- 1Reneco International Wildlife Consultants, LLC, Abu Dhabi, United Arab Emirates
- 2Emirate Center for Wildlife Propagation, Missour, Morocco
- 3Emirates Centre for the Conservation of Houbara, Navoi, Uzbekistan
In conservation translocation, released animals should have comparable fitness to their wild counterparts to effectively contribute to the species demography. Captive-bred animals frequently exhibit lower fitness performances, which can often be attributed to an inadequate release strategy. Untimely release of migrant animals may interfere with key events such as their migration and breeding. In Kazakhstan and Uzbekistan, declining wild populations of Asian houbara (Chlamydotis macqueenii) are reinforced in their breeding grounds with captive-bred individuals. Using data from 6 years of monitoring, we compare eight breeding traits and the productivity of wild and captive-bred females released in two distinct seasons (autumn vs. spring) considering the effects of age and time in the season. Females released in the spring nest prior to their first migration, and females released in the autumn nest following their first migration. Our results highlight that captive-bred and wild females have similar breeding traits and productivity. Breeding probability, laying date, and egg volume varied, depending on the release season and female age. One-year-olds released in autumn have a significantly lower breeding probability compared to wild and spring-released females. However, 1-year-old females released in the spring nest later and lay smaller eggs than wild and autumn-released birds; effects which appear to be carried over with age. Age has a positive effect on breeding probability, egg volume, re-clutching probability and advancement of nesting date. These findings suggest a complex interplay of release timing with migration and breeding, resulting in short- and long-term effects on population demography, emphasizing its importance in conservation translocation.
Introduction
Captive-breeding and animal translocation are increasingly being used to restore declining populations (Griffith et al., 1989; Wolf et al., 1996; Fischer and Lindenmayer, 2000; Sheean et al., 2012). Such conservation initiatives are upheld by advancements in artificial breeding and rearing techniques, resulting in more captive-bred animals being readily available for conservation (Armstrong and Seddon, 2008). Theoretical studies have questioned the contribution of captive-bred released animals to wild populations' demography, with reported evidence on their lower fitness in comparison to their wild counterparts, resulting from genetic and non-genetic issues. Genetic issues include outbreeding depression (Huff et al., 2011), inbreeding and drift loads (Kirchner et al., 2006; Robert, 2011), as well as adaptation to captivity (Lynch and O'Hely, 2001; Araki et al., 2007; Frankham, 2008). Non-genetic issues are associated with rearing and release processes, leading to a complex set of interactions between individual phenotypes with their new environment. Environmental conditions such as food availability, weather conditions, conspecific interaction (Hardouin et al., 2014, 2015; Robert et al., 2015), and predator naivete (Ross et al., 2019) have all been documented as important factors affecting released individual performances. These are also expected to vary temporally, and timing of management activities may affect individuals and populations in numerous and unforeseen ways (Facka et al., 2016).
Timing can indeed be critical for many life-history events of animals, and failure to consider how it influences management actions may jeopardize efforts to restore species or populations (Bright and Morris, 1994; Facka et al., 2016). In translocation programs, the timing of release is therefore a key parameter to consider in the selected tactic (Tavecchia et al., 2009; Batson et al., 2015; Wilson et al., 2020), although it has hitherto received little attention in analysis and planning (Griffith et al., 1989; Armstrong and Seddon, 2008). This is particularly true for migratory species, where the untimely release of animals may interfere with key life history stages (fattening, molt, migration, or breeding), and individuals may then experience subsequent effects on their physiology, survival rates or reproduction (Parker et al., 2012; Jachowski et al., 2016; Teitelbaum et al., 2019a).
Release of captive-bred animals is a major event in their life histories because it is associated with substantial energy demands due to adaptation to a novel habitat and post-release stress (Dickens et al., 2010; Hamilton et al., 2010). Similarly, migration is a high energy demanding stage in birds' annual life cycle, resulting in significant physiological effects on metabolic rate, body mass, and size of several organs (Wikelski et al., 2003; McKechnie, 2008). Likewise, the reproductive stage has an indispensable cost on breeders' fitness (Williams, 2012). The successful integration of these successive life stages is usually regulated by trade-off or carry-over mechanisms which ultimately shape fitness of migrants (Schmidt-Wellenburg et al., 2008; Fayet et al., 2016). Therefore, timing of release of captive-bred animals relative to their key life stages remains one of the critical questions facing translocations of migrant species because it can have long lasting effects on individual fitness, population demography, and, importantly, the success of the translocation (Facka et al., 2016; Teitelbaum and Mueller, 2019).
The Asian houbara bustard, Chlamydotis macqueenii, (hereafter “houbara”), is a medium-sized bustard which is classified as vulnerable (BirdLife International, 2017) due to significant populations decline caused by the continuous increase in unregulated hunting and poaching across its range, associated with habitat loss and degradation (Riou et al., 2011). The species has an extensive range, with resident and migratory populations (Riou et al., 2012). Migrant houbara have a wide breeding range across the deserts and semi-deserts of Central Asia, extending from northern Iran and the eastern Caspian Sea through Uzbekistan and Kazakhstan to the Gobi Desert in China and Mongolia (Gubin, 2008). Migrants use three migration routes described by Combreau et al. (2011) to spend the winter in the southern part of their distribution range, which stretches from east Iraq to west India, across the south of Iran and Pakistan with some long-distance migrant wintering in the Arabian Peninsula. In Central Asia, comprehensive conservation programs have been implemented, combining ex-situ (conservation breeding) and in-situ measures (e.g., reinforcement, hunting regulation, education programs) to mitigate the decline of wild migrant populations. Similarly to the African houbara bustard (Chlamydotis undulata undulata) translocation program in Morocco (Lacroix et al., 2003), an intensive long-term monitoring of wild and captive-bred released individuals has been carried out. In Morocco, studies have revealed that the timing of release influences population demographics. While African houbara released in spring had better short-term survival (Hardouin et al., 2014), they had lower breeding performances compared to autumn releases and wild-born individuals (Bacon et al., 2018). These variations in breeding parameters were observed at 1 year old but also later in life, suggesting a long-lasting effect of the timing of release. Houbara are released as juveniles (<1-year-old), and after spending several months in aviaries without parental care, they complete their growth and learning in the wild, which is a completely novel environment for them. Such early life environment and experience may then be determinant later in life, for example, influencing movement pattern (migration), interactions with conspecifics, with other species [predator naivete, (Ross et al., 2019)], and in fine individual performances (Whiteside et al., 2015; Brandl et al., 2019).
In this study we test the effect of timing of release on the reproductive traits and productivity of migrant Asian houbara females in Central Asia. Two release seasons were considered: the autumn releases, completed from late August to the end of September, and the spring releases, with houbara kept in aviaries over winter and released in late March to early May of the following year. Juveniles released in autumn can nest following their first full migration when they are around 1 year old, whereas juveniles released in spring can nest shortly after their release on the breeding ground and prior to their first migration, resulting in different life histories and experiences prior to key events (breeding and migration). Assessing reproductive success requires evaluation of all composite traits involved, beginning with the female decision to breed through egg laying and incubation, and ending with chick survival traits, all of which influence productivity (Azar et al., 2018). Here, we provide the first estimates of breeding traits of captive-bred migrant houbara using a large-scale data set from 519 houbara nests monitored between 2013 and 2018 in Uzbekistan and central Kazakhstan (two regions in the core breeding range of migrant Asian houbara), and we investigate (1) the effects of female origin (wild vs. captive-bred), (2) timing of release (autumn vs. spring), and (3) age on eight breeding parameters.
Materials and Methods
Study Area
Field work was conducted from 2013 to 2018 in Betpakdala; south-central Kazakhstan (43° 47′-46° 56′ N, 65°90′-70°90′ E), and south Navoi and far-western Samarkand province, Uzbekistan (39° 22′-41°41′ N, 64°06′-66°01′ E), with a few nests located north of Navoi city toward the Kyzylkum Desert (Figure 1). Both areas are classified as deserts and xeric shrublands representing two ecoregions; Central Asian northern desert in Kazakhstan and Central Asia southern deserts in Uzbekistan (Olson et al., 2001). These areas possess a cold desert climate corresponding to a Köppen classification of “BWk” (Kottek et al., 2006). From January to July, average temperatures range from −7 to 31°C in Kazakhstan and from −2 to 35°C in Uzbekistan (Beaudoing and Rodell, 2020). Mean annual precipitation ranges between 150 and 300 mm with the northern parts receiving more rain (Mirzabaev, 2013). The topography of both areas is largely flat with elevation ranging from 200 to 300 m above sea level (Figure 1). The substratum is mainly loess silt but becomes sandier toward the Kyzylkum Desert in north Uzbekistan. The dominant vegetation is Artemisia spp. mainly A. terrae albae, and A. turanica in the northern parts, with A. kemrudica, A. diffusa, and A. dimoana becoming dominant toward the south. Sandy areas are dominated by Haloxylon persicum.
