- 1Department of Applied Ecology, North Carolina State University, Raleigh, NC, United States
- 2Center for Evolutionary Hologenomics, University of Copenhagen, Copenhagen, Denmark
- 3Department of Biology, University of Kentucky, Lexington, KY, United States
- 4Louis Calder Center and Department of Biological Sciences, Fordham University, New York, NY, United States
- 5Living Earth Collaborative, Washington University in St. Louis, St. Louis, MO, United States
- 6Department of Biology, Washington University in St. Louis, St. Louis, MO, United States
- 7Department of Ecology and Conservation Biology, Texas A&M University, College Station, TX, United States
- 8Department of Biology, Case Western Reserve University, Cleveland, OH, United States
- 9Department of Entomology, Texas A&M University, College Station, TX, United States
- 10Department of Biology, University of North Carolina Chapel Hill, Chapel Hill, NC, United States
- 11Zhejiang Tiantong Forest Ecosystem National Observation and Research Station, School of Ecological and Environmental Sciences, East China Normal University, Shanghai, China
- 12Unidad de Genómica Avanzada (Langebio), Cinvestav-IPN, Irapuato, Guanajuato, Mexico
Many of the choices humans make with regard to infrastructure, urban planning and other phenomena have impacts that will last thousands of years. This can readily be seen in modern cities in which contemporary streets run along street grids that were laid out thousands of years prior or even in which ancient viaducts still play a role. However, rarely do evolutionary biologists explicitly consider the future of life likely to be associated with the decisions we are making today. Here, we consider the evolutionary future of species in cities with a focus on the origin of lineages and species. We do so by adjusting evolutionary predictions from the theory of island biogeography so as to correspond to the unique features of cities as islands. Specifically, the species endemic to cities tend to be associated with the gray habitats in cities. Those habitats tend to be dominated by human bodies, pet bodies and stored food. It is among such species where the origin of new lineages is most likely, although most research on evolution in cities has focused on green habitats. We conclude by considering a range of scenarios for the far future and their implications for the origin of lineages and species.
Introduction
Globally, cities are growing both in number and in size, with dozens of cities now containing more than 10 million people (United Nations Statistics Division, 2017) and new megacities predicted in the coming decades (Terando et al., 2014). Roughly 3% of Earth's terrestrial area is now urban (Johnson and Munshi-South, 2017) with much more suburban. At the same time, the number of people living in cities continues to grow, both in absolute terms and as a proportion of the global population (United Nations, 2018), such that the evolution occurring in cities has the potential to affect and even be seen by billions of people. Recently, there have been calls to conserve evolutionary processes and, in doing so, to ensure their ability to engender future diversity (Des Roches et al., 2018). If we are to watch, understand and thoughtfully conserve and manage evolution in all of its details anywhere, surely where we live should be one of those places. This should occur while also recognizing that biodiversity can provide both services and disservices (Dunn, 2010; von Döhren and Haase, 2015) and, so too, new species can be benign, beneficial, or deadly. Here we consider evolution as it relates to the origin of new species. As a group, we have spent decades studying the ecology and evolution of rodents (Munshi-South), pigeons (Carlen), ants (Martin), household species (Dunn), insect pests (Vargo), plants and their microbes (Cibrian-Jaramillo), lice (Light), and even viruses that infect bacteria that infect household pests (Yitbarek). We have done so with studies of many cities, but with a special focus on Manhattan in New York (Figure 1). Yet, like others, we have written little about the origin of species in cities. Here we take the opportunity to begin to remedy that gap.
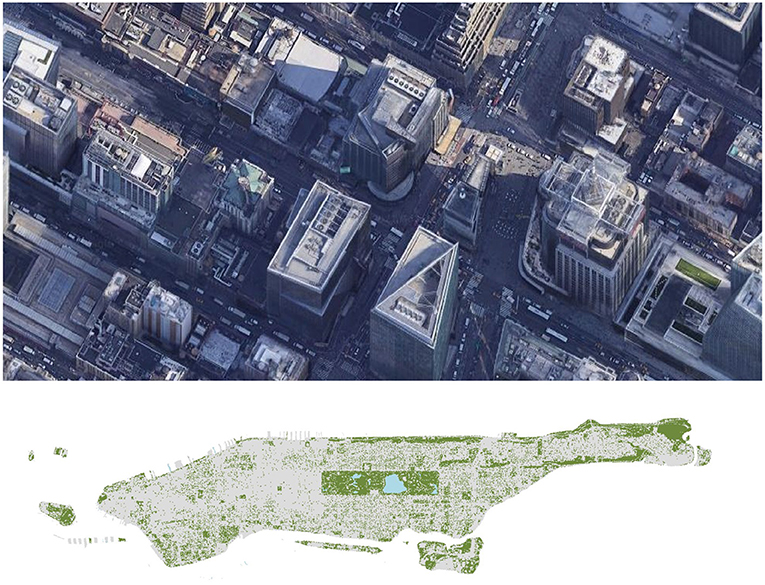
Figure 1. At top, a satellite image of Times Square in Manhattan. In this image, the only visible green habitat is a small green rooftop on the Stephen Sondheim Theater (at far right). As a result, the living biomass of this part of Manhattan is nearly entirely human and part of the gray habitat. Image courtesy of Google Earth. At bottom, a view of all the major green habitats in broader Manhattan as well as the more expansive gray habitats that surround them. In the green parts of the city, plant biomass dominates; in the gray parts of the city, human biomass does (Department of Information Technology Telecommunication, 2018).
In considering the origin of species in cities, we take four steps. First, we lay out a general theory of diversification among cities. Second, we consider the role that differences among cities play in diversification, via effects on mutation rates, generation times and the strength and direction of selection. Third, we consider evidence for divergence of species among cities, with a focus on insights from Manhattan in New York (Figure 1) and, more generally, cities in the United States. Fourth, we discuss potential types of future cities in order to facilitate conversations about the choices we might make to favor one evolutionary outcome relative to another.
The temptation in considering the future is to consider the next quarter, as businesses often do, the next year, the next decade or, if really extending the temporal scale, the years 2050, 2080, or 2100. Here we extend the temporal scale and consider the far future hundreds or even thousands of years from today. Our focus on the far future of life may seem frivolous or even like the misdirection of time and resources at a time of ecological crises. Yet, many of our current actions will greatly influence the far future of biodiversity—we are laying the foundation stones for the evolutionary future today. Most of the infrastructure being built will have a legacy of decades and, in some cases, centuries (Liu et al., 2014). As a result, plans should, at the very least, entail century-thinking. In addition, consideration of the far future allows us to ponder not only the most immediate scenarios (ever more, ever larger cities, connected within regions and sometimes among regions), but also scenarios that are more remote from our daily experiences and yet might nonetheless be possible.
