- 1Laboratoire de Biologie et Ecologie animales (LBEA), Unité de Formation et de Recherche/Science de la Vie et de la Terre (UFR/SVT), Université Joseph KI-ZERBO, Ouagadougou, Burkina Faso
- 2Faculty of Sciences and Technics, Department of Natural Sciences, University of Kara, Kara, Togo
- 3Faculty of Sciences Laboratory of Applied Hydrology and Environment Boîte Postale (BP), University of Lomé, Lomé, Togo
- 4Centre Universitaire Polytechnique de Tenkodogo, Tenkodogo, Burkina Faso
- 5Université de Dédougou, Dédougou, Burkina Faso
Birds, amphibians, fish, and macroinvertebrates are useful indicators of ecological conditions and ensure considerable conservation value and tools for decision making in management of wetland ecosystems. However, the use of these organisms to monitor wetland ecosystems has rarely been explored in Western Africa. Whereas, we are currently facing to growing multiple anthropogenic pressures and climate warming that impact negatively our wetlands and the biodiversity. Notably, there is an urgent need of cost-effective tools for wetland ecosystems health assessment in Burkina Faso. In this study, we examined the taxonomic composition of birds, amphibians, fish, and macroinvertebrates and explored their potential use for monitoring wetland ecosystems. From our findings, measures of taxa composition and diversity respond to the gradients of anthropogenic alterations. Our results revealed that the highest diversity of fish and macroinvertebrates taxa was recorded in protected sites, whereas the lowest diversity was obtained in degraded sites. Additionally, the findings showed a strong and positive correlation between macroinvertebrates taxa and key water variables, whereas fish taxa were strongly correlated to xylal (deadwood) substrates. Most of bird’s species were recorded in tree-shrubs, and amphibians of protected wetlands were distinguished by identifying indicator taxa through indicator value index. African wetland ecosystems and biodiversity may be sustainably preserved through responsive monitoring programs of wetlands by limnologists.
Introduction
Research that explores biological responses to anthropogenic disturbances in specific ecosystems is necessary for long-term management and restoration purposes. Wetlands are an important complex network ecosystem, which provide valuables services to local communities. Wetlands have, for a long time, been considered as a driver of intense agricultural and rural development, leading to conceal wetland policy and management compared with those of river basins (Gopal, 2013). Therefore, wetland ecosystem preservation and restoration remain major challenges for all governments, as evidenced by numerous public and private projects, which are focused on the urgent need for tools to assess the gravity of human pressures and guide action (Clarkson et al., 2004; Wetlands International, 2017; Barratt Heitmann, 2018). It is demonstrated that wetland ecosystems provide some goods and services such as carbon sequestration by trees, reduction of pollution effects through filtration, water storage, protection from storms, flood control and prevention, drought buffering, erosion control, groundwater recharge and discharge, shoreline stabilization, and stabilization of local climate conditions (Rafiq et al., 2013; Thapa et al., 2020; Spieles, 2022). The use of goods and services, which wetlands provide for the human population, should not compromise the ecological integrity of the wetlands.
However, wetland ecosystems are threatened by multiple factors that contribute to the decline of their valuable functions affecting their capacity to support and maintain some natural biological systems. In fact, the pressure to feed and provide livelihoods for a rapidly growing population is leading to the dramatic wetland ecosystems habitat and biodiversity loss. In addition, poor policy enforcement, linked to increasing human pressures, negatively affects ecosystem services (Saunders et al., 2013; Camacho-Valdez et al., 2014; Parienté, 2017). Habitats degradation, intense agriculture practices, and untreated wastes from municipalities have altered organisms (fauna and flora), including overall wetland environment health Egler et al., 2012; Kaboré et al., 2016; Newton et al., 2020). For example, changes in hydrology, water pollution, invasion by weeds, and pests can lead to severely impaired wetland habitats (Junk, 2002; Clarkson et al., 2004). Therefore, with the ongoing human pressures and increasing climatic variability, the urgent need for tools (e.g., use of local organisms) for ecological health assessment is a priority in conservation efforts and efficient management of wetlands in developing countries (Kaboré et al., 2018). This is true for many tropical wetlands such as Asia, Africa, Latin America, and the Caribbean where continually changes in quantity and quality of wetland areas are receiving considerable attention (Sarkar et al., 2020; Adeeyo et al., 2022).
In Africa, most of the endangered species (e.g., amphibians, birds, mammals, invertebrates, and fish) and highly diverse natural habitats are confined in protected wetlands, and most of them are under anthropogenic disturbances (Clarkson et al., 2004; Camacho-Valdez et al., 2014; Arowosafe et al., 2019; Stephenson et al., 2020). For example, in South Africa, Adeeyo et al. (2022); Sinthumule and Netshisaulu (2022) address the ranges of threats affecting wetland ecosystems functioning and provide guidelines for long term management. According to Adeeyo et al. (2022), the current state of several wetlands across African countries calls for urgent attention. The unclear management status linked to policy and legislation weaknesses and the scarcity of biological data and tools to monitor wetlands are central problems that need to be solved urgently by scientists and policy makers to avoid irreversible extinction of existing wetlands (Gumbricht et al., 2017; Kaboré et al., 2018; Stephenson et al., 2020; Adeeyo et al., 2022).
In Burkina Faso, impaired wetlands in urban areas have, for a long time, been the overall recipient of industrial wastes, untreated wastewater, and wastewater disposal (Lamizana/Diallo et al., 2008; Haro et al., 2013; Alikhani et al., 2021). There is evidence of intense agricultural practices including additional nutrient input, deforestation, and mining activities, as well as water deviation rendering these wetland ecosystems more vulnerable, and posing potential threats to both human health and the integrity of wetlands (Junk, 2002; Ayanlade and Proske, 2016). In contrast, protected wetlands are exposed to the lowest levels of human impacts, where natural intactness of wetland vegetation provides more opportunities for food, shelter for variety of terrestrial wild and aquatic fauna, as well as river bank stabilization (Kaboré et al., 2018). To reinforce the conservation and protection measures of wetland ecosystems, there is a need for basic tools for early warning systems of habitat degradation. However, quantitative and distributional analyses of biological community needed to estimate sensitivity to land use change and promoting potential use in bioassessment programs are missing. Wetland fauna that are well adapted to their environment are potential indicators of environmental perturbations. The knowledge of essential birds, amphibians, fish, and macroinvertebrate is an important management tool for ecosystem health monitoring. Birds, amphibians, fish, and macroinvertebrate must become a well-established indicator to support better wetland management for Burkina Faso.
This study aimed to investigate the use of birds, amphibians, fish, and macroinvertebrate as tools to monitor the effects of different wetland use types. The key objectives of this work were to (1) evaluate the diversity of birds, amphibians, fish, and macroinvertebrates in two categories of wetland and (2) discuss their potential use as tools in bio-assessment.
Material and methods
Study area
Burkina Faso is located in the heart of West Africa, in the sub-Saharan region (12° 16′N, 2° 4′W, Figure 1). The climate is characterized by highly irregular rainfall patterns with marked differences between wet and dry seasons, which lead to chronic water scarcity and episodes of severe drought.
Burkina Faso is drained by four main catchments, namely, Nakanbé, Mouhoun, Comoé, and Niger, and constitutes its hydrological network (Kaboré et al., 2022). The most destructive changes to wetland ecosystems are a result of intensive agriculture and deforestation coupled with urban center expansion and industrial activities (Kaboré et al., 2016; Ola and Benjamin, 2019).
The data of fish, macroinvertebrates, birds, and amphibians were gathered from protected and impaired wetlands. We used the terminology “area” and showed them on the map to avoid confusion about sampling site, representing geographically confined area and can consist of several investigation sites.
Sampled protected wetland areas include Nazinga, Comoé-Léraba, and Deux Balé. These sites are characterized by natural vegetation cover and preserved natural habitats. The vegetation of Comoé-Léraba (9.836N, −4.623W) and Deux Balé (11.677N, −2.836W) is characterized by trees, shrubs, and herbaceous savannahs typical to area > 80%, which constitute shelters for diverse fauna (e.g., mammals, reptiles, and birds). Nazinga park lies in southern Burkina Faso (11.330N, −1.716 W) (Héma et al., 2010). The area is relatively flat and is drained by the valley consisting of Sissili River and its two tributaries, which have intermittent flows. The mean annual rainfall is about 900 mm. The vegetation is characterized by grasses, trees, and shrubs, showing mainly riverine forest, savannah woodlands, and shrub savannah.
The impaired sampling areas include Ouagadougou and Koubri. Ouagadougou (12.130N, −1.4575W) is the capital city of Burkina Faso. In Ouagadougou, the sampling site named “Bangr-Weoogo” is surrounded by residential and industrial areas and then is exposed to industrial and domestic waste. The hydrographic network consists of Massili River that receives waters from small streams draining the city of Ouagadougou.
In Koubri (12.422N, −1.552W), five sites were selected, namely, “Napagbtenga, Arzoumbaogo, Poedgo, Noungou, and Nabazana.” Koubri is located 40 km southeast of Ouagadougou. The area is characterized by high reservoir and dykes, intensive small-scale agriculture using fertilizer and pesticides, livestock husbandry, as well as, water abstraction, and livestock watering at all sampling sites. The area is drained by one main river called “Nariale”, a tributary of Nakanbé River.
Data collection
In situ parameters such as, pH, electrical conductivity (Cond), temperature, and dissolved oxygen (Oxy) were measured with field multimeters (WTW 340i) before fish and macroinvertebrate sampling.