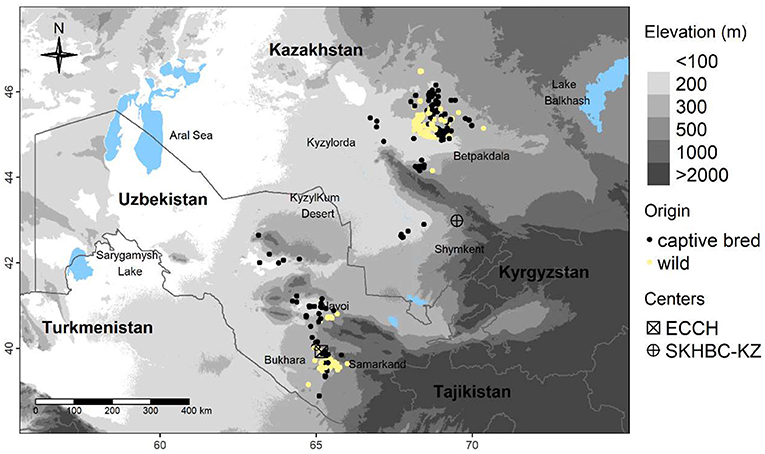
Figure 1. Nests locations in our study areas in central Kazakhstan and Uzbekistan between 2013 and 2018 from wild-born (n = 316) and captive-bred (n = 203) migrant Asian houbara (Chlamydotis macqueenii).
Reinforcement Program
Houbara released in Kazakhstan were produced by the International Fund for Houbara Conservation (IFHC, www.houbarafund.org) at the Sheikh Khalifa Houbara Breeding Center in Kazakhstan (SKHBC-KZ), or one of the two breeding Centers in the UAE; the National Avian Research Center (NARC), and the Sheik Khalifa Houbara Breeding Center (SKHBC-AD). Asian houbara released in Uzbekistan were mainly produced at the Emirates Center for Conservation of Houbara (ECCH, see Appendix A). All these conservation breeding centers are managed by Reneco International Wildlife Consultants LLC, (www.reneco.net). Captive flocks, used to reinforce wild populations, were established following egg collections from local breeding wild populations in Central Asia. Captive-bred houbara were produced using artificial insemination following strict genetic management aimed at minimizing deleterious genetic effects that might occur in captivity, while preserving populations' genetic diversity (Lesobre, 2008). Releases started in 2009 in Kazakhstan (n = 9) and 2012 in Uzbekistan (n = 23). However, larger-scale releases started in 2012 in Kazakhstan (n = 238) and 2013 in Uzbekistan (n = 159). Within the study areas, c. 30,900 individuals were released by 2020, of which c. 15,500 were released in central Kazakhstan and c. 15,400 in Uzbekistan (Appendix A), with females representing ~49% of released birds. Houbara were released in autumn on 6 September (±5 days) in Kazakhstan and 13th of September (±6 days) at a mean age of 4.5 (±0.74 SD) months, and in spring with houbara kept in aviaries over winter and released on 21st of March (±4 days) in Uzbekistan and 8th of April (±9 days) in Kazakhstan of the following year at a mean age of 11.00 (±0.97 SD) months. The IFHC and ECCH release houbara on both hunting and non-hunting grounds. Releases on designated hunting grounds reduce hunting pressure on wild individuals.
Female Monitoring
Released captive-bred houbara were marked with metal and plastic engraved rings, allowing for their individual identification through photos taken by camera traps or direct observation. In Kazakhstan, 448 females were released with solar-powered satellite platform transmitter terminals (PTT, 30 g backpack; Microwave Telemetry Inc., Columbia, MD, USA) and 31 with very high frequency solar-powered radio-transmitters (VHF, 20 g backpack style; Merlin Systems, Inc.), of which 13 were replaced with PTT in subsequent years. In Uzbekistan, 244 females were released with PTT. Wild females were trapped on nests during egg collection campaigns (2010–2013 and 2016 in Uzbekistan; and 2009–2011 and 2016–2018 in Kazakhstan) using nylon snares and were fitted with PTT (63 in Kazakhstan and 26 in Uzbekistan). These resulted in 143 nests collected in Kazakhstan and 131 in Uzbekistan. In addition, 25 wild-born females were captured as juveniles (Kazakhstan = 14, Uzbekistan = 11) and fitted with PTT using elastic Teflon harnesses (Combreau et al., 2011). PTT transmitters have no effect on breeding traits of Asian Houbara (Burnside et al., 2019).
Nest and Chick Monitoring
Nests were located either by circular observations completed by two observers using telescopes to detect females with potential nesting behavior, or by tracking transmitter-equipped females. Females with VHF were searched for twice a week to locate their nests, while coordinates of PTT tracked females were checked twice weekly in a geographic information system to identify any cluster of locations indicating potential nesting activity. Camera traps (Reconyx HC600, HC500, Wisconsin, USA; Moultrie, M-990i, Alabama, USA) were deployed at 353 nests within 1 week of discovery, allowing accurate quantification of nest fate and number of eggs hatched. Nests monitored with camera traps were visited every 10.19 (±5.14 SD) days after deployment, while those without camera traps were monitored by regular visits on average every 6.11 (±4.71 SD) days to determine nest survival and hatching rate. Only nests from identified females were considered in this study. Chicks were monitored via tracking equipped females. Individually tracked brooding females were tracked biweekly and observed with a telescope to count the number of alive chicks or to determine whether the entire brood was lost based on females' behavior (for example, movement patterns, vigilance). Only brood with known hatching dates and number of chicks hatched were considered for analysis.
Breeding Parameters
Eight parameters were evaluated: (1) breeding probability, estimated from tracked females monitored for a minimum of 60 days on breeding range, (2) nest initiation date, measured for all nesting attempts, as day of year (DOY), estimated from the mean incubation stages of all eggs in the clutch (Combreau et al., 2002) or by subtracting the species average incubation period (23 days) from the observed hatching date, (3) egg volume, calculated following Hoyt (1979), (4) clutch size, given as the maximum number of eggs observed in a nest, (5) daily nest survival (DNS), the daily probability of a nest to survive, (6) hatching rate, the proportion of hatched eggs to clutch size, (7) female re-clutching probability following nest failure, estimated from tracked females, (8) chick survival, estimated from tracked females seen with chicks after hatching. Productivity (number of chicks per female on breeding range), was the product of breeding probability, clutch size, nest survival, hatching rate, and chick survival (Azar et al., 2018).
Statistical Analysis
Breeding parameters were modeled using generalized linear mixed models conducted in the “glmmTMB” package (Brooks et al., 2017) in R v.2.15.1 (R Development Core Team, 2015). To compare breeding traits between wild-born and captive-bred houbara released in spring and autumn, a dummy variable “origin” containing three levels was used as a predictor. Age groups (1 and ≥2 years), study areas (Kazakhstan and Uzbekistan), and “origin” (wild, spring-released captive, and autumn-released captive) were main predictors used in all models. Female identity was used as a random effect in all models to account for repeated measures, except for DNS and chick survival. Breeding probability had binomial responses (1 nested/0 no nest) and logit link functions. Nest initiation date and egg volume were fitted with gaussian distributions and identity link functions. Nest initiation date and clutch size were additional predictors in egg volume models, with nest identity used as an additional random effect. Clutch size was fitted with the Conway-Maxwell-Poisson distribution (compois) to account for under dispersion with log link function, and nest initiation date as additional predictors. Hatching rate had a compois distribution and a log link function, with log clutch size used as an offset and nest initiation date as additional predictor. DNS models had binomial responses (1 alive/0 dead) encoded from date of nest discovery until last known nest fate using logistic exposure method (Shaffer, 2004), and time in season (DOY) in its linear and quadratic forms used as additional predictors. Nest identity was used as a random factor. Probability of re-clutching constituted a binomial response (1 re-nested/0 no re-nest) and a logit link function with time in season of failure of previous nest as an additional predictor. Bi-weekly chick encounter histories were compiled from identified brooding females with known hatching date and rates. Chick survival was estimated using the logistic exposure method, with a binomial response (1 alive/0 dead) encoded from the date of nest hatching to the last known chick fate within 2 months of hatching. Female identity was used as a random factor to account for pseudo replication. Time since hatching was then grouped into 2 monthly occasions and used as a predictor. Using the “dredge” function in the “MuMIn” package (Barton, 2019), all subsets of models were generated from global models. Akaike's information criterion corrected for small sample sizes, QAICc was used to rank models, and ΔQAICc and Akaike weights (w) were used to assess model likelihood and uncertainty (Burnham and Anderson, 2010). Competing models (≤ 2 ΔQAICc) were averaged to calculate parameter estimates and relative variable importance (RVI). Model-averaged parameters were considered strongly supported when their 95% unconditional confidence intervals did not span zero. Normality and homoscedasticity of the random residuals were checked graphically for all global models. All continuous predictors were scaled and centralized by subtracting the mean and dividing by the standard deviation. To account for the uncertainty in the estimated productivity, we generated 1,000 iteration values for clutch size using beta estimates and variance covariance matrix, and for other breeding traits using β-distributions (Schaub et al., 2009).