General Theory
In developing general theory for the future evolution of species in cities, we rely on insights from island biogeography, metapopulation theory and macroecology. In this context, we offer six primary sets of hypotheses and predictions.
First, we hypothesize that new species are more likely to evolve in big cities and big groups of cities or megalopolises than in small cities. This prediction is an extension of the area-speciation hypothesis articulated by MacArthur and Wilson (1967) and refined by Kisel and Barraclough (2010). This prediction has three forms. It might apply to species evolving (a) relative to their non-urban ancestors/relatives, (b) relative to urban populations in other cities, or (c) relative to subpopulations in the same city.
Second, the probability of extinction of species from urban islands decreases as a function of the size of the city. This prediction follows directly from MacArthur and Wilson's area-extinction hypothesis (1967), as well as from more recent models that build area-extinction expectations up from species' traits and metapopulation models (Burger et al., 2019). City wide extinctions due to the small area of cities may seem impossible to document. However, they have been observed in the context of heavily managed species such as bed bugs or rats (Brown, 1948). Bed bugs were essentially eradicated in many cities globally by about 1960 (Davies et al., 2012); urban biogeography theory, as well as empirical observations from islands (Toft and Schoener, 1983), predict that such eradication was likely to have been easier in smaller cities and in more isolated cities (where recolonization was less likely). As a result, we predict new species to be more likely to evolve in bigger cities, but then also to persist once they have evolved. It appears that some novel coronaviruses, for example, are more likely to evolve in big cities (particularly those with many unvaccinated individuals, the population sizes of which are the most direct metric of “island size” for the virus), and also more likely to avoid local extinction in such cities (Stier et al., 2020).
Third, the main island biogeographic rules in cities apply most strongly to those species that live primarily in cities, species we call “urban endemics.” For urban endemics, the area between cities is most directly analogous to the sea between oceanic islands. However, the biogeographic rules also apply to populations of non-urban endemic species that acquire independence from other populations of the same species (whether by isolation or due to local selection and adaptation) living outside of cities. By analogy with the disease ecology literature, we call such species “spillover species.”
Fourth, in comparing taxa, the likelihood of divergence of species within or among cities is strongly influenced by their gene flow, as Kisel and Barraclough (2010) have documented. Gene flow prevents local adaptation. Current evidence from over 150 studies indicates that species vary widely in their extent of gene flow and hence differentiation within and among cities (Miles et al., 2018). In considering islands, the probability of the evolution of new species is greatest for species with less gene flow (Kisel and Barraclough, 2010); the same should be true for cities.
Fifth, unlike oceanic islands, the traits that facilitate gene flow (and foil differentiation) within and among cities are likely to be those related to whether species are able to move with humans such that the taxa likely to diverge in cities might be quite different from those on oceanic islands. For example, spiders are relatively likely to diversify within and among oceanic islands (Opatova and Arnedo, 2014). However, in cities this might not necessarily be the case if (or when) spiders are frequently transported from city to city by humans. Recently, Western black widow spiders have been shown to have more gene flow among cities than among geographically matched rural populations (Miles et al., 2018). Similarly, some microbe lineages have diversified among oceanic islands (Lachance and Fedor, 2014), but with urban islands there is the potential for microbes to be moved among cities (Neiderud, 2015) as recently became apparent with the virus that causes COVID-19. Not only did that virus spread globally, but so too have most of the new variants of the virus, often within weeks (Li B. et al., 2021).
Just which species humans move depends on transportation systems, border policies and many other factors. For centuries, the predominant mode of movement of people and goods over long distances was ships. Thanks to the movement of ships, some rat (Rattus spp., such as the Norway rat) and mouse (e.g., Mus musculus, the house mouse) species appear to have moved frequently in previous centuries among ports and their cities (Armitage, 1993). Until as recently as the 1930s, it was assumed that most or even all large ships would host rats and sanitation measures focused on distinguishing ships with an “ordinary number of rats,” from those with an excessive number of rats (Pierce, 1930; Jones et al., 2013). However, hygiene on ships has led them to be ever less likely to transport rats and mice (Russell et al., 2007; Sjodin et al., 2021). As a result, for example, the movement of Norway rats (R. norvegicus) has decreased among ports and cities such that Norway rats have begun to diverge among cities (Lack et al., 2013; Puckett et al., 2016) and among neighborhoods within cities (Combs et al., 2018a,b; Byers et al., 2021).
The key generalism here is that human behaviors (with regard to shipping and hygiene) can have as much influence on which species move among cities and how these species move among cities, as do the dispersal traits of the species themselves. In some scenarios of human behavior (see the end of this article) movement of species by humans will make isolation of taxa within cities or megacities unlikely. In other scenarios, it will be the norm (see Figure 2 for a visualization of the ways in which cities can be both corridors and archipelagos, dependent both on the organism being considered but also the scale of analysis).
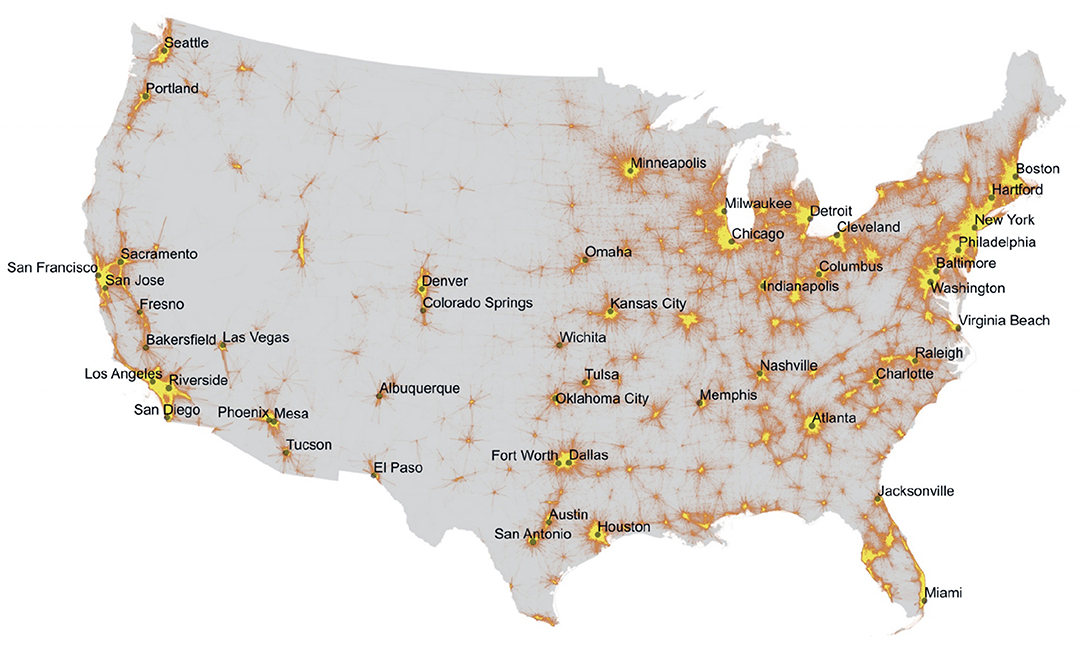
Figure 2. Urban Archipelagos and Corridors. This map shows patterns of commuter movement into and out of cities in the United States, color coded as a function of the density of commuters. Yellow shows areas with high densities of people and movement. Orange indicates an intermediate density of overlapping commutes. On the one hand, this map emphasizes the isolation of many cities, particularly, for example, cities of the intermountain West, and also the isolation of some groups of cities, such as those of California, relative to other cities, such as those of the Northeast. On the other hand, however, this map shows a measure of the extent to which cities can serve as corridors for species able to move with humans in vehicles or along roads. Highlighted here as well are cities mentioned elsewhere in the article, including the Northeastern megacity (note the modest break located around Connecticut) and, within it, Manhattan. Data are from Nelson and Rae (2016).