Fish communities were sampled in Koubri (05 sites) and Nazinga (06 sites) using two types of equipment including electric fishing and cast net, and the habitats type were noted following sampling approach described by (Meulenbroek et al., 2019). For electric fishing, the backpack-generator ELT60-IIH from Hans Grassl was applied. Two professional local fishermen were recruited to conduct the “traditional” cast net fishing method. Two different kinds of nets with 10/25 mm mesh size and a diameter of 4.3/4.5 m were used. Fish were identified to species level by using morphological characters [Paugy et al., 2003] and then were counted.
The macroinvertebrates were sampled in impaired sites including Bangr-Weoogo (02 sites) and Koubri (03 sites) and in protected area including Nazinga (05 sites) using hand-net (25 × 25 cm, a mesh size of 500 μm) following the multi-habitat sampling approach from Moog (2007). A pooled sample, consisting of 20 sampling units, taken from all habitats in each sampling site was used. All taxa were sorted and identified following procedure described by Kaboré et al. (2016).
Bird surveys were conducted in Nazinga and Bangr-Weoogo located in protected and impaired areas, respectively, based on local managers and experts’ designs (Thiollay, 2006). The roads and small trails were divided into separate transects. All birds seen were counted within 100 m and the raptors within 1 km. On either side of the trails, visual assessment and auditory cues were considered to record each species. In order to capture peak activity, allowing for an accurate count of species, the bird’s samples were taken between 9 a.m. and 2 p.m. of day. All birds were identified with binoculars (Opticron 8 × 30, Super Zenith 8 × 40) by experts and using field standard guide (Balança et al., 2007).
In order to provide sufficient data of amphibians in wetland, we used the data from literature researches conducted by (Ayoro et al., 2020) whom produced unprecedented database of amphibians in Burkina Faso. However, only the field data from protected “Comoé-Léraba, Deux Balé” and impaired wetlands “Ouagadougou and Koubri” were taken into account in this study. Field amphibian’s data were gathered using visual searches in various habitat (e.g., ponds, forest, rocks, and logs), as well as acoustic signals and dip netting for tadpoles were employed (see also Rödel and Ernst, 2003; Ayoro et al., 2020).
Data analysis
Fish, macroinvertebrates, birds, and amphibians’ community structures were described through relative abundance and diversity parameters. Species diversity parameters such as taxa richness, species heterogeneity using Shannon–Weiner diversity index (H), and Margalef diversity were calculated, and True estimator “Chao 1 diversity” (Chao, 1987; Colwell and Coddington, 1994) was used to calculate the estimated true species diversity in the two categories of wetland, respectively. The total taxa richness was simply taken as a count of number of taxa or species present in each station, and then Indicator value (IndVal) was computed for the amphibians’ data using Dufrêne & Legendre (1997) equation in PCord (version 6) to determine the potential bioindicators of wetland sites.
We used Boxplots and Mann–Whitney U test (p< 0.05) to compare fish and macroinvertebrates diversity between protected and impaired wetlands.
Canonical correspondence analysis (CCA) was performed to define composite environmental variables that correspond to the major patterns of macroinvertebrates and fish species community occurrence. Before deciding on using CCA, we conducted a test for unimodality and linearity based on Detrended Correspondence Analysis (DCA), and the gradient lengths for both macroinvertebrates and fish species returned > 4 for the DCA first axis (Lepš and Šmilauer, 2003). Species with an abundance of less than 10 specimens were not included in the analysis. The statistical significance of each variable selected was judged by a Monte Carlo permutation test (p< 0.05). The Canoco (Canonical Ordination of the Communities) for Windows package, version 4.5 (Ter Braak and Smilauer, 2002) was used for the analysis.
All, these indices were calculated following the equations (1–4).
where pi is the proportion of individuals in the “ith” taxon of the community and s is the total number of taxa in the community.
where S is total number of species in area sampled and N total number of individuals observed
Sobs= the observed number of species, n1 is the number of species with only a single occurrence in the sample and n2 is the number of species with exactly two occurrences in the sample.
where Aij = N individualsij/N individualsi is a measure of specificity based on abundance values, whereas Bij = N sitesij/N sitesi is a measure of fidelity computed from the presence data. IndVals in percentage range from 0 (no indication) to 100 (perfect indication).
Results
Fish communities as indicator of human pressures
Table 1 shows a significant decrease of fish sizes across the sampling stations due to the sensitivity of fish to environmental degradation. From Table 1, the highest mean fish sizes were caught in protected wetland (total sizes = 133.33 ± 1.59, p< 0.05), whereas the lowest fish sizes (total sizes = 71.23 ± 1.98) were caught in impaired wetland. Indeed, across different wetland categories, we found a general deviation from the expected balance in terms of species sizes in wetland communities, as revealed using fish sizes group-based surrogate measures of ecosystem attributes. All the stations were dominated by Mormyridae (> 20%) followed by Cichlidae (16%), Cyprinidae, and Alestidae (15.02%), whereas the lowest abundances (< 1%) were observed in the families of Anabantidae, Citharinidae, Distichodontidae, and Polypteridae. Figures 2A–D illustrates fish community structure clustered at two categories of sampling sites. Metrics based on diversity indices showed higher sensitivity to different levels of environmental degradation and a clear decrease across the gradient of human impact intensity. All richness measures and diversity were efficient to clearly discriminate the two categories of land use: species richness, Shannon–Wiener diversity, Margalef diversity, and Chao 1 diversity per site was highest in protected wetlands 30.00 ± 1.53, 2.50 ± 0.24, 3.94 ± 0.32, and 31.94±1.56, respectively, and then dropped to a minimum of 12.33 ± 2.02, 1.54 ± 0.50, 1.82 ± 0.42, and 12.44 ± 2.13, respectively, per site in impaired wetlands (Figure 2). Ten taxa were recorded in protected wetlands: (Mormyridae, Polypterus, Brycinus, Hydrocynus, Heterobranchus, Synodontis, Labeo, Citharinus, Lates niloticus, and Heterotis niloticus). Brabus, Brycinus, Clarias, Oreochromis, Schilbe, Coptodon, and Hemichromis were mostly recorded in impaired wetlands.
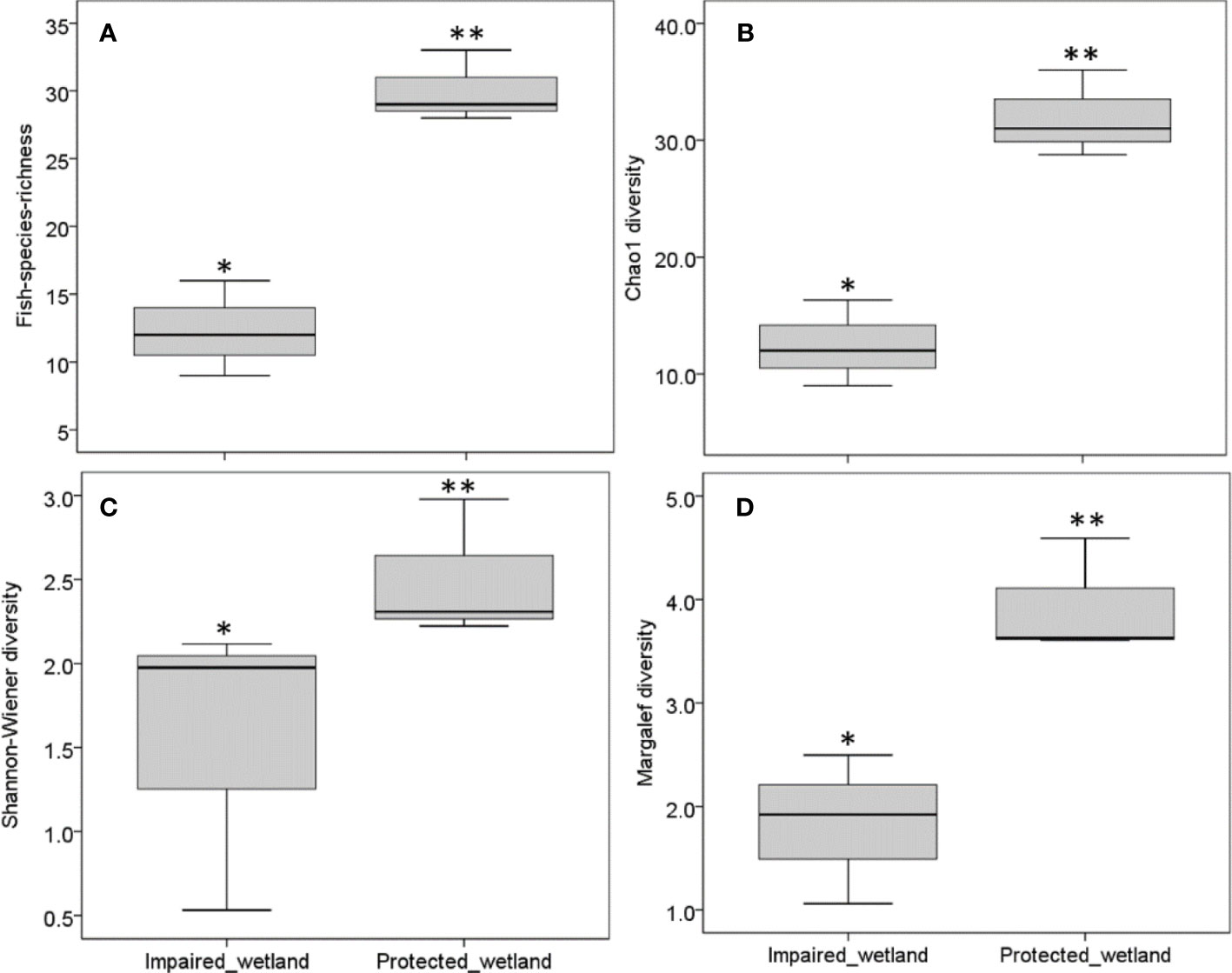
Figure 2 Box plot comparing fish diversity between impaired wetland and protected wetland. Median value is shown in each box; vertical bars correspond to the minimum and maximum values. (A) Fish-species-richness, (B) Chao 1 diversity, (C) Shannon–Weiner diversity, (D) Margalef diversity. *indicates statistical significance (Mann–Whitney U test, p< 0.05).
