Corrigendum: Seed sourcing strategies for ecological restoration under climate change: A review of the current literature
- 1Negaunee Center for Plant Conservation Science and Action, Chicago Botanic Garden, Glencoe IL, United States
- 2Department of Natural Resources, Lake County Forest Preserve District, Libertyville, IL, United States
- 3Program in Plant Biology and Conservation, Northwestern University, Evanston, IL, United States
- 4Conservation Department, Native Plant Trust, Framingham, MA, United States
- 5The Wetlands Initiative, Chicago, IL, United States
- 6Pollinator Program, The Xerces Society for Invertebrate Conservation, Portland, OR, United States
- 7Resource Management, Forest Preserves of Cook County, River Forest, IL, United States
- 8Keller Science Action Center, The Field Museum, Chicago, IL, United States
Climate change continues to alter the seasonal timing and extremes of global temperature and precipitation patterns. These departures from historic conditions along with the predicted variability of future climates present a challenge to seed sourcing, or provenance strategy decisions, within the practice of ecological restoration. The “local is best” for seed sourcing paradigm is predicated upon the assumption that ecotypes are genetically adapted to their local environment. However, local adaptations are potentially being outpaced by climate change, and the ability of plant populations to naturally migrate or shift their distribution accordingly may be limited by habitat fragmentation. Restoration practitioners and natural area managers have a general understanding of the importance of matching the inherent adaptations of source populations with the current and/or future site conditions where those seeds or propagules are planted. However, for many species used in seed-based restoration, there is a lack of empirical evidence to guide seed sourcing decisions, which are critical for the longevity and ecological function of restored natural communities. With the goal of characterizing, synthesizing, and applying experimental research to guide restoration practice, we conducted a systematic review of the literature on provenance testing of taxa undertaken to inform seed sourcing strategies for climate resiliency. We found a strong bias in the choice of study organism: most studies have been conducted on tree species. We also found a strong bias regarding where this research has been conducted, with North America (52%) and Europe (31%) overrepresented. Experiments were designed to assess how propagule origin influences performance across both climatic (26%) and geographic (15%) distance, with some studies focused on determining how climate normal conditions (39%) impacted performance related to survivorship, growth and other parameters. We describe the patterns and gaps our review identified, highlight specific topics which require further research, and provide practical suggestions of immediate and longer-term tools that restoration practitioners can use to guide and build resilient natural communities under future climate scenarios.
Introduction
Climate change is altering both biotic communities and abiotic environments globally (Parmesan, 2006; Diffenbaugh et al., 2018), with the potential to disrupt locally adapted populations and drive new patterns of dispersal (Anderson and Wadgymar, 2020). Cumulatively, this is likely to result in changes in species distribution and community composition (Löf et al., 2019) at landscape scales. The impact of climate change on the study and practice of restoration has been extensively discussed (e.g., Hobbs et al., 2006; Corlett, 2016). For example, climate change may disrupt community composition in such a way that systems may retain only some historic components or may be completely novel, meaning that intact reference habitats will not be available to guide our restoration and management plans (Hobbs et al., 2009). Not only is the practice of restoration challenged by climate change, but restoration itself is now considered to be an important tool to combat its effects (Brancalion and Holl, 2020, Simonson et al., 2021).
For many ecosystems, the vegetative community is fundamental, and seed augmentation is a critical component of restoration (Breed et al., 2018; Gann et al., 2019). Indeed, a primary consideration in undertaking management decisions to ensure the climate resiliency of natural areas, and the native species that comprise them, is the sourcing of propagules for restoration, reforestation, and rare species reintroduction efforts (Houseal and Smith, 2000; Havens et al., 2015; Török et al., 2021). Most restoration projects rely on seed addition during initial phases to establish a new habitat or during later phases to augment community diversity. Restoration seedings are undoubtedly impacted by climate change. For example, changes in soil water availability and temperature with climate change are likely to influence the success of seedling establishment. (Muñoz-Rojas et al., 2016; Groves and Brudvig, 2018). Despite its importance to the successful outcome of restoration projects, seed procurement is largely dependent upon local and regional availability; both the species diversity and volume of seed available frequently fail to meet the needs of land managers (Houseal and Smith, 2000; White et al., 2018; Camhi et al., 2019; Oldfield, 2019; Harrison et al., 2020; Barak et al., 2021; Török et al., 2021).
Traditional restoration practice has operated under the assumption that seed sourced from wild populations near the project location ensures the greatest chance for successful establishment and growth (Aitken and Bemmels, 2016; Breed et al., 2018; Risk et al., 2021). This paradigm is often referred to as the “local is best” approach (Hancock and Hughes, 2014; Reiker et al., 2015; Breed et al., 2018). However, provenance guidelines may vary regionally or across different land management agencies (Saari and Glisson, 2012). The relevance of a local provenance strategy is challenged when practitioners attempt to design seed mixes with long-term climate resiliency in mind (Hancock and Hughes, 2014; Reiker et al., 2015; Breed et al., 2018), especially when evidence for local adaptation across multiple plant traits is lacking for at least some species within a region (Giencke et al., 2018). This had led to recommendations for strategies that could be useful in sourcing climate resilient seed such as: establishing a supply chain for source identified seed to aid in seed mix design (Havens et al., 2015), sourcing that is biased towards the predicted direction of climate change (Havens et al., 2015; Prober et al., 2015), using regional or other mixed provenance strategies to increase genetic diversity and the potential for adaptation (Prober et al., 2011; Bucharova et al., 2019) and the use of species distribution modeling to gain insight into both the direction and distance from which to source seeds for any given project or region (Ramalho et al., 2017). Taken as a whole, however, resilient seed will likely be from sources that closely match the conditions of the restoration site, currently and into the future, regardless of the geographic distance between project site and seed source (Vitt et al., 2010), but this relationship may be species-specific (Rushing et al., 2021).
Many restoration practitioners and land management agencies recognize and likely already incorporate the general observation that plant species ranges will shift poleward in latitude and upward in elevation (Etterson, 2004a; Parmesan and Hanley, 2015; Anderson and Wadgymar, 2020) in their seed sourcing decisions and in the design of their seed mixes (Figure 1). However, there is a dearth of knowledge about sourcing plant materials for any given species, locale, or project as climate change raises questions about where species may thrive in the future (Löf et al., 2019), and the magnitude and timeframe of change is uncertain (Diffenbaugh et al., 2018). Phenological mismatches may complicate seed sourcing decisions even more, as they can result in low fecundity (Etterson, 2004a), driven by the failure of fruit to mature, failure of seasonally appropriate bud or leaf expansion, or failure to flower (Anderson and Wadgymar, 2020). The demographic consequences of poor seed sourcing decisions may remain obscured in the early stages of a restoration, when seed germination, seedling establishment, and high relative growth rates are paramount to restoration success (James et al., 2011; Larson and Funk, 2016). Once established, however, population dynamics of longer-lived species may be buffered against climate change, relative to short-lived ones (Morris et al., 2008; Török et al., 2021), thus appearing more resilient in the near-term.
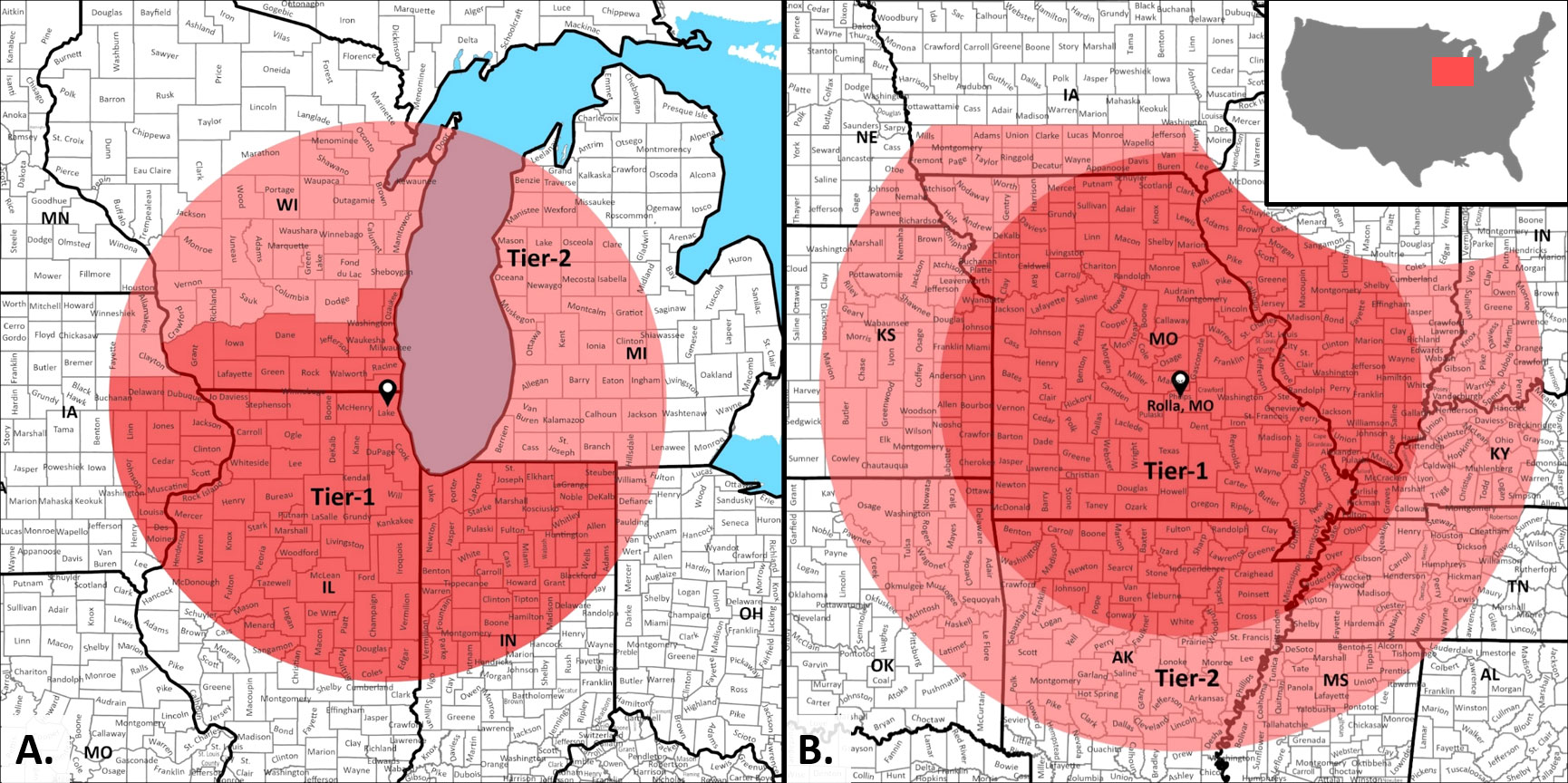
Figure 1 Seed procurement specifications from Lake County Forest Preserve District (LCFPD), Illinois, USA. (A) Standard seed sourcing specification used during the bidding process is biased for seed regionally produced south of Lake County, Illinois which is indicated by the map centroid. The District has a two-tier seed provenance system for purchase consideration of native plant material. Seed sourced by vendors from Tier 1 - (Deep Red) is given priority over seed sourced from Tier 2 (Light Red) during purchasing decisions. (B) Lake County Forest Preserve District has undertaken a test of climate predictive provenancing, with updated Tiers 1 and 2 that were determined by applying Species Distribution Models of multiple species used in standard restoration of abandoned agricultural lands. Overlapping regions between the standard seed provenance (A) and the predictive provenance are cut out.
We undertook this study to establish the “state of the science” and thus to provide a systematic update to the general recommendations regarding seed sourcing strategies to explicitly address climate change. We wanted to determine how sourcing decisions under climate change are likely to alter the performance of seed during the establishment phase of a restoration, as well as during later stages when community composition and structure may overshadow the effects of the population dynamics of individual species. We also wanted to explore if any performance or fitness patterns could be discerned from the literature that might inform the development of specific recommendations for sourcing seed that would provide more predictable outcomes as the climate changes. We were particularly interested in summarizing the literature around climate resilient seed sourcing so that we could make specific recommendations for land managers and other practitioners that go beyond provisional seed transfer zones (Bower et al., 2014).
We conducted a review of the literature for studies that had explicit or potential application for seed sourcing under climate change. We sought out experimental studies that assessed differences in performance of plants collected from different populations, i.e., studies that focused on evaluating different seed provenances, and analyzed plant responses to climate either implicitly or explicitly across multiple provenances. We had five primary objectives for this review of the literature. We sought to determine:
1. The range of species commonly included in these studies, as well as their life histories (e.g., were trees or long-lived herbaceous perennials common subjects of research)?.
2. The context in which these studies had been conducted (e.g., continent, country, and habitat type).
3. The experimental design of these studies, including the types of experiments used (e.g., common garden experiments, or if genetic effects such as maternal effects, plasticity or heritability were considered), and the duration of each study. In addition, we were interested in how researchers addressed the effects of climate change (e.g., did studies use actual climate variables such as mean annual temperature or mean annual rainfall as predictors, or did they use “proxy” variables that are strongly correlated with climate such as elevation or latitude).
4. The range of response variables studied, and which, if any, performance or fitness variables could be used to measure a successful “match” between seed origin and the geography of the restoration project.