Sixth, the “size” of an urban island may be a function of more than just its geographic area. Larger oceanic islands tend to have more kinds of habitats, more available biomass (and/or habitat) and more net primary productivity per unit time (which is to say, a greater flux of energy). All of these factors can lead to increased rates of divergence and speciation (Losos and Schluter, 2000; Warren et al., 2015) and alter the dynamics of speciation; they might also do so in cities. However, the nature of these factors differs in cities relative to oceanic habitats in that in cities each of these factors is strongly influenced by human actions.
The number of kinds of habitats in cities is controlled by public and private land management and ownership and by human population density. To the extent that the origin of species on islands is mediated by habitat diversity, the origin of species in cities will be subject to the ways in which urban habitats are managed and inhabited. Currently, such differences in management are strongly influenced by the age of cities, among other factors, including culture and socioeconomics (e.g., Norton et al., 2016). In the future, their drivers may be very different (see “scenario thinking” below).
As for the total biomass in cities, it is heavily influenced by the amount of green space and by the nature of human food subsidies. The first of these points is obvious; the more green space there is, the more primary productivity a city is likely to have. The second of these points is perhaps less obvious. Human food subsidies in cities are poorly studied by ecologists and yet they create and modulate much of the resource base available to organisms in cities. For example, 1.63 million people live in the borough of Manhattan in New York City (U.S. Census Bureau, 2019; see Figure 1 for geographic context). The average adult American body weighs 81 kilograms (180 pounds; Walpole et al., 2012) and contains 12 kg carbon (C; Bar-On et al., 2018). As a result, the average resident (nighttime) biomass of humans (anthromass) in Manhattan is nearly 20 million kilograms C (19.6 million). Compare this with the biomass of plants in Manhattan. On average, total aboveground and belowground plant biomass in temperate forests is about 12,006 g/m2 (say 12 million kg/km2; calculated based on Fahey et al., 2005). If roughly 28% of Manhattan's 59 km2 is green space (Natural Area Conservancy, 2021), then the total plant biomass in Manhattan is 200 million kg C. This is almost certainly an overestimate inasmuch as the green spaces in Manhattan includes habitats with low plant biomass such as grassy parks and street medians. Pulling these pieces back together, this crude math suggests that, on its own, the anthromass in Manhattan is roughly one-tenth the biomass of plants. This is vastly different from most terrestrial ecosystems where, on average, animal biomass tends to be just one two hundredth that of plant biomass (Bar-On et al., 2018). However, our estimate is deceptive for two reasons. First, the population of Manhattan doubles during the day when people come to “the city” to work, or at least it did before the COVID-19 pandemic (McKenzie et al., 2010). As a result, during the day, anthromass in Manhattan may be one-fifth that of plant biomass. Second, our estimates average across the entirety of Manhattan. In truth the city consists of relatively discrete areas where plants grow (such as parks and medians) and those in which they don't. As a result, it is probably more useful to think of cities in two parts, green habitats that are relatively high in plant biomass and that also support the biomass of species dependent on plants and gray habitats that are lower in total biomass and dominated by human biomass. The distribution of these habitats can be seen in the lower panel of Figure 1.
Notably, the food web in the gray habitats of Manhattan and other cities is inverted. In most cases, plant biomass is tiny—and reflected in the occasional house plant or balcony flower—compared to the biomass of humans or, more broadly, humans, pets and other indoor animals. This inversion of the human-supported indoor trophic pyramid is due to agricultural subsidies from rural areas to urban areas. This rural subsidy to cities is analogous to marine subsidies to terrestrial life on a small island (Polis et al., 1997). This rural trophic subsidy is a hidden component of urban ecosystems, though we can provide a rough estimate as to some aspects of its dimensions. If the average New Yorker consumes 2.5 kg of food per day (data from U.S. and Department of Agriculture Economic Research Service, 2021) and keeps about a month worth of stored food (Golem and Byrd-Bredbenner, 2015), then the average person has at least 75 kilograms of food (30 days * 2.5 kg/day) in their house (per capita), amounting to roughly 122 million kg of stored food at any moment in Manhattan, more than half the total C biomass of plants in Manhattan (Golem and Byrd-Bredbenner, 2015). This stored food represents a habitat and food for many species, including species now restricted to cities or human dwellings, some of which have been living in dwellings for many thousands of years (Panagiotakopulu et al., 2010).
Moreover, in New York City roughly 3 million kg of food is wasted daily (~0.5 kg per person per day; Conrad et al., 2018). Once stored food is discarded (and turned into waste), it becomes a food resource for wildlife and a habitat for parasites and pathogens in the form of garbage. In many countries, less than half of municipal solid waste, a large portion of which is food waste, is collected (Adhikari et al., 2006 and references therein). In cities in developed countries, however, such as New York City, the majority of the food waste resource is moved out of the city to landfills or dumps (Kaza et al., 2018), much as feces is displaced to waste treatment facilities. These displaced components of the urban ecosystem have the potential to be evolutionary hotspots. However, our focus here is on the waste that makes its way to streets and green spaces, as well as the feces that makes its way, one defecation at a time, to those same places. The quantity of pet feces on the streets of cities can be immense (Rubel and Wisnivesky, 2005) and is typically complemented by a smaller but consequential density of human feces.