Figure 3 shows the biplots of species and physical–chemical parameters with eigenvalues for axes 1–2, 0.278, 0.169, respectively, and only the two axes were used for interpretation. The first two axes of CCA captured about 63.9% of information in species-environment correlations. The chemical variables, such as conductivity (r = 0.66) and pH (r = 0.57), are positively correlated with axis 1. The variations in the values of these variables are often an indication of water pollution due to human activities. Whereas, the physical habitats, such as Xylal (r = 0.44), sediment substrates (r = 0.93), are positively related to axis 2.
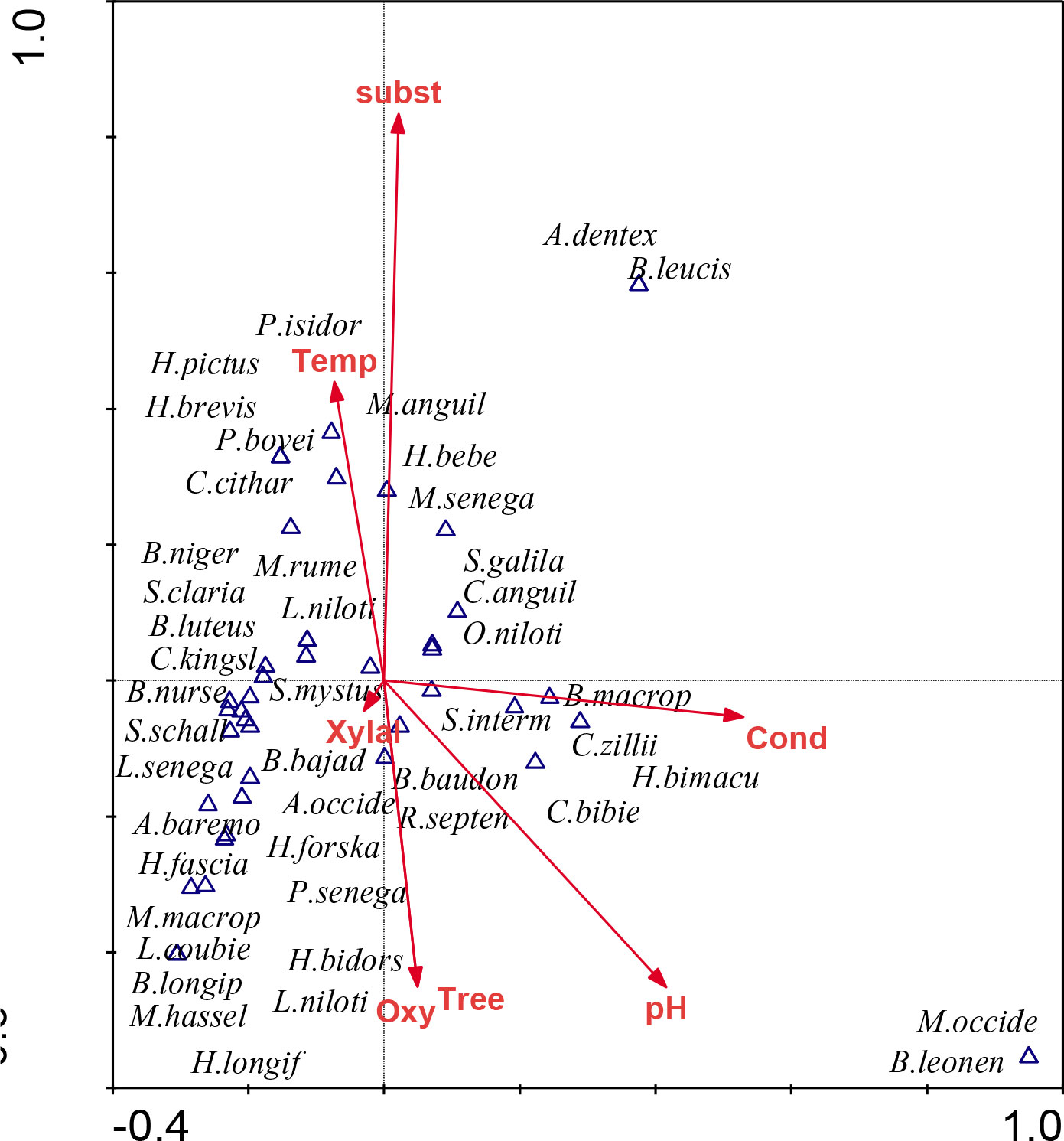
Figure 3 Relationship between habitats, physico-chemical variables, and fish based on canonical correspondence analysis (CCA). A. baremoze, Alestes baremoze; A. dente, Alestes dentex; A. occidentalis, Auchenoglanis occidentalis; B. bajad, Bagrus bajad ; B. baudoni, Barbus baudoni; B. leonensis, Barbus leonensis; B. macrops, Barbus macrops; B. longipinnis, Brycinus longipinnis; B. luteus, Brycinus luteus; B. nurse, Brycinus nurse; C. bibie, Chelaethiops bibie; C. anguillaris, Clarias anguillaris; H. bimaculatus, Hemichromis bimaculatus; H. fasciatus, Hemichromis fasciatus; H. bidorsalis, Hemichromis bidorsalis; H. pictus, Hippopotamyrus pictus; H. forskahlii, Hemicromis forskahlii; H. bebe, Hyperopisus bebe; L. coubie, Labeo coubie; L. senegalensis, Labeo senegalensis; L. niloticus, Lates niloticus; M. senegalensis, Marcusenius senegalensis; M. rume, Mormyrus rume; O. niloticus, Oreochromis niloticus; P. bovei, Petrocephalus bovei; P. isidori, Pollimyrus isidori; R. septentrionalis, Rhabdalestes septentrionalis; S. galilaeus, Sarotherodon galilaeus; S. intermedius, Schilbe intermedius;S. mystus, Schilbe Mystus; S. schall, Synodontis schall; C. zillii, Cooptodon zillii.
Macroinvertebrates communities as indicator of human pressures
In total, 40 macroinvertebrate taxa distributed among 10 orders were recorded. Insects have the highest percentage composition (92%) by number, whereas Bivalvia, Annelids, and Arachnids were less represented (< 1%) by number. The tolerant Chironomus were reported only in impaired sites. Notably, overall means of metrics related to macroinvertebrates community structures such as taxa richness (25 ± 3.21), Shannon–Wiener (2.20 ± 0.31), Margalef (3.80 ± 0.19), and true diversity (30.67 ± 7.67) per site clearly distinguished protected wetland from impaired wetland 9.50 ± 0.86, 1.21 ± 0.21, 1.33 ± 0.12, and 9.62 ± 0.9, respectively (Figure 4).