5. The motivation for the studies (e.g., restoration, assisted migration or assisted gene flow, commercial forestry), and whether studies were explicitly designed to assess seed sourcing. We were also interested in determining which funding sources supported the work.
As a practical synthesis and application of the above objectives, we wanted to use our review of the literature to understand - and work towards bridging - the gap between the research regarding how plants respond to climate change and the practice of sourcing seed for restoration. To this end, we provide recommendations in the Discussion.
Methods
To understand the current state of the science with regard to seed sourcing under climate change, we performed a comprehensive review of the literature and subsequent analysis.
Literature search
To compile a list of studies that test seed provenances, we conducted a total of nine searches in February 2021 on Web of Science using combinations of keywords including “seed sourcing,” “restoration,” and “climate change.” This process returned 2442 unique titles; 120 titles were returned in three or more of these nine search word combinations. Twelve references were incomplete and couldn’t be located during specific title searches and were therefore discarded. The resulting bibliography was uploaded into Rayyan (https://rayyan.ai), an online tool designed to provide a collaborative platform during the title/abstract screening phases of the review process that allows multiple reviewers to vote for inclusion or exclusion in their study. We utilized the available tools to ensure that no reviewer was able to see the recommendations or comments of any other reviewer (i.e.: the review was “blind”. Each title and abstract of the remaining references were reviewed by at least two randomly assigned reviewers to determine relevance to our primary question of seed sourcing under climate change; a subset was reviewed by more than two of the authors because of the blind anonymous review process. Of the papers thus evaluated, 182 were considered germane by all reviewers, while 1770 titles were excluded by all reviewers. One hundred seventy-three titles (173) required further review of the full text to determine relevance and the reviewers were conflicted on the remaining 305 titles. To reconcile conflicts among reviewers with regards to these titles, two additional reviewers conducted a subsequent blind review of these titles and their abstracts to determine if the studies were relevant. Relevant studies were defined as those that evaluated plant materials (seeds and/or seedlings) from more than one source or origin (provenance) and that did so under experimental conditions where these materials were planted in a common environment such that phenotypic or performance traits could be objectively measured. We included studies that were conducted in greenhouses, growth chambers, or in field conditions and that were designed as common garden studies and/or reciprocal transplants. Ultimately, 378 references were determined to be relevant to our question about seed sourcing under climate change and were included in the next step of the review (Figure 2).
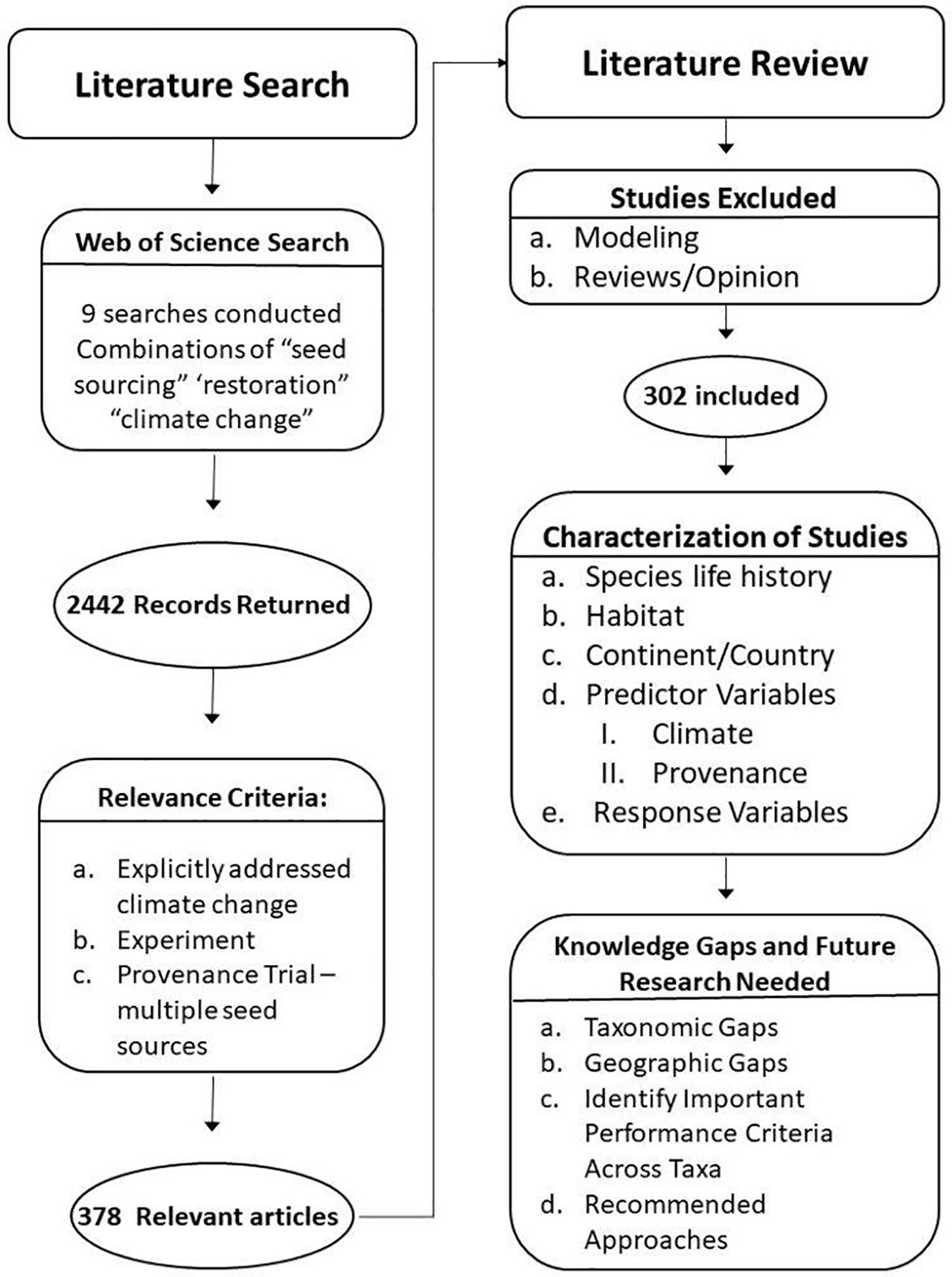
Figure 2 Process flow diagram illustrating literature search, review, and characterization of studies.
Literature review
Of the 378 articles that were deemed relevant, further review of the abstracts and methods revealed that 63 were reviews of the issues or otherwise provided background information important to consideration of our topic, and 13 were papers that used modeling approaches. Therefore, a total of 302 papers appeared to be experiments related to provenance testing using reciprocal transplants, common garden studies, or other field-based or greenhouse trials designed to determine how the origin of a seed collection performs in an environment outside of the one in which it naturally occurs (Figure 2).
Characterization of the literature reviewed
Each of the 302 papers was randomly assigned to one reviewer, who undertook an initial screening to determine if the paper met the following criteria: An experimental study regarding the performance or fitness of more than one origin or source of plant material, i.e.: more than one “provenance.” Non-experimental studies including review, opinion/perspective, modeling, and methods papers that had not been previously filtered out were excluded in this screening phase and did not undergo further review. Data collection on the included papers was iterative and took place in two phases.
Included papers were subsequently assessed for the inclusion of terms and/or concepts indicated in Table 1; the data were gathered and managed via Google Forms. To assist our evaluations, we developed a rubric for terminology that was applied inconsistently across the literature we reviewed. Maternal effects were constrained to papers in which individual maternal lines (e.g., a single parent plant) were followed from seed collection through to experimental set-up and were tracked during the measurements of response variables. To account for plasticity, an experiment needed to assess at least half-sib families and to follow clones or siblings in multiple environments so that a single genetic signature could be followed and measured with replication. Lastly, an experimental design that assessed heritability would require full sibs to be evaluated as well as measurements to be taken across different environments to determine the breadth of the expression of the genotype-by-environment interaction.
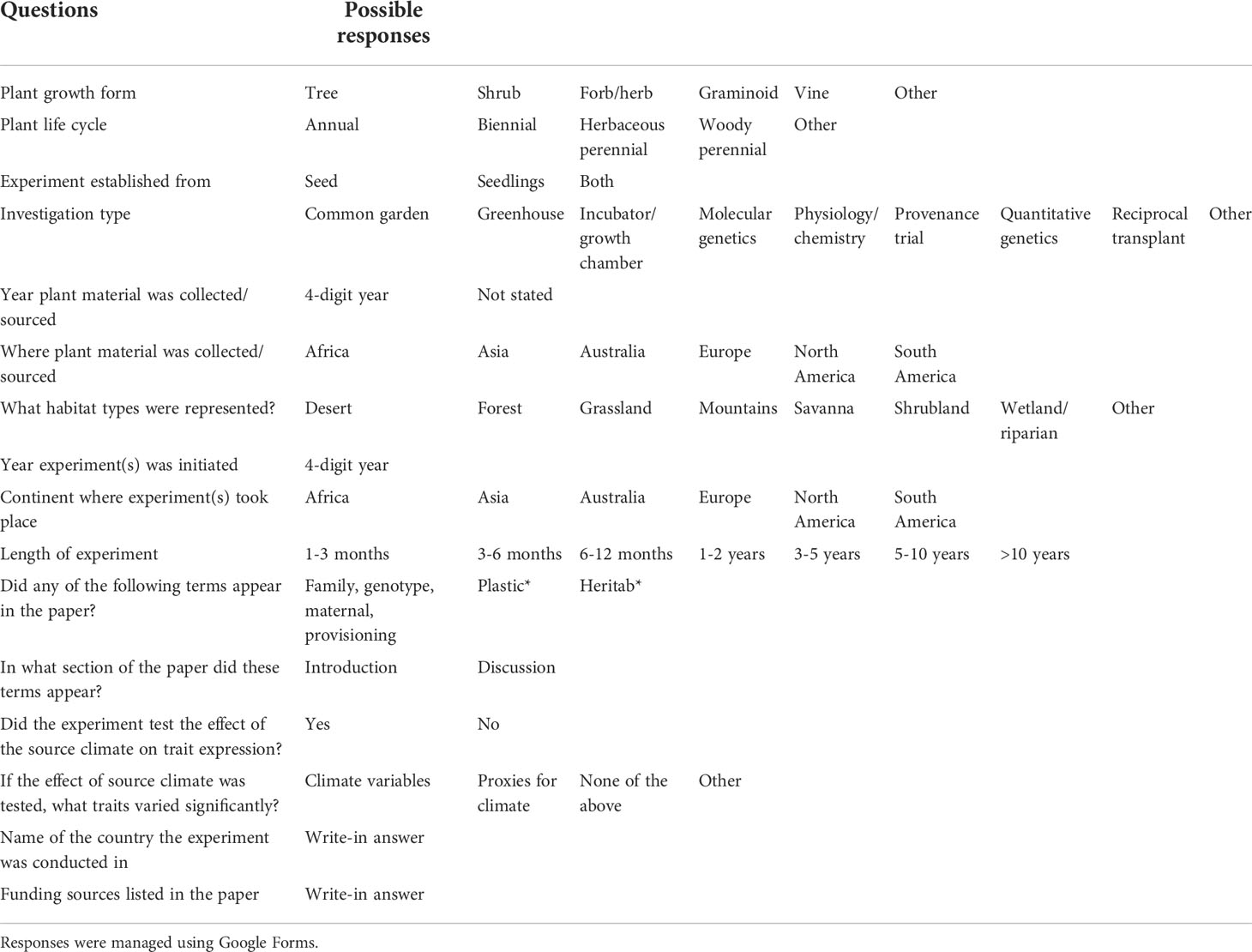
Table 1 Terms or concepts considered during the review, with their associated multiple-choice responses.
Although many studies acknowledged that genetic factors such as plasticity or heritability are important to consider, not all were specifically designed to account for them. To evaluate this discrepancy, we assessed 176 papers that were experimental papers relevant to our research questions (see Results below). We undertook keyword searches within the text of each paper for “maternal effects”, “plasticity”, “heritability”, and related terms (see Table 1). We were also interested in establishing if these effects were explicitly integrated into the experimental design and analysis (i.e., methods/results), or used as framing concepts (i.e., found only in the introduction or discussion, but not accounted for in the experimental design).
We determined whether the experimental design tested the effect of the source climate (climate where provenance was collected) on trait expression using one of the following variables: proxies for climate (e.g., provenance, latitude, elevation), climate variables (e.g., BIOCLIM variables, mean annual temperature etc.), none of the above, or other. If the experiment was determined to have tested the effect of source climate, reviewers listed the traits that varied significantly by source climate, including traits that varied significantly as an interaction with another model term. To further refine the geographic range of the studies represented in the literature, reviewers indicated the identity of the country or countries in which experiments took place.
Lastly, we compiled sources of funding listed in the acknowledgments. Each funding source was then categorized as follows: federal or national funding (e.g., a national agency or ministry); European Union (funding by EU commissions, or specific EU grants aimed at international collaboration); state or provincial funding (provided by the state/provincial governments); university-based funding sources (e.g., funding provided by university fellowships, or by specific departments or programs); industrial or commercial; non-profit organizations and foundations (including non-profit umbrella organizations that unite various stakeholders); indeterminate; and not listed.
