- 1The Nature Conservancy, Stewardship Department, San Diego, CA, United States
- 2United States (US) Geological Survey, Western Ecological Research Center, San Diego, CA, United States
- 3San Diego Natural History Museum, Biodiversity Research Center of the Californias, San Diego, CA, United States
- 4Conservación de Fauna del Noroeste, Ensenada, Mexico
- 5United States Fish and Wildlife Service, Conservation Partnerships Program, Carlsbad, CA, United States
Conservation translocations, the human-mediated movement and release of a living organism for a conservation benefit, are increasingly recommended in species’ recovery plans as a technique for mitigating population declines or augmenting genetic diversity. However, translocation protocols for species with broad distributions may require regionally specific considerations to increase success, as environmental gradients may pose different constraints on population establishment and persistence in different parts of the range. Here we report on ongoing, genetically informed translocations of a threatened amphibian, California red-legged frog (Rana draytonii), from Baja California, México, to extirpated parts of the range in southern California in the United States, where contemporary stressors related to urbanization, invasive species, and aridification add to the natural environmental challenges already present for amphibians at this ‘warm edge’ of the range. We describe the collaborative binational planning required to jumpstart the effort, the fine-tuning of protocols for collection, transport, headstarting, and release of individuals, and results of multiple translocations, where time will tell whether the successes to date have reached their full potential. The steps outlined in this paper can serve as a template to inform future conservation translocations of imperiled amphibians across the U.S./México border, where the phylogenetics, historical biogeography and future habitat availability of a focal species are blind to political boundaries and critical to guiding recovery actions across the range.
1 Introduction
Global declines in biodiversity are necessitating bold and rapid action to abate threats and reduce the risk of extirpation or extinction. Translocation of individuals from certain parts of a species range to others is one such method that has been used to good effect for species recovery, but the process is often challenging, and myriad considerations are necessary to achieve success (Dodd and Seigel, 1991; Reinert, 1991; IUCN/SCC, 2013; Seddon et al., 2014; Berger-Tal et al., 2020). Types of translocations include reintroduction (i.e., the release of organisms into extirpated parts of the native range), reinforcement (i.e., release of organisms to enhance existing populations), assisted colonization (i.e., release of an organisms outside the indigenous range to avoid extinction), and ecological replacement (i.e., release of organisms outside the range to perform a specific ecological function), some or all which may be required for the most imperiled populations, species, or ecosystems (Fischer and Lindenmayer, 2000; IUCN/SCC, 2013; Hayward and Slotow, 2016). That 40% of the world’s nearly 7,000 amphibian species are threatened with extinction underscores a need to evaluate translocation and other forms of intervention as tools that can promote recovery (Wake and Vredenburg, 2008; Linhoff et al., 2021).
Translocation planning includes identification of source populations and recipient sites, willing landowners, threat abatement, long-term ecological management, information about population genetics and phylogenetics, post-release monitoring, and funding (IUNC/SCC, 2013; Linhoff et al., 2021). Accounting for taxon-specific behavioral traits and life history is also important to improve outcomes, particularly for protected species (Berger-Tal et al., 2020). For those with broad distributions, environmental heterogeneity can introduce limiting factors in parts of a species’ range that may reduce the efficacy of a translocation protocol that worked well in other parts of the range. Therefore, knowledge built through experimentation in different parts of the range may be necessary to optimize the technique in different environments.
In the United States, reintroduction is often identified as a recovery action for species listed under the Federal Endangered Species Act of 1973, such as the threatened California red-legged frog (Rana draytonii) (USFWS, 2002). Prior to its decline, R. draytonii was abundant in California and Baja California, México, occupying coastal drainages from Mendocino County and the Sierra Nevada in northern California south into northwestern Baja California, México. Today, the species occupies less than 30% of its historical range (Thompson et al., 2016). Populations in southern California (i.e., San Bernardino, Riverside, Orange and San Diego counties) and much of Baja California are now extirpated, leaving a ~420 km gap in the southern part of the species’ range (Peralta-García et al., 2016; Backlin et al., 2018).
The population genetic structuring and ancestry of R. draytonii in northern Baja California and southern California have been examined using mitochondrial, microsatellite, and genomic data (Shaffer et al., 2004; Richmond et al., 2013; Peralta García, 2017; Backlin et al., 2018; USGS unpub. data). These studies confirm that all populations in Baja California, México, form a single, well-supported lineage within R. draytonii that is distinctive from other lineages north of the Los Angeles Basin in California (‘Baja California Lineage’).
The causes of decline rangewide include habitat conversion and fragmentation; modified hydrology; pollution; mining; poorly managed livestock grazing; overharvesting for food in the late 19th and early 20th centuries; proliferation of nonnative predators (e.g., American bullfrog (Rana catesbeiana), red swamp crayfish (Procambarus clarkii), and a variety of nonnative predatory fish species); prolonged drought (USFWS, 2002; Peralta-García et al., 2016; Thompson et al., 2016); and possibly the amphibian fungal disease chytridiomycosis (Adams et al., 2017).
Since its U.S. federal listing (USFWS, 1996), recovery actions for the Baja California Lineage of R. draytonii have languished in the southern range gap, despite measures in other parts of the state that include translocation (e.g., Santa Monica Mountains National Recreation Area, Pinnacles National Park, Golden Gate National Recreation Area, and Yosemite National Park). Reintroduction planning at the warm, arid edge of the species’ range south of the Los Angeles Basin (in contrast with the cooler, wetter edge in northern California) was stalled due to a lack of approved reintroduction sites, persistent threats (namely invasive exotic species and drought), unidentified source populations, and naivety regarding the international permit process. Meanwhile, the last known population of R. draytonii at the Santa Rosa Plateau, in Riverside, California, became extirpated sometime after 2002.
During the nearly 20-year period that followed, organizations including Conservación de Fauna del Noroeste A.C. (FAUNO), San Diego Natural History Museum (SDNHM), The Nature Conservancy (TNC), U.S. Fish and Wildlife Service (USFWS), and U.S. Geological Survey (USGS) laid the foundation for reintroduction of R. draytonii through habitat protection and restoration, threat management, genetics research, source population assessment, development of translocation methods, and investigation of the international permitting process. In 2019, these organizations formed a coordinated working group focused on the reintroduction of R. draytonii from México, including the development of a translocation feasibility analysis and reintroduction plan that identified the following short-term goals: (1) conduct three annual translocations from Baja California to two sites (see Methods) in the extirpated parts of the species’ range in San Diego and Riverside counties in southern California (2020 to 2022); (2) conduct annual monitoring at both reintroduction sites for six years (2020 – 2025); (3) conduct annual monitoring of each source population in Baja California for six years (2020 – 2025); and (4) have fully established populations (i.e., reproducing) at both recipient sites by 2025.
In this paper we describe our collective translocation of R. draytonii across the U.S./México border, steps that made the intervention possible, and determinants of success to date. The ~420 km gap now separating populations in southern California and northern Baja California makes this one of the largest distances ever covered for a translocation experiment in frogs (Figure 1). Our objectives for the effort were threefold: (1) to increase genetic representation of the declining Baja California lineage within R. draytonii; (2) to repatriate parts of the historic range in southern California, an action outlined in the species Recovery Plan (USFWS, 2002); and (3) to establish a process for the binational recovery of R. draytonii. This effort adds to a growing list of translocations for R. draytonii in California, including further north in the Santa Monica Mountains of Los Angeles County, where a single population remained until managers used the technique to mitigate further retraction of the range in coastal southern California. Because additional translocations will take place in the coming years, documenting lessons from the first three years of this effort will improve success for this form of intervention not only in R. draytonii, but for other anuran species of conservation concern that straddle the international border with México.