Our calculations of the biomass in Manhattan are very rough, but they should make our main point clear. The bulk of biomass of gray habitats of cities is stored food, human bodies and, also, pet bodies, along with various types of waste. Plant biomass and the species that depend on it, although the focus of the vast majority of urban ecological research, are a minor component of the most “lived in” parts of cities. We may underestimate the amount of green life indoors (we know of no studies of the biomass of houseplants in an individual neighborhood, much less a city, and which likely varies due to cultural trends) but even if plant biomass in houses is larger than we have estimated, it will remain dwarfed by other forms of biomass in gray habitats. This appears to have long been true. Uruk, one of the first cities in the world, is described in the epic of Gilgamesh as being one third dwellings, one third clay pits (for brick making), and one third orchards. In other words, like many modern cities, Uruk was about two thirds gray habitat. However, this depiction underrepresents the extent to which cities, be they modern Manhattan or ancient Uruk, are gray inasmuch as the vertical dimension of buildings leads them to have more floor area and volume than would be suggested based on their footprint alone. For example, a NESCent Working Group on the Evolutionary Biology of the Built Environment et al. (2015) calculated that the floor space of Manhattan is twice that of the outdoor habitat (green and gray together) due to extensive multilevel buildings.
Superficially, one might imagine that there are relatively few species that live primarily in the gray habitats of cities. However, recent studies of bodies (McDonald et al., 2018) and homes (Dunn et al., 2013; Barberán et al., 2015; Bertone et al., 2016) suggest that the diversity of species in urban gray habitats can be high. Host dependent taxa make up a relatively large percentage of all species in cities. For example, human bodies, as well as those of pets, can host many thousands of bacteria species (McDonald et al., 2018), thousands of bacteriophage species (Camarillo-Guerrero et al., 2021), hundreds or perhaps even thousands of kinds of viruses that infect human cells (Liang and Bushman, 2021), tens of fungal species (Huseyin et al., 2017), and a modest diversity of protists. Most of these species are only found in environments where humans or pets are present. Human homes can contain tens of thousands of bacteria and fungal species (Barberán et al., 2015), thousands of animal species (Madden et al., 2016), and many other taxa. No one has yet estimated what proportion of these species are primarily house dwellers, but for those taxa that are well-studied, the number seems high. Of the more than one thousand arthropod species found in homes in Raleigh, North Carolina (Bertone et al., 2016), no fewer than a hundred of those species dwell primarily in homes (M. Trautwein, pers. comm.) and many of those animal species seem likely to harbor host-specific bacteria and other microbe species and, in turn, host specific bacteriophages (Raymond and Erdos, 2022).
Another component of island “area” that has been argued to be important is the flux of energy, typically measured as Net Primary Productivity (NPP). In the green habitats of cities, NPP is probably a reasonable proxy for energy flux. However, in the gray parts of cities, energy flux is most strongly influenced by human birth and immigration rates (which can be approximated by population size) and per capita flux of food into cities (which varies by an order of magnitude among cultures, cities and regions) as well as per capita food use efficiency/waste production.
Based solely on their biomass or flux of energy, green habitats seem more likely to favor the origin of new species in low density cities and gray habitats in high density cities. However, we hypothesize that the species living in the gray habitats of cities are more likely than those in green habitats to be isolated relative to surrounding environments and, hence, be more likely to diverge. For example, a mourning dove living in the forest of Inwood Park in Manhattan is less likely to be isolated by the boundaries of New York City than a pigeon living in the gray habitats of the city. The mourning dove can move north into more suburban Westchester County and Connecticut (green space), while a pigeon may be limited to areas with greater urbanization (gray space; Carlen and Munshi-South, 2021). Similarly, lineages of Culex pipiens f. molestus living in the very gray habitats of the London Underground (Byrne and Nichols, 1999) or the New York City subway system seem far more likely to diverge among cities than closely related lineages of Culex pipiens f. pipiens living in greener habitats above ground (Aardema et al., 2020).
The Climates of Cities
We also predict that regional climate will shape the evolution that occurs in cities.
One body of thinking, metabolic theory, directly links temperature to rates of evolution via the effects of temperature on metabolic rate. Where conditions are hot, metabolic rates are faster and, as a result, so too are generation times (Brown et al., 2004), especially for ectothermic taxa, be they bacteria, fungi or insects (Sibly et al., 2012). Because the units of time relevant to evolution are not months or years but generations, this should lead to increases in the rate of divergence of hot cities relative to cooler cities (Brown et al., 2004; Burger, 2021). On top of the direct effects of temperature, more tropical conditions (which is to say, both hot and wet) have been argued to be associated with both faster speciation rates and lower extinction rates in some taxa, such as mammals (Rolland et al., 2014), although not necessarily others, including plants and birds (Rabosky et al., 2015; Igea and Tanentzap, 2020).
Generally speaking, we predict that the rate of the origination of new lineages should be highest in large, tropical cities. Per our earlier predictions, the rate of origination of new species in those big, tropical cities should be greatest among those species in gray habitats. A simple test of the prediction that hot cities will be hotspots of evolution would be to consider the extent of population differentiation among and within populations of the same species from cities of differing sizes along climatic gradients, from tundra to tropics (or whatever part of the gradient is possible given the species being studied). We expect to find a tendency toward higher population differentiation (as measured by FST), and a greater frequency of lineage divergence in populations from hotter temperatures when compared to conspecific populations from colder temperatures.
Climate can also indirectly influence the rates of speciation in cities via its effects on the potential for spillover species to colonize urban habitats, become isolated from their rural conspecifics and then diverge from those conspecifics as they adapt to urban habitats. Where conditions favor high regional biodiversity, such as in the tropics, the species pool of taxa able to potentially colonize cities, adapt to those cities and spillover to become urban endemics is high. This phenomenon has been best studied in the context of parasites (we use the term broadly so as to include pathogens). The regional diversity of human parasite species, as well as parasites with the potential to become human parasite species, is most diverse where mammals and birds are most diverse (Dunn et al., 2010). This is both because a subset of parasites (zoonotics) are dependent on wild bird or mammal species (and have diverged with them via codiversification) and because the same factors that have favored bird and mammal diversity have favored parasite diversity. As a result, disease ecologists predict that the potential for the evolution of new emergent diseases in humans to evolve anew is greatest in tropical settings, especially where human populations are large (big cities) and where big human populations and their gray habitats are adjacent to big green habitats (Jones et al., 2008). For vectored parasites, an added complexity of such spill-over scenarios, particularly in regions with high biodiversity, relates to the layers of evolutionary change that can occur in vectors, parasites, hosts and alternate hosts. For example, the tube and subway-dwelling lineage of Culex pipiens, Culex pipiens f. molestus, is more likely than the aboveground lineage C. pipiens f. pipiens to feed on mammals than on birds. But the hybrid of the two lineages appears to feed on both birds and mammals. As a result, it has been hypothesized the hybrid of these two mosquito lineages is more likely to vector West Nile virus from birds to mammals such as humans (Huang et al., 2009). Were C. pipiens f. pipiens to become more exclusively dependent on mammals (due perhaps to isolation in underground habitats with less contiguity with aboveground habitats), it might facilitate the origin of an exclusively mammal-dependent variant of West Nile virus.