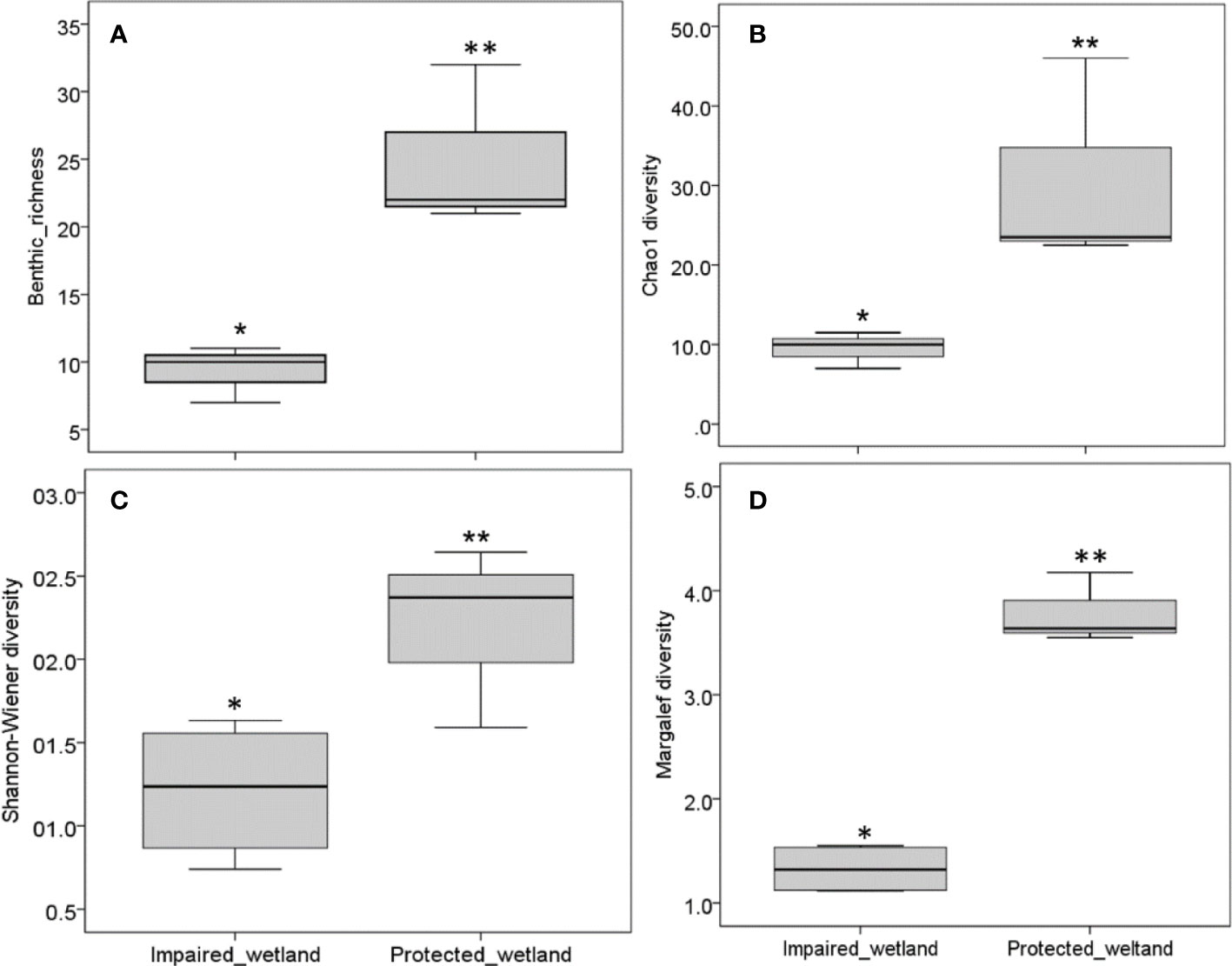
Figure 4 Box plot comparing macroinvertebrate diversity between impaired wetland and protected wetland. Median value is shown in each box; vertical bars correspond to the minimum and maximum values. (A) Benthic-richness, (B) Chao 1 diversity, (C) Shannon–Weiner diversity, (D) Margalef diversity. *indicates statistical significance (Mann–Whitney U test, p< 0.05).
Figure 5 shows biplots of taxa and keys water variables with eigenvalues of 0.826 and 0.279 for axis 1 and axis 2; both axes explained nearby 100% of the variance of overall variables about information of species–environment correlations according to Monte Carlo test (p< 0.05). Thus, dissolved oxygen (r = 0.91) is strongly and positively related to axis 1, whereas the conductivity (r = 0.91) is associated with axis 1. Most of sensitive taxa such as Atyidae, Paleomonidae, Heptageniid and all trichopteran frequency showed a strong correlation with oxygen, while the tolerant taxa including red Chironomus, Hirudinae, and Simulidae are associated with conductivity. The temperature (r = 0.66) is positively correlated with the axis 2. The majority of Molluscs (e.g., Gastropods) were closed to axis 2.
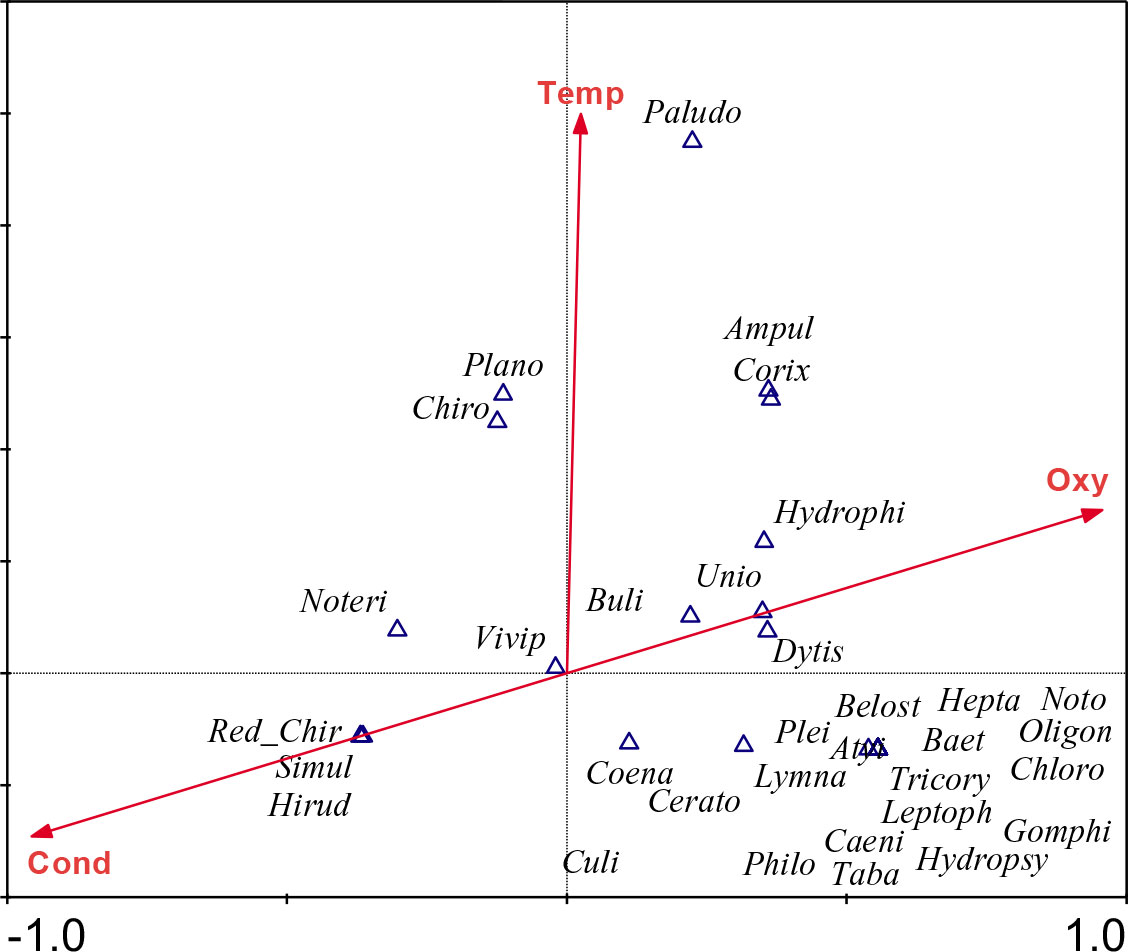
Figure 5 Relationship between physico-chemical variables and benthic macroinvertebrates based on canonical correspondence analysis (CCA). Ampul, Ampullariidae; Atyi, Atyidae; Baet, Baetidae; Belost, Belostomatidae; Buli, Bulinidae; Caeni, Caenidae; Cerato, Ceratopogonidae; Chaob, Chaoboridae; Chiro, Chironomidae; Chloro, Chlorolestidae; Coena, Coenagrionidae; Corbu, Corbuculidae; Corix, Corixidae; Culi, Culicidae; Dytis, Dytiscidae; Elmi, Elmidae; Gerri, Gerridae; Gomphi, Gomphidae; Helotre, Helotrephidae; Hepta, Heptagenidae; Hirud, Hirudinae; Hydroc, Hydrochidae; Hydrophi, Hydrophilidae; Hydropsy, Hydropsychidae; Iridi, Iridinae; Leptoph, Leptophlebeidae; Libel, Libellulidae; Limoni, Limoniidae; Lymna, Lymnaeidae; Macromi, Macromidae; Muteli, Mutelidae; Nauco, Naucoridae; Nepi, Nepidae; Noteri, Noteridae; Noto, Notonectidae; Oligoc, Oligochaeta; Oligon, Oligoneuridae; Palae, Palaemonidae; Paludo, Paludomidae; Philo, Philopotamidae; Pili, Pilidae; Plano, Planorbidae; Plei, Pleidae; Polyce, Polycentropodidae; Polymi, Polymitarcyidae; Ranat, Ranatridae; Red_Chiro, Red_Chironomus; Simul, Simulidae; Sphaer, Sphaeridae; Syrphi, Syrphidae; Taba, Tabanidae; Tanyp, Tanypodinae; Thiari, Thiaridae; Tipul, Tipulidae; Tricory, Tricorythidae; Unio, Unionidae; Vivip, Viviparidae.
Bird and amphibian community structures
In total, 30 species of wild bird were recorded. Most of them were reported in trees and shrubs (19 species) followed by soil ground (eight species). For amphibians, the high species richness was reported in protected wetland, whereas the lower richness was recorded in impaired wetland excepted Chao-1 true diversity (Table 2).
