- 1Institute of Ecological Conservation and Restoration, Chinese Academy of Forestry, Beijing, China
- 2Ecology and Nature Conservation Institute, Chinese Academy of Forestry, Beijing, China
- 3Key Laboratory of Biodiversity Conservation of National Forestry and Grassland Administration, Beijing, China
- 4Changqing National Nature Reserve, Hanzhong, China
Similar species may co-occur in sympatry because of the partitioning of habitat use and resources at different spatial and temporal scales. Understanding coexistence patterns of species may contribute to further uncovering the underlying coexistence mechanisms, and ultimately benefit the conservation of threatened species. In this study, camera trapping was used to investigate spatial and temporal activity patterns of sympatric giant pandas (Ailuropoda melanoleuca) and Asiatic black bears (Ursus thibetanus) in Changqing National Nature Reserve in Qinling Mountains, China. Our study obtained 281 independent detections of giant pandas and 185 of Asiatic black bears during 93,606 camera-trap days from April 2014 to October 2017. We performed occupancy modeling and temporal overlap analyses to examine the spatial-temporal relationships between pandas and bears, and results showed that: (1) giant pandas had higher detection probabilities than Asiatic black bears, while having lower occupancy probabilities; (2) Elevation positively predicted giant panda and negatively predicted Asiatic black bear occupancy, understory vegetation type negatively predicted giant panda occupancy, and distance to nearest settlement positively predicted Asiatic black bear occupancy; (3) giant pandas were more active in spring and winter, while Asiatic black bears were more active in summer, and the two species had low spatial overlap with one another throughout the year; (4) both giant pandas and Asiatic black bears showed mainly diurnal activity patterns, and had high temporal overlap with one another in spring and moderate temporal overlap with one another in autumn. Our results provide detailed information of the spatial and temporal ecology of sympatric giant pandas and Asiatic black bears in the Qinling Mountains of China, which could act as a guide to construct conservation priorities as well as design efficient management programs.
1 Introduction
Understanding the drivers of coexistence between sympatric wildlife is crucial to explaining species and functional diversity (Noor et al., 2017; Pastore et al., 2021). Interspecific interactions play an important role in community ecology, including by explaining how communities are shaped, why some species are members of a community and some are not, and which factors mediate predator densities and predation patterns (Farris et al., 2016). In order to reduce agonistic interactions within communities, species often partition resources along three main niche dimensions (temporal, spatial and resource) to promote coexistence, a process known as niche differentiation (Carvalho and Cardoso, 2020). Knowledge about niche differentiation can contribute to further understanding the underlying coexistence mechanisms, and ultimately benefit the conservation of ecological communities (Farris et al., 2020).
Large mammalian carnivores play an important role in terrestrial landscapes, as their top-down effects can regulate prey populations with consequences spreading across the entire food web (Steinmetz et al., 2021). Nevertheless, most carnivores are neither large nor at the apex of their ecological communities (Li et al., 2022). Bears (Family: Ursidae) are the among the largest of the extant Carnivora comprising only eight extant species in the world (Penteriani and Melletti, 2021). Being opportunistic omnivores, bears appear to be more resistant to environmental disturbances than other large Carnivora that depend on a narrower meat diet, such that loss of bears from a given habitat could be an early sign of significant environmental degradation or heavy poaching (Liu et al., 2009). Bears have come under pressure through encroachment of their habitat, poaching, climate change, and illegal trade in their body parts (Garshelis and Steinmetz, 2020), so much so that the International Union for Conservation of Nature (IUCN) lists six bear species as Vulnerable (https://www.iucnredlist.org/). With the widespread extirpation of apex predators in some ecosystems, bears play an increasingly important role in mediating predator-prey interactions and structuring ecosystems, though some have relatively loose relationships with prey (Zager and Beecham, 2006). The reduction of apex predators can contribute to an increase the abundance of bears in some areas, which can result in intensifying predation pressure and intraguild competition (Ordiz et al., 2013). However, research on the interspecific interactions among sympatric bears has received comparatively little attention (Steinmetz et al., 2013; Ji et al., 2022).
The giant panda (Ailuropoda melanoleuca) is an endemic species to south-central China and is mainly distributed in six isolated mountain ranges in Sichuan, Shaanxi, and Gansu provinces (State Forestry and Grassland Administration, 2021). The past decades have witnessed a remarkable increase in the biological and ecological knowledge on the giant panda, making the panda an excellent candidate for testing the much-touted conservation approach (Ran et al., 2009). The Chinese government has implemented China’s national conservation plan to strictly protect and improve habitat for giant pandas. Many of the known key threats (e.g. poaching, habitat destruction) for the species have been mitigated; populations that have been low for many generations are now beginning to increase (Wei et al., 2015). Giant pandas have occupied a global biodiversity hotspot and have shared a distribution with multiple large mammal species whose ranges are broader and populations are larger, such as wild boar (Sus scrofa), takin (Budorcas taxicolor), and Asiatic black bears (Ursus thibetanus) (Wang et al., 2015; Li et al., 2017). The success of giant panda conservation appears to have benefited other sympatric large mammal species (e.g. takin, wild boar), as their numbers and distribution ranges have increased substantially, resulting in interspecific competition, especially in places where top predators are virtually absent (Liu et al., 2020; Li et al., 2022a; Li et al., 2022b). While the habitat requirements, diet, evolution and behavior of giant pandas have been well studied during the past decades (Wei et al., 2015), the interspecific interactions between giant pandas and other large mammals remains unclear (Wang et al., 2015; Diao et al., 2021).
Morphologically similar sympatric species are expected to have higher niche overlap and competition under conditions of limited resources (Deng et al., 2021). The Asiatic black bear greatly overlaps the range of giant pandas in south-central China. The two species are much alike, as both are solitary carnivores of similar body size, have similar habitat requirements, climb trees and used tree cavities or rock caves as dens, as well as consume bamboo and are mainly active in daytime (Schaller et al., 1985). Different dietary characteristics between giant pandas and Asiatic black bears may be a main factor for coexistence of the two species. Although they belong to the order Carnivora, giant pandas have evolved to specialize on bamboo for 99% of their diet, retaining only a simple digestive tract from their carnivore ancestors (Zhao et al., 2013). While Asiatic black bears feed on a wide range of foods and their diet is mainly composed of fruit and green vegetation, they also feed on insects such as bees and ants, and scavenge livestock and wild animals for meat (Koike et al., 2013; Seryodkin, 2015). Unlike Asiatic black bears living in temperate zone, giant pandas do not hibernate and are active throughout the year (Zhang et al., 2015). Asiatic black bears are effective seed dispersers among mammals, and are believed to alter giant panda habitat selection through their foraging on young bamboo shoots (Gong et al., 2006; Takahata et al., 2014). Aside from a difference in diet, spatial avoidance and temporal partitioning may be two other behavioural mechanisms facilitating coexistence between sympatric giant pandas and Asiatic black bears (He et al., 2018). However, research on coexistence patterns between these two niche dimensions is scarce, largely because their secretive behavior, low densities, solitary nature and dense forest habitats make them difficult to study.
Understanding mechanisms of coexistence among sympatric wildlife is an important component for the conservation of threatened species (Croose et al., 2019). In this study, we conducted an intensive long-term camera trapping effort to survey the fine-scale spatially and temporally-driven coexistence patterns between giant pandas and Asiatic black bears in Changqing National Nature Reserve in the Qinling Mountains, a region virtually free of top predators (e.g., Panthera pardus was recorded only once during our survey period). Our primary objective was to (i) evaluate habitat variables that explain spatial distribution patterns of the giant panda and Asiatic black bear, (ii) describe daily, seasonal and annual activity patterns of the two species, and (iii) quantify spatial and temporal overlap between the two species. The results describe possible coexistence mechanisms between giant pandas and Asiatic black bears, which may contribute to the development of effective conservation and management strategies for forest communities in this mountainous region.
2 Materials and methods
2.1 Study area
The Changqing National Nature Reserve (107°25′to 107°45′ E, 33°26′to 33°43′ N) is located on the southern slopes of the Qinling mountains in Yangxian county, central China (Figure 1). The reserve was established in 1994 and was further upgraded to a national park in 2017, primarily for conservation of the giant panda and other sympatric endangered species. The reserve covers an area of approximately 299 km2, with an altitude range from 800 to 3,000 m. Mean annual temperature is 7°C and mean annual rainfall is 814 mm in the study area. Based on the local climate characteristics, we divided the year into four seasons (spring: Mar-May; summer: Jun to Aug; Autumn: Sep-Oct; winter: Dec to Feb) (Ren et al., 2002). Vegetation varies with elevation. Deciduous broadleaf forest is mainly found at lower elevations, mixed broadleaf-conifer forest is found at mid-elevations, and coniferous forest interspersed with subalpine shrubs and meadows is found at higher elevations (Ren et al., 2002). Although there is a history of human disturbance in the region, currently, the reserve is under strict protection, no residents live within reserve, the habitats are nearly pristine, and the influence of human activity is negligible. The diverse habitats support a large number of wildlife. Of all mammal species present, 2 were evaluated as Endangered by the IUCN RedList, 6 as Vulnerable and 4 as Near Threatened (He et al., 2016; Li et al., 2020).The fourth national survey from 2011 to 2014 estimated that there were approximately 345 wild giant pandas residing in the Qinling mountains, and 57 wild giant pandas inhabiting the Changqing National Nature Reserve (State Forestry and Grassland Administration, 2021).
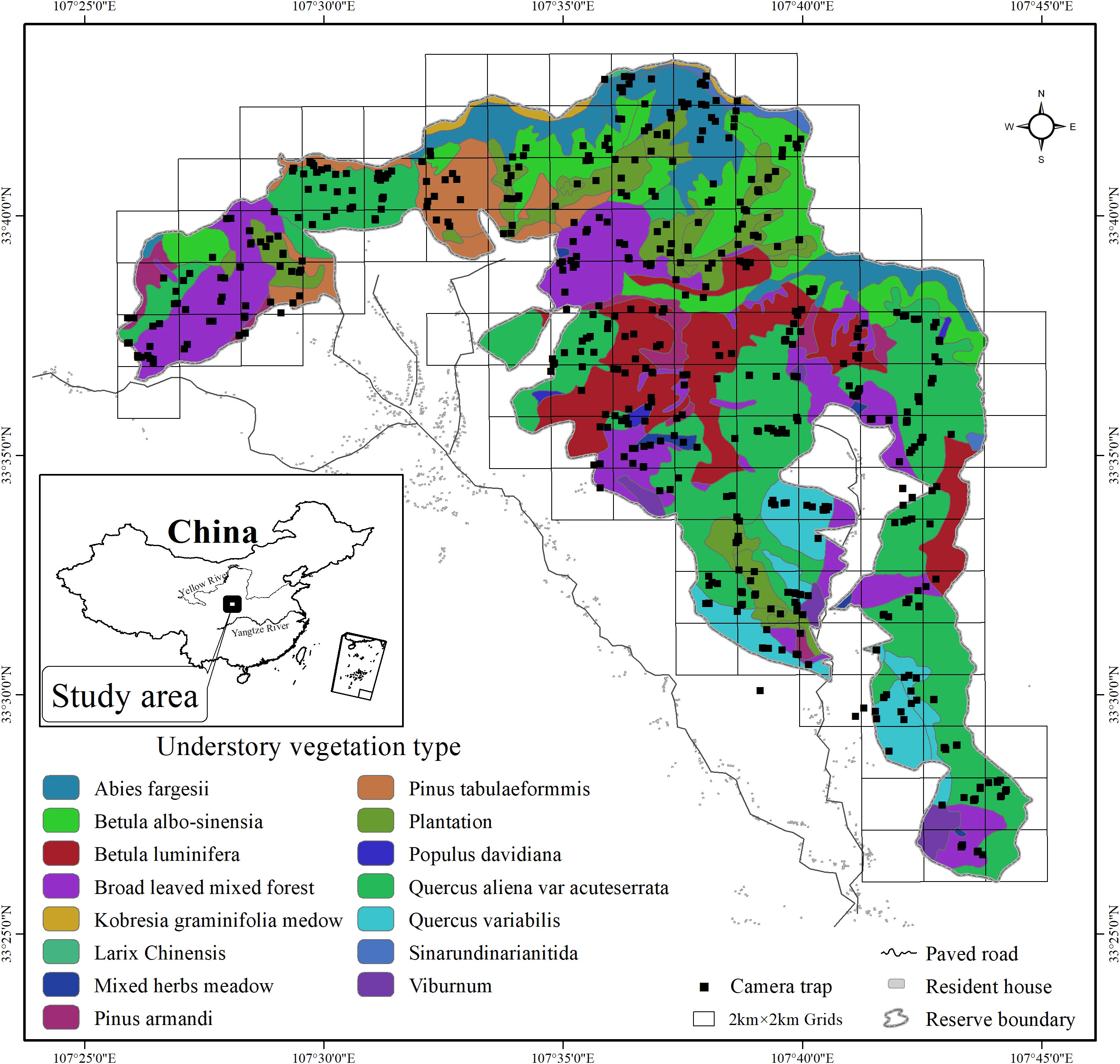
Figure 1 Locations of camera trap sites in 4 km2 grids and understory vegetation types in Changqing National Nature Reserve. Inset map shows the study area location in central China.
2.2 Camera trapping
We deployed infrared-triggered cameras (Ltl-6210; Shenzhen creation) to systematically survey mammals in the Changqing National Nature Reserve continuously from April 2014 to October 2017, covering all four seasons. We created 4 km2 grids (total of 118 grid cells; Figure 1) as potential sampling grids in the study area. Harsh terrain allowed us to sample only 90 grids (76% of total grids). Each grid was divided into four smaller cells (cell size 1 km2). For each grid, we placed one infrared camera in one of the four 1 km2 cells for approximately 4 to 6 months, and then considered whether to relocate to another 1 km2 cell within the same 4 km2 grid according to the detection rate of mammals.
During any single survey period, we positioned the cameras more than 300 m apart from the nearest neighboring camera to allow for spatial independence. Cameras were placed on trees 50-80 cm above ground level and in suitable habitat with mammal signs (e.g. feces and footprints) or along trails with little human disturbance within grids, based on field staff experience (Li et al., 2020). Cameras were operated 24 h/day and passive infrared sensors were set to moderate. When triggered, cameras took two photos and a 15-sec video, with a delay of a 2 min interval between consecutive events, and automatically recorded information on date and time of each trigger event. SD cards and batteries were replaced upon movement of cameras between cells. No bait or lure was used to attract wildlife.
2.3 Data analysis
Photographs and videos were summarized by site, hour, and date at each camera placement site. To ensure independence of photographic capture events, we considered as an “independent detection (n)” to be all photographs of the same species from the same camera trap site recorded in ≤30 min, to limit pseudoreplication bias, and only independent detections were considered for all analyses (Blake et al., 2011). The number of effective camera trap days was calculated from the time the camera was placed in operation to the time the last photograph or video was taken (based on date and time stamp).
Spatial distribution-Occupancy models make use of spatial-temporal replicated data and combine the detections/non-detections of target species to describe maximum likelihood estimation of occupancy (ψ) and detection probability (P) (Moreno-Sosa et al., 2022). We assumed that data from the same site were independent across years. To increase the detection probability for each sampling period and reduce zero inflation in the dataset, we considered whether the target species was detected (1) or not (0) during each 30-day period, resulting in 6 sampling occasions (0–30, 31–60, 61–90, 91–120, 121–150, >150 days). Based on current knowledge of giant panda and Asiatic black bear ecology (Hull et al., 2014; Swaisgood et al., 2016; Garshelis and Steinmetz, 2020; Penteriani and Melletti, 2021), we selected distance to nearest river (Disriv), distance to nearest settlement (Disset), understory vegetation type (Veg), elevation (Ele) and aspect (Asp) as variables in the occupancy models for both species (as predictors of both occupancy and detection probability). In addition, we used understory bamboo distribution (Bamboo), elevation range and Asiatic black bears’ occupancy probability (Bearoccu) as covariates in models for predicting giant panda detection probability. Because Asiatic black bears hibernate in winter, we used season as a covariate for this species only. We divided year into two seasons: cold season (from December to February) and rest of year, ultimately including understory vegetation type, season and giant panda detected or not as covariates for the models predicting Asiatic black bear detection probability. We obtained settlements and understory vegetation type data from Changqing administration. Elevation was derived from a Digital Elevation Model (DEM) with a resolution of 30 m from the Resource and Environment Science Data Center (https://www.resdc.cn/Default.aspx, accessed on 20 October 2021) and aspect data was derived from the elevation layer. All covariates were first normalized to z scores before analysis.We fitted 108 single-species occupancy models with a logit link function to model occupancy and detection as a function of all possible combinations of covariates. The linear model structure for covariates in occupancy models is presented as ~ Psi (.) ~ P (.). For each model set, we ranked candidate models using Akaike’s information criterion (AIC), and only considered the top ranked models (ΔAIC ≤ 2) as the most likely determinant(s) of the species’ occupancy (Lesmeister et al., 2015). Occupancy analyses were performed with the “unmarked” package in R (R Development Core Team).
Spatial overlap analysis-We used seasonal presence/absence data for giant pandas and Asiatic black bears obtained from photographs within the 4 km2 grids to evaluate spatial overlap between giant pandas and Asiatic black bears, Sørensen similarity index (Sij) was calculated using the following formula (Sørensen, 1948; Torretta et al., 2015):
Where aij represented the number of 4 km2 grids with the presence of both giant panda and Asiatic black bear (in any photograph in the same season), and bij and cij were numbers of grids with the presence of only one species. The index ranged from Sij =0, no overlap, to Sij=1, total overlap.
Temporal analysis-We used relative activity indices (RAI) to estimate annual activity patterns of giant pandas and Asiatic black bears (Blake et al., 2014). Each camera’s independent detections were summed for each month and then divided by the number of effective camera trap days for each month, and multiplied by 1000 camera trap days, RAI= (No. of independent detections/No. of effective camera trap days) ∗ 1000 camera trap days.
We used pooled datasets to examine whether giant panda and Asiatic black bear circadian activity patterns were diurnal, nocturnal or crepuscular (i.e., active 1 h before sunrise and 1 h after sunset) (Mella-Méndez et al., 2019). Since time of sunrise and sunset differs according to seasonal changes, we standardized our observations by transforming the clock-recorded time of each detection to the average sunrise and sunset times. Average sunrise and sunset time were at 06:20 and 19:22 in spring, 05:55 and 19:50 in summer, in 06:54 and 18:23 in autumn and 07:42 and 18:07 in winter from April 2014 to October 2017 in Yangxian county, respectively. We calculated selection rations (wi) of used to available time periods for giant pandas and Asiatic black bears with the following calculation (Manly et al., 2002):
Where wi was the selection ratio for period i; oi was the proportion of detections in period i; and pi was the proportion of length in period i relative to the length of all periods. wi >1 indicated that the time period was selectively used; wi ≤1 indicated the time period was avoided (Noor et al., 2017).
Temporal overlap-We measured activity patterns of giant pandas and Asiatic black bears by estimating the coefficient of overlap (Δ), which ranges from 0 (no overlap) to 1 (complete overlap) to measure the extent of overlap between two kernel density estimates (Linkie and Ridout, 2011). We used Δ4 to estimate small sample sizes (n >75), but otherwise used Δ1 (Meredith and Ridout, 2021), and created 95% confidence intervals of overlap by generating 10,000 simulations with nonparametric estimator bootstraps. Temporal overlap analyses were performed in program R using the “overlap” package (Meredith and Ridout, 2021). Finally, we classified the activity overlap between giant pandas and Asiatic black bears as follows: high overlap (Δ>0.75), moderate overlap (0.5<Δ≤0.75) and low overlap (Δ≤0.5) (Monterroso et al., 2014).
3 Results
3.1 General summary
A total of 620 camera sites were surveyed. Of these, 47 failed due to camera malfunction, damage, or loss, resulting in 573 sites deemed suitable for data analysis. We obtained 281 independent detections (out of 363 total detections) of giant pandas and 185 (out of 232) of Asiatic black bears, during 93,606 camera-trap days (surveying each site an average of 162 ± 51 days) from April 2014 to October 2017. Giant pandas were detected at 72 sites (independent detections n=118) in spring, 13 sites (n=15) in summer, 27 sites (n=36) in autumn and 44 sites (n=112) in winter. Asiatic black bears were detected at 31 sites (n=36) in spring, 63 sites (n=95) in summer, 36 sites (n=49) in autumn and only 5 sites (n=5) in early winter.
3.2 Occupancy models
The Asiatic black bear had a higher occupancy probability than the giant panda based on our top model (ΔAIC ≤ 2; Table 1), with average occupancy probability estimates of 0.484 (CI: 0.25-0.80) and 0.276 (CI:0.06-0.65), respectively. Occupancy of giant pandas was positively predicted by elevation (β=0.632 ± 0.279; Figure 2; Table 2), and negatively predicted by understory vegetation type (β=-0.313 ± 0.188). Occupancy of Asiatic black bear was negatively predicted by elevation (β=0.257 ± 0.309) and positively predicted by distance to nearest settlement (β=0.215 ± 0.258). Spatial projection of both species’ occupancy probabilities in Changqing reserve are shown in Figure 3.
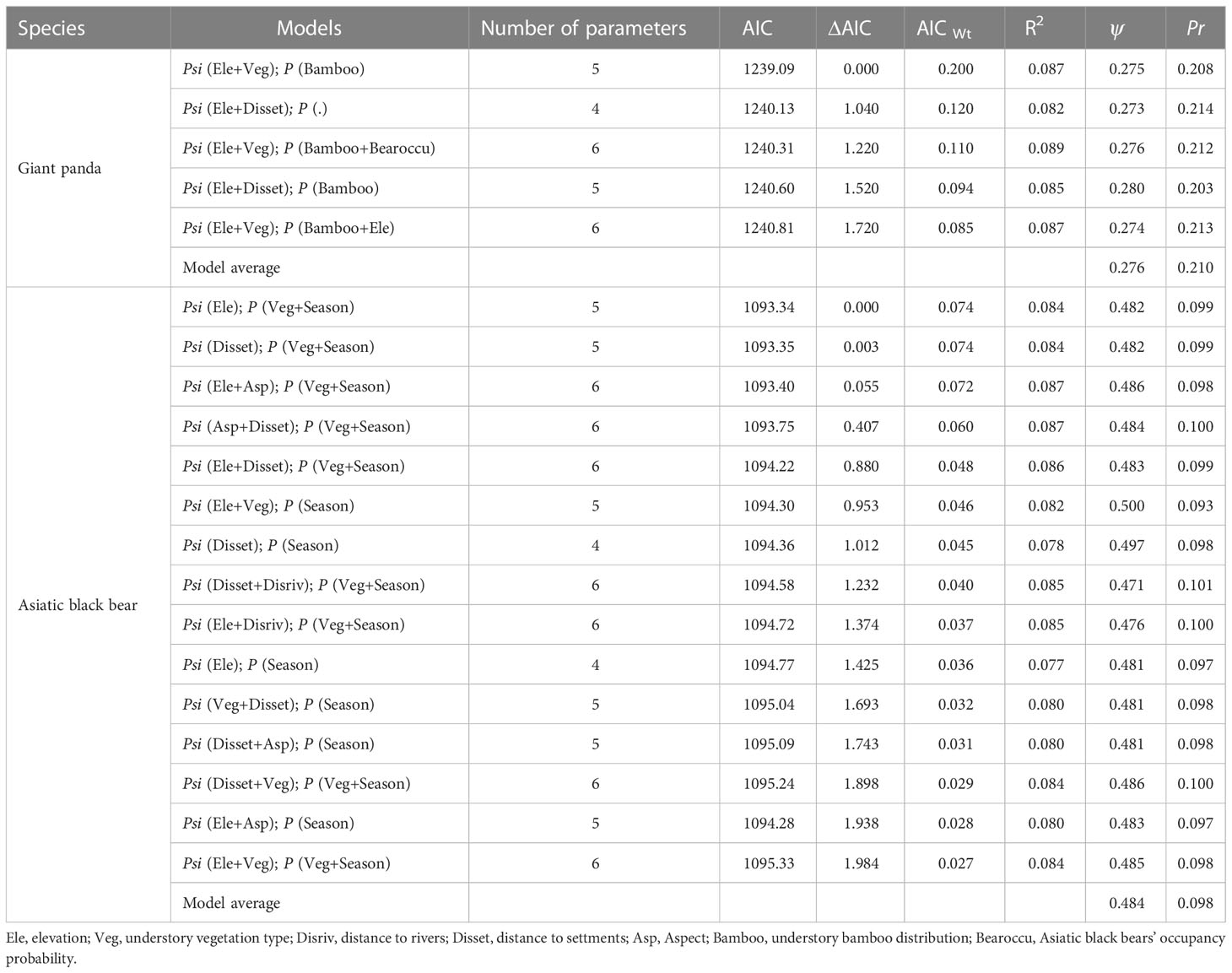
Table 1 Summary of model selection results for giant panda and Asiatic black bear occupancy in Changqing National Nature Reserve in the Qinling mountains, showing estimated occupancy rate and detection probability for the optimal models (ΔAIC ≤ 2).
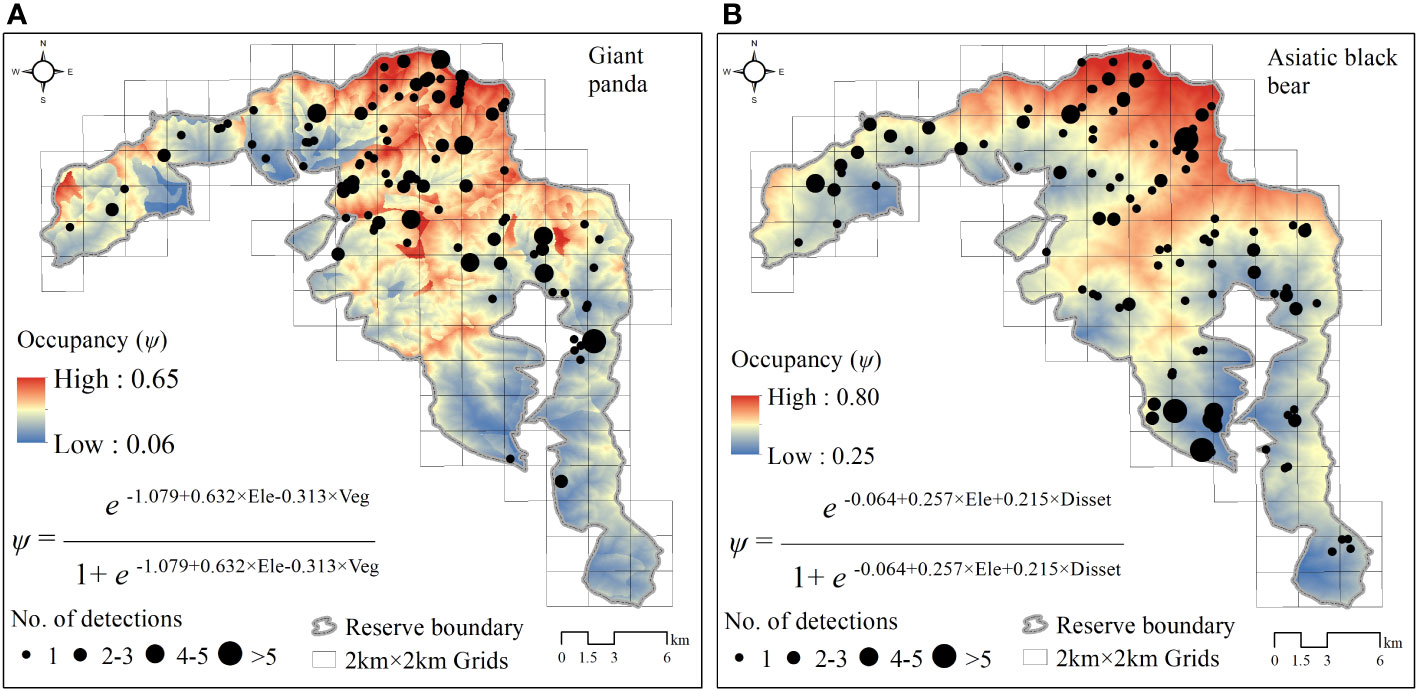
Figure 2 Spatial projection of giant panda (A) and Asiatic black bear (B) occupancy probability and model structure in Changqing National Nature Reserve, based on the optimal models (ΔAIC ≤ 2). Black points show the location of giant pandas and Asiatic black bears detected by camera traps, and their size indicates the number of independent detections. Ele, elevation; Veg, understory vegetation type; Disset, distance to nearest settment.
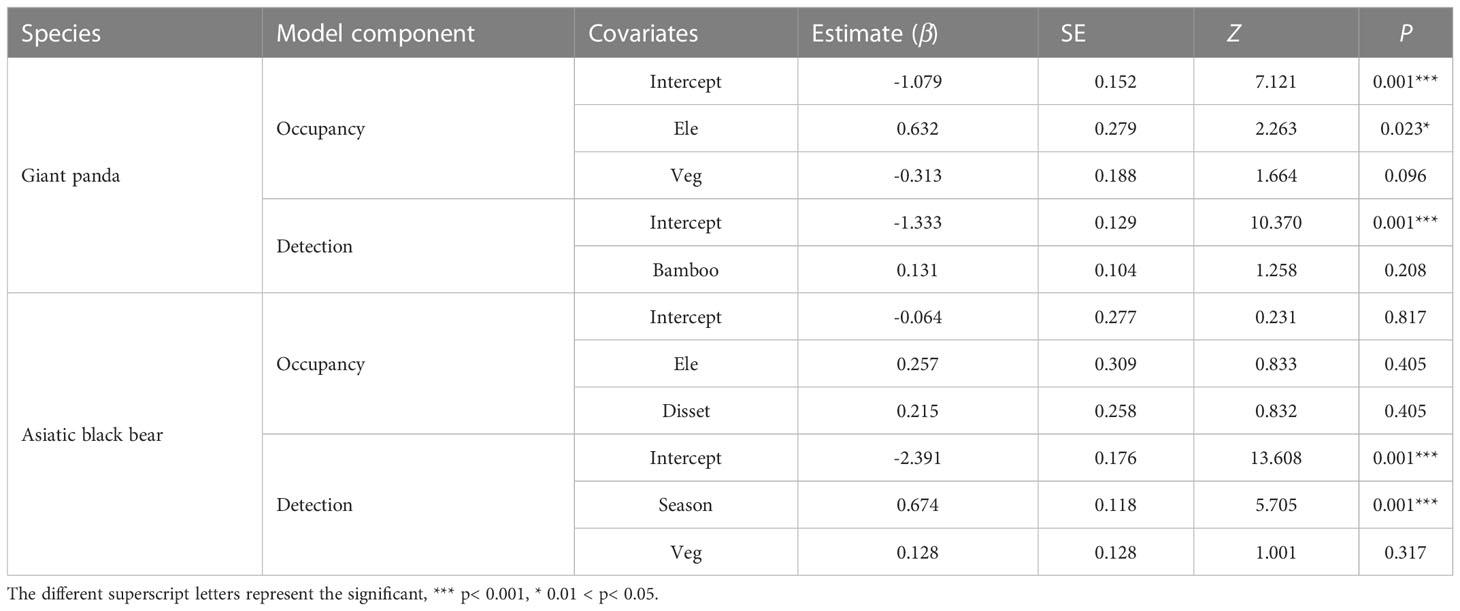
Table 2 Estimated parameter coefficients (β) for covariates influencing giant panda and Asiatic black bear occupancy and detection probability, based on the optimal models (ΔAIC ≤ 2).
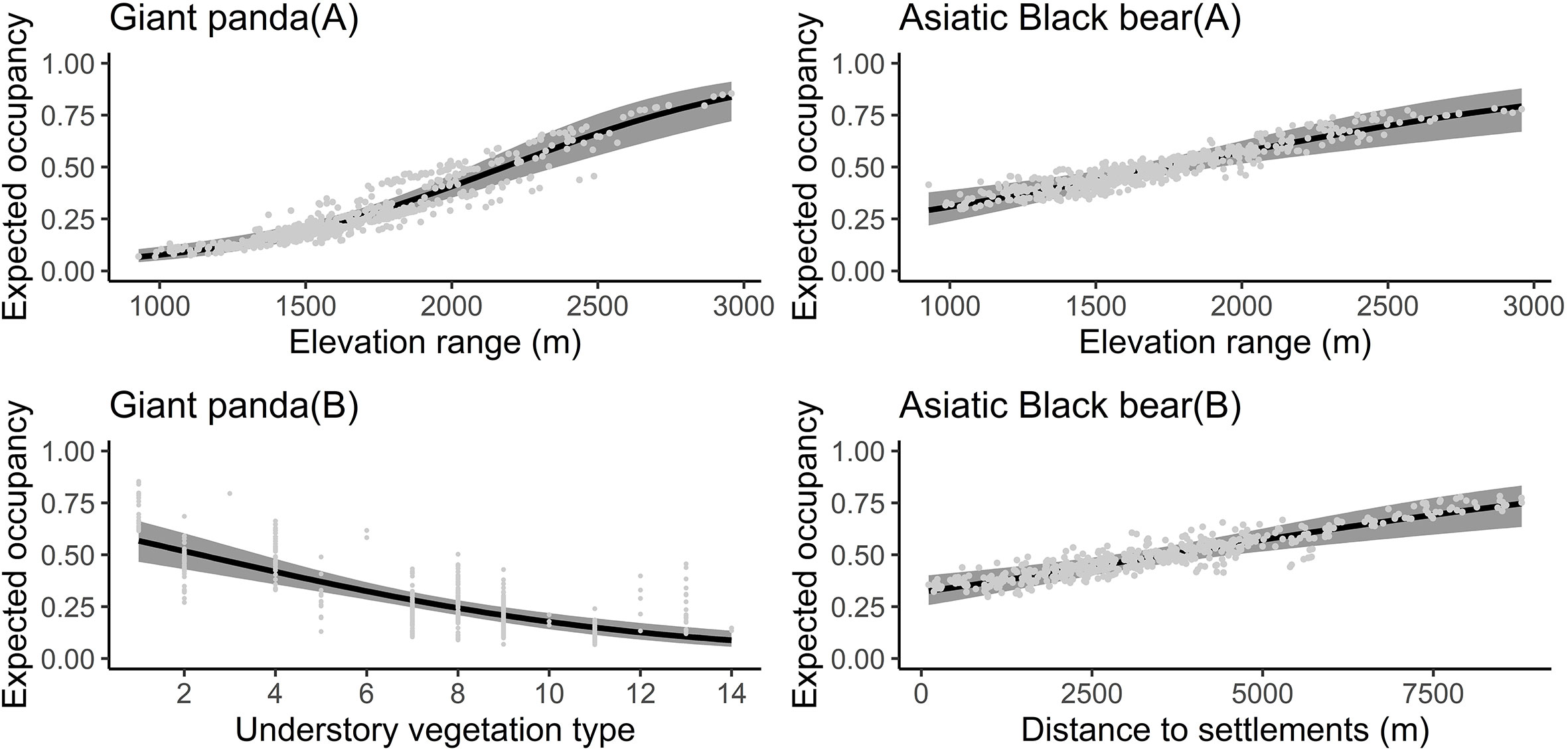
Figure 3 The effect of covariates on giant panda (elevation range and understory vegetation type) and Asiatic black bear (elevation range and distance to nearest settlement) occupancy probability predicted by the optimal models (ΔAIC ≤ 2) in the Changqing National Nature Reserve. The black curve was fitted with polynomial regression; gray area indicated 95% confidence intervals.
Average detection probabilities were P=0.210 for giant panda and P=0.098 for Asiatic black bear. Detection probability for giant pandas was positively predicted by understory bamboo (β=0.131 ± 0.104). Season (β=0.674 ± 0.118) and understory vegetation type (β=0.128 ± 0.128) positively predicted the detection probability of Asiatic black bear (Table 2).
3.3 Spatial distribution and spatial overlap
The giant panda was detected at 49 (41.5%) of the 4 km2 grids in Changqing reserve (spring: 36 grids; summer: 13 grids; autumn: 21 grids and winter: 28 grids). The Asiatic black bear was detected at 56 (47.4%) of 4 km2 grids (spring: 22 grids; summer: 40 grids; autumn: 25 grids and winter: 4 grids). The giant panda and Asiatic black bear had low spatial overlap with each other throughout the year (Sørensen similarity index (Sij) was 0.448 in spring, 0.302 in summer, 0.348 in autumn, and 0.00 in winter).
3.4 Annual activity patterns
The mean survey effort was 7,832 ± 1,212 camera-days per month. Maximum effort was 9,111 camera-days in May, and minimum effort was 6,099 camera-days in February. Based on relative activity indices, activity levels of giant pandas in spring and winter were higher than in summer and autumn (Figure 4). Giant pandas had low activity from June to October with a gradual increasing in January followed by a peak in March and gradual decline until low levels were reached again in June.
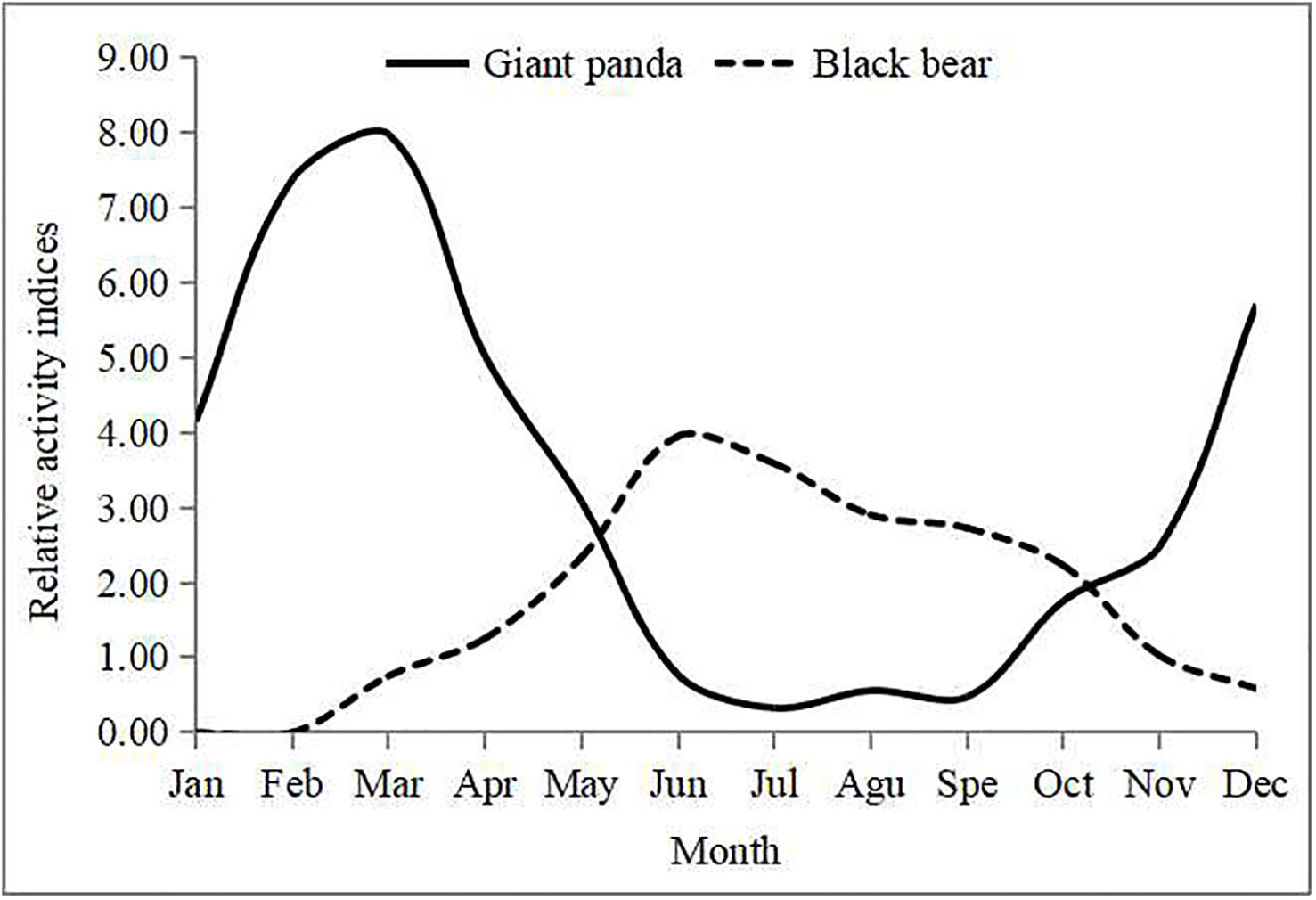
Figure 4 Relative activity indices (RAI) of giant pandas and Asiatic black bears for each month in Changing National Nature Reserve.
In contrast, Asiatic black bears were more active in summer than other seasons. The species showed low levels of activity in March with a gradual increase from April to May followed by a peak in June and gradual decline until the lowest level in early December. Asiatic black bears hibernated during winter; we detected no activity from January to February, and only detected five instances of activity in early December, even with a large survey effort.
3.5 Daily activity patterns across seasons
Based on selection ratios, giant pandas were mainly active during diurnal and crepuscular (wi>1) times in spring and winter (Table 3), with activity peaks at around 15:00-17:00 in spring and 14:00-16:00 in winter (Figure 5), and with low levels of activity during nocturnal times (wi<1). Giant pandas were more active during diurnal times (wi=1.35) than during nocturnal (wi=0.89) and crepuscular times (wi=0.33) in autumn, with activity peaks at around 09:00-12:00 and low activity at around 03:00-05:00. Interestingly, though most of the giant pandas were mainly active in diurnal and crepuscular times, some pandas were still moving during nocturnal times. Independent detections (n=15) of giant pandas in summer were too few for the analysis of daily activity patterns.
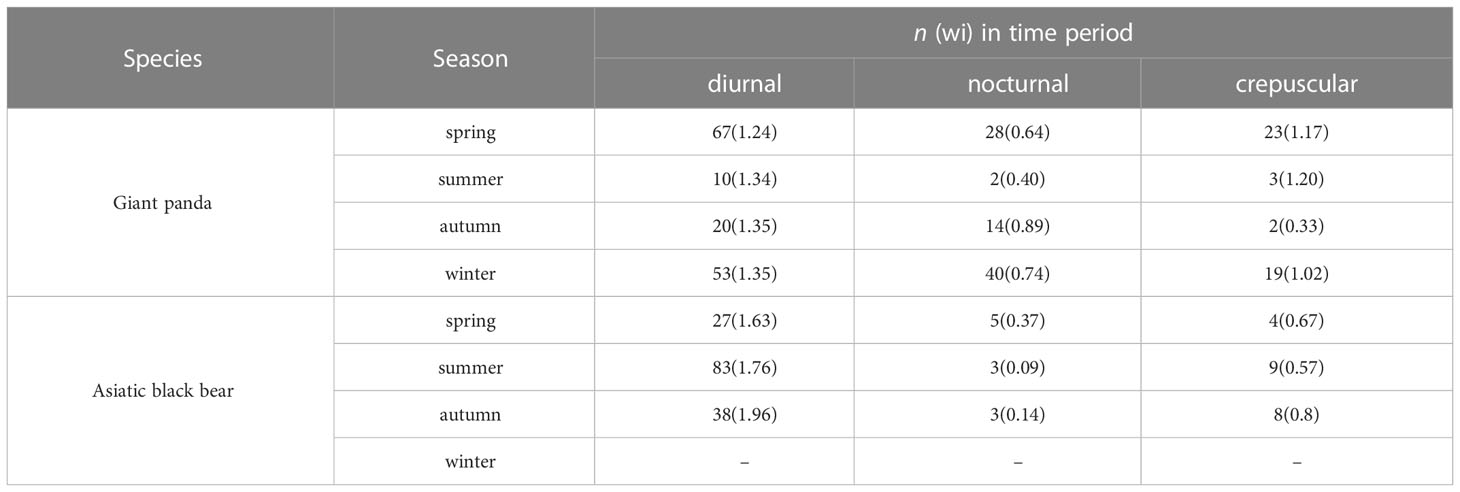
Table 3 The number of independent detections n (selection ratio: wi) of diurnal, nocturnal, and crepuscular time periods for giant pandas and Asiatic black bears in the Changqing National Nature Reserve, China from April 2014 to October 2017.
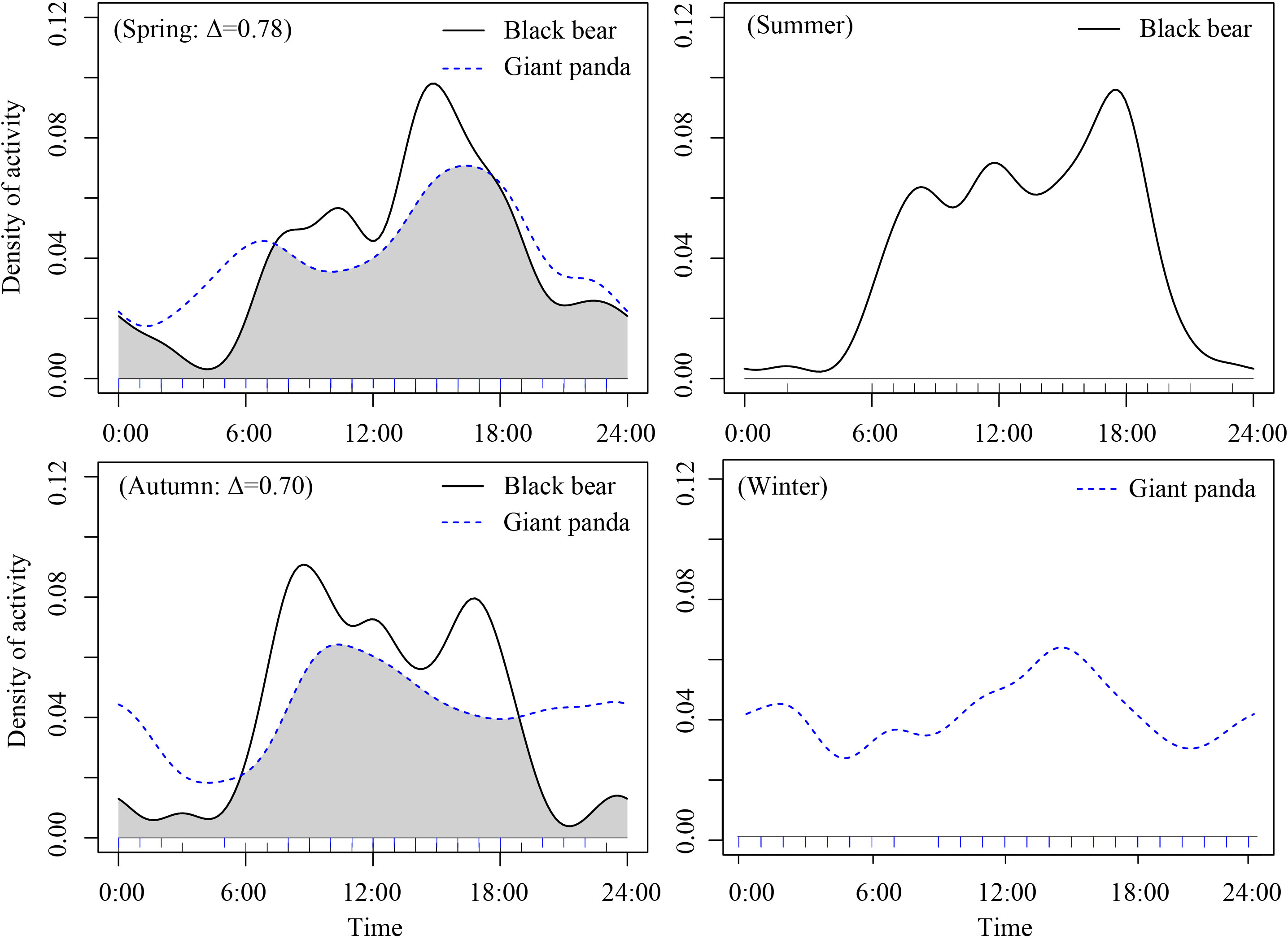
Figure 5 Density estimates of daily activity patterns and temporal overlap (∆) of giant panda and Asiatic black bears across the four seasons in in Changing National Nature Reserve, China. The y-axis is the “density ofactivity”. The overlap area is denoted in grey.
Asiatic black bears mainly had diurnal (wi>1) activity patterns across difference seasons, but there were difference in the timing of the highest activity peak. Bears had one active peak around 15:00-17:00 in spring and summer, and two active peaks in autumn, at about 07:00-08:00 and 16:00-17:00.
3.6 Temporal overlap
The giant panda and Asiatic black bear had high temporal overlap in spring (overlap coefficient (Δ) = 0.78, CI:0.72-0.92) and moderate temporal overlap in autumn (Δ) =0.70, CI: 0.57-0.85, Figure 5). We did not analyze temporal overlap in summer or winter because independent detections of giant pandas (in summer) and Asiatic black bears (in winter) were too few for the analysis.
4 Discussion
Our intensive camera-trap survey elucidated spatial co-occurrence and temporal activity patterns of sympatric giant pandas and Asiatic black bears, information which is meaningful for conservation of these threatened species. Camera trapping provides analytical methods that enable researchers to quantify the presence and distribution of cryptic and low density species, as cameras can be unattended in the field for several months to collect extensive time and space data on multiple species without disturbance (Agha et al., 2018). Furthermore, camera trapping provides detailed daily and seasonal activity patterns, yielding important insights into potential interspecific interactions of sympatric species (Frey et al., 2017; Murphy et al., 2018).
The occupancy model results from this study suggest that the giant panda and Asiatic black bear had different habitat use patterns with respect to elevation. Seasonal migration patterns of the giant panda correspond with bamboo growing seasons and temperature shifts. In the Qinling mountains, giant pandas spend most of the year (approximately November to April) at lower elevation (900-2000 m) to forage Bashania fargesii and shift to higher elevation (2000-2300 m) during the remainder of the year for the Fargesia qinlingensis growing season (Pan, 2014). Giant pandas gradually ascended to high-elevations ranges in summer and autumn as more nutritious foraging becames available and there is less human disturbance. In addition, the higher elevation brought fewer biting insects and safer cub rearing locations, with 75% of births occuring in August and September (Zhu et al., 2010). In contrast, we found that seasonal migration patterns of Asiatic black bears went from high-elevation in spring, low-elevation in summer and mid-elevation in autumn, which is similar to findings from past work. After emerging from winter dens in high-elevation ranges in more southerly ranges, mothers and cubs-of-the-year remain in the vicinity of their place of hibernation until late spring (Penteriani and Melletti, 2021), and mainly feed on new growth of herbaceous plants and shrubs. The bears gradually descended to low-elevation ranges in summer as more nutritious foraging became available in deciduous broadleaf forest and crops (mainly corn) in farmland. Then the bears return to mid-elevation ranges in autumn in search of mast trees in mixed broadleaf-conifer forest to accumulate energy for the coming winter (Reid et al., 1991). Thus, the giant panda and Asiatic black bear showed high spatial avoidance throughout the year, and seasonal migration may be one important mechanism of coexistence.
Regarding activity patterns, we found that giant pandas exhibited seasonality in activity patterns, with higher activity in winter and spring and lower activity in summer and autumn, similar to previous findings from raidio-collared pandas (Zhang et al., 2017). The fact that giant pandas exhibit higher activity in winter and spring can be attributed to foraging and reproduction strategy. Giant pandas need to move longer distances in search of bamboo during winter when resources are relatively poor, and allocate more energy toward travelling and fighting for mating rights in spring (Nie et al., 2015). During summer and autumn, pandas mainly feed on Fargesia qinlingensis in the Qinling mountains, a bamboo that has relatively higher nutritious value and is only distribution in high elevation, causing movement and activity levels to be relatively low (Loucks et al., 2003; Hull et al., 2015). Interestingly, Asiatic black bear showed the opposite pattern to the giant panda; activity patterns were high in late spring and summer, then gradually declined in autumn, similar from results in Sichuan, China, where habitat, available forage and mammal communities are similar (Reid et al., 1991). In fact, seasonal activity patterns of bears are consistent with food availability and mating activity; foods included succulent vegetation (shoots, forbs and leaves) in spring, and insects and a variety of tree and shrub-borne fruits in summer, as well as during the mating season (approximately from May to August) when more travel is required (Koike et al., 2010; Koike et al., 2013; Garshelis and Steinmetz, 2020). Asiatic black bears rely heavily on hard mast (e.g. Quercus, Castanea, Corylus) in autumn, in part to attain sufficient fat reserves for winter denning; thus bears spend most of their time feeding in hard mast trees above, and break branches and pile them up forming what appears to be a platform, causing movement and activity levels to be relatively low underground (Kozakai et al., 2011; Kozakai et al., 2013; Kozakai et al., 2017). According to optimal foraging theory, bears would be expected to maximize their energy intake by spending more time and effort on foraging during autumn. We speculate that the differences in annual activity patterns between giant pandas and Asiatic black bears may result in lower competition between the two species.
Daily temporal partitioning can reduce competition and thus facilitate species coexistence in cases where species segregate between diurnal, crepuscular, and nocturnal domains (Marinho et al., 2020). Based on our results, giant panda and Asiatic black bear exhibited a high (Δ=0.78) degree of temporal overlap in their daily activity patterns in spring and moderate (Δ=0.70) overlap in autumn, suggesting that daily differentiation in activity patterns was not a major factor contributing to their coexistence in the Qinling mountains. Giant pandas exhibited a unimodal daily activity pattern during winter and spring, with peaks around 14:00-17:00, which may reflect a strategy of energy conservation (Zhang et al., 2015). Peak activity of pandas occurred in the afternoon, a period in which they consume a large amount of bamboo, so that they have more time to rest during the cold night, and there may be less need for a second foraging bout to meet their energy needs (Pan, 2014). Because pandas have a low digestive efficiency, peaks in digestion behavior often occurred adjacent to peaks in foraging at night, and digestion was often accompanied with resting (Zhang et al., 2017). Pandas may lay in a rock cave or old-growth tree cavity, overall reducing their movement and energy expenditure during these periods (Zhang et al., 2011).
Our study is the first to use camera traps to elucidate daily activity patterns of the Asiatic black bear in the Qinling mountains. Bears were generally active in the diurnal period and far less active in the crepuscular and nocturnal time, similar to previous studies of this species in other study areas (Hwang and Garshelis, 2007). Asiatic black bears showed unimodal daily activity patterns in the afternoon during spring and summer, similar to pandas, which could also be a strategy of energy conservation. However, Asiatic black bears maintained bimodal daily activity patterns in autumn with peaks around 07:00-09:00 and 15:00-17:00. Although we cannot infer causality from our results, we speculate autumn may be a critical part of the yearly cycle when bears accumulate fat reserves before hibernation, thus, time budgets maybe vary during this season according to the availability of food.
Despite the fact that the range of the giant panda overlaps extensively with Asiatic black bear in the Qinling mountains, their relatively exclusive use of some resources may reflect niche partitioning and dietary differences that gave promoted the coexistence of pandas and Asiatic black bears on a finer scale. Our results suggest that occupancy of Asiatic black bears did not have a negative effect on the detection of giant pandas, and these two species did not show significant negative or positive co-occurrences. This suggests that there were unlikely to be strong interactions between the two species in the Qinling mountains, largely because of their low densities in the study area (State Forestry and Grassland Administration, 2021). The co-occurrence pattern may be unsurprising given that the two species are not closely related within Ursidae and exhibit morphological similarities that may largely be due to convergence (Vy et al., 2017).
Previous studies have documented that wildlife were rarely found below 1,360 m in the study area, a boundary between forest and agriculture (Pan, 2014). However, our camera traps detected giant pandas and Asiatic black bears at lower elevation zones (1265 m and 1109 m, respectively). This change may be due to the implementation of reforestation programs, having low-elevation farmland returned to bamboo forests (Li et al., 2013). Human presence and lower quality habitat in low-elevation zones will likely create new conservation challenges for both giant pandas and Asiatic black bears. While it is unclear if human activities influence the species’ activity pattern and seasonal migration in the study area, the daily lives of local villagers are closely linked to reserve resources (e.g., via collection of bamboo, mushrooms and herbs). Asiatic black bears may forage on honey and damage local agriculture (e.g., corn) in low-elevation areas, creating potential sources of conflict between humans and bears. Additionally, giant panda conservation programme have contributed to an increased in the abundance of sympatric wildlife, as wildlife (e.g. ungulate, mesocarnivore) population increases have also brought about new challenges for conservation and management (Li et al., 2022a; Li et al., 2022b). Yet little is known about the consequences of these population increases in their ecological system in the Qinling mountains, where top predators are virtually absent. These many knowledge gaps and potential threats to giant panda and Asiatic black bear conservation warrant the establishment of long-term monitoring programs that regularly assess changes in wildlife communities and ongoing anthropogenic threats.
Our study has some limitations. First, we were unable to sample some locations due to the difficulty of navigating to remote areas, which may result in detection error or bias, whereby giant pandas and Asiatic black bears may have been present at these sites regardless of our ability to survey them. Second, activity patterns and detection probability may be influenced by other factors that we did not account for in this study, including factors related to species ecology (e.g. home range, density dependence), and environmental factors (e.g. human disturbances, sympatry with other mammals) (Sollmann et al., 2013; Ikeda et al., 2016; Broadley et al., 2019). Further research is needed to determine micro-habitat use of both species before further generalised conclusions are made regarding their co-occurrence patterns on a micro-habitat scale. Because of the limitations of camera-trapping surveys, future studies are required to clarify how the activity patterns of giant pandas and Asiatic black bears relate to specific behaviors, combining survey tools with telemetry surveys and direct observations. In addition, further studies should focus on the relationship between the coexistence patterns and both population density and relative abundance of the two species, and adopt a multi-species occupancy modeling approach to tease apart some of these factors.
5 Conclusion
Our study provides novel information on the spatial and temporal ecology of sympatric giant pandas and Asiatic black bears in forest ecosystems. The results are important to set a baseline of understanding of mechanisms explaining interactions between giant pandas and Asiatic black bears. Furthermore, the results could be used for assessments of conservation status in the study area, which could act as a guide to construct conservation priorities as well as efficient management programs.
Data availability statement
The original contributions presented in the study are included in the article/supplementary materials. Further inquiries can be directed to the corresponding author.