Climate can also indirectly influence rates of speciation and extinction by modulating the selection acting in different regions (Jezkova and Wiens, 2018) or, in our case, cities. Imagine two cities that are close together geographically but have very different climates. One might be at a low elevation (Santa Cruz, Bolivia, for instance) and the other at a high elevation (La Paz, Bolivia). In this scenario, the difference in climate between cities is likely to lead to more rapid divergence of species between those two cities as a function of selection imposed by that divergent climate. For example, cooler, high-elevation conditions have long been predicted to lead to selection for larger body size than warmer, low-elevation conditions (Smith et al., 1995). As a result, selection might be expected to favor the evolution of larger body size in populations in La Paz compared to their conspecifics in Santa Cruz.
Beyond the effects of differences between cities in climate, differences between cities in culture, management and, more generally, human behavior, have the potential to influence the particular traits that are favored and that shape and magnitude of selection imposed on these traits and their divergence between or among cities. Human actions likely to influence selective pressures differently in different cities include the provisioning of food (e.g., Bosse et al., 2017; Guiry and Buckley, 2018), the influence of buildings and pavement on temperatures and humidity (Diamond and Martin, 2020), the proliferation of light (Hopkins et al., 2018), sound (Derryberry and Luther, 2021), and pollution, and the use of biocides (e.g., Brans et al., 2021). While many of these selective pressures are ubiquitous across cities, the strength of their effects will often vary, within and between cities, due to differences in urban design, planning and management (Rivkin et al., 2019; Des Roches et al., 2021). Moreover, the altered biodiversity of cities can lead to changes in biotic interactions and their associated selection pressures. For example, reduced forest cover in cities has changed patterns of selection on gall size in the classic goldenrod gall fly system by reducing the incidence of bird predation on gallmakers (Start et al., 2018). Similarly, but less well explored, the city environment can alter microbial abundance and diversity, potentially affecting selection on important host-microbe associations (e.g., Barnes et al., 2021; Berlow et al., 2021; Murray-Stoker and Johnson, 2021). Although a full consideration of the directions of selection in cities is beyond our scope (see Diamond and Martin, 2021), we consider some of the traits likely to be favored by selection in cities in Box 1 in Supplementary Material.
Evidence to Date
Here, we consider some of this divergence documented to date in different urban habitats and the extent to which it follows hypotheses and predictions 1 through 6. We draw examples from around the world, but with a particular focus on Manhattan and, more generally, New York City, where our team has many years of experience.
Gray Habitats (Bodies)
As we've noted, host dependent taxa make up a relatively large percentage of all species in the gray habitats of cities. Taxa that depend on the bodies of their hosts have the potential to diverge more rapidly than their hosts in part due to the effects of generation times. Pigeons were introduced to North America, and North American cities in particular, in the 1600s. Since then, 200-350 generations of pigeons have transpired (pigeon generation range from seven months to two years; Shao et al., 2020). Pigeon body and feather lice, on the other hand, have experienced roughly 7,000 generations in the same time period (Martin, 1934). No study has compared the relative rates of divergence of pigeons and pigeon lice within or among cities (though doing so would be an excellent test of urban biogeographic theory). However, one recent study considered divergence of two species of pigeon lice within a single Utah city. Divergence was discovered to have occurred among populations of pigeon lice, even though they were separated by no physical barriers and were within seven kilometers of each other (DiBlasi et al., 2018). And, just as predicted based on urban biogeographic theory, their divergence reflected their mode of dispersal: pigeon body lice, which disperse from parents to offspring (i.e. vertical transmission), diverged more than did pigeon wing lice, which can disperse from one group of pigeons to another, by riding like tiny cowboys on pigeon-dependent flies (DiBlasi et al., 2018).
In considering human associates, we can study the origin of new lineages by looking to the past when human populations were separated by geographic barriers and geographically isolated groups of people might have acted, in essence, like proto-cities. Many species associated with human bodies show divergence among hominin and, more narrowly, human populations. For example, human lice (genus Pediculus) have diverged relative to the lice of chimpanzees, but human Pediculus have further diverged into five genetically distinct lineages (Reed et al., 2004; Amanzougaghene et al., 2020) and are also recognized as two ecological forms: head and body lice. Head and body lice are often described as the same species (Buxton, 1947; Burgess, 1995; Light et al., 2008) as are the different lineages of Pediculus lice. The divergence among some of these lineages is several-fold greater than that, for example, between species of mockingbirds in the Galapagos that helped to crystallize Darwin's thinking on the origin of species (Arbogast et al., 2006).
Similar divergence has occurred in many of the parasites and pathogens that have been well-studied (Perry, 2014), including face mites (Palopoli et al., 2015), and Mycobacterium tuberculosis, the bacteria responsible for causing tuberculosis (Freschi et al., 2021) as well as species that do not live full-time on human bodies. The common bed bug (Cimex lectularius) likely evolved from blood feeding ectoparasites of cave-dwelling bats, with at least one lineage switching to hominins some 245,000 years ago (Usinger, 1966; Balvín et al., 2012; Roth et al., 2019) either in Africa or the Middle East. Today, this species is confined to buildings and dependent on human mediated dispersal. Populations of C. lectularius show greater genetic differentiation at larger spatial scales (Saenz et al., 2012; Rosenfeld et al., 2016) in line with the expectation for divergence to occur among cities or groups of cities. Across human body associates, we predict that where urban human populations remain relatively separate, there remains the potential for further divergence. Where such populations move frequently, such divergence is likely to be foiled by gene flow.
In addition to heirloom body associates, the origin of new lineages and species has also been (all too often) witnessed in the context of spillover species; such species are most often noted when they are emerging infectious diseases (Jones et al., 2008; Allen et al., 2017).
Gray Habitats (Stored Food, Food Waste)
Recent research has revealed thousands of species of arthropods living in homes, including many indoor endemics (Bertone et al., 2016; Madden et al., 2016), many of which are found in all continents except Antarctica. The ubiquity of these species, and many others like them, present a kind of paradox. The paradox is that these species have spread to hundreds or even thousands of cities (as can be seen for the Indian mealmoth in Figure 3) with extraordinarily different conditions and yet have not diverged into distinct lineages or species. Assuming these globally distributed taxa have had such a distribution for many generations (as often seems to be the case) there are just two resolutions to this paradox of ubiquity. One is that global gene flow of these species among cities is far greater than appreciated and that, in essence, a vast network of movements of household spiders, centipedes, flies, moths and cockroaches has gone unnoticed. The other possibility is that divergence has actually occurred in some, many or all of these species, but that such divergence has simply gone unstudied. An intermediate scenario is one in which gene flow is maintained within regions but divergence is occurring among them, as has recently been documented for pavement ants (Tetramorium immigrans; Zhang et al., 2019). For many species, either of these scenarios is possible, in part because few urban species have been studied in sufficient detail with regard to their movement, molecular evolution or morphological diversity so as to allow the test of these scenarios.