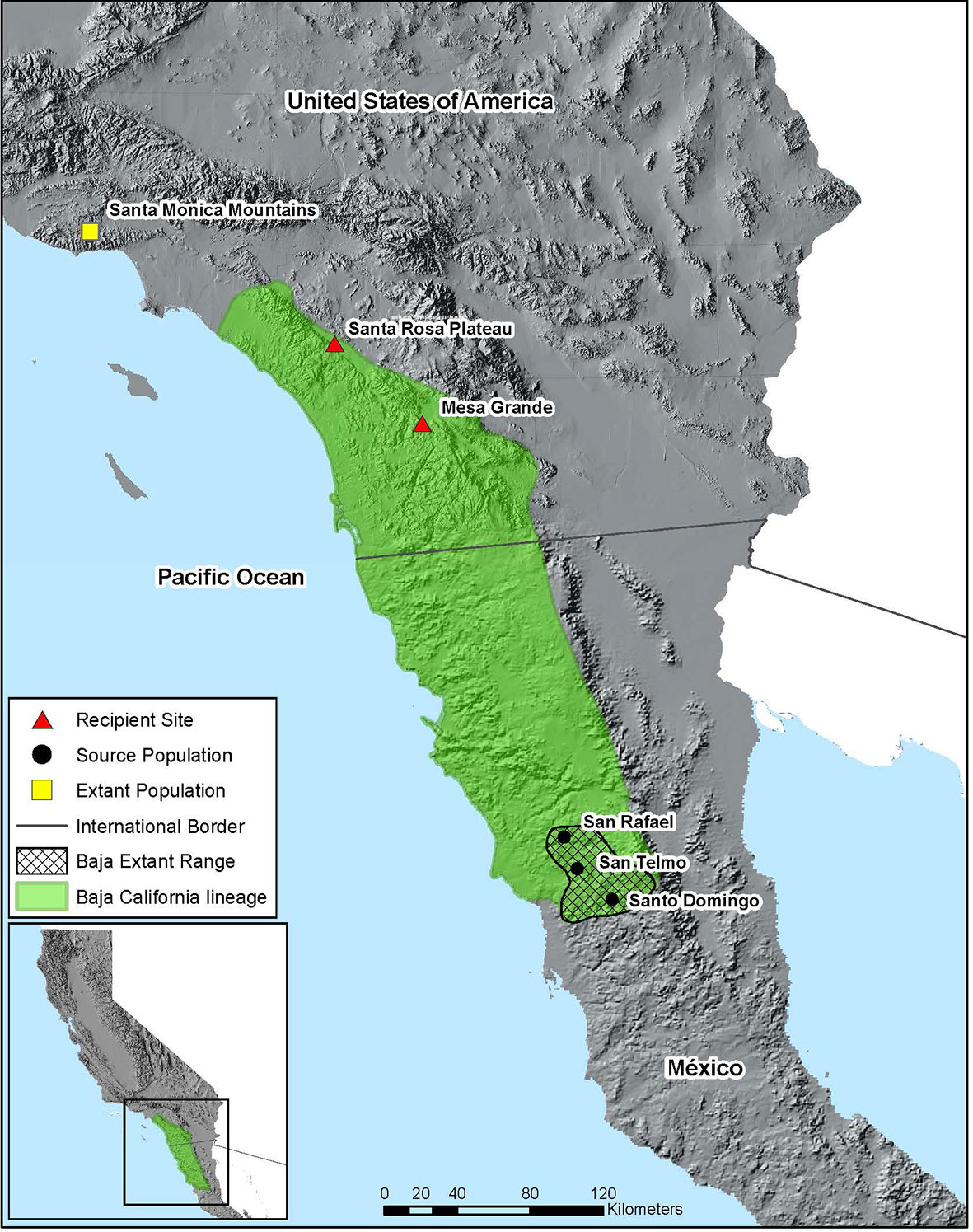
Figure 1 Map of translocation project area. Green polygon shows the approximate distribution of the Baja California lineage of R. draytonii based on available genetic data and museum specimens (records not shown). Polygon with cross-hatching shows R. draytonii occupancy in Baja California, México. Triangles represent the two recipient sites in California and circles represent the source populations in the Sierra de San Pedro Martir mountains. The single yellow square represents the most southern R. draytonii population in California (in the Santa Monica Mountains), which is the northern limit of the 420 km range gap.
2 Methods
2.1 Metrics of Success
Our team determined the following metrics for success for the project: (A) translocation of egg masses must occur at two or more recipient sites (achieved in 2020, 2021 and 2022); (B) egg masses, juveniles and adults are present at the recipient sites within six years following the initial translocation (partially achieved at both sites in 2021); and (C) population demography at recipient sites is stable or increasing at six years (although ‘stable’ in this part of the species’ range includes fluctuating demographic structure to due seasonal and multi-year climate variability (Richmond et al., 2014).
2.2 Selecting the Source Population
Determining the most suitable source population required multiple considerations, beginning with genetic data that narrowed the search to four genetic groups within the 10 extant populations in the Baja California Lineage, located in the Sierra de San Pedro Mártir mountains. Each of these populations is considered threatened due to their relatively low abundance, limited geographic reach and exposure to threats. Population surveys in 2013-14 (Peralta-García et al., 2016) and regular surveys since informed our most recent abundance estimates. We also considered other factors such as relationships with local landowners and managers, the equivalency of elevation between the candidate source and recipient sites (due to the potential for adaptive variation at different elevations), road access to occupied habitat, helicopter landing suitability, and whether habitat enhancement projects were ongoing at any specific sites. Two sites in separate watersheds in the Sierra de San Pedro Mártir were selected as suitable sources in Years 1 and 2 (hereafter ‘San Telmo’ and ‘Santo Domingo’) (Table 1). A third site in the same mountain range was added in Year 3 (hereafter ‘San Rafael’). All source sites are benefactors of ongoing habitat enhancement, relatively accessible, and have evidence of regular reproduction and recruitment.
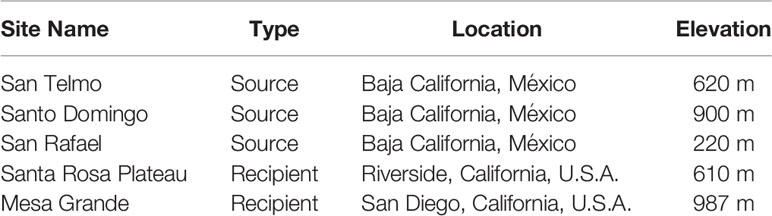
Table 1 Overview of source and recipient sites for reintroduction of the Baja California lineage of R. draytonii.
2.3 Selecting the Recipient Sites
Prioritization of recipient sites in southern California was based on land protection status, landowner interest, habitat suitability (microhabitat conditions, water availability, site elevation and natural dispersal opportunities), threat abatement, active management, and prioritization by the USFWS Recovery Plan for California Red-legged Frog (USFWS, 2002). We ultimately selected two sites, one being a known historic locality and the other within the species’ historic range but lacking verifiable records.
The known locality was the Santa Rosa Plateau in Riverside County, California, a state-designated Ecological Reserve of ~3,650 hectares supporting oak woodland, grassland, vernal pool and riparian habitats (elev. 610 m). It is currently owned by California Department of Fish and Wildlife (CDFW) and Riverside County Parks and managed by TNC. The primary historical threat to R. draytonii, the invasive American bullfrog, was eradicated after a major removal effort in the early 2000s. In addition, three ponds were artificially constructed by TNC in 2018 to provide refugia for aquatic species during extended dry periods, an issue that is predicted to worsen due to anthropogenic climate change (Polade et al., 2014).
The second recipient site is a privately-owned 162-hectare cattle ranch at Mesa Grande situated in the headwaters of a major watershed in San Diego County (elev. 987 m). This property supports grassland, chaparral, oak woodland, freshwater seeps, low growing scrub communities, and is protected in perpetuity by a Conservation Easement owned by TNC. It is managed by USFWS through a Cooperative Agreement that has led to numerous recovery actions for the benefit of aquatic species, including western pond turtle (Actinemys pallida), which is being evaluated for listing under the U.S. Endangered Species Act of 1973 (USFWS, 2015). The largest pond on the property (~4,000 m2) was drained, purged of nonnative fish, and lined with bentonite to maintain surface water, providing perennial water during drought years. Bullfrogs have been effectively eradicated. Despite eradication we continue to monitor and manage to maintain their absence. Stepwise eradication is currently being performed in the downstream direction.
2.4 Collection Parameters
Rana draytonii is a large frog with mature adults reaching up to 13 cm in length (Stebbins & McGinnis, 2012). The window of reproductive activity is relatively short at approximately two months. Eggs are laid during winter and early spring, with the onset and peak of egg production dependent on the local environmental conditions of a particular year (Alvarez et al., 2013). Sexually mature females lay a single large egg mass of 500 to 4,000 eggs each year (Stebbins & McGinnis, 2012). The large size of the egg masses makes them relatively easy to detect and distinguishable from other native anuran species.
We used egg masses for translocation to avoid problems with the homing instinct of juveniles and adults and to maximize the number of individuals transported at any one time (Gill, 1979; Reinert, 1991; Bloxam & Tonge, 1995; Rathbun and Schneider, 2001; Semlitsch, 2002; Tocher & Brown, 2004), as amphibian translocations can be mired by small sizes of newly re-established populations (Germano and Bishop, 2009). Due to a lack of keratin tissue, egg masses are not susceptible to infection by the pathogen Batrachochytrium dendrobatidis (hereafter Bd: Hyatt, 2016), which can lead to the often-fatal disease chytridiomycosis in amphibians. While the degree to which Bd has influenced mortality in R. draytonii is uncertain (but see Adams et al., 2020), there are no documented mass mortality events due to the disease in this species. Although the pathogen is present at both the donor and recipient sites (Peralta-García et al., 2018; Santos-Barrera & Peralta-García, 2018), we implemented a biosecurity protocol (Appendix 1) to minimize the potential for spread.We surveyed source populations for eggs on a weekly basis from January through April, with slight variation in the timing of when surveys began depending on the site and year. We collected half of one egg mass for every five fertile egg masses detected at each site (10% of reproductive output) to minimize demographic impacts on the sources.