Author contributions
JL and DL conceived the ideas and designed methodology, collected the data, analysed the data and writing of the manuscript. WD collected the data. All authors contributed to the article and approved the submitted version.
Acknowledgments
This work was supported by National Science & Technology Pillar Program during the Twelfth Five-year Plan Period (No.2013BAC09B02), and Nature Reserves Biological Specimen Resources Sharing Subplatform (No. 2005DKA21404). We thank all staff member of the Changqing Nature Reserve for helping to set camera traps in the study area. We also thank Charlotte Hacker and Yadong Xue for valuable suggestions for the manuscript.
Conflict of interest
The authors declare that the research was conducted in the absence of any commercial or financial relationships that could be construed as a potential conflict of interest.
Publisher’s note
All claims expressed in this article are solely those of the authors and do not necessarily represent those of their affiliated organizations, or those of the publisher, the editors and the reviewers. Any product that may be evaluated in this article, or claim that may be made by its manufacturer, is not guaranteed or endorsed by the publisher.
References
Agha M., Batter T., Bolas E. C., Collins A. C., Da Rocha D. G., Monteza-Moreno C. M., et al. (2018). A review of wildlife camera trapping trends across Africa. Afr. J. Ecol. 56 (4), 694–701. doi: 10.1111/aje.12565
Blake J. G., Mosquera D., Guerra J., Loiselle B. A., Romo D. (2014). A hotspot for jaguars Panthera onca (Carnivora: Felidae)? camera-traps and jaguar activity at tiputini biodiversity station, Ecuador. Rev. Biol. Trop. 62 (2), 689–698. doi: 10.15517/rbt.v62i2.11115
Blake J. G., Mosquera D., Guerra J., Loiselle B. A., Romo D., Swing K. (2011). Mineral licks as diversity hotspots in lowland forest of eastern Ecuador. Diversity 3 (2), 217–234. doi: 10.3390/d3020217
Broadley K., Burton A. C., Avgar T., Boutin S. (2019). Density-dependent space use affects in interpretation of camera trap detection rates. Ecol. Evol. 9 (24), 14031–14041. doi: 10.1002/ece3.5840
Carvalho C. J., Cardoso P. (2020). Decomposing the causes for niche differentiation between species using hypervolumes. Front. Ecol. Evol. 8, 243. doi: 10.3389/fevo.2020.00243
Croose E., Bled F., Fowler N. L., Beyer D. E., Belant J. L. (2019). American Marten and fisher do not segregate in space and time during winter in a mixed-forest system. Ecol. Evol. 9, 4906–4913. doi: 10.1002/ece3.5097
Deng S. T., Li J. Q., Qu Y. S., He J., Liu K., Xue H., et al. (2021). Camera trap reveals the co-occurrence patterns of two sympatric muntjac species in southern anhui province, China: no spatial segregation. Ecol. Evol. 11, 17801–17809. doi: 10.1002/ece3.8307
Diao Y. X., Zhao Q., Weng Y., Gu B. J., Wang F. (2021). Temporal shifts as elusive responses to anthropogenic stressors in a stressors in a mammal community. Biodiversity Conserv. 30 (8), 2529–2544. doi: 10.1007/s10531-021-02208-5
Farris Z. J., Gerber B. D., Karpanty S., Murphy A., Wampole E., Ratelolahy F., et al. (2020). Exploring and interpreting spatiotemporal interactions between native and invasive carnivores across a gradient of rainforest degradation. Biol. Invasions 22 (3), 2033–2047. doi: 10.1007/s10530-020-02237-1
Farris Z. J., Kelly M. J., Karpanty S., Ratelolahy F. (2016). Patterns of spatial co-occurrence among native and exotic carnivores in north-eastern Madagascar. Anim. Conserv. 19 (2), 189–198. doi: 10.1111/acv.12233
Frey S., Fisher J. T., Burton A. C., Volpe J. P. (2017). Investigating animal activity patterns and temporal niche partitioning using camera-trap data: challenges and opportunities. Remote Sens. Ecol. Conserv. 3 (3), 123–132. doi: 10.1002/rse2.60
Garshelis D., Steinmetz R. (2020). Ursus thibetanus (amended version of 2016 assessment) (The IUCN Red List of Threatened Species).
Gong H. S., Zeng Z. G., Gao X. B., Liang Q. H., Song Y. L. (2006). Species of birds and mammals feeding on bamboo in foping national nature reserve. Chin. J. Zool. 41 (3), 31–36.
He X. M., Chen L. X., Luo G., Gu X. D., Wang G., Ran J. H. (2018). Suitable habitat prediction and overlap analysis of two sympatric species, giant panda (Ailuropoda melanoleuca) and Asiatic black bear (Ursus thibetanus) in liangshan mountains. Biodiversity Sci. 26 (11), 1180–1189. doi: 10.17520/biods.2018167
He B. S., Sun R. Q., Cheng P., Dong W., Wang J., Wang D. J., et al. (2016). Baseline survey of mammal and bird diversity using camera-trapping in the changqing national nature reserve of shaanxi province. Acta Theriol. Sin. 36 (3), 348–356. doi: 10.16829/j.slxb.201603011
Hull V., Roloff G., Zhang J. D., Liu W., Zhou S. Q., Huang J. Y., et al. (2014). A synthesis of giant panda habitat selection. Ursus 25 (2), 146–162. doi: 10.2192/URSUS-D-13-00011.1
Hull V., Zhang J., Zhou S., Huang J., Li R., Liu D., et al. (2015). Space use by endangered giant pandas. J. Mammal 96, 230–236. doi: 10.1093/jmammal/gyu031
Hwang M. H., Garshelis D. L. (2007). Activity patterns of Asiatic black bear (Ursus thibetanus) in the central mountains of Taiwan. J. Zool. 271, 203–209. doi: 10.1111/j.1469-7998.2006.00203.x
Ikeda T., Uchida K., Matsuura Y., Takahashi H., Yoshida T., Kaji K., et al. (2016). Seasonal and diel activity patterns of eight sympatric mammals in norther Japan revealed by an intensive camera-trap survey. PloS One 11 (10), e016362. doi: 10.1371/journal.pone.0163602
Ji Y. R., Liu F., Li D. Q., Chen Z. Y., Chen P. (2022). Spatial-temporal patterns of sympatric Asiatic black bears (Ursus thibetanus) and brown bears (Ursus arctos) in northeastern China. Animals 12, 1262. doi: 10.3390/ani12101262
Koike S., Masaki T., Nemoto Y., Kozakai C., Yamazaki K., Kasai S., et al. (2010). Estimate of the seed shadow created by the Asiatic black bear ursus thibetanus and its characteristics as a seed disperser in Japanese cool-temperate forest. Oikos 120, 280–290. doi: 10.1111/j.1600-0706.2010.18626.x
Koike S., Nakashita R., Naganawa K., Koyama M., Tamura A. (2013). Changes in diet of a small, isolate bear population over time. J. Mammal 74, 631–368. doi: 10.2307/23488367
Kozakai C., Nemoto Y., Nakajima A., Koike S., Ohnishi N., Yamazaki K. (2017). Influence of food availability on matrilineal site fidelity of female Asian black bears. Mammal Study 42 (4), 1–12. doi: 10.3106/041.042.0404
Kozakai C., Yamazaki K., Nemoto Y., Nakajima A., Koike S., Abe S., et al. (2011). Effect of mast production on home range use of Japanese black bears. J. Wildlife Manage. 75, 867–875. doi: 10.1002/jwmg.122
Kozakai C., Yamazaki K., Nemoto Y., Nakajima A., Umemura Y., Koike S., Goto Y., et al. (2013). Fluctuation of daily activity time budgets of Japanese black bears: relationship to sex, reproductive status, and hardmast availability. J. Mammal 94, 351–360. doi: 10.1644/11-MAMM-A-246.1
Lesmeister D. B., Nielsen C. K., Schauber E. M., Hellgren E. C. (2015). Spatial and temporal structure of a mesocarnivore guild in midwestern north America. Wildlife Monogr. 191 (1), 1–61. doi: 10.1002/wmon.1015
Li J., Li D. Q., Hacker C., Dong W., Wu B., Xue Y. D. (2022a). Spatial co-occurrence and temporal activity patterns of sympatric mesocarnivores guild in qinling mountains. Global Ecol. Conserv. 36, e02129. doi: 10.1016/j.gecco.2022.e02129
Li J., Liu F., Xue Y. D., Zhang Y., Li D. Q. (2017). Assessing vulnerability of giant panda to climate change in the qinling mountains of China. Ecol. Evol. 7, 4003–4015. doi: 10.1002/ece3.2981
Linkie M., Ridout M. S. (2011). Assessing tiger-prey interactions in Sumatran rainforests. J. Zool. 3 (3), 224–229. doi: 10.1111/j.1469-7998.2011.00801.x
Liu Z. X., Dayananda D., Jeffree R. A., Tian C., Zhang Y. Y., Yu B., et al. (2020). Giant panda distribution and habitat preference: the influence of sympatric large mammals. Global Ecol. Conserv. 4, e01221. doi: 10.1016/j.gecco.2020.e01221
Liu F., McShea W., Garshelis D., Zhu X. J., Wang D. J., Gong J. E., et al. (2009). Spatial distribution as a measure of conservation needs: an example with Asiatic black bears in south-western China. Diversity Distribution 15, 649–659. doi: 10.1111/j.1472-4642.2009.00571.x
Li Y., Viña A., Yang W., Chen X. D., Zhang J. D., Ouyang Z. Y., et al. (2013). Effects of conservation policies on forest cover change in giant panda habitat regions, China. Land Use Policy 33, 42–53. doi: 10.1016/j.landusepol.2012.12.003
Li J., Wang X. L., Yang M. Y., Cheng D. X., Wang X. J., Luo P., et al. (2020). Construction progress of camera-trapping database from the nature reserves biological specimen resources sharing Sub-platform. Biodiversity Sci. 28 (9), 1081–1089. doi: 10.17520/biods.2019292
Li J., Xue Y. D., Liao M. F., Dong W., Wu B., Li D. Q. (2022b). Temporal and spatial activity patterns of sympatric wild ungulates in qinling mountains, China. Animals 12, 1666. doi: 10.3390/ani12131666
Loucks C. J., Lv Z., Dinerstein E., Wang D. J., Fu D. L., Wang H. (2003). The giant pandas of the qinling mountains, China: a case study in designing conservation landscapes for elevational migrants. Conserv. Biol. 17 (2), 558–565. doi: 10.1046/j.1523-1739.2003.01494.x
Manly B. F. J., McDonald L. L., Thomas D. L., McDonald T. L., Erickson W. P. (2002). Resource selection by animals: statistical design and analysis for field studies. 2ed (Netherlands: Springer).