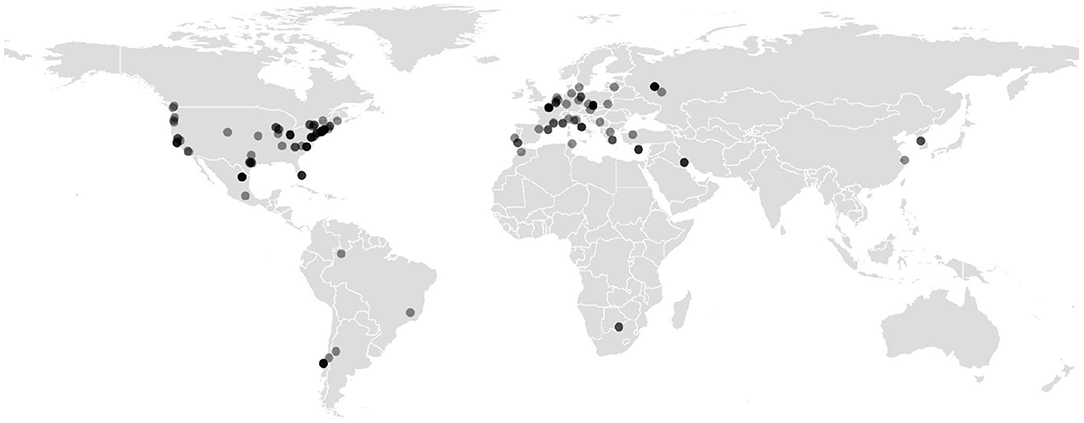
Figure 3. The paradox of ubiquity. The Indian mealmoth (Plodia interpunctella) like many indoor species is a cosmopolitan pest with an extraordinarily broad geographic range, as shown here based on observations made by citizen scientists using the iNaturalist platform. Though its biogeographic history has never been studied, this moth is often described as having evolved from tropical regions and then having spread around the world via commercial ocean freight shipments (though its biogeographic history has never been studied; Cox, 1978; Schulten and Roorda, 1984). Because it is soft-bodied, it is unlikely to be recovered from archaeological sites. Like many grain pests, it may have colonized human settlements along with the domestication of crops and the storage of grain. The paradox with this moth, as with many indoor species, is how it could have spread so widely and yet failed to diverge or even speciate. This paradox has layers; the Indian mealmoth is host to specialist parasites, including baculoviruses. Have they diverged among regions? We do not know. One intriguing possibility is that indianmeal moth baculoviruses, by shuffling genetic material into and out of hosts (Gilbert et al., 2014; Raymond and Erdos, 2022) might have actually facilitated evolution and local adaptation in Indian mealmoths. Testing whether this has occurred as well as the many predictions about where the diversity of novel lineages is greatest (in big, hot, cities) or least (in smaller cities and or regions with more movements of grains) is a straightforward project that awaits an interested researcher.
Two of the handful species in which the potential for regional adaptation and divergence has been studied are house flies (Musca domestica) and German cockroaches (Blattella germanica). Detailed studies of both of these species suggest that they are examples in which ubiquitous urban-associated insect taxa hide cryptic divergence that has occurred and continues to unfold. Beginning in the 1970s, studies of house flies collected outdoors in North America (where they are introduced) by Bryant (1977), revealed a latitudinal gradient in fly body size, with larger flies at higher elevations and latitudes, in accordance with Bergmann's rule (Bergmann, 1848). Based on laboratory rearing studies, Bryant concluded that a substantial component of this variation was due to adaptive genetic differentiation among fly populations (Bryant, 1977). Bryant's work suggests the possibility for isolation, divergence and local adaptation even in urban-associated animal species capable of flying hundreds of meters (Legner and McCoy, 1966).
The earliest record of the German cockroach, B. germanica, is 1763 from Denmark, but it has been associated with humans much longer. Tang et al. (2019) proposed that B. germanica evolved from its free-living close relative, the Asian cockroach, B. asahinai. According to this hypothesis, B. asahinai was then much later transported from southern Asia along agricultural routes into Europe during the 16th and 17th centuries. Unable to survive the cold winters in Europe, it sought shelter in buildings where it scavenged on waste and stored foods. The German cockroach eventually became specialized on, and presumably (although this has yet to be documented) adapted to indoor environments (it became an indoor endemic). As it did, it lost the ability to fly.
Genetic studies show increasing levels of differentiation of German cockroach populations at greater geographic scales, from populations within single apartment buildings, to populations from different cities within a continent, to populations across continents (Crissman et al., 2010; Vargo et al., 2014). These results indicate that the dispersal of German cockroaches on foot is largely limited to movement within a building. As a result, the extent of divergence among buildings, cities and regions is likely to be determined by human movement patterns and control measures. Generally speaking, German cockroaches seem to have the potential to diverge among cities, contingent on just how much and where humans unwittingly move (or control the movement of) the cockroaches. Because of their ubiquity, German cockroaches would be an excellent candidate to study with regard to the rate of their divergence as a function of city size and climate. To date, however, no genetic studies of German cockroaches have explicitly considered the effects of city size, and the population genetics and phylogeography of tropical German cockroaches are essentially unknown.
In studying oceanic islands, Kisel and Barraclough (2010) concluded that, among the taxa they studied, snails were the poorest dispersers and required a minimum area for speciation of 0.8 km2, roughly the size of Tesla's plant in Fremont, California. For German cockroaches, it appears as though divergence and even speciation could conceivably occur at even smaller scales. Crissman et al. (2010) found panmixia when comparing rooms within apartment buildings in Raleigh, North Carolina, but genetic differentiation among apartment buildings separated by just a few kilometers. They conclude that gene flow among apartments is sufficiently rare that human assisted movement of German cockroaches is uncommon and that divergence among apartments is likely to be even greater when selection pressures differ (Crissman et al., 2010). The strongest selection pressure on German cockroaches, like many urban insects, is often due to insecticides. Insecticide resistance has evolved repeatedly and rapidly in German cockroaches (Fardisi et al., 2019). Where insecticide use is common in one building, but not in those that surround it, reduced gene flow together with selection have the potential to lead to very rapid divergence.
As the evolution of more urban arthropod species is studied, it is likely that the details of their evolutionary dynamics and trajectory will differ due to details of their biology. For example, bed bug populations are generally founded by a very small number of individuals resulting in low levels of observed heterozygosity, high levels of relatedness and high levels of inbreeding (Booth et al., 2012, 2015; Saenz et al., 2012; Fountain et al., 2014; Raab et al., 2016), whereas populations of the German cockroach exhibit high levels of observed heterozygosity and low coefficients of relatedness (Booth, 2018). If there are no additional population introductions, the low levels of genetic diversity in bed bug populations should render genetic drift a potent force in the evolution of bed bug populations, whereas selection will be the predominant force in the more genetically diverse populations of German cockroaches. As more species found in the gray habitats of cities are studied, it is likely that their evolutionary stories are even more divergent than those of the species that are well studied to date.