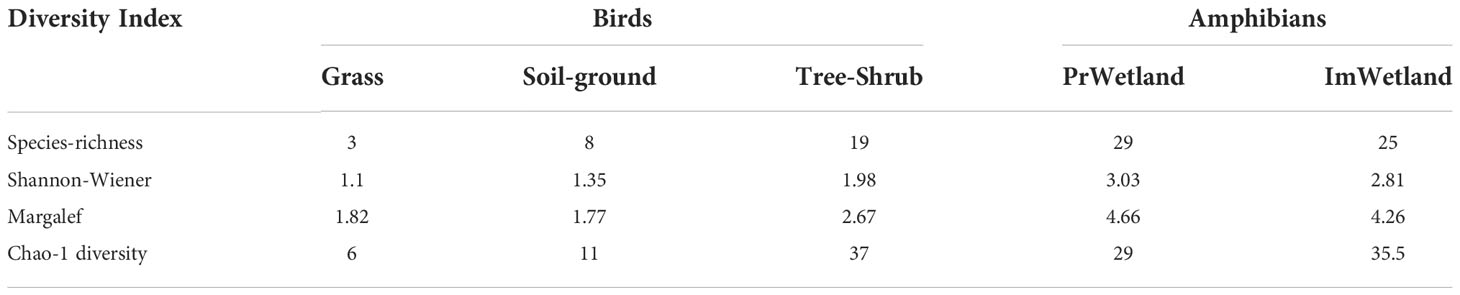
Table 2 diversity of birds and amphibians reported in different habitats of wetland PrWetland (protected wetland), ImWetland (impaired wetland).
For IndVal calculation index conducted between protected and impaired wetlands, we found five amphibians’ potential indicator: genera Hildebrandtia (79), Afrixalus (66.9), Tomopterna (66.8), Hyperolius (50.5), and Leptopelis (50) for the protected wetland. These results highlighted that amphibians and birds can provide specific information of wetland habitat conditions.
Discussion
Wetland biodiversity as an indicator of human pressures
In West Africa, human activities are the main cause of dramatic changes in the structure of wild animals’ communities in the wetland areas. In response to these threats, the species composition, diversity, and functional traits are often used to assess the impact of habitats modification and overall ecosystem health. Here, we covered several biological communities testing their potential use to assess both the diversity and habitats quality of wetlands in Burkina Faso. The variation in fish sizes and abundance, macroinvertebrates, birds, and amphibians revealed a clear distinction between communities’ sensitivity to various types of stressors and might be explained with the taxonomic structures revealed by Figures 2 and 4. Our results confirmed that the assessment of ecological integrity of wetland ecosystems should benefit from comparing multiple systems, because wetlands are an important complex network ecosystem including forests and water networks, as well as an extraordinarily diverse endemic and sensitive biota (Strayer and Dudgeon, 2010; Fonseca et al., 2018; Théau et al., 2018). In spite of the strong evidence of multiple sampling techniques and efforts, the use of valuable taxonomic groups that shows strong associations with the environment can offer additional tools for the bioassessment of wetland ecosystems. Using different bio-indicators can reduce misinterpretation of the data and provide a powerful weight-of-evidence approach for assessing the overall condition of a site, because they differ in their sensitivity to different stressors (Griffith et al., 2005). Most wetlands in western African region, especially in Burkina Faso, are often characterized by forests, rivers/streams, and several stocking waters, including different communities making them ecologically and socio-economically important network habitat types. In this study, we found most of them as reported in (Melcher et al., 2012; Mano et al., 2020), which have already been suggested as bio-indicators of environmental conditions in tropical freshwater ecosystems (Aboua et al., 2012; Meulenbroek et al., 2019). Importantly, using fish community-based approaches (frequencies and structure) and/or sentinel species was well known to measure habitat conditions or overall condition of wetland ecosystems. We found that fish sizes and abundances in protected wetlands differed significantly from impaired wetlands. This could be justified by the protected status of this area with almost natural conditions and less human pressures on water and biological resources. We found 10 taxa of fish confined to protected wetland, most probably due to the sensitivity of these groups to the degree of efficient oxygenation water network and to the availability of specific habitats. These findings are similar to those of Kaboré et al. (2018); Meulenbroek et al. (2019), and Mano et al. (2020) who demonstrate that protected sites are associated with high species diversity, as well as sensitive, rare species, making them the main hotspots of biodiversity in sahelian area. Whereas, impaired wetlands that were exposed to human activities suggest that man made influences were responsible for decreasing overall fish species diversity revealed by Figure 2 and increasing number of tolerant species found here. For example, the high-relative abundances of cyprinids and cichlids observed in impaired wetland can be explained by the fact that these taxa can survive in highly stressed environments and turbid habitats (Witte et al., 2012; Meulenbroek et al., 2019). We found in most impaired wetlands evidence of physical habitats degradation (e.g., flooded and riparian vegetation clearing), leading to the alteration connectivity of wetland network used by fish for reproduction and feeding, and that might reduce opportunities in terms of possible ecological niches Table 1. All impaired wetlands land cover has been replaced by intensive agricultural, mining, and residential activities implying increased anthropogenic pressure on the wetland resources.
Our findings showed that fish community has a high potentiality in ecological indicators, because they reflected various habitats condition (see Figure 3). Our results lie in the same line with those of Stranzl (2014); Kaboré et al. (2016), and Meulenbroek et al. (2019) who demonstrate the habitats modification such as oxygen, conductivity, and PH, substrates, and vegetation, among others may have strong negative effects on fish community, resulting in productivity loss, ecosystem health deterioration, and species declining. Most of physicochemical parameter changes are associated with anthropogenic activities, for example, the higher values of electrical conductivity are an indicator of severe sedimentation resulting from land degradation due to increasing agricultural activities, urbanization and others activities (Twesigye et al., 2011). Our results are also in agreement with Paller et al. (2000) and Bain et al. (2000) who argue that fish community structure could be used to measure restoration success of impaired sites because they can reflect the condition of surrounding riparian habitats which are often associated with human pressures that affect negatively the overall aquatic ecosystem health.
The use of macroinvertebrates as bioindicators in lentic and lotic waters has been largely studied, because they have well-known life history and ecology, and adapted to local environment (Barbour et al., 2000). For example, Kaboré et al. (2016) had demonstrated that diversity measures were better at detecting land use change. The tolerant dipterans found in the present study (e.g., red chironomids) can reflect chronic effects of bad habitats condition. In the present study, most of tolerant taxa were reported in impaired wetlands. For example, some impaired wetland such as Bangr-Weoogo receives domestic and industrial wastes leading to impoverishment of water quality and habitats condition and may explain the strong dominance of tolerant taxa and the decline of local biodiversity. We observed a drastic decrease of macroinvertebrates diversity in impaired wetland. This could be explained by the fact that impaired wetlands are prone to threats, emanating from anthropogenic activities. These results are similar to those of Kaboré et al. (2016) and Tampo et al. (2021) who show that changes in macroinvertebrates structure are often associated with sites riparian land use and water pollution. Promoting different approaches using benthic macroinvertebrates in bioassessment through multimetric and scoring systems could be extended to natural wetland, as far they include several water bodies types (e.g. rivers/streams and ponds), like others, the authors have proven its use for riverine ecosystems (Ofenböck et al., 2010; Tampo et al., 2020; Tampo et al., 2021; Kaboré et al., 2022). This can help to perform better assessment methods of wetland ecosystems including overall habitat condition and biota. Our findings revealed that benthic macroinvertebrates may have different levels of sensitivity to physicochemical parameters (Figure 5). The results support other studies that found that sensitive taxa such as heptageniids, trichopterans, and shrimp’s taxa are often the most abundant group reported in protected sites with a sufficiently dissolved oxygen content and good habitats condition. Here, tolerant taxa, such as Chironomus, leeches, and molluscs found in impaired sites, often indicate organic pollution coming from intensive agricultural activities (e.g., use of fertilizers) and allochthonous substrate, as confirmed in previous studies (Kaboré et al., 2016; Tampo et al., 2021; Bancé, 2022). Some authors have demonstrated that the combined effect of riparian land use and local scale human activities and physico-chemical acts to influence the diversity, composition of macroinvertebrate assemblages at the sampled sites (Kaboré et al., 2016; Tampo et al., 2021).
Bird communities are useful indicators, because they are integrators of the cumulative effects of multiple environmental influences in a landscape (Environmental Protection Agency USEPA, 2002; Zwartsa et al., 2018). In West Africa, especially in Burkina Faso, there is still a need for developing bird biological integrity index because avifaunal indices (abundance, diversity, and trophic measures) can mainly act as an indicator of land use alteration, habitat fragmentation, as we have seen in this study. We found many bird species in the study areas dwell in diverse ecological niches. The high diversity of bird in protected wetlands demonstrates the natural intactness of the area characterized by natural preserved habitats. The naturalness of these wetlands (e.g., density of shrubs, trees, and vegetation cover) enhances environmental heterogeneity, provides more niches, or improves food and nest sites conditions for birds. According to Lee and Martin (2017) and Bellanthudawa et al. (2019), habitats are valuable factors that determine bird community composition and diversity and may reflect the condition of the particular area. Furthermore, Adamus et al. (2001) and Ladin et al. (2016) argue that bird communities can reflect the overall ecosystem condition and its components, including water quality, vegetation composition, and productivity. The lower diversity of bird reported in grass lands and ground can show that most of grasslands are used for local human activities such as agriculture and animals breeding, as demonstrated by Kaboré et al. (2022). Despite the limitation of data to provide deeper insight into the ecological organization of birds, we address the necessity of using birds in bioassessment in Sahel wetland ecosystems. Because of bird’s well-known life history, their high societal value and their sensitivity to environmental changes link to their wide distribution can make them remarkably attractive for conservationists, managers, and policy makers, as demonstrated by Fahrig (2003) and Hevia et al. (2015). Developing indicators that environmental managers and regulators can easily use to communicate and implement policy could be an important tool for suitable management of wetland Niemi and McDonald (2004). Avian metrics (e.g., taxonomic diversity) have proven useful in many assessments’ methods (O’Connell et al., 2000; O’Connell et al., 2007) but still limited in West African Sahel region and particularly in Burkina Faso. This study is the first attempt for a large-scale assessment of several taxonomic groups in the wetland areas of Burkina Faso, but more data and detailed analyses are still needed to understand interactions between bird communities and complex network ecosystems.