Results
Between 2013 and 2018, 774 nests were found (Figure 1) belonging to wild-born (316), captive-bred (203) or unidentified (255) (excluded from analysis) female origins. Nests of identified females belonged to 351 distinct females tracked by PTT (275), VHF (39), or identified by their engraved plastic rings (37). Nests of one-year-old females belonged to 11 wild-born houbara that nested after their first migration, 111 captive-bred houbara released in spring and nested directly after release and prior to their first migration, and 20 captive-bred houbara released in autumn and nested in the following calendar year after their first migration. Nests of females ≥2 years old belonged to wild-born (305), captive-bred released in spring (43) and captive-bred released in autumn (32), all completing at least one spring migration. Summaries of breeding parameters are presented in Table 1 and top models in Appendix B.
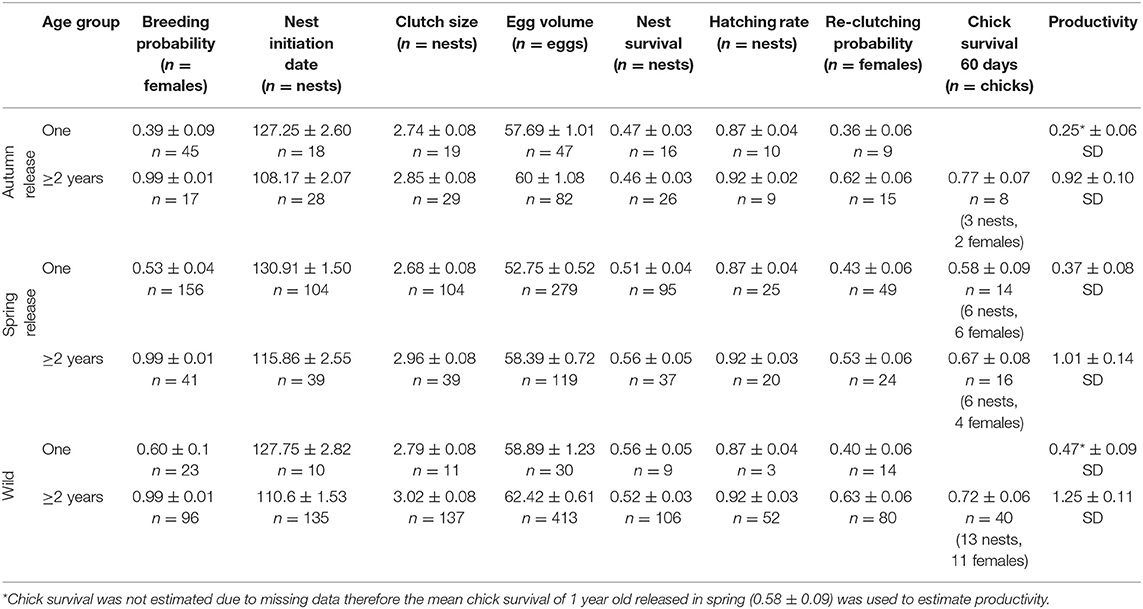
Table 1. Mean (± SE) breeding parameters for wild, and captive-bred migrant Asian houbara (Chlamydotis macqueenii) released in autumn (late August to the end of September) and spring (late March to early May of the following year) and from two age groups: one (females <2 years old) and ≥2 years (females two or more years old).
Female Breeding Probability
Mean breeding probabilities of 1-year-old females were similar between wild-born and captive-bred birds released in spring and both were significantly higher than captive-bred birds released in autumn (Figure 2). Mean breeding probability was positively influenced by age and for females ≥2 years was high (0.99 ± 0.01 SE) and similar, irrespective of bird origin. Area appeared in top models however its effect was not significant (Table 2).
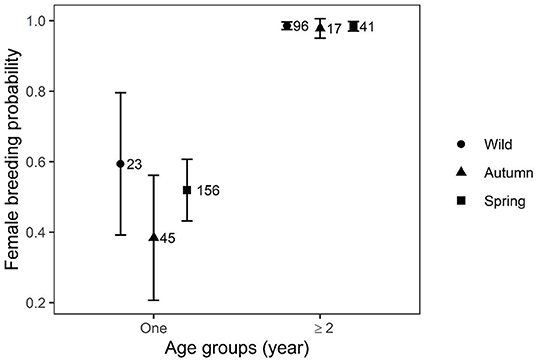
Figure 2. Predictions of female mean breeding probabilities (± CI) of wild-born and captive-bred migrant Asian houbara (Chlamydotis macqueenii) released in spring and autumn.
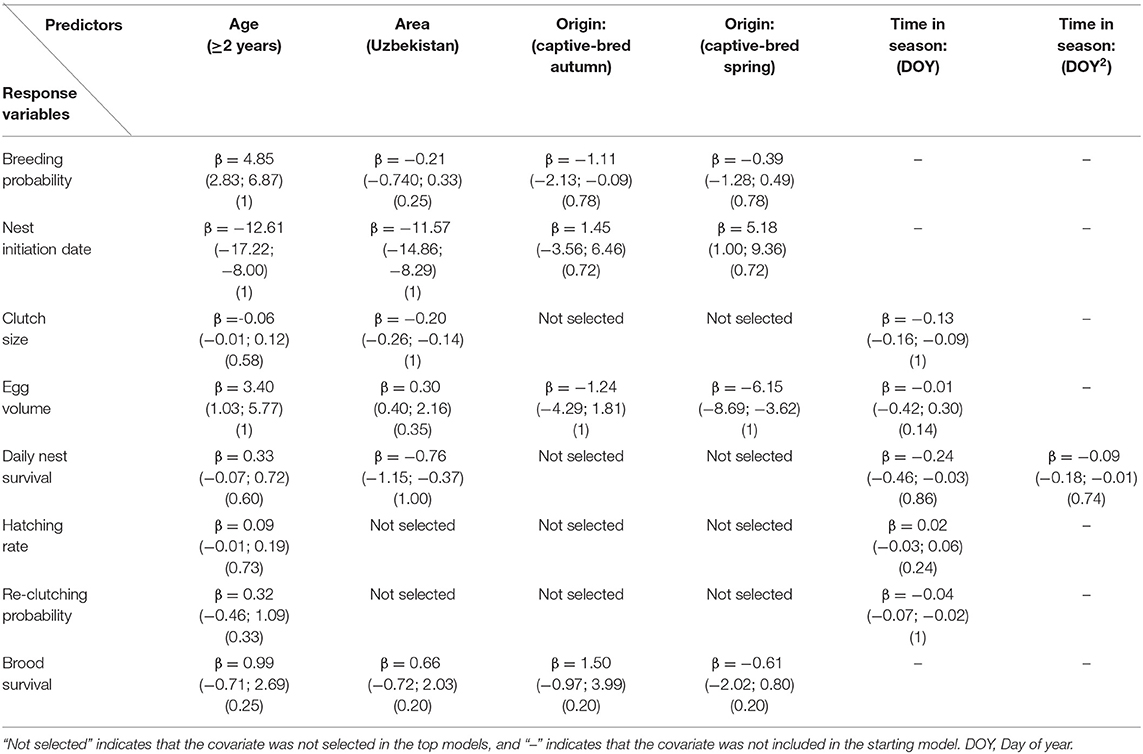
Table 2. Estimated beta coefficients (β) (± 95% CIs) and relative variable importance (RVI) for variables selected in the best models (ΔAICc ≤ 2) with respect to breeding parameters of migrant Asian houbara (Chlamydotis macqueenii) in Central Asia.
Nest Initiation Date
Laying dates ranged between 10 March and 25 May in Uzbekistan and 28 March−10 June in Kazakhstan. Top models of nest initiation date showed strong effects of nesting area, female age, and origin (Table 2). Females nested (17 days) earlier in Uzbekistan and older females nested on average 17 days earlier than 1-year-old birds (Figure 3). There was no difference in nest timing between wild-born and autumn released birds, however, spring released birds nested significantly later.
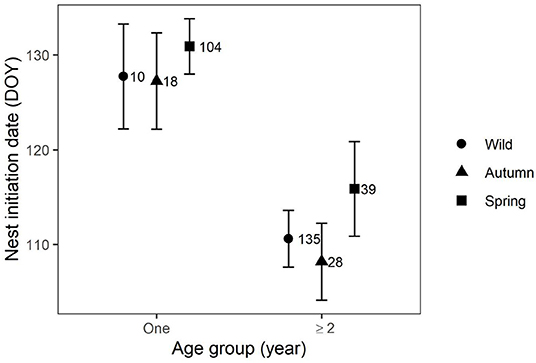
Figure 3. Predictions of mean nest initiation dates (± CI), for wild-born and captive-bred migrant Asian houbara (Chlamydotis macqueenii) released in spring and autumn from two age groups.