As for species other than arthropods in homes, the same paradox of ubiquity seen in indoor arthropod species appears, for now, to prevail. Many indoor fungal species, including many species of Penicillium, now appear to have relatively global distributions. Early work tended to assume that these species dispersed readily around the globe and perhaps even long had global distributions even in the absence of movements by humans. For some species, this may indeed be the case; Penicillium chrysogenum appears to include more than one lineage/species with relatively frequent gene flow among continents (Henk et al., 2011). However, as other ubiquitous fungal species have been studied, many have been revealed to contain cryptically divergent lineages or even species (Taylor et al., 2007). Some ubiquitous household fungi are like German cockroaches in that they have achieved their global distribution recently, followed by divergence. For example, brewer's yeast, Saccharomyces cerevisiae, appears to have evolved in China and subsequently spread globally, but has since diverged into multiple regional lineages; these lineages have not been well enough studied within particular cities to know if more local divergence and adaptation is occurring (Pontes et al., 2020).
Gray Habitats (Outdoors)
Waste is a key element of outdoor gray habitats in cities. Anthropogenic waste spills over into the outdoors in cities in a variety of forms. These forms include intentional litter (as Figure 4 demonstrates), open trash cans, poorly sealed trash cans and dumpsters, illegal dumping, feces from people (Burt et al., 2021), dogs, cats, canaries and the occasional ferret, and food that is intentionally given to animals, whether in the form of bread tossed to birds, bird seed, or bowls of food left out for stray domestic animals (Burt et al., 2021). It seems very likely that the total quantity of this waste scales with population density and Gross National Income (this is true at least for food waste; Thi et al., 2015). Undoubtedly, the amount of spilled waste differs per capita among cities, but the key factors governing this variation also remain largely unexplored except for individual cities. In New York City, for example, a combination of development patterns (i.e. lack of alleys to house sealed dumpsters), sanitation practices, and cultural tolerance of littering and public garbage have led to much greater levels of human waste available to commensal species than is found in other cities (Nagle, 2014).
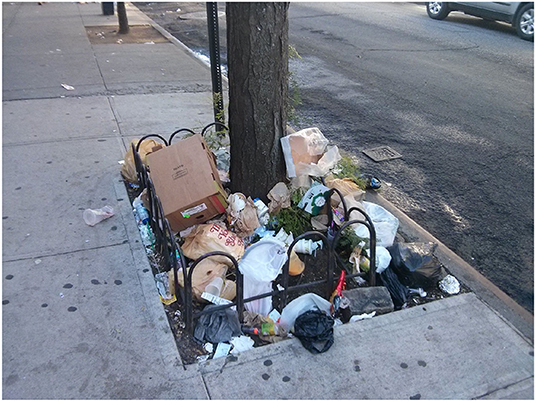
Figure 4. An example of a trophic subsidy from the gray habitats of Manhattan into a green habitat. Here one sees a small “green” habitat in the form of a tree in a tree pit in New York City. In this scene, the organisms living in the gray habitat around the tree–such as pavement ants–are subsidized both by the carbon produced by the tree and the plants growing around the tree and by the human garbage mounded around the tree. Photo by Jason Munshi-South, 2018.
Among the best-studied species dependent on human food waste in cities are mice (Mus spp.) and rats (Rattus spp.) These rodents spread early around the world with humans (Armitage, 1993; Puckett et al., 2016), but have subsequently become relatively isolated (Puckett et al., 2016), particularly in the last decades as shipboard sanitation procedures have become more rigorous. Thanks to this history of spread and isolation, combined with their relatively short generation times (at least for mammals), the potential for divergence, diversification and adaptive radiation among human associated rodents is great.
The evolving relationship between mice, rats and human food began many thousands of years ago. The house mouse (Mus musculus) was naturally present in western Asia and the Middle East, and evolved commensalism as early as 15,000 ya alongside increasingly sedentary forager settlements (Weissbrod et al., 2017). House mice expanded out of the Levant as humans developed agricultural settlements and large stores of domesticated grain (Cucchi et al., 2012; Li Y. et al., 2021). Today, house mice live in virtually every region that humans live and their abundance is likely to scale with human population sizes. The history of movements of house mice can be reconstructed because of the genetic differentiation that has already occurred among house mouse populations (Jones et al., 2013). The functional morphological differentiation associated with this molecular divergence has not been fully explored, but results from latitudinal gradients have revealed substantial genomic and morphological evolution (Ferris et al., 2021). Given that house mouse habitat ranges from subarctic cities to wet tropical cities to desert cities which vary in climate, the food resources available, and composition of pathogens and predators, it is likely that many house mouse adaptations to novel environments remain undiscovered. In coming decades and, especially, millenia, it is conceivable that house mice would diverge among cities and megacities such that each of many cities has its own endemic house mouse lineage.
The black rat, Rattus rattus, evolved in southern India, potentially in association with the Indus River Valley civilization or its progenitors (Ervynck, 2002), and appears in the zooarchaeological record as early as the 3rd millennium BC in Mesopotamia. A few thousand years later they were present in the Mediterranean, and then widely spread by the Roman Empire by the thirteenth century AD (Yu et al., 2021). Indian Ocean trade networks also moved the black rat along the eastern coast of Africa and several islands beginning in the seventh Century AD.
A separate lineage that was likely a different species (Rattus tanezumi) spread out of southeast Asia but the historical record is less clear on timing. Rattus exulans evolved in the Indonesian archipelago (possibly Flores) before spreading to other islands, parts of southeast Asia, and Oceania (Thomson et al., 2014).
The brown (or Norway) rat, Rattus norvegicus, evolved in northern China, but did not spread to other areas until much later than the other commensal rats. Around the thirteenth century AD, the brown rat had spread throughout China into southeast Asia, and then were moved westward across Eurasia by humans following coastal trade routes. Once established in western Europe, the brown rat was moved all over the world by European imperial powers (Puckett et al., 2016, 2020).
While none of these rat species were restricted to urban settings during their years of regional and global movement, their dependence on human food sources meant that they tended to be most dense where humans were most dense, which is to say, in cities. Where modern divergence among populations of these rat species has been studied, it has been observed among regions (as a function of ancient movements via ships; Puckett et al., 2016), among cities within regions (as a function of modern patterns of movement; Combs et al., 2018a) and even within cities (related to very local barriers). Divergence has even recently been documented within the borough of Manhattan within New York city (Combs et al., 2018b).