To maximize the resilience of embryos during handling and transportation we considered their Gosner (1960) developmental stage when evaluating the suitability of an egg mass for translocation. Egg masses containing embryos beyond Gosner stage 20 were not considered for translocation because of the risk of premature emergence, and whenever possible we preferentially translocated those egg masses that were the earliest along in development.
Egg masses were transported in a coarse-mesh nylon bag (hereafter, transport bag) suspended in filtered pond water in a 3L plastic container. A protocol describing the egg mass detection surveys, egg harvesting and transport, and biosecurity measures is provided in Appendix I. To protect the health of personnel, field activities conducted after March 15, 2020, followed standard Covid-19 protocols.
On each day of transport, we obtained clearance to move eggs to the United States from Mexican wildlife officials (Procuraduría Federal de Protección al Ambiente; PROFEPA) in the city of Ensenada. In Years 1 and 2, egg masses were transported in modified YETI® coolers by helicopter to the Guadalupe Valley, and then transferred to a vehicle and driven to the international border in the city of Tecate. Entry into the United States was approved by the U.S. Fish and Wildlife Service and U.S. Customs and Border Protection. From the port of entry, the eggs were then driven to the recipient sites. In Year 3, to reduce uncertainty in planning for use of a helicopter, egg masses travelled exclusively by vehicle from the source sites to the release sites. Travel time was slightly shorter with the helicopter, but both took approximately 8-10 hours and were dependent on border-crossing wait times.
2.5 Headstarting and Release at Recipient Sites
We conducted ‘soft releases’ at recipient sites using in situ holding pens to support egg and tadpole development while affording protection from predators. Prior to placement in the holding pens, we rinsed each transport bag (containing each half-egg mass) three times in containers with recipient pond water to reduce the potential spread of Bd spores and allow the eggs to acclimate to the new water. After acclimating the eggs in plastic containers for 15-20 min, we suspended the transport bag inside a larger, fine-mesh nylon bag supported by a PVC pipe frame, referred to as the holding pen. Each holding pen floated at the surface of the water and was anchored seven weeks prior to translocation to allow algal growth, providing a food source for tadpoles. After hatching, the tadpoles could swim out of the transport bag and into the holding pen. We initiated supplemental feeding once algal material started to decline within the holding pens. We also measured temperature, pH, conductivity, and dissolved oxygen of the water during every site visit.
2.6 Post-Release Monitoring
Annual post-release monitoring for newly metamorphosed frogs began in August and continued through mid-November. This consisted of nighttime visual searches along the shoreline or by kayak using flashlights and headlamps. We captured frogs by hand or with dip nets and recorded life-stage, sex, weight, length and GPS location. We implanted a permanent passive integrated transponder tag (PIT-tag) into individuals with a snout-to-urostyle length of ≥45 mm, which allows us to track the age and survival of individual frogs from each cohort.
3 Results
3.1 Egg Mass Detection at Source Populations
3.1.1 San Telmo
In 2020, we conducted egg mass surveys at San Telmo (620 m) from January 28 to April 7, during which we found 20 egg masses (Figure 2). We detected most of the egg masses (n = 13) during the first week of February, with 65% located in artificial breeding ponds constructed outside of the main stream channel. In 2021, we initiated surveys one week earlier, starting on January 19, and continued until no new egg masses were detected for two consecutive surveys, with surveys ending on March 15. Far more egg masses (n = 35) were found in 2021, with all detected from January 19 to February 18; 66% were found in the artificial breeding ponds. In 2022, weekly egg mass surveys began on January 12 and continued until February 25; 43 egg masses were detected. Breeding seemed to peak both in mid-January (n = 25) and in early February (n = 11). As in previous years, the majority (63%) of egg masses were detected in the artificial breeding ponds in 2022.
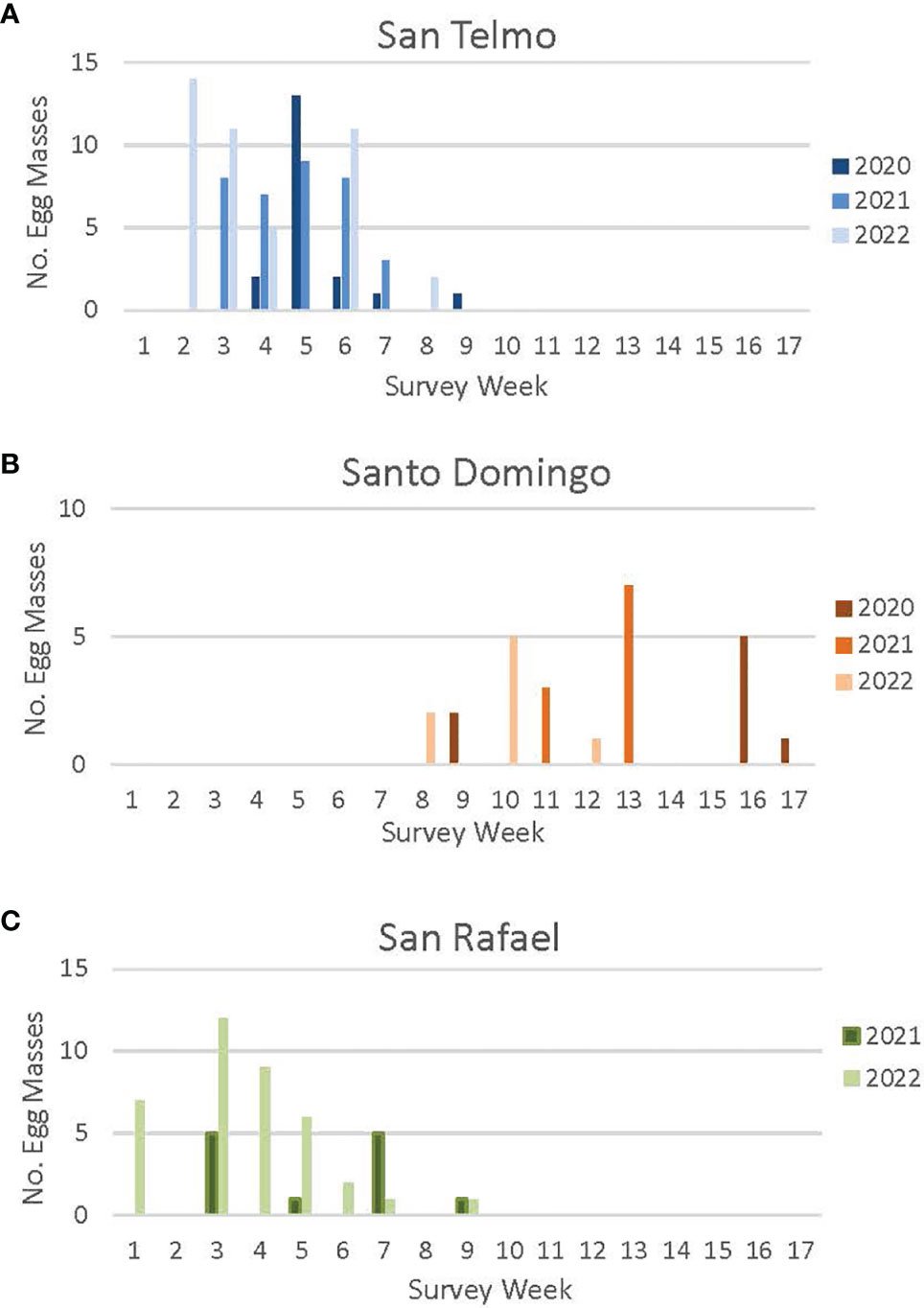
Figure 2 Egg mass detections at three R. draytonii source sites [San Telmo (A), Santo Domingo (B), San Rafael (C)] in Baja California in 2020, 2021 and 2022. Week 1 = First week of January; Week 17 = Fifth week of April. Annual surveys started earlier each year as the timing of breeding was better understood (surveys were initiated the fourth week of January in 2020, the third week of January in 2021, and the first week of January in 2022). Survey schedules varied from year to year. See Appendix II for a complete survey schedule for each site.