Clutch Size
Clutch size ranged between one to five eggs and top models had strong effects of nesting area, time in the season and age (Table 2). Females laid smaller clutches in Uzbekistan (2.77 ± 0.79 SE) than in Kazakhstan (2.97 ± 0.80 SE). Globally, clutch size decreased with the advancement in the season (Appendix C). Female age had a positive but not significant effect on clutch size and there was no difference between wild-born and captive-bred as origin did not appear in top models.
Egg Volume
Top models of egg volume included effects of origin, age, and their interaction. Wild-born and autumn released birds had similar egg volume, however birds released in spring laid significantly smaller eggs (Table 2). Female age had a positive influence on egg volume (Figure 4); however, interaction of age and release season was not significant for both autumn (RVI = 1.00, β = −1.27, 95% CI = −4.71–2.16) and spring releases (RVI = 1.00, β = −2.18, 95% CI = −0.48–4.83). Tukey post-hoc test showed a significant difference between females ≥2 years released in spring and autumn (4.00 ± 0.85, P-values < 0.001).
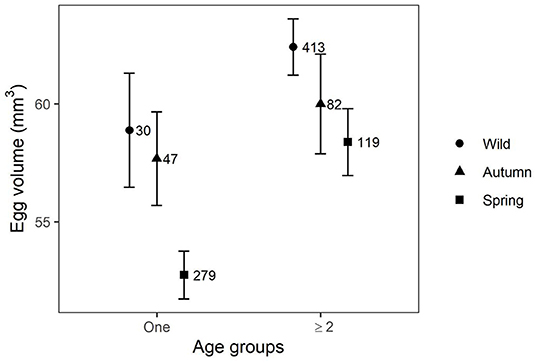
Figure 4. Predictions of mean egg volume (± CI) for wild-born and captive-bred migrant Asian houbara (Chlamydotis macqueenii) released in spring and autumn from two age groups.
Daily Nest Survival
Top models of daily DNS included effects of area, quadratic effect of DOY and age. DNS had quadratic pattern with DOY and was lower in Uzbekistan (Figure 5). Female age had a positive but not significant effect (Table 2). There was no significant difference between wild-born and captive-bred birds as origin did not appear in top models. Mean nest survival to 23 days, from the mean nest initiation date, of wild-born females was higher in Kazakhstan (1-year-old = 0.52 ± 0.04, ≥2 years = 0.63 ± 0.06) compared with Uzbekistan (1-year-old = 0.30 ± 0.02, ≥2 years = 0.42 ± 0.03). Likewise, nest survival of captive-bred birds was higher in Kazakhstan (spring: 1-year-old = 0.49 ± 0.03, ≥2 years = 0.62 ± 0.05, autumn: 1-year-old = 0.52 ± 0.04, ≥2 years = 0.63 ± 0.06) compared with Uzbekistan (spring: 1-year-old = 0.28 ± 0.02, ≥2 years = 0.41 ± 0.02, autumn: 1-year-old = 0.31 ± 0.02, ≥2 years = 0.41 ± 0.02). Mean nest survival to 23 days for all groups in Uzbekistan was 0.38 (±0.02 SE) and 0.54 (±0.04 SE) in Kazakhstan. Nests monitored with camera traps (Kazakhstan: n = 131, Uzbekistan: n = 222) allowed identification of causes of nest failure. Foxes (Vulpes spp.) were the main predators in both Kazakhstan and Uzbekistan, accounting, respectively, for 60 and 35% of failed nests. Desert monitor lizard (Varanus griseus) was present only in Uzbekistan and accounted for 23% of failed nests there (Appendix D).
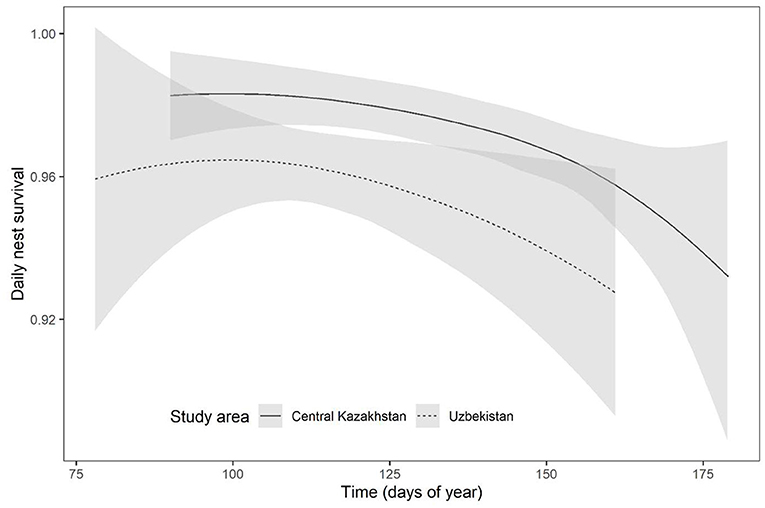
Figure 5. Predictions of mean daily nest survival (± CI) for migrant Asian houbara (Chlamydotis macqueenii) nesting in central Kazakhstan and Uzbekistan with the progress of breeding season.
Hatching Rate
Top models of hatching rate included effects of age and time in season, however their effects were not significant (Table 2). Wild-born and captive-bred birds had similar hatching rate as “origin” did not appear in top models. Mean hatching rates across all groups was 0.90 (±0.06 SE).
Re-clutching Probability
Top models of re-clutching probability included time in season and age effects. Time in season negatively influenced re-clutching probability while female age had a positive but not significant effect (Table 2).
Chick Survival
The top model included effects of age, area, origin, and months after hatching. However, none of these variables had a significant effect on chick survival (Table 2). Mean chick survival for 60 days after hatching was 0.68 (±0.08 SE).
Female Productivity
Average productivity (± SD) of wild-born females was higher in Kazakhstan (1-year-old = 0.58 ± 0.12, ≥2 years = 1.32 ± 0.19) compared with Uzbekistan (1-year-old = 0.27 ± 0.06, ≥2 years = 0.81 ± 0.09). Likewise, productivity of captive-bred birds appeared higher in Kazakhstan (spring: 1-year-old = 0.42 ± 0.07, ≥2 years =1.23 ± 0.15, autumn: 1-year-old = 0.38 ± 0.09, ≥2 years = 1.14 ± 0.16) compared with Uzbekistan (spring: 1-year-old = 0.22 ± 0.20, ≥2 years = 0.82 ± 0.09, autumn: 1-year-old = 0.20 ± 0.05, ≥2 years = 0.77 ± 0.08). We do not compare productivity statistically because of small sample size used to estimate brood survival of some groups.
Discussion
The aim of a conservation translocation is to provide measurable benefits to the target species by arresting or reversing declines and to restore wild populations (IUCN/SSC, 2013). In the case of population reinforcement an early measure of success is when released individuals can survive and have similar breeding parameters to their wild counterparts, if productivity of the wild is not the cause behind population decline (Bubac et al., 2019). Therefore, long-term monitoring of wild and captive-bred demographic parameters is essential to infer the success of conservation translocation (Armstrong and Seddon, 2008; Batson et al., 2015). The 6 years of monitoring data for reinforced populations of houbara in Central Asia reported here provides the first estimates of breeding parameters for captive-bred migrant houbara and comparisons to their wild counterparts. Our results highlight the conservation value of the translocation program in restoring wild populations, with captive-bred over 2 years old having productivity close to their wild counterparts. The study also reveals the importance of timing of release, with significant variations in some breeding parameters, associated with female age, and time in the season.
The breeding traits of wild-born and captive-bred houbara were not significantly different, except for egg volume and nest initiation of spring releases and breeding probability at 1-year-old of autumn releases. This is a key result for the houbara translocation programs in Uzbekistan and Kazakhstan, confirming that translocated captive-bred houbara can successfully breed and contribute to wild population demography. Interestingly, released 1-year-old females were able to breed before (spring releases) and after (autumn releases) the completion of their first migration. However, this shift in release timing was not without consequences and had varying effects on some reproductive traits, particularly in 1-year-old females, i.e., breeding probability, the nest initiation date, and the egg volume.