Pigeons–Pigeons (Columbia livia), also known as rock doves, were domesticated as a food source 5,000-10,000 years ago in the Mediterranean, north Africa, and near east where they nested on rocky cliffs and fed on seeds. Pigeons were moved around the world as Europeans colonized the Americas, Africa, and Asia. In these new cities, pigeons thrived on buildings that mimicked cliffs while feeding upon spilled grain and discarded food waste. Despite being closely associated with evolution and a model of artificial selection since the theory was first proposed by Charles Darwin (Darwin, 1868), to date, relatively minimal population genetic research has been conducted on pigeons. Jacob et al. (2015) found that for pigeons in European cities genetic distance increased with geographic distance, suggesting that different urban populations of pigeons have the potential to become genetically isolated. In 2018, Tang et al. found that pigeons in Singapore form a single genetic cluster, likely arising from an introduction in the early 1900s (Tang et al., 2018). Despite the proximity of Singapore to other Asian cities (Johor Bahru, Malaysia is just 20 kilometers away) Singaporean pigeons are isolated from and likely diverging from populations of other cities. More recently, Carlen and Munshi-South (2021) showed that pigeons in the United States Northeastern megacity, which ranges from Boston, MA to Washington, DC, formed two genetic clusters—one cluster that includes Boston, MA and Providence, RI samples, and a second cluster that included all samples collected south of Providence, RI. Carlen and Munshi-South (2021) proposed that this clustering may be a result of the decreased urbanization across Connecticut; this less urban area acts as a barrier for pigeons which prefer “city life.” Interestingly, the fate of the pigeons isolated in Singapore and those diverging among cities in the Northeastern megacity is likely to be quite different. It seems likely that the pigeons of Singapore will continue to diverge from those of other tropical Asian cities, particularly, as Tang et al. (2018) note, given that pigeons do not like to cross water. In contrast, if the Northeastern megacity becomes more connected in Connecticut (Arnold et al., 2020), gene flow may foil the ongoing divergence between populations.
Green Habitats
The vast majority of studies of aspects of island biogeography in cities have been in green areas, whether focusing on insect diversity in parks (Fattorini et al., 2017), or, say, the diversity of ants, fungi and bacteria in medians and other green space (Reese et al., 2016). Similarly, recent reviews of urban evolution have focused on these green habitats (Johnson et al., 2015; Johnson and Munshi-South, 2017), perhaps because the number of gray habitat species is mistakenly believed to be, as Johnson and Munshi-South (2017) put it,” small but consequential.” Yet, as we've already pointed out, we suspect urban green habitats are less likely to be areas to be centers for the origin of new species than are gray habitats.
In searching for the origin of new species in the green habitats of cities, one might look for species that have begun to adapt to aspects of those habitats that are differentiated from those of surrounding green areas (in other words, spillover species in the process of becoming urban endemics). In their recent review, Johnson and Munshi-South (2017) identify a number of features of the green habitats in cities that can favor the kind of local adaptation associated with spillover (we point readers to their review for a broader consideration). Green habitats in cities are almost invariably warmer than those of surrounding areas, which can favor adaptations for those warmer conditions, as has been observed in Crested anoles (Campbell-Staton et al., 2020), water fleas (Brans et al., 2017) and one species of acorn ant (Martin et al., 2021). Urban habitats are also likely to be higher in concentrations of biocides and other pollutants and thus can favor adaptations that confer resistance. Urban adaptation to heavy-metal pollution, for instance, appears to be taxonomically widespread (e.g., Andrew et al., 2019; Jacquier et al., 2021). Urban green habitats are also often patchier than non-urban habitats, which can lead to adaptations for dealing with such patchiness. For example, Crepis sancta, an annual Mediterranean plant, has adapted its seeds to pavement by investing less in seed dispersal in cities than in nearby non-fragmented rural populations (Cheptou et al., 2008). In addition, species from green habitats can adapt to gray habitats, as is the case for the subset of emerging infectious diseases that are transmitted human to human, such as some coronaviruses, for example.
Implicit in these examples of urban adaptation in green habitat species is the strength of selection has been sufficiently great relative to rates of gene flow from non-urban populations so as to allow local adaptation. Eventually, we predict that a subset of these urban adapted populations become urban endemic species, as is the case for many emerging infectious diseases (Wolfe et al., 2007; Jones et al., 2008), but also some other types of organisms. For example, urban adapted populations of one plant species, Lepidium virginicum, have spread to other cities (Yakub and Tiffin, 2017); whether they have begun to diverge among those cities has not yet been studied.
Another context in which divergence might have already occurred in green habitats in cities is for the subset of such habitats that do not correspond to the green habitats immediately outside of cities. In some cases, humans intentionally create new habitats with no precise analog. For example, green rooftops represent a novel habitat type and are becoming common in some cities and one can imagine scenarios (see below) in which the tops of buildings across entire cities are green. At least to date, green roof tops tend to be dominated by a small number of typically introduced species of Sedum (sometimes called “stonecrop”) that are not common outside of cities (Oberndorfer et al., 2007). Species dependent on such rooftop plant species might show divergence among cities or, for very poorly dispersing species with short generation times, even among roofs. Similar dynamics could, at least in theory, occur for species dependent on horticultural plants that are common in cities but not outside of them. For example, there are many thousands of cultivars of Rhododendrons, some common in cities, and the species dependent on these plants might diverge among cultivars, among cities or both (Lewis et al., 2019). In other cases new versions of existing habitat types might be recreated in cities, but distant from their natural analogs. For example, Larson (2004) has argued that many of the species that thrive in cities are cliff-dwelling species for which buildings and sidewalks offer conditions similar to those found on talus slopes and shear rock faces. Except in very particular situations, most of the cliff species that thrive in cities are unlikely to be able to maintain gene flow with natural populations of the same species living on cliffs.
Discussion
We conclude by discussing the implications of our understanding of the origin of species in cities through the use of “scenario thinking.” Scenarios are, as Raskin et al. (2005) put it, “plausible, challenging and relevant stories about how the future might unfold.” Scenario thinking has a long history when considering the future, but is perhaps most often encountered in considering population growth (Meadows, 1972) and climate change (Milbrath, 1989; Burrows et al., 1992; Leggett et al., 1992). In the context of climate change, climate scientists have elaborated a variety of scenarios with regard to the key actions humans might take and how those actions will affect climate (Leggett et al., 1992; van Vuuren et al., 2011). Yet, although these scenarios are increasingly detailed (see O'Neill et al., 2014), they remain vague with regard to factors most likely to affect evolutionary trajectories. Here, we consider scenarios that differ with regard to the human actions most likely to affect the evolution of new species among urban islands. The time horizon for the climate scientists is typically relatively short term (2100 is a common benchmark); we take a longer view.
We begin by considering a variety of transportation scenarios, given the importance (prediction 5) of human-mediated movement of organisms in cities. Arthur C. Clarke (Michael, 1964), echoing earlier futurists (de Sola Pool, 1977), posited that a tradeoff exists between travel and communication technology wherein as communication technology increases in ease of use, