- 1Wildlife Health and Agricultural Ecosystems Functioning Service, French Biodiversity Agency (OFB), Orléans, France
- 2Unit of Hygiene and Quality of Poultry & Pork Products, Laboratory of Ploufragan-Plouzané-Niort, French Agency for Food Environmental and Occupational Health & Safety (ANSES), Ploufragan, France
- 3Protected Areas Service, French Biodiversity Agency (OFB), Giffaumont, France
- 4Data and Methodological Support Service, French Biodiversity Agency (OFB), Auffargis, France
- 5Pasteur Institute, Paris-Cité University, National Reference Centre for Anaerobic Bacteria and Botulism, Paris, France
- 6Conservation and Sustainable Management of Exploited Species Service, French Biodiversity Agency (OFB), Arles, France
- 7Rabies and Wildlife Laboratory, ANSES, Malzéville, France
Botulism in wild birds is a widespread and potentially lethal disease raising major conservation issues. Botulism is also of public health concern. Due to the action of botulinum neurotoxins, mostly produced by Clostridium botulinum, botulism can affect wild birds, livestock, and humans. This study is part of a project aimed at improving our understanding of the pathogenesis of botulism in wild avifauna, which is still poorly understood. Indeed, the prevalence and dynamics of C. botulinum in the digestive tract or in bird tissue, whether as intermittent carriage related to environmental contamination or as part of the normal avian microbiota, is still unknown. In this study, we specifically addressed the presence of a healthy carrier status of wild birds, and its role in outbreaks. To answer this question, we monitored the estimated prevalence of C. botulinum in wild birds through samples from banded and swabbed birds as well as from hunted bird organs. Our results do not support the hypothesis of a healthy carriage outside of outbreaks, which raises the question of the bioavailability of the bacterium and toxin in the environment. Finally, the gene encoding botulinum neurotoxin type E was detected in keel muscle from a hunted bird, showing that recommendations on the consumption of wild bird meat are needed following a botulism outbreak.
1. Introduction
Avian botulism is considered to be one of the main causes of loss of waterfowl and shorebirds worldwide, inducing up to one million deaths in some wetlands (Rocke, 2006; Rocke and Bollinger, 2007; Hardy and Kaldhusdal, 2013). It can therefore have a huge impact on endangered species, on local populations, or on birds with limited distribution (Rocke, 2006). Studies from the literature have reported 15% loss of the western population of American white pelican (Pelecanus erythrorhynchos) in California (Rocke et al., 2004) and 7% loss of the total population of Black-faced spoonbills (Platalea minor) in Taiwan (BirdLife International, 2004) due to botulism outbreaks. The disappearance of the Great black-backed gulls (Larus marinus) from eastern Lake Ontario (Shutt et al., 2014) and declines in the population of Northern pintail (Anas acuta) in the United States (Friend et al., 2001) have been linked to botulism. An impact on White-headed duck (Oxyura leucocephala) and on Marbled teal (Marmaronetta angustirostris), both endangered and vulnerable species, has also been strongly suggested in Spain (Vidal et al., 2013). Botulism has been diagnosed in 264 species of birds, mostly belonging to waterbird families (Rocke, 2006), with the Anatidae and more precisely the Anatinae, covering dabbling ducks such as mallards (Anas platyrhynchos) and Common teal (Anas crecca), being the most affected family at least in France, Spain and South Africa (Anza et al., 2016; Ventujol et al., 2017; Russell et al., 2019). Multiple studies have been published over the last decade reporting avian botulism outbreaks in many places around the world (Table 1). The impact of avian botulism is expected to increase in the future due to climate change, increasing urbanization, eutrophication, and invasive aquatic species (Anza, 2014; Espelund and Klaveness, 2014; Russell et al., 2019).
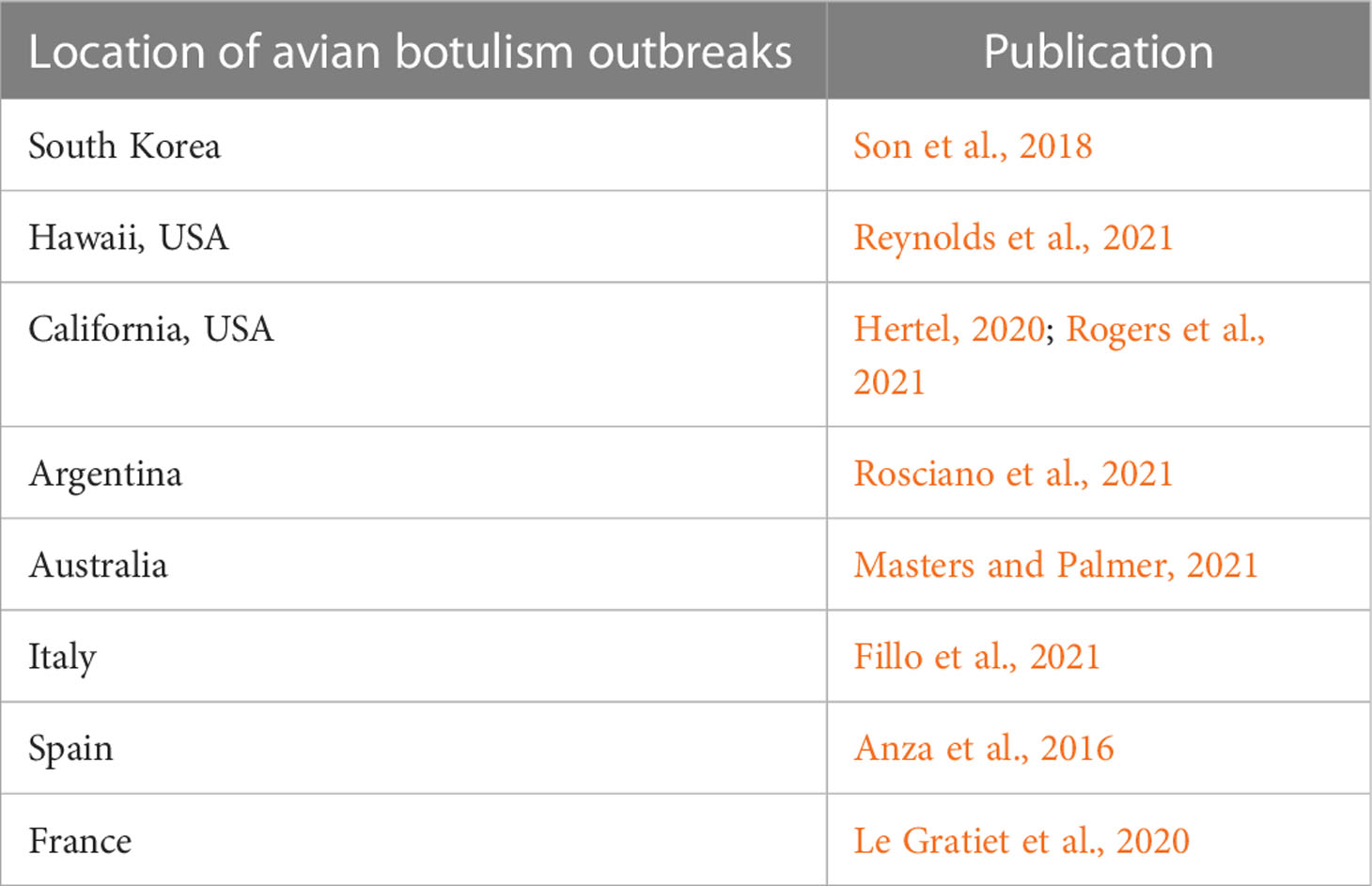
Table 1 Examples of publications reporting avian botulism outbreaks around the world, over the last decade.
Botulism is caused by the action of the botulinum neurotoxin (BoNT) that prevents the release of acetylcholine in the nerve endings, resulting in flaccid paralysis. Major clinical signs of avian botulism include difficulty or inability of birds to use their legs and wings to swim and fly, difficulty holding their neck in the final stages of the disease (Badagliacca et al., 2018), and prolapse of the nictitating membrane (Holmes, 2019), all common signs of botulism (Rocke, 2006). The disease often leads to respiratory problems and drowning of affected wild birds.
BoNT is typically produced by Clostridium botulinum, a motile, Gram-positive, spore-forming and strictly anaerobic bacterium (Rasetti-Escargueil et al., 2019). Among all different BoNT types and subtypes described so far, avian botulism is mostly associated with BoNT type C/D (Woudstra et al., 2012; Anza, 2014; Le Gratiet et al., 2020), type C and type E (Lan Chun et al., 2015) in wild birds. BoNT C/D is currently the most common BoNT involved in avian botulism in Europe, while BoNT E is reported around the Great Lakes in the USA and Canada. BoNT C/D is produced by C. botulinum group III, part of the C. novyi sensu lato genogroup (Skarin et al., 2011). Mosaic BoNT C/D was first described in 2005 (Takeda et al., 2005) and differentiation between BoNT C and C/D requires the use of dedicated tools able to distinguish mosaic from non-mosaic types, such as subtyping PCR (Nakamura et al., 2010; Anniballi et al., 2012; Woudstra et al., 2012; Anniballi et al., 2013) or Endopep-MS (Bjornstad et al., 2014, Hansbauer et al., 2016).
The pathogenesis of avian botulism and the factors behind the initiation of an outbreak in wild birds are not completely understood. Two different scenarios have been put forward: clinical signs may be due to intoxication through direct ingestion of preformed BoNT (Holmes, 2019), or to toxico-infection through ingestion of C. botulinum followed by in situ production of BoNT in the digestive tract (Rocke, 2006). The second scenario has been described in poultry in particular, where BoNT production in ceca is required to induce clinical signs (Miyazaki and Sakaguchi, 1978; Hyun and Sakaguchi, 1989). Persistence of C. botulinum in the digestive tract of sick wild birds has been reported, suggesting possible toxico-infection in wild birds, as already described in poultry (Anza et al., 2016). The extent of these intoxication/toxico-infection pathways and their role in avian botulism are currently unknown, but they likely coexist (Skarin et al., 2015).
Carcasses are known to play a major role in avian botulism, as decaying matter is favorable for C. botulinum growth and BoNT production. Invertebrates, maggots in particular, are not sensitive to BoNTs and can concentrate BoNT and C. botulinum when they feed on decomposing tissue. Ingestion of these infected invertebrates by healthy birds will induce their death, resulting in perpetuation and amplification of the botulism outbreak, a phenomenon called the carcass-maggot cycle (Wobeser, 1997). The concomitant presence of decaying carcasses (due to any cause of bird death) and of C. botulinum (as a normal inhabitant of the bird digestive tract or after recent ingestion by the bird from its contaminated environment) can lead to a botulism outbreak (Rocke, 2006; Anza et al., 2016). Initiation of an avian botulism outbreak may therefore occur in any bird carcass when spores of C. botulinum are present in the digestive tract (Reynolds et al., 2021). Clostridium botulinum can be found in bird tissue (Reed and Rocke, 1992) and in bird feces (Matveev and Konstantinova, 1974). The prevalence and dynamics of C. botulinum in the digestive tract or in bird tissue, whether as intermittent carriage related to environmental contamination or as part of the normal avian microbiota, are however unknown.
The objective of our study was to investigate the prevalence of C. botulinum in wild bird tissue by collecting samples in areas with or without botulism outbreaks, so as to provide new insights into the potential existence of C. botulinum carriage by apparently healthy birds (i.e., birds without clinical signs associated to botulism).
2. Materials and methods
2.1. Study sites
Data used in this study were collected at four different sites in France (Figure 1): (A) Grand Birieux pond (148 hectares, 80 ha for the pond only), (B) the natural reserve of Baie de l’Aiguillon (5,000 ha), (C) the wildlife reserve of Grand’Mare (147 hectares), and (D) the wildlife reserve of Lac du Der (5,700 ha in total).
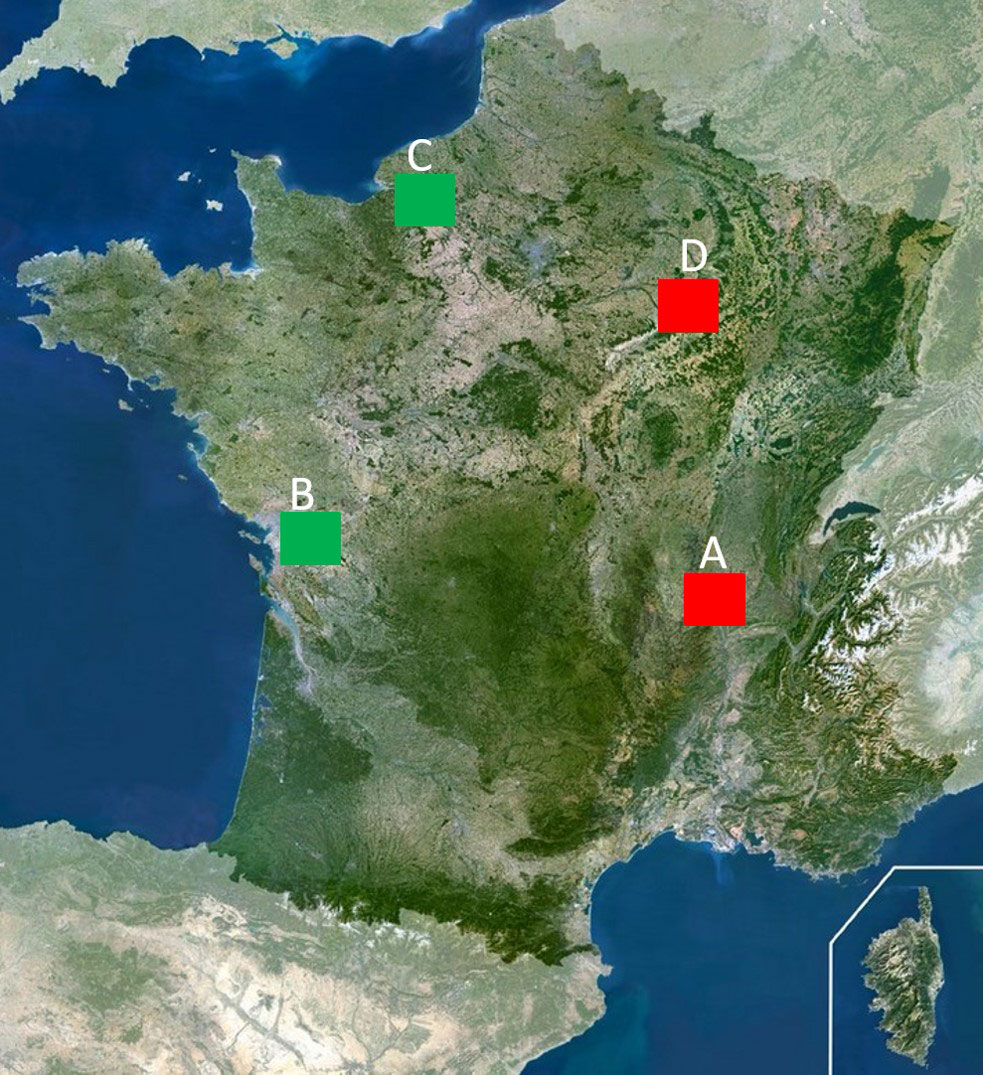
Figure 1 Location of the four study sites. (A) Etang du Grand Birieux, (B) Baie de l’Aiguillon, (C) Grand’Mare, (D) Lac du Der. The green squares indicate sites where prevalence was estimated (see text) and no botulism outbreak was detected. The red squares indicate sites where prevalence was estimated and botulism outbreaks were detected.
2.2. Data collection
2.2.1. Sampling to estimate prevalence
First, we assessed the prevalence of C. botulinum in birds at the four sites, annually from 2018 to 2020, through sampling of hunted birds at site A, and through cloacal swab sampling at sites B, C and D.
At sites B, C and D, the sampling strategy was opportunistic: we took advantage of capture and banding operations that already took place (generally between September and March, using traps such as cages or nets) to carry out cloacal swabbing on banded birds. All cloacal swabs were realized by trained and qualified banders. Swabbing was mostly carried out on two species, mallards and common teals, because these were the most frequently captured and banded birds, and because they are both of interest for the study of botulism (see 2.3 Data processing).
In total, 171 banded birds were swabbed: 42 at Baie de l’Aiguillon (B), 23 at Grand’Mare (C), and 106 at Lac du Der (D). More banded birds were swabbed at site D because a botulism outbreak was detected there (based on clinical signs and detection of C. botulinum type C/D in symptomatic bird liver), so additional efforts were deployed to follow the dynamics of infection.
At site A, a botulism outbreak was detected in September and October 2018. It was confirmed based on a white stork (Ciconia ciconia) found dead on the pond, whose liver was collected and analyzed and found to be positive for a gene coding for BoNT C/D and E (using the method developed in Le Maréchal et al., 2017). A post-mortem study was also carried out on 29 birds hunted around this pond in October 2018, with birds stored frozen at a temperature below -18°C (as recommended in Le Maréchal et al., 2017), until necropsy in November 2018. Necropsies were conducted in the local veterinarian laboratory by an experienced team that routinely conducts necropsies. Five organs were tested for the presence of C. botulinum: cecum, intestinal contents, liver, gizzard, and keel muscle from each hunted bird. To estimate prevalence, the analyzed ceca of these dead birds were considered equivalent to swabs collected on live banded birds at the other three sites, as the literature suggests that cloacal swabs and cecum can be used for C. botulinum type C/D detection, giving similar results (Vidal et al., 2013).
2.2.2. Clostridium botulinum detection and diagnostic procedure
Clostridium botulinum was detected using real-time PCR targeting the gene encoding BoNT C/D and E, after enrichment as previously described (Le Maréchal et al., 2016; Le Maréchal et al., 2017; Souillard et al., 2021). A sample was considered positive when characteristic amplification was detected by PCR. The methods used in this study did not allow for a distinction between spores and vegetative cells as no thermal treatment was applied before the enrichment step.
Regarding sampled organs, ceca, livers and keel muscles were analyzed at ANSES (French National Reference Laboratory for Avian Botulism, Ploufragan laboratory) using the method previously mentioned, while gizzards and intestinal contents were analyzed by the French National Reference Center for Anaerobic Bacteria and Botulism (Institut Pasteur) using the protocol described in Le Maréchal et al. (2019).
The current recommendation for confirmation of avian botulism in France is the analysis of livers collected from at least four birds showing clinical signs (Le Maréchal et al., 2016). When sampling poultry, this standard protocol is easily applied, it is more difficult in wild avifauna where livers are collected from a small number of birds (usually on carcasses) and then tested.
For the prevalence study at sites B, C and D, C. botulinum detection was based on cloacal swabs analyzed using real-time PCR for banded birds. Here, a positive case was defined as a swabbed banded bird for which the gene coding for BoNT was detected after the enrichment step followed by DNA extraction as previously described (Le Maréchal et al., 2016; Souillard et al., 2021).
For the prevalence study at site A, post-mortem diagnosis was based on organs (cecum, intestinal contents, gizzard, keel muscle, and liver) analyzed using real-time PCR for hunted birds. Here, a positive case was defined as a hunted bird with an organ in which the gene coding for BoNT was detected.
2.3. Data processing
We pooled common teals and mallards for analyses (i.e., we did not distinguish between the two species) as they share many biological and ecological characteristics: both are dabbling ducks (Anatinae) and are susceptible to botulism, they share the same mostly granivorous diet during winter, and are both abundant in France and in the three study areas. Pooling the two species also made it possible to work with a larger dataset. Ultimately, the swabbing dataset consisted of a total of 171 swabbed banded birds for sites B, C and D, and 29 hunted birds for site A (also common teals and mallards).
To evaluate the dynamics of infection in the studied population at sites B, C and D, we estimated the prevalence at each site during each period of interest, defined as periods of the year with weather conditions as homogeneous as possible between the different sites and years (mean temperatures, humidity, and rainfall). Exposure of birds to C. botulinum is known to remain constant during consistent weather conditions. Since October did not have consistent meteorological conditions, we did not estimate prevalence during this month of the year. Indeed, we wanted to focus on sure and consistent periods from year to year, and after performing a PCA of three weather variables (temperature, precipitation, humidity), October was not constant among 2018, 2019 and 2020. At sites B, C and D, we therefore estimated botulism prevalence during the periods August/September, November/December and January/February, depending on the availability of data for each year. At site A, the available data provided only a snapshot of the prevalence (as they were collected over only one day), and it was not possible to study the dynamics of the outbreak.
2.4. Data analysis
Once sufficient data were available, i.e., number of swabbed birds N > 10, we estimated the prevalence of C. botulinum detection with a Bayesian approach: under the assumption of a botulism outbreak in a site with a C. botulinum prevalence equal to p, the number n positive birds in the sample can be supposed to follow a binomial distribution, with parameters N (the total number of swabbed birds in the sample) and p. To estimate p, we supposed a uniform prior distribution on p (i.e., we supposed that the prevalence could take a priori any value between 0 and 100% with the same probability, so that the estimated prevalence depended only on the collected data and not on any expert prior knowledge). Under these conditions, it is well known that the posterior distribution on p is a beta distribution with parameters alpha = n+1 and beta = N-n+1 (Gelman et al., 2014). We were therefore able to estimate the limits of an 80% credible interval on the prevalence (interval with 80% chance of including the true value of the prevalence) with the function qbeta from the R software.
For site A, we estimated C. botulinum prevalence in November 2018 by evaluating detection in ceca, but also separately for four other organs (intestinal contents, liver, gizzard, and keel muscle), to assess C. botulinum tropism. All statistical analyses were performed with R 4.0.3 and the interface Rstudio version 1.1.463.
3. Results
3.1. Prevalence
3.1.1. Prevalence in banded birds (sites B, C and D)
Among the three sites where banded birds were swabbed, outbreaks were detected only in the pond at Lac du Der (site D). Among all the banded birds swabbed in 2018, 2019 and 2020, C. botulinum type C/D was detected only in one cloacal swab in 2018 (on August 27) at site D. Estimated prevalence for this study site is presented in Table 2. In Baie de l’Aiguillon (site B) and Grand’Mare (site C), no botulism outbreaks were detected and the bacterium was not detected in any of the sampled birds. As noted above, the Bayesian estimation of prevalence based on these data yielded a maximum possible prevalence for each site and period (Table 2).
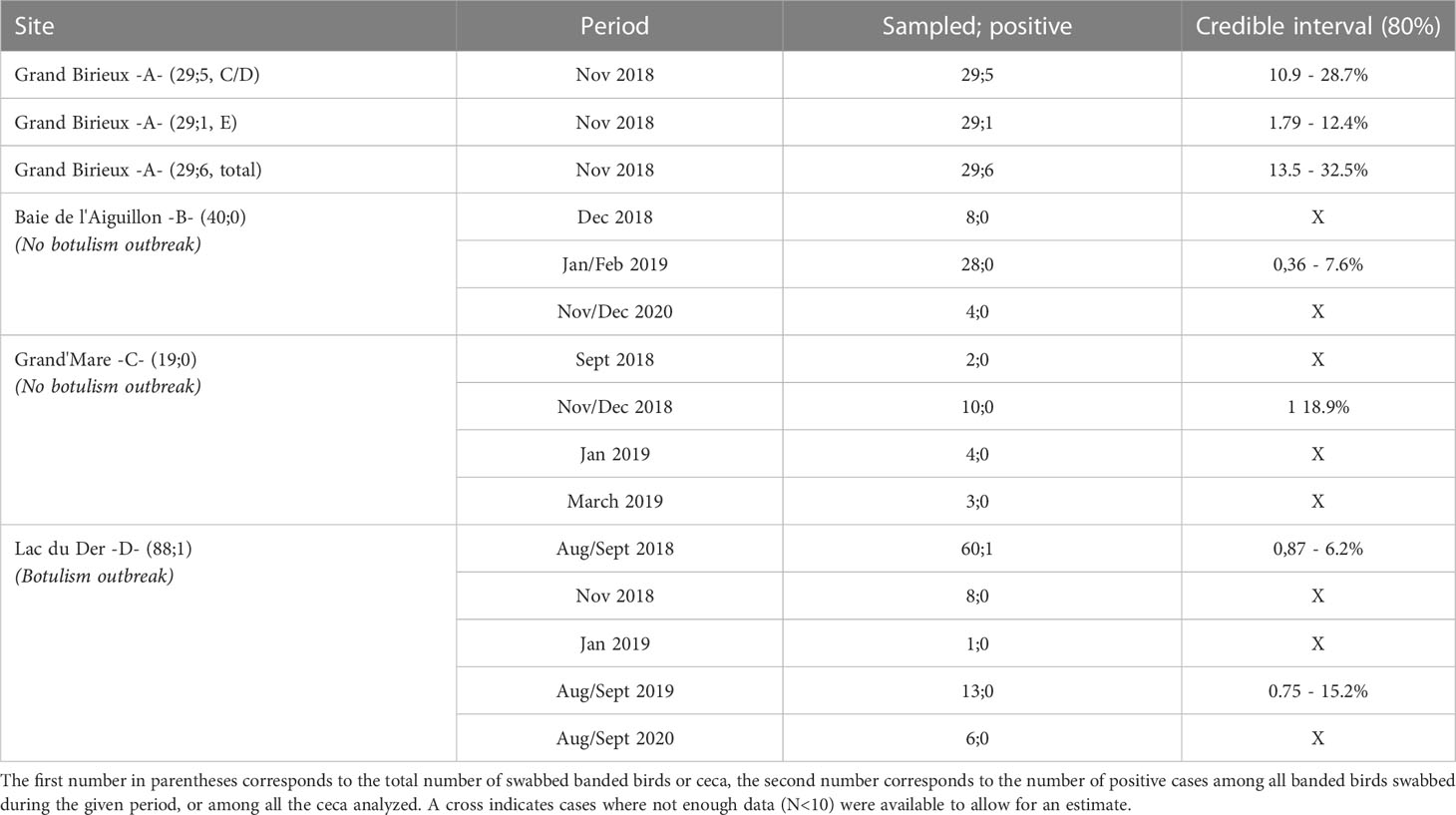
Table 2 Credible interval (80%) on prevalence of positive swabbed banded birds in Baie de l’Aiguillon (B), Grand’Mare (C) and Lac du Der (D) and of positive ceca of hunted birds in Grand Birieux (A).
Table 2 shows that it was not possible to estimate credible intervals for many periods (5 out of 13), because an insufficient number of swabbed banded birds were available to allow for an estimate of informative intervals. Among all periods, no major differences in estimated prevalence were found between sites where botulism outbreaks were detected and sites where no outbreaks were detected, from the data on swabbed banded birds (from sites B, C and D). As a result, we could not rule out the possibility of an outbreak at sites B and C, but outbreaks were not detected with our limited sample size. Nevertheless, if we compare the credible interval of site A (for BoNT C/D) to the three other sites, it seems higher in positive ceca of hunted birds of site A (10.9-28.7%) than in swabbed banded birds of sites B, C and D.
3.1.2. Prevalence in organs (site A)
The aim was to better understand the contamination of birds during an ongoing botulism outbreak by studying tissue and organ contamination by C. botulinum, with an analysis of five matrices.
Twenty-nine birds were hunted at this site, among which seven were positive for C. botulinum in at least one matrix. At the tissue level, among the 145 organs analyzed using real-time PCR, there were eight organs in which genes encoding BoNT C/D or E were detected. More precisely, the gene encoding BoNT C/D was detected in one gizzard and five ceca, and the gene encoding BoNT E was detected in a keel muscle and a cecum. Overall, among the eight positive organs, six were ceca. Considering this result, prevalence of C. botulinum in each organ was calculated (Table 3).
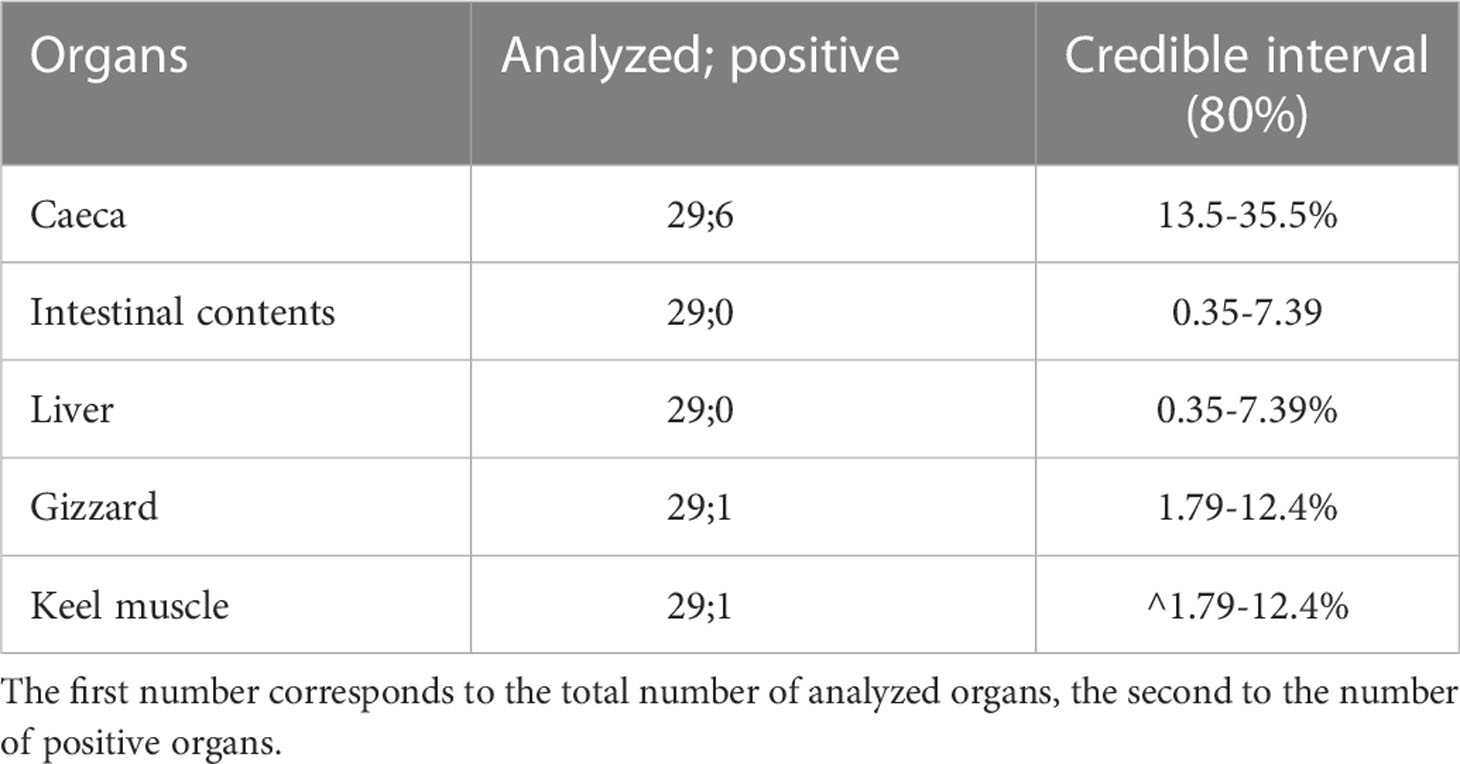
Table 3 Credible interval (80%) on prevalence obtained for each analyzed organ of hunted birds in October 2018 at Grand Birieux (site A).
4. Discussion
The main objective of our study was to assess whether healthy carriers could take part in the mechanisms of emergence or persistence of a botulism outbreak. We found no major differences in estimated C. botulinum prevalence between sites where a botulism outbreak was detected and sites with no detected outbreak. The same result was reported in broilers in a study conducted to evaluate the detection of BoNT type C/D in the cecal contents of 100 flocks, in which the authors failed to detect C. botulinum using a similar method (Hardy and Kaldhusdal, 2013). Both this study and our results, conducted in the wild, therefore suggest that C. botulinum does not seem to be widespread in bird digestive tracts, even during an outbreak. Whether or not botulism outbreaks are detected, the bacterium is only rarely found in non-symptomatic birds.
It is therefore still unclear whether C. botulinum is part of normal avian microbiota. It has been suggested that the C. botulinum level in the avian digestive tract is low, at least under the limit of detection of our methods (Popoff, 1989). This raises some new issues, such as possible upkeep mechanisms in the digestive tract. Asymptomatic carriage of BoNT type D/C by broilers has recently been demonstrated using the exact same method as the one used here (Souillard et al., 2021), supporting the hypothesis that the absence of detection could be interpreted as the absence of carriage or low excretion, and not as a methodological issue.
Nevertheless, this result should be taken with caution, as the sample size in some areas during some periods were too small a sample to draw firm conclusions. It would therefore be interesting to determine a minimum sample size to obtain sufficiently informative credible intervals.
Concerning the hunted birds during an ongoing botulism outbreak at the Grand Birieux site, we found that six of the eight positive organs were ceca. This result is consistent with other studies suggesting the specific role of ceca in the production of toxin. For example, in Reed and Rocke (1992), it was determined that mallards had “detectable type-C botulism spores in [ … ] livers or ceca” (Reed and Rocke, 1992); in Table II of the Haagsma study (1973), the authors also report 21 out of 41 mallards in which C. botulinum type C was found in ceca (Haagsma, 1973).
Our investigations also revealed that a keel muscle was positive for the gene encoding BoNT E in a bird hunted during an ongoing botulism outbreak. The mechanism leading to contamination of this tissue is not known.
We could hypothesize either ante-mortem translocation, or post-mortem contamination during the necropsy. Due to the small sample size (only one keel muscle found positive), it is difficult to draw definite conclusions regarding the possibility of contamination of muscles by C. botulinum. However, if confirmed, this result could be of major importance regarding public health: keel muscles are a prime part of the duck body consumed by humans after harvest. Efforts should be made to increase awareness of this risk and recommendations made available to limit the risk, for instance concerning cutting methods and cooking procedures.
5. Conclusion
Through this study that aimed at estimating the prevalence of C. botulinum in wild birds, we were unable to rule out the hypothesis that there is no healthy carriage in wild birds outside of a botulism outbreak. Our results, however, confirmed that such carriage does not seem to be common and that a larger population of sampled birds is required to accurately determine C. botulinum prevalence. Considering the absence of detection of C. botulinum in avian digestive tracts, this study also raises the question of the role of bird exposure to C. botulinum and/or BoNT in the environment, and more generally the question of C. botulinum and/or BoNT bioavailability. This seems to be a crucial issue to better understand the mechanisms of infection of wild birds by C. botulinum and its pathogenesis, and should therefore be investigated further.
Data availability statement
The original contributions presented in the study are included in the article/supplementary files. Further inquiries can be directed to the corresponding author.
Ethics statement
Ethical review and approval were not required for the animal study because all capture and handling operations were carried out as part of a national dabbling duck banding program, approved by the National Museum of Natural History (CRBPO PP No. 331), and carried out by trained and qualified banders (either Anatidae banding specialists or generalist banders allowed to band any bird species). All these manipulations, including cloacal sampling, are outside the scope of the regulations on the use of animals for scientific purposes in unhoused wildlife.
Author contributions
All authors listed on the title page have contributed significantly to the work, have read and commented on the manuscript, attest to the validity and legitimacy of the data and its interpretation, and agree to its submission.
Acknowledgments
We would like to thank: Natacha Piffeteau (Departmental Federation of Hunters of the Eure – FDC27), Emmanuel Joyeux and Sylvain Haie (OFB) for their valuable help with banding and counting data, and other technical activities, and the Departmental laboratory of analyses of the Ain and Aube (LDA 01 and LDA 10) for their support in the autopsies and analyses of birds. We also thank all members of the Departmental Federation of Hunters of the Marne (FDC51) and the Departmental Service of the Marne (OFB - SD51) for their contribution to data collection in this study.
Conflict of interest
The authors declare that the research was conducted in the absence of any commercial or financial relationships that could be construed as a potential conflict of interest.
Publisher’s note
All claims expressed in this article are solely those of the authors and do not necessarily represent those of their affiliated organizations, or those of the publisher, the editors and the reviewers. Any product that may be evaluated in this article, or claim that may be made by its manufacturer, is not guaranteed or endorsed by the publisher.