Data analysis
We used the R environment for statistical computing, version 4.0.4 ‘Lost Library Book’ (R Core Team, 2021) to manipulate, summarize, and visualize data. Open-ended responses, and “other” responses were assigned to posteriori thematic categories by individual authors with design input, review, and approval from the authorship team. Visualizations were created with the following packages: forcats (Wickham, 2021), ggfittext (Wilkins, 2021a), ggmosaic (Jeppson et al., 2021), ggplot2 (Wickham, 2016), ggthemes (Arnold, 2021), tidyr (Wickham, 2021), treemapify (Wilkins, 2021b).
Results
Of the 302 papers screened through the literature review process, 124 were excluded during the data collection phase. These included 35 experimental papers that did not address our research questions, 42 modeling papers, 19 review papers, 18 opinion and perspective papers, and 5 methods papers. Five papers were excluded for miscellaneous reasons - e.g., poor experimental methods, etc. One hundred and seventy-six (176) studies were experimental papers relevant to our research questions and are included in the results.
Species and life histories
In the literature we reviewed, provenance trials on tree species have the earliest history, appearing in the scientific literature more than 60 years ago, and interest in tree species responses to climate change has greatly increased since the mid-2000’s (Figure 3). The number of studies on perennial herbaceous species, including grasses and forbs, also began to increase around the same time, but remains outpaced by published studies on tree species. Additionally, the length of time devoted to tree trials is much longer than for other growth forms (Figure 4A). Similarly plants with longer life histories, including woody perennials, have been studied more intensively than annual or biannual plants, (Figure 4B). The bias towards tree species is also reflected in the frequency with which journal titles were observed, as Forest Ecology and Management was the most frequently observed (Supplementary Figure S2). While the most-represented journals were tree centric, the journal Evolutionary Applications, which has a broader scope beyond forestry, was also frequently encountered among the papers we reviewed. Furthermore, while there were approximately 175 species included in the studies we reviewed, the ten most studied species were trees. The species that appeared most frequently in these studies were Picea abies (Norway spruce, found in 14 papers, 8%), and Picea glauca (white spruce, 7%), followed by Pinus sylvestris (Scots pine, 5%) and Pseudotsuga menziesii (Douglas fir, 5%), all in the Pinaceae family. Only one Angiosperm taxon was frequently observed, Fagus sylvatica (European beech, Fagaceae, 4%).
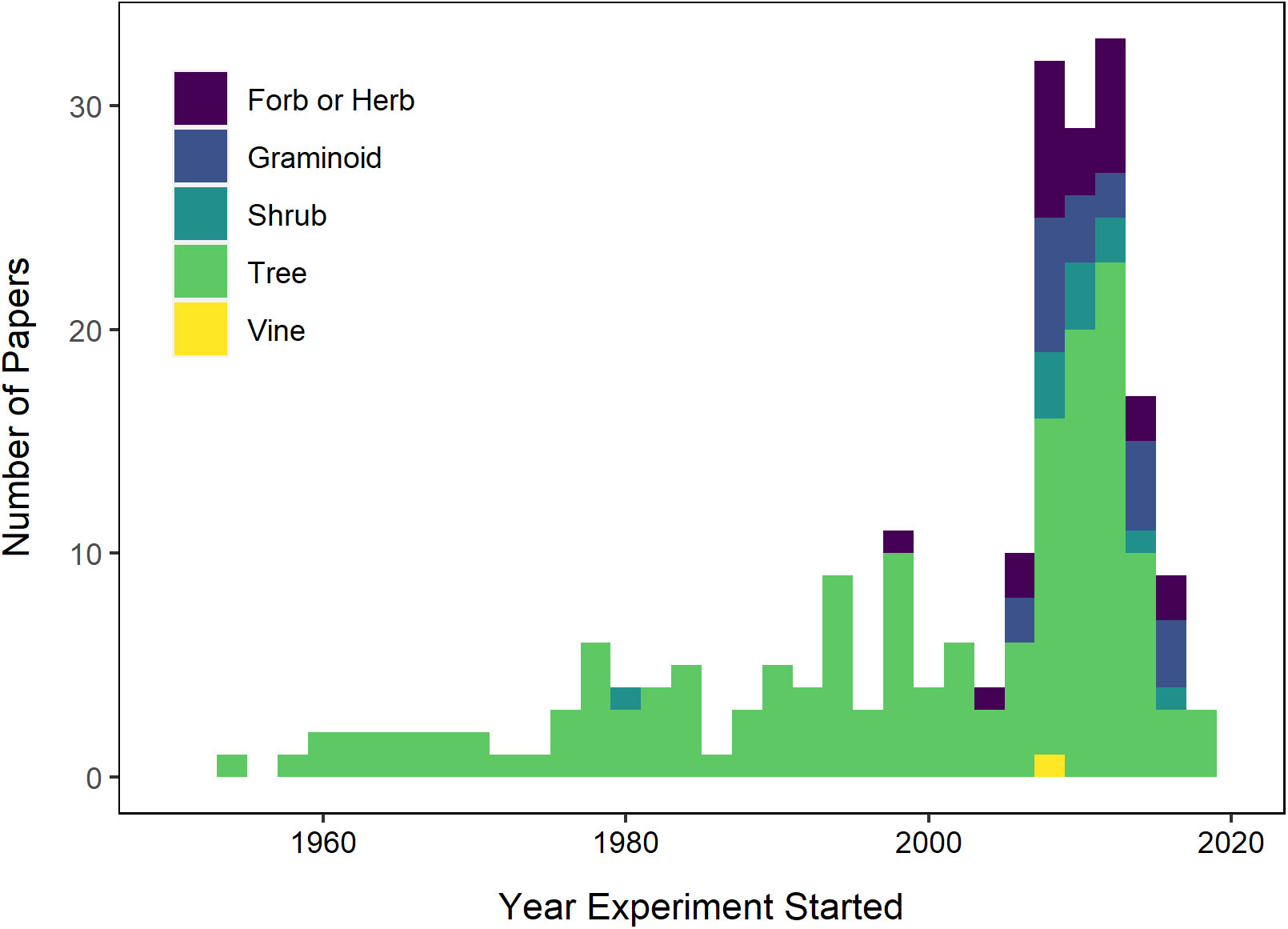
Figure 3 Histogram of included papers by plant growth form(s) examined and the year the experiment was initiated.
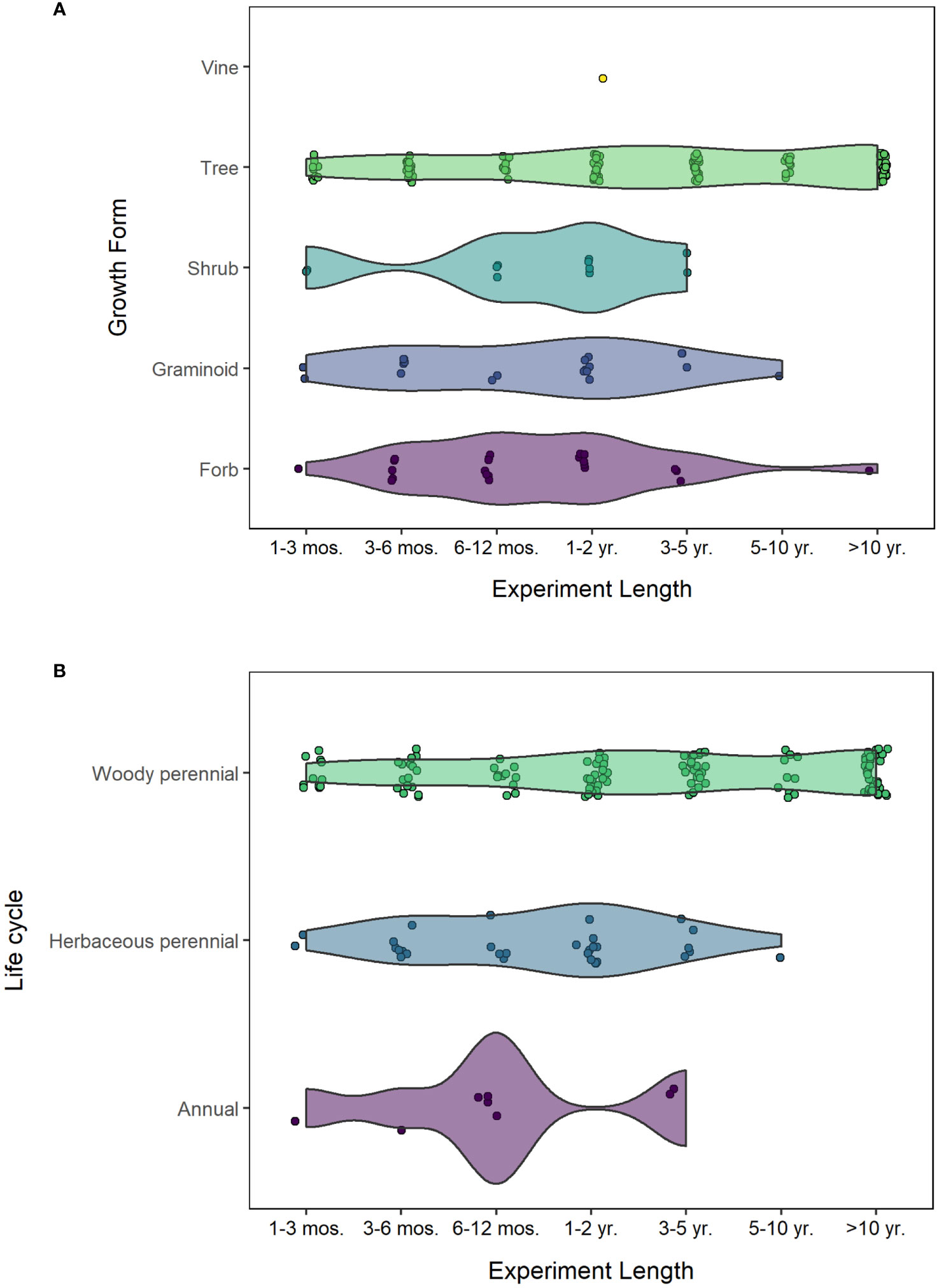
Figure 4 (A) Experiment length by plant growth form. Studies of forbs and graminoids are typically between 3 months and 1-2 years in length. Few are 3-5 years in length, and almost none are over 5 years. Whereas woody plants have good representation across experiment lengths (from 1-3 months through >10 years). This is consistent with documentation that many restorations are only tracked for the first few years, despite including many long-lived perennials (15+ years). (B) Number of studies by experiment length and plant life cycle. A single study of an herbaceous biennial is omitted from this visualization.
Geographic and habitat context
Most of the trials were conducted in North America (52%) or Europe (31%), with 41% of the studies conducted in the United States and Canada (Figure 5). Our search found very few studies from the South American (2%) and African (3%) continents (Figure 5). Most of the studies assessed species found in woodland habitats (68%), while species found in montane and grassland habitats were assessed in 17% and 16% of the papers, respectively (Figure 6).
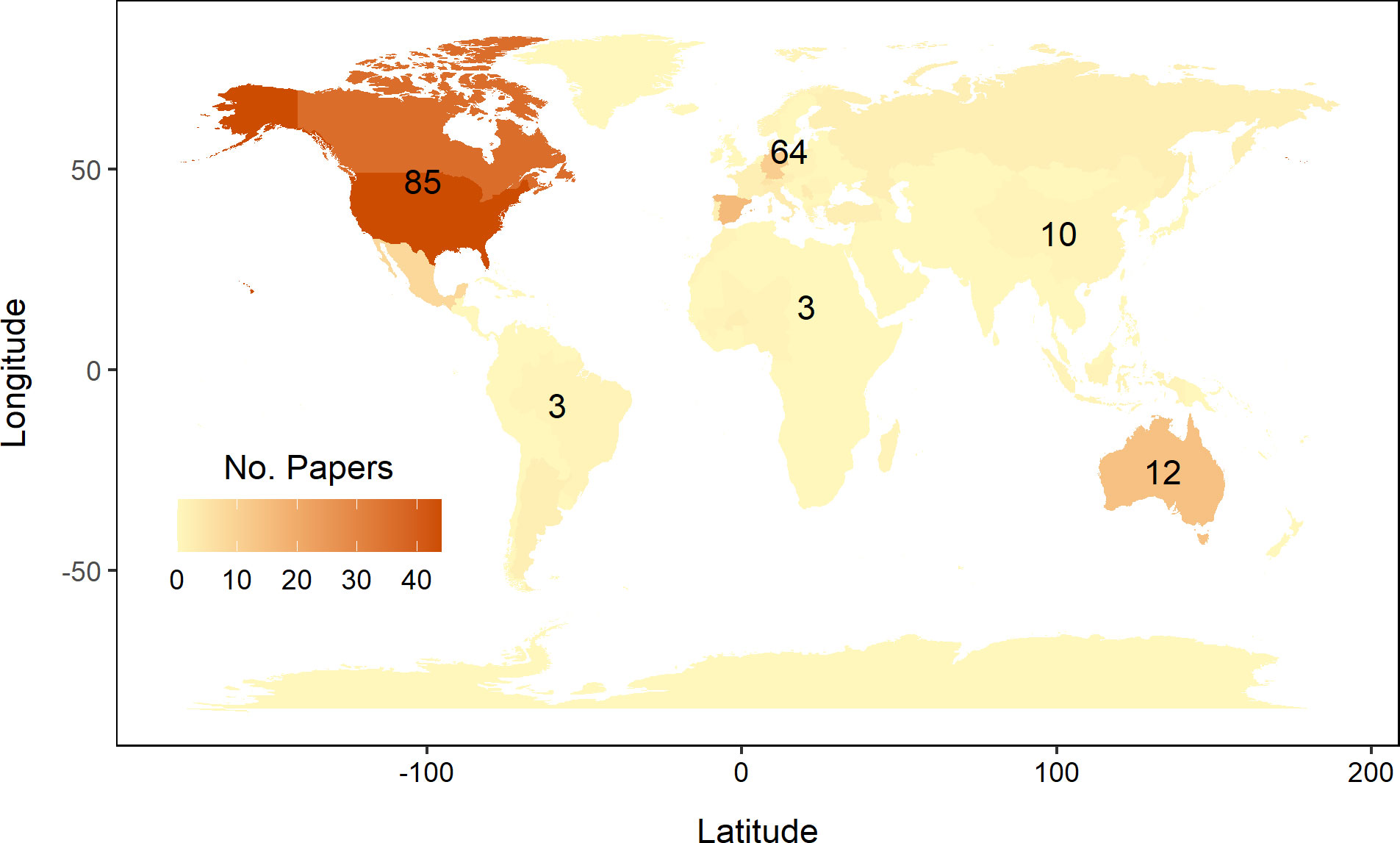
Figure 5 Indicates the number of studies by continent where plant material was sourced. The color shading of the countries indicates the number of studies conducted within each country. The majority of studies took place on the North American continent, with a greater number from the United States than from Canada. Countries represented by a single study are omitted from this visualization (n=31).
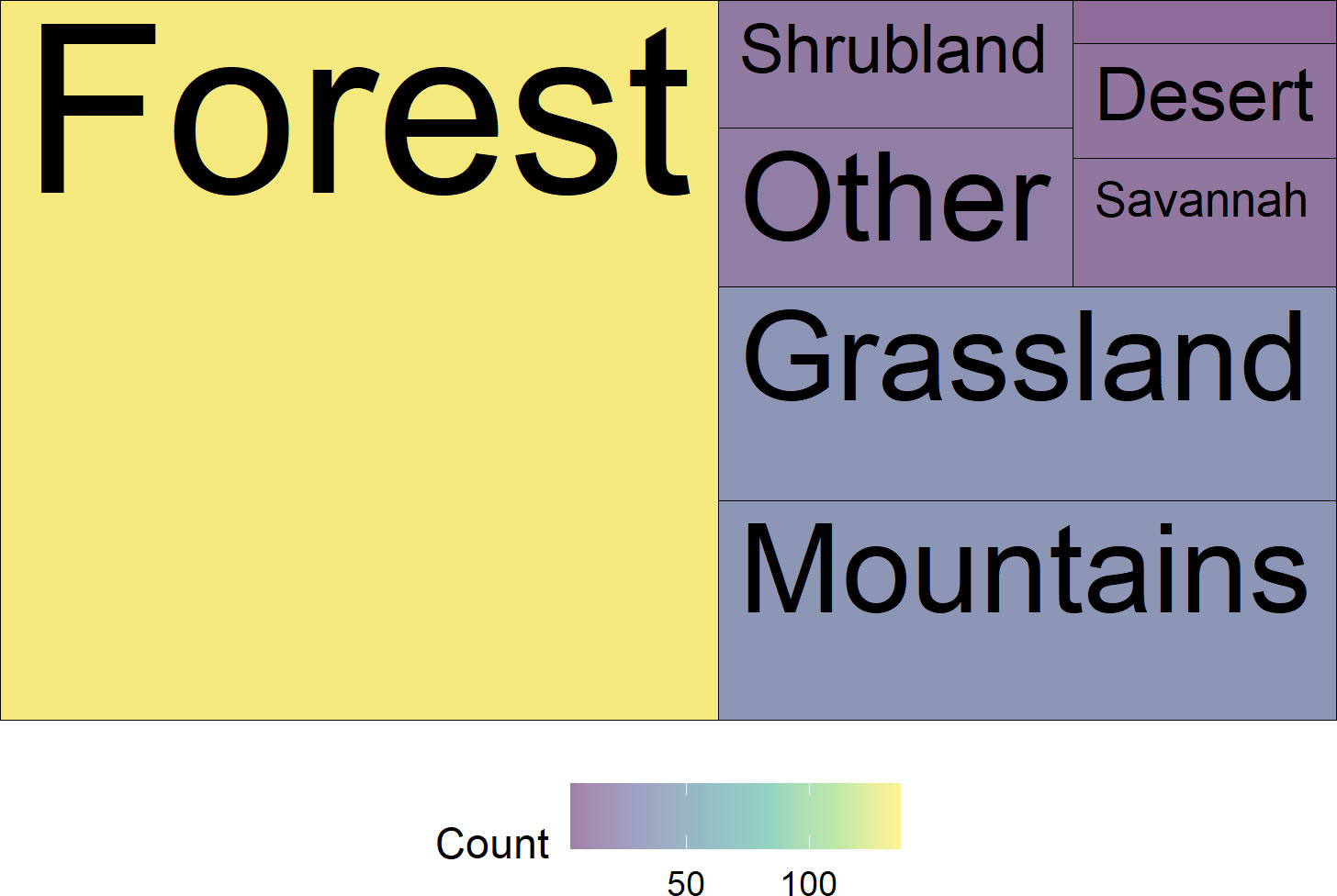
Figure 6 Treemap of the number of papers per habitat type. The label is omitted from the smallest category in the upper righthand corner, “Wetland and riparian” (n=3). “Other” includes categories such as “home gardens,” “coastal,” and “disturbed”.
Experimental design
Experiments were designed to assess how propagule origin influences performance across geographic distance (15.0%), or climatic distance (37%), or how the climate normal conditions decreased or increased performance relative to those found at the common garden or other study site outside of the origin (27%). Most of the studies regarding the performance of woody species utilized seedlings (51%) rather than seeds (25%), while studies on shorter-lived species, especially herbaceous perennials, used seeds with nearly the same frequency (37%; Figure 7).
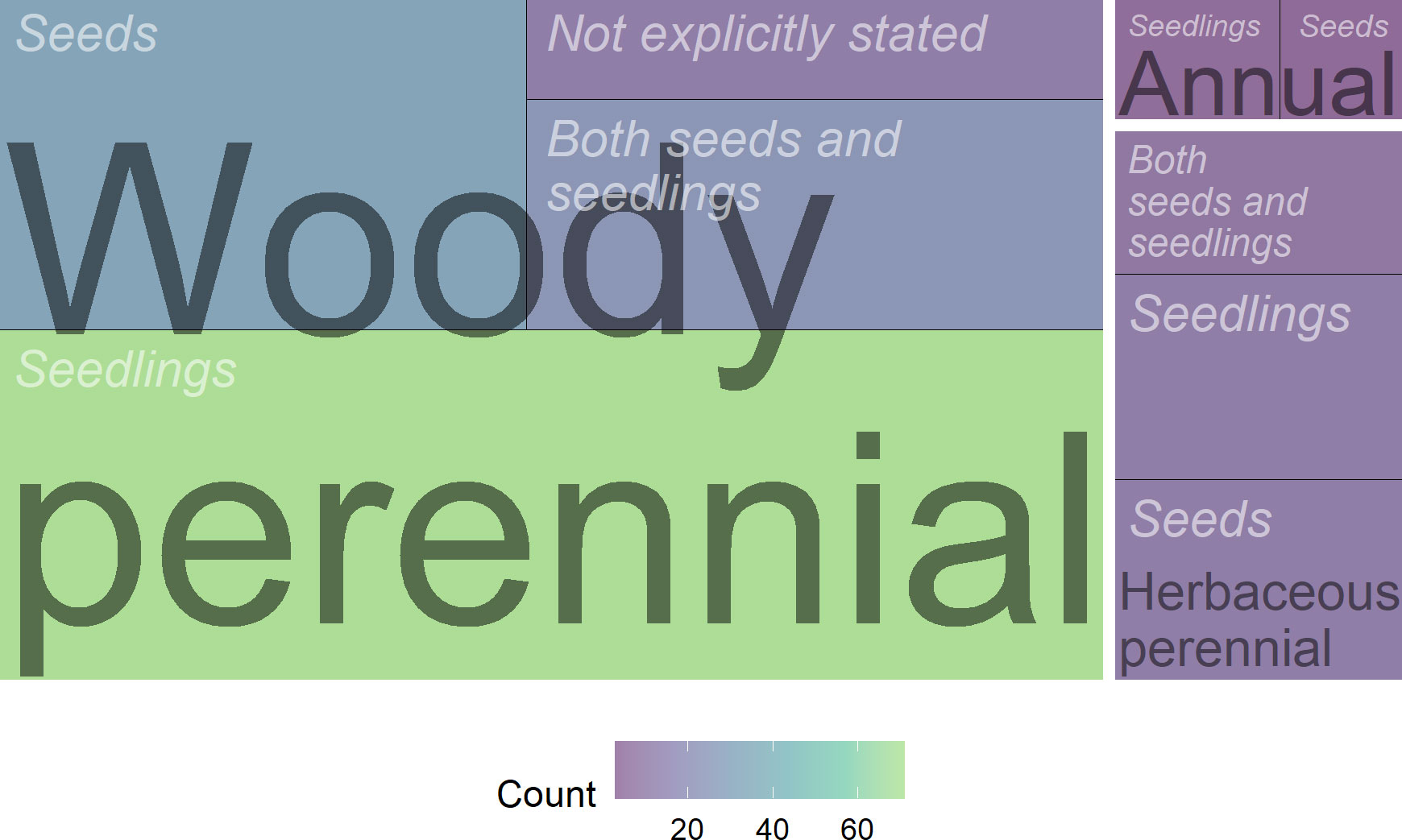
Figure 7 Treemap of the number of studies by source of plant material (seeds, seedlings, or both) and life cycle (woody perennial, herbaceous perennial, and annual). The sole study of a herbaceous biennial started using seeds is omitted from this visualization.
We also categorized the types of investigations used in these studies, noting that some papers reported results from more than one type of study. Two types of field experiments, common gardens (48%), and provenance trials (41%) were the most common experimental types. These were followed by incubator or growth chamber experiments (19%), greenhouse experiments (18%), reciprocal transplant field experiments (11%), quantitative (10%) and molecular (6%) genetics approaches, and studies of physiology and/or chemistry (4%). Fewer than 2% of papers included each of the following: nursery studies, lab experiments that were not specifically incubator experiments, in situ/observational studies, assisted migration studies, and field experiments of types not captured elsewhere, while 7% of studies included experiment types that did not fit into any of these categories.
Predictor variables
The most common predictor variables were provenance/site of origin (98% of studies), and experimental treatment/study site (53%) (Figure 8). Climate and geographic factors, such as climate normal of provenance origin (26%), geographic (15%) and climatic (15%) distance between collection and experimental sites, and elevation (8%) were also studied. Forty-eight percent (48%) of papers used proxies for climate such as provenance, latitude, and elevation as predictor variables in their analysis, while 41% of papers incorporated bioclimatic variables such as mean annual temperature, 38% included both types of variables, and 2% did not include variables of either type (Figure 9).
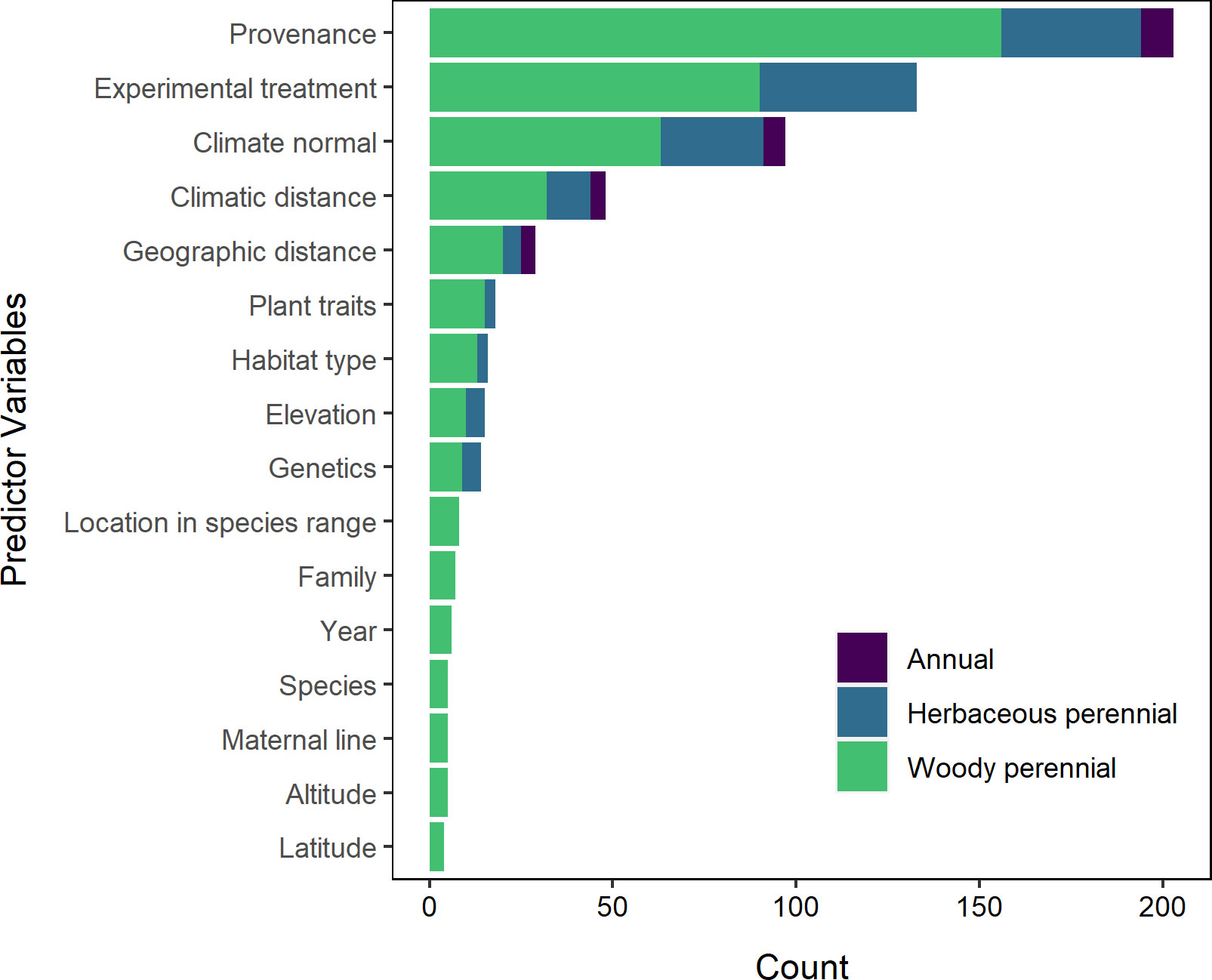
Figure 8 Predictor variables by plant life cycle. Predictors by life cycle pairs represented by two or fewer studies, as well as a single study of an herbaceous perennial, are omitted from this visualization. The most common predictor variables seen in statistical analyses were the provenance (or site of origin), followed by the experimental treatment or study site. However, this trend is largely driven by studies of woody perennials. Studies of woody perennials also appear to consider a greater diversity of predictor variables than studies of herbaceous perennials and annual plants.
