- 1Institute of Zoology, Zoological Society of London, London, United Kingdom
- 2Department of Genetics, Evolution and Environment, University College London, London, United Kingdom
- 3Key Laboratory of Aquatic Biodiversity and Conservation, Institute of Hydrobiology, Chinese Academy of Sciences, Wuhan, China
- 4Durrell Wildlife Conservation Trust, Les Augrès Manor, Trinity, Jersey
The impacts of fisheries interactions on cetaceans can be challenging to determine, often requiring multiple complementary investigative approaches. The Yangtze finless porpoise (Neophocaena asiaeorientalis asiaeorientalis), one of the few Critically Endangered cetaceans, is endemic to the middle-lower Yangtze drainage, a system impacted by multiple anthropogenic pressures. Bycatch mortality is implicated in regional porpoise decline, but the significance and dynamics of porpoise interactions with fishing activities and other threats remain poorly understood. We conducted boat-based surveys to map seasonal distributions and spatial congruence of porpoises and two potential threats (fishing and sand-mining), and an interview survey of fishing communities to understand temporal patterns and drivers of regional fishing activity, across Poyang Lake and the adjoining Yangtze mainstem. Variation in harmful and non-harmful gear use (non-fixed nets versus static pots and traps) between these landscapes might be an important factor affecting local porpoise status. Within Poyang Lake, spatial correlations between porpoises and threats were relatively weak, seasonal porpoise and threat hotspots were located in different regions, and two protected areas had higher porpoise encounter rates and densities than some unprotected sections. However, porpoise hotspots were mostly in unprotected areas, threats were widely observed across reserves, and more fishing and sand-mining was seasonally observed within reserves than within unprotected areas. Compared to null distributions, porpoises were detected significantly closer to fishing activities in summer and further from sand-mining in winter, indicating possible spatial risks of gear entanglement and disturbance. Reported porpoise bycatch deaths are associated with fixed and non-fixed nets, hook-based gears, and electrofishing. Longitudinal patterns in reported gear use indicate that hook-based fishing has decreased substantially and is generally conducted by older fishers, and significantly fewer respondents now practice fishing as their sole source of income, but electrofishing has increased. This combined research approach indicates a continued potential risk to porpoises from changing fisheries interactions and other threats, highlighting the importance of fishing restrictions and appropriate support for fishing communities impacted by this legislation. A potential “win–win” for both biodiversity and local livelihoods could be achieved through wider use of static pots and traps, which are not associated with bycatch deaths.
Introduction
Fisheries bycatch (incidental death of non-target species) is a major recognized cause of mortality in many populations of small cetaceans worldwide, as well as for many other aquatic taxa (Gray and Kennelly, 2018; Brownell et al., 2019; Tulloch et al., 2020). Bycatch levels can be unsustainable and fisheries interactions have been implicated in catastrophic cetacean declines and losses, including the extinction of the Yangtze River dolphin or baiji (Lipotes vexillifer), and the critical depletion of the vaquita (Phocoena sinus) and Māui dolphin (Cephalorhynchus hectori maui) to tiny numbers of surviving individuals (Turvey et al., 2007; Taylor et al., 2017; Slooten and Dawson, 2021).
However, robust data are often unavailable to determine the population-level effects of fisheries interactions and the extent to which bycatch might impact cetacean populations, hindering the potential for evidence-based conservation management, and the question of how to understand and reduce bycatch of threatened species is recognized as a key outstanding issue in marine conservation (Parsons et al., 2014). This is a particular problem across social–ecological systems where local fisheries employ multiple gears and extraction methods, where fishery dynamics show spatial and/or temporal variation, and where fisheries represent only one of many anthropogenic pressures that can have direct and indirect effects on cetaceans. Such complex and confounding conditions characterize the highly converted and industrialized landscapes that support surviving populations of threatened Asian freshwater cetaceans, and also provide food, livelihoods, resources, and ecosystem services for high-density, low-income human populations (Reeves et al., 2000; Kelkar et al., 2010). It is therefore crucial to determine the dynamics of fisheries interactions and their impacts on freshwater cetacean populations, including patterns of both spatial and temporal variation in threat intensity, to assess the effectiveness of existing mitigation strategies and guide identification of locally-appropriate management approaches that also support human wellbeing (Aburto-Oropeza et al., 2018; Alava et al., 2019).
The Yangtze finless porpoise (Neophocaena asiaeorientalis asiaeorientalis) is a freshwater cetacean restricted to the middle-lower Yangtze drainage in eastern China, where it occurs in the river mainstem and two major appended lake systems (Dongting and Poyang). Its population has declined severely, falling in the mainstem from ~2,700 individuals in the 1990s (Zhang et al., 1993) to ~450 in 2017, with another ~450 individuals in Poyang Lake and ~100 in Dongting Lake (Huang et al., 2020). It is one of the few cetaceans listed as Critically Endangered by the IUCN (2021). The middle-lower Yangtze is heavily industrialized and supports an extremely large human population, and is impacted by multiple anthropogenic stressors including high vessel traffic, pollution, resource depletion, and habitat loss, degradation and disturbance; these activities are all proposed as possible drivers of porpoise decline (Wang et al., 2000; Zhao et al., 2008; Wang, 2009; Turvey et al., 2013; Mei et al., 2014; Huang et al., 2017; Mei et al., 2021). This system also supports local fisheries that use a variety of legal and illegal methods (Chen et al., 2009; Turvey et al., 2015; Mei et al., 2019). These fisheries have collapsed over recent decades due to overexploitation and other factors such as water impoundment, pollutant discharge, and habitat modification and fragmentation (Fang et al., 2006; Turvey et al., 2012; Zhang et al., 2020). Several legislative interventions have been introduced to support fish stock recovery and protect porpoises from potential fishery impacts, including periodic fishery closures, and establishment of in situ reserves in both mainstem and appended lakes (Wang, 2009; Huang et al., 2017; Zhang et al., 2020). However, other destructive environmental practices that might impact porpoises directly or indirectly, notably sand-mining (dredging of sand by vessels from river and lake beds), have increased across the Yangtze system in recent years but remain largely unregulated and unstudied (Larson, 2018; Yao et al., 2019; Koehnken et al., 2020). Sand-mining has a range of impacts on abiotic properties of freshwater systems (channel morphology, flow regime, water quality, sediment composition and movement) and on wider biodiversity (Zou et al., 2019; Koehnken et al., 2020). Porpoises might also be affected directly by underwater noise associated with sand-mining (de Leeuw et al., 2010), as anthropogenic noise from vessels and industrial activities is a known source of sub-lethal environmental stress (Zhao et al., 2008; Wang, 2009), causing physiological damage, hormonal stress, and behavioral alteration including disruption of foraging and communication and avoidance behavior (Li et al., 2008; Kastelein et al., 2015; Kastelein et al., 2017; Wisniewska et al., 2018). Sand-mining is now considered a primary threat to porpoises in some areas (Xu, 2015; Li et al., 2021).
Conservation research on many marine mammals aims to assess relationships between threatened populations and threatening anthropogenic activities (Breen et al., 2016; Braulik et al., 2018). Such studies of human–wildlife interactions within linked social–ecological systems, in which local communities interact closely with threatened biodiversity, can benefit from multiple complementary investigative approaches using both ecological and social science methods, to understand human dimensions as well as biodiversity baselines (Newing, 2011; Liu et al., 2016). Conversely, previous studies on Yangtze finless porpoises have focused primarily on understanding population size, distribution and trends, including identification of regions of higher relative porpoise densities, but have rarely investigated porpoise distribution in relation to co-occurring human activities to understand threat dynamics or evaluate effectiveness of conservation actions.
Some specific gears (notably hook-based gears comprising longlines with hundreds of unbaited hooks and electrofishing) have been highlighted as possible drivers of Yangtze cetacean decline (Zhou and Wang, 1994; Zhou et al., 1998; Zhang et al., 2003; Turvey et al., 2007; Turvey et al., 2013), and legislation prohibiting electrofishing is widely promoted and enforced in the mainstem and appended lakes (Turvey et al., 2015; Mei et al., 2019). However, investigation of porpoise bycatch has been largely restricted to opportunistic reporting of observed deaths in gear entanglement (Zhou and Wang, 1994; Wang et al., 2000; Wang et al., 2015a; Mei et al., 2019). Analysis of porpoise mortality data obtained through interviews with artisanal fishers by Turvey et al. (2013) suggested that bycatch mortality has decreased over time, and might therefore merely be tracking rather than driving population decline. Mogensen et al. (2022) also found limited spatial correlation between observed porpoise deaths and fishing activity patterns at multiple spatial scales, but population modeling suggested that reported bycatch mortality might exceed sustainable levels. The dynamics of how bycatch and other potential threats act and interact across the different multi-threat landscapes of the Yangtze system, their specific relationships with porpoises (e.g., levels and patterns of spatial overlap), and how these might vary at different scales across time and space, remain poorly understood. There is therefore an urgent need for further research into the spatial and temporal dynamics of different anthropogenic activities across the range of the Yangtze finless porpoise, together with assessment of the effectiveness of existing mitigation efforts to reduce potential fisheries and other impacts, to identify key threats and key regions of higher and lower threat intensity (cf. Karpouzi et al., 2007; Tulloch et al., 2015).
To establish a strengthened evidence-base on porpoise–fisheries interactions across the Yangtze system, and to understand the changing dynamics of these interactions and their relative importance in the context of other regional anthropogenic pressures, we conducted new conservation research using both ecological and social science methods, collecting data on porpoises and threats in the same combined survey effort. This research aimed to determine the distributions, spatiotemporal overlap, and potential interactions between porpoises and potential threats at multiple spatial and temporal scales, and their relationship with protected areas established to reduce the pressure of these threats, to help guide decision-making and improve the effectiveness of existing mitigation efforts. More widely, our study presents a mixed-methods approach to threat assessment that can be employed to understand potential fisheries impacts in other complex social–ecological systems with limited existing data.
Materials and methods
Study region
Fieldwork was conducted in Poyang Lake, and along the ~150-km adjoining section of the lower Yangtze mainstem from the mouth of Poyang Lake downstream to Anqing (Figure 1). These two regions have been identified as “high conservation value areas” for porpoises (Zhao et al., 2013). Poyang Lake supports almost half the remaining porpoise population (Huang et al., 2020), porpoise encounter rates have remained relatively constant in this region over recent decades during boat-based visual and acoustic surveys (Zhao et al., 2008; Dong et al., 2015; Huang et al., 2020), and significantly fewer fishers in this region consider that porpoises have declined locally in comparison to perceptions of fishers in the mainstem (Turvey et al., 2013). Within the mainstem, porpoise encounter rates were highest within the Poyang Lake–Anqing section (>0.35 porpoises/km) in 2006 (Zhao et al., 2013), and relatively high densities have been recorded in this section in subsequent surveys (Mei et al., 2014; Huang et al., 2020).
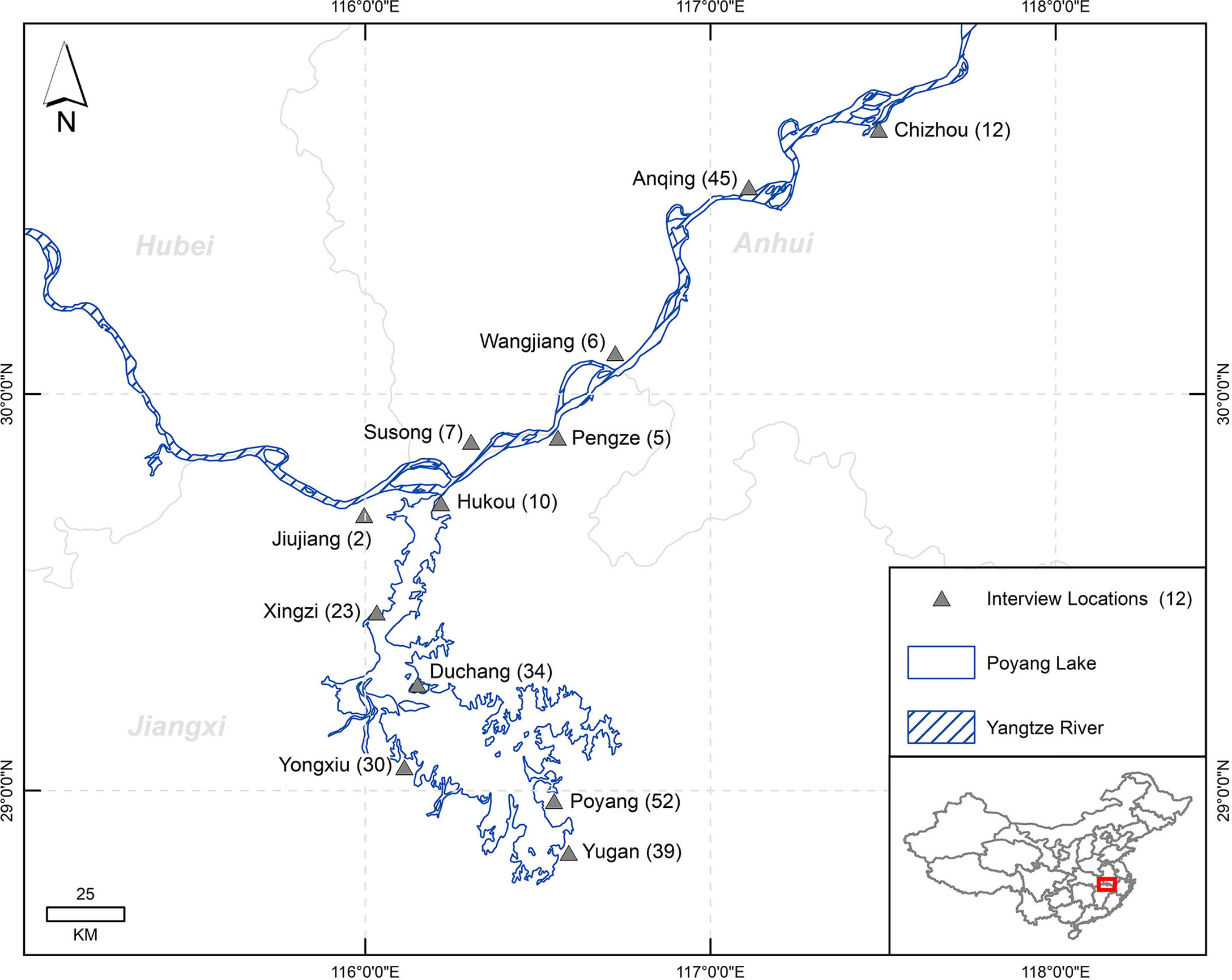
Figure 1 Poyang Lake and the adjoining section of Yangtze mainstem, showing fishing communities where interviews were conducted.