One-year-old females released in autumn had a significantly lower breeding probability than wild and spring releases, which had similar breeding probabilities (Figure 2). An opposite pattern was observed in the African houbara, with females released in autumn having a higher breeding performance than females released in spring (Bacon et al., 2018). Considering that survival and reproductive parameters may positively covary (Cam et al., 2016), and because a higher mortality has been observed in autumn releases (Hardouin et al., 2014), the authors hypothesized that observed differences in breeding performance can be attributed to environmental pressure, which selects for the most performant females, resulting in autumn released females being higher quality breeders. Based on such selection theory, our result therefore appears counterintuitive considering the lower survival of Asian houbara released in autumn, compared to spring releases (ECCH unpublished data; Burnside et al., 2016) and potential selection for better breeders in the following season. However, the major life history event that differentiates released Asian houbara from African Houbara is their migration behavior. In autumn, birds are released as juveniles before the end of September, and shortly before autumn migration starts around mid-October (Madon et al., 2015). Therefore, they have a limited time to adapt to their new environment and to prepare for their first migration which represents a highly energy-demanding life stage (Battley et al., 2000; Lindström et al., 2000; Wikelski et al., 2003; McKechnie, 2008). The overlap of these two high energy-demanding stages can potentially influence fitness, not only in terms of survival, but through carry-over effects on breeding traits in the following breeding season (Schmidt-Wellenburg et al., 2008; Fayet et al., 2016). The timing of specific life history traits (e.g., migration or reproduction) spans a temporal continuum, and changes in one trait can have cascading effects on others (Mitchell et al., 2012; Touchon et al., 2013). Birds released in spring, contrary to autumn release, are not urged to migrate. They are released between late March and early May, at the peak of the breeding season when environmental conditions (e.g., food resources and temperature) are at their optimal, promoting their breeding probability. However, they are kept in aviaries over winter for an average of 11 months (±0.97 SD), and once released, post-release stress and time of acclimatization to their novel environment might reduce their fitness and affect breeding expression via delaying their breeding attempts (Saltz and Rubenstein, 1995; Dickens et al., 2010; Hamilton et al., 2010). Such an adaptation period seemed to be necessary for spring release houbara females as they laid the latest (Figure 3).
In the houbara, the laying time in the season is a major factor in the regulation of breeding success, affecting females' investment in terms of clutch size, egg volume and re-clutching probability (Bacon et al., 2017; Azar et al., 2018). This is usually explained in relation to underlying physiological mechanisms determining female breeding investment such as reduction in food availability or increase in predation intensity (Decker et al., 2012). Nest laid later in the season are probably replacement clutches which are known to have lower female investment and productivity (Nisbet, 2019). In our study, nest survival followed a quadratic relationship with time in the season (Figure 5) with survival declining toward the end, possibly due to a progressive increase in predation risk. Along the season, predator breeding may induce a rise in their nutritional demand as they hunt to feed their offspring (Wilson et al., 2007). In addition, the guild of predator species might increase or change, with species more specialized in nest predation (Ibáñez-Álamo et al., 2015), as in Uzbekistan with the emergence of seasonal predators such as the monitor lizard (Varanus griseus) (Appendix D). Re-clutching probability also decreased with the advancement of breeding season, probably because it is constrained by migration timing. Nest survival and re-clutching probability did not differ with female origin; however, spring released females laying later had smaller eggs (Figure 4), possibly reflecting a mismatch between nest initiation date and optimal resources availability. Age had positive effects on breeding parameters, resulting in older females nesting earlier, having a higher breeding probability, laying larger eggs, and having a higher re-clutching probability. These findings are consistent with previous results on resident Asian houbara in UAE (Azar et al., 2018), African houbara in Morocco (Bacon et al., 2017), and supporting the compelling evidence of age effect on breeding parameters (Christians, 2002; Williams, 2012).
Even though aging improved most breeding parameters for captive-bred and wild females, the delay in laying date persisted for spring released females (Figure 3), as did their lower investment in egg volume (Figure 4), potentially supporting the existence of a long-lasting effect, as observed in the African houbara (Bacon et al., 2018). In our study, several hypotheses can also be proposed to explain the observed patterns. One explanation could be the so-called “domino effect” in which a delay in one life stage of a migratory bird can carry-over to successive life stages (Briedis et al., 2018). For example, late breeders may experience delays in departure date and arrival date on wintering grounds which can carry over to delay next year's departure from wintering grounds, arrival date on breeding grounds, and nest initiation (Stutchbury et al., 2011; Catry et al., 2013; Beltran et al., 2019). A recent work on migratory performances of captive-bred juveniles of Asian houbara found a delay in departure date in autumn compared to wild juveniles (Diele-Viegas et al., 2020), implying the need to investigate further potential carryover effects of early life stages in migrant Asian houbara. Another explanation is carryover effects of early life experiences; if age and experience are assumed to covary, there is growing evidence that early experiences are important in determining individual performance later in life (Teitelbaum et al., 2019b; Rotics et al., 2021). This is especially true for precocial species raised in captivity, where the lack of parental learning may influence their behavior later in life and affect demographic traits such as survival, dispersal, and breeding (Dreitz, 2009). Translocated captive-bred animals usually lack parental care and will need more time and exposure to their novel environment to gather information and learn how to best exploit local resources (Mertes et al., 2019). Recent animal behavior research demonstrates that early life stages play a key role in shaping the personality and cognitive capacity of released animals (Whiteside et al., 2015, 2016; Germano et al., 2017). In our study, the early life experience of autumn and spring released groups differs dramatically (time in the wild vs. time in captivity; migration vs. breeding; weather conditions, food availability, etc.), leading to differences in breeding performance in the first year that persisted in subsequent years.
Due to the extensive breeding range of the houbara, their breeding parameters are expected to vary geographically with change in habitat quality, local climate, and migration strategies. Even though our study covered a limited area of the species' breeding grounds, variation in breeding parameters were noted. In Kazakhstan, houbara nested later, laid larger clutches, and had higher nest survival compared to Uzbekistan. The later nesting date in Kazakhstan is probably due to the longer migration route (Madon et al., 2015) or a delayed spring at higher latitudes (McNamara et al., 2008). Differences in clutch size may result from differences in the quality of wintering and breeding areas (Ockendon et al., 2013) or migration strategies (Arzel et al., 2014). However, these hypotheses require further investigation in the case of the houbara. Higher nest survival in Kazakhstan supports a positive latitudinal trend reported in many migratory species, which is usually explained by a decrease in predator density (Freeman et al., 2019). Furthermore, more intensive herding activity in Uzbekistan (Koshkin et al., 2016) resulted in more nest failures (10.91%) compared to Kazakhstan (6.66%), primarily due to livestock disturbance and trampling, predation by domestic dogs, or being poached by shepherds (Appendix D). Wild nest survival in Uzbekistan (0.41 ± 0.03 SE) was lower in our study than previously reported in Bukhara province (0.51 ± 0.09 CI, Koshkin et al., 2016), most likely due to the longer breeding season considered in our study (until mid-June compared to early May), resulting in the inclusion of nests initiated later in the season having lower survival (Figure 5). Other factors influencing survival may include habitat variations between local nesting areas or annual variation of predation risk. As such, a study on breeding parameters encompassing large geographical and temporal scales is necessary to fully uncover spatial and temporal demographic variability and to predict population trends. Chick survival of precocial birds is difficult to monitor due to the limitations in monitoring and tagging techniques and their negative impact on survival. In our study, chick survival did not differ by geography, female age, or release season, however this results should be considered with caution due to the small sample sizes from 1-year-old spring releases in Kazakhstan (n = 9) and Uzbekistan (n = 5), or 2-year-old spring release in Kazakhstan (n = 5) and Uzbekistan (n = 11), or missing groups from 1-year autumn release and 1-year wild (Table 1), therefore further analysis based on larger sample size is necessary. Finally, it is important to note that grouping females into two age categories may have created an unbalance in age structure; the mean age of captive-bred females ≥2 years old was 2.73 (±0.93, range: 2–6) years, while the true age of wild-born females was unknown as most of them were caught as adults on their nests. Knowing that the breeding success increases with age (Bacon et al., 2017), we might expect an increase in the productivity of the captive-bred females associated with age.
Conservation Implications
This study demonstrates that translocation programs in Central Asia are contributing to population demography, with captive-bred released houbara having close, but slightly lower, breeding success to their wild counterparts. It also emphasizes the importance of long-term monitoring and an experimental approach to unravel causes of the observed variations. Captive-bred animals often face extraordinary challenges. In the absence of parental care, they require longer adaptation time, and it may overlap with key life history events such as breeding and migration. The timing of release then becomes fundamental, potentially affecting their performance not only in their first year, but also later in life. Our study illustrates such effect on breeding success, but more research is needed to assess the effect of release timing on other demographics such as survival and migration patterns in the short- and long-terms. Beyond the case of the Asian houbara, these results may inform current and future release tactics of other captive bred bustards, as the Little Bustard (Tetrax tetrax), the Great bustard (Otis tarda), the Arabian bustard (Ardeotis arab) and the Great Indian Bustard (Ardeotis nigriceps), for which migratory behaviors have been observed in some populations (Villers et al., 2010; Burnside et al., 2012; Kessler et al., 2013; Mahood et al., 2019). Finally, our study provides original information on two main sources of variation in productivity: geography and age. Interestingly, 1-year-old migrant Asian houbara in Central Asia had relatively high productivity compared to resident Asian houbara in the Middle East (Azar et al., 2018) or the African houbara in Morocco (Bacon et al., 2017, 2018). Therefore, in migrant Asian houbara, population dynamic modeling should consider the contribution of 1-year-old birds (wild and captive-bred), and viability studies should consider variation between geographical populations in order to better estimate the contribution of captive-bred birds to houbara conservation efforts.
Data Availability Statement
Requests to access the datasets should be directed to the research department at Reneco International Wildlife Consultants: www.reneco.net/contact.
Ethics Statement
Houbara release, trapping, and nest monitoring were all done under proper permits from the State Committee of the Republic of Uzbekistan on Ecology and Environmental Protection and the Institute of Zoology at the Science Committee of the Ministry of Education and Science of the Republic of Kazakhstan. Releases in Kazakhstan were supervised by the Kazakh Scientific Center for Quarantine and Zoonotic Diseases.
Author Contributions
JA analyzed the data and led the writing of the manuscript. CF and CL coordinated fieldwork and data collection in Kazakhstan and Uzbekistan. YH conceptualized the study and contributed to the manuscript's writing. All authors contributed to the article and approved the submitted version.
Funding
This study was supported by the International Fund for Houbara Conservation (IFHC) and the Emirate Center for the Conservation of Houbara (ECCH).
Conflict of Interest
The authors are employed by Reneco International Wildlife Consultants, LLC.
Publisher's Note
All claims expressed in this article are solely those of the authors and do not necessarily represent those of their affiliated organizations, or those of the publisher, the editors and the reviewers. Any product that may be evaluated in this article, or claim that may be made by its manufacturer, is not guaranteed or endorsed by the publisher.
Acknowledgments
We are grateful to HH Sheikh Mohamed bin Zayed Al Nahyan, Crown Prince of Abu Dhabi and Founder of the IFHC, HH Sheikh Theyab Bin Mohamed Al Nahyan, Chairman of the IFHC, HE Mohammed Ahmed Al Bowardi, Deputy Chairman, for their support, and HH Sheikh Mohammed Bin Rashid Al Maktum funder of the ECCH. This study was completed under the guidance of Reneco International Wildlife Consultants, LLC, a consulting company managing IFHC and ECCH houbara conservation programs. We are thankful to Dr. F. Lacroix, managing director of Reneco. We sincerely thank all IFHC and ECCH employees in particular the ecology division involved in data collection. We are grateful to Dr. Thomas Martin for improving the English text.
Supplementary Material
The Supplementary Material for this article can be found online at: https://www.frontiersin.org/articles/10.3389/fcosc.2022.815506/full#supplementary-material
References
Araki, H., Cooper, B., and Blouin, M. S. (2007). Genetic effects of captive breeding cause a rapid, cumulative fitness decline in the wild. Science. 318, 100–103. doi: 10.1126/science.1145621
Armstrong, D. P., and Seddon, P. J. (2008). Directions in reintroduction biology. Trends Ecol. Evol. 23, 20–25. doi: 10.1016/j.tree.2007.10.003
Arzel, C., Dessborn, L., Pöysä, H., Elmberg, J., Nummi, P., and Sjöberg, K. (2014). Early springs and breeding performance in two sympatric duck species with different migration strategies. Ibis 156, 288–298. doi: 10.1111/ibi.12134
Azar, J. F., Chalah, T., Rautureau, P., Lawrence, M., and Hingrat, Y. (2018). Breeding success and juvenile survival in a reintroduced captive-bred population of Asian houbara bustards in the United Arab Emirates. Endanger. Species Res. 35, 59–70. doi: 10.3354/esr00874
Bacon, L., Hingrat, Y., and Robert, A. (2017). Evidence of reproductive senescence of released individuals in a reinforced bird population. Biol. Conserv. 215, 288–295. doi: 10.1016/j.biocon.2017.08.023
Bacon, L., Robert, A., and Hingrat, Y. (2018). Long lasting breeding performance differences between wild-born and released females in a reinforced North African Houbara bustard (Chlamydotis undulata undulata) population: a matter of release strategy. Biodivers. Conserv. 28, 553–570. doi: 10.1007/s10531-018-1651-6
Barton, K. (2019). Package ‘MuMIn’: Multi-Model Inference. R Package Version 1.43.46. Available online at: http://CRAN.R-project.org/package=MuMIn
Batson, W. G., Gordon, I. J., Fletcher, D. B., and Manning, A. D. (2015). REVIEW: translocation tactics: a framework to support the IUCN Guidelines for wildlife translocations and improve the quality of applied methods. J. Appl. Ecol. 52, 1598–1607. doi: 10.1111/1365-2664.12498
Battley, P. F., Piersma, T., Dietz, M. W., Tang, S., Dekinga, A., and Hulsman, K. (2000). Empirical evidence for differential organ reductions during trans-oceanic bird flight. Proc. Royal Soc. B. 267, 191–195. doi: 10.1098/rspb.2000.0986
Beaudoing, H., and Rodell, M., NASA/GSFC/HSL (2020), GLDAS Noah Land Surface Model L4 3 hourly 0.25 x 0.25 degree V2.1, Greenbelt, Maryland, USA, Goddard Earth Sciences Data and Information Services Center (GES DISC). (accessed April 15, 2020], 10.5067/E7TYRXPJKWOQ.
Beltran, R. S., Kirkham, A. L., Breed, G. A., Testa, J. W., and Burns, J. M. (2019). Reproductive success delays moult phenology in a polar mammal. Sci. Rep. 9, 5221. doi: 10.1038/s41598-019-41635-x
BirdLife International (2017). Chlamydotis Macqueenii (Amended Version of 2016 Assessment). The IUCN Red List of Threatened Species 2017. Available online at: http://dx.doi.org/10.2305/IUCN.UK.2017-3.RLTS.T22733562A118585210.en (accessed October 28, 2019).
Brandl, H. B., Farine, D. R., Funghi, C., Schuett, W., and Griffith, S. C. (2019). Early-life social environment predicts social network position in wild zebra finches. Proc. Biol. Sci. 286, 20182579. doi: 10.1098/rspb.2018.2579
Briedis, M., Krist, M., Král, M., Voigt, C. C., and Adamík, P. (2018). Linking events throughout the annual cycle in a migratory bird—non-breeding period buffers accumulation of carry-over effects. Behav. Ecol. Sociobiol. 72, 93. doi: 10.1007/s00265-018-2509-3
Bright, P. W., and Morris, P. A. (1994). Animal translocation for conservation: performance of dormice in relation to release methods, origin and season. J. Appl. Ecol. 31, 699. doi: 10.2307/2404160
Brooks, M. E., Kasper, K., Koen, J., van, B., Arni, M., Casper, W., et al. (2017). glmmTMB balances speed and flexibility among packages for zero-inflated generalized linear mixed modeling. R J. 9, 378–400. doi: 10.32614/RJ-2017-066
Bubac, C. M., Johnson, A. C., Fox, J. A., and Cullingham, C. I. (2019). Conservation translocations and post-release monitoring: identifying trends in failures, biases, and challenges from around the world. Biol. Conserv. 238, 108239. doi: 10.1016/j.biocon.2019.108239
Burnham, K. P., and Anderson, D. R. (2010). Model Selection and Multimodel Inference: A Practical Information-Theoretic Approach. New York, NY: Springer.
Burnside, R. J., Carter, I., Dawes, A., Waters, D., Lock, L., et al. (2012). The UK great bustard Otis tarda reintroduction trial: a 5-year progress report. Oryx. 46, 112–121. doi: 10.1017/S0030605311000627
Burnside, R. J., Collar, N. J., Scotland, K. M., and Dolman, P. M. (2016). Survival rates of captive-bred Asian Houbara Chlamydotis macqueenii in a hunted migratory population. Ibis 158, 353–361. doi: 10.1111/ibi.12349
Burnside, R. J., Guilherme, J. L., Collar, N. J., and Dolman, P. M. (2019). Backpack-mounted satellite transmitters do not affect reproductive performance in a migratory bustard. Eur. J. Wildl. Res. 65, 98. doi: 10.1007/s10344-019-1332-0
Cam, E., Aubry, L. M., and Authier, M. (2016). The conundrum of heterogeneities in life history studies. Trends Ecol. Evol. 31, 872–886. doi: 10.1016/j.tree.2016.08.002
Catry, P., Dias, M. P., Phillips, R. A., and Granadeiro, J. P. (2013). Carry-over effects from breeding modulate the annual cycle of a long-distance migrant: an experimental demonstration. Ecology 94, 1230–1235. doi: 10.1890/12-2177.1
Christians, J. K. (2002). Avian egg size: variation within species and inflexibility within individuals. Biol. Rev. Camb. Philos. Soc. 77, 1–26. doi: 10.1017/S1464793101005784
Combreau, O., Qiao, J., Lawrence, M., Gao, X., Yao, J., Yang, W., et al. (2002). Breeding success in a Houbara Bustard Chlamydotis [undulata] macqueenii population on the eastern fringe of the Jungar Basin, People's Republic of China. Ibis 144, E45–E56. doi: 10.1046/j.1474-919X.2002.00054.x
Combreau, O., Riou, S., Judas, J., Lawrence, M., and Launay, F. (2011). Migratory pathways and connectivity in Asian houbara bustards: evidence from 15 years of satellite tracking. PLoS ONE 6, e20570. doi: 10.1371/journal.pone.0020570
Decker, K. L., Conway, C. J., and Fontaine, J. J. (2012). Nest predation, food, and female age explain seasonal declines in clutch size. Evol. Ecol. 26, 683–699. doi: 10.1007/s10682-011-9521-7
Dickens, M. J., Delehanty, D. J., and Michael Romero, L. (2010). Stress: an inevitable component of animal translocation. Biol. Conserv. 143, 1329–1341. doi: 10.1016/j.biocon.2010.02.032
Diele-Viegas, L. M., Hingrat, Y., Eliezer, G. E., Luca, C., Folta, C., Su, S., et al. (2020). “Migratory performance of wild and captive bred released Asian Houbara Bustards, Chlamydotis macqueenii [Conference presentation],” in Advanced Research in Bustard Ecology and Conservation (Abu Dhabi).