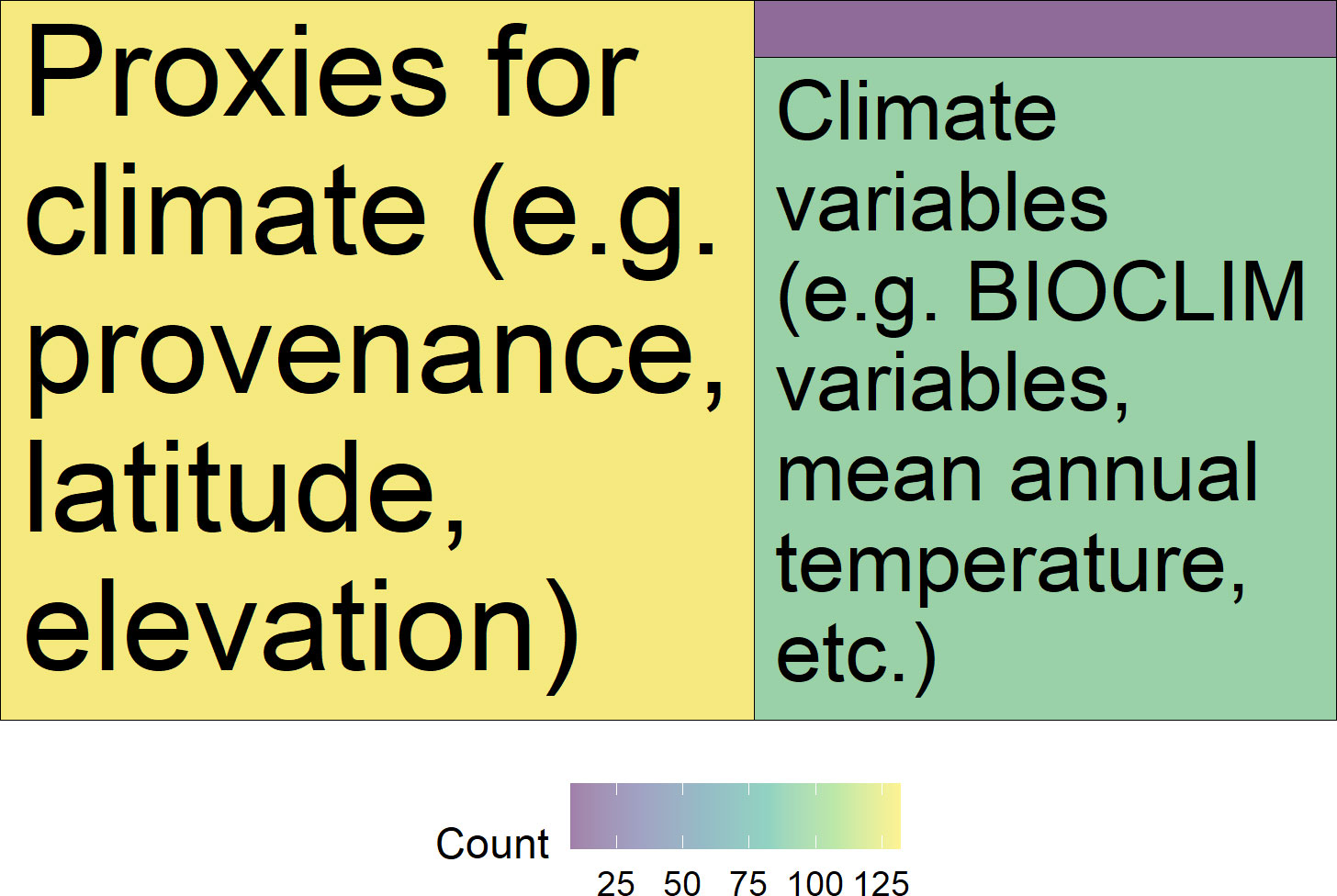
Figure 9 Treemap of the number of papers which modeled plant performance in response to source climate. The majority of studies included proxies for source climate (76%) or climate variables (54%) when modeling performance variation among provenances. Only 6% of studies did not include source climate or climate proxies in modeling plant performance by provenance.
Genetic considerations
While most of the papers assessed climate-related performance of a single taxon, approximately half (51%) did not control for or mention genetic effects, such as maternal effects, heritability, or plasticity, that might influence these traits depending upon the environment in which they are expressed and measured (Figure 10). Twenty-nine percent (29%) of the studies considered these genetic effects to frame the context of their experiment—i.e., they referred to them in either the introduction or discussion—but only 20% explicitly designed their experiments to account for or to measure these effects (Figure 10).
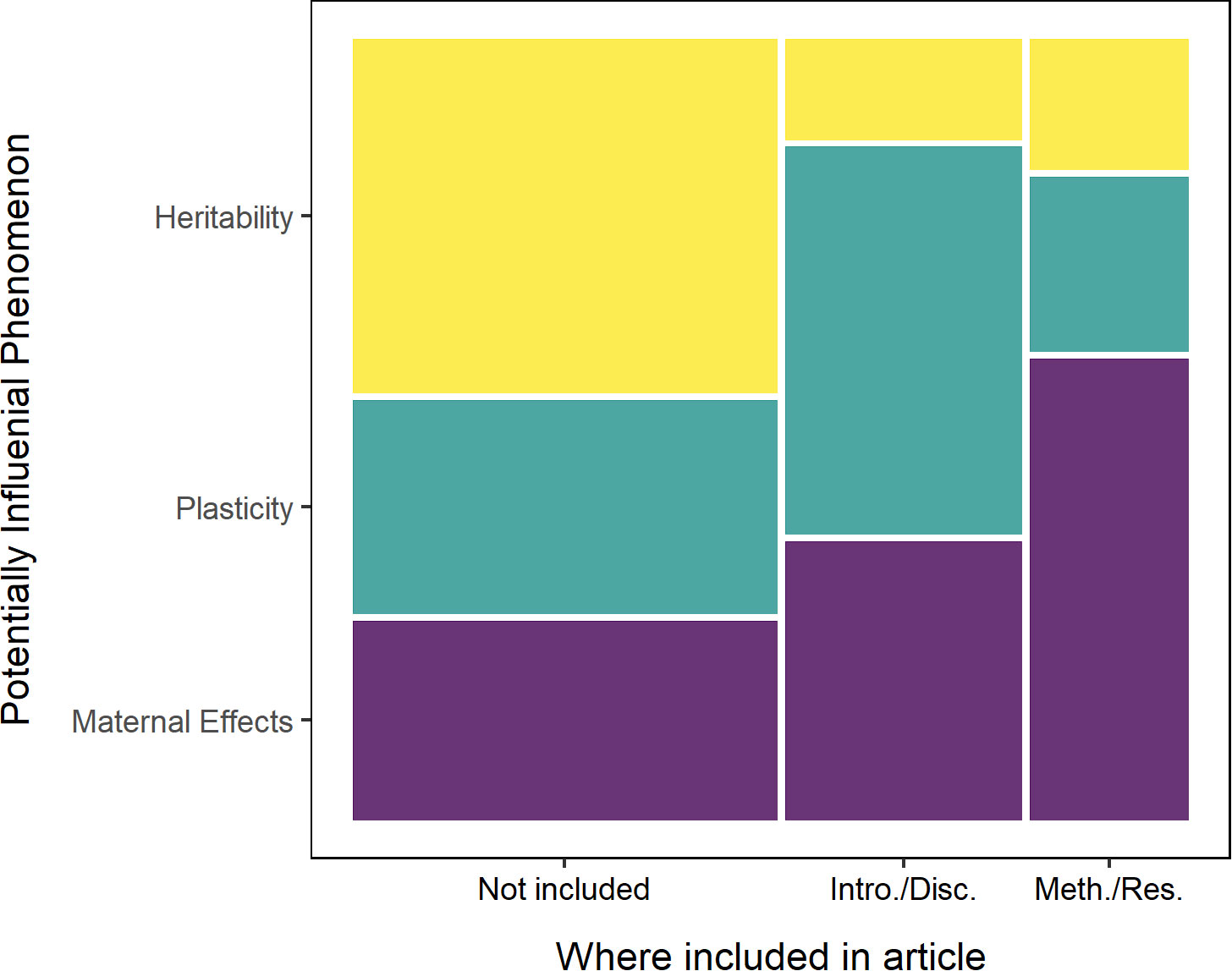
Figure 10 Mosaic plot showing the proportion of studies that discussed (Introduction or Discussion) or included (Methods or Results) the potentially influential phenomena of trait heritability, plasticity, and maternal effects. Approximately half of studies did not include any discussion of these phenomena (51%), almost a third addressed their role in the broader context of their study (29%), and only a fifth explicitly included them in their methodology and data analysis (20%). Of the three, the topic of plasticity was most frequently included in the ‘Introduction or Discussion’ (50%), followed by maternal effects (35%), and heritability (15%). The top two topics switch for inclusion in the ‘Methods or Results,’ with maternal effects (60%) followed by plasticity (24%), and heritability (16%).
Response variables
We found that response variables varied quite broadly, with a total of 249 unique variables reported upon in the relevant literature. Many of these disparate variables could be broadly categorized as morphological or other character traits to visualize them more readily; when combined, morphological traits far outpaced exceeded other types of response variables (Figure 11). Survivorship was an important measure of performance in 41% of the experiments we reviewed, while plant height was assessed in 46% and was particularly prevalent in studies that focused on tree species (Figure 11). Other traits of interest included germination (22%), biomass (22%), and phenology (19%), while 23% of the experiments measured how important physiological traits such as photosynthetic rates, stomatal conductance, and water use efficiency might be impacted.