Among the vertebrate, amphibians serve as vital links in food webs and between wetland and upland habitats and constitute an important component of wetland ecosystems (Ayoro et al., 2020). Here, we encountered high diversity of amphibians in protected wetland. This could be explained by the fact that the protected wetland ecosystems have conditions favorable for amphibian survival. The lower diversity reported in impaired wetland could be explained by various human-induced processes, including land use change, introduction of predators, and spread of toxic substances that affect the diversity (Camacho-Valdez et al., 2014; Xu et al., 2019). The failure of true Chaos diversity index as indicator metric is the proof of general concerns about the ongoing general degradation of our environment due to human activities and climatic variability and may lead amphibian’s species to change habitats. Amphibians are closely associated with water and riparian vegetation and can respond to chemical, physical, and ecological stress by migrating. Our results revealed that amphibians’ genera such as Hildebrandtia, Afrixalus, Tomopterna, and Hyperolius are specially associated with protected wetland and may be used as sensitive taxa. The indicator taxa based on amphibians may be very useful for monitoring wetlands in Burkina Faso, because amphibians’ species shelter in various habitats reflecting possible overall wetland ecosystems health, as demonstrated by Ayoro et al. (2020). However, extensive research is still needed to explore the behaviors of each single species with water and surrounding landscapes features. For this reason, we encourage scientists and local managers to examine amphibian communities as a possible tool for Sahel wetland monitoring and biodiversity conservation.
National wetland management and biodiversity conservation
Wetlands are an important complex ecosystem with many valuable functions and services for human well-being (Mexia et al., 2018; Stephenson et al., 2020). In West Africa, wetlands constitute an important natural resource with high economic, cultural, and scientific value. However, the actual threats to wetlands in this region arise from the hydro-morphological modifications, pollution, deforestation caused by intense urbanization, agriculture using fertilizers and pesticides, as well as industry activities leading to biodiversity and sensitive species loss, goods disappearance, and soil erosion. We found that human responses to threats and maintaining the interaction between physicochemical parameters, biological community, and immediate environment are crucial to the preservation of wetland ecological integrity (Figure 6). Despite that many management programmes undertaken over the world, the policies aimed at wetland conservation are either non-existent or very weak (Gopal, 2013; Adeeyo et al., 2022), particularly in western African countries. Promoting tools that include local benthic macroinvertebrates, fish, amphibians, and birds to preserve the remaining wetlands and habitats may be a very good approach to improve wetlands management and decision making because, when the interaction between wetland biota and the environment is disrupted, many functions and services provided to humans by wetlands will be altered or lost. Importantly, any research should encompass systemic assessment of how links and interactions within and between the biotic components including threats to wetland ecosystems, as well as climatic changes and the socio-economic sides to improve a national wetland assessment programs (Stephenson et al., 2020). We also recognize that the specific sampling design, which involves local managers, could lead to a co-creation of knowledge and produce standardized tools to be used by local population. Data on environmental variables are still needed to explore the relationships between each environmental variable and biological parameters. Extending the sampling to others taxonomic (zooplankton, phytoplankton, macrophytes, and trees) can provide additional valuable information on other human influences and biodiversity. According to Stephenson et al. (2015), the biodiversity and species abundance is still one of the most pertinent indicators for conservation.
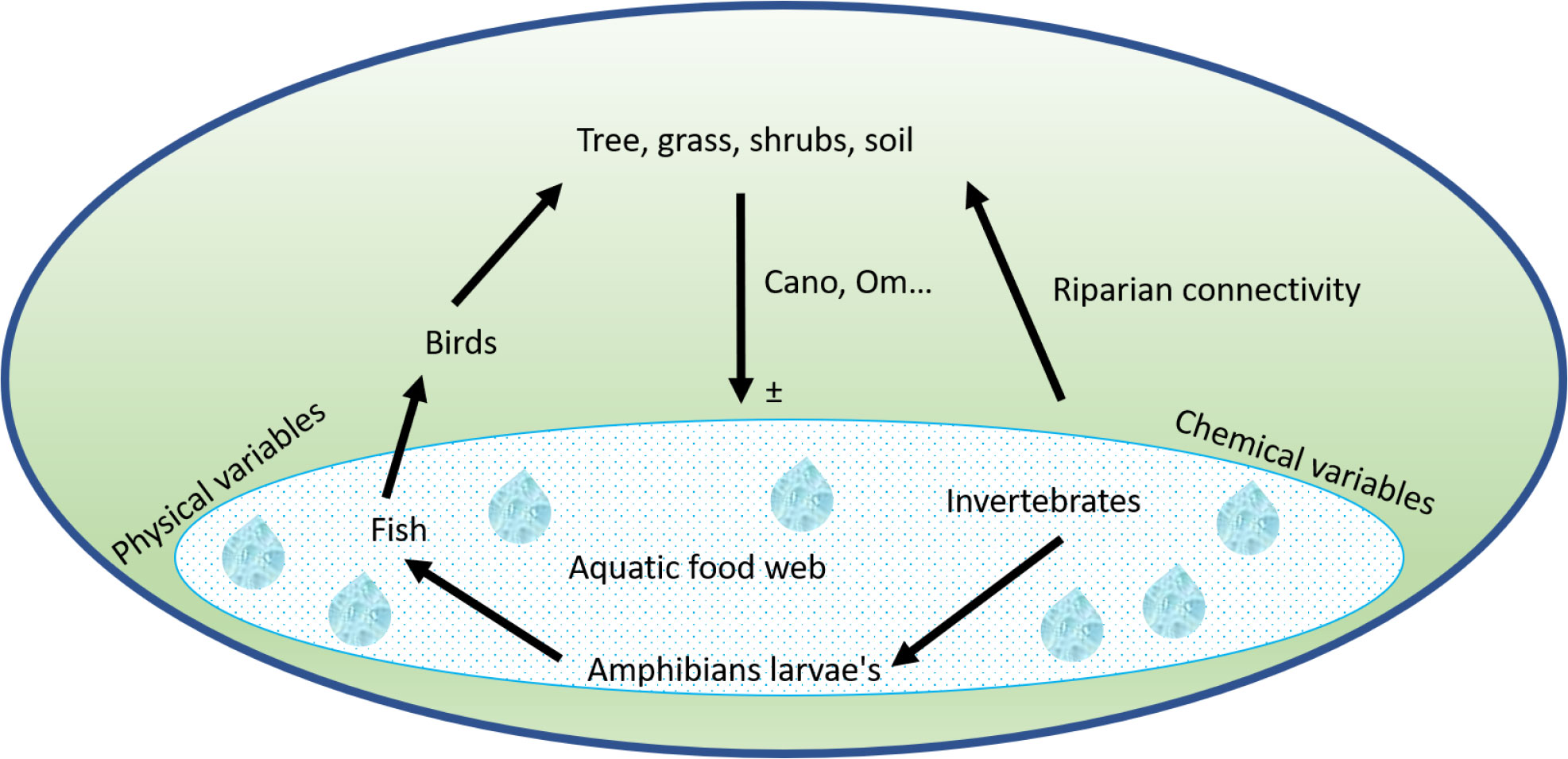
Figure 6 Wetland ecological integrity functioning, (Cano) Canopy, and (Om) organic matter; ± indicates positive/negative effects of vegetation in water network.
Conclusions
Wetlands in West Africa, particularly in Burkina Faso, are extremely overexploited and impaired by many human activities, as well as climate variability. Therefore, the need for wetland biomonitoring is evident, and assessing the ecological status of wetland and identifying the threats is essential to develop adequate restoration and conservation strategies. This study revealed that wetland ecosystems bear high birds, amphibians, fish, and macroinvertebrates richness and are particularly effective as indicators of wetland health conditions in Burkina Faso. These organisms were found to be very sensitive to human pressures and can be used for earlier detection of wetland ecosystem disturbance. Also, this research explored methods of using wetland biota to implement and improve strategies of management and conservation of wetland ecosystem networks. However, further studies and more data are still needed to sustain an intensive research effort to generate definitive results useful for policy. Therefore, to the best of our knowledge, these preliminary results are the first step toward the promotion of large use of local biota in the development of wetland biomonitoring program for long-term sustainability of biodiversity and wetland ecosystems for future generations in Sahel area.
Data availability statement
The original contributions presented in the study are included in the article/Supplementary Material. Further inquiries can be directed to the corresponding author.
Author contributions
IK conceived the manuscript and wrote the paper with LT and VB. IK, VB and AHJ collected and analysed the data. AJ and CD assisted IK in the laboratory work. KM and AO supervised the laboratory analyses. IK and TL corrected the English language. KM and AO supervised the study and contributed valuable comments to the manuscript. All authors contributed to the article and approved the submitted version.
Acknowledgments
We are grateful to PhD students of department of Biology and physiology animals from university Joseph KI-ZERBO for their help in data collection. We also are extremely grateful to Bella Nyamukure for English proof reading, and to two anonymous reviewers for many insightful comments which largely helped to improve the manuscript.
Conflict of interest
The authors declare that the research was conducted in the absence of any commercial or financial relationships that could be construed as a potential conflict of interest.