Marinho P. H., Fonseca C. R., Sarmento P., Fonseca C., Venticinque E. M. (2020). Temporal niche overlap among mesocarnivores in a caatinga dry forest. Eur. J. Wildlife Res. 66 (2), 34. doi: 10.1007/s10344-020-1371-6
Mella-Méndez I., Flores-Peredo R., Pérez-Torres J., Hernández-González S., González-Urbie D. U., Bolívar-CiméB S. (2019). Activity patterns and temporal niche partitioning of dogs and medium-sized wild mammals in urban parks of xalapa, Mexico. Urban Ecosyst. 22 (6), 1061–1070. doi: 10.1007/s11252-019-00878-2
Meredith M., Ridout M. (2021) Overview of the “Overlap” package. Available at: http://cran.cs.wwu.edu/web/packages/overlap/vignettes/overlap.pdf (Accessed 10 November 2021).
Monterroso P., Alves P. C., Ferreras P. (2014). Plasticity in circadian activity patterns of mesocarnivores in southwestern Europe: Implications for species coexistence. Behav. Ecol. Sociobiol. 68 (9), 1403–1417. doi: 10.1007/s00265-014-1748-1
Moreno-Sosa A. M., Yacelga M., Craighead K. A., Kramer-Schadt S., Abrams J. F. (2022). Can prey occupancy act as a surrogate for mesopredator occupancy? a case study of ocelot (Leopardus pardalis). Mamm. Biol. 102 (1), 163–175. doi: 10.1007/s42991-022-00232-8
Murphy A., Kelly M. J., Karpanty S. M., Andrianjakarivelo V., Farris Z. J. (2018). Using camera traps to investigate spatial co-occurrence between exotic predators and native prey species: a case study from northeastern Madagascar. J. Zool. 307, 265–273. doi: 10.1111/jzo.12645
Nie Y. G., Zhang Z. J., Raubenheimer D., Elser J. J., Wei W., Wei F. (2015). Obligate herbivory in an ancestrally carnivorous lineage: the giant panda and bamboo from the perspective of nutritional geometry. Funct. Ecol. 29 (1), 26–34. doi: 10.1111/1365-2435.12302
Noor A., Mir Z. R., Veeraswami G. G., Habib B. (2017). Activity patterns and spatial co-occurrence of sympatric mammals in the moist temperate forest of the Kashmir himalaya, India. Folia Zool. 66 (4), 231–241. doi: 10.25225/fozo.v66.i4.a4.2017
Ordiz A., Bishchof R., Swenson J. E. (2013). Saving large carnivores, but losing the apex predator? Biol. Conserv. 168, 128–133. doi: 10.1016/j.biocon.2013.09.024
Pan W. S. (2014). A chance for the lasting survival: ecology and behavior of wild giant pandas (Washington, DC: Smithsonian institution scholarly press).
Pastore A. I., Barabás G., Bimler M. D., Mayfield M. M., Miller T. E. (2021). The evolution of niche overlap and competitive differences. Nat. Ecol. Evol. 5 (3), 330–337. doi: 10.1038/s41559-020-01383-y
Penteriani V., Melletti M. (2021). Bears of the world: ecology, conservation and management (New York: University printing house).
Ran J., Du B., Yue B. (2009). Conservation of the endangered giant panda ailuropoda melanoleuca in China: successes and challenges. Oryx 43 (2), 176–178. doi: 10.1017/S0030605309432010
Reid D., Jiang M. D., Teng Q. T., Qin Z. S., Hu J. C. (1991). Ecology of the Asiatic black bear (Ursus thibetanus) in sichuan. Mammalia 55 (2), 221–238. doi: 10.1515/mamm.1991.55.2.221
Ren Y., Yang X. Z., Wang X. J. (2002). Wildlife resource in changqing national nature reserve (Northwestern University Press).
Sørensen T. A. (1948). Method of establishing groups of equal amplitude in plant sociology based on similarity of species content and its application to analyses of the vegetation on Danish commons. Kong Danish Vidensk Selsk Biol. Skr (Copenhagen) 5, 1–34.
Schaller G., Hu J. C., Pan W. S., Zhu J. (1985). The giant panda of wolong (Chiccago: Chiacago university press).
Seryodkin I. V. (2015). Diet composition of brown bear and Asiatic black bear in the middle sikhote-alin (Russian far east): comparative study. Bull. Irkutsk State Univ. 12, 32–38.
Sollmann R., Mohamed A., Samejima H., Wilting A. (2013). Risky business or simple solution – relative abundance indices from camera-trapping. Biol. Conserv. 159 (1), 405–412. doi: 10.1016/j.biocon.2012.12.025
State Forestry and Grassland Administration (2021). The 4th national survey report on giant panda in China (Beijing: Science press).
Steinmetz R., Garshelis D. L., Chuptipong W., Seuaturien N. (2013). Foraging ecology and coexistence of Asiatic and sun bears in a seasonal tropical forest in southeast Asia. J. Mammal 94 (1), 1+18. doi: 10.1644/11-MAMM-A-351.1
Steinmetz R., Seuaturien N., Intanajitjuy P., Inrueang P., Prempree K. (2021). The effects of prey depletion on dietary niches of sympatric apex predators in southeast Asia. Integr. Zool. 16 (1), 19–32. doi: 10.1111/1749-4877.12461
Swaisgood R., Wang D., Wei F. (2016). Ailuropoda melanoleuca (errata version published in 2017) (The IUCN Red List of Threatened Species).
Takahata C., Nielsen S. E., Takii A., Izumiyama S. (2014). Habitat selection of a large carnivore along human-wildlife boundaries in a highly modified landscape. PloS One 9 (1), e86181. doi: 10.1371/journal.pone.0086181
Torretta E., Serafini M., Puopolo F., Schenone L. (2015). Spatial and temporal adjustments allowing the coexistence among carnivores in liguria (N-W Italy). Acta Ethol 19 (2), 123–132. doi: 1007/s10211-015-0231-y
Vy N. T., Ngoprasert D., Gale G. A., Browne S. T., Savini T. (2017). Co-Occurrence of two sympatrc galliform species on a landscape-scale. Paffles Bull. Zool. 65, 60–67.
Wang F., McShea W. J., Wang D. J., Li S. (2015). Shared resources between giant panda and sympatric wild and domestic mammals. Biol. Conserv. 186, 319–325. doi: 10.1016/j.biocon.2015.03.032
Wei F. W., Swaisgood R., Hu Y. B., Nie Y. G., Yan L., Zhang Z. J., et al. (2015). Progress in the ecology and conservation of giant panda. Conserv. Biol. 29, 1497–1507. doi: 10.1111/cobi.12582
Zager P., Beecham J. (2006). The role of American black bears and brown bears as predators on ungulates in north America. Ursus 17 (2), 95–108. doi: 10.2192/1537-6176(2006)17[95:TROABB]2.0.CO;2
Zhang J. D., Hull V., Huang J., Zhou S. Q., Xu W. H., Yang H. B., et al. (2015). Activity patterns of the giant panda (Ailuropoda melanoleuca). J. Mammal 96 (6), 1116–1127. doi: 10.1093/jmammal/gyv118
Zhang J. D., Hull V., Ouyang Z. Y., He L., Connor T., Yang H. B., et al. (2017). Modeling activity patterns of wildlife using time-series analysis. Ecol. Evol. 78 (8), 2575–2584. doi: 10.1002/ece3.2873
Zhang Z. J., Swaisgood R. R., Zhang S., Nordstorm L. A., Wang H., Gu X., et al. (2011). Old growth forest in what giant pandas really need. Biol. Lett. 7, 403–406. doi: 10.1098/rsbl.2010.1081
Zhao S. C., Zheng P. P., Dong S. S., Zhan X. J., Wu Q., Guo X. S., et al. (2013). Whole-genome sequencing of giant pandas provides insights into demographic history and local adaptation. Nat. Genet. 45 (1), 67–73. doi: 10.1038/ng.2494
Keywords: camera trapping, interspecific interaction, temporal overlap, occupancy model, coexistence patterns
Citation: Li J, Li D and Dong W (2023) Coexistence patterns of sympatric giant pandas (Ailuropoda melanoleuca) and Asiatic black bears (Ursus thibetanus) in Changqing National Nature Reserve, China. Front. Conserv. Sci. 4:1029447. doi: 10.3389/fcosc.2023.1029447
Received: 27 August 2022; Accepted: 20 January 2023;
Published: 03 February 2023.
Edited by:
Vanessa Hull, University of Florida, United StatesReviewed by:
Qiang Dai, Chengdu Institute of Biology, Chinese Academy of Sciences (CAS), ChinaHong Mingsheng, China West Normal University, China
Amira Sharief, Zoological Survey of India, India
Copyright © 2023 Li, Li and Dong. This is an open-access article distributed under the terms of the Creative Commons Attribution License (CC BY). The use, distribution or reproduction in other forums is permitted, provided the original author(s) and the copyright owner(s) are credited and that the original publication in this journal is cited, in accordance with accepted academic practice. No use, distribution or reproduction is permitted which does not comply with these terms.
*Correspondence: Diqiang Li, lidiqiang_caf@163.com