3.1.2 Santo Domingo
We conducted egg mass surveys at the highest elevation site, Santo Domingo (900 m), from January 28 to April 27, 2020, with a total of nine egg masses detected across the 14-week survey period (Figure 2). The first egg masses were detected in the last week of February, a one-month delay in egg detection compared to San Telmo the same year; 80% of egg masses were detected in the main stream channel and the rest in a natural oxbow pond. Fifty percent of egg masses (n = 5) were detected during the third week of April, 2020. Heavy rains across the region prevented full stream surveys at both sites from March 11 to April 13, and high flow led to a documented loss of two egg masses at Santo Domingo after the initial detections in early March.
In 2021, we started surveys at Santo Domingo one week earlier (January 19) and continued through April. We decided not to collect eggs at Santo Domingo in 2021 due to the low number of egg masses detected in 2020, and as such, transitioned to a biweekly survey schedule. All breeding detected in 2021 occurred from March 19 to April 4 (n = 10).
Biweekly surveys in 2022 started on February 25 and continued through April 19, 2022. Due to the two consecutive years of low reproductive output at Santo Domingo, we again avoided the site for egg harvest in 2022. The biweekly survey schedule allowed for accurate detection of egg masses while balancing personnel hours required for the project. However, a more labor-intensive weekly schedule is required for sites designated for egg harvest, as the eggs need to be identified for translocation at the earliest possible stage in their development as possible. Off-channel ponds constructed at Santo Domingo in 2019 have yet to be colonized by breeding adults of R. draytonii. Based on the three-year delay in use of the off-channel San Telmo ponds, we estimate the Santo Domingo ponds could be used for breeding in 2023.
3.1.3 San Rafael
Egg mass monitoring started at San Rafael in 2021 to evaluate the possibility of adding this population as a source for translocation. We conducted four biweekly surveys at this low elevation (220 m) site from January 19 to March 3, with 12 masses observed in 2021 (Figure 2). Surveys in 2022 were initiated one week earlier and conducted from January 7 to February 28 and detected over three times as many egg masses as in 2021 (n = 38). A large (500 m2) off-channel pond constructed in 2021 to provide additional breeding habitat for the species is yet to be utilized. The increase of egg masses at this site in 2022, resulted from the construction of an in-stream pond in March 2021 by local ranchers who used it as a source of irrigation water. Our experience with such ponds at other sites in México indicates that they are generally compatible with frog breeding in the short-term. Such in-stream ponds are generally colonized by frogs quickly, but they can be filled in with sediment during heavy flow events or over-utilized for agricultural irrigation. Thus, our current strategy is focused exclusively on pond construction outside of the main stream channel in order to provide long term resilience.
3.2 Egg Mass Collection and Transport
Although egg mass deposition peaked at San Telmo in the first week of February, 2020, delays in obtaining permits led to the first collections on March 13, 2020. Due to this late stage in the season, only one egg mass was available for translocation. We split this single egg mass (Gosner 20) into two halves and held them in situ until the following day, when one half was transported to Mesa Grande and the other to the Santa Rosa Plateau (site SRP 1). Embryos were hatching upon arrival, with tadpoles and remaining eggs all in good condition. Eggs mass surveys continued at Santo Domingo until April 27, when one half-mass (Gosner 18) was collected and transported to Mesa Grande the following day. Due to the earlier developmental stage of this egg mass, no eggs hatched during transport and the mass remained in good condition.
Twice as many (n = 6) egg masses were translocated in 2021 as in 2020 (n = 3), all sourced from San Telmo. We transported two egg masses per week for three consecutive weeks from late January to mid-February, with three sent to each recipient site. All egg masses collected in 2021 were at an earlier developmental stage compared to the previous year (Gosner 10 – 16). At Santa Rosa Plateau we used two separate release areas within the same creek in 2021: the same pond used in 2020 (SRP 1), and an in-stream pool located approximately 2 km away (SRP 2).
In 2022, egg mass collection increased again (n = 9) with the addition of the new source, San Rafael, which supplied one egg mass to each recipient site. The timing of breeding mirrored the previous year, facilitating the collection of all nine egg masses in just two transport events during the final two weeks of January. Four egg masses (Gosner 9 – 14) were sent to Mesa Grande, and 5 were sent to the Santa Rosa Plateau (SRP 1). An overview of which source and recipient site were used each year is shown in Table 2.
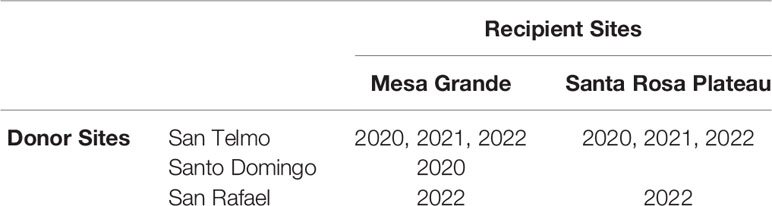
Table 2 Account of years that source and recipient sites were used in translocation of R. draytonii Baja California lineage.
3.3 Headstarting and Release at Recipient Sites
We conducted two translocations in 2020, one in March and one in April. Following the first translocation on March 14, 2020, we conducted seven weekly welfare checks until May 12. After nearly two months of healthy growth in the holding pens, we observed a decline in the number of tadpoles at both sites within a week’s time and decided to release those that remained. Decline was more extreme at the Santa Rosa Plateau; however neither site had significant changes or abnormalities in water quality or temperature. Analysis of the mortality at the Santa Rosa Plateau by USGS National Wildlife Health Center did not establish a cause of death. This initial translocation resulted in the release of 17 and three tadpoles at Mesa Grande and Santa Rosa Plateau, respectively, in 2020 (Table 3).
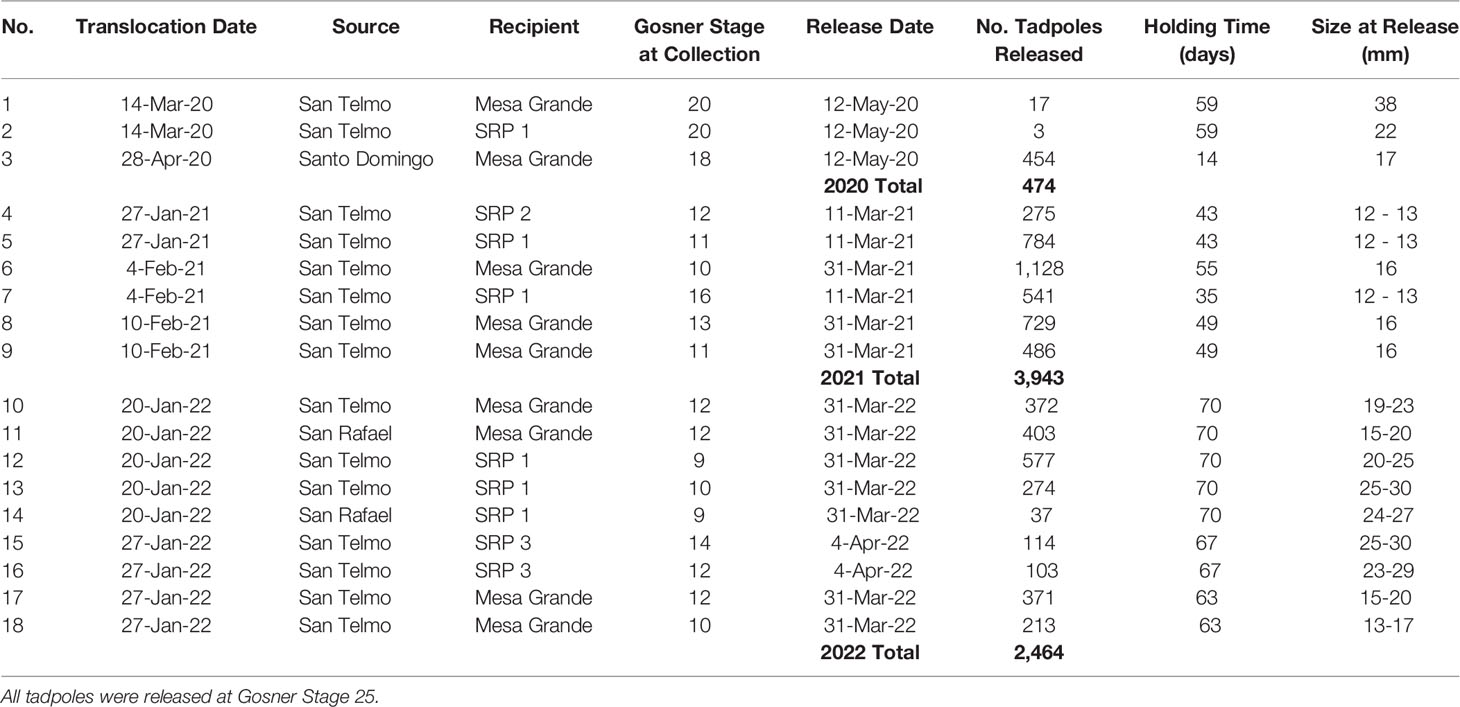
Table 3 Headstarting results for 18 R. draytonii half-egg masses translocated from Baja California, México, to California, U.S.A. (2020 – 2022).
Following the second translocation to Mesa Grande on April 28, 2020, we conducted three welfare checks between April 30 and the release date on May 12. This second translocation in 2020 resulted in the release of 454 tadpoles, all in good condition and with little mortality. We released the tadpoles in this group after 14 days (17 mm) to avert potential mortality as seen with the first two egg masses (released at 38 mm and 22 mm, respectively).
In 2021, we conducted daily welfare checks of egg masses at Mesa Grande and Santa Rosa Plateau until their release on two dates in March. Approximately 1,600 tadpoles (12-13 mm) were released at the Santa Rosa Plateau after 35 – 43 days of headstarting (SRP 1: n = 1,325; SRP 2: n = 275). At Mesa Grande, about 2,343 tadpoles (16 mm) were released after 49 – 55 days of headstarting. We again released tadpoles earlier and at a smaller size compared to the previous year to avoid potential mortality associated with penned tadpoles at larger sizes.
Following the 2022 translocations, we monitored egg masses twice per week from January 22 until April 4, 2022. After 63-70 days, about 2,464 tadpoles (15-30 mm) were released from the holding pens at Mesa Grande. At Santa Rosa Plateau, we released a total of 1,105 tadpoles in 2022. Three of five egg masses in SRP 1 were released into that pond after 70 days (n = 888; 20-30 mm). The remaining two egg masses (n = 217; 23-30 mm) were transported from SRP 1 to a third site (SRP 3), where they were released in an off-channel pond constructed and supplied with well water to provide drought refugium. SRP 3 is hydrologically connected to and located between SRP 1 and SRP 2. A total of 1,359 tadpoles were released to Mesa Grande and Santa Rosa Plateau in 2022.
Starting in Year 2 (2021), we removed the floating PVC frame and stabilized the holding pens by securing them to t-posts staked to the bottom of the pond (Figure 3). This modified, hammock-like design allowed us to monitor the eggs more efficiently and adjust the height of the eggs in the water column if necessary. We also eliminated the seven-week advance placement of the holding pen to reduce the possibility of damselfly larvae colonization.
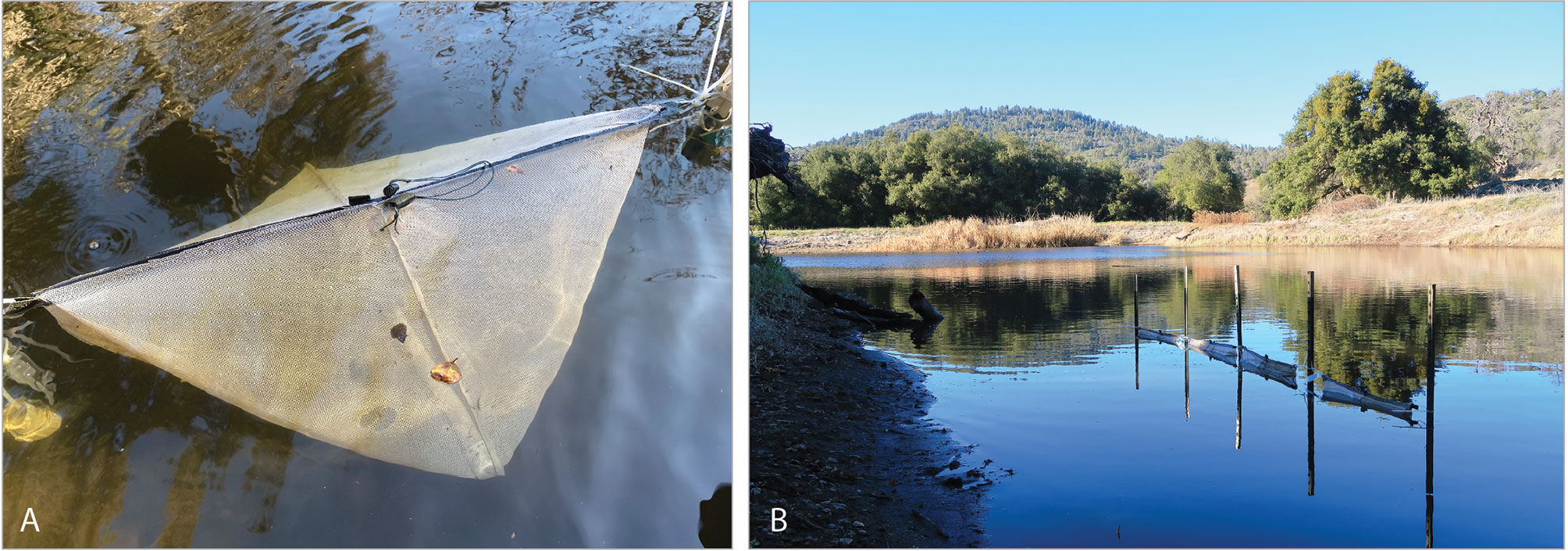
Figure 3 (A) Example of the modified egg mass holding pen. (B) Example of consecutive holding pens (n = 4) attached to posts.
In Year 1, we examined the eggs/tadpoles two days after the translocation, and then approximately every seven days thereafter. In Year 2, we monitored the egg masses daily, and in Year 3 we monitored twice per week. Tadpoles were released from their holding pens with a target size of 35-40 mm in length and Gosner stage 25, which was later revised to 15 mm and Gosner 25.
3.4 Post-Release Monitoring
Three surveys at Mesa Grande conducted between September and November 2020 detected 71 newly metamorphosed frogs, of which 18 were large enough to PIT-tag. Three surveys at Mesa Grande in September and October 2021 detected 13 adults and 8 subadults.
We conducted two surveys targeting subadults at the Santa Rosa Plateau on August 27 and September 22, 2020, but did not detect any frogs. Five surveys conducted from July to October, 2021, detected 25 subadults and one adult frog at the Santa Rosa Plateau, proving some survivorship of the 2020 cohort that experienced the die-off. During this period, water levels at SRP 1 and SRP 2 diminished approximately 90% from January to October due to below average rainfall in 2021.
4 Discussion
Translocations of amphibian species have a mixed record of success, in part because each life-history phase is subject to unique threats to survival (Germano and Bishop, 2009; Scheele et al., 2021). Success can also be affected by environmental gradients that impose different constraints on population establishment and survivorship in different parts of the range. These factors can lead to unanticipated challenges in the early stages of the process, yet the knowledge gained through these ‘growing pains’ are key to refining techniques and ultimately improving success. Below, we draw attention to early challenges for this project that are likely to extend to other amphibian species, describe modifications to the initial protocol to mitigate them, and highlight results that demonstrate the effort is on a positive trajectory.
4.1 Refining Translocation Procedures
The biggest challenges to this project have involved uncertainty of the weather, timing of breeding at different elevations, and travel restrictions due to the Covid-19 pandemic. As shifting circumstances closed our window of opportunity for the initial translocation in 2020, we were left with only two late-season egg masses that were slightly beyond the preferred developmental stage for movement (Gosner 20). We proceeded with the translocation because it was agreed that this would be the last opportunity to do so in 2020, and at that point there was no compelling reason to believe that the slightly older egg masses would jeopardize success.
A definitive cause for the higher-than-anticipated and rapid onset of mortality of the first egg masses is unknown. Possibilities include the late stage of egg development at the time of translocation (Gosner 20 vs. 9-18 for subsequent translocations), excessive duration of in situ rearing (59 days vs. 14-49 days), inability of the tadpoles to properly thermoregulate in the water column while in the holding pen, presence of damselfly larvae in the holding pens (i.e., predation), and/or some unknown environmental stressor. Tadpole growth, behavior, and survivorship is affected by temperature (Goldstein et al., 2017), although in this case water temperature and quality (unpublished data) showed no striking fluctuation or anomalies prior to or during the mortality event.
We modified the following procedures to address several factors: 1) timing of egg mass surveys; 2) target lifestage for egg harvest; 3) design of holding pens; 4) advanced placement of holding pens; 5) monitoring frequency during headstarting; and 6) tadpole size at release. After gaining insight on breeding phenology over successive years, we found that egg mass surveys are best initiated in early January at the low elevation sites (San Rafael and San Telmo), and in March at the higher elevation site (Santo Domingo) due to the influence of temperature on breeding.
We are now targeting Gosner stage 14 or lower as the optimum stage for harvest, and stage 18 as the upper limit based on success of the second translocation in 2020. This approach increases the overall acclimatization time to the recipient environment by exposing embryos to the new conditions at an earlier stage of development, providing greater opportunity for their genotypes to respond to any novel stimuli. This may be important for translocations involving large translocation distances, where local environments at the source and recipient sites are more likely to differ than they would over shorter distances (Marshall et al., 2010). The ability to produce different phenotypes from the same genotype (Schlichting, 1989; Nicotra et al., 2010), or phenotypic plasticity, may therefore facilitate a better phenotype-environment match at recipient sites if the eggs are translocated earlier in development, and ultimately improve survivorship. Realistically, collecting at the targeted Gosner stage is constrained by year-to-year variation in the timing of egg laying, the influence of temperature on development, undetected egg masses, and the ability to quickly mobilize a complex cross-border operation.
Modification of the holding pen design has increased monitoring ease and efficiency. To avoid colonization of damselfly larvae in the holding pens (leading to potential predation), they are no longer set up in advance of a translocation event and we manually remove any damselfly larvae throughout headstarting. The frequency of egg mass and tadpole monitoring varied from year to year (2020: weekly; 2021: daily; 2022: twice weekly) until we struck a balance between minimizing disturbance and monitoring frequently enough to detect any problems as early as possible (e.g., mold growth, mortality, predation). Lastly, to reduce any potential risk associated with excessive time in the holding pens, the minimum size for release was decreased from 35-40 mm to 15 mm and Gosner 25, which gives the tadpoles more opportunity to self-regulate their feeding and preferred temperature.
High mortality is expected for wild (i.e., non-headstarted) larvae. For example, natural survivorship from egg to subadult in a population of R. aurora was reported in the literature as 5% (Calef, 1973). Thus, detection of subadults and adults at both recipient sites is an encouraging sign for population establishment. Precise estimates of recruitment were difficult to obtain because we were limited to PIT-tagging individuals greater than ≥45 mm snout-to-urostyle, an issue that will ameliorate as more frogs grow to larger sizes. Nonetheless, the number of translocated egg masses increased with each year (2020 = 3 egg masses/474 tadpoles; 2021 = 6 egg masses/3,951 tadpoles; 2022 = 9 egg masses/2,464 tadpoles), suggesting that modifications to the original protocol are working and active management at the source sites is promoting breeding.
4.2 Binational Translocation Planning
In California, at least 40 species listed under the U.S. Federal Endangered Species Act of 1973 have ranges that extend into Baja California, but cooperative binational recovery planning can be constrained by several complex factors (distinct environmental regulations, unique permitting processes, spending restrictions associated with public funding, differing degrees of land protection and ecological management norms, socio-economic circumstances on the ground, and language barriers). Yet, translocation projects across the US-México border are not unprecedented (e.g., California condor (Gymnogyps californianus) (Walters et al., 2010); Tarahumara frog (Rana tarahumarae) (Rorabaugh et al., 2020); Mexican wolf (Canis lupus baileyi) (Parsons, 1998); multiple subspecies of pronghorn antelope (Antilocapra americana) (Ramirez et al., 1999); and black-tailed prairie dog (Cynomys ludovicianus) (Hale et al., 2013). This project distinguishes itself in that it was planned, permitted, and initiated in less than one year, suggesting that expediency for this and other international recovery efforts will continue to improve as successes become more frequent.
The complexity of translocation planning can be untangled by undertaking the systematic process described by the IUCN (2013); we offer a flowchart for the process undertaken here (Figure 4). Identification of binational partners with clear roles was a major catalyst for success. Translocation projects can be stalled during negotiations with landowners and in procuring permits from both countries (see Acknowledgements for a list of required permits), a challenge we mitigated by streamlining the process over time and establishing a rapport with the regulatory agencies involved with permitting. We also prioritized financial and technical support of FAUNO to ensure continued cooperation with local ranchers in México in managing threats and providing long-term resilience of aquatic habitat. Persistence of frogs in the Sierra de San Pedro Mártir is far from guaranteed, as increased groundwater extraction and surface diversions for agriculture are ongoing threats that are exacerbated by invasive species occupancy and aridification. Future plans within México include additional support from U.S. collaborators, continued habitat enhancement, and in-country translocation to add resilience to the few remaining populations.
4.3 Future Directions
Successful translocations for Rana draytonii have occurred in different parts of the species’ range, covering a breadth of habitat/environments at different latitudes. This effort represents the first conducted across an international border at the ‘warm edge’ of the range, where the species ecology is very different than in the cooler parts of the range in central coastal and northern California. Inland and upland suitable habitat in southern California and northern Baja California is more ephemeral, and perennial water sources occur mainly in the form of seeps and springs (Levick et al., 2008; Hershkovitz and Gasith, 2013). Ponds, lakes and reservoirs are not a part of the natural landscape (Stephenson and Calcarone, 1999), and nonnative invasive species are a persistent problem in these habitats (Moyle and Marchetti, 2006). Presence of permanent or near permanent water is a requirement for R. draytonii. Therefore, considerable challenges exist for identifying stable recipient sites that can support the species in this part of the range due to climate change, which is predicted to increase the duration, frequency and intensity of droughts and heat waves in the region (Kalansky et al., 2018).
We will continue to prioritize source and recipient sites where management can proactively provide aquatic refugia under more extreme climatic conditions, such as the newly constructed artificial ponds supplied with well water at the Santa Rosa Plateau and those constructed at source populations in Baja California. Artificial ponds can provide security for aquatic species, including access to water for temperature regulation, predation avoidance, foraging and breeding, although care must be taken to ensure that nonnative species do not colonize these sites.
We expect that propagule pressure will be key to population establishment, as it takes time to reach stable predatory-prey relationships and some critical mass of mature frogs that are ready to breed (Lockwood et al., 2005; Gillespie et al., 2012). Hossack et al. (2022) evaluated 25 years of survey and translocation data for the Chiricahua leopard frog (Rana chiricahuensis) and found an increased probability of site occupancy and years of population persistence with more translocation events. With these points in mind, our team has agreed to conduct translocations for an additional three years, and we anticipate that breeding at recipient sites will begin in 2023-2024. Once established (i.e., reproducing), we will monitor the genetic health of translocated populations by comparing them to source populations to measure genetic diversity and levels of inbreeding between sites.
We intend to use the two U.S. populations as sources for translocations to new sites that are currently under consideration. To the extent possible, future reintroduction sites will be clustered in close proximity to increase the probability of natural recolonization by neighboring populations (Hossack et al., 2022). More fine-scale resiliency of re-established populations may be generated by attempting to restore metapopulation structure, with the goal of promoting natural dispersal among populations within the same watershed (and where possible, between watersheds). Ideally, our goal is to re-create self-sustaining metapopulations that require minimal or no human intervention for survival.
Berger-Tal et al. (2020) emphasize that outcomes for translocation interventions can be improved by accounting for taxon-specific behavioral traits and life history. We add that success also requires different considerations depending on geography, particularly for species like R. draytonii that are distributed across a broad latitudinal gradient. If translocation is to be used as a staple method for reversing population declines, success will likely require a geographically informed protocol based on experimentation before it can be put to effective use in certain parts of a species’ range.
Data Availability Statement
The raw data supporting the conclusions of this article will be made available by the authors, without undue reservation.
Ethics Statement
Ethical review and approval was not required for the animal study because the following permits did not require such a review: México Scientific Collection Permit (SGPA/DGVS/11447/19; 03618/20; 05807/21), México Export Permit (No. 47442; 48269; 49510), issued by Secretaría del Medio Ambiente y Recursos Naturales. Registros de Verificación PFPA/110/00888/2020,/01229/2020; 0259/2021, 0421/2021, 0527/2021; 400/2022, 495/2022) issued by Procuraduría Federal de Protección al Ambiente. U.S. Import Permit (MA63444D-0), and a California Department of Fish and Wildlife Scientific Collecting Permit (SCP) 838 and a federal (Section 10(a)1(A)) Recovery Permit (TE-045994) for R. draytonii issued to the USGS Western Ecological Research Center. This project was conducted in compliance with the following California of Fish and Wildlife (CDFW) policies: Department Bulletin 2017-04 (Captive Propagation of Fish, Wildlife and Plants for Conservation Purposes) and 2017-05 (Policy and Procedures for Conservation Translocations of Animals and Plants). None of the above permits required an ethical review for this project, which was conducted specifically to aid in recovery of the species.
Author Contributions
All authors listed have made a substantial, direct, and intellectual contribution to the work and approved it for publication.