Publisher’s note
All claims expressed in this article are solely those of the authors and do not necessarily represent those of their affiliated organizations, or those of the publisher, the editors and the reviewers. Any product that may be evaluated in this article, or claim that may be made by its manufacturer, is not guaranteed or endorsed by the publisher.
References
Aboua B. R. D., Kouaméla E. P., N’Douba V. (2012). Development of a fish-based index of biotic integrity (FIBI) to assess the quality of bandama river in côte d’Ivoire. Knowl. Managt. Aquat. Ecosyst. 404, 08. doi: 10.1051/kmae/2012002
Adamus P. R., Danielson T. J., Gonyaw A. (2001). Indicators for monitoring biological integrity of inland, freshwater wetlands: a survey of north American technical literature, (1990-2000) (Washington D.C: U.S. Environmental Protection Agency, Office of Water, Wetlands Division).
Adeeyo A. O., Ndlovu S. S., Ngwagwe L. M., Mudau M., Alabi M. A., Edokpayi J. N. (2022). Wetland resources in south Africa: Threats and metadata study. Resources 11, 54. doi: 10.3390/resources11060054
Alikhani S., Nummi P., Ojala A. (2021). Urban wetlands: A review on ecological and cultural values. Water 13, 3301. doi: 10.3390/w13223301
Arowosafe F. C., Wuleka K. C. J., Emma E-M. M. R. (2019). Perceptions on benefits of ecotourism development by residents of fringe communities to two national parks inWest Africa. Am. J. Tourism. Manage. 8 (1), 8–18. doi: 10.5923/j.tourism.20190801.02
Ayanlade A., Proske U. (2016). Assessing wetland degradation and loss of ecosystem services in the Niger delta, Nigeria. Mar. Freshw. Res. 67, 828–836. doi: 10.1071/MF15066
Ayoro H. J., Segniagbeto G. H., Hema E. M., Penner J., Oueda A., Dubois A., et al. (2020). : List of amphibian species (Vertebrata, tetrapoda) of Burkina Faso. Zoosystema 42 (28), 515550. doi: 10.5252/zoosystema2020v42a28
Bain M. B., Harig A. L., Loucks D. P., Goforth R. R., Mills K. E. (2000). Aquatic ecosystem protection and restoration: advances in methods for assessment and evaluation. Environ. Sci. Policy 3, 89–98. doi: 10.1016/S1462-9011(00)00029-0
Bancé V. (2022). Macroinvertébrés des écosystèmes lacustres du Burkina Faso : diversité, écologie et définition d’un indice biotique (Burkina Faso: Université Joeph KI-ZERBO), 183 p.
Barbour M. T., Swietlik W. F., Jackson S. K., Courtemanch D. L., Davies S. P., Yoder C. O. (2000). Measuring the attainment of biological integrity in the USA: A critical element of ecological integrity. Hydrobiologia 422/423, 453–464. doi: 10.1023/A:1017095003609
Barratt Heitmann J. R. (2018). "National parks and development in Sub-Saharan Africa: and examination of social and economic program effectiveness in the development paradigm" (2018) Vol. 2018 (Senior Projects Spring), 216. Available at: https://digitalcommons.bard.edu/senproj_s2018/216.
Bellanthudawa B. K. A., Nawalage N. M. S. K., Subanky S., Panagoda P. A. B. G., Weerasinghe H.W. G. A. S., Tharaka L. K. D. N., et al. (2019). Composition and diversity variation of avifauna, along different vegetative habitat types in a human-modified area, university of kelaniya, Sri Lanka. Int. J. Zool. Volume 2019, 16 pages. doi: 10.1155/2019/9727609
Clarkson B. R., Sorrell B. K., Reeves P. N., Champion P. D., Partridge T. R., Clarkson B. D. (2004). Handbook for monitoring wetland condition. Coordinated monitoring of New Zealand wetlands. A Ministry for the Environment SMF funded project (Wellington: Ministry for the Environment). doi: 10.7931/J2Z60KZ3
Camacho-Valdez V., Ruiz-Luna A., Ghermandi A., Berlanga-Robles C. A., Nunes P. A. L. D. (2014). Effects of land use changes on the ecosystem service values of coastal wetlands. Environ. Manage. 54, 852–864. doi: 10.1007/s00267-014-0332-9
Chao A. (1987). Estimating the population size for capture-recapture data with unequal catchability. Biometrics 43 (4), 783–791. doi: 10.2307/2531532
Colwell R. K., Coddington J. A. (1994). Estimating terrestrial biodiversity through extrapolation. Philos. Trans. R. Soc. Lond. B. Biol. Sci. 345 (1311), 101–118. doi: 10.1098/rstb.1994.0091
Dufrêne M., Legendre P. (1997). Species assemblages and indicator species: The need for a flexible asymmetrical approach. Ecol. Monogr. 67, 345–366. doi: 10.2307/2963459
Egler M., Buss D. F., Moreira J. C., Baptista D. F. (2012). Influence of agricultural land-use and pesticides on benthic macroinvertebrate assemblages in an agricultural river basin in southeast Brazil. Ecol. Braz. J. Biol. 72 (3), 437–443. doi: 10.1590/S1519-69842012000300004
Fahrig L. (2003). Effects of habitat fragmentation on biodiversity. Annu. Rev. Ecol. Evol. S. 34, 487–515. doi: 10.1146/annurev.ecolsys.34.011802.132419
Fonseca B. M., de Mendonça-Galvão L., Sousa F. D. R., Elmoor-Loureiro L. M. A., Gomes-e-Souza M. B., Pinto R. L., et al. (2018). Biodiversity in pristine wetlands of central Brazil: a multi-taxonomic approach. Wetlands 38, 145–156. doi: 10.1007/s13157-017-0964-7
Gopal B. (2013). Future of wetlands in tropical and subtropical Asia, especially in the face of climate change. Aquat. Sci. 75, 39–61. doi: 10.1007/s00027-011-0247
Griffith M. B., Hill B. H., McCormick F. H., Kaufmann P. R., Herlihy A. T., Selle A. R. (2005). Comparative application of indices of biotic integrity based on periphyton, macroinvertebrates, and fish to southern rocky mountain streams. Ecol. Indic 5, 117–136. doi: 10.1016/j.ecolind.2004.11.001
Gumbricht T., Roman-Cuesta R. M., Verchot L., Herold M., Wittmann F., Householder E., et al. (2017). An expert system model for mapping tropical wetlands and peatlands reveals south America as the largest contributor. Glob. Change Biol. 23, 3581–3599. doi: 10.1111/gcb.13689
Haro M., Guiguemde I., Diendere F., Bani I., Kone M., Soubeiga M., et al. (2013). Effect of the kossodo industrial wastewater discharges on the physico chemical quality of massili river in Burkina Faso. Res. J. Chem. Sci. 3, 85–91.
Héma E. M., Barnes R. F. W., Guenda W. (2010). The seasonal distribution of savannah elephants (Loxodonta africana africana) in nazinga game ranch, southern Burkina Faso. Pachyderm 48, 33–40.
Hevia V., Carmona C. P., Azcárate F. M., Torralba M., Alcorlo P., Ariño R., et al. (2015). Effects of land use on taxonomic and functional diversity: a cross-taxon analysis in a Mediterranean landscape. Oecologia 181, 959–970. doi: 10.1007/s00442-015-3512-2
Junk W. J. (2002). Long-term environmental trends and the future of tropical wetlands. Environ. Conserv. 29 (4), 414–435. doi: 10.1017/S0376892902000310
Kaboré I., Moog O., Alp M., Guenda W., Koblinger T., Mano K., et al. (2016). Using macroinvertebrates for ecosystem health assessment in semi-arid streams of Burkina Faso. Hydrobiologia 766 (1), 57–74. doi: 10.1007/s10750-015-2443-6
Kaboré I., Moog O., Oueda A., Sendzimir J., Ouedraogo R., Guenda W., et al. (2018). Developing reference criteria for the ecological status of West African rivers. Environ. Monit. Assess. 190 (1), 2. doi: 10.1007/s10661-017-6360-1
Kaboré I., Ouéda A., Moog O., Meulenbroek P., Tampo L., Bancé V., et al. (2022). Benthic invertebrates-based biotic index to assess the ecological status of West African sahel rivers, Burkina Faso. J. Environ. Manage. 307 (16), 114503. doi: 10.1016/j.jenvman.2022.114503
Ladin Z. S., Higgins C. D., Schmit J. P., Sanders G., Johnson M. J., Weed A. S., et al. (2016). Using regional bird community dynamics to evaluate ecological integrity within national parks. Ecosphere 7 (9), e01464. doi: 10.1002/ecs2.1464
Lamizana/Diallo M. B., Kenfack S., Millogo/Rasolodimby J. (2008). Evaluation of the physicochemical quality of water from a temporary stream of Burkina Faso - the case of the massili in kadiogo. South Sci. Technol. 16), 23–28.
Lee M.-B., Martin J. A. (2017). Avian species and functional diversity in agricultural landscapes: Does landscape heterogeneity matter? PloS One 12 (1), e0170540. doi: 10.1371/journal.pone.0170540
Lepš J., Šmilauer P. (2003). Multivariate analysis of ecological data using CANOCO. (Cambridge: Cambridge University Press).