Dreitz, V. J. (2009). Parental behaviour of a precocial species: implications for juvenile survival. J. Appl. Ecol. 46, 870–878. doi: 10.1111/j.1365-2664.2009.01658.x
Facka, A. N., Lewis, J. C., Happe, P., Jenkins, K., Callas, R., and Powell, R. A. (2016). Timing of translocation influences birth rate and population dynamics in a forest carnivore. Ecosphere 7, e01223. doi: 10.1002/ecs2.1223
Fayet, A. L., Freeman, R., Shoji, A., Kirk, H. L., Padget, O., Perrins, C. M., et al. (2016). Carry-over effects on the annual cycle of a migratory seabird: an experimental study. J. Anim. Ecol. 85, 1516–1527. doi: 10.1111/1365-2656.12580
Fischer, J., and Lindenmayer, D. B. (2000). An assessment of the published results of animal relocations. Biol. Conserv. 96, 1–11. doi: 10.1016/S0006-3207(00)00048-3
Frankham, R. (2008). Genetic adaptation to captivity in species conservation programs. Mol. Ecol. 17, 325–333. doi: 10.1111/j.1365-294X.2007.03399.x
Freeman, B. G., Scholer, M. N., Boehm, M. M. A., Heavyside, J., and Schluter, D. (2019). Adaptation and latitudinal gradients in species interactions: nest predation in birds. Am. Nat. 196, E160–E166. doi: 10.1086/711415
Germano, J. M., Nafus, M. G., Perry, J. A., Hall, D. B., and Swaisgood, R. R. (2017). Predicting translocation outcomes with personality for desert tortoises. Behav. Ecol. 28, 1075–1084. doi: 10.1093/beheco/arx064
Griffith, B., Scott, J. M., Carpenter, J. W., and Reed, C. (1989). Translocation as a species conservation tool: status and strategy. Science. 245, 477–480. doi: 10.1126/science.245.4917.477
Gubin, B. M. (2008). A Natural History of the Houbara Bustard. Abu Dhabi-UAE: Environment Agency - Abu Dhabi (EAD) in association with Motivate Publishing.
Hamilton, L. P., Kelly, P. A., Williams, D. F., Kelt, D. A., and Wittmer, H. U. (2010). Factors associated with survival of reintroduced riparian brush rabbits in California. Biol. Conserv. 143, 999–1007. doi: 10.1016/j.biocon.2010.01.015
Hardouin, L. A., Hingrat, Y., Nevoux, M., Lacroix, F., and Robert, A. (2015). Survival and movement of translocated houbara bustards in a mixed conservation area. Anim. Conserv. 18, 461–470. doi: 10.1111/acv.12196
Hardouin, L. A., Robert, A., Nevoux, M., Gimenez, O., Lacroix, F., and Hingrat, Y. (2014). Meteorological conditions influence short-term survival and dispersal in a reinforced bird population. J. Appl. Ecol. 51, 1494–1503. doi: 10.1111/1365-2664.12302
Hoyt, D. F. (1979). Practical methods of estimating volume and fresh weight of bird eggs. Auk. 96, 73–77.
Huff, D. D., Miller, L. M., Chizinski, C. J., and Vondracek, B. (2011). Mixed-source reintroductions lead to outbreeding depression in second-generation descendents of a native North American fish. Mol. Ecol. 20, 4246–4258. doi: 10.1111/j.1365-294X.2011.05271.x
Ibáñez-Álamo, J. D., Magrath, R. D., Oteyza, J. C., Chalfoun, A. D., Haff, T. M., Schmidt, K. A., et al. (2015). Nest predation research: recent findings and future perspectives. J Ornithol 156, 247–262 doi: 10.1007/s,10336-015-1207-4
IUCN/SSC (2013). Guidelines for Reintroductions and Other Conservation Translocations. Gland: IUCN Species Survival Commission.
Jachowski, D., Millspaugh, J. J., Angermeier, P. L., and Slotow, R. H., eds. (2016). Reintroduction of Fish and Wildlife Populations. Oakland, CA: University of California Press.
Kessler, A. E., Batbayar, N., Natsagdorj, T., Batsuur, D., and Smith, A. T. (2013). Satellite telemetry reveals long-distance migration in the Asian great bustard Otis tarda dybowskii. J. Avian Biol. 44, 311–320. doi: 10.1111/j.1600-048X.2013.00072.x
Kirchner, F., Robert, A., and Colas, B. (2006). Modelling the dynamics of introduced populations in the narrow-endemic Centaurea corymbosa: a demo-genetic integration. J. Appl. Ecol. 43, 1011–1021. doi: 10.1111/j.1365-2664.2006.01179.x
Koshkin, M., Burnside, R. J., Packman, C. E., Collar, N. J., and Dolman, P. M. (2016). Effects of habitat and livestock on nest productivity of the Asian houbara Chlamydotis macqueenii in Bukhara Province, Uzbekistan. Eur. J. Wildl. Res. 62, 447–459. doi: 10.1007/s10344-016-1018-9
Kottek, M., Grieser, J., Beck, C., Rudolf, B., and Rubel, F. (2006). World Map of the Köppen-Geiger climate classification updated. Meteorol. Z. 15, 259–263. doi: 10.1127/0941-2948/2006/0130
Lacroix, F., Seabury, J., Al Bowardi, M., and Renaud, J. (2003). The Emirates Center for Wildlife Propagation: developing a comprehensive strategy to secure a self-sustaining population of Houbara Bustards in eastern Morocco. Houbara News 5, 2. Available online at: http://wmenews.com/Information/Other/Section3Lacroixetalpp60-62pdf.pdf
Lesobre, L. (2008). Genetic structure of endangered populations of Houbara bustard (Chlamydotis undulata undulata) in Morocco. Implications for the management of a captive breeding program and the reinforcement of wild populations (PhD thesis). Muséum National d'Histoire Naturelle, Paris, France.
Lindström, A., Kvist, A., Piersma, T., Dekinga, A., and Dietz, M. W. (2000). Avian pectoral muscle size rapidly tracks body mass changes during flight, fasting and fuelling. J. Exp. Biol. 203, 913–919. doi: 10.1242/jeb.203.5.913
Lynch, M., and O'Hely, M. (2001). Captive breeding and the genetic fitness of natural populations. Conserv. Genet. 2, 363–378. doi: 10.1023/A:1012550620717
Madon, B., Le Nuz, E., Ferlat, C., and Hingrat, Y. (2015). Insights into the phenology of migration and survival of a long migrant land bird. bioRxiv [Preprint], (2015). Available online at: https://www.biorxiv.org/content/10.1101/028597v1 (accessed May 10, 2021).
Mahood, S. P., Jha, R. R. S., Rahmani, A. R., Thakuri, J. J., Chamnan, H., Bhargava, R., et al. (2019). A systematic expert-based assessment of progress and priorities for conservation of the Bengal Florican Houbaropsis bengalensis. Conservat. Sci. Prac. 1:e121. doi: 10.1111/csp2.121
McKechnie, A. E. (2008). Phenotypic flexibility in basal metabolic rate and the changing view of avian physiological diversity: a review. J. Comp. Physiol. B 178, 235–247. doi: 10.1007/s00360-007-0218-8
McNamara, J. M., Barta, Z., Wikelski, M., and Houston, A. I. (2008). A theoretical investigation of the effect of latitude on avian life histories. Am. Nat. 172, 331–345. doi: 10.1086/589886
Mertes, K., Stabach, J. A., Songer, M., Wacher, T., Newby, J., Chuven, J., et al. (2019). Management background and release condition's structure post-release movements in reintroduced ungulates. Front. Ecol. Evol. 7, 470. doi: 10.3389/fevo.2019.00470
Mirzabaev, A. (2013). Climate volatility and change in Central Asia: economic impacts and adaptation (PhD thesis). University of Bonn, Bonn, Germany.
Mitchell, G. W., Newman, A. E. M., Wikelski, M., and Norris, D. R. (2012). Timing of breeding carries over to influence migratory departure in a songbird: an automated radiotracking study. J. Anim. Ecol. 81, 1024–1033. doi: 10.1111/j.1365-2656.2012.01978.x
Nisbet, I. C. T. (2019). Consequences of renesting in common terns (Sterna hirundo): changes in clutch size, egg mass, and productivity. Waterbirds 42, 393. doi: 10.1675/063.042.0404
Ockendon, N., Leech, D., and Pearce-Higgins, J. W. (2013). Climatic effects on breeding grounds are more important drivers of breeding phenology in migrant birds than carry-over effects from wintering grounds. Biol. Lett. 9, 20130669. doi: 10.1098/rsbl.2013.0669
Olson, D. M., Dinerstein, E., Wikramanayake, E. D., Burgess, N. D., Powell, G. V. N., Underwood, E. C., et al. (2001). Terrestrial ecoregions of the world: a new map of life on earth. Anim. Conserv. 51, 933. doi: 10.1641/0006-3568(2001)051[0933:TEOTWA]2.0.CO;2
Parker, K. A., Dickens, M. J., Clarke, R. H., and Lovegrove, T. G. (2012). “The theory and practice of catching, holding, moving and releasing animals,” in Reintroduction Biology: Integrating Science and Management, ed. J. G. Ewen (Oxford: Wiley-Blackwell), 105–137.
R Development Core Team (2015). R: A Language and Environment for Statistical Computing. Vienna: The R Foundation for Statistical Computing.
Riou, S., Combreau, O., Judas, J., Lawrence, M., Al Baidani, M. S., and Pitra, C. (2012). Genetic differentiation among migrant and resident populations of the threatened Asian houbara bustard. J. Hered. 103, 64–70. doi: 10.1093/jhered/esr113
Riou, S., Judas, J., Lawrence, M., Pole, S., and Combreau, O. (2011). A 10-year assessment of Asian Houbara Bustard populations: trends in Kazakhstan reveal important regional differences. Bird Conserv. Int. 21, 134–141. doi: 10.1017/S0959270910000377
Robert, A. (2011). Find the weakest link. A comparison between demographic, genetic and demo-genetic metapopulation extinction times. BMC Ecol. Evol. 11, 260. doi: 10.1186/1471-2148-11-260
Robert, A., Colas, B., Guigon, I., Kerbiriou, C., Mihoub, J.-B., Saint-Jalme, M., et al. (2015). Defining reintroduction success using IUCN criteria for threatened species: a demographic assessment. Anim. Conserv. 18, 397–406. doi: 10.1111/acv.12188
Ross, A. K., Letnic, M., and Blumstein, D. T. Moseby, K. E. (2019). Reversing the effects of evolutionary prey naiveté through controlled predator exposure. J Appl Ecol. 56:1761–1769. doi: 10.1111/1365-2664.13406
Rotics, S., Turjeman, S., Kaatz, M., Zurell, D., Wikelski, M., Sapir, N., et al. (2021). Early-life behaviour predicts first-year survival in a long-distance avian migrant. Proc. Royal Soc. B. 288, 20202670. doi: 10.1098/rspb.2020.2670
Saltz, D., and Rubenstein, D. I. (1995). Population dynamics of a reintroduced asiatic wild ass (Equus Hemionus) Herd. Ecol. Appl. 5, 327–335. doi: 10.2307/1942025
Schaub, M., Zink, R., Beissmann, H., Sarrazin, F., and Arlettaz, R. (2009). When to end releases in reintroduction programmes: demographic rates and population viability analysis of bearded vultures in the Alps. J. Appl. Ecol. 46, 92–100. doi: 10.1111/j.1365-2664.2008.01585.x
Schmidt-Wellenburg, C. A., Visser, G. H., Biebach, B., Delhey, K., Oltrogge, M., Wittenzellner, A., et al. (2008). Trade-off between migration and reproduction: does a high workload affect body condition and reproductive state? Behav. Ecol. 19, 1351–1360. doi: 10.1093/beheco/arn066
Shaffer, T. L. (2004). A unified approach to analyzing nest success. Auk. 121, 526–540. doi: 10.1642/0004-8038(2004)121[0526:AUATAN]2.0.CO;2
Sheean, V. A., Manning, A. D., and Lindenmayer, D. B. (2012). An assessment of scientific approaches towards species relocations in Australia. Austral Ecol. 37, 204–215. doi: 10.1111/j.1442-9993.2011.02264.x
Stutchbury, B. J. M., Gow, E. A., Done, T., MacPherson, M., Fox, J. W., and Afanasyev, V. (2011). Effects of post-breeding moult and energetic condition on timing of songbird migration into the tropics. Proc. Royal Soc. B. 278, 131–137. doi: 10.1098/rspb.2010.1220
Tavecchia, G., Viedma, C., Martínez-Abraín, A., Bartolomé, M.-A., Gómez, J. A., and Oro, D. (2009). Maximizing re-introduction success: assessing the immediate cost of release in a threatened waterfowl. Biol. Conserv. 142, 3005–3012. doi: 10.1016/j.biocon.2009.07.035
Teitelbaum, C. S., Converse, S. J., Fagan, W. F., and Mueller, T. (2019a). “Movement ecology of reintroduced migratory Whooping Cranes,” in Whooping Cranes: Biology and conservation, ed. P. Nyhus (Cambridge, MA: Academic Press), 217–238.
Teitelbaum, C. S., Converse, S. J., and Mueller, T. (2019b). The importance of early life experience and animal cultures in reintroductions. Conserv. Lett. 12, e12599. doi: 10.1111/conl.12599
Teitelbaum, C. S., and Mueller, T. (2019). Beyond migration: causes and consequences of nomadic animal movements. Trends Ecol. Evol. 34, 569–581. doi: 10.1016/j.tree.2019.02.005
Touchon, J. C., McCoy, M. W., Vonesh, J. R., and Warkentin, K. M. (2013). Effects of plastic hatching timing carry over through metamorphosis in red-eyed treefrogs. Ecology 94, 850–860. doi: 10.1890/12-0194.1
Villers, A., Millon, A., Jiguet, F., Lett, J.-M., Attie, C., Morales, M. B., et al. (2010). Migration of wild and captive-bred Little Bustards Tetrax tetrax: releasing birds from Spain threatens attempts to conserve declining French populations. Ibis 152, 254–261. doi: 10.1111/j.1474-919X.2009.01000.x
Whiteside, M. A., Sage, R., and Madden, J. R. (2015). Diet complexity in early life affects survival in released pheasants by altering foraging efficiency, food choice, handling skills and gut morphology. J. Anim. Ecol. 84, 1480–1489. doi: 10.1111/1365-2656.12401
Whiteside, M. A., Sage, R., and Madden, J. R. (2016). Multiple behavioural, morphological and cognitive developmental changes arise from a single alteration to early life spatial environment, resulting in fitness consequences for released pheasants. R. Soc. Open Sci. 3, 160008. doi: 10.1098/rsos.160008
Wikelski, M., Tarlow, E. M., Raim, A., Diehl, R. H., Larkin, R. P., and Visser, G. H. (2003). Costs of migration in free-flying songbirds. Nature. 423, 704. doi: 10.1038/423704a
Williams, T. D. (2012). Physiological Adaptations for Breeding in Birds. Princeton: Princeton University Press.
Wilson, B. A., Evans, M. J., Evans, M. J., Batson, W. G., Banks, S. C., Gordon, I. J., et al. (2020). Adapting reintroduction tactics in successive trials increases the likelihood of establishment for an endangered carnivore in a fenced sanctuary. PLoS ONE 15, e0234455. doi: 10.1371/journal.pone.0234455
Wilson, S., Martin, K., and Hannon, S. J. (2007). Nest survival patterns in willow ptarmigan: influence of time, nesting stage, and female characteristics. Condor. 109, 377–388. doi: 10.1093/condor/109.2.377
Keywords: animal translocation, conservation, migration, reinforcement, release timing, reproduction
Citation: Azar JF, Ferlat C, Landsmann C and Hingrat Y (2022) Timing of Release Influence Breeding Success of Translocated Captive-Bred Migrant Asian Houbara Bustard. Front. Conserv. Sci. 3:815506. doi: 10.3389/fcosc.2022.815506
Received: 15 November 2021; Accepted: 14 January 2022;
Published: 22 February 2022.
Edited by:
Carlos R. Ruiz-Miranda, State University of the North Fluminense Darcy Ribeiro, BrazilReviewed by:
Robert Burnside, University of East Anglia, United KingdomAdrian Manning, Australian National University, Australia
Copyright © 2022 Azar, Ferlat, Landsmann and Hingrat. This is an open-access article distributed under the terms of the Creative Commons Attribution License (CC BY). The use, distribution or reproduction in other forums is permitted, provided the original author(s) and the copyright owner(s) are credited and that the original publication in this journal is cited, in accordance with accepted academic practice. No use, distribution or reproduction is permitted which does not comply with these terms.
*Correspondence: Joseph F. Azar, azar.joseph@gmail.com