Ghislain M. (2007). Extensive simple sequence repeat genotyping of potato landraces support a major reevaluation of their gene pool structure and classification. Proc. Natl. Acad. Sci. 104, 19398–19403.
Suter S., Barrett B., Welden N. (2023). Do biodiversity monitoring citizen science surveys meet the core principles of open science practices? Environ. Monit. Assess. 195, 295. doi: 10.1007/s10661-022-10887-y
Thompson M. S. A., Couce E., Webb T. J., Grace M., Cooper K. M., Schratzberger M. (2021). What’s hot and what’s not: Making sense of biodiversity ‘hotspots’. Global Change Biol. 27, 521–535.
Tiranti B., Negri V. (2007). Selective micro-environmental effects play a role in shaping genetic diversity and structure in a *Phaseolus vulgaris* l. landrace: implications for on-farm conservation. Mol. Ecol. 16, 4942–4955
Ureta C., González-Salazar C., González E. J., Álvarez-Buylla E. R., Martínez-Meyer E. (2013). Environmental and social factors account for Mexican maize richness and distribution: A data mining approach. Agriculture Ecosyst. environment. 179, 25-34. doi: 10.1016/j.agee.2013.06.017
Veteläinen M., Negri V., Maxted N. (2009). European Landraces: On-farm conservation, management and use. Bioversity Tech. Bull. (Rome, Italy) 15, 1–359.
Zabel F., Müller C., Elliott J., Minoli S., Lägermeyr J., Schneider J. M., et al. (2021). Large potential for crop production adaptation depends on available future varieties. Global Change Biol. 27, 16. doi: 10.1111/gcb.15649
Zimmerer K. S. (1991a). The regional biogeography of native potato cultivars in highland Peru. J. Biogeography 18, 165–178.
Keywords: Andes, complementary reserve analysis, genetic resources, GIS, in situ conservation, multilevel indicators, observatories
Citation: Dawson T, Juarez H, Maxted N and de Haan S (2023) Identifying priority sites for the on-farm conservation of landraces and systematic diversity monitoring through an integrated multi-level hotspot analysis: the case of potatoes in Peru. Front. Conserv. Sci. 4:1130138. doi: 10.3389/fcosc.2023.1130138
Received: 22 December 2022; Accepted: 29 March 2023;
Published: 08 May 2023.
Edited by:
Jesus V. Jorrin Novo, University of Cordoba, SpainReviewed by:
Alex McAlvay, New York Botanical Garden, United StatesCopyright © 2023 Dawson, Juarez, Maxted and de Haan. This is an open-access article distributed under the terms of the Creative Commons Attribution License (CC BY). The use, distribution or reproduction in other forums is permitted, provided the original author(s) and the copyright owner(s) are credited and that the original publication in this journal is cited, in accordance with accepted academic practice. No use, distribution or reproduction is permitted which does not comply with these terms.
*Correspondence: Stef de Haan, s.dehaan@cgiar.org
Disclaimer: All claims expressed in this article are solely those of the authors and do not necessarily represent those of their affiliated organizations, or those of the publisher, the editors and the reviewers. Any product that may be evaluated in this article or claim that may be made by its manufacturer is not guaranteed or endorsed by the publisher.
Research integrity at Frontiers
Learn more about the work of our research integrity team to safeguard the quality of each article we publish.