Whereas the lower Yangtze mainstem maintains broadly similar year-round conditions, Poyang Lake is a highly seasonal environment that experiences major inundation changes between winter low-water and summer high-water periods (from 714.1 km2 in October 2009 to 3,162.9 km2 in August 2010; Feng et al., 2012). Distributions of porpoises and threats may therefore be expected to exhibit seasonal variation within this dynamic system (Li et al., 2021). Fisheries interactions and sand-mining are proposed as the two main threats to porpoises in Poyang Lake (Xu, 2015). An in situ porpoise reserve with two spatially separate management areas (Longkou and Laoyemiao) was officially designated in Poyang Lake in 2004, and is managed by the Duchang and Poyang fisheries bureaus, respectively. At the time of our fieldwork, there was an annual 2-month fishing ban in Poyang Lake (April–May) and 3-month ban in the mainstem (April–June) to support fish stock recovery.
Boat surveys
Combined porpoise and threat surveys were conducted on 1–7 March 2016 (winter, low water) and 7–13 September 2017 (summer, high water), using visual transect surveys from a single-engine fishing vessel. Surveys followed an approximately north–south route along the channel midline, as well as a ~20-km side channel west of Xingzi as a detour when heading north (Figure S1; Table S1). This route represents the only navigable route during winter, and so was followed in both surveys to permit comparison of seasonal differences. High ship traffic density also meant that our survey had to follow a standard route designated by ship traffic safety legislation in both seasons. Each survey was conducted twice across this route, collecting data on both passes of the same route (north–south and south–north).
Surveys comprised dual-observer teams at an eyeline ≥2.5-m above water level, comprising two experienced observers using binoculars (Opticron Adventurer Wp 8×42) and laser range-finder (Hawke LRF 600). Left and right observers searched 90° off the bow and 10° beyond the track-line. All porpoise, fishing, and sand-mining observations were recorded in passing mode (Dawson et al., 2008), with tracks and observations recorded using a Garmin MAP64 GPS. Surveys were halted on days where porpoises could not be seen beyond 200 m due to bad weather. Porpoise sighting data included time, distance from nearest bank, and distance and direction from vessel (using an angle board to estimate distance; Buckland et al., 2001). Group size and number of calves/group were also recorded, with a group defined as any gathering of individuals estimated as being within 100 m of each other. Direction and distance of active fishing and sand-mining vessels were also recorded; moored/inactive vessels were not recorded.
GPS tracks were edited using GPS Track Editor and converted to shapefiles in ArcGIS v10.3.1 (ESRI, 2014). Porpoise observations were plotted by distance from survey vessel to estimate effective strip width. Porpoise and threat density along the survey line were mapped in 1 × 1 km grid cells, with observations corrected to their actual positions using ArcPy in AcGIS, based on recorded angles and distances from bank/vessel.
The north–south survey area was subdivided in two ways for analysis: (1) three sections (northern, Hukou-Xingzi; central, Xingzi-Duchang; southern, Duchang-Ruihong) and (2) protected versus non-protected areas (Hukou–Laoyemiao Reserve; Laoyemiao Reserve; Laoyemiao Reserve–Longkou Reserve; Longkou Reserve; Xingzi channel; and Longkou Reserve–Ruihong) (Figures 2, 3; Figure S1). Differences in the distance at which porpoises were detected from the survey line between seasons and across the three sections (using observations >250 m from survey line) were investigated using Mann–Whitney U tests and Kruskal–Wallis tests. Individual encounter rates (IER; individuals/km) were used to investigate spatiotemporal variation in porpoise density (Zhao et al., 2013; Rone et al., 2017; Braulik et al., 2018), with seasonal and geographic differences in IER tested using Mann–Whitney U tests. Analyses were conducted in R v3.3.3 (R Development Core Team, 2017). Absolute abundance calculation to correct for animals missed on the survey line was not attempted, as no acoustic data or second independent survey vessel data were available to calibrate detection probabilities, and sampling could only be conducted along a restricted survey route (excluding zig-zag sampling or randomized routes across potential environmental gradients), thus preventing compliance with distance sampling assumptions (Buckland et al., 2001; Thomas et al., 2007).
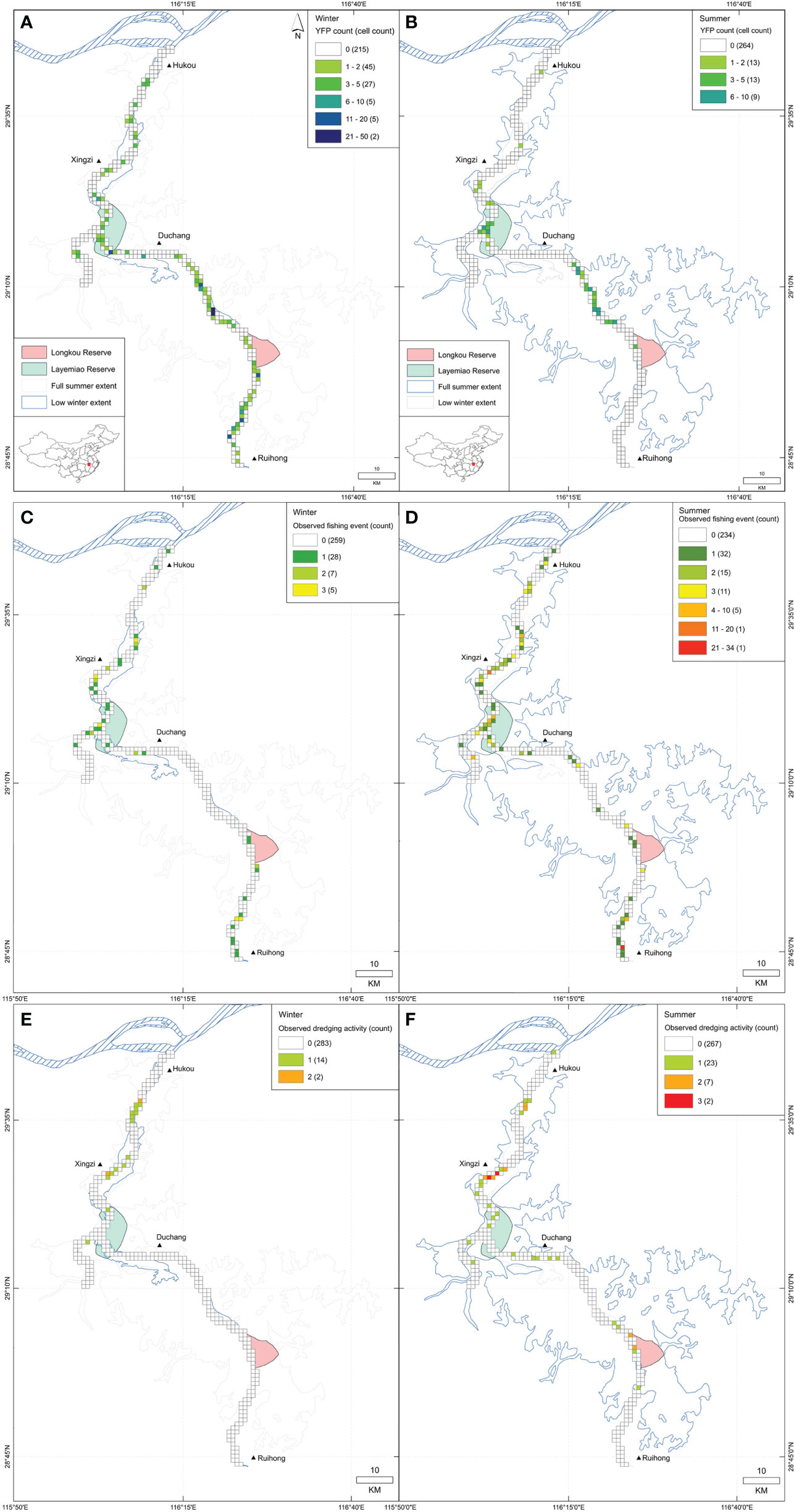
Figure 2 Observations of (A, B) porpoises, (C, D) fishing activity, and (E, F) sand-mining activity in Poyang Lake during winter (A, C, E) and summer (B, D, F).
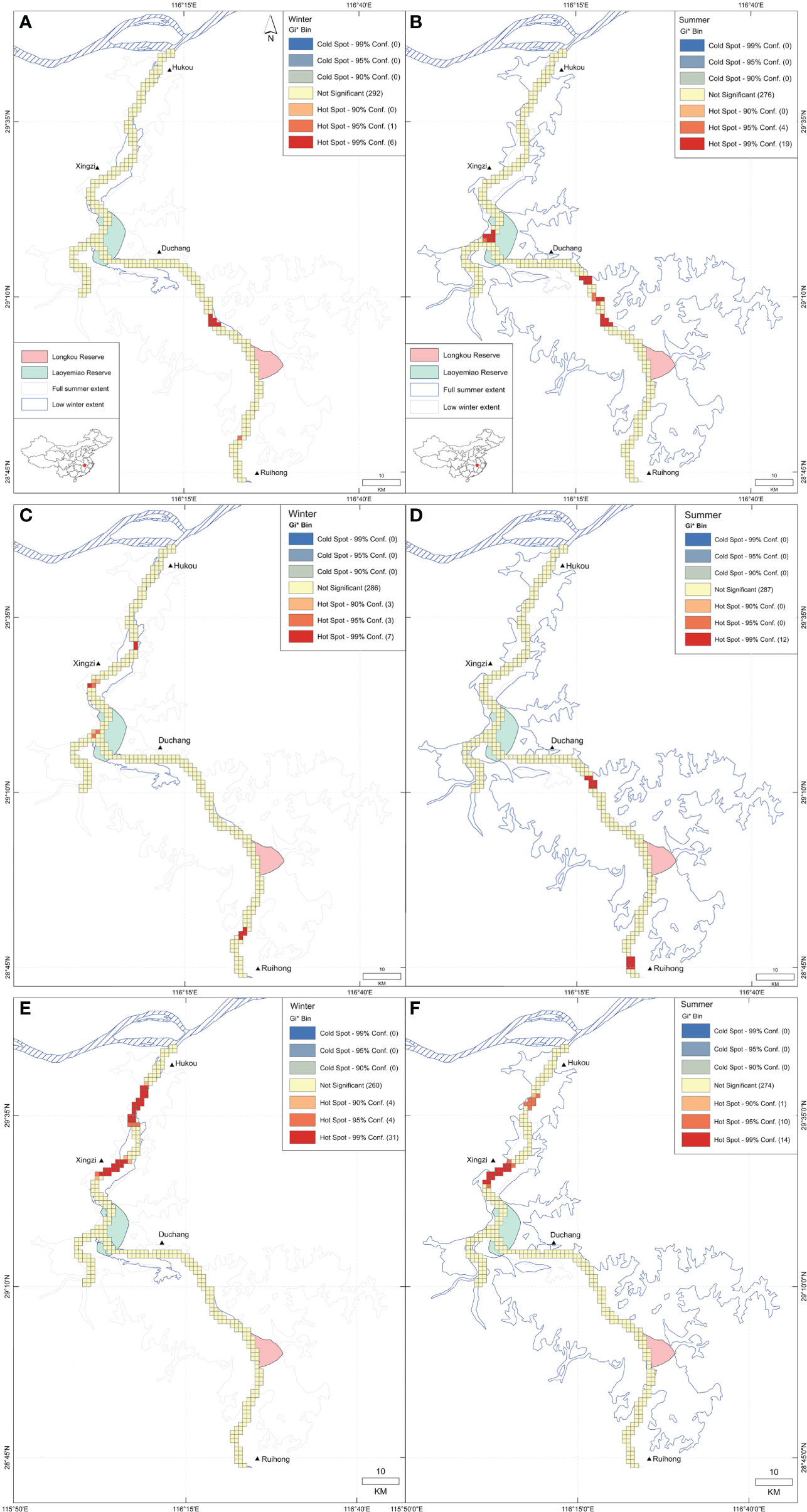
Figure 3 Statistically significant hotspots calculated using Getis-Ord* statistic for (A, B) porpoises, (C, D) fishing activity, and (E, F) sand-mining activity observed in Poyang Lake during winter (A, C, E) and summer (B, D, F).
Spatial and temporal differences in porpoise and threat densities between grid cells were analyzed using Optimised Hot Spot Analysis in the Spatial Statistics toolbox in ArcGIS v10.3.1, using the Getis-Ord* statistic to identify significant clusters of high or low values (Getis and Ord, 1992). Relative spatial distributions were calculated by measuring distances between individual porpoise and fishing/sand-mining vessel observations in summer and winter using the RANN package in R v3.3.3 (Arya et al., 2015).
To investigate whether there was any correlation between the spatial distribution of porpoises and threats across Poyang Lake, pixel-by-pixel Pearson’s correlations were conducted between rasters of porpoise and fishing/sand-mining vessel counts in both seasons in R v4.0.4. Areas in which porpoises or threats were dominant were visually highlighted using bivariate overlap plots produced in QGIS v3.16.4.
To determine whether porpoises and threats were distributed non-uniformly in relation to the two Poyang Lake reserve management areas, chi-squared tests were conducted to compare densities of porpoises and threats exclusively inside and outside each reserve with expected density across the entire survey region. Counts of porpoises and threats within each reserve and across the survey region were divided by the area of each zone to estimate density. As multiple tests were conducted simultaneously for this analysis, a Bonferroni correction was used to adjust the significance threshold, dividing the original threshold (0.05) by number of tests (12) to generate a new threshold at which a difference was considered significant (0.004).
To determine whether porpoises were randomly distributed in relation to threats at a finer scale, identical sample sizes of random points were generated within the same buffer shapefile using the “sp” package in R v3.3.3 (Pebesma and Bivand, 2005), and distances between random points and observed threat data were measured. This process was repeated 10,000 times to create null distributions against which mean distance values from observed data were compared.
Interview survey
Interviews were conducted between 12 September and 14 November 2016, in fishing communities in all seven municipalities/counties around Poyang Lake (Duchang, Hukou, Jiujiang, Poyang, Xingxi, Yongxiu, Yugan), and all five municipalities along the Yangtze mainstem from the mouth of Poyang Lake to Anqing (Anqing, Chizhou, Pengze, Susong, Wangjiang) (Figure 1). Stratified random sampling was used to determine target sample sizes based on available data on numbers of registered fishers for 2011–2012 (Turvey et al., 2015). A target of 3% of registered fishers was aimed for in communities around Poyang Lake, and 10% in communities along the Yangtze mainstem (which had fewer registered fishers), and an upper limit of 50 complete interviews was set per community for logistic reasons. However, targets were not met in every community due to fisher availability (Table 1).
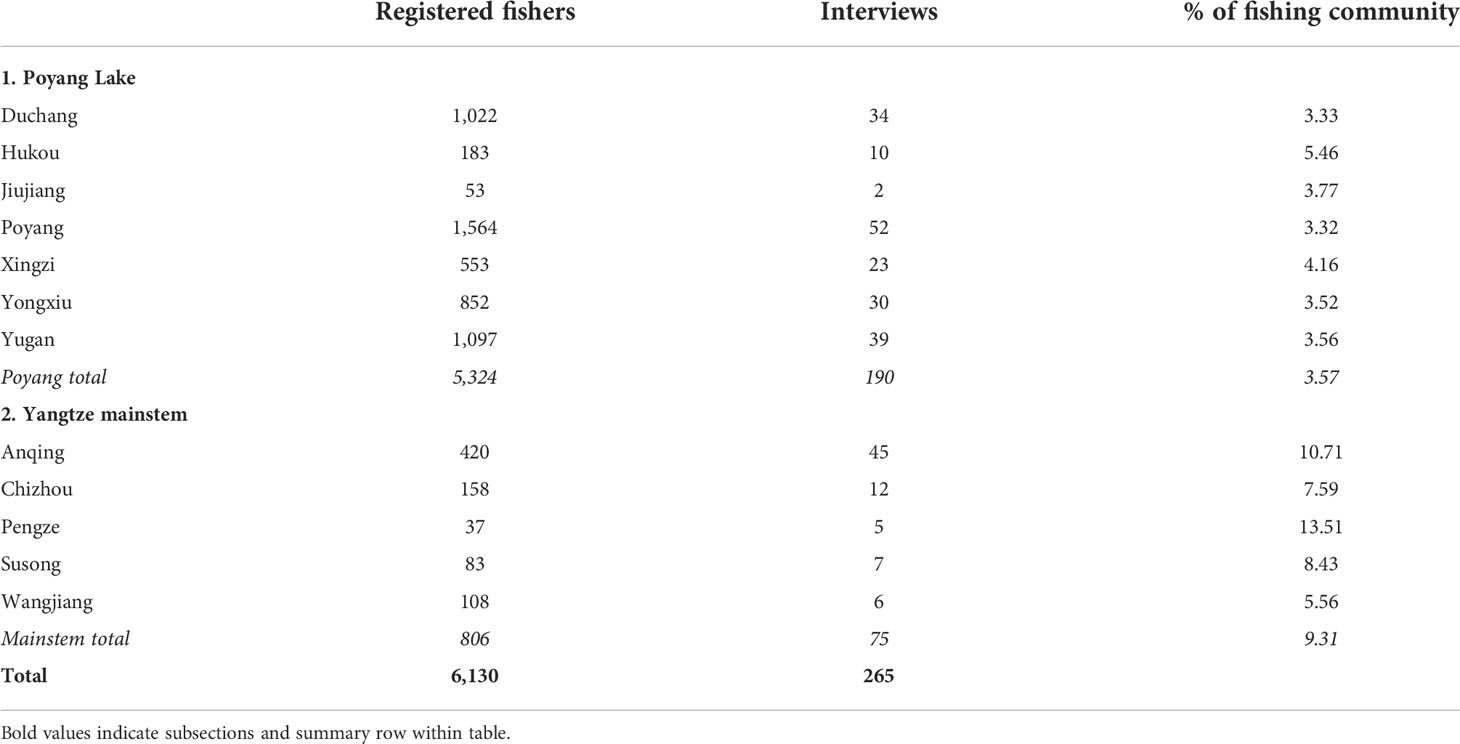
Table 1 Fishing community interviews in Poyang Lake and the Yangtze mainstem. Data on registered fishers from Turvey et al. (2015).
Respondents were identified by representatives from fishing communities known through local project partners, or by visiting known fishing ports to inquire about local fishing villages, and then selecting participants opportunistically by asking anyone encountered whether they were fishers and were happy to be interviewed. Respondents were informed about study aims, that interviews were anonymous, and that they could withdraw at any time or choose not to answer any question; participation was voluntary, with interviews only conducted following verbal consent. Only people aged 18 or above were interviewed. Only one person was interviewed per household to ensure independence of responses. All interviews were conducted on a one-to-one basis in standard Mandarin by native speakers.
A standard questionnaire including closed and open questions was used for all interviews, which took ~45 min to complete (Supplementary Material). Questionnaire design and interview protocols were refined during a pilot survey in fishing markets in Wuhan before the main survey. The survey included questions on respondents’ demographic characteristics; fishing gear use and fishing activity; whether they had seen or heard of dead porpoises, and what they thought had killed them; fishing income and economic background; perceptions of local fish stock status (at the time of the survey and 5 and 10 years earlier); and further questions about hook-based gears, electrofishing, and fishing activities during the fishing ban period, including estimating the proportion of their local community that used hook-based gears and electrofishing at the time of the survey and 5 and 10 years earlier (categories: none, very few, below 25%, up to half, over half, and everyone).
All statistical analyses were conducted in R v3.3.3 (R Development Core Team, 2017). Gears were grouped into functional categories for analysis. Newly collected data were compared with available baseline datasets on regional fishing activities from 2008 (Turvey et al., 2010) and 2011–2012 (Turvey et al., 2015), to assess longitudinal changes in fishery dynamics and bycatch. Wording of questions in our questionnaire was matched directly to questions from these previous surveys to enable direct data comparisons. Demographic differences between respondent samples from different surveys were investigated using ANOVAs.
Differences in relative numbers of reported porpoise deaths associated with different gear categories, and in reported deaths compared to relative numbers of respondents using each gear category, were investigated using chi-squared tests or Fisher’s exact tests (if count data were less than five). Overall and monthly differences in gear use were investigated using two-proportion Z-tests, and differences in perceptions of how many people continue fishing during the ban were investigated using a t-test. Demographic and socioeconomic predictors of gear use were investigated using generalized linear mixed models (GLMMs): respondent income from fishing (scaled to a mean of zero and a range of −1 to 1) and age were included as fixed effects, because these variables are known to influence gear choice in other small-scale fisheries (Wallner-Hahn et al., 2016; Limuna et al., 2018; Samah et al., 2019; Herrón et al., 2020), and interview location was included as a random effect to control for possible spatial differences in gear use (Harrison et al., 2018). Changes in perceived local use over time of two gear categories that have been associated with mortality in Yangtze cetaceans (hook-based gears, electrofishing) were further investigated using Z-tests, first by comparing estimates across all three time intervals (now, 5 years ago, ten years ago) using three-proportion tests, and then by comparing estimates for now and 10 years ago using two-proportion tests if there was no significant difference. Longitudinal changes in use of hook-based gears were also investigated using a three-proportion Z-test through direct comparison of reported levels of its use across the 2008, 2011–2012 and 2016 surveys. Direct data on reported electrofishing use are not available for 2008 or 2011–2012, so relative numbers of respondents who reported porpoises killed by electrofishing were compared instead between 2011–2012 and 2016 using a two-proportion Z-test, as an indirect metric. Perceived changes in fish catch over time were investigated using an ANOVA, and relative numbers of fishers who practice fishing as their sole source of income were compared between 2011–2012 and 2016 using a two-proportion Z-test.
Results
Boat surveys
Mean on-effort survey speed was 10.70 km/h (winter) and 11.59 km/h (summer). Continuous vessel speed data recorded using GPS show that mean speed was significantly faster during winter due to stronger currents (two-sample t-test6079.9 = 6.2077, p < 0.001). However, all mean survey speeds are well above mean porpoise swimming speed (4.5 ± 0.5 km/h; Akamatsu et al., 2002); hence, likelihood of double-counting is very low for both seasons.
There was no significant difference between the distance of all porpoise observations from the survey vessel in winter and summer (Spearman rank correlation: ρ = 0.43, p = 0.299), so they were treated as comparable for further analysis. Visual data inspection showed a drop-off in observations >250 m from the survey line (Figure S2); observations beyond this distance were excluded from further analysis, resulting in a 500-m-wide survey transect. The final dataset (observations within a 500-m transect) contained 334 winter porpoise observations (88.6% of total winter dataset, with 148 groups) and 133 summer porpoise observations (80.3% of total summer dataset, with 53 groups). There were no differences in the distance at which porpoises were observed from the survey line between seasons or sections (all temporal and spatial comparisons, p > 0.05). No seasonal differences were observed in mean group size (summer = 2.51 individuals; winter = 2.24 individuals; two-sample t177 = 0.84, p = 0.400) or mean number of calves/group (summer = 0.23; winter = 0.19; two-sample t94.46 = 0.54, p = 0.591). In winter, porpoises were observed across the survey region at relatively high density, with significant hotspots in central and south Poyang Lake. In contrast, large distribution gaps existed in summer, with no porpoises observed south of Longkou Reserve and very few observed north of Xingzi, and with significant hotspots only in central Poyang Lake (Figures 2A, B, 3A, B).
IER was significantly higher in winter than in summer in northern Poyang Lake (w = 2,995, p < 0.01) and southern Poyang Lake (w = 8,245.5, p < 0.01), but did not differ seasonally in central Poyang Lake (w = 4,812, p = 0.096). In both seasons, IER was higher in southern Poyang Lake compared to northern Poyang Lake (winter, w = 8,104, p < 0.001; summer, w = 6,791, p < 0.01) and was also higher in southern Poyang Lake compared to central Poyang Lake in winter (w = 9,512, p < 0.001). IER was lowest in northern Poyang Lake in summer (w = 3,824, p < 0.05).
Porpoises were observed in both reserves in both summer and winter, although very few porpoises were observed in Longkou Reserve in summer (Figures 2A, B). Laoyemiao Reserve had higher IER compared to the Hukou-Laoyemiao section in winter and summer (winter, w = 1,446.5, p = 0.0246; summer, w = 1,451.5, p < 0.01), the Xingzi channel in winter (w = 456, p < 0.01), and the Longkou-Ruihong section in summer (w = 1,291.5, p < 0.001). Longkou Reserve had higher IER than the Xingzi channel in winter (w = 263, p < 0.05), but showed no statistical differences to other sections in summer. The Longkou Reserve–Ruihong section could not be tested using this analysis in summer, as there were no porpoise observations in this section during this season. One porpoise hotspot was within Laoyemiao Reserve in winter, but no hotspots were detected within either reserve in summer (Figures 3A, B). Density comparisons show that, compared to unprotected parts of the survey area, there are significantly fewer porpoises than expected within Longkou Reserve in summer (χ2 = 20.01, p < 0.001), and significantly more than expected within Longkou Reserve in winter (χ2 = 9.76, p < 0.01) and Laoyemiao Reserve in summer (χ2 = 94.75, p < 0.001) (Table 2).
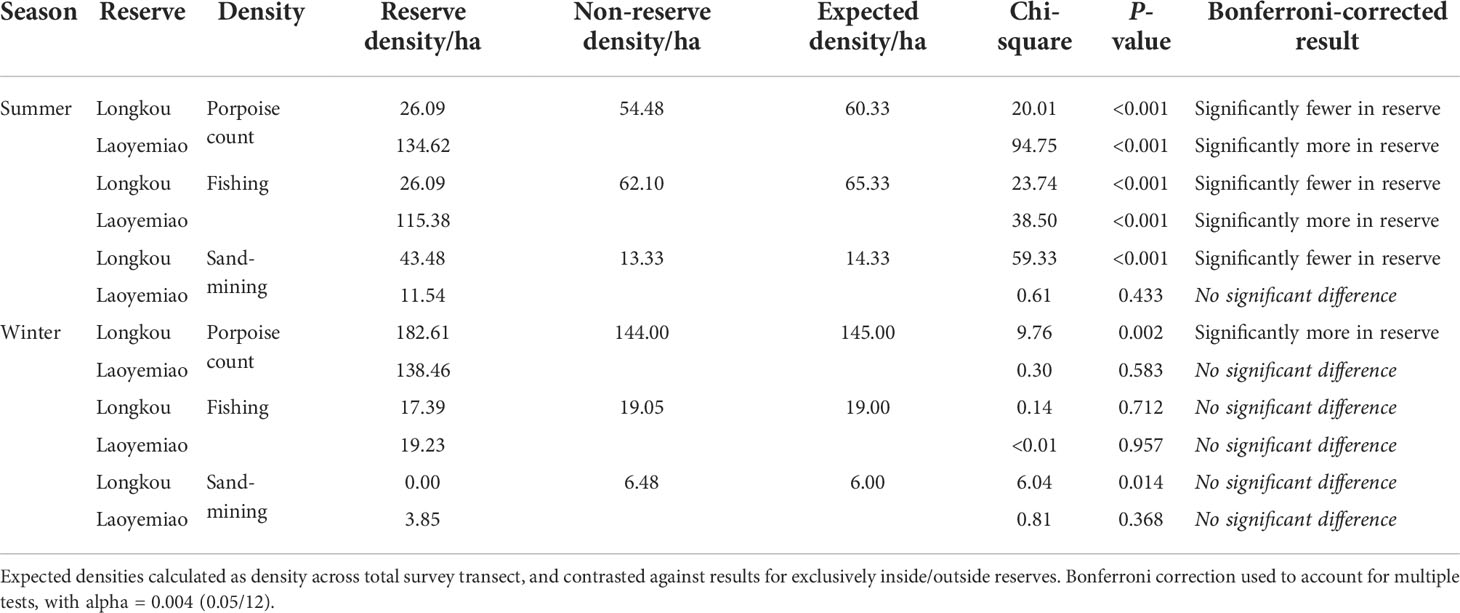
Table 2 Chi-squared tests for significant differences between porpoise, fishing vessel, and sand-mining vessel densities inside and outside Poyang Lake reserves, in contrast with expected uniform density across survey area.
Both threats were observed significantly more frequently in summer (fishing, summer = 93, winter = 56, χ2 = 9.188, p < 0.01; sand-mining, summer = 50, winter = 18, χ2 = 15.059, p < 0.001). Fishing hotspots were present in winter in northern Poyang Lake (Hukou–Duchang, n = 3) and in southern Poyang Lake near Ruihong, and in summer near Ruihong and between Duchang and Longkou Reserve. Fishing was observed within both reserves in summer and winter, with numerous observations within Laoyemiao Reserve, and with a fishing hotspot within this reserve in winter (Figures 2C, D, 3C, D). Sand-mining hotspots were present in both seasons in northern Poyang Lake (around Xingzi and between Xingzi and Hukou), but sand-mining also occurred further south in summer. Sand-mining was observed within Laoyemiao Reserve in winter, and within both reserves in summer (Figures 2E, F, 3E, F). Density comparisons show that, compared to unprotected parts of the survey area, there is significantly more fishing than expected within Laoyemiao Reserve in summer (χ2 = 38.50, p < 0.001), and significantly less than expected within Longkou Reserve in summer (χ2 = 23.74, p < 0.001); and there is significantly more sand-mining than expected within Longkou Reserve in summer (χ2 = 59.33, p < 0.001) (Table 2).
All spatial correlations between porpoises and threats were relatively weak at a landscape scale across Poyang Lake (Figure 4). The strongest correlation was between porpoises and fishing vessels in summer (Pearson’s correlation: 0.225). All other correlations were near zero, suggesting no correlation between variables (fishing, winter: 0.092; sand-mining, summer: 0.044; sand-mining, winter: −0.005).
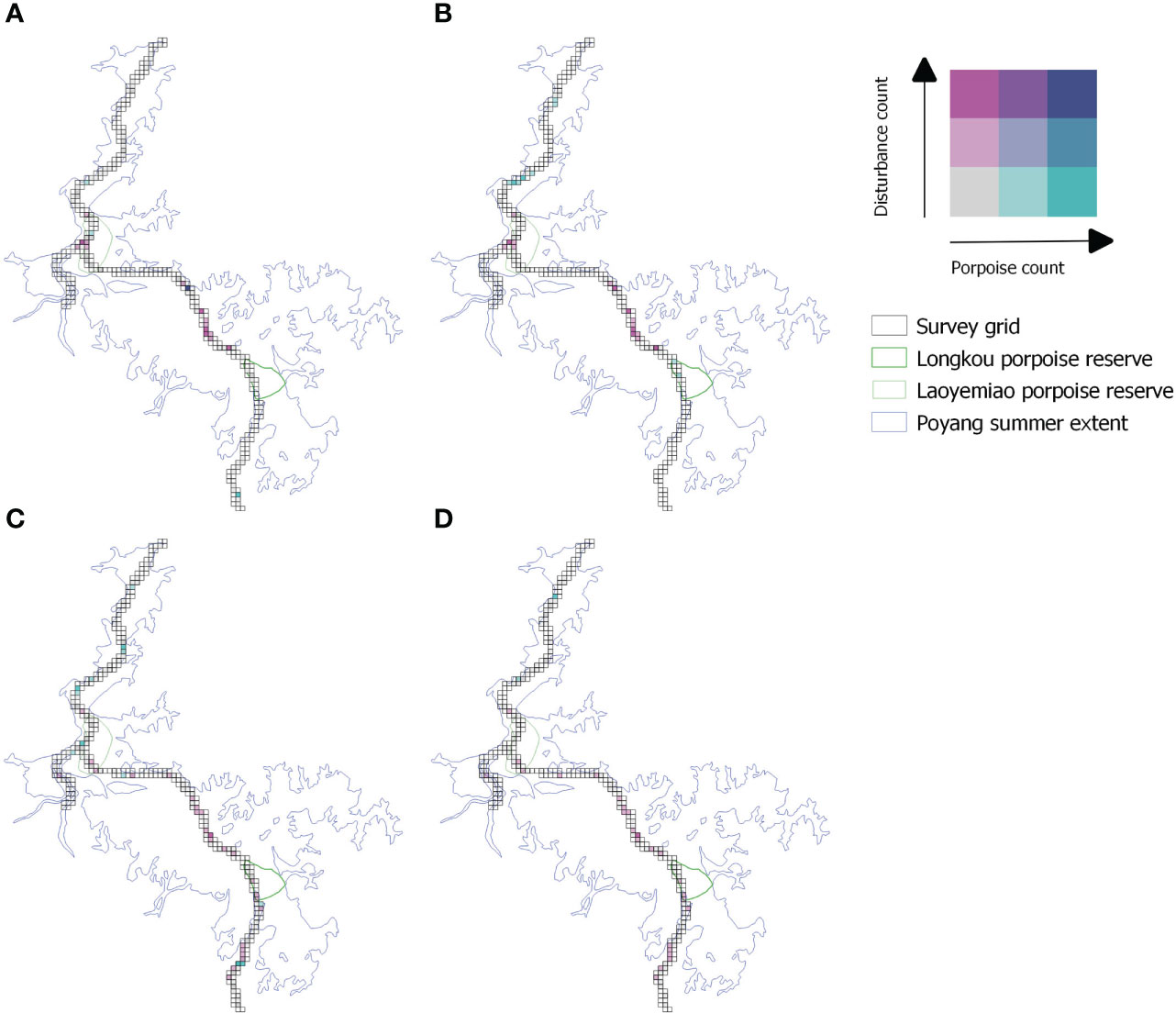
Figure 4 Bivariate plots of porpoise and threat counts: (A) porpoises versus fishing vessels (summer); (B) porpoises versus sand-mining vessels (summer); (C) porpoises versus fishing vessels (winter); (D) porpoises versus sand-mining vessels (winter).
Compared to null distributions, porpoises were distributed significantly further from sand-mining in winter (p < 0.001), and significantly closer to fishing in summer (p < 0.001). There was no difference between observed and null porpoise distributions in relation to sand-mining in summer (p = 0.133) or fishing in winter (p = 0.135) (Figure 5).
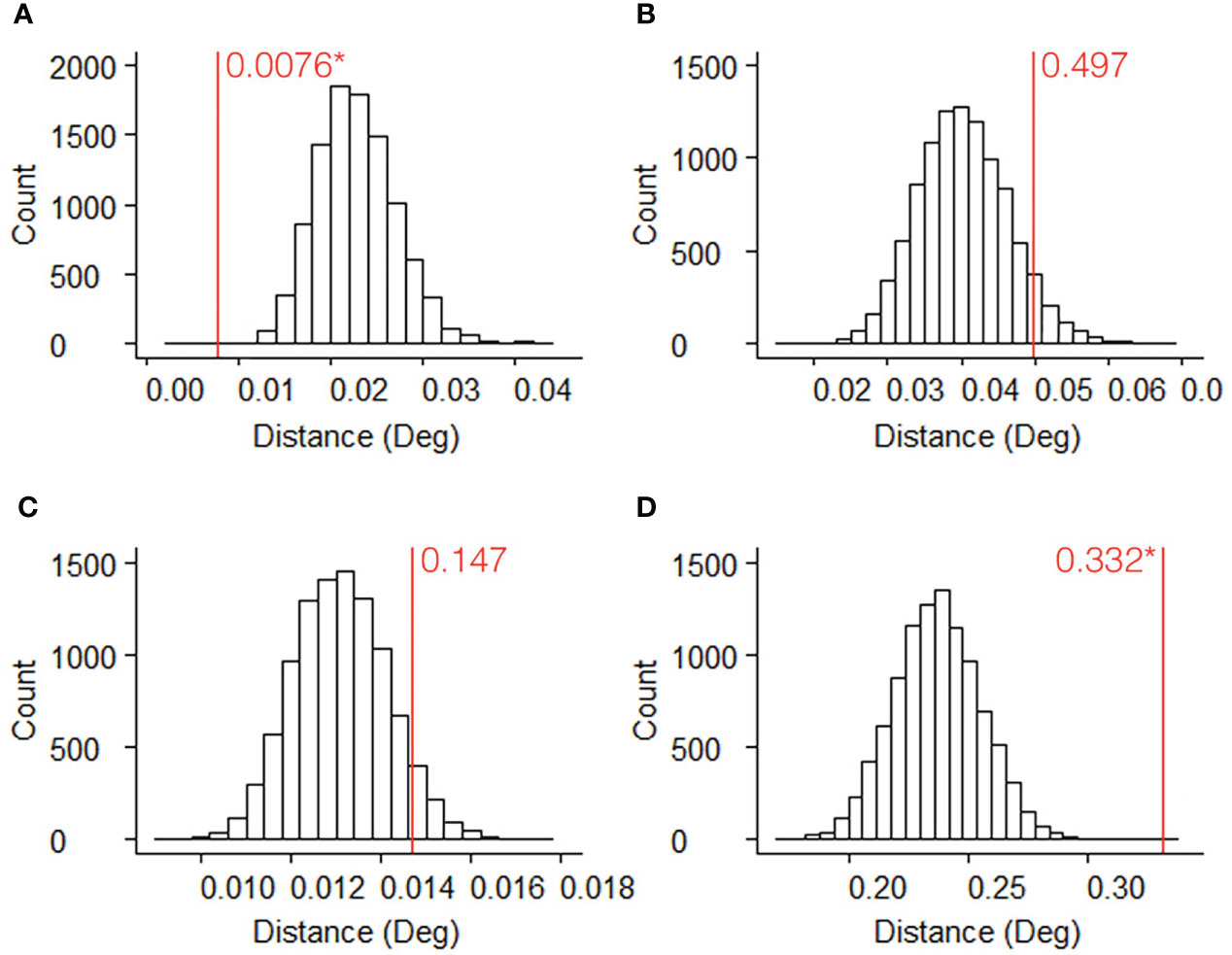
Figure 5 Comparison of simulated null distributions and mean observed distances (red bars and values) between porpoises and threats: (A) fishing (summer); (B) fishing (winter); (C) sand-mining (summer); and (D) sand-mining (winter). Asterisks denote significant differences at the 1% level.
Interview survey
A total of 265 interviews were conducted (190 at Poyang Lake, 75 along the Yangtze mainstem), although not all respondents answered all questions (Table 1). Mean respondent age was 50.8 years (range = 25–77; SD = 10.1); this was not significantly different from the age profile of respondents in the 2008 survey (52 ± 12.8 years, n = 527; ANOVA: F(2,1202) = 7.198, p = 0.377) or the 2011–2012 survey (49.1 ± 10.9 years, n = 417; ANOVA: F(2,1202) = 7.198, p = 0.147). Mean respondent income from fishing was 31,136 CNY (range = 2,500–150,000 CNY; SD = 21,454).
Reported gears represent six main functional categories: (1) non-fixed nets (includes both free-floating gill nets and drag nets, as some net types are reportedly used in both ways and/or local net names are variably used; reported by 70.8% of respondents); (2) static pots and traps for catching crabs, shrimp, and small fish (67.3%); (3) fixed nets (semi-permanently fixed to river/lake bed; 18.5%); (4) hook-based gears (13.8%); (5) cormorant fishing (8.1%); and (6) electrofishing (6.9%) (“other” gears also reported by 3.1% of respondents). Respondents reported using between one (23.2%), two (44.3%), three (27.1%), and four (5.3%) different gear categories.
In total, 67 porpoise bycatch deaths were reported. Dates for many deaths were not reported, but 32 were stated to have occurred during the 12-month period preceding the survey. Deaths were reported in non-fixed nets (n = 31), electrofishing (n = 19), fixed nets (n = 10), and hook-based gears (n = 7). Porpoise bycatch mortality data are also available for the study region from 2008 and 2011–2012 (combined deaths reported across all three surveys, n = 145: non-fixed nets, n = 55; hook-based gears, n = 41; electrofishing, n = 28; fixed nets, n = 21). Relative numbers of deaths associated with the six gear categories are significantly different for both the combined 2008–2016 data and the 2016-only data (Fisher’s exact test, p < 0.001 for both tests), and across the four categories for which deaths were reported in both datasets (2008–2016: χ2 = 24.818, df = 3, p < 0.0001; 2016: χ2 = 27.761, df = 3, p < 0.001). For the 2016 dataset, count data were also compared across gears, in terms of number of reported deaths per gear category as a proportion of the number of fishers reporting use of that category [fixed nets, 10/48 (0.208); hook-based gears, 7/36 (0.194); non-fixed nets, 31/184 (0.168); pots and traps, 0/175; cormorant fishing, 0/21]; electrofishing was removed from these comparisons because of the likelihood of fishers underreporting use of this illegal method (see Discussion). These comparisons show significant differences across all categories (Fisher’s exact test, p < 0.001), but not across the three categories with reported deaths (χ2 = 0.478, df = 2, p = 0.788).
Gear use differs between Poyang Lake and the mainstem, with a higher proportion of fishers in the mainstem reporting use of non-fixed nets (mainstem = 89.3%, Poyang = 61.9%; χ2 = 19.3, df = 1, p < 0.001), and a higher proportion in Poyang Lake reporting use of pots and traps (mainstem = 51.2%, Poyang = 75.0%; χ2 = 13.6, df = 1, p < 0.001), electrofishing (mainstem = 0%, Poyang = 10.2%; χ2 = 7.7, df = 1, p < 0.05), and cormorant fishing (mainstem = 0%, Poyang = 11.9%; χ2 = 9.4, df = 1, p < 0.05) (Figure 6). Fishing activity is highly seasonal in both regions, with highest activity in summer and a peak in June (89.7% of respondents active) in Poyang Lake, and highest activity in late summer–autumn and a peak in September (82.9% of respondents active) in the mainstem. The proportion of active fishers differs significantly between the two regions in January (χ2 = 8.6, df = 1, p < 0.05), February (χ2 = 13.7, df = 1, p < 0.001), May (χ2 = 4.4, df = 1, p < 0.05), June (χ2 = 1.1, df = 1, p < 0.001), July (χ2 = 15.1, df = 1, p < 0.001), September (χ2 = 8.8, df = 1, p < 0.01), October (χ2 = 25.8, df = 1, p < 0.001), November (χ2 = 27.5, df = 1, p < 0.001) and December (χ2 = 13.3, df = 1, p < 0.001) (Figure S3). Many respondents reported fishing even during months when it is banned completely in both Poyang Lake (April, 21.6% of respondents; May, 27.6%) and the mainstem (April, 10.5%; May, 14.5%; June, 15.8%), with respondents in Poyang Lake tending to estimate that a greater percentage of their local community continued to fish during the ban compared to mainstem respondents (Poyang Lake mean estimate = 10.2%, mainstem = 4.3%; t = −2.9, df = 197.1, p < 0.001).
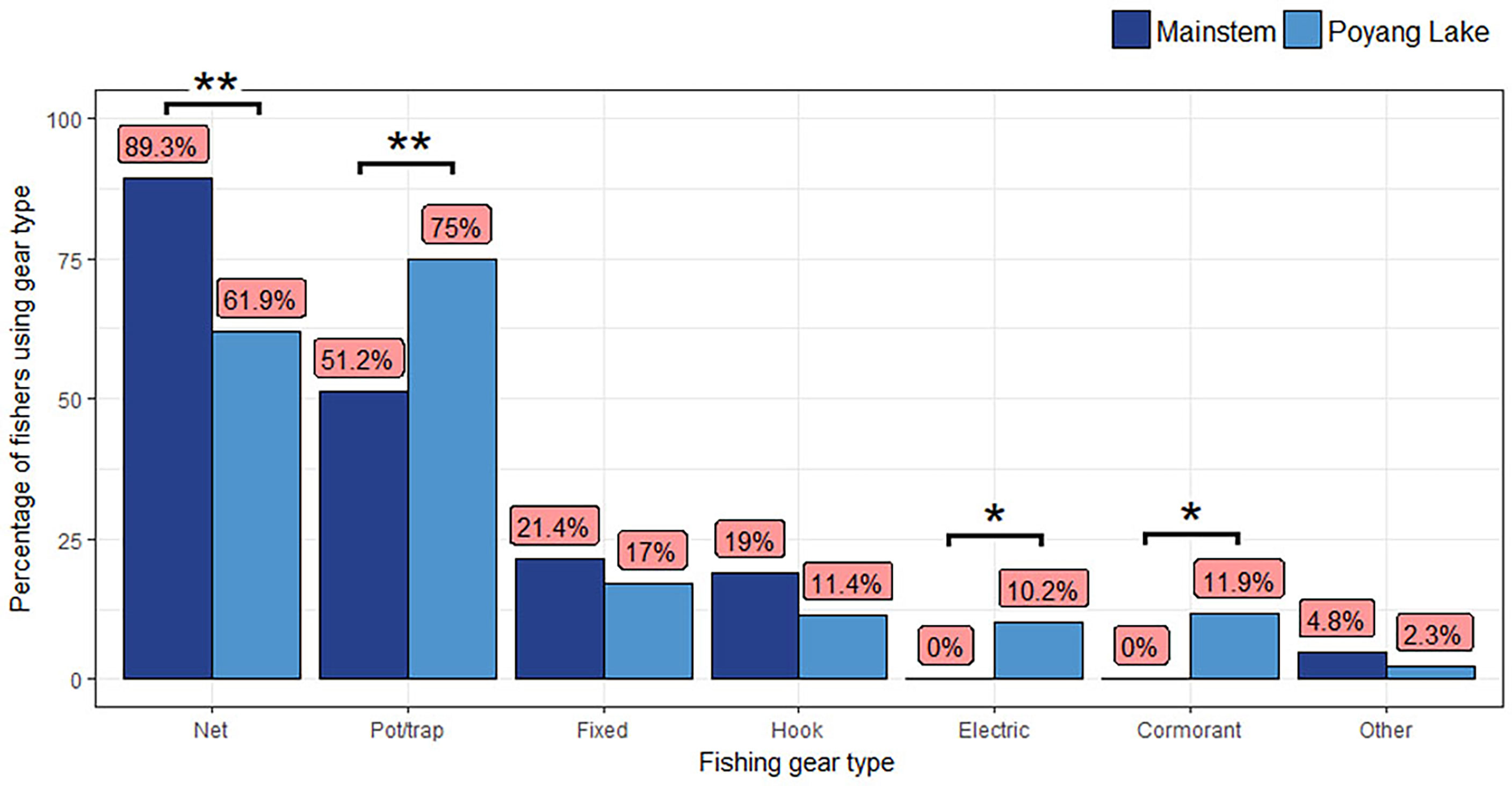
Figure 6 Differences in functional fishing gear use by fishers in Yangtze mainstem and Poyang Lake. Asterisks indicate significant differences between regions at the 5% level (*) and 1% level (**).
Use of hook-based gears is positively correlated with respondent age (effect size = 0.076, SE = 0.024, p < 0.01), and use of pots/traps is negatively correlated with respondent age (effect size = -0.054, SE = 0.017, p < 0.01); no predictors were significantly correlated with use of other gears in GLMMs (Table S2). The percentage of respondents using hook-based gears declined significantly over time across the three surveys, from 36.1% in 2008 to 27.3% in 2011–2012 and 13.8% in 2016 (χ2 = 42.265, df = 2, p < 0.001). Respondents in 2016 also estimated that the percentage of the local community who used hook-based gears had declined from 10 years ago to 5 years ago to the present, with lowest-use categories increasing over these progressive time periods (none: χ2 = 21.6, df = 2, p < 0.001; very few: χ2 = 12.2, df = 2, p < 0.05), and higher-use categories decreasing over time (below 25%: χ2 = 6.2, df = 2, p < 0.05; up to half: χ2 = 12.0, df = 2, p < 0.05; over half: χ2 = 17.5, df = 2, p < 0.05; everyone: χ2 = 23.7, df = 2, p < 0.05) (Figure 7). Reported reasons for using hook-based gears included because they were traditional and/or they catch bigger fish.
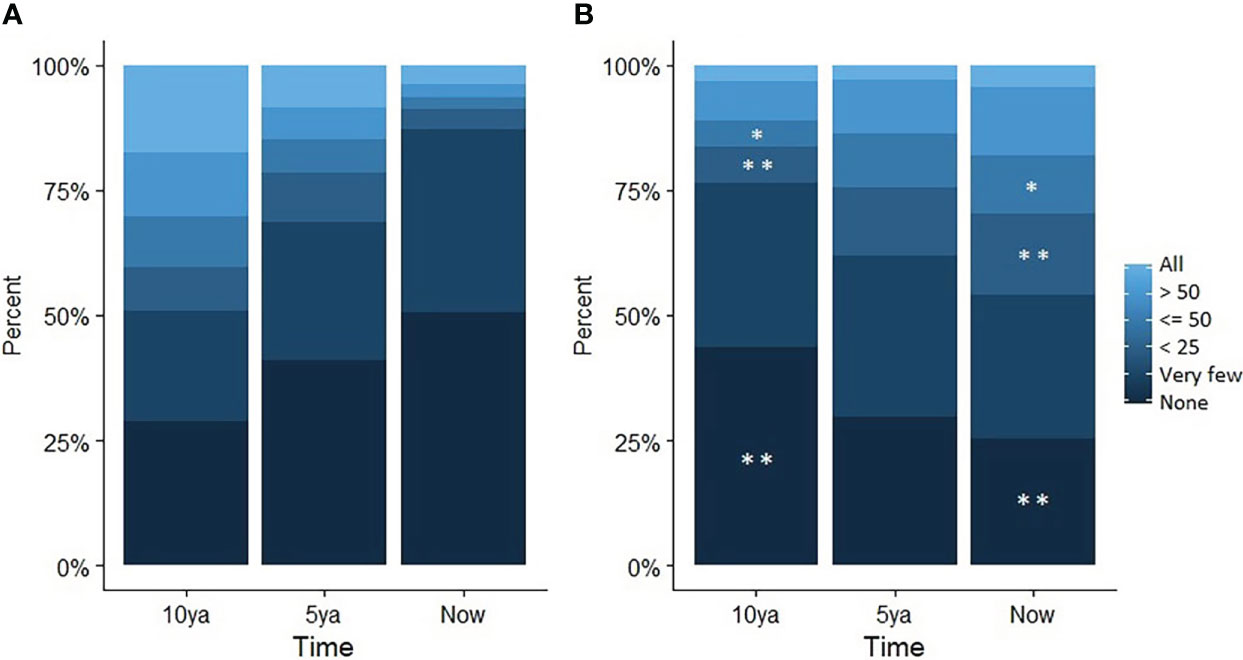
Figure 7 Changes in fishers’ perceptions of changing use over time (now, 5 years ago, and 10 years ago) for (A) hook-based gears, (B) electrofishing. Color categories indicate proportion of local community that respondents thought used different gears now and in the past. For changing hook-based gear use, all years and all groups are significantly different; for changing electrofishing use, asterisks indicate significant differences within same abundance categories between years at the 5% level (*) and 1% level (**).
Conversely, respondents estimated that electrofishing had increased from 10 years ago, with the “none” category decreasing over these progressive time periods (χ2 = 14.3, df = 1, p < 0.001), and two higher-use categories increasing over time (below 25%: χ2 = 7.3, df = 1, p < 0.001; up to half: χ2 = 4.4, df = 1, p < 0.05) (Figure 7). Reported reasons for practicing electrofishing included because there are fewer fish now, and this method is easy to use, can catch fish more easily compared to other gears, and/or can make more money. The percentage of respondents who reported a porpoise death from electrofishing also increased significantly from 7.7% in 2011–2012 to 12.8% in 2016 (χ2 = 3.90, df = 1, p < 0.05).
The percentage of respondents who stated fishing was their sole source of income was 67.3%, representing a significant decrease across the same region from 94.5% in 2011–2012 (χ2 = 80.9, df = 1, p < 0.001). In total, 60.1% of respondents reported that their income was unstable, due to decrease in fish stocks (20.0% of respondents) unstable catch (16.4%) or water-level fluctuations (10.0%), and 86.5% stated they would be willing to adopt alternative livelihoods if offered. Mean and median values for mean daily catch were progressively lower from 2005 to 2015, but data showed high standard deviations and no significant difference over time [F(1,513) = 1.276, p = 0.259].
Discussion
Our study combines existing and novel ecological and social science datasets to establish an integrated baseline on threats to Yangtze finless porpoises. We provide novel insights into the distribution of potential threats facing an at-risk cetacean population across protected and unprotected landscapes, overlap between porpoises and threats at different spatial scales, and patterns of seasonal and longer-term variation in these threats. Analysis across multiple spatial and temporal scales provides new information about interactions and risks from different anthropogenic activities, and possible factors associated with local porpoise vulnerability or resilience and differing status between landscapes. Together, these findings help to understand the dynamics and implications of porpoise–threat interactions across different conservation-priority landscapes, to inform regional mitigation and management.
Although distance sampling is widely used for estimating cetacean abundances and distribution patterns, this approach could not be employed in our study because we were forced to follow a restricted survey route in Poyang Lake. However, encounter rates are also widely used to understand relative abundance and distribution patterns for cetaceans (Dan, 1988; O’Hern et al., 2014; Marcoux et al., 2016; Moore and Barlow, 2017; Rone et al., 2017) and investigate spatial relationships between cetaceans and threats (Braulik et al., 2018). Estimation of encounter rates has also been the primary survey method for previous porpoise monitoring in Poyang Lake, with population trends for 2006–2017 based on encounter rate analysis (Zhao, 2009; Huang et al., 2020). Encounter rates provide a comparative index of abundance if survey methods and conditions are consistent; although absolute abundances are underestimated through decreasing detectability further from the observer, this does not invalidate relative comparisons if detectability does not vary between surveys (Dan, 1988). Our dataset was obtained using consistent visual-only observation methods, and shows no consistent biases in detectability or variation in negative encounter rate bias with increasing distance (e.g., due to weather). It is thus suitable for making spatial and temporal comparisons of porpoise and threat distributions, and although we recognize the risk of type II statistical error (reduced ability to detect genuine differences between surveys due to decreased power), we interpret statistical differences detected in our comparative analytical approach as representing valid differences within the underlying system. Distance sampling involves estimation of how many individuals were undetected by observers at differing distances, and thus would also not be a suitable framework for investigating spatial relationships between observed porpoises and observed threats; as our data show no consistent biases in porpoise detectability across Poyang Lake or between seasons, we can also include comparative assessment of this important baseline.
As we were forced to follow a restricted survey route in Poyang Lake, interpretation of boat survey data must be restricted to our transect strip, rather than extrapolated to infer possible conditions elsewhere across the lake system. This is particularly the case for summer survey data, when lake area extended far beyond the 500-m strip. Despite this obvious and unavoidable limitation in survey design and spatial representativeness, we consider it important to report these and other available survey data for Poyang Lake; otherwise, a scientific evidence-base on the relative status of porpoises across this conservation-priority landscape will remain unavailable for management planning. Our boat survey data thus provide a starting baseline to guide future broader-scale spatial investigation and decision-making.
Between-landscape differences in threats
At the broadest scale, our fisher interviews demonstrate significant differences in local fishing activities between Poyang Lake and the adjacent Yangtze mainstem, with differing temporal fishing activity patterns and differences in widely used gears. Importantly, the locally commonest gears are associated with differing numbers of reported porpoise bycatch deaths, raising the possibility that varying porpoise status between landscapes might be associated with differential usage of varyingly harmful gears.
Static fishing pots and traps, the most widely used gear in Poyang Lake, are not associated with any reported porpoise deaths. Similar creel fisheries are typically associated elsewhere with bycatch only in large cetaceans (Johnson et al., 2007; Dolman and Brakes, 2018), and have reduced impacts on other non-target species or damage to local habitats (Shester and Micheli, 2011). It is therefore possible that greater usage of pots and traps in Poyang Lake might be a factor contributing to greater local porpoise abundance and more stable population status.
In contrast, non-fixed nets (gill nets and drag nets) are the most widely-used gear in the adjacent Yangtze mainstem, and are associated with 31 reported porpoise bycatch deaths in our dataset. Non-fixed nets are not the most lethal gear in this system in relative terms, as statistically similar numbers of porpoise deaths were also associated with both fixed nets and hook-type gears when measured as a proportion of the number of fishers using each gear. However, they are the most lethal gear in absolute terms: they are used by at least two-thirds of fishers in both the mainstem and Poyang Lake, meaning that they are associated with the highest overall number of reported bycatch deaths. We recognize that our analyses were conducted using only broad functional gear categories, and recommend further research into the dimensions and fine-scale spatial and temporal usage patterns of particularly harmful gears reported to kill porpoises (Dewhurst-Richman et al., 2020; Kelkar and Dey, 2020). However, we highlight spatial variation in gear use as a potential factor to account for varying porpoise status between these landscapes.
Within-landscape variation in porpoise and threat distribution
Despite the potentially limited representativeness of our restricted transect-strip data for the wider Poyang system, our boat surveys provide insights into changing patterns of porpoise and threat distribution across seasons and transect sections. Porpoises are distributed widely along the narrow surveyed lake channel in winter, but become more restricted to central sections of the trackline in summer, with shifting spatial hotspots and density patterns between seasons. Fewer individuals were also observed along the trackline in summer, as expected due to increased lake size during this season. It is important to understand which ecological or anthropogenic factors regulate these distributional patterns (Liu et al., 2021). Our analyses indicate little spatial correlation between porpoise and threat counts within 1 × 1 km grid cells along our trackline, and seasonal porpoise and threat hotspots are generally distributed in different transect regions, indicating that porpoises tend to be distributed in areas with reduced fishing or sand-mining along the narrow surveyed section. Previous research has shown that Yangtze finless porpoises can feed in areas of high ship density, and can tolerate disturbance from large vessels in port areas when trying to find prey (Wang et al., 2014; Wang et al., 2015b); the spatial patterns we observe might therefore reflect indirect factors such as restriction of sand-mining activities to north Poyang Lake in winter due to shallow water (de Leeuw et al., 2010), contrasting with the wider distribution of winter porpoise hotspots. However, these findings raise concerns over possible spatial avoidance or exclusion of porpoises from areas with high threat levels, possibly due to high local disturbance, as documented for small cetaceans in other landscapes containing intensive fishing or vessel traffic (Morteo et al., 2012; Campana et al., 2017).
Evaluation of the effectiveness of management actions is crucial, but has not yet been conducted in a transparent manner for conservation efforts aiming to mitigate threats to porpoises across the Yangtze system. Our findings provide worrying insights into the effectiveness of existing protective measures in Poyang Lake. Our transect sections within both reserve management areas had higher porpoise encounter rates than some unprotected sections based on IER analysis, one winter porpoise hotspot fell within the trackline through Laoyemiao Reserve, and there were significantly more porpoises than expected along the trackline through Longkou Reserve in winter and Laoyemiao Reserve in summer based on density comparisons. However, porpoise hotspots were mostly in unprotected parts of our transect, and there were significantly fewer porpoises than expected within Longkou Reserve compared to other transect sections in summer based on density comparisons. Fishing was observed across both reserves in both seasons, and sand-mining was observed across both reserves in summer and Laoyemiao Reserve in winter. Although there was significantly less fishing than expected within Longkou Reserve compared to transect sections in summer based on density comparisons, there was otherwise little evidence of reduced threats within either reserve. In fact, density comparisons with unprotected transect sections showed more fishing within Laoyemiao Reserve and more sand-mining within Longkou Reserve in summer, and a winter fishing hotspot was detected within the trackline through Laoyemiao Reserve. These findings therefore suggest that Poyang Lake’s protected areas are neither particularly well-placed to protect porpoises, nor well-managed to reduce threats, highlighting the need for further wider-scale evaluation of their effectiveness.
Fine-scale interactions between porpoises and threats
In contrast to generally low spatial correlation between distributions of porpoises and threats at the landscape level across the Poyang Lake transect, our fine-scale distance-to-threat analyses demonstrate that porpoises are affected in different ways by different anthropogenic processes in different seasons, showing temporal variation in local-scale movements of individual animals and how they interact spatially with two potential threats.
Local distribution of porpoises within Poyang Lake showed no spatial relationship with sand-mining in summer, but porpoises were distributed significantly further from this activity in winter. This pattern might indicate that porpoises are actively distancing themselves from sand-mining during winter, when porpoises and human activities are spatially concentrated within the narrow lake channel and can come into closer proximity. These results highlight the possibility that sand-mining causes seasonal behavioral disruption to porpoises due to underwater noise and other disturbance, which could have energetic and fitness consequences as porpoises must forage nearly continuously (Wisniewska et al., 2016). Quantifying the acoustic effects of sand-mining should be investigated urgently, including the distance at which noise dissipates to levels that do not cause injury, distress, or behavioral disruption to porpoises. However, the geographic restriction and reduced level of sand-mining in Poyang Lake during winter suggests that direct impacts on porpoises may be less severe than if possible avoidance behavior was detected during summer.
Conversely, porpoises showed no fine-scale spatial relationship with fishing during winter, but were observed significantly closer to this activity during summer. Porpoises and fishing vessels in summer also showed the highest spatial correlation in broader landscape-level analysis. This contrasting pattern might reflect exploitation of similar resources by porpoises and fishers; Yangtze finless porpoises are known to prefer areas with higher fish densities (Kimura et al., 2012; Wang et al., 2014; Mei et al., 2017), which are also preferentially targeted by fishing activities. Spatial overlap with fishing activities is demonstrated for several small marine and freshwater cetaceans (Hastie et al., 2004; Kelkar et al., 2010; Breen et al., 2016; Revuelta et al., 2018; Paudel et al., 2020), but comparable patterns have not previously been demonstrated for porpoises within the Yangtze system. These findings provide important evidence for fine-scale spatial overlap of porpoises with fishing activity within a key conservation landscape, highlighting the potential for fisheries-related mortality through incidental bycatch.
The contrasting temporal pattern of porpoise–fisheries overlap might suggest reduced bycatch risk within Poyang Lake, as porpoises and anthropogenic activities are not concentrated into a narrow channel in summer. However, our boat survey data and interview data both indicate that fishing activity is significantly greater during summer within Poyang Lake. Our results thus demonstrate an increased risk of bycatch due to fine-scale spatial overlap when fishing activities are most intensive.
Seasonal and longitudinal changes in threats
Seven porpoise deaths associated with bycatch in hook-based gears were reported by our respondents, and 41 further bycatch deaths in this gear were recorded in the 2008 and 2011–2012 surveys (Turvey et al., 2013; Turvey et al., 2015). Whereas there are no spatial differences in hook-based gear use between Poyang Lake and the mainstem, our results demonstrate that it is considered “traditional” and associated with older fishers, with reported use decreasing by about two-thirds in this region between 2008 and 2016. These findings thus provide a note of hope, as they indicate that a harmful fishing method has declined in use. However, whereas hook-based gears are often identified as a particularly lethal gear for Yangtze cetaceans (Zhou and Wang, 1994; Zhou et al., 1998; Turvey et al., 2007; Turvey et al., 2013), we found no significant differences in porpoise mortality associated with fixed nets and non-fixed nets, which were both used more widely by respondents. These findings highlight the need for more systematic investigation of porpoise deaths in the Yangtze system, to obtain direct data on deaths by different gears (e.g., through a regional strandings investigation program; Wang et al., 2015a).
Worryingly, electrofishing has reportedly increased over the same period. The negative impact on porpoises of this illegal method remains difficult to determine. Although several porpoise deaths from electrofishing were reported by respondents, it is challenging for non-specialists to identify electrofishing as a cause of death due to the lack of distinctive external marks it leaves on cetacean carcasses, leaving the accuracy of these reports unclear (Thomas et al., 2019). The relatively high number of reported porpoise deaths from electrofishing also contrasts with the very low level of electrofishing admitted directly by respondents, a pattern also seen in previous interview surveys in Yangtze fishing communities (Turvey et al., 2013; Mei et al., 2019). This is likely to indicate that respondents are underreporting their own involvement in this illegal behavior; in contrast to other regional fishing legislation, nearly all fishers across the region know electrofishing is illegal, as they have been exposed to extensive outreach programs and enforcement of electrofishing legislation (Turvey et al., 2015). Conversely, it is possible that electrofishing is conducted by different people to the fishers we interviewed (e.g., opportunistic fishers aiming to supplement non-fishing income, instead of full-time traditional fishers), as our respondents reported that this method is easy to use and does not require as much specialist knowledge or equipment compared to other gears. Understanding actors, usage patterns, and impacts on porpoises and other biodiversity are key knowledge gaps about electrofishing within the Yangtze system that require urgent further study, not only through direct investigation of porpoise mortality but also by using social science techniques specifically designed to obtain information about sensitive behaviors (Nuno and St John, 2014).
Our interviews also reveal that a substantial proportion of the regional fishing community continued to fish during the ban period in 2016, especially in Poyang Lake. A new 10-year ban on all commercial fishing came into force in January 2020 across the Yangtze mainstem and its adjoining lakes, in an effort to provide better protection for threatened aquatic biota (Mei et al., 2020). We hope that this new ban will prove effective for Yangtze biodiversity. Indeed, many respondents highlighted the ongoing decrease in fish stocks and unstable fish catch that make improved conservation measures for the wider Yangtze ecosystem a necessity, and also raise concerns about a declining prey base for porpoises. However, as our results demonstrate that considerable fishing has continued in recent years during previous bans, some fishing activity may therefore be expected to continue illegally within priority porpoise landscapes despite this new legislation (with greatest risk of ongoing fishing during summer, based on current seasonal fishing patterns). Indeed, although the number of people within the Yangtze region who depend on fishing as their sole source of income has decreased over the past decade, and the great majority of respondents were potentially willing to adopt alternative livelihoods if offered, over two-thirds were still entirely dependent on fishing for their livelihoods in 2016. These findings highlight the need both for vigilance in enforcing the new ban and for provision of effective and appropriate support for the many low-income communities that will be impacted economically, socially, and culturally by this new legislation.
Conservation recommendations
The challenges to overcoming bycatch are complex, especially in systems such as the Yangtze that support large, low-income human populations who have been historically dependent on fishery resources. The optimal long-term solution is conversion to alternative efficient and inexpensive fishing methods that can replace gears known to be harmful to cetaceans, but without jeopardizing local livelihoods (Brownell et al., 2019). Our results suggest that one potential “win–win” option for local biodiversity and human wellbeing could be a shift toward wider use of static fishing pots and traps as a viable “porpoise-friendly” alternative to other methods. This gear is already used extensively across the region (including over three-quarters of Poyang Lake respondents), especially by younger fishers, and is associated with zero known porpoise mortality. We recommend further investigation of the potential for increasing pot and trap fishing across the Yangtze system, in terms of its spatiotemporal use and how this relates to porpoise habitat use and landscape-level distribution; its sustainability for target catch species and other components of regional biodiversity; and in an economic context for maintaining local fishery livelihoods (Königson et al., 2015; Petetta et al., 2021).
The unavoidable spatial limitations of boat-based surveys in Poyang Lake highlight the need for additional survey replicates to increase the power of our results, and additional porpoise survey methods to complement existing baselines and fill known data gaps, especially for our spatially restricted summer survey dataset (e.g., shore-based surveys, aerial surveys; Embling et al., 2015; Fürstenau Oliveira et al., 2017). Furthermore, our data cannot in themselves indicate whether bycatch is a more serious or significant threat than sand-mining or other uninvestigated anthropogenic pressures, necessitating further research into the dynamics of human–environmental relationships within this system. In particular, additional research is required into individual-level and population-level effects on porpoises of other vessel traffic, other sources of anthropogenic noise and habitat alteration, and pollutants known to affect cetacean reproductive success and survivorship, all of which remain poorly understood in the Yangtze (Mei et al., 2021; Mogensen et al., 2022). We encourage future investigation to determine multi-scale relationships between porpoises, threats, and biotic and abiotic environmental variables that might regulate porpoise habitat use, along with investigation of additional anthropogenic activities, to work towards establishing a more nuanced understanding of the sustainability of different threats to porpoises.
However, our study establishes a new baseline on the spatiotemporal relationships and interactions between a highly threatened freshwater cetacean and different regional threats, providing novel insights into both potential bycatch risk and sand-mining impacts within a partly protected social–ecological system that also supports a dense human population dependent on natural resources. Our findings emphasize the need for further evidence-based evaluation of reserve effectiveness, improved threat mitigation within protected areas, and re-designation of reserves at sites that maximize benefits to porpoises. More widely, effective conservation decision-making in social–ecological systems requires both ecological baselines on threatened species and social science baselines on human activities and their economic or cultural drivers. We recommend the use of multiple complementary methods and longitudinal datasets to determine the status of other poorly known species, the sustainability and dynamics of different human pressures, and the effectiveness of conservation actions for cetaceans and other wildlife in complex multi-threat landscapes (e.g., other Asian river dolphins; small cetaceans occurring along developed coastal regions; Kiszka et al., 2022). In such situations, population trend data alone are insufficient to identify, contextualize, and mitigate key causes of decline, and our integrated interdisciplinary approach can provide a useful template for applied conservation research in other systems.
Data availability statement
The original contributions presented in the study are included in the article's Supplementary Material. Further inquiries can be directed to the corresponding author.
Ethics statement
The studies involving human participants were reviewed and approved by Zoological Society of London’s Ethics Committee (BME713). Written informed consent for participation was not required for this study in accordance with national legislation and institutional requirements.
Author contributions
LM and ST designed the study. LM performed the sampling with assistance from ZM, YH, and DW. LM, MH, and ST analyzed data. ST and LM led the writing of the manuscript. All authors contributed to the article and approved the submitted version.
Funding
Funding was provided by a PhD studentship from the Natural Environment Research Council doctoral training partnership, the Mohamed bin Zayed Species Conservation Fund, the New England Aquarium Marine Conservation Action Fund, and Synchronicity Earth.
Acknowledgments
We thank Helen Chatterjee for logistical support, all of the individuals who kindly agreed to be interviewed and gave their time to participate in this study, and the student volunteers who assisted with interviews.
Conflict of interest
The authors declare that the research was conducted in the absence of any commercial or financial relationships that could be construed as a potential conflict of interest.
Publisher’s note
All claims expressed in this article are solely those of the authors and do not necessarily represent those of their affiliated organizations, or those of the publisher, the editors and the reviewers. Any product that may be evaluated in this article, or claim that may be made by its manufacturer, is not guaranteed or endorsed by the publisher.
Supplementary material
The Supplementary Material for this article can be found online at: https://www.frontiersin.org/articles/10.3389/fcosc.2022.929959/full#supplementary-material
References
Aburto-Oropeza O., López-Sagástegui C., Moreno-Báez M., Mascareñas-Osorio I., Jiménez-Esquivel V., Johnson A. F., et al. (2018). Endangered species, ecosystem integrity, and human livelihoods. Conserv. Lett. 11, e12358. doi: 10.1111/conl.12358
Akamatsu T., Wang D., Wang K., Wei Z., Zhao Q., Naito Y. (2002). Diving behaviour of freshwater finless porpoises (Neophocaena phocaenoides) in an oxbow of the Yangtze River, China. ICES J Mar. Sci. 59, 438–443. doi: 10.1006/jmsc.2001.1159
Alava J. J., Tatar B., Barragán M. J., Castro C., Rosero P., Denkinger J., et al. (2019). Mitigating cetacean bycatch in coastal Ecuador: Governance challenges for small-scale fisheries. Mar. Policy 110, 102769.
Arya S., Mount D., Kemp S., Jefferis G. (2015). RANN: Fast nearest neighbour search (Wraps Arya and Mount’s ANN library) (R Package).
Braulik G. T., Kasuga M., Wittich A., Kiszka J. J., MacCaulay J., Gillespie D., et al. (2018). Cetacean rapid assessment: An approach to fill knowledge gaps and target conservation across large data deficient areas. Aquat. Conserv. Mar. Freshw. Ecosyst. 28, 216–230. doi: 10.1002/aqc.2833
Breen P., Brown S., Reid D., Rogan E. (2016). Modelling cetacean distribution and mapping overlap with fisheries in the northeast Atlantic. Ocean Coast. Manage. 134, 140–149. doi: 10.1016/j.ocecoaman.2016.09.004
Brownell R. L. Jr., Reeves R. R., Read A. J., Smith B. D., Thomas P. O., Ralls K., et al. (2019). Bycatch in gillnet fisheries threatens critically endangered small cetaceans and other aquatic megafauna. Endanger. Species Res. 40, 285–296. doi: 10.3354/esr00994
Buckland S. T., Anderson D. R., Burnham K. P., Laake J. L., Borchers D. L., Thomas L. (2001). Introduction to distance sampling: Estimating abundance of biological populations (Oxford: Oxford University Press).
Campana I., Angeletti D., Crosti R., Luperini C., Ruvolo A., Alessandrini A., et al. (2017). Seasonal characterisation of maritime traffic and the relationship with cetacean presence in the Western Mediterranean Sea. Mar. Pollut. Bull. 115, 282–291. doi: 10.1016/j.marpolbul.2016.12.008
Chen D., Xiong F., Wang K., Chang Y. (2009). Status of research on Yangtze fish biology and fisheries. Environ. Biol. Fishes 85, 337–357. doi: 10.1007/s10641-009-9517-0
Dan R. S. (1988). Humpback whale encounter rates offshore of Maui, Hawaii. J. Wildl. Manage. 52, 301–304. doi: 10.2307/3801238
Dawson S., Wade P., Slooten E., Barlow J. (2008). Design and field methods for sighting surveys of cetaceans in coastal and riverine habitats. Mammal Rev. 38, 19–49. doi: 10.1111/j.1365-2907.2008.00119.x
de Leeuw J., Shankman D., Wu G., de Boer W. F., Burnham J., He Q., et al. (2010). Strategic assessment of the magnitude and impacts of sand mining in Poyang Lake, China. Reg. Environ. Change 10, 95–102. doi: 10.1007/s10113-009-0096-6
Dewhurst-Richman N. I., Jones J. P. G., Northridge S., Brook S., Freeman R., Jepson P., et al. (2020). Fishing for the facts: Drivers and levels of river dolphin bycatch in a freshwater artisanal fishery in Bangladesh. Anim. Conserv. 23, 160–170. doi: 10.1111/acv.12523
Dolman S. J., Brakes P. (2018). Sustainable fisheries management and the welfare of bycaught and entangled cetaceans. Front. Vet. Sci. 5, 287. doi: 10.3389/fvets.2018.00287
Dong L., Wang D., Wang K., Li S., Mei Z., Wang S., et al. (2015). Yangtze finless porpoises along the main channel of Poyang Lake, China: Implications for conservation. Mar. Mammal Sci. 31, 612–628. doi: 10.1111/mms.12181
Embling C. B., Walters A. E. M., Dolman S. J. (2015). How much effort is enough? The power of citizen science to monitor trends in coastal cetacean species. Glob. Ecol. Conserv. 3, 867–877. doi: 10.1016/j.gecco.2015.04.003
Fang J., Wang Z., Zhao S., Li Y., Tang Z., Yu D., et al. (2006). Biodiversity changes in the lakes of the central Yangtze. Front. Ecol. Environ. 4, 369–377. doi: 10.1890/1540-9295(2006)004[0369:BCITLO]2.0.CO;2
Feng L., Hu C., Chen X., Cai X., Tian L., Gan W. (2012). Assessment of inundation changes of Poyang Lake using MODIS observations between 2000 and 2010. Remote Sens. Environ. 121, 80–92. doi: 10.1016/j.rse.2012.01.014
Fürstenau Oliveira J. S., Georgiadis G., Campello S., Brandão R. A., Ciuti S. (2017). Improving river dolphin monitoring using aerial surveys. Ecosphere 8, e01912. doi: 10.1002/ecs2.1912
Getis A., Ord J. K. (1992). The analysis of spatial association by use of distance statistics. Geogr. Anal. 24, 189–206. doi: 10.1111/j.1538-4632.1992.tb00261.x
Gray C. A., Kennelly S. J. (2018). Bycatches of endangered, threatened and protected species in marine fisheries. Rev. Fish Biol. Fish. 28, 521–541. doi: 10.1007/s11160-018-9520-7
Harrison X. A., Donaldson L., Correa-Cano M. E., Evans J., Fisher D. N., Goodwin C. E. D., et al. (2018). A brief introduction to mixed effects modelling and multi-model inference in ecology. PeerJ 6, e4794. doi: 10.7717/peerj.4794
Hastie G. D., Wilson B., Wilson L. J., Parsons K. M., Thompson P. M. (2004). Functional mechanisms underlying cetacean distribution patterns: Hotspots for bottlenose dolphins are linked to foraging. Mar. Biol. 144, 397–403. doi: 10.1007/s00227-003-1195-4
Herrón P., Kluger L. C., Castellanos-Galindo G. A., Wolff M., Glaser M. (2020). Understanding gear choices and identifying leverage points for sustainable tropical small-scale marine fisheries. Ocean Coast. Manage. 188, 105074. doi: 10.1016/j.ocecoaman.2019.105074
Huang J., Mei Z., Chen M., Han Y., Zhang X., Moore J. E., et al. (2020). Population survey showing hope for population recovery of the critically endangered Yangtze finless porpoise. Biol. Conserv. 241, 108315. doi: 10.1016/j.biocon.2019.108315
Huang S. L., Mei Z., Hao Y., Zheng J., Wang K., Wang D. (2017). Saving the Yangtze finless porpoise: Time is rapidly running out. Biol. Conserv. 210, 40–46. doi: 10.1016/j.biocon.2016.05.021
IUCN (2021) The IUCN Red List of threatened species. Available at: http://www.iucnredlist.org.
Johnson A. J., Kraus S. D., Kenney J. F., Mayo C. A. (2007). “The entangled lives of right whales and fishermen: Can they coexist?,” in The urban whale: North Atlantic right whales at the crossroads. Eds. Kraus S. D., Rolland R. M. (Cambridge, MA: Harvard University Press), 380–408.
Karpouzi V. S., Watson R., Pauly D. (2007). Modelling and mapping resource overlap between seabirds and fisheries on a global scale: A preliminary assessment. Mar. Ecol. Prog. Ser. 343, 87–99. doi: 10.3354/meps06860
Kastelein R. A., Huybrechts J., Covi J., Helder-Hoek L. (2017). Behavioral responses of a harbor porpoise (Phocoena phocoena) to sounds from an acoustic porpoise deterrent. Aquat. Mamm. 43, 233–244. doi: 10.1578/AM.43.3.2017.233
Kastelein R. A., van den Belt I., Helder-Hoek L., Gransier R., Johansson T. (2015). Behavioral responses of a harbor porpoise (Phocoena phocoena) to 25-kHz FM sonar signals. Aquat. Mamm. 41, 311–326. doi: 10.1578/AM.41.3.2015.311
Kelkar N., Dey S. (2020). Mesh mash: Legal fishing nets cause most bycatch mortality of endangered South Asian river dolphins. Biol. Conserv. 252, 108844. doi: 10.1016/j.biocon.2020.108844
Kelkar N., Krishnaswamy J., Choudhary S., Sutaria D. (2010). Coexistence of fisheries with river dolphin conservation. Conserv. Biol. 24, 1130–1140. doi: 10.1111/j.1523-1739.2010.01467.x
Kimura S., Akamatsu T., Li S., Dong L., Wang K., Wang D., et al. (2012). Seasonal changes in the local distribution of Yangtze finless porpoises related to fish presence. Mar. Mammal Sci. 28, 308–324. doi: 10.1111/j.1748-7692.2011.00490.x
Kiszka J. J., Bejder L., Davis R., Harcourt R., Meekan M., Rodriguez D. H., et al. (2022). Small cetacean conservation: Current challenges and opportunities. Front. Mar. Sci. 9, 957002. doi: 10.3389/fmars.2022.957002
Koehnken L., Rintoul M. S., Goichot M., Tickner D., Loftus A. C., Acreman M. C. (2020). Impacts of riverine sand mining on freshwater ecosystems: A review of the scientific evidence and guidance for future research. River Res. Appl. 36, 362–370. doi: 10.1002/rra.3586
Königson S. J., Fredriksson R. E., Lunneryd S. G., Strömberg P., Bergström U. M. (2015). Cod pots in a Baltic fishery: Are they efficient and what affects their efficiency? ICES J. Mar. Sci. 72, 1545–1554.
Larson C. (2018). Asia’s hunger for sand takes toll on ecology. Science 359, 964–965. doi: 10.1126/science.359.6379.964
Li S., Akamatsu T., Wang D., Wang K., Dong S., Zhao X., et al. (2008). Indirect evidence of boat avoidance behavior of Yangtze finless porpoises. Bioacoustics 17, 174–176. doi: 10.1080/09524622.2008.9753806
Li Q., Lai G., Liu Y., Devlin A. T., Zhan S., Wang S. (2021). Assessing the impact of the proposed Poyang Lake hydraulic project on the Yangtze finless porpoise and its calves. Ecol. Indic. 129, 107873. doi: 10.1016/j.ecolind.2021.107873
Limuna M. M., Sitaula B. K., Njaya F., Storebakken T. (2018). Evaluation of small-scale fishers’ perceptions on climate change and their coping strategies: Insights from Lake Malawi. Climate 6, 34.
Liu J., Hull V., Yang W., Viña A., Chen X., Ouyang Z., et al. (2016). Pandas and people: Coupling human and natural systems for sustainability (Oxford: Oxford University Press).
Liu X., Mei Z., Zhang J., Sun J., Zhang N., Guo Y., et al. (2021). Seasonal Yangtze finless porpoise (Neophocaena asiaeorientalis asiaeorientalis) movements in the Poyang Lake, China: Implications on flexible management for aquatic animals in fluctuating freshwater ecosystems. Sci. Total Environ. 807, 150782. doi: 10.1016/j.scitotenv.2021.150782
Marcoux M., Young B. G., Asselin N. C., Watt C. A., Dunn J. B., Ferguson S. H. (2016). Estimate of Cumberland Sound beluga (Delphinapterus leucas) population size from the 2014 visual and photographic aerial survey. DFO Can. Sci. Advis. Sec. Res. 037, 1–19.
Mei Z., Cheng P., Wang K., Wei Q., Barlow J., Wang D. (2020). A first step for the Yangtze. Science 367, 1314. doi: 10.1126/science.abb5537
Mei Z., Chen M., Li Y., Huang S. L., Haung J., Han Y., et al. (2017). Habitat preference of the Yangtze finless porpoise in a minimally disturbed environment. Ecol. Modell. 353, 47–53. doi: 10.1016/j.ecolmodel.2016.12.020
Mei Z., Han Y., Dong L., Turvey S. T., Hao Y., Wang K., et al. (2019). The impact of fisheries management practices on the survival of the Yangtze finless porpoise in China. Aquat. Conserv. Mar. Freshw. Ecosyst. 29, 639–646. doi: 10.1002/aqc.3078
Mei Z., Han Y., Turvey S. T., Liu J., Wang Z., Nabi G., et al. (2021). Mitigating the effect of shipping on freshwater cetaceans: The case study of the Yangtze finless porpoise. Biol. Conserv. 257, 109132. doi: 10.1016/j.biocon.2021.109132
Mei Z., Zhang X., Huang S. L., Zhao X., Hao Y., Zhang L., et al. (2014). The Yangtze finless porpoise: On an accelerating path to extinction? Biol. Conserv. 172, 117–123. doi: 10.1016/j.biocon.2014.02.033
Mogensen L. M. W., Mei Z., Hao Y., Harrison X. A., Wang D., Turvey S. T. (2022). Precautionary principle or evidence-based conservation? Assessing the information content of threat data for the Yangtze finless porpoise. Front. Mar. Sci. 8, 791484. doi: 10.3389/fmars.2021.791484
Moore J. E., Barlow J. (2017). Population abundance and trend estimates for beaked whales and sperm whales in the California Current from ship-based visual line-transect survey data 1991-2014. NOAA Tech. Memorandum NOAA-TM-NMFS-SWFSC-585.
Morteo E., Rocha-Olivares A., Arceo-Briseño P., Abarca-Arenas L. G. (2012). Spatial analyses of bottlenose dolphin–fisheries interactions reveal human avoidance off a productive lagoon in the Western Gulf of Mexico. J. Mar. Biolog. Assoc. 92, 1893–1900. doi: 10.1017/S0025315411000488
Newing H. (2011). Conducting research in conservation: A social science perspective (Abingdon, UK: Routledge).
Nuno A., St John F. A. V. (2014). How to ask sensitive questions in conservation: A review of specialized questioning techniques. Biol. Conserv. 189, 5–15. doi: 10.1016/j.biocon.2014.09.047
O’Hern J. E., Sculley M., Jean-Smith K., Biggs D., Slowey N., Ruales D. A., et al. (2014). Marine mammal distribution in Ecuador: Surveys aboard a ship of opportunity as a means of monitoring relative abundance. LAJAM 12, 24–28.
Parsons E. C. M., Favaro B., Aguirre A. A., Bauer A. L., Blight L. K., Cigliano J. A., et al. (2014). Seventy-one important questions for the conservation of marine biodiversity. Conserv. Biol. 28, 1206–1214. doi: 10.1111/cobi.12303
Paudel S., Koprowksi J. L., Cove M. V. (2020). Seasonal flow dynamics exacerbate overlap between artisanal fisheries and imperiled Ganges River dolphins. Sci. Rep. 10, 18798. doi: 10.1038/s41598-020-75997-4
Petetta A., Virgili M., Guicciardi S., Lucchetti A. (2021). Pots as alternative and sustainable fishing gears in the Mediterranean Sea: An overview. Rev. Fish Biol. Fish. 31, 773–795. doi: 10.1007/s11160-021-09676-6
R Development Core Team (2017). R: A language and environment for statistical computing (Vienna: R Foundation for Statistical Computing).
Reeves R. R., Smith B. D., Kasuya T. (2000). Biology and conservation of freshwater cetaceans in Asia. Occas. Pap. IUCN Species Surviv. Commun. 23, 1–152.
Revuelta O., Domènech F., Fraija-Fernández N., Gozalbes P., Novillo O., Penadés-Suay J., et al. (2018). Interaction between bottlenose dolphins (Tursiops truncatus) and artisanal fisheries in the Valencia region (Spanish Mediterranean Sea). Ocean Coast. Manage. 165, 117–125. doi: 10.1016/j.ocecoaman.2018.08.001
Rone B. K., Zerbini A. N., Douglas A. B., Weller D. W., Clapham P. J. (2017). Abundance and distribution of cetaceans in the Gulf of Alaska. Mar. Biol. 164, 23. doi: 10.1007/s00227-016-3052-2
Samah A. A., Shaffril H. A. M., Hamzah A., Samah B. A. (2019). Factors affecting small-scale fishermen’s adaptation toward the impacts of climate change: Reflections from Malaysian fishers. SAGE Open 9, 3.
Shester G. G., Micheli F. (2011). Conservation challenges for small-scale fisheries: Bycatch and habitat impacts of traps and gillnets. Biol. Conserv. 144, 1673–1681. doi: 10.1016/j.biocon.2011.02.023
Slooten E., Dawson S. M. (2021). Delays in protecting a small endangered cetacean: Lessons learned for science and management. Front. Mar. Sci. 8, 606547. doi: 10.3389/fmars.2021.606547
Taylor B. L., Rojas-Bracho L., Moore J., Jaramillo-Legoretta A., Ver Hoef J. M., Cardenas-Hinojosa G., et al. (2017). Extinction is imminent for Mexico’s endemic porpoise unless fishery bycatch is eliminated. Conserv. Lett. 10, 588–595. doi: 10.1111/conl.12331
Thomas P. O., Gulland F. M. D., Reeves R. R., Kreb D., Wang D., Smith B., et al. (2019). Electrofishing as a potential threat to freshwater cetaceans. Endanger. Species Res. 39, 207–220. doi: 10.3354/esr00962
Thomas L., Williams R., Sandilands D. (2007). Designing line transect surveys for complex survey regions. J. Cetacean Res. Manag. 9, 1–13.
Tulloch V., Pirotta V., Grech A., Crocetti S., Double M., How J., et al. (2020). Long-term trends and a risk analysis of cetacean entanglements and bycatch in fisheries gear in Australian waters. Biodivers. Conserv. 29, 251–282. doi: 10.1007/s10531-019-01881-x
Tulloch V. J. D., Tulloch A. I. T., Visconti P., Halpern B. S., Watson J. E. M., Evans M. C., et al. (2015). Why do we map threats? Linking threat mapping with actions to make better conservation decisions. Front. Ecol. Environ. 13, 91–99. doi: 10.1890/140022
Turvey S. T., Barrett L. A., Hart T., Collen B., Hao Y., Zhang L., et al. (2010). Spatial and temporal extinction dynamics in a freshwater cetacean. Proc. R. Soc. B 277, 3139–3147.
Turvey S. T., Hao Y., Wang D. (2015). Identifying conservation solutions for the Yangtze finless porpoise (Neophocaena asiaeorientalis asiaeorientalis) through community research (Cambridge: International Whaling Commission). Available at: http://www.iucn-csg.org/wp-content/uploads/2010/03/Turvey_IWC_final_report.pdf.
Turvey S. T., Pitman R. L., Taylor B. L., Barlow J., Akamatsu T., Barrett L. A., et al. (2007). First human-caused extinction of a cetacean species? Biol. Lett. 3, 537–540.
Turvey S. T., Risley C. L., Barrett L. A., Hao Y., Wang D. (2012). River dolphins can act as population trend indicators in degraded freshwater systems. PloS One 7, e37902. doi: 10.1371/journal.pone.0037902
Turvey S. T., Risley C. L., Moore J. E., Barrett L. A., Hao Y., Zhao X., et al. (2013). Can local ecological knowledge be used to assess status and extinction drivers in a threatened freshwater cetacean? Biol. Conserv. 157, 352–360. doi: 10.1016/j.biocon.2012.07.016
Wallner-Hahn S., Molander F., Gallardo G., Villasante S., Eklöf J. S., Jiddawi N. S., et al. (2016). Destructive gear use in a tropical fishery: Institutional factors influencing the willingness–and capacity to change. Mar. Policy 72, 199–210. doi: 10.1016/j.marpol.2016.07.001
Wang D. (2009). Population status, threats and conservation of the Yangtze finless porpoise. Bull. Chin. Acad. Sci. 27, 3473–3484. doi: 10.1007/s11434-009-0522-7
Wang Z., Akamatsu T., Mei Z., Dong L., Imaizumi T., Wang K., et al. (2015b). Frequent and prolonged nocturnal occupation of port areas by Yangtze finless porpoises (Neophocaena asiaeorientalis): Forced choice for feeding? Integr. Zool. 10, 122–132. doi: 10.1111/1749-4877.12102
Wang Z., Akamatsu T., Wang K., Wang D. (2014). The diel rhythms of biosonar behavior in the Yangtze finless porpoise (Neophocaena asiaeorientalis asiaeorientalis) in the port of the Yangtze river: The correlation between prey availability and boat traffic. PloS One 9, e97907. doi: 10.1371/journal.pone.0097907
Wang D., Liu R., Zhang X., Yang J., Wei Z., Zhao Q., et al. (2000). “Status and conservation of the Yangtze finless porpoise,” in Biology and conservation of freshwater cetaceans in Asia. Eds. Reeves R. R., Smith B. D., Kasuya T. (Gland, Switzerland: IUCN), 81–85.
Wang Y., Li W., van Waerebeek K. (2015a). Strandings, bycatches and injuries of aquatic mammals in China 2000-2006, as reviewed from official documents: A compelling argument for a nationwide strandings programme. Mar. Policy 51, 242–250. doi: 10.1016/j.marpol.2014.07.016
Wisniewska D. M., Johnson M., Teilmann J., Rojano-Doñate L., Shearer J., Sveegaard S., et al. (2016). Ultra-high foraging rates of harbor porpoises make them vulnerable to anthropogenic disturbance. Curr. Biol. 26, 1441–1446. doi: 10.1016/j.cub.2016.03.069
Wisniewska D. M., Johnson M., Teilmann J., Siebert U., Galatius A., Dietz R., et al. (2018). High rates of vessel noise disrupt foraging in wild harbour porpoises (Phocoena phocoena). Proc. R. Soc B 285, 20172314. doi: 10.1098/rspb.2017.2314
Xu Z. (2015). The study of the Yangtze finless porpoise population status and behavior characteristics and protection in the key waters of Poyang Lake (Nanchang: PhD dissertation, Nanchang University).
Yao J., Zhang D., Li Y., Zhang Q., Gao J. (2019). Quantifying the hydrodynamic impacts of cumulative sand mining on a large river-connected floodplain lake: Poyang lake. J. Hydrol. 579, 124156. doi: 10.1016/j.jhydrol.2019.124156
Zhang H., Kang M., Shen L., Wu J., Li J., Du H., et al. (2020). Rapid change in Yangtze fisheries and its implications for global freshwater ecosystem management. Fish Fish. 21, 601–620. doi: 10.1111/faf.12449
Zhang X., Liu R., Zhao Q., Zhang G., Wei Z., Wang X., et al. (1993). The population of finless porpoise in the middle and lower reaches of Yangtze River. Acta Theriol. Sin. 13, 260–270.
Zhang X., Wang D., Liu R., Wei Z., Hua Y., Wang Y., et al. (2003). The Yangtze River dolphin or baiji (Lipotes vexillifer): Population status and conservation issues in the Yangtze River, China. Aquat. Conserv. Mar. Freshw. Ecosyst. 13, 51–64. doi: 10.1002/aqc.547
Zhao X. (2009). Investigation of survey methodology on the abundance of cetaceans in riverine systems and its applications (Ph.D. Dissertation, Institute of Hydrobiology: Wuhan).
Zhao X., Barlow J., Taylor B. L., Pitman R. L., Wang K., Wei Z., et al. (2008). Abundance and conservation status of the Yangtze finless porpoise in the Yangtze River, China. Biol. Conserv. 141, 3006–3018. doi: 10.1016/j.biocon.2008.09.005
Zhao X., Wang D., Turvey S. T., Taylor B., Akamatsu T. (2013). Distribution patterns of Yangtze finless porpoises in the Yangtze River: Implications for reserve management. Anim. Conserv. 16, 509–518. doi: 10.1111/acv.12019
Zhou K., Sun J., Gao A., Würsig B. (1998). Baiji (Lipotes vexillifer) in the lower Yangtze River: Movements, numbers threats and conservation needs. Aquat. Mamm. 24.2, 123–132.
Zhou K., Wang X. (1994). Brief review of passive fishing gear and incidental catches of small cetaceans in Chinese waters. Rep. IWC 15, 347–354.
Keywords: bycatch, freshwater cetacean, interview survey, Neophocaena asiaeorientalis, sand-mining, social-ecological system, Yangtze finless porpoise
Citation: Mogensen LMW, Mei Z, Hao Y, Hudson MA, Wang D and Turvey ST (2022) Spatiotemporal relationships of threatened cetaceans and anthropogenic threats in the lower Yangtze system. Front. Conserv. Sci. 3:929959. doi: 10.3389/fcosc.2022.929959
Received: 29 June 2022; Accepted: 12 October 2022;
Published: 02 November 2022.
Edited by:
Jean Hugé, Open University of the Netherlands, NetherlandsReviewed by:
Helder Araújo, University of Aveiro, PortugalKim Magnus Bærum, Norwegian Institute for Nature Research (NINA), Norway
Copyright © 2022 Mogensen, Mei, Hao, Hudson, Wang and Turvey. This is an open-access article distributed under the terms of the Creative Commons Attribution License (CC BY). The use, distribution or reproduction in other forums is permitted, provided the original author(s) and the copyright owner(s) are credited and that the original publication in this journal is cited, in accordance with accepted academic practice. No use, distribution or reproduction is permitted which does not comply with these terms.
*Correspondence: Samuel T. Turvey, samuel.turvey@ioz.ac.uk