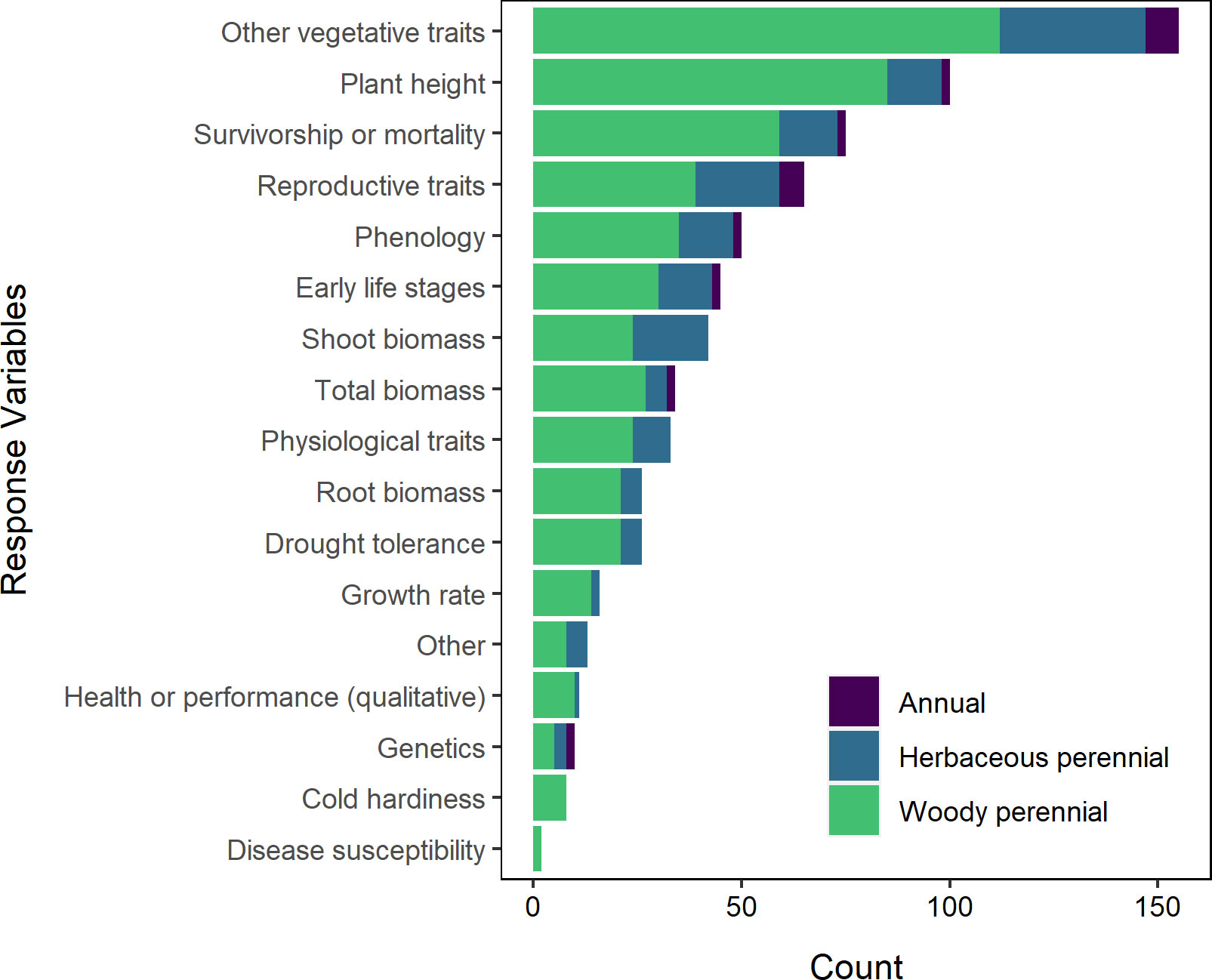
Figure 11 Response variables by plant life cycle. A single study of an herbaceous perennial is omitted from this visualization. “Other vegetative traits” consists of vegetative, morphological traits not listed separately (e.g., diameter, number of leaves, branching habit, etc.).
Approximately half of the papers (53%) reported that performance varied among provenances, meaning that the site of propagule origin significantly influenced performance of one or more traits.
Motivation of study
The most common study motivation we found was commercial forestry (42% of papers), followed by ecological restoration (27%), and three conservation topics—assisted migration, natural dispersal, and reintroduction (18%, 16%, and 7%). Finally, 5% of studies were conducted in the context of agriculture or crop science. There were 47 “other” responses, among these were 10 papers (6%) that studied responses or adaptation to climate change, and five papers (3%) on evolution.
We found that the majority of the relevant experimental papers returned from our literature search were explicitly designed to address how seed sourcing affects performance (71%) and we concluded that 86% of the papers offer insights into seed sourcing strategies under climate change.
Funding sources
Many studies had more than one funding source. Out of the 369 funding sources recorded, governmental sources were most common (60%). Most government-based funding came from national agencies (44%), 14% was provided by state/provincial agencies, while the European Union provided 2% of funding (Supplementary Figure S2). Industry support comprised 7% of funding sources, somewhat lower than the 13% provided by nonprofits. Of the studies reviewed, 17% did not record any funding sources and fewer than 2% had undetermined sources of funding.
Discussion
Globally, climate is predicted to shift from historic patterns (IPCC and Core Writing Team, 2014), with the potential to disrupt the structure and function of ecosystems, as well as the services they provide. For example, the Great Lakes region of the Midwestern United States, where most of the authors are located and where several of us practice restoration, is predicted to experience increased air temperatures between 3 ± 1°C under lower emissions scenarios and 5.0 ± 1.2°C under higher scenarios by the end of the century, and regionally the number of summer days with very high temperatures is also expected to increase (Pryor et al., 2014). The severity and frequency of heavy rain events will increase, leading to heightened flooding across the region (Pryor et al., 2014). Increases in winter and spring precipitation of up to 20-30% are projected, but future summer and fall patterns remain uncertain (Hayhoe et al., 2010). As a result, the challenges associated with managing and restoring natural habitats will become increasingly complex. Given the magnitude of the predicted changes, and the uncertainty that surrounds them, planning for habitat management and the restoration of degraded natural areas is becoming increasingly complex, despite the importance of undertaking these activities. Indeed, restoration actions are increasingly advanced as nature-based solutions to mitigate the effects of climate change (Brancalion and Holl, 2020; Simonson et al., 2021). This is a tall order despite the focus on restoration during this, the UN Decade of Ecosystem Restoration (https://www.decadeonrestoration.org/), when so much uncertainty remains regarding the fundamentals of sourcing plant materials.
We began this review hopeful that a critical mass of studies was available that had accumulated a body of results that would elucidate some general guidelines about sourcing seed to foster climate resiliency in seed-based restoration projects. To this end, we anticipated that we would at least find some commonality among predictor, and especially response, variables that would lead to general conclusions that could be broadly applied to the adaptive management of on-the-ground restoration projects at the seed sourcing stage (Perring et al., 2015; Baughman et al., 2019; Leger et al., 2021; Rushing et al., 2021). However, that is not what we found. Instead, our results showed that seed sourcing trials are primarily focused on tree species of economic importance, rather than on species that are the focus of ecological restoration. Indeed, the breadth of the data for forest trees is sufficient to support the development of a database for North American tree species compiled specifically to address future syntheses and/or meta-analysis (Risk et al., 2021). The data on the species included in this resource corresponds to those species we found have been most studied, which appear to be forest trees with economic value in extractive, production forestry. We base this conclusion upon the large number of studies that focused on trees, as well as upon the response variables that were chosen to determine performance as climate changes. These were frequently parameters such as biomass, tree or wood volume, DBH etc., rather than measures that are generally useful in monitoring success of restoration projects, such as germination and establishment (Broadhurst et al., 2016), survival, phenology, and fecundity (i.e., traits that are important for reproductive success and a trajectory of persistence and community function over longer time scales). In our view, the dearth of papers that assessed fecundity speaks to a near-term vision of land management, rather than the long-term restoration goal of establishing self-sustaining populations of a community of species that is resilient over time (Principle 9 - FAO, IUCN CEM and SER 2021).
Common garden and reciprocal transplant experiments, especially when the maternal identity of propagation materials is maintained throughout the experiment, are the optimal way to test how climate and other environmental conditions interact with the genetic background of individuals and population to affect heritable traits and phenotypic plasticity (Schwinning et al., 2022). Such genecological studies undertaken for tree species have far outpaced those focused upon shorter-lived species (Aitken and Bemmels, 2016). Sedentary and long-lived species may not be able to track conditions through migration, and very long-lived species such as trees are even more likely to be challenged to respond adaptively to climate change (Kremer et al., 2012). Conversely, the population and genetic structure of many forest tree species, including high amounts of genetic variation, and high heritabilities coupled with large populations and high fecundity rates, might increase their adaptive potential (Alberto et al., 2013). However, predicting such adaptations is complex (Nadeau and Urban, 2019), thus it is understandable that the majority of provenance trials undertaken to determine sourcing strategies for climate resilience are performed on tree species. Although trees also are dominant functional components of habitats globally, it may be their economic importance that has driven the result underpinning the current review given the emphasis on biomass and other more “resource-related” response variables that we found, and the finding that only tree studies were funded by industry sources. This finding is even more remarkable given the long timeframe that has been devoted to such provenance trials, frequently more than 10 years, when most studies tend to have much shorter durations, driven by funding cycles and the need to publish results quickly, and generally tend to focus on novel methods, questions, or context (Lindenmayer et al., 2012).
Long-term studies are valuable as they can be used to determine whether trends favoring certain provenances over the short term hold after decades or after extreme weather events, which are expected to increase under climate change. For example, St. Clair et al. (2020) showed that survival rates changed drastically after an interval of 20 years in a century old Douglas-fir provenance trial. Further, relationships between growth, survivorship, or other measures of fitness and the effects of site may vary across the life span of individuals. Thus, when experimental plantings are installed for long-term studies, and where multiple provenances are included, these may be repurposed to evaluate performance across time as climate changes. This approach was recommended long ago for trees (Mátyás, 1994), and was implemented in many of the studies on trees that we reviewed here. Similar insights for long-lived herbaceous perennial species would be useful in determining the likelihood that plants surviving the early stages of a restoration might prove resilient over time.
In the early stages of de novo restoration, most projects are focused on creating a community matrix, usually with herbaceous perennial species installed via seed and later adding woody components such as trees and shrubs, which are usually installed as pot-grown or bare root plants. Most of the papers we reviewed studied a single taxon, and did not measure any plant community related pressures and interactions. In degraded woodlands, restoration efforts generally concentrate on removing invasive trees and shrubs, and then restoring any remnant plant communities by re-establishing natural processes such as fire and augmenting with graminoid and forb seed if needed. Therefore, it is unclear whether studies on long-lived tree species that measure response variables like biomass production, have relevance to seed sourcing for restoration projects. Furthermore, while we found that most papers offered insights that could inform seed sourcing decisions, these insights may be dependent upon species-, context-, or life-history stage. This may be especially true for herbaceous species, which have a tremendous diversity of species, ranges, and life-histories. On the other hand, Etterson (2004a) utilized a reciprocal common garden design to demonstrate that Chamaecrista fasciculata, an annual nitrogen-fixing forb, was best adapted to its local environment, and when sourcing distance increased, performance—measured as fecundity—decreased. Phenology played a critical role in this relationship. Furthermore, some measures of performance traits had low heritabilities, thus limiting the adaptive potential of these populations, especially on the edges of the distribution (Etterson, 2004b). Etterson’s paired studies would support a “local is best” strategy for this, and perhaps other, annual species. However, a study using cutleaf monkeyflower (Mimulus laciniatus), another annual forb, showed that this species can adapt to quickly changing conditions after experiencing a severe drought (Dickman et al., 2019). In a study published after our data collection was complete, Woolridge et al. (2022) demonstrate the complexity of sourcing seed for climate resilience. They assessed the performance of three restoration-relevant forb species in a common garden and found that the influence of site and climate varied based on species as well as life-stage. They found that one species had highest lifetime fitness when sourced locally, a second had highest fitness when sourced from north of the common garden site, and a third when sourced from south of the site. Altogether, these findings present a conundrum for land managers tasked with restoring biodiverse and resilient local habitats that will withstand changing climatic conditions.
We recognize that the question we posed is likely too broad. Seed sourcing in an era of climate change is incredibly complex. If we had conducted a more focused search on a particular region or community type, e.g., the Great Basin of North America as did Shryock et al. (2018) or Baughman et al. (2019), we might have found a critical mass of information on functional or other traits that would prove useful for seed sourcing decisions in the manner that we envisioned. But climate change provides an existential challenge that has been broadly addressed by research for many decades. Therefore, we are surprised by the lack of data with which to undertake any meta-analysis, even for well-studied forest trees. This was largely driven by the high number of response variables we encountered. We recorded a total of 329 unique response variables, and while we were able to place them into broad categories, there was no single category that provided sufficient data for a meta-analysis. Many studies did use survivorship as a response variable, but the life stage at which they measured survivorship was also highly variable, making direct comparisons difficult. Another response variable we encountered with high frequency was plant height. While plant height has successfully been used in autecological and demographic studies, comparison across multiple unrelated species with a diversity of life histories, e.g., annuals and trees, with highly divergent growth rates, and assessed at different stages in the life span of an organism, was untenable. A common set of parameters established for use in evaluating plant performance from an ecological perspective, across climate gradients, or climate proxies would provide the foundation for powerful analysis in future work.
Recommendations and conclusions
We anticipated that we would discover general trends in plant performance across a gradient of environmental conditions that would prove useful for seed sourcing decisions for restoration practitioners. We found that trees, particularly those species that have economic value, are well-represented by provenance studies in the literature. Developing an aggregated database for these species might provide useful data for meta-analysis. We found a dearth of similar studies specific to important forb or graminoid species that might be broadly used in restoration projects, so-called “workhorse” species (Havens et al., 2015). In light of these findings, we have several recommendations that might bridge the gap between study and practice.
1. Source Identified Seed. It can be a challenge to find sufficient quantities of seed with the collection origin and the production data readily available. Seed with a certified origin, or “yellow-tag” seed (Houseal and Smith, 2000), is the exception rather than the rule. If the provenance details were more readily available across multiple producers, practitioners would then have the option of sourcing outside their region, and/or the opportunity to use environmental versus geographic distances in their seed choices; and/or to screen multiple sources for the best fit for their project location (Houseal and Smith, 2000; Havens et al., 2015; Leger et al., 2021). Seeds banked in regional and/or national seedbanks can provide the foundation for climate resilient restoration, as multiple species are collected across wide geographic areas (Chapman et al., 2019; Barga et al., 2020), and provenance information on each collection includes information on the location, as well as biotic and abiotic characteristics of the site. Moreover, a central database of seed origin and availability would make seed purchases easier and could be a tool for the industry for tracking demand (e.g., https://appliedeco.org/restoration/nativeseednetwork/). In addition, documenting the outcome of on-the-ground restoration projects using source-identified seed would provide valuable insights into plant performance from different sources.
2. Species Distribution Modeling (SDM). Ramalho et al. (2017) provided a very tangible and workable framework for the application of SDM’s to seed sourcing decisions. The approach they outlined included the use of user-friendly internet resources that could be implemented by practitioners to choose source locations beyond their typical protocols. A similar approach was recently implemented by McKone and Hernández (2021) in a prairie planting in Minnesota, United States. However, they advocate the introduction of species that might naturally expand their range, as predicted by models of climate-based range envelopes, rather than sourcing ecotypes of species outside their local region.
Another approach taken by one of the authors here (P. Vitt) involved the installation of an experimental seed mix design utilizing the species palette typical for de novo prairie restorations but sourced using SDM-based climate envelopes (Figure 1B). Exploratory modeling was undertaken using the tools presented by Ramalho et al. (2017). The results were used to set the source specifications for seed purchased through a standardized competitive bidding process. The experimental planting was paired with the same suite of species sourced in a similar bidding process specifying the seed origin as outlined in Figure 1A. The first seeding was implemented in February 2021 but failed because of a prolonged and deep regional drought. A second seeding was installed in February 2022. No data are yet available.
3. Regional Seed Networks. We know of several efforts to develop appropriate native seed supply regionally, including the Colorado Plateau Native Plant Program (United States), the Nevada Native Seed Partnership (United States), Northern Illinois Native Seed Network (United States), Ontario Plant Restoration Alliance (Canada), Southern Alberta Native Seed Collaborative (Canada), and the Xingu Native Seed Network (Brazil). Networks of all sizes can help ensure the availability of native seed through cooperative collection and production, and they can provide feedback on the performance of seed across regional restoration projects. They can also collaboratively provide resources such as the seed collection database created by the Minnesota, North Dakota, and South Dakota Chapter of The Nature Conservancy (2020) (https://tnc.maps.arcgis.com/home/group.html?id=453b14cdc5164015bfae43662d50aec7#overview). Efforts such as these can help expand the availability and use of source-identified seed. We advocate for the creation of more such networks.
4. Dynamic Seed Transfer Zones. Seed transfer zones are spatially explicit regions within which plant material may be moved while also minimizing the loss of fitness via maladaptation as well as avoiding the disruption of population genetic patterns. We recommend developing regional spatiotemporal seed transfer zones (Marinoni et al., 2021) or other approaches to create dynamic seed transfer zones that expand upon provisional seed transfer zones (e.g., Bower et al., 2014).
5. Increased integration between practice and theory. When researchers and practitioners work collaboratively on restoration projects, the results may prove particularly insightful (Dickens and Suding, 2013; Jellinek et al., 2021; Leger et al., 2021). Many studies have focused on extending basic ecological concepts to the practice of restoration (Dickens and Suding, 2013), but many of these are done in plot-based experiments that may or may not be correlated with patterns and dynamics at play in at-scale restoration projects. This science-practice gap exacerbates the uncertainties facing practitioners attempting future-forward climate resilient restoration. Many of the recommendations listed here will be most informative if applied in at-scale experiments done in collaboration. For example, the long-term resiliency of restored populations may be addressed by a regional collection approach that ensures a diversity of genotypes are introduced to restoration sites (Saari and Glisson, 2012; Herman et al., 2014, Bucharova et al., 2019) and then monitored for performance across climate gradients. More powerfully, purposefully moving populations beyond their current range (predictive provenancing) to be proactive in response to climate change (Havens et al., 2015), coupled with adequate monitoring, analysis, and communication in appropriate outlets for both scientists and practitioners would provide either a powerful proof of concept, or an appropriate repudiation of this approach. Either way, addressing these questions within the context of real-world restoration projects would be tremendously informative.
6. Addressing research needs. Havens et al. (2015) and the United States National Seed Strategy (Bureau of Land Management, 2021. US National Seed Strategy. https://www.blm.gov/programs/natural-resources/native-plant-communities/national-seed-strategy) among others, call for research that should focus on the genecological studies of broadly distributed foundational species. For example, screening multiple sources for the best fit or broadest adaptive potential (Leger et al., 2021). We call for funding to support long-term studies which are extremely important for ecology in general, and for understanding the impacts of climate change in particular (Kuebbing et al., 2018). Also, we advocate for a generalized set of response variables to be used in this type of study (see Supplementary Table 2), to contribute to a unified understanding of the impacts of climate change on plant populations, and implications for seed sourcing. For example, phenological changes affect >90% of plant species (Parmesan and Hanley, 2015), which may be particularly informative when coupled with an analysis of geographic variation in the responses (Parmesan and Hanley, 2015), and performance of individual species (Etterson, 2004a). Furthermore, while many studies that we reviewed focused on height and biomass production as response variables, only a few focused on the reproductive traits of herbaceous perennials such as seed production, viability, germination, etc. However, as reproductive traits are key to understanding restoration outcomes in the long term, and are often uncorrelated to vegetative traits (e.g., Hoyle et al., 2015), we believe they should be considered as central to questions of seed sourcing for climate change. Additionally, we found that most studies only consider a single taxon, while the expectation is that climate change is likely to change community composition. Therefore, research that addresses how community dynamics might be influenced by seed sourcing should also be undertaken.
7. Finally, we recommend that researchers and practitioners alike consider depositing data and lessons learned with a Restoration Project Clearinghouse to communicate how plantings undertaken with different seed designs are faring over time (Havens et al., 2015; https://www.ser-rrc.org/project-database/; https://www.globalrestoreproject.com/). Many seeding experiments are undertaken on a small scale, or as part of an on-the-ground restoration project. Data collected by land managers, while an important part of project monitoring, may be informal or accomplished across sporadic timeframes, which may be difficult to analyze. In combination with the results of formal monitoring however, these observations, as well as data from failed experiments (many of which do not get published) would provide a more comprehensive and deepened understanding of the processes at work. It may be necessary to develop an incentive to encourage land managers to spend the time submitting the necessary data through adding such requirements to grants or other funding opportunities. One approach is that taken by the Chicago Wilderness (2021), an alliance of regional land management, academic and private organizations working to advocate for nature in a highly developed landscape. Their Excellence in Ecological Restoration Program (www.chicagowilderness.org/page/EERPProgram) provides practitioners the opportunity to highlight their projects and be recognized for quality land management. The process of the program includes a site visit by a commission of regional professionals who evaluate the work during an extended site visit. These types of interactions provide a critical opportunity for discourse and information exchange.
Although many of the actions that we are calling for here are not new, perhaps there is a greater urgency for implementation. We are nearing the threshold for important climate change tipping points and the need for tools to ensure climate resilient restoration has never been more urgent.
Author contributions
All authors conceived and designed the research and collected data. JF led the data analysis and visualization. PV led the writing of the manuscript. All authors contributed to writing, editing, and analysis, and approved the final manuscript.
Funding
PV formed the Climate Resilient Seed Working Group, to which all authors belong, with support provided by the Wildlife Conservation Society’s Climate Adaptation Fund. JF was supported in part by the Oak Spring Garden Foundation’s Fellowship in Plant Conservation Biology. SF was supported under contribution agreement 68-3A75-18-048 with the United States Department of Agriculture-Natural Resources Conservation Service (USDA-NRCS).
Acknowledgments
Thank you to cassi saari who was involved in the early stages of developing these ideas, Imeña Valdes who helped locate full-text manuscripts, and Nicholas Spittlemeister who prepared the seed procurement maps. We also thank Rodolfo Gentili and Christopher Cockel who provided insightful comments that greatly improved the narrative.
Conflict of interest
The authors declare that the research was conducted in the absence of any commercial or financial relationships that could be construed as a potential conflict of interest.
Publisher’s note
All claims expressed in this article are solely those of the authors and do not necessarily represent those of their affiliated organizations, or those of the publisher, the editors and the reviewers. Any product that may be evaluated in this article, or claim that may be made by its manufacturer, is not guaranteed or endorsed by the publisher.
Supplementary material
The Supplementary Material for this article can be found online at: https://www.frontiersin.org/articles/10.3389/fcosc.2022.938110/full#supplementary-material
References
Aitken S. N., Bemmels J. B. (2016). Time to get moving: assisted gene flow of forest trees. Evol. Appl. 9 (1), 271–290. doi: 10.1111/eva.12293
Alberto F. J., Aitken S. N., Alía R., González-Martínez S. C., Hänninen H., Kremer A., et al. (2013). Potential for evolutionary responses to climate change–evidence from tree populations. Global Change Biol. 19 (6), 1645–1661. doi: 10.1111/gcb.12181
Anderson J. T., Wadgymar S. M. (2020). Climate change disrupts local adaptation and favours upslope migration. Ecol. Lett. 23 (1), 181–192. doi: 10.1111/ele.13427
Arnold J. B. (2021) Ggthemes: Extra themes, scales and geoms for 'ggplot2'. r package version 4.2.4. Available at: https://CRAN.R-project.org/package=ggthemes.
Barak R. S., Ma Z., Brudvig L. A., Havens K. (2021). Factors influencing seed mix design for prairie restoration. Restor. Ecol. 30 (5), e13581. doi: 10.1111/rec.13581
Barga S. C., Olwell P., Edwards F., Prescott L., Leger E. A. (2020). Seeds of success: A conservation and restoration investment in the future of US lands. Conserv. Sci. Pract. 2 (7), e209. doi: 10.1111/csp2.209
Baughman O. W., Agneray A. C., Forister M. L., Kilkenny F. F., Espeland E. K., Fiegener R., et al. (2019). Strong patterns of intraspecific variation and local adaptation in great basin plants revealed through a review of 75 years of experiments. Ecol. Evol. 9 (11), 6259–6275. doi: 10.1002/ece3.5200
Bower A. D., Clair J. B. S., Erickson V. (2014). Generalized provisional seed zones for native plants. Ecol. Appl. 24 (5), 913–919. doi: 10.1890/13-0285.1
Brancalion P. H. S., Holl. K. D. (2020). Guidance for successful tree planting initiatives. J. Applied Ecology. 57(12), 2349–2361. doi: doi.org/10.1111/1365-2664.13725
Breed M. F., Harrison P. A., Bischoff A., Durruty P., Gellie N. J., Gonzales E. K., et al. (2018). Priority actions to improve provenance decision-making. BioScience 68 (7), 510–516. doi: 10.1093/biosci/biy050
Broadhurst L. M., Jones T. A., Smith F. S., North T., Guja L. (2016). Maximizing seed resources for an uncertain future. BioScience 66 (1), 73–79. doi: 10.1093/biosci/biv155
Bucharova A., Bossdorf O., Hölzel N., Kollmann J., Prasse R., Durka W. (2019). Mix and match: regional admixture provenancing strikes a balance among different seed-sourcing strategies for ecological restoration. Conserv. Genet. 20 (1), 7–17. doi: 10.1007/s10592-018-1067-6
Bureau of Land Management, United States Department of the Interior (2021) National seed strategy. Available at: https://www.blm.gov/programs/natural-resources/native-plant-communities/national-seed-strategy (Accessed May 6, 2022).
Camhi A. L., Perrings C., Butterfield B., Wood T. (2019). Market-based opportunities for expanding native seed resources for restoration: A case study on the Colorado plateau. J. Environ. Manage. 252, 109644. doi: 10.1016/j.jenvman.2019.109644
Chapman T., Miles S., Trivedi C. (2019). Capturing, protecting and restoring plant diversity in the UK: RBG kew and the millennium seed bank. Plant Diversity 41 (2), 124–131. doi: 10.1016/j.pld.2018.06.001
Chicago Wilderness (2021) Excellence in restoration program. Available at: www.chicagowilderness.org/page/EERPProgram (Accessed May 5, 2022).
Corlett R. T. (2016). Plant diversity in a changing world: Status, trends, and conservation needs. Plant Diversity. 38 (1), 10–16. doi: 10.1016/j.pld.2016.01.001
Dickens S. J. M., Suding K. N. (2013). Spanning the science-practice divide: why restoration scientists need to be more involved with practice. Ecol. Restor. 31 (2), 134–140. doi: 10.3368/er.31.2.134
Dickman E. E., Pennington L. K., Franks S. J., Sexton J. P. (2019). Evidence for adaptive responses to historic drought across a native plant species range. Evol. Appl. 12 (8), 1569–1582. doi: 10.1111/eva.12803
Diffenbaugh N. S., Singh D., Mankin J. S. (2018). Unprecedented climate events: Historical changes, aspirational targets, and national commitments. Sci. Adv. 4 (2), eaao3354. doi: 10.1126/sciadv.aao3354
Etterson J. R. (2004a). Evolutionary potential of Chamaecrista fasciculata in relation to climate change. 1. clinal patterns of selection along an environmental gradient in the great plains. Evolution 58 (97), 1446–1458. doi: 10.1554/04-053
Etterson J. R. (2004b). Evolutionary potential of Chamaecrista fasciculata in relation to climate change. II. genetic architecture of three populations reciprocally planted along an environmental gradient in the great plains. Evolution 58 (97), 1459–1471. doi: 10.1111/j.0014-3820.2004.tb01727.x
FAO, IUCN CEM and SER (2021). Principles for ecosystem restoration to guide the united nations decade 2021–2030 (Rome: FAO).