Funding
Financial support for this project was provided by in-kind contributions from all partners, and funding contributions from the following: The Nature Conservancy (2021 Grant Agreement 04062020-14297, 2022 Grant Agreement 0917202115206), U.S. Fish and Wildlife Service Ecological Services Program, U.S. Fish and Wildlife Service Carlsbad Field Office, U.S. Fish and Wildlife Service Partners for Fish and Wildlife Program, San Diego Association of Governments Transnet funding, Dennis and Carol Wilson, William Cole Foundation, The Hervey Family Fund at The San Diego Foundation, and Evolutionary Venture Fund at the San Diego Natural History Museum.
Conflict of Interest
The authors declare that the research was conducted in the absence of any commercial or financial relationships that could be construed as a potential conflict of interest.
Publisher’s Note
All claims expressed in this article are solely those of the authors and do not necessarily represent those of their affiliated organizations, or those of the publisher, the editors and the reviewers. Any product that may be evaluated in this article, or claim that may be made by its manufacturer, is not guaranteed or endorsed by the publisher.
Acknowledgments
All translocations were performed in accordance with the following permits: México Scientific Collection Permit (SGPA/DGVS/11447/19; 03618/20; 05807/21), México Export Permit (No. 47442; 48269; 49510), issued by Secretaría del Medio Ambiente y Recursos Naturales. Registros de Verificación PFPA/110/00888/2020,/01229/2020; 0259/2021, 0421/2021, 0527/2021; 400/2022, 495/2022) issued by Procuraduría Federal de Protección al Ambiente. U.S. Import Permit (MA63444D-0), and a California Department of Fish and Wildlife Scientific Collecting Permit (SCP) 838 and a federal (Section 10(a)1(A)) Recovery Permit (TE-045994) for R. draytonii issued to the USGS Western Ecological Research Center. This project was conducted in compliance with the following California of Fish and Wildlife (CDFW) policies: Department Bulletin 2017-04 (Captive Propagation of Fish, Wildlife and Plants for Conservation Purposes) and 2017-05 (Policy and Procedures for Conservation Translocations of Animals and Plants). Thank you to the ranchers in Baja California who provided access to their land and supported the recovery of R. draytonii. Thank you to YETI for donating the transport coolers. This is contribution number 812 of the USGS Amphibian Research and Monitoring Initiative (ARMI). Any use of trade, firm, or product names is for descriptive purposes only and does not imply endorsement by the U.S. Government.
Supplementary Material
The Supplementary Material for this article can be found online at: https://www.frontiersin.org/articles/10.3389/fcosc.2022.908929/full#supplementary-material
References
Adams A. J., Pessier A. P., Briggs C. J. (2017). Rapid Extirpation of a North American Frog Coincides With an Increase in Fungal Pathogen Prevalence: Historical Analysis and Implications for Reintroduction. Ecol. Evol. 7 (23), 10216–10232. doi: 10.1002/ece3.3468
Adams A. J., Pessier A. P., Cranston P., Grasso R. L. (2020). Chytridiomycosis-Induced Mortality in a Threatened Anuran. PloS One 15, e0241119. doi: 10.1371/journal.pone.0241119
Alvarez J. A., Cook D. G., Yee J. L., Van Hattem M. G., Fong D. R., Fisher R. N. (2013). Comparative Microhabitat Characteristics at Oviposition Sites of the California Red-Legged Frog. Herpetolog. Conserv. Biol. 8 (3), 539–551.
Backlin A. R., Richmond J. Q., Gallegos E. A., Christensen C. K., Fisher R. N. (2018). An Extirpated Lineage of a Threatened Frog Species Resurfaces in Southern California. Oryx 52 (4), 718–722. doi: 10.1017/S0030605317001168
Berger-Tal O., Blumstein D. T., Swaisgood R. R. (2020). Conservation Translocations: A Review of Common Difficulties and Promising Directions. Anim. Conserv. 23, 121–131. doi: 10.1111/acv.12534
Bloxam Q. C., Tonge S. J. (1995). Amphibians: Suitable Candidates for Breeding-Release Programmes. Biodivers. Conserv. 4, 636–644. doi: 10.1007/BF00222519
Calef G. W. (1973). Natural Mortality of Tadpoles in a Population of Rana aurora. Ecology 54, 741–758. doi: 10.2307/1935670
Dodd C. K. Jr., Seigel R. A. (1991). Relocation, Repatriation, and Translocation of Amphibians and Reptiles: Are They Conservation Strategies That Work? Herpetologica 47, 336–350.
Fischer J., Lindenmayer D. B. (2000). An Assessment of the Published Results of Animal Relocations. Biol. Conserv. 96 (1), 1–11. doi: 10.1016/S0006-3207(00)00048-3
Germano J. M., Bishop P. J. (2009). Suitability of Amphibians and Reptiles for Translocation. Conserv. Biol. 23, 7–15. doi: 10.1111/j.1523-1739.2008.01123.x
Gill D. E. (1979). Density Dependence and Homing Behavior in Adult Red-Spotted Newts (Notophthalmus viridescens) (Rafinesque). Ecology 60, 800–813. doi: 10.2307/1936616
Gillespie R. G., Baldwin B. G., Waters J. M., Fraser C. I., Nikula R., Roderick G. K. (2012). Long-Distance Dispersal: A Framework for Hypothesis Testing. Trends Ecol. Evol. 27, 47–56. doi: 10.1016/j.tree.2011.08.009
Goldstein J. A., Hoff K. V. S., Hillyard S. D. (2017). The Effect of Temperature on Development and Behaviour of Relict Leopard Frog Tadpoles. Conserv. Physiol. 5, cow075. doi: 10.1093/conphys/cow075
Gosner K. L. (1960). A Simple Table for Staging Anuran Embryos and Larvae With Notes on Identification. Herpetologica 16 (3), 183–190. doi: 10.2307/3890061
Hale S. L., Koprowski J. L., Hicks H. (2013). “Review of Black-Tailed Prairie Dog Reintroduction Strategies and Site Selection: Arizona Reintroduction,” in Merging Science and Management in a Rapidly Changing World: Biodiversity and Management of the Madrean Archipelago III and 7th Conference on Research and Resource Management in the Southwestern Deserts; 2012 May 1-5; Tucson, AZ. Proceedings. RMRS-P-67. Eds. Gottfried G. J., Ffolliott P. F., Gebow B. S., Eskew L. G., Collins L. C. (Fort Collins, Colorado: U.S. Department of Agriculture, Forest Service, Rocky Mountain Research Station), 310–315.
Hayward M. W., Slotow R. (2016). “Management of Reintroduced Wildlife Populations,” in Reintroduction of Fish and Wildlife Populations. Eds. Jachowski D. S., Millspaugh J. J., Angermeier P. L., Slotow R. (Oakland, California: University of California Press), 319–340.
Hershkovitz Y., Gasith A. (2013). Resistance, Resilience, and Community Dynamics in Mediterranean-Climate Streams. Hydrobiologia 719, 59–75. doi: 10.1007/s10750-012-1387-3
Hossack B. R., Howell P. E., Owens A. K., Cobos C., Goldberg C. S., Hall D., et al. (2022). Identifying Factors Links With Persistence of Reintroduced Populations: Lessons Learned From 25 Years of Amphibian Translocations. Global Ecol. Conserv. 25, e02078. doi: 10.1016/j.gecco.2022.e02078
Hyatt A. D. (2016). Infection With Batrachochytrium dendrobatidis. Manual of Diagnostic Tests for Aquatic Animals (Paris, France: World Organisation for Animal Health (OIE).
International Union for Conservation of Nature Species Survival Group (IUCN/SCC) (2013). Guidelines for Reintroductions and Other Conservation Translocations. Version 1.0 (Gland, Switzerland: IUCN Species Survival Commission), viiii–57 pp.
Kalansky J., Cayan D., Barba K., Walsh L., Brouwer K., Boudreau D. (2018). “San Diego Summary Report,” in California’s Fourth Climate Change Assessment (San Diego, California: University of California, San Diego), 114.
Levick L. R., Goodrich D. C., Hernandez M., Fonseca J., Semmens D. J., Stromberg J. C., et al. (2008). “The Ecological and Hydrological Significance of Ephemeral and Intermittent Streams in the Arid and Semi-Arid American Southwest. U.S,” in Environmental Protection Agency, Office of Research and Development and USDA/ARS Southwest Watershed Research Center (Tucson, Arizona; U.S: Environmental Protection Agency and USDA/ARS Southwest Watershed Research Center), pp 116.
Linhoff L. J., Soorae P. S., Harding G., Donnelly M. A., Germano J. M., , Hunter, et al. (2021). IUCN Guidelines for Amphibian Reintroductions and Other Conservation Translocations. 1st ed. (Gland, Switzerland: IUCN).
Lockwood J. L., Cassey P., Blackburn T. (2005). The Role of Propagule Pressure in Explaining Species Invasions. Trends Ecol. Evol. 20, 223–228. doi: 10.1016/j.tree.2005.02.004
Marshall D. J., Monro K., Bode M., Keough M. J., Swearer S. (2010). Phenotype–environment Mismatches Reduce Connectivity in the Sea. Ecol. Lett. 13 (1), 128–140. doi: 10.1111/j.1461-0248.2009.01408.x
Moyle P. B., Marchetti M. P. (2006). Predicting Invasion Success: Freshwater Fishes in California as a Model. Trends Ecol. Evol. 56, 515–524. doi: 10.1641/0006-3568(2006)56[515:PISFFI]2.0.CO;2
Nicotra A. B., Atkin O. K., Bonser S. P., Davidson A. M., Finnegan E. J., Mathesius U., et al. (2010). Plant Phenotypic Plasticity in a Changing Climate. Trends Plant Sci. 15 (12), 684–692. doi: 10.1016/j.tplants.2010.09.008
Parsons A. (1998). “Green Fire” Returns to the Southwest: Reintroduction of the Mexican Wolf. Wildlife Society Bulletin 26 (4), 799–807.
Peralta García A. (2017). “Distribucioín, Amenazas Y Estructura Geneítica De La Rana De Patas Rojas (Rana draytonii) En Baja California,” in PhD. Dissertation (La Paz, Baja California Sur: Centro de Investigaciones Biológicas del Noroeste, Baja California, México), 154 pp. doi: 10.3354/dao03202
Peralta-García A., Adams A. J., Galina-Tessaro P., Briggs C. J., Valdez-Villavicencio J. H., Hollingsworth B., et al. (2018). Occurrence of Batrachochytrium dendrobatidis in Anurans of the Mediterranean Region of Baja California, México. Dis. Aquat. Organisms 127193–200.
Peralta-García A., Hollingsworth B. D., Valdez-Villavicencio J. H., Richmond J. Q., Ruiz-Campos G., Fisher R. N., et al. (2016). Population Status of the California Red-Legged Frog (Rana draytonii) in Baja California, México. Herpetological Conserv. Biol. 11 (1), 168–180.
Polade S. D., Pierce D. W., Cayan D. R., Gershunov A., Dettinger M. D. (2014). The Key Role of Dry Days in Changing Regional Climate and Precipitation Regimes. Sci. Rep. 4, 4364. doi: 10.1038/srep04364
Ramirez F., Cancino J., Reyes J. M., Sanchez-Sotomayor V. (1999). Collaborative Programs for México and the United States to Enhance Pronghorn: A Review. Pronghorn Antelope Workshop 18, 74–80.
Rathbun G. B., Schneider J. (2001). Translocation of California Red-Legged Frogs (Rana aurora draytonii). Wildlife Soc. Bull. 29, 1300–1303.
Reinert H. K. (1991). Translocation as a Conservation Strategy for Amphibians and Reptiles: Some Comments, Concerns, and Observations. Herpetologica 47, 357–363.
Richmond J. Q., Backlin A. R., Tatarian P. J., Solvesky B. G., Fisher R. N. (2014). Population Declines Lead to Replicate Patterns of Internal Range Structure at the Tips of the Distribution of the California Red-Legged Frog (Rana draytonii). Biol. Conserv. 172, 128–137. doi: 10.1016/j.biocon.2014.02.026
Richmond J. Q., Barr K. R., Backlin A. R., Vandergast A. G., Fisher R. N. (2013). Evolutionary Dynamics of a Rapidly Receding Southern Range Boundary in the Threatened California Red-Legged Frog (Rana draytonii). Evolutionary Appl. 6 (5), 808–822. doi: 10.1111/eva.12067
Rorabaugh J. C., Owens A. K., King A., Hale S. F., Poulin S., Sredl M. J., et al. (2020). Reintroduction of the Tarahumara Frog (Rana tarahumarae) in Arizona: Lessons Learned. Herpetological Conserv. Biol. 15 (2), 372–389.
Santos-Barrera G., Peralta-García A. (2018). Earliest Record of Batrachochytrium dendrobatidis in Amphibian Populations of Baja California, México. Herpetolog. Rev. 49 (4), 689–691.
Scheele B. C., Hollanders M., Hoffmann E. P., Newell D. A., Lindenmayer D. B., , McFadden, et al. (2021). “Conservation Translocations for Amphibian Species Threatened by Chytrid Fungus: A Review, Conceptual Framework, and Recommendations,” in Conservation Science and Practice 3 (11), e524. doi: 10.1111/csp2.524
Schlichting C. D. (1989). Phenotypic Integration and Environmental Changes. Bioscience 39 (7), 460–464. doi: 10.2307/1311138
Seddon P. J., Griffiths C. J., Soorae P. S., Armstrong D. P. (2014). Reversing Defaunation: Restoring Species in a Changing World. Science 345, 406–412. doi: 10.1126/science.1251818
Semlitsch R. (2002). Critical Elements for Biological Based Recovery Plans of Aquatic-Breeding Amphibians. Conserv. Biol. 16, 619–629. doi: 10.1046/j.1523-1739.2002.00512.x
Shaffer H. B., Fellers G. M., Voss S. R., Oliver J. C., Pauly G. B. (2004). Species Boundaries, Phylogeography and Conservation Genetics of the Red-Legged Frog (Rana aurora/draytonii) Complex. Mol. Ecol. 13, 2667–2677. doi: 10.1111/j.1365-294X.2004.02285.x
Stebbins R. C., McGinnis J. (2012). Field Guide to the Amphibians and Reptiles of California (Berkeley, California, USA: University of California Press).
Stephenson J. R., Calcarone G. M. (1999). Southern California Mountains and Foothills Assessment: Habitat and Species Conservation Issues (No. Gen. Tech. Rep. GTR-PSW-172) (Albany, CA: U.S. Department of Agriculture, Forest Service, Pacific Southwest Research Station).
Thompson R. C., Wright A. N., Shaffer H. B. (2016). California Amphibian and Reptile Species of Special Concern (Oakland California: California Department of Fish and Wildlife and University of California Press).
USFWS (United States Fish and Wildlife Service) (1996). “Endangered and Threatened Wildlife and Plants: Determination of Threatened Status for the California Red-Legged Frog Rana Draytonii,” in Federal Registrar, vol. 61 (101), 25813–25833.
USFWS (U.S. Fish and Wildlife Service). (2002). “Recovery Plan for the California Red-Legged Frog (Rana aurora draytonii),” in U.S. Fish and Wildlife Service, Portland, Oregon, USA (Portland, Oregon U.S: Fish and Wildlife Service), 173.
USFWS (U.S. Fish and Wildlife Service). (2015). Endangered and Threatened Wildlife and Plants; 90-Day Findings on 10 Petitions. Fed. Regist. 80, 19259–19263.
Wake D. B., Vredenburg V. T. (2008). Are We in the Midst of a Sixth Mass Extinction? A View From the World of Amphibians. Proc. Natl. Acad. Sci. 105, 11466–11473. doi: 10.1073/pnas.0801921105
Keywords: translocation, amphibian, headstart, international, reintroduction, recovery
Citation: North S, Richmond JQ, Santana FE, Peralta-García A, Gallegos EA, Backlin AR, Hitchcock CJ, Hollingsworth BD, Valdez-Villavicencio JH, Principe Z, Fisher RN and Winchell CS (2022) Taking the Leap: A Binational Translocation Effort to Close the 420-Km Gap in the Baja California Lineage of the California Red-Legged Frog (Rana draytonii). Front. Conserv. Sci. 3:908929. doi: 10.3389/fcosc.2022.908929
Received: 31 March 2022; Accepted: 02 May 2022;
Published: 02 June 2022.
Edited by:
Doug P. Armstrong, Massey University, New ZealandReviewed by:
Qiang Dai, Chengdu Institute of Biology (CAS), ChinaNicola J. Van Wilgen, South African National Parks, South Africa
Copyright © 2022 North, Richmond, Santana, Peralta-García, Gallegos, Backlin, Hitchcock, Hollingsworth, Valdez-Villavicencio, Principe, Fisher and Winchell. This is an open-access article distributed under the terms of the Creative Commons Attribution License (CC BY). The use, distribution or reproduction in other forums is permitted, provided the original author(s) and the copyright owner(s) are credited and that the original publication in this journal is cited, in accordance with accepted academic practice. No use, distribution or reproduction is permitted which does not comply with these terms.
*Correspondence: Susan North, c3VzYW4ubm9ydGhAdG5jLm9yZw==