References
Anniballi F., Auricchio B., Delibato E., Antonacci M., De Medici D., Fenicia L. (2012). Multiplex real-time PCR SYBR green for detection and typing of group III clostridium botulinum. Veterinary Microbiol. 154 (3-4), 332–338. doi: 10.1016/j.vetmic.2011.07.018
Anniballi F., Auricchio B., Woudstra C., Fach P., Fiore A., Skarin H., et al. (2013). Multiplex real-time PCR for detecting and typing clostridium botulinum group III organisms and their mosaic variants. Biosecur. Bioterrorism 11 (SUPPL. 1), S207–S214. doi: 10.1089/bsp.2012.0084
Anza I., Skarin H., Vidal D., Lindberg A., Båverud V., Mateo R. (2014). The same clade of clostridium botulinum strains is causing avian botulism in southern and northern Europe. Anaerobe 26, 20–23. doi: 10.1016/j.anaerobe.2014.01.002
Anza I., Vidal D., Feliu J., Crespo E., Mateo R. (2016). “Differences in the vulnerability of waterbird species to botulism outbreaks in Mediterranean wetlands: An assessment of ecological and physiological factors,” in Applied and environmental microbiology, vol. 82 . Ed. Schaffner D. W., 3092–3099. doi: 10.1128/AEM.00119-16
Badagliacca P., Pomilio F., Auricchio B., Sperandii A. F., Di Provvido A., Di Ventura M., et al. (2018). Type C/D botulism in the waterfowl in an urban park in Italy. Anaerobe 54, 72–74. doi: 10.1016/j.anaerobe.2018.07.010
BirdLife International (2004). State of the world’s birds 2004: indicators for our changing world (Cambridge, UK: BirdLife International).
Bjornstad K., Tevell Aberg A., Kalb S. R., Wang D., Barr J. R., Bondesson U., et al. (2014). Validation of the endopep-MS method for qualitative detection of active botulinum neurotoxins in human and chicken serum. Anal. Bioanal Chem. 406 (28), 7149–7161. doi: 10.1007/s00216-014-8170-4
Espelund M., Klaveness D. (2014). Botulism outbreaks in natural environments - an update. Front. Microbiol. 5. doi: 10.3389/fmicb.2014.00287
Fillo S., Giordani F., Tonon E., Drigo I., Anselmo A., Fortunato A., et al. (2021). Extensive genome exploration of clostridium botulinum group III field strains. Microorganisms 9 (11), 2347. doi: 10.3390/microorganisms9112347
Friend M., McLean R. R., Dein F. J. (2001). Disease emergence in birds: challenges for the twenty-first century. Auk 118, 290–303. doi: 10.1093/auk/118.2.290
Gelman A., Carlin J. B., Stern H. S., Dunson D. B., Vehtari A., Rubin D. B. (2014). Bayesian Data analysis third edition. Chapman & Hall/CRC Texts in Statistical Science 3, 677. doi: 10.1201/b16018
Haagsma J. (1973). The etiology and epidemiology of botulism in waterfowl in The Netherlands. Hydrobiological Bull. 7, 96–105. doi: 10.1007/BF02275610
Hansbauer E. M., Skiba M., Endermann T., Weisemann J., Stern D., Dorner M. B., et al. (2016). Detection, differentiation, and identification of botulinum neurotoxin serotypes C, CD, D, and DC by highly specific immunoassays and mass spectrometry. The Analyst 141 (18), 5281–5297. doi: 10.1039/c6an00693k
Hardy S. P., Kaldhusdal M. (2013). Type c and C/D toxigenic clostridium botulinum is not normally present in the intestine of healthy broilers. Veterinary Microbiol. 165 (3–4), 466–468. doi: 10.1016/j.vetmic.2013.03.022
Hertel M. (2020). “Avian botulism kills 40,000 birds at national wildlife refuge,” in The revelator. (San Francisco: Audubon California). Available at: https://therevelator:org/avian-botulism-klamath/.
Holmes P. (2019). "Avian botulism - a recurring paralytic disease of wild UK waterbirds. Veterinary Rec. 185 (9), 261–262. doi: 10.1136/vr.l5417
Hyun S. H., Sakaguchi G. (1989). "Implication of coprophagy in pathogenesis of chicken botulism." nihon juigaku zasshi. Japanese J. veterinary Sci. 51 (3), 582–586. doi: 10.1292/jvms1939.51.582
Lan Chun C., Kahn C. I., Borchert A. J., Byappanahalli M. N., Whitman R. L., Peller J., et al. (2015). Prevalence of toxin-producing clostridium botulinum associated with the macroalga cladophora in three great lakes: growth and management. Sci. Total Environ. 511, 523–529. doi: 10.1016/j.scitotenv.2014.12.080
Le Gratiet T., Poëzevara T., Rouxel S., Houard E., Mazuet C., Chemaly M., et al. (2020). Development of an innovative and quick method for the isolation of clostridium botulinum strains involved in avian botulism outbreaks. Toxins 12 (1), 42. doi: 10.3390/toxins12010042
Le Maréchal C., Ballan V., Rouxel S., Bayon-Auboyer M. H., Baudouard M. A., Morvan H., et al. (2016). Livers provide a reliable matrix for real-time PCR confirmation of avian botulism. Anaerobe 38, 7–13. doi: 10.1016/j.anaerobe.2015.10.014
Le Maréchal C., Hulin O., Macé S., Chuzeville C., Rouxel S., Poezevara T., et al. (2019). A case report of a botulism outbreak in beef cattle due to the contamination of wheat by a roaming cat carcass: From the suspicion to the management of the outbreak. Animals 9 (12), 1025. doi: 10.3390/ani9121025
Le Maréchal C., Rouxel S., Ballan V., Houard E., Poezevara T., Bayon-Auboyer M. H., et al. (2017). "Development and validation of a new reliable method for the diagnosis of avian botulism." edited by G. F. Browning. PloS One 12 (1), e0169640. doi: 10.1371/journal.pone.0169640
Masters A. M., Palmer D. G. (2021). Confirmation of botulism diagnosis in Australian bird samples by ELISA and RT RtPCR. J. Veterinary Diagn. Invest. 33, 104063872110144. doi: 10.1177/10406387211014486
Matveev K. I., Konstantinova N. D. (1974). The role played by mirating birds in the distribution of the botulism agent. Hygiene Sanitation 12, 91–92.
Miyazaki S., Sakaguchi G. (1978). Experimental botulism in chickens: the cecum as the site of production and absorption of botulinum toxin. Jpn J. Med. Sci. Biol. 31 (1), 1–15. doi: 10.7883/yoken1952.31.1
Nakamura K., Kohda T., Umeda K., Yamamoto H., Mukamoto M., Kozaki S. (2010). Characterization of the D/C mosaic neurotoxin produced by clostridium botulinum associated with bovine botulism in Japan. Vet. Microbiol. 140 (1-2), 147–154. doi: 10.1016/j.vetmic.2009.07.023
Popoff M. R. (1989). Revue sur l'épidémiologie du botulisme bovin en France et analyse de sa relation avec les élevages de volailles. Rev. scientifique technique 8 (1), 129–145. doi: 10.20506/rst.8.1.404
Rasetti-Escargueil C., Lemichez E., Popoff M. R. (2019). Public health risk associated with botulism as foodborne zoonoses. Toxins 12 (1), 17. doi: 10.3390/toxins12010017
Reed T. M., Rocke T. E. (1992). The role of avian carcasses in botulism epizootics. Wildlife Soc. Bull. 20 1973–2006, 175–182. http://www.jstor.org/stable/3782892.
Reynolds M., Kyoko J., Schvaneveldt E., Dewey D., Uyehara K. J., S.C. H. (2021). Efficacy of detection canines for avian botulism surveillance and mitigation. Conserv. Sci. Pract. 3. doi: 10.1111/csp2.397
Rocke T. E. (2006). “The global importance of avian botulism,” in Waterbirds around the world. Eds. Boere G. C., Galbraith C. A., Stroud D. A. (Edinburgh, UK: The Stationery Office), 422–426.
Rocke T. E., Bollinger T. K. (2007). Avian botulism." infectious diseases of wild birds. Eds. Thomas N. J., Hunter D. B., Atkinson C. T. (Oxford, UK: Blackwell Publishing Ltd), 377–416.
Rocke T. E., Nol P., Pelizza C., Sturm K. (2004). Type c botulism in pelicans and other fish-eating birds at the salton Sea. Stud. Avian Biol. 27, 137–140.
Rogers K. H., Gonzales Viera O., Uzal F. A., Peronne L., Mete A. (2021). Mortality of Western gulls (Larus occidentalis) associated with botulism type a in coastal southern California, USA. J. Wildlife Dis. 57 (3), 657–661. doi: 10.7589/JWD-D-20-00153
Rosciano N. G., Cossa N. A., Farace M. I., Ojeda V., Seijas S. (2021). Outbreak of type c botulism in aquatic birds on the nahuel huapi lake and national park area, Argentina. Hornero 36 (1), 71–80.
Russell I. A., Randall R. M., Zimmerman D., Govender D. (2019). Outbreak of avian botulism and its effect on waterbirds in the wilderness lakes, south Africa. KOEDOE - Afr. Protected Area Conserv. Sci. 61 (1). doi: 10.4102/koedoe.v61i1.1553
Shutt J. L., Andrews D. W., Weseloh D. V. C., Moore D. J., Hebert C. E., Campbell G. D., et al. (2014). The importance of island surveys in documenting disease-related mortality and botulism e in great lakes colonial waterbirds. J. Great Lakes Res. 40, 58–63. doi: 10.1016/j.jglr.2014.01.001
Skarin H., Hafström T., Westerberg J., Segerman B. (2011). Clostridium botulinum group III: A group with dual identity shaped by plasmids, phages and mobile elements. BMC Genomics 12. doi: 10.1186/1471-2164-12-185
Skarin H., Lindgren Y., Jansson D. S. (2015). Investigations into an outbreak of botulism caused by clostridium botulinum type C/D in laying hens. Avian Dis. 59 (2), 335–340. doi: 10.1637/10861-051214-Case
Son K., Kim Y. K., Woo C., Wang S. J., Kim Y., Oem J. K., et al. (2018). Minimizing an outbreak of avian botulism (Clostridium botulinum type c) in incheon, south Korea. J. Veterinary Med. Sci. 80 (3), 553–556. doi: 10.1292/jvms.17-0519
Souillard R., Grosjean D., Le Gratiet T., Poezevara T., Rouxel S., Balaine L., et al. (2021). Asymptomatic carriage of c. botulinum type D/C in broiler flocks as the source of contamination of a massive botulism outbreak on a dairy cattle farm. Front. Microbiol. 12. doi: 10.3389/fmicb.2021.679377
Takeda M., Tsukamoto K., Kohda T., Matsui M., Mukamoto M., Kozaki S., et al. (2005). Characterization of the neurotoxin produced by isolates associated with avian botulism. Avian Dis 49, 376–381. doi: 10.1637/7347-022305R1.1
Ventujol A., Decors A., Le Maréchal C., Toux J. Y., Allain V., Mazuet C., et al. (2017). Le botulisme aviaire en France : étude des cas signalés dans la faune sauvage et dans les élevages par deux réseaux de surveillance entre 2000 et 2013. Epidémiol. et santé anim 72, 857–102.
Vidal D., Anza I., Taggart M. A., Perez-Ramirez E., Crespo E., Hofle U., et al. (2013). Environmental factors influencing the prevalence of a clostridium botulinum type C/D mosaic strain in nonpermanent Mediterranean wetlands. Appl. Environ. Microbiol. 79 (14), 4264–4271. doi: 10.1128/AEM.01191-13
Wobeser G. (1997). Avian botulism–another perspective. J. wildlife Dis. 33 (2), 181–186. doi: 10.7589/0090-3558-33.2.181
Keywords: Clostridium botulinum, waterfowl, botulism, prevalence, bioavailability
Citation: Meloni E, Le Maréchal C, Millot F, Payne A, Calenge C, Mazuet C, Chemaly M, Rouxel S, Poezevara T, Avouac A, Plaquin B, Guillemain M, Richomme C and Decors A (2023) Exposure of waterfowl to Clostridium botulinum in France. Front. Conserv. Sci. 4:1011555. doi: 10.3389/fcosc.2023.1011555
Received: 17 August 2022; Accepted: 10 January 2023;
Published: 25 January 2023.
Edited by:
Mario Diaz, Spanish National Research Council (CSIC), SpainReviewed by:
Andrea Bogomolni, University of Massachusetts Boston, United StatesShannon Dundas, NSW Government, Australia
Copyright © 2023 Meloni, Le Maréchal, Millot, Payne, Calenge, Mazuet, Chemaly, Rouxel, Poezevara, Avouac, Plaquin, Guillemain, Richomme and Decors. This is an open-access article distributed under the terms of the Creative Commons Attribution License (CC BY). The use, distribution or reproduction in other forums is permitted, provided the original author(s) and the copyright owner(s) are credited and that the original publication in this journal is cited, in accordance with accepted academic practice. No use, distribution or reproduction is permitted which does not comply with these terms.
*Correspondence: Emma Meloni, emma.meloni@ofb.gouv.fr