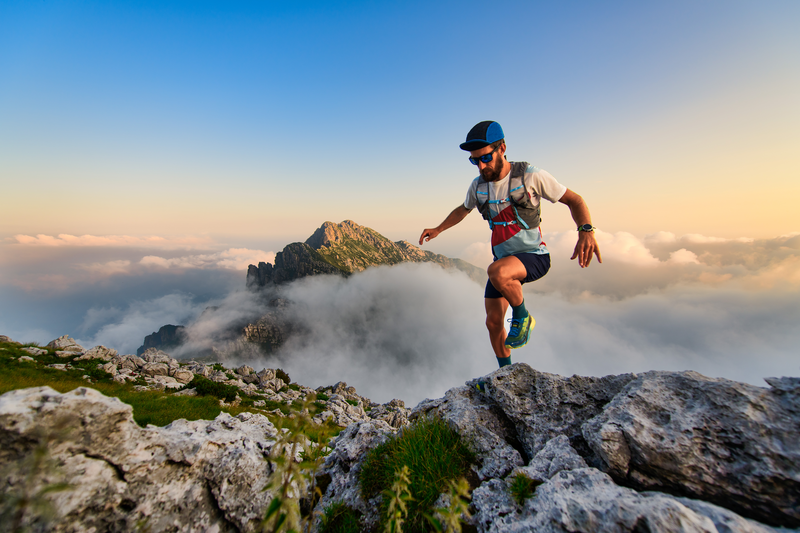
94% of researchers rate our articles as excellent or good
Learn more about the work of our research integrity team to safeguard the quality of each article we publish.
Find out more
ORIGINAL RESEARCH article
Front. Conserv. Sci. , 17 June 2022
Sec. Human-Wildlife Interactions
Volume 3 - 2022 | https://doi.org/10.3389/fcosc.2022.850309
The impact of humans on biodiversity, in the form of the spatially extensive occurrence of humans and subsequent habitat degradation, leads to negative interactions between humans and native wildlife. However, knowledge of the spatial and temporal interface between humans and wildlife is necessary to understand the root cause of such negative interactions, yet considerably understudied in the context of human-dominated landscapes in south and south-eastern Asia. We took this opportunity, gaining insights on seasonal spatial interaction and spatio-temporal overlap between sloth bears (Melursus ursinus) and humans, and subsequently predicted the conflict source sites and dispersion (i.e., hotspots) based on the robust geographic profiling (GP) method in the Sanjay Tiger Reserve (STR), a human-dominated landscape of central India. Detection data of sloth bear and human were obtained from camera trap survey conducted for two years (2017–2018) and records of conflict incidents (2009–2019) were collected from forest department. We found that sloth bears can co-occur with humans independently of seasons, based on occupancy models. However, during summer, higher temporal overlap (Δ4 = 0.46) and lower spatial overlap (0.31) were observed between sloth bears and humans. Contrastingly, lower temporal overlap (Δ4 = 0.29) and higher spatial overlap (0.44) were observed between the same two during winter. The activity patterns of sloth bears and humans differed significantly across seasons and within the same species in different seasons. Our findings indicated that significant changes in human activity, especially during summer, increased the likelihood of sloth bear-human interaction and subsequent conflict incidents. The mapping of conflict source and dispersion (with high accuracy) also predicted a greater probability of conflict during summer, compared to winter, and thus showed the successful application of GP models in this field. Also, camera trap data alone were able to predict the occurrence of hotspots, demonstrating the use of camera trap records in the successful prediction of source-dispersion of conflict. This study would be useful for decision-makers to alleviate sloth bear–human conflict based on insights on seasonal variation of spatio-temporal overlap between the two and direct conservation efforts accordingly.
The ever-expanding human population and the resultant degradation and fragmentation of habitat have inadvertently affected wildlife and increased the proximity-driven interaction between humans and wildlife (Messmer, 2000; Messmer, 2009; Inskip and Zimmermann, 2009; Elfström et al., 2014; Joshi et al., 2016). Often, these human–wildlife interactions pose challenges to conservation initiatives due to the significant damage in the form of livestock depredation, human-mauling, crop loss, and retaliatory killing of the concerned species (Rajpurohit and Krausman, 2000; Madhusudan, 2003; Treves and Karanth, 2003; Bargali et al., 2005; Karanth et al., 2012). However, the identification of underlying reasons behind such conflict scenarios is necessary to address human–wildlife conflict (Sutherland et al., 2009). Among other species of wildlife, conflict with large carnivores (including bears) has a significant impact on the health and livelihood of humans worldwide (Treves and Karanth, 2003; Chapron et al., 2014). Human–carnivore interaction has been studied widely to understand the nature and extent of conflict and draw inferences on possible conflict mitigation (Redpath et al., 2013; Nyhus, 2016).
The expansion of anthropogenic activities has affected the global distribution of carnivores as well as their temporal and spatial use of the habitat, globally (Dirzo et al., 2014; Gaynor et al., 2018; Tucker et al., 2018). Also, humans impact directly or indirectly on the mortality and physiological fitness of carnivores (Rode et al., 2007; Darimont et al., 2015). However, it was observed that human–wildlife interaction could be quite complex and dependent on several factors (Tablado and Jenni, 2017). Wild animals, especially carnivores, can respond to increased or persistent anthropogenic activities in a variety of ways (Ordiz et al., 2011; Carter et al., 2012; Elfström et al., 2014; Tablado and Jenni, 2017; Suraci et al., 2019b), including strong fear responses (Suraci et al., 2019a). The response of carnivores towards human occurrences may be governed by the spatial extent and intensity of the anthropogenic pressure prevailing in the concerned landscape. In a human-dominated landscape, especially with year-round high occurrence of humans can affect the space use pattern of carnivores, leading to avoidance of areas where human disturbance is high (Chi and Gilbert, 1999; Fortin et al., 2016). Many wildlife species, including bears, consider humans as a perceived threat or predator as they elicit similar responses in the presence of natural predators (Joshi et al., 1999; Frid and Dill, 2002; Ciuti et al., 2012). However, bears were also found to be frequently attracted to human settlements for opportunistic foraging, especially crop raiding, livestock depredation, and feeding on garbage (food wastes) (Charoo et al., 2011; Morales-González et al., 2020; Prajapati et al., 2021). Despite these exceptions, it is likely that animals will avoid places where human pressure persists (Dröge et al., 2017). Similarly, if the temporal pattern of human occurrence is more predictable to the animals, it is also possible that animals would segregate their movement by increasing the time of activity when humans are least active (Suraci et al., 2019b), instead of avoiding spatially (Nickel et al., 2020), which would in turn facilitate co-existence (Fagen and Fagen, 1994; Fortin et al., 2016; Srivastava et al., 2020).
It is expected that human–wildlife conflict is a direct outcome of the high degree of human–wildlife interface (Pack et al., 2013). However, unlike in Europe and North American countries, in South and South-east Asian regions, the prevalence of human–wildlife conflict is primarily due to rich and endangered biodiversity and associated developmental activities in and around forest areas (Madhusudan and Karanth, 2002; Sodhi et al., 2010). A high degree of dependence on natural resources driven by poverty and an expanding human population impacts adversely on biodiversity (Sodhi et al., 2010; Chao, 2012) and subsequently increases conflict scenarios due to highly overlapping resource use (Treves et al., 2006). In this view, there is a paucity of studies that take into consideration the spatio-temporal interactions between humans and carnivores with global distribution, such as Asian bear species, to address the issues of conflict more effectively (Can et al., 2014; Anand and Radhakrishna, 2017).
The sloth bear (Melursus ursinus) is one of the four bear species found in the Indian subcontinent and endemic to this region (Prater, 1965; Johnsingh, 2003; Sathyakumar et al., 2012). Most of the sloth bear populations are restricted to the moist and dry deciduous forests of the Western Ghats and central Indian landscape (Yoganand et al., 2006). The sloth bear is a myrmecophagous (ant and termite eating) ursid, which is notably involved in conflict with humans in some parts of its distributional range, especially human-dominated landscapes (Rajpurohit and Krausman, 2000; Bargali et al., 2005; Akhtar and Chauhan, 2008; Garcia et al., 2016; Debata et al., 2017; Dhamorikar et al., 2017; Singh et al., 2018; Sharp et al., 2020; Prajapati et al., 2021). However, except for a few anecdotal evidences, attacks on humans by sloth bears are mostly defensive, rather than intentional or predatory in nature, as they perceive humans as threats or predators (Sharp et al., 2020). In human-dominated forests of central India, it was found that most of the victims belonged to tribal communities with high natural resource dependency and were attacked inside the forest while collecting non-timber forest produce (NTFP) (Dhamorikar et al., 2017), as well as adjacent to the village peripheries and agricultural lands (Dhamorikar et al., 2017; Singh et al., 2018). The seasonal and temporal patterns of these attacks varied between different landscapes (Bargali et al., 2005; Dhamorikar et al., 2017; Singh et al., 2018). Sloth bears are mainly nocturnal and crepuscular in activity (Yoganand, 2005; Bargali et al., 2012; Ramesh et al., 2013) but could be active throughout the daylight hours in some parts of its distribution (Joshi et al., 1999). It was believed that the majority of the conflict cases happened due to the overlapped activity between sloth bears and humans during the crepuscular period (Akhtar et al., 2004; Bargali et al., 2005). However, there is a shortfall of studies which describe the fine-scale interaction and overlap (spatial and temporal) between sloth bears and humans utilizing the spatially independent point locations (such as camera traps) in a human-dominated landscape. Also, to our knowledge, no research work has been conducted to predict probable conflict sources and hotspots based on occurrence records of sloth bears and humans.
In this study, we used the season-specific detection records of sloth bears and humans, obtained from a camera trap survey conducted in the Sanjay Tiger Reserve (STR), central India, to understand the interaction between sloth bears and humans. Firstly, based on detection and non-detection data of sloth bears and humans, two-species occupancy models (Richmond et al., 2010) were developed to assess the species interaction in terms of association or segregation. Furthermore, the extent of spatial and temporal overlap was estimated using the relative abundance index (RAI) (O’Brien et al., 2003; Rovero and Marshall, 2009) of sloth bears and humans. We expected that sloth bears would avoid humans spatially regardless of seasons, as evident from other studies (Paudel et al., 2022; Pokharel et al., 2022). However, we also assumed that sloth bears would be more nocturnal in disturbed habitats to avoid human encounters during daylight hours. Finally, we used geographic profiling/geo-profiling (GP) to identify conflict sources and dispersion (i.e., hotspots), a novel approach to tackle wildlife crime and predict conflict hotspots (Faulkner et al., 2018; Struebig et al., 2018), which was earlier only restricted to criminology (Rossmo, 2000). With this background, we aimed to understand the seasonal spatial interaction and spatiotemporal overlap between sloth bears and humans in the context of a human-dominated landscape. Subsequently, we also attempted to identify probable conflict source sites and hotspots that can serve as a basis for implementing sloth bear–human conflict mitigation strategies.
This study was conducted at the Sanjay Tiger Reserve (STR), which is spread over two districts, i.e., Sidhi and Shahdol, in the Indian state of Madhya Pradesh, central India (Figure 1). As per the biogeographic classification of India by Rodgers & Panwar (1988), STR comes under the “Central Highlands.” Sanjay National Park (SNP) and Dubri Wildlife Sanctuary (DWLS) together constitute the core area (831.25 km2) of STR. Surrounding the core, an additional area of 812.58 km2 was demarcated as a buffer zone for STR.
Figure 1 Location of Sanjay Tiger Reserve (STR) in India, along with land use land cover (LULC) classes and camera trap locations.
The forest type of STR is dominated by Shorea robusta (Sal). It falls under the sub-group "North Indian moist deciduous forests", with subtype "Moist sal-bearing forest" and subdivision "Moist peninsular sal forest", according to the revised classification by Champion & Seth (1968). Other major tree species found in STR include Buchanania cochinchinensis (Char), Diospyros melanoxylon (Tendu), Madhuca longifolia, (Mahua), Lagerstroemia parviflora (Sedha), and Cassia fistula (Amaltas). Land use land cover types of STR are categorized into six classes, i.e., dense forest, open forest, scrubland, barren land, water bodies, and agricultural land (Figure 1). The elevation of the STR varied from 239 to 770 m. The topography is generally undulating and hilly in the eastern part and moderately sloped to flat terrain in the western part with varying slopes, ranging from 0° to 61°. Temperature varies between 7.4 and 41.8°C with distinct seasonal variation viz. summer (March to June), monsoon (July to October), and winter (November to February). The mean annual precipitation in STR is 1,303 mm. Apart from sloth bear, STR harbors diverse mammal species such as the Panthera tigris (Tiger), Panthera pardus (Leopard), Hyaena hyaena (Striped hyena), Canis lupus (Indian wolf) Cuon alpinus (Asiatic wild dog), Axis axis (Chital), Rusa unicolor (Sambar), Tetracerus quadricornis (Four-horned antelope), etc. There are thirty-nine villages inside the core area of the Tiger Reserve. People living in these villages, along with their substantial livestock population, are highly dependent on forest resources, which pose immense anthropogenic pressure on the core habitat of STR.
Systematic camera trapping exercises were conducted over two consecutive years, from December 2016 to April 2017 (henceforth, 2017) and January 2018 to April 2018, in the core area of STR. The core area was gridded into 4 km2 blocks (henceforth, sites), following the Indian tiger estimation protocol (Jhala et al., 2015). A total of 143 and 144 sites were selected for the deployment of camera traps during 2017 and 2018, respectively. Due to logistical constraints, we divided the core area into two blocks, viz., SNP (466.6 km2) and DWLS (364.6 km2) for the deployment of camera traps. First, we deployed camera traps at 86 sites during winter 2017, i.e., December 2016 to February 2017 in SNP, followed by 57 sites in DWLS during summer 2017, i.e., March 2017 to April 2017. During winter 2018 (January to February) and summer 2018 (March to April), camera traps were deployed in 57 sites in DWLS and 87 sites in SNP, respectively.
Double-sided automated camera traps (Cuddeback C1 and Cuddeback Ambush) were placed preferably on dirt roads, dry riverbeds, and animal trails to maximize the detectability of large carnivores (Karanth et al., 2011), including sloth bears, at a height of 30–40 cm above the ground. Furthermore, the placement of double-sided camera traps, opposite to each other, at each site would preferably increase the detection probability of animals (Pease et al., 2016). Each camera trap was set to take photographs at a 15-second interval upon detection of the animals. The survey duration of camera traps at each block was continued for 45 days, and camera traps were active on a 24 × 7 basis. However, in 2017, due to rampant forest fires at DWLS during summer, camera traps were active for an average period of 36 days. Each camera trap location was recorded using Garmin e-Trex handheld GPS units (Garmin Inc.).
After retrieving the camera trap data, detections of sloth bears and humans were considered independent events (or independent photographs) if two consecutive photographs of each species were taken at a ≥30-minute interval, to limit the risk of pseudoreplication (O’Brien et al., 2003; Xiao et al., 2018; Haswell et al., 2020). We chose a 30-minute interval for humans as we expected a substantial number of repeated photo-captures of the same individual (single and groups) within a short period, due to the curiosity of people detecting camera traps. The entire survey duration (45 days) was collapsed into 15 temporal replicates per site by considering each three days as a single temporal replicate and pooling the detection–nondetection records of species accordingly. Collapsing the survey duration into a smaller number of temporal replicates facilitated the reduction of overdispersion (variance > mean) and increased the temporal independence of each detection (Penjor et al., 2019). Lastly, we used camera trap data from two summers and two winters obtained from two blocks representing the entire core area of STR for each season (overall winter and summer).
In the first step, we used two-species conditional occupancy models (Richmond et al., 2010) to assess if sloth bears show any spatial avoidance toward human occurrence in two different seasons (winter and summer). The two-species occupancy model estimates the conditional probability of occupancy and detectability of a less dominant species in the presence of a dominant species (Richmond et al., 2010). We interpreted the “probability of occupancy” of a species as the probability of “use” since we believed that the size of the site would potentially violate the closure assumption and independence between sites (MacKenzie et al., 2017, p447). If species A and B are to co-occur and A is dominant over B, the following parameters are estimated in the two-species model:
i. ΨA (Probability of occupancy of A)
ii. ΨBa (Probability of occupancy of B when A is absent)
iii. ΨBA (Probability of occupancy of B when A is present)
iv. pA (Detection probability of A when B is absent)
v. pB (Detection probability of B when A is absent)
vi. rA (Detection probability of A when both A and B were present)
vii. rBA (Detection probability of B when A was present and detected)
viii. rBa (Detection probability of B when A is present but not detected).
In this study, we assumed that human (species A) activity does not depend on the occurrence of sloth bear (species B). Sloth bears probably perceive humans as a threat equivalent to predators (tigers and leopards), with which they are sympatric (Laurie and Seidensticker, 1977; Yoganand, 2005). Given the extensive anthropogenic pressure in STR, it is very likely that sloth bears would adjust their space use in accordance with human occurrence. In this view, we assumed sloth bears to be a subordinate species in the two-species occupancy model. However, considering humans as the dominant species in two-species occupancy models was not common [but see Parsons et al. (2016)], our intention was to assess the degree of spatial avoidance of sloth bears in response to the extensive occurrence of a perceived threat (i.e., humans) in the context of the human-dominated landscape.
The species interaction factor (henceforth, SIF) is a derived parameter estimated from the two-species occupancy model. A value of SIF >1 shows the degree of species aggregation rather than avoidance, and an SIF value <1 indicates spatial avoidance or segregation, where two species are less likely to occur with each other than expected under the hypothesis of species independence. An SIF value of ~1 indicates that both species can occur independently. Following Richmond et al. (2010), SIF can be calculated as:
We calculated SIF and compared it between seasons to assess the avoidance of space used by sloth bears in the presence of humans. We also carefully inspected the values of other parameter estimates (ΨBa, ΨBA, pB, rBA and rBa), and compared them between seasons. Model comparison and selection were based on Akaike’s Information Criterion (AIC) (Burnham and Anderson, 2002). We used the program Presence (Version 2.13.11) (Hines and USGS-PWRC, 2006) to estimate occupancy and related parameters of the two species.
In the second step, the extent of spatial overlap between sloth bears and humans was estimated for summer and winter as we were interested in assessing the interaction on a finer scale, which might not always be discernible from occupancy models. In order to do so, the relative abundance index (RAI; O’Brien et al., 2003; Rovero & Marshall, 2009) was calculated at each camera trap location for both species using the following formula:
RAI = (No. of independent photographs/total no. of days during which each camera trap was operating) × 100.
Considering each location of the camera trap as spatially independent of other locations, we calculated the spatial overlap by applying Pianka’s overlap index (Pianka, 1974). This method takes account of the proportion of RAI values of a pair of species with respect to different camera trap locations. The output value (estimate of overlap) ranges between 0, i.e., no overlap and 1 or complete overlap. Although it was developed initially to estimate the niche overlap, several studies have applied Pianka’s index to estimate spatial overlap between co-occurring species and human activities (Ramesh et al., 2012b; Yang et al., 2019; Mori et al., 2020; Zhao et al., 2020). We also estimated 95% confidence interval (CI) of the spatial overlap estimate from 10,000 bootstrapped replicates. All analyses related to spatial overlap estimation were carried out in R (R Core Team, 2019) using the package “spaa” (Zhang et al., 2013).
To assess the activity pattern of sloth bears and humans for summer and winter, we considered the same camera trap locations that were used to compute the spatial overlap. Following the methodology described by Ridout & Linkie (2009), a non-parametric kernel density function was fitted to the circular time data obtained from the camera trap photographs of both species, which would produce the pairwise (sloth bear–human) activity patterns. Then, the coefficient of overlap (delta, Δ) was estimated from the area under the curve of two different density functions (sloth bear and human). Values of Δ ranged between 0 and 1, where 0 denotes no overlap and 1 denotes complete overlap (Linkie & Ridout, 2011; Meredith & Ridout, 2014). We calculated Δ4 when the number of independent events of both sloth bear and human, were >75, except for one season (winter 2018), when, number of sloth bear photographs was <75 and hence, Δ1 was calculated, as mentioned by Linkie & Ridout (2011). The 95% CI was generated by 10,000 bootstrapped samples of overlap coefficient to check the precision of the estimates (Linkie and Ridout, 2011). Analyses regarding the estimation of overlap coefficient were carried out in the package “overlap” (Meredith and Ridout, 2021), implemented in R (R Core Team, 2019).
One-sample Watson’s U2 test (Watson, 1961) is a goodness of fit test, which was performed to determine whether the distribution of detection time of each species significantly deviated from the uniform distribution of activity. Similarly, we performed two-sample Watson’s U2 tests (Watson, 1962) to assess if the activity distributions of sloth bears and humans were significantly different from each other between summer and winter. We also tested for the difference in activity of sloth bears and humans separately between seasons through two–sample Watson’s U2 tests. Both one-sample and two-sample Watson’s U2 tests were performed using the software “Oriana” (Kovach, 2011).
Records of conflict incidents between sloth bears and humans in the form of human injuries and deaths were obtained from the forest department of STR for 10 years (2009–2019). We retained the records only for the core area of the STR, as our camera trapping exercise was conducted in the core area only. However, GPS locations of these incidents were generally not recorded by the forest department; only the name of the concerned forest beat and compartment was mentioned. As compartments are the smallest (average size of 2.12 km2) administrative units of our study area (and all other Tiger Reserves), we generated centroids of compartments of forest beats where conflict incidents occurred and used this information for further analysis.
Geographic profiling (GP) is a technique used to estimate the probable sources of spatial encounters (human–sloth bear encounters in this case) when the sources are unknown (villages or sloth bear territories). It works on the Bayesian framework based on a distance decay process (Verity et al., 2014). We used the dirichlet process mixture (DPM) (Verity et al., 2014; Faulkner et al., 2017) model to produce a geographic profile of sloth bear–human encounters using the package Rgeoprofile 2.1.0 in R (https://github.com/bobverity/Rgeoprofile). GP has been proven to be advantageous over conventionally used prediction models such as kernel density and ensemble modeling for mapping of invasive species as well as conflict or risk assessment (Stevenson et al., 2012; Struebig et al., 2018). The process consists of two parts: the incident locations where the conflict occurred and the source locations from where the conflict might have arisen. The model divides the incident locations into “n” clusters. Then the source sites are predicted using the Gibbs sampler (Geman and Geman, 1984) within a Markov Chain Monte Carlo framework until it converges on the posterior distribution of sigma (σ). Sigma (σ) is the standard deviation of the dispersion distribution, and it means how far we expect the sources to travel from the incident sites. There are two approaches to testing the model efficiency: one is by using the hit score percentage (HS%, ranging from 0 to 100%), and the second is using the Gini coefficient (G, ranging from 0 to 1) (Gini, 1921; Faulkner et al., 2018). The GP model provides an optimal search process of the source locations or anchor points. The hit score percentage (i.e., the proportion of the area covered by the "conflict/encounter" divided by the total area) was used to test the efficiency of the model, with a lower hit score value depicting more accuracy of the search process. The Gini coefficient was used to assess the model performance; the higher the Gini coefficient, the more accurate the geographic profile (Faulkner et al., 2018).
We produced a geographic profile of sloth bear–human encounters using a) conflict data of sloth bear attacks on humans and b) camera trap data where both the sloth bears and humans were detected together (but at different times). We used the compartment centroids for the conflict data and camera trap data consisting of only sloth bears or humans were excluded. Duplicate points were removed from the data as the DPM model tends to fit very low sigma values with repeated locations (Struebig et al., 2018). The mean sigma (σ) used for the analysis was 2 km [assuming 87% of the dispersal events occur within 2 km of the source; following Faulkner et al. (2017)] and we ran the full data set with locations of conflict and camera trap, using 10 chains with a burn-in of 10,000. Firstly, the resultant model will identify the probable conflict source sites, followed by conflict hotspots or dispersion around the source sites. Next, we compared any seasonal shift in the conflict-probable areas in STR using the summer and winter camera trap locations separately, where sloth bear and human both were detected. In this study, we assumed the camera trap data where both the sloth bears and humans were detected to be a probable conflict site. Therefore, to test the efficacy of camera trap data (n = 88) alone to predict the future probable conflict areas, model validation was conducted. We generated a geographic profile based solely on camera trap data (where both sloth bears and humans were detected). Sloth bear attack sites (n = 57) were then overlaid on the geographic profile layer to check whether the conflict sites were falling over the predicted probable conflict areas generated by the camera trap data.
A total of 179 and 311 independent detections of sloth bears were obtained from camera traps in winter and summer, respectively, during the entire survey period of 12,430 trap-nights (winter = 6,435 trap-nights; summer = 5,995 trap-nights). Detection of sloth bears was substantially higher in SNP (winter = 122; summer = 235) than in DWLS (winter = 57; summer = 76), irrespective of the season (Figure 2). Similarly, the detection of humans was found to be higher in SNP (winter = 3,396; summer = 2,565) than in DWLS (summer = 1,749; winter = 1,862) (Figure 2).
Figure 2 Block-wise photographic captures of sloth bears (A) and humans (B) in winter and summer, obtained from camera trap surveys during 2017–2018 in the core zone of Sanjay Tiger Reserve, Madhya Pradesh, India.
During winter and summer, SIF values (SIF value ± Standard Error) were found to be 0.98 ± 0.007 (95% CI: 0.97–0.99) and 1.00 ± 0.03 (95% CI: 0.93–1.07), respectively (Table 1). Thus, no difference in species interaction was observed between seasons. The closeness of the SIF value toward 1 indicated no distinguishable pattern of spatial avoidance shown by sloth bears toward human occurrences. The probability of use of sites by sloth bears in the presence of humans was found to be less [ΨBA (SE) = 0.71 (0.04)] than when humans are absent [ΨBa (SE) = 1.00 (0.00)] during winter. However, during summer, the probability of use of sites by sloth bears did not differ much [ΨBA (SE) = 0.79 (0.04); ΨBa (SE) = 0.77 (0.17)] in the presence or absence of humans.
Table 1 Values of species interaction factor (SIF), probability of use (ΨBA) of sites by sloth bears (denoted as “B”) in the presence of humans (denoted as “A”), probability of use (ΨBa) of sites by sloth bears in the absence of humans (denoted as “a”) and detection SIF (delta, δ), along with associated standard error (SE), calculated for two seasons (winter and summer) in the Sanjay Tiger Reserve, Madhya Pradesh, India, during 2017–2018.
A higher spatial overlap (0.44) between sloth bears and humans was observed during winter than in summer (0.31). A high variation in spatial overlap between sloth bears and humans was observed between seasons in DWLS compared to SNP (Table 2).
Table 2 Spatial overlap index values of species pair (sloth bear and human), with associated 95% confidence interval (CI), during 2017–2018 in the Sanjay Tiger Reserve, Madhya Pradesh, India.
It was found that, during summer, the temporal overlap between sloth bears and humans was higher in comparison to winter (Table 3; Figure 3). Sloth bears were found to be more crepuscular and nocturnal (Watson’s U2 = 0.09; p <0.05 for winter; Watson’s U2 = 0.51; p <0.005 for summer) irrespective of the seasons, whereas human activity was found to be primarily diurnal (Watson’s U2 = 2.25; p <0.005 for winter; Watson’s U2 = 3.85; p <0.005 for summer; Table 4). During summer, the activity of humans started before 06:00 and extended as long as 22:00 (Figure 3). However, during winter, human activities mostly started after 07:00 and continued till 20:00 (Figure 3). Watson’s two-sample U2 test revealed that sloth bear and human activity patterns significantly differed (Watson’s U2 = 9.84, p <0.001 for winter; Watson’s U2 = 11.13, p <0.001 during summer; Table 4) from each other for both the seasons. However, between seasons, the activity of sloth bears and humans also differed significantly (U2 = 0.22, p <0.05 for sloth bears; U2 = 19.61, p <0.001 for humans).
Table 3 Estimates of temporal overlap (Δ4) between sloth bear and human, with associated 95% confidence interval (CI), during 2017–2018 in the Sanjay Tiger Reserve, Madhya Pradesh, India.
Figure 3 Activity overlap and overlap coefficient (Δ4), i.e., gray shaded area, between sloth bear (blue solid line) and human (red solid line) for overall winter (A) and summer (B) in the Sanjay Tiger Reserve, Madhya Pradesh, India, during 2017–2018.
Table 4 Circular statistics (include one-sample and multi-sample tests) of seasonal patterns of temporal activity of sloth bears and humans during 2017–2018 in the Sanjay Tiger Reserve, Madhya Pradesh, India.
A total of 57 records of attacks on humans by sloth bears occurred in the core area of STR from 2009 to 2019. Centroids of compartments were generated for 57 conflict incidents.
The first model was run to produce the overall geographic profile of sloth bear–human encounters in STR using locations (n = 57) of bear attacks on humans and 88 camera trap locations that detected both sloth bears and humans. The top 10% of the probable source sites (n = 8) are mentioned in Table 5 and Figure 4. The Gini coefficient of the model was 0.97. For the second model, the seasonal shift in the probable conflict sites was assessed using 81 and 66 camera trap locations for summer and winter, respectively. The model identified 9 sites and 5 sites as probable sources of conflict in summer and winter, respectively, details of which are provided in the supplementary material (Supplementary Table 1; Supplementary Figures 1, 2). The Gini coefficient for the summer and winter models was 0.90 and 0.99, respectively.
Table 5 Hit score percentages of the probable source locations estimated from the Dirichlet Process Mixture (DPM) modeling using conflict (sloth bear attacks on humans from 2009 to 2019) and camera trap locations (where sloth bears and humans were both photo-captured) as incident sites in the Sanjay Tiger Reserve, Madhya Pradesh, India, during 2017–2018.
Figure 4 Map showing the top 10% of the probable sloth bear–human conflict areas (probable source sites and dispersion or hotspots around source sites) in the Sanjay Tiger Reserve, Madhya Pradesh, India, based on locations of sloth bear attacks on humans (from 2009 to 2019) and camera trap surveys (locations where sloth bears and humans were both photo-captured) conducted during 2017–2018; blue square points (n = 8) were probable source locations (or anchor points) of conflict; red points were the camera trap locations which photo-captured both sloth bears and humans; lighter color shade indicated high conflict probabilities.
A third model was run using 88 camera trap locations where sloth bears and humans were detected together, to test the efficacy of camera trap data for conflict prediction (Figure 5). A profile based on camera trap data alone produced a similar conflict prediction and could identify 21% of the sloth bear attacks on humans (12 attack sites out of 57) by searching less than 10% of the study area and 50% of these conflicts (29 attack sites out of 57) by searching just 20% of the study area (Supplementary Table 2). Thus, it validated the model performance (Gini coefficient = 0.91).
Figure 5 Prediction of the source-dispersion of sloth bear–human conflict using camera trap surveys conducted in the Sanjay Tiger Reserve during 2017–2018, Madhya Pradesh, India; the lighter shade areas indicate high conflict probable areas and the blue squared points were the probable source sites (n = 8) of conflict hotspots; light green points were the conflict sites where attacks on humans by sloth bears occurred between 2009 and 2019; red points were the camera trap locations which photo-captured both sloth bears and humans; twenty-nine conflict sites fall within <20% of the search area and 12 conflict sites within <10%, thus validating the model performance.
We reported spatial interaction and spatio-temporal overlap between sloth bears and humans with respect to seasons, i.e., winter and summer, in STR. Sloth bears independently co-occurred with humans in terms of space use, which was in contradiction to our expectations. However, distinct seasonal variation of spatial and temporal overlap between sloth bears and humans was observed at a finer scale, which, we believe, perhaps enables sloth bears to co-exist with humans in a human-dominated landscape. Finally, in the context of extensive human activities in sloth bear habitat and resultant conflict incidents, we identified the probable conflict source sites and hotspots in STR based on available conflict records and detections of sloth bears and humans from camera traps.
Two-species occupancy models showed that sloth bears could independently occur with humans irrespective of seasons, which, to our knowledge, is not an entirely novel situation. In Mudumalai Tiger Reserve, the occupancy of sloth bears was uninfluenced by the distance to the nearest human settlements (Ramesh et al., 2012a). Sloth bears can be habituated to persistent human presence and are attracted to human settlements in search of abundant seasonal fruits (Ziziphus mauritiana, Syzygium cumini), cultivated crops (groundnut, maize), and even garbage (food wastes) (Akhtar et al., 2004; Palei et al., 2020; Prajapati et al., 2021), which also increased the sloth bear–human conflict (Bargali et al., 2005; Prajapati et al., 2021). However, increased human activity or encounter with humans would facilitate the shifting of temporal activities of many mammalian species, including carnivores (Gaynor et al., 2018), instead of spatial avoidance (Nickel et al., 2020), or could induce habituation towards human activity (Albert and Bowyer, 1991; Barnes and Wilker, 2000; Elfström et al., 2014). On the other hand, bears show strong avoidance of human activities both spatially and temporally (Chi and Gilbert, 1999; Tollefson et al., 2005; Ladle et al., 2018). We believe the spatial distribution of humans or anthropogenic activities could be the governing factor of such spatial avoidance or spatial co-occurrence. In the human-dominated landscape, it is not always possible to avoid humans spatially as it would cost to miss out on potential resource-rich areas, which would negatively affect the foraging success and overall fitness of the animal. In STR, human occurrence and livestock grazing in sloth bear habitat are extensive. We found high occupancy (ΨA = 0.96 ± 0.02 in winter; ΨA = 0.84 ± 0.03 in summer) of humans in STR, which indicated the substantial level of anthropogenic pressure throughout the study area, regardless of seasons. Despite this extensive human pressure, the probability of use of sites by sloth bears was also found to be high (ΨBA = 0.71 ± 0.04 in winter; ΨBA = 0.79 ± 0.04 in summer; Table 2), which indicated that irrespective of seasons, sloth bears are utilizing sites independently of humans. It was also found that detection SIF (delta, δ) was slightly higher than 1 (δ = 1.05 ± 0.06 in winter; δ = 1.06 ± 0.07 in summer; Table 2), indicating some sort of association in detection between sloth bears and humans. It could be attributed to the relatively high preference for trail use by wide-ranging carnivores (Harmsen et al., 2010; Karanth et al., 2011; Kays et al., 2017; Nickel et al., 2020), apart from humans, which would facilitate animals to cover a larger distance in an energetically efficient way (Dickie et al., 2017).
However, spatial overlap estimation based on Pianka’s overlap index (Pianka, 1974) revealed seasonal variation between sloth bears and humans. The intensity of space use by sloth bears was lower (0.31) during summer than during winter (0.44), which indicated that bears used sites less frequently during summer in the presence of humans than in winter. Given the widespread distribution of human activities during winter and summer, it was expected to have more overlapped areas between the two without any seasonal variation. Less spatial overlap between sloth bears and humans during the summer season could be attributed to comparatively higher temporal overlap during summer with respect to winter (see Table 4). In STR, human activities were found to be primarily diurnal regardless of seasons. However, during summer, the activities of humans started well before 06:00 o’clock in the morning and continued till late evening hours, which substantially overlapped with the crepuscular activity of sloth bears (Yoganand, 2005; Bargali et al., 2012; Ramesh et al., 2013). Increased human activity in the early morning and crepuscular hours during summer (especially from March to the first half of April) is primarily associated with the collection of flowers of Mahua, which is used to prepare alcoholic beverages (Kumar and Rao, 2007; Mewada, 2012). The selling of dry Mahua flowers significantly helps in the income generation of indigenous people (Islam and Quli, 2017). Hence, it is widely considered an important livelihood option for indigenous people in India (Kumar et al., 2018; Mishra and Poonia, 2019). The Mahua flowers also significantly contributed to the diet of sloth bears during the onset of summer (Bargali et al., 2004; Yoganand, 2005), which, coupled with its crepuscular activity, increased the interaction between sloth bears and humans (Akhtar et al., 2004). In STR, based on our camera trap survey, it was found that the frequency of occurrence of sloth bears and humans at per hour intervals was high during the early morning (05:00–07:00) and evening (19:00–20:00) in summer (Supplementary Figure 3). To avoid human encounters during the crepuscular period, it is possible that sloth bears used sites less intensively where chances of human encounters were high. Contrastingly, during winter, high spatial overlap could be associated with low temporal overlap between sloth bears and humans. In winter, human activities remained more concentrated during broad daylight hours for a comparatively short span of time, but did not start in the early morning hours, like summer (see Figure 3). In line with this, the morning (07:00–08:00) and early evening hours (15:00–16:00) showed the maximum frequency of occurrence of sloth bears and humans per hour interval, although the magnitude of the frequency of occurrence was less in winter compared to summer (Supplementary Figure 3).
We found that the activity patterns of humans varied significantly between seasons, which was also the case for sloth bears. Our results indicated that sloth bears used sites more extensively during winter regardless of human occurrences, with a distinct temporal avoidance. Studies elsewhere have shown that carnivores could avoid human occurrences temporally (Tollefson et al., 2005), instead of avoiding them spatially, in a human-dominated landscape (Wang et al., 2015; Nickel et al., 2020). The marginal difference (p <0.05) in the activity of sloth bears between seasons indicated that sloth bears tended to avoid a more predictable pattern of human activity, despite already being strictly nocturnal and crepuscular in a human-dominated landscape (Bargali et al., 2012). Since human activity is not dependent on sloth bear activity, our study revealed that a significant shift in human activity between seasons influenced the likelihood of interaction between sloth bears and humans temporally and spatially.
However, despite distinct spatio-temporal avoidance, the conflict between sloth bears and humans in terms of human-mauling is prevalent in STR. It is attributed to the year-round existing immense anthropogenic pressure posed by human settlements inside the core area of STR. Similar observations were also made in the human-dominated landscapes of central India (Bargali et al., 2005; Dhamorikar et al., 2017) and elsewhere (Garcia et al., 2016; Debata et al., 2017; Singh et al., 2018). Based on our camera trap survey, we found that during summer, the number of detections of sloth bears and humans per hour interval was higher (n = 53) than during winter (n = 33), indicating that during summer, there is a relatively high probability of conflict incidents inside the forest, as reported by Dhamorikar et al. (2017). We assumed that sites where sloth bears and humans were detected more frequently within a specific bin (1 h in this case) of time are more prone to accidental encounters between them, while humans are engaged in different activities, especially in and around those sites. In previous studies, it has also been observed that most of the attacks occur during sudden close encounters with sloth bears (Dhamorikar et al., 2017; Singh et al., 2018), especially while collecting non-timber forest produce (NTFP) separately and silently inside the forest (Dhamorikar et al., 2017).
Finally, we have made an attempt to identify the conflict source sites and dispersion around source sites based on the present camera trap survey and records of conflict incidents obtained from the forest department. Our primary aim was to identify areas inside the forest where humans and sloth bears occurred more frequently, assuming that areas where sloth bears and humans were more likely to get exposed to each other, lead to conflict scenarios. Furthermore, records from the forest department were used to validate our model and future conflict predictions. We found that, based on detections of sloth bears and humans obtained from camera traps and validating them with registered conflict records, the GP model produced a reasonably good prediction (Gini coefficient = 0.92) of conflict source and dispersion. The GP model was found to be especially robust when fewer data points are provided and can potentially outcompete any other spatial statistics used in different contexts and scenarios of model-based predictions (Stevenson et al., 2012). However, a distinct seasonal variation was observed in the current prediction of conflict hotspots. More probable source sites (n = 9) of conflict were identified during summer, in comparison to winter (n = 5), indicating a greater chance of conflict in forest areas during summer, which was also earlier reported in the central Indian landscape (Dhamorikar et al., 2017). It could be attributed to the much higher number of detections of sloth bears (n = 312) during summer, in comparison to winter (n = 180, see Table 1), and associated high temporal overlap with humans. We found that seasonal variation in the temporal overlap between sloth bears and humans was also reflected in the predicted source-dispersion modeling of conflict in STR. As probable source sites and hotspots were mostly associated with villages’ locations, it was evident that the probability of conflict was also higher in areas adjacent to villages. Relocation of villages from the core zone of STR, especially those situated around the probable source sites shown here, would be a primary step for managers to reduce conflict between sloth bears and humans. However, despite being a contentious and gradual process, voluntary relocation of villages from the core zone of Tiger Reserves was proved to be one of the successful means to restore wildlife population and its habitat (Lasgorceix and Kothari, 2009), with special reference to tiger conservation in India (Jhala et al., 2021). Also, elsewhere in India, communities living inside Tiger Reserves prefer to be relocated willingly due to the decreased productivity of forests, lack of basic amenities, and human–wildlife conflict (Harihar et al., 2014). Thus, we believe that the findings of geographic profiling could be one means for forest managers to identify the villages that need to be relocated on a priority basis or rationalize resources or techniques for conflict mitigation. However, in between the process of relocation, an effort could be made to make people living in conflict hotspots aware of the avoidance of conflict scenarios based on the findings of this study and studies carried out elsewhere in central India (Bargali et al., 2005; Dhamorikar et al., 2017; Dhamorikar et al., 2018). Although we could not identify the conflict sources and hotspots outside the core zone of STR, conducting awareness programs among the local people as mitigation measures is especially important in the buffer zone and other human-dominated forest areas outside the Tiger Reserve.
Our study had several limitations. Due to logistical constraints, we could not broaden our understanding of conflict prediction in the buffer zone of STR. Also, camera traps were not deployed in the monsoon, which restricted us to drawing inferences during this season. We have deployed camera traps on the trails and forest roads in order to maximize the capture of tiger and other co-predators, including sloth bears. Previous studies showed that placement of camera traps on trails or forest roads significantly increased the detection of certain mammal species and bears as well (Wearn et al., 2013; Kolowski and Forrester, 2017; Tanwar et al., 2021). On the other hand, human activities inside the forest, such as the collection of NTFPs, are not restricted to the roads and trails but can be conducted anywhere in the forest depending upon resource availability and accessibility. In general, people do not walk alone on the forest roads or trails, especially during dark hours, as moving in groups would reduce the chance of being attacked by bears (Garrote et al., 2017). Despite knowing these facts, we assumed that in the locations where humans and bears were frequently detected, the chance of conflict could also be high in and around those locations. Although widely used, the efficacy of trail-based camera traps in estimating anthropogenic pressure has rarely been assessed. However, Miller et al. (2017) showed how wildlife monitoring cameras (on-trail) can also efficiently capture human activities and could be used for long-term wildlife–human interaction-based studies. Our study indicated fairly accurate conflict prediction through the GP model by using only camera trap data, although we recommend careful considerations while deploying camera traps (on-road and off-road) in future similar studies.
This study revealed that the interaction between sloth bears and humans was primarily governed by the seasonal variation of spatio-temporal overlap, especially the significant changes in human activity between seasons. From the species perspective, fine scale segregation of temporal and spatial activity was observed to co-exist with humans. However, extensive anthropogenic pressure throughout STR exacerbated the situation and led to conflict scenarios. Therefore, radio-telemetry-based research, along with monitoring through camera traps, is recommended to understand the spatio-temporal activity of sloth bears in a human-dominated landscape. In this study, the application of novel methods like GP models provided an opportunity to predict the source-dispersion of conflict precisely, even using secondary data sets obtained from camera traps. It would be insightful for forest managers to reduce conflict incidents derived from sloth bear–human interface with a more area-specific targeted approach and promote coexistence in a human-dominated landscape.
The datasets presented in this study can be found in online repositories. The names of the repository/repositories and accession number(s) can be found in the article/Supplementary Material.
SK and RK conceived the project. RK, SK, SS, and SC designed the study. SC and RR collected the data. SC and RR carried out data preparation prior to analysis. SC and MB completed data analysis, prepared tables and figures and wrote the first draft of the manuscript. SS, RK, and SK supervised and critically reviewed the manuscript. All authors listed have made a substantial, direct, and intellectual contribution to the work and approved it for publication.
This work was supported by the Madhya Pradesh Forest Department, Madhya Pradesh, India.
The authors declare that the research was conducted in the absence of any commercial or financial relationships that could be construed as a potential conflict of interest.
All claims expressed in this article are solely those of the authors and do not necessarily represent those of their affiliated organizations, or those of the publisher, the editors and the reviewers. Any product that may be evaluated in this article, or claim that may be made by its manufacturer, is not guaranteed or endorsed by the publisher.
We are grateful to the Madhya Pradesh Forest Department (Mr. Narendra Kumar, Dr. Suhas Kumar, Mr. Jitendra Agarwal, Mr. R.K. Srivastava, Dr. Deleep Kumar, and Mr. Vincent Rahim) for supporting this research through providing research permission and logistics during field work and the Wildlife Institute of India (Dr. V.B. Mathur, Dr. Dhananjai Mohan, Dr. Parag Nigam, and Professor Qamar Qureshi) for the institutional support, respectively. We also thank Ms. Snehaa Sundaram, Ms. Maithili Devadas, and Mr. Griffith Victor for helping us during initial field data collection and data preparation. We are thankful to all our field assistants (Mr. Amritlal Yadav, Mr. Ramsakha Yadav, Mr. Rakesh Yadav, Mr. Rajkaran Yadav, and Mr. Satendra Sahu) and the ground level staff of Sanjay Tiger Reserve for their untiring work during data collection in the field. We greatly appreciate the timely logistics provided by the forest range officers (Mr. M.S. Marawi, Mr. V.B.S. Parihar, and Mr. R.K. Jhariya) and the veterinary officer (Dr. Abhay Sengar) of the Sanjay Tiger Reserve. Special thanks are due to all the friends and colleagues in the Wildlife Institute of India, especially Dr. Rahul De, Mr. Dibyadeep Chatterjee, Mr. Shrutarshi Paul, Mr. Nilanjan Chatterjee, and Dr. Ankita Sinha, who have provided valuable suggestions during the preparation of this manuscript.
The Supplementary Material for this article can be found online at: https://www.frontiersin.org/articles/10.3389/fcosc.2022.850309/full#supplementary-material
Akhtar N., Bargali H. S., Chauhan N. P. S. (2004). Sloth Bear Habitat Use in Disturbed and Unprotected Areas of Madhya Pradesh, India. Ursus 15, 203–211. doi: 10.2192/1537-6176(2004)015<0203:SBHUID>2.0.CO;2
Akhtar N., Chauhan N. P. S. (2008). Status of Human Wildlife Conflict and Mitigation Strategies in Marwahi Forest Division, Bilaspur Chhattisgarh. Indian For. 134, 1349–1358.
Albert D. M., Bowyer T. R. (1991). Factors Related to Grizzly Bear: Human Interactions in Denali National Park. Wildl. Soc. Bull. 19, 339–349. doi: 10.2307/3782527
Anand S., Radhakrishna S. (2017). Investigating Trends in Human-Wildlife Conflict: Is Conflict Escalation Real or Imagined? J. Asia-Pac. Biodivers. 10, 154–161. doi: 10.1016/j.japb.2017.02.003
Bargali H. S., Akhtar N., Chauhan N. P. S. (2004). Feeding Ecology of Sloth Bears in a Disturbed Area in Central India. Ursus 15, 212–217. doi: 10.2192/1537-6176(2004)015<0212:FEOSBI>2.0.CO;2
Bargali H. S., Akhtar N., Chauhan N. P. S. (2005). Characteristics of Sloth Bear Attacks and Human Casualties in North Bilaspur Forest Division, Chhattisgarh, India. Ursus 16, 263–267. doi: 10.2192/1537-6176(2005)016[0263:COSBAA]2.0.CO;2
Bargali H. S., Akhtar N., Chauhan N. P. S. (2012). The Sloth Bear Activity and Movement in Highly Fragmented and Disturbed Habitat in Central India. World J. Zool. 7, 312–319. doi: 10.5829/idosi.wjz.2012.7.4.64180
Barnes V. G. Jr., Wilker G. A. (2000). Brown Bear Activity, Behavior, and Response to Public Use at Karluk Lake, Alaska (Alaska, USA: US Fish and Wildlife Service Report).
Burnham K. P., Anderson D. R. (2002). A Practical Information-Theoretic Approach (New York: Springer).
Can Ö.E., D’Cruze N., Garshelis D. L., Beecham J., Macdonald D. W. (2014). Resolving Human-Bear Conflict: A Global Survey of Countries, Experts, and Key Factors. Conserv. Lett. 7, 501–513. doi: 10.1111/conl.12117
Carter N. H., Shrestha B. K., Karki J. B., Pradhan N. M. B., Liu J. (2012). Coexistence Between Wildlife and Humans at Fine Spatial Scales. Proc. Natl. Acad. Sci. 109, 15360–15365. doi: 10.1073/pnas.1210490109
Champion H. G., Seth S. K. (1968). A Revised Survey of the Forest Types of India (Government of India, New Delhi, India: Manager of Publications).
Chao S. (2012). Forest Peoples: Numbers Across the World (UK: Forest Peoples Programme Moreton-in-Marsh).
Chapron G., Kaczensky P., Linnell J. D. C., Von Arx M., Huber D., Andrén H., et al. (2014). Recovery of Large Carnivores in Europe’s Modern Human-Dominated Landscapes. Science 346, 1517–1519. doi: 10.1126/science.1257553
Charoo S. A., Sharma L. K., Sathyakumar S. (2011). Asiatic Black Bear-Human Interactions Around Dachigam National Park, Kashmir, India. Ursus 22, 106–113. doi: 10.2192/URSUS-D-10-00021.1
Chi D. K., Gilbert B. K. (1999). Habitat Security for Alaskan Black Bears at Key Foraging Sites: Are There Thresholds for Human Disturbance? Ursus 11, 225–237. doi: 10.2307/3873005
Ciuti S., Northrup J. M., Muhly T. B., Simi S., Musiani M., Pitt J. A., et al. (2012). Effects of Humans on Behaviour of Wildlife Exceed Those of Natural Predators in a Landscape of Fear. PLoS One 7, 1–13. doi: 10.1371/journal.pone.0050611
Darimont C. T., Fox C. H., Bryan H. M., Reimchen T. E. (2015). The Unique Ecology of Human Predators. Science 349, 858–860. doi: 10.1126/science.aac4249
Debata S., Swain K. K., Sahu H. K., Palei H. S. (2017). Human-Sloth Bear Conflict in a Human-Dominated Landscape of Northern Odisha, India. Ursus 27, 90–98. doi: 10.2192/URSUS-D-16-00007.1
Dhamorikar A., Gore K., Bargali H. S. (2018). How to Avoid Conflict With Sloth Bears- a Pictorial Handbook (Mumbai: The Corbett Foundation).
Dhamorikar A. H., Mehta P., Bargali H. S., Gore K. (2017). Characteristics of Human-Sloth Bear (Melursus Ursinus) Encounters and the Resulting Human Casualties in the Kanha-Pench Corridor, Madhya Pradesh, India. PLoS One 12, 1–18. doi: 10.1371/journal.pone.0176612
Dickie M., Serrouya R., McNay R. S., Boutin S. (2017). Faster and Farther: Wolf Movement on Linear Features and Implications for Hunting Behaviour. J. Appl. Ecol. 54, 253–263. doi: 10.1111/1365-2664.12732
Dirzo R., Young H. S., Galetti M., Ceballos G., Isaac N. J. B., Collen B. (2014). Defaunation in the Anthropocene. Science 345, 401–406. doi: 10.1126/science.1251817
Dröge E., Creel S., Becker M. S., M’Soka J. (2017). Risky Times and Risky Places Interact to Affect Prey Behaviour. Nat. Ecol. Evol. 1, 1123–1128. doi: 10.1038/s41559-017-0220-9
Elfström M., Zedrosser A., Støen O. G., Swenson J. E. (2014). Ultimate and Proximate Mechanisms Underlying the Occurrence of Bears Close to Human Settlements: Review and Management Implications. Mamm. Rev. 44, 5–18. doi: 10.1111/j.1365-2907.2012.00223.x
Fagen J. M., Fagen R. (1994). Interactions Between Wildlife Viewers and Habituated Brown Bear-1992. Nat. Area. J. 14, 159–164.
Faulkner S. C., Stevens M. C. A., Romañach S. S., Lindsey P. A., Le Comber S. C. (2018). A Spatial Approach to Combatting Wildlife Crime. Conserv. Biol. 32, 685–693. doi: 10.1111/cobi.13027
Faulkner S. C., Verity R., Roberts D., Roy S. S., Robertson P. A., Stevenson M. D., et al. (2017). Using Geographic Profiling to Compare the Value of Sightings vs Trap Data in a Biological Invasion. Divers. Distrib. 23, 104–112. doi: 10.1111/ddi.12498
Fortin J. K., Rode K. D., Hilderbrand G. V., Wilder J., Farley S., Jorgensen C., et al. (2016). Impacts of Human Recreation on Brown Bears (Ursus Arctos): A Review and New Management Tool. PLoS One 11, 1–26. doi: 10.1371/journal.pone.0141983
Frid A., Dill L. (2002). Human-Caused Disturbance Stimuli as a Form of Predation Risk. Conserv. Ecol. 6, 11. doi: 10.5751/es-00404-060111
Garcia K. C., Joshi H. M., Dharaiya N. (2016). Assessment of Human-Sloth Bear Conflicts in North Gujarat, India. Ursus 27, 5–10. doi: 10.2192/URSUS-D-15-00012.1
Garrote P. J., Delgado M. M., López-Bao J. V., Fedriani J. M., Bombieri G., Penteriani V. (2017). Individual Attributes and Party Affect Large Carnivore Attacks on Humans. Eur. J. Wildl. Res. 63, 1–7. doi: 10.1007/s10344-017-1142-1
Gaynor K. M., Hojnowski C. E., Carter N. H., Brashares J. S. (2018). The Influence of Human Disturbance on Wildlife Nocturnality. Science 360, 1232–1235. doi: 10.1017/S1473550416000240
Geman S., Geman D. (1984). Stochastic Relaxation, Gibbs Distributions, and the Bayesian Restoration of Images. IEEE Trans. Pattern Anal. Mach. Intell. PAMI 6, 721–741. doi: 10.1109/TPAMI.1984.4767596
Harihar A., Ghosh-harihar M., Macmillan D. C. (2014). Human Resettlement and Tiger Conservation – Socio-Economic Assessment of Pastoralists Reveals a Rare Conservation Opportunity in a Human-Dominated Landscape. Biol. Conserv. 169, 167–175. doi: 10.1016/j.biocon.2013.11.012
Harmsen B. J., Foster R. J., Silver S., Ostro L., Doncaster C. P. (2010). Differential Use of Trails by Forest Mammals and the Implications for Camera-Trap Studies: A Case Study From Belize. Biotropica 42, 126–133. doi: 10.1111/j.1744-7429.2009.00544.x
Haswell P. M., Kusak J., Jones K. A., Hayward M. W. (2020). Fear of the Dark? A Mesopredator Mitigates Large Carnivore Risk Through Nocturnality, But Humans Moderate the Interaction. Behav. Ecol. Sociobiol. 74, 1–9. doi: 10.1007/s00265-020-02831-2
Hines J. E., USGS-PWRC (2006) PRESENCE- Software to Estimate Patch Occupancy and Related Parameters. Available at: http://www.mbr-pwrc.usgs.gov/software/presence.html.
Inskip C., Zimmermann A. (2009). Human-Felid Conflict: A Review of Patterns and Priorities Worldwide. Oryx 43, 18–34. doi: 10.1017/S003060530899030X
Islam M. A., Quli S. M. S. (2017). The Role of Non-Timber Forest Products (NTFPs) in Tribal Economy of Jharkhand, India. Int. J. Curr. Microbiol. Appl. Sci. 6, 2184–2195. doi: 10.20546/ijcmas.2017.610.259
Jhala Y., Gopal R., Mathur V., Ghosh P., Negi H. S., Narain S., et al. (2021). Recovery of Tigers in India: Critical Introspection and Potential Lessons. People Nat. 3, 281–293. doi: 10.1002/pan3.10177
Jhala Y. V., Qureshi Q., Gopal R. (2015). The Status of Tigers, Cpredators & Prey in India 2014 (Dehradun: National Tiger Conservation Authority, New Delhi & Wildlife Institute of India).
Joshi A. R., Dinerstein E., Wikramanayake E., Anderson M. L., Olson D., Jones B. S., et al. (2016). Tracking Changes and Preventing Loss in Critical Tiger Habitat. Sci. Adv. 2, 1–8. doi: 10.1126/sciadv.1501675
Joshi A. R., Smith J. L. D., Garshelis D. L. (1999). Sociobiology of the Myrmecophagous Sloth Bear in Nepal. Can. J. Zool. 77, 1690–1704. doi: 10.1139/z99-131
Karanth K. K., Gopalaswamy A. M., Defries R., Ballal N. (2012). Assessing Patterns of Human-Wildlife Conflicts and Compensation Around a Central Indian Protected Area. PLoS One 7, 1–13. doi: 10.1371/journal.pone.0050433
Karanth K. U., Gopalaswamy A. M., Kumar N. S., Vaidyanathan S., Nichols J. D., Mackenzie D. I. (2011). Monitoring Carnivore Populations at the Landscape Scale: Occupancy Modelling of Tigers From Sign Surveys. J. Appl. Ecol. 48, 1048–1056. doi: 10.1111/j.1365-2664.2011.02002.x
Kays R., Parsons A. W., Baker M. C., Kalies E. L., Forrester T., Costello R., et al. (2017). Does Hunting or Hiking Affect Wildlife Communities in Protected Areas? J. Appl. Ecol. 54, 242–252. doi: 10.1111/1365-2664.12700
Kolowski J. M., Forrester T. D. (2017). Camera Trap Placement and the Potential for Bias Due to Trails and Other Features. PLoS One 12, 1–20. doi: 10.1371/journal.pone.0186679
Kovach W. L. (2011). Oriana–circular Statistics for Windows, Ver. 4 (Pentraeth, Wales, UK: Kovach Computer Service).
Kumar R., Kumar J., Siddiqui M. H. (2018). Studies on Production and Collection Aspects of Mahua (Madhuca latifolia) in Jharkhand. J. Pharmacognsy. Phytochem. SP1, 2154–2158.
Kumar V., Rao R. (2007). Some Interesting Indigenous Beverages Among the Tribals of Central India. Indian J. Tradit. Knowl. 6, 141–143.
Ladle A., Steenweg R., Shepherd B., Boyce M. S. (2018). The Role of Human Outdoor Recreation in Shaping Patterns of Grizzly Bear-Black Bear Co-Occurrence. PLoS One 13, 1–16. doi: 10.1371/journal.pone.0191730
Lasgorceix A., Kothari A. (2009). Displacement and Relocation of Protected Areas: A Synthesis and Analysis of Case Studies. Econ. Polit. Wkly. 44, 37–47. doi: 10.2307/25663860
Laurie A., Seidensticker J. (1977). Behavioural Ecology of the Sloth Bear (Melursus Ursinus). J. Zool. 182, 187–204. doi: 10.1111/j.1469-7998.1977.tb04155.x
Linkie M., Ridout M. S. (2011). Assessing Tiger-Prey Interactions in Sumatran Rainforests. J. Zool. 284, 224–229. doi: 10.1111/j.1469-7998.2011.00801.x
MacKenzie D. I., Nichols J. D., Royle J. A., Pollock K. H., Bailey L. L., Hines J. E. (2017). Occupancy Estimation and Modeling: Inferring Patterns and Dynamics of Species Occurrence, 2nd ed. (London, United Kingdom: Academic Press). doi: 10.1016/C2012-0-01164-7
Madhusudan M. D. (2003). Living Amidst Large Wildlife: Livestock and Crop Depredation by Large Mammals in the Interior Villages of Bhadra Tiger Reserve, South India. Environ. Manage. 31, 466–475. doi: 10.1007/s00267-002-2790-8
Madhusudan M. D., Karanth U. K. (2002). Local Hunting and the Conservation of Large Mammals in India. Ambio 31, 49–54. doi: 10.1579/0044-7447-31.1.49
Meredith M., Ridout M. S. (2021) Overlap: Estimates of Coefficient of Overlapping for Animal Activity Patterns. R Package Version 0.3.4. Available at: https://CRAN.R-project.org/package=overlap.
Meredith M., Ridout M. S. (2014) Overview of the overlap package. Available at: https://cran.microsoft.com/snapshot/2016-08-05/web/packages/overlap/vignettes/overlap.pdf.
Messmer T. A. (2000). The Emergence of Human-Wildlife Conflict Management: Turning Challenges Into Opportunities. Int. Biodeterior. Biodegrad. 45, 97–102. doi: 10.1016/S0964-8305(00)00045-7
Messmer T. (2009). Human-Wildlife Conflicts: Emerging Challenges and Opportunities. Human-Wildl. Interact. 3, 10–17.
Mewada T. P. (2012). Mahua Flowers and Intoxicating Brew Bring Sloth Bears in Conflict With Tribal People in Banaskantha District of North Gujarat, India. Int. Bear. News 21, 20–21.
Miller A. B., Leung Y. F., Kays R. (2017). Coupling Visitor and Wildlife Monitoring in Protected Areas Using Camera Traps. J. Outdoor. Recreat. Tour. 17, 44–53. doi: 10.1016/j.jort.2016.09.007
Mishra A., Poonia A. (2019). Mahua (Madhuca Indica): A Boon for Tribal Economy. Indian Food Ind. Mag. 38, 19–25. doi: 10.13140/RG.2.2.23132.74888
Morales-González A., Ruiz-Villar H., Ordiz A., Penteriani V. (2020). Large Carnivores Living Alongside Humans: Brown Bears in Human-Modified Landscapes. Glob. Ecol. Conserv. 22, 1–13. doi: 10.1016/j.gecco.2020.e00937
Mori E., Bagnato S., Serroni P., Sangiuliano A., Rotondaro F., Marchianò V., et al. (2020). Spatiotemporal Mechanisms of Coexistence in an European Mammal Community in a Protected Area of Southern Italy. J. Zool. 310, 232–245. doi: 10.1111/jzo.12743
Nickel B. A., Suraci J. P., Allen M. L., Wilmers C. C. (2020). Human Presence and Human Footprint Have non-Equivalent Effects on Wildlife Spatiotemporal Habitat Use. Biol. Conserv. 241, 1–11. doi: 10.1016/j.biocon.2019.108383
Nyhus P. J. (2016). Human-Wildlife Conflict and Coexistence. Annu. Rev. Environ. Resour. 41, 143–71. doi: 10.1146/annurev-environ-110615-085634
O’Brien T. G., Kinnaird M. F., Wibisono H. T. (2003). Crouching Tigers, Hidden Prey: Sumatran Tiger and Prey Populations in a Tropical Forest Landscape. Anim. Conserv. 6, 131–139. doi: 10.1017/S1367943003003172
Ordiz A., Støen O. G., Delibes M., Swenson J. E. (2011). Predators or Prey? Spatio-Temporal Discrimination of Human-Derived Risk by Brown Bears. Oecologia 166, 59–67. doi: 10.1007/s00442-011-1920-5
Pack S., Golden R., Walker A. (2013). Comparison of National Wildlife Management Strategies: What Works Where, and Why (St. Rosa, CA, USA: Wildl. Consult. Assoc).
Palei H. S., Debata S., Sahu H. K. (2020). Diet of Sloth Bear in an Agroforest Landscape in Eastern India. Agrofor. Syst. 94, 269–279. doi: 10.1007/s10457-019-00389-1
Parsons A. W., Bland C., Forrester T., Baker-Whatton M. C., Schuttler S. G., McShea W. J., et al. (2016). The Ecological Impact of Humans and Dogs on Wildlife in Protected Areas in Eastern North America. Biol. Conserv. 203, 75–88. doi: 10.1016/j.biocon.2016.09.001
Paudel R. P., Kadariya R., Lamichhane B. R., Subedi N., Sashika M., Shimozuru M., et al. (2022). Habitat Occupancy of Sloth Bear Melursus Ursinus in Chitwan National Park, Nepal. Ecol. Evol. 12, 1–14. doi: 10.1002/ece3.8699
Pease B. S., Nielsen C. K., Holzmueller E. J. (2016). Single-Camera Trap Survey Designs Miss Detections: Impacts on Estimates of Occupancy and Community Metrics. PLoS One 11, 1–14. doi: 10.1371/journal.pone.0166689
Penjor U., Tan C. K. W., Wangdi S., Macdonald D. W. (2019). Understanding the Environmental and Anthropogenic Correlates of Tiger Presence in a Montane Conservation Landscape. Biol. Conserv. 238, 108196. doi: 10.1016/j.biocon.2019.108196
Pianka E. R. (1974). Niche Overlap and Diffuse Competition. Proc. Natl. Acad. Sci. 71, 2141–2145. doi: 10.1073/pnas.71.5.2141
Pokharel M., Subba A., Rai D., Bhandari S., Ghimirey Y. (2022). Fine-Scale Ecological and Anthropogenic Variables Predict the Habitat Use and Detectability of Sloth Bears in the Churia Habitat of East Nepal. Ecol. Evol. 12, 1–12. doi: 10.1002/ece3.8512
Prajapati U., Koli V. K., Sundar K. S. G. (2021). Vulnerable Sloth Bears are Attracted to Human Food Waste: A Novel Situation in Mount Abu Town, India. Oryx, 1–9. doi: 10.1017/S0030605320000216
Rajpurohit K. S., Krausman P. R. (2000). Hunan-Sloth Bear Conflicts in Madhya Pradesh, India. Wildl. Soc Bull. 28, 393–399. doi: 10.2307/3783697
Ramesh T., Kalle R., Sankar K., Qureshi Q. (2012a). Factors Affecting Habitat Patch Use by Sloth Bears in Mudumalai Tiger Reserve, Western Ghats, India. Ursus 23, 78–85. doi: 10.2192/URSUS-D-11-00006.1
Ramesh T., Kalle R., Sankar K., Qureshi Q. (2012b). Spatio-Temporal Partitioning Among Large Carnivores in Relation to Major Prey Species in Western Ghats. J. Zool. 287, 269–275. doi: 10.1111/j.1469-7998.2012.00908.x
Ramesh T., Kalle R., Sankar K., Qureshi Q. (2013). Activity Pattern of Sloth Bear Melursus Ursinus (Mammalia: Ursidae) in Mudumalai Tiger Reserve, Western Ghats, India. J. Threat. Taxa. 5, 3989–3992. doi: 10.11609/JoTT.o3071.3989-92.Copyright
R Core Team (2019) R: A Language and Environment for Statistical Computing. Available at: http://www.R-project.org/ (Accessed 20 February 2020).
Redpath S. M., Young J., Evely A., Adams W. M., Sutherland W. J., Whitehouse A., et al. (2013). Understanding and Managing Conservation Conflicts. Trends Ecol. Evol. 28, 100–109. doi: 10.1016/j.tree.2012.08.021
Richmond O. M. W., Hines J. E., Beissinger S. R. (2010). Two-Species Occupancy Models: A New Parameterization Applied to Co-Occurrence of Secretive Rails. Ecol. Appl. 20, 2036–2046. doi: 10.1890/09-0470.1
Ridout M. S., Linkie M. (2009). Estimating Overlap of Daily Activity Patterns From Camera Trap Data. J. Agric. Biol. Environ. Stat. 14, 322–337. doi: 10.1198/jabes.2009.08038
Rode K. D., Farley S. D., Fortin J., Robbins C. T. (2007). Nutritional Consequences of Experimentally Introduced Tourism in Brown Bears. J. Wildl. Manage. 71, 929–939. doi: 10.2193/2006-075
Rodgers W. A., Panwar H. S. (1988). Planning a Wildlife Protected Area Network in India (Dehradun: Wildlife Institute of India).
Rovero F., Marshall A. R. (2009). Camera Trapping Photographic Rate as an Index of Density in Forest Ungulates. J. Appl. Ecol. 46, 1011–1017. doi: 10.1111/j.1365-2664.2009.01705.x
Sathyakumar S., Kaul R., Ashraf N. V. K., Mookherjee A., Menon V. (2012). National Bear Conservation and Welfare Action Plan (India: Ministry of Environment and Forests, Wildlife Institute of India, and Wildlife Trust of India).
Sharp T. R., Swaminathan S., Arun A. S., Smith T., Satyanarayan K., Seshamani G. (2020). Sloth Bear Attacks on the Deccan Plateau of Karnataka, India. Ursus 31, 1–11. doi: 10.2192/URSUS-D-18-00027.3
Singh N., Sonone S., Dharaiya N. (2018). Sloth Bear Attacks on Humans in Central India: Implications for Species Conservation. Human-Wildl. Interact. 12, 338–347. doi: 10.26077/2mgq-fs29
Sodhi N. S., Posa M. R. C., Lee T. M., Bickford D., Koh L. P., Brook B. W. (2010). The State and Conservation of Southeast Asian Biodiversity. Biodivers. Conserv. 19, 317–328. doi: 10.1007/s10531-009-9607-5
Srivastava N., Krishnamurthy R., Sathyakumar S. (2020). Avoidance or Coexistence? The Spatiotemporal Patterns of Wild Mammals in a Human-Dominated Landscape in the Western Himalaya. Mt. Res. Dev. 40, R20–R31. doi: 10.1659/MRD-JOURNAL-D-19-00046.1
Stevenson M. D., Rossmo D. K., Knell R. J., Le Comber S. C. (2012). Geographic Profiling as a Novel Spatial Tool for Targeting the Control of Invasive Species. Ecography 35, 704–715. doi: 10.1111/j.1600-0587.2011.07292.x
Struebig M. J., Linkie M., Deere N. J., Martyr D. J., Millyanawati B., Faulkner S. C., et al. (2018). Addressing Human-Tiger Conflict Using Socio-Ecological Information on Tolerance and Risk. Nat. Commun. 9, 1–9. doi: 10.1038/s41467-018-05983-y
Suraci J. P., Clinchy M., Zanette L. Y., Wilmers C. C. (2019a). Fear of Humans as Apex Predators has Landscape-Scale Impacts From Mountain Lions to Mice. Ecol. Lett. 22, 1578–1586. doi: 10.1111/ele.13344
Suraci J. P., Frank L. G., Oriol-Cotterill A., Ekwanga S., Williams T. M., Wilmers C. C. (2019b). Behavior-Specific Habitat Selection by African Lions may Promote Their Persistence in a Human-Dominated Landscape. Ecology 100, 1–11. doi: 10.1002/ecy.2644
Sutherland W. J., Adams W. M., Aronson R. B., Aveling R., Blackburn T. M., Broad S., et al. (2009). One Hundred Questions of Importance to the Conservation of Global Biological Diversity. Conserv. Biol. 23, 557–567. doi: 10.1111/j.1523-1739.2009.01212.x
Tablado Z., Jenni L. (2017). Determinants of Uncertainty in Wildlife Responses to Human Disturbance. Biol. Rev. 92, 216–233. doi: 10.1111/brv.12224
Tanwar K. S., Sadhu A., Jhala Y. V. (2021). Camera Trap Placement for Evaluating Species Richness, Abundance, and Activity. Sci. Rep. 11, 1–12. doi: 10.1038/s41598-021-02459-w
Tollefson T. N., Matt C., Meehan J., Robbins C. T. (2005). Quantifying Spatiotemporal Overlap of Alaskan Brown Bears and People. J. Wildl. Manage. 69, 810–817. doi: 10.2193/0022-541X(2005)069[0810:QSOOAB]2.0.CO;2
Treves A., Karanth K. U. (2003). Human-Carnivore Conflict and Perspectives on Carnivore Management Worldwide. Conserv. Biol. 17, 1491–1499. doi: 10.1111/j.1523-1739.2003.00059.x
Treves A., Wallace R. B., Naughton-Treves L., Morales A. (2006). Co-Managing Human-Wildlife Conflicts: A Review. Hum. Dimens. Wildl. 11, 383–396. doi: 10.1080/10871200600984265
Tucker M. A., Böhning-gaese K., Fagan W. F., Fryxell J. M., Moorter B.V., Alberts S. C., et al. (2018). Moving in the Anthropocene: Global Reductions in Terrestrial Mammalian Movements. Science 359, 466–469. doi: 10.1126/science.aam9712
Verity R., Stevenson M. D., Rossmo D. K., Nichols R. A., Le Comber S. C. (2014). Spatial Targeting of Infectious Disease Control: Identifying Multiple, Unknown Sources. Methods Ecol. Evol. 5, 647–655. doi: 10.1111/2041-210X.12190
Wang Y., Allen M. L., Wilmers C. C. (2015). Mesopredator Spatial and Temporal Responses to Large Predators and Human Development in the Santa Cruz Mountains of California. Biol. Conserv. 190, 23–33. doi: 10.1016/j.biocon.2015.05.007
Watson G. S. (1961). Goodness-Of-Fit Tests on a Circle. Biometrika 48, 109–114. doi: 10.1093/biomet/48.1-2.109
Watson G. S. (1962). Goodness-Of-Fit Tests on a Circle. II. Biometrika 49, 57–63. doi: 10.1093/biomet/49.1-2.57
Wearn O. R., Rowcliffe J. M., Carbone C., Bernard H., Ewers R. M. (2013). Assessing the Status of Wild Felids in a Highly-Disturbed Commercial Forest Reserve in Borneo and the Implications for Camera Trap Survey Design. PLoS One 8, 1–9. doi: 10.1371/journal.pone.0077598
Xiao W., Hebblewhite M., Robinson H., Feng L., Zhou B., Mou P., et al. (2018). Relationships Between Humans and Ungulate Prey Shape Amur Tiger Occurrence in a Core Protected Area Along the Sino-Russian Border. Ecol. Evol. 8, 11677–11693. doi: 10.1002/ece3.4620
Yang H., Han S., Xie B., Mou P., Kou X., Wang T., et al. (2019). Do Prey Availability, Human Disturbance and Habitat Structure Drive the Daily Activity Patterns of Amur Tigers (Panthera Tigris Altaica)? J. Zool. 307, 131–140. doi: 10.1111/jzo.12622
Yoganand K. (2005). Behavioural Ecology of Sloth Bear (Melursus Ursinus) In Panna National Park, Central India. [Dissertation/Master's Thesis] (Rajkot, Gujarat, India: Saurashtra University).
Yoganand K., Rice C. G., Johnsingh A. J. T., Seidensticker J. (2006). Is the Sloth Bear in India Secure? A Preliminary Report on Distribution, Threats and Conservation Requirements. J. Bombay. Nat. Hist. Soc 103, 172–181.
Zhang J., Ding Q., Huang J. (2013) Spaa: Species Association Analysis. R Package Version 0.2.1. Available at: https://github.com/helixcn/spaa.
Keywords: camera trap survey, human-dominated landscape, interaction, conflict hotspot, Melursus ursinus, geographic profiling
Citation: Chaudhuri S, Bandyopadhyay M, Rajaraman R, Kalyanasundaram S, Sathyakumar S and Krishnamurthy R (2022) Spatio-Temporal Patterns and Source-Dispersion Modeling Towards Sloth Bear–Human Conflict Management in Central India. Front. Conserv. Sci. 3:850309. doi: 10.3389/fcosc.2022.850309
Received: 07 January 2022; Accepted: 09 May 2022;
Published: 17 June 2022.
Edited by:
Alexandra Zimmermann, University of Oxford, United KingdomReviewed by:
Himanshu Shekhar Palei, North Orissa University, IndiaCopyright © 2022 Chaudhuri, Bandyopadhyay, Rajaraman, Kalyanasundaram, Sathyakumar and Krishnamurthy. This is an open-access article distributed under the terms of the Creative Commons Attribution License (CC BY). The use, distribution or reproduction in other forums is permitted, provided the original author(s) and the copyright owner(s) are credited and that the original publication in this journal is cited, in accordance with accepted academic practice. No use, distribution or reproduction is permitted which does not comply with these terms.
*Correspondence: Ramesh Krishnamurthy, cmFtZXNoQHdpaS5nb3YuaW4=; Sankarshan Chaudhuri, Y2hhdWRodXJpc2Fua2Fyc2hhbkBnbWFpbC5jb20=
Disclaimer: All claims expressed in this article are solely those of the authors and do not necessarily represent those of their affiliated organizations, or those of the publisher, the editors and the reviewers. Any product that may be evaluated in this article or claim that may be made by its manufacturer is not guaranteed or endorsed by the publisher.
Research integrity at Frontiers
Learn more about the work of our research integrity team to safeguard the quality of each article we publish.