Mano K., Oueda A., Ouedraogo R., Ouedraogo I., Kaboré I., Kabré G. B., et al. (2020). Fish assemblages in the upper part of the Volta river, Burkina Faso: A link analysis towards fisheries management and conser. Int. J. Biol. Chem. Sci. 13 (6), 2560–2572. doi: 10.4314/ijbcs.v13i6.11
Melcher A. H., Ouédraogo R., Schmutz S. (2012). Spatial and seasonal fish community patterns in impacted and protected semi-arid rivers of Burkina Faso. Ecol. Eng. 48, 117–129. doi: 10.1016/j.ecoleng.2011.07.012
Meulenbroek P., Stranzl S., Oueda A., Sendzimir J., Mano K., Kabore I., et al. (2019). Fish communities, habitat use, and human pressures in the upper Volta basin, Burkina Faso, West Africa. Sustainability 11 (19), 5444. doi: 10.3390/su11195444
Mexia T., Vieiraa J., Príncipea A., Anjosa A., Silvab P., Lopesb N., et al. (2018). Ecosystem services: Urban parks under a magnifying glass. Environ. Res. 160, 469–478. doi: 10.1016/j.envres.2017.10.023
Moog O. (2007). Deliverable 8 – part 1. manual on pro-rata multi-habitat-sampling of benthic invertebrates from wadeable rivers in the HKH-region. BOKU (Vienna: University of Natural Resources and Applied Life Sciences), 28 p. Available at: www.assess-hkh.at.
Newton A., Icely J., Cristina S., Perillo G. M. E., Turner R. E., Ashan D., et al. (2020). Anthropogenic, direct pressures on coastal wetlands. Front. Ecol. Evol. 8. doi: 10.3389/fevo.2020.00144
Niemi G. J., McDonald M. E. (2004). Application of ecological indicators. Annu. Rev. ecol. evol. Systemat 35, 89–111. doi: 10.1146/annurev.ecolsys.35.112202.130132
O’Connell T. J., Bishop J. A., Brooks R. P. (2007). Sub-Sampling data from the north American breeding bird survey for application to the bird community index, an indicator of ecological condition. Ecol. Indic. 7(2007), 679–691. doi: 10.1016/j.ecolind.2006.07.007
O’Connell J. T., Jackson L. E., Brooks R. P. (2000). Bird guilds as indicators of ecological condition in the central appalachians. Ecol. Appl. 10 (6), 1706–1721. doi: 10.1890/1051-0761(2000)010[1706:BGAIOE]2.0.CO;2
Ofenböck T., Moog O., Sharma S., Korte T. (2010). Development of the HKHbios: a new biotic score to assess the river quality in the Hindu kush-himalaya. Hydrobiologia 651 (1), 39–58. doi: 10.1007/s10750-010-0289-5
Ola O., Benjamin E. (2019). Preserving biodiversity and ecosystem services in West African forest, watersheds, and wetlands: A review of incentives. Forests 10 (6), 479. doi: 10.3390/f10060479
Paller M. H., Reichert M. J. M., Dean J. M., Seigle J. C. (2000). Use of fish community data to evaluate restoration success of a riparian stream. Ecol. Eng. 15 (2000), 171–187. doi: 10.1016/S0925-8574(99)00082-8
Parienté W. (2017). Urbanization in Sub-Saharan Africa and the challenge of access to basic services. J. Demogr. Eco. 83 (1), 31–39. doi: 10.1017/dem.2017.3
Paugy D., Lévêque C., Teugels G. G. (2003). Poissons d’Eaux Douces et Saumâtres de l’Afrique de l’Ouest, Tome 2. Paris: IRD.
Rafiq R., Alam S., Rahman M., Islam I. (2013). Conserving wetlands: Valuation of indirect use of benefits of a wetland of Dhaka. Inter. J. Environ. Sci. Dev. 5, 64–69. doi: 10.7763/IJESD.2014.V5.452
Rödel M.-O., Ernst R. (2003). The amphibians of marahoué and mont péko national parks, ivory coast. Herpetozoa 16, 23–39.
Sarkar U. K., Mishal P., Borah S., Karnatak G., Chandra G., Kumari S., et al. (2020). Status, potential, prospects, and issues of floodplain wetland fisheries in India: Synthesis and review for sustainable management. Rev. Fisheries. Sci. Aquacult 29, 1–32. doi: 10.1080/23308249.2020.1779650
Saunders M. J., Kansiime F., Jones M. B. (2013). Reviewing the carbon cycle dynamics and carbon sequestration potential of cyperus papyrus l. wetlands in tropical Africa. Wetlands Ecol Manage 22, 143–155. doi: 10.1007/s11273-013-9314-
Sinthumule N. I., Netshisaulu K. H. (2022). Wetland resource use and conservation attitudes of rural vs. urban dwellers: A comparative analysis in thohoyandou, Limpopo province, south Africa. Water 14, 1290. doi: 10.3390/w140812
Spieles D. J. (2022). Wetland construction, restoration, and integration: A comparative review. Land 11, 554. doi: 10.3390/land11040554
Stephenson P. J., Ntiamoa-Baidu Y., Simaika J. P. (2020). The use of traditional and modern tools for monitoring wetlands biodiversity in Africa: Challenges and opportunities. Front. Environ. Sci. 8. doi: 10.3389/fenvs.2020.00061
Stephenson P. J., O’Connor S., Reidhead W., Loh J. (2015). Using biodiversity indicators for conservation. Oryx 49, 396. doi: 10.1017/S0030605315000460
Stranzl S. (2014). Quantification of human impacts on fish assemblages in the upper Volta catchment, Burkina Faso (Vienna, Austria: University of Natural Resources and Life Sciences), p 90.
Strayer D. L., Dudgeon D. (2010). Freshwater biodiversity conservation: recent progress and future challenges. North Am. Benthol. Soc. 29 (1), 344–358. doi: 10.1899/08-171.1
Tampo L., Kaboré I., Alhassan H. E., Ouéda A., Bawa M. L., Boundjou G. D. (2021). Benthic macroinvertebrates as ecological indicators: Their sensitivity to the water quality and human disturbances in a tropical river. Front. Water 3, 662765. doi: 10.3389/frwa.2021.662765
Tampo L., Lazar I. M., Kaboré I., Oueda A., Akpataku K. V., Djaneye-Boundjou G., et al. (2020). A multimetric index for assessment of aquatic ecosystem health based on macroinvertebrates for the zio river basin in Togo. Limnologica 83, 125783. doi: 10.1016/j.limno.2020.125783
Ter Braak C. J., Smilauer P. (2002) CANOCO reference manual and CanoDraw for windows user's guide: software for canonical community ordination (version 4.5). Available at: www.canoco.com.
Thapa S., Wang L., Koirala A., Shrestha S., Bhattarai S., Aye W. N. (2020). Valuation of ecosystem services from an important wetland of Nepal: A study from begnas watershed system. Wetlands 40, 1071–1083. doi: 10.1007/s13157-020-01303-7
Théau J., Trottier S., Graillon P. (2018). Optimization of an ecological integrity monitoring program for protected areas: Case study for a network of national parks. PloS One 13 (9), e0202902. doi: 10.1371/journal.pone.0202902
Thiollay J. M. (2006). Large Bird declines with increasing human pressure in savanna woodlands (Burkina Faso). Biodivers. Conserv. 15, 2085–2108. doi: 10.1007/s10531-004-6684-3
Twesigye C. K., Onywere S. M., Getenga Z. M., Mwakalila S. S., Nakiranda J. K. (2011). The impact of land use activities on vegetation cover and water quality in the lake Victoria watershed. Open Environ. Eng. J. 4, 66–77. doi: 10.2174/1874829501104010066
USEPA (2002). Guidelines for ensuring and maximizing the quality, objectivity, utility, and integrity of information disseminated by the environmental protection agency. Washington, DC: U.S. Environmental Protection Agency, Office of Environmental Information (2810).
Wetlands International (2017). Water shocks: Wetlands and human migration in the sahel (The Netherlands: Wetlands International).
Witte F., Wanink J. H., Kishe-Machumu M. A., Rensing M., Goldschmidt T. (2012). Cichlid species diversity in naturally and anthropogenically turbid habitats of lake Victoria, East Africa. Aquat. Sci. 75, 169–183. doi: 10.1007/s00027-012-0265-4
Xu T., Weng B., Yan D., un Wang K., Li X., Bi W., et al. (2019). Wetlands of international importance: Status, threats, and future protection. Int. J. Environ. Res. Public Health 16 (10), 1818. doi: 10.3390/ijerph16101818
Keywords: wetland, networks, biota, integrity, dryland
Citation: Kaboré I, Tampo L, Bancé V, Daboné C, Mano K, Ayoro HJ and Ouéda A (2022) Preliminary biological data of Sahel wetland ecosystems in Burkina Faso: Implications for ecological health assessment. Front. Conserv. Sci. 3:913333. doi: 10.3389/fcosc.2022.913333
Received: 23 June 2022; Accepted: 07 November 2022;
Published: 25 November 2022.
Edited by:
Vanessa Hull, University of Florida, United StatesReviewed by:
Augustine Ovie Edegbene, Federal University of Health Sciences Otukpo, NigeriaAdeeyo Ao, University of Venda, South Africa
Copyright © 2022 Kaboré, Tampo, Bancé, Daboné, Mano, Ayoro and Ouéda. This is an open-access article distributed under the terms of the Creative Commons Attribution License (CC BY). The use, distribution or reproduction in other forums is permitted, provided the original author(s) and the copyright owner(s) are credited and that the original publication in this journal is cited, in accordance with accepted academic practice. No use, distribution or reproduction is permitted which does not comply with these terms.
*Correspondence: Idrissa Kaboré, ikabore16@yahoo.fr