Gann G. D., McDonald T., Walder B., Aronson J., Nelson C. R., Jonson J., et al. (2019). International principles and standards for the practice of ecological restoration. second edition. Restor. Ecol. 27 (S1), S1–S46. doi: 10.1111/rec.13035
Giencke L. M., Carol Denhof R., Katherine Kirkman L., Stribling Stuber O., Brantley S. T. (2018). Seed sourcing for longleaf pine ground cover restoration: using plant performance to assess seed transfer zones and home-site advantage. Restor. Ecol. 26 (6), 1127–1136. doi: 10.1111/rec.12673
Groves A. M., Brudvig L. A. (2018). Interannual variation in precipitation and other planting conditions impacts seedling establishment in sown plant communities. Restor. Ecol. 27 (1), 128–137. doi: 10.1111/rec.12708
Hancock N., Hughes L. (2014). Turning up the heat on the provenance debate: testing the ‘local is best’ paradigm under heatwave conditions. Austral Ecol. 39 (5), 600–611. doi: 10.1111/aec.12122
Harrison S. P., Schoen R., Atcitty D., Fiegener R., Goodhue R., Havens K., et al. (2020). Preparing for the need for a supply of native seed. Ecol. Restor. 38 (4), 203–206. doi: 10.3368/er.38.4.203
Havens K., Vitt P., Still S., Kramer A. T., Fant J. B., Schatz K. (2015). Seed sourcing for restoration in an era of climate change. Natural Areas J. 35 (1), 122–133. doi: 10.3375/043.035.0116
Hayhoe K., VanDorn J., Croley T., Schlegal N., Wuebbles D. (2010). Regional climate change projections for Chicago and the US Great Lakes. Journal of Great Lakes Research. 36 (2), 7–21.
Herman B., Packard S, Pollack C., Houseal G., Sinn S., O’Leary C., et al (2014) “Decisions . . . Decisions . . . How to source plant material for native plant restoration projects.” Ecological Restoration 32, no. 3, 236–238. muse.jhu.edu/article/552439.
Hobbs R. J., Arico S., Aronson J., J., Baron S., Bridgewater P., V., Cramer A., et al. (2006). Novel ecosystems: theoretical and management aspects of the new ecological world order. Global Ecology and Biogeography. 15 (1), 1–7. doi: 10.1111/j.1466-822X.2006.00212.x
Hobbs R. J., Higgs E., Harris J. A. (2009). Novel ecosystems: implications for conservation and restoration. Trends Ecol. Evol. 24 (11), 599–605. doi: 10.1016/j.tree.2009.05.012
Houseal G., Smith D. (2000). Source-identified seed: the Iowa roadside experience. Ecol. Restor. 18 (3), 173–183. doi: 10.3368/er.18.3.173
Hoyle G. L., Steadman K. J., Good R. B., McIntosh E. J., Galea L. M., Nicotra A. B. (2015). Seed germination strategies: an evolutionary trajectory independent of vegetative functional traits. Front. Plant Sci. 6, 731. doi: 10.3389/fpls.2015.00731
IPCC, Core Writing Team (2014). Climate change, (2014): Synthesis report. contribution of working groups I, II and III to the fifth assessment report of the intergovernmental panel on climate change. Eds. Pachauri R. K., Meyer L. A. (Geneva, Switzerland: IPCC), 151 pp.
James J. J., Svejcar T. J., Rinella M. J. (2011). Demographic processes limiting seedling recruitment in arid grassland restoration. J. Appl. Ecol. 48 (4), 961–969. doi: 10.1111/j.1365-2664.2011.02009.x
Jellinek S., Lloyd S., Catterall C., Sato C. (2021). Facilitating collaborations between researchers and practitioners in ecosystem management and restoration. Ecol. Manage. Restor. 22 (2), 208–213. doi: 10.1111/emr.12465
Jeppson H., Hofmann H., Cook D., Wickham H. (2021) Ggmosaic: Mosaic plots in the 'ggplot2' framework. r package version 0.3.3. Available at: https://CRAN.R-project.org/package=ggmosaic.
Kremer A., Ronce O., Robledo-Arnuncio J. J., Guillaume F., Bohrer G., Nathan R., et al. (2012). Long-distance gene flow and adaptation of forest trees to rapid climate change. Ecol. Lett. 15 (4), 378–392. doi: 10.1111/j.1461-0248.2012.01746.x
Kuebbing S. E., Reimer A. P., Rosenthal S. A., Feinberg G., Leiserowitz A., Lau J. A., et al. (2018). Long-term research in ecology and evolution: A survey of challenges and opportunities. Ecol. Monogr. 88 (2), 245–258. doi: 10.1002/ecm.1289
Larson J. E., Funk J. L. (2016). Regeneration: an overlooked aspect of trait-based plant community assembly models. J. Ecol. 104 (5), 1284–1298. doi: 10.1111/1365-2745.12613
Leger E. A., Barga S., Agneray A. C., Baughman O., Burton R., Williams M. (2021). Selecting native plants for restoration using rapid screening for adaptive traits: methods and outcomes in a great basin case study. Restor. Ecol. 29 (4), e13260. doi: 10.1111/rec.13260
Lindenmayer D. B., Likens G. E., Andersen A., Bowman D., Bull C. M., Burns E., et al. (2012). Value of long-term ecological studies. Austral Ecol. 37 (7), 745–757. doi: 10.1111/j.1442-9993.2011.02351.x
Löf M., Madsen P., Metslaid M., Witzell J., Jacobs D. F. (2019). Restoring forests: regeneration and ecosystem function for the future. New Forests 50 (2), 139–151. doi: 10.1007/s11056-019-09713-0
Marinoni L., Quijano Parra M., J., Zabala M., J., Pensiero F., J., Iriondo M. (2021). Spatiotemporal seedtransfer zones as an efficient restoration strategy in response to climate change. Ecosphere 12(5):e03462. doi: 10.1002/ecs2.3462
Mátyás C. (1994). Modeling climate change effects with provenance test data. Tree Physiol. 14 (7-8-9), 797–804. doi: 10.1093/treephys/14.7-8-9.797
McKone M. J., Hernández D. L. (2021). Community-level assisted migration for climate-appropriate prairie restoration. Restor. Ecol. 29 (7), e13416. doi: 10.1111/rec.13416
Morris W. F., Pfister C. A., Tuljapurkar S., Haridas C. V., Boggs C. L., Boyce M. S., et al. (2008). Longevity can buffer plant and animal populations against changing climatic variability. Ecology 89 (1), 19–25. doi: 10.1890/07-0774.1
Muñoz-Rojas M., T., Erickson E., D., Martini C., K., Dixon W., D., Merritt J. (2016). Climate and soil factors influencing seedling recruitment of plant species used for dryland restoration. SOIL 2, 287–298. doi: 10.5194/soil-2-287-2016
Nadeau C. P., Urban M. C. (2019). Eco-evolution on the edge during climate change. Ecography 42 (7), 1280–1297. doi: 10.1111/ecog.04404
Oldfield S. (2019). The US national seed strategy for rehabilitation and restoration: Progress and prospects. Plant Biol. 21 (3), 380–382. doi: 10.1111/plb.12851
Parmesan C. (2006). Ecological and evolutionary responses to recent climate change. annual review. Ecol. Systematics 37, 637–669. doi: 10.1146/annurev.ecolsys.37.091305.110100
Parmesan C., Hanley M. E. (2015). Plants and climate change: complexities and surprises. Ann. Bot. 116 (6), 849–864. doi: 10.1093/aob/mcv169
Perring M. P., Standish R. J., Price J. N., Craig M. D., Erickson T. E., Ruthrof K. X., et al. (2015). Advances in restoration ecology: rising to the challenges of the coming decades. Ecosphere 6 (8), 131. doi: 10.1890/ES15-00121.1
Prober S. M., Dunlop M. (2011). Climate change: a cause for new biodiversity conservation objectives but let’s not throw the baby out with the bathwater. Ecological Management and Restoration 12 (1), 2
Prober S. M., Byrne M., McLean E. H., Steane D. A., Potts B. M., Vaillancourt R. E., et al. (2015). Climate-adjusted provenancing: a strategy for climate-resilient ecological restoration. Front. Ecol. Evol. 3. doi: 10.3389/fevo
Pryor S. C., Scavia D., Downer C., Gaden M., Iverson L., Nordstrom R., et al (2014). Ch. 18: Midwest. Climate Change Impacts in the United States: The Third National Climate Assessment Melillo J. M., Richmond T. C., Yohe G. W., eds., U.S. Global Change Research Program, 418–440. doi: 10.7930/J0J1012N.
Ramalho C. E., Byrne M., Yates C. J. (2017). A climate-oriented approach to support decision-making for seed provenance in ecological restoration. Front. Ecol. Evol., 95. doi: 10.3389/fevo.2017.00095
R Core Team (2021). R: A language and environment for statistical computing. r foundation for statistical computing (Vienna, Austria: The R development core team). Available at: https://www.R-project.org/.
Reiker J., Schulz B., Wissemann V., Gemeinholzer B. (2015). Does origin always matter? evaluating the influence of nonlocal seed provenances for ecological restoration purposes in a widespread and outcrossing plant species. Ecol. Evol. 5 (23), 5642–5651. doi: 10.1002/ece3.1817
Risk C., McKenney D. W., Pedlar J., Pengixin Lu (2021). A compilation of north American tree provenance trials and relevant historical climate data for seven species. Sci. Data 8, 29. doi: 10.1038/s41597-021-00820-2
Rushing N. S., Flint S. A., Shaw R. G. (2021). Latitude of seed source impacts flowering phenology and fitness in translocated plant populations. Restor. Ecol. 29 (8), e13464. doi: 10.1111/rec.13464
Saari c., Glisson W. (2012). Survey of Chicago region restoration seed source policies. Ecol. Restor. 30 (3), 162–165. doi: 10.3368/er.30.3.162
Schwinning S., Lortie C. J., Esque T. C., DeFalco L. A. (2022). What common-garden experiments tell us about climate responses in plants. J. Ecology, 110, 986–996. doi: 10.1111/1365-2745.13887
Shryock D. F., DeFalco L. A., Esque T. C. (2018). Spatial decision-support tools to guide restoration and seed-sourcing in the desert southwest. Ecosphere 9 (10), e02453. doi: 10.1002/ecs2.2453
Simonson W. D., Miller E., Jones A., García-Rangel S., Thornton H., McOwen C. (2021). Enhancing climate change resilience of ecological restoration–a framework for action. Perspect. Ecol. Conserv. 19 (3), 300–310. doi: 10.1016/j.pecon.2021.05.002
St. Clair J. B., Howe G. T., Kling J. G. (2020). The 1912 Douglas-fir heredity study: long-term effects of climatic transfer distance on growth and survival. J. Forestry 118 (1), 1–13. doi: 10.1093/jofore/fvz064
The Nature Conservancy (2020) The prairie seed collection group. Available at: https://tnc.maps.arcgis.com/home/group.html?id=453b14cdc5164015bfae43662d50aec7#overview (Accessed May 5, 2022).
Török P., Brudvig L. A., Kollmann J., N Price J., Tóthmérész B. (2021). The present and future of grassland restoration. Restor. Ecol. 29, e13378. doi: 10.1111/rec.13378
Vitt P., Havens K., Kramer A. T., Sollenberger D., Yates E. (2010). Assisted migration of plants: changes in latitudes, changes in attitudes. Biological Conservation 143(1), 18–27.
White A., Fant J. B., Havens K., Skinner M., Kramer A. T. (2018). Restoring species diversity: assessing capacity in the US native plant industry. Restor. Ecol. 26 (4), 605–611. doi: 10.1111/rec.12705
Wickham H. (2016). ggplot2: Elegant graphics for data analysis (New York: Springer-Verlag). Available at: https://ggplot2.tidyverse.org, ISBN: ISBN 978-3-319-24277-4.
Wickham H. (2021) Forcats: Tools for working with categorical variables (Factors). r package version 0.5.1. Available at: https://CRAN.R-project.org/package=forcats.
Wilkins D. (2021a) Ggfittext: Fit text inside a box in 'ggplot2'. r version 0.9.1. Available at: https://CRAN.R-project.org/package=ggfittext.
Wilkins D. (2021b) Treemapify: Draw treemaps in 'ggplot2'. r package version 2.5.5. Available at: https://CRAN.R-project.org/package=treemapify.
Keywords: climate change, common garden, ecological restoration, provenance, reciprocal transplant, reforestation, seed sourcing
Citation: Vitt P, Finch J, Barak RS, Braum A, Frischie S and Redlinski I (2022) Seed sourcing strategies for ecological restoration under climate change: A review of the current literature. Front. Conserv. Sci. 3:938110. doi: 10.3389/fcosc.2022.938110
Received: 07 May 2022; Accepted: 11 August 2022;
Published: 06 October 2022.
Edited by:
David W. Inouye, University of Maryland, College Park, United StatesReviewed by:
Rodolfo Gentili, University of Milano-Bicocca, ItalyChristopher Cockel, Royal Botanic Gardens, Kew, United Kingdom
Copyright © 2022 Vitt, Finch, Barak, Braum, Frischie and Redlinski. This is an open-access article distributed under the terms of the Creative Commons Attribution License (CC BY). The use, distribution or reproduction in other forums is permitted, provided the original author(s) and the copyright owner(s) are credited and that the original publication in this journal is cited, in accordance with accepted academic practice. No use, distribution or reproduction is permitted which does not comply with these terms.
*Correspondence: Pati Vitt, p-vitt@northwestern.edu
†These authors have contributed equally to this work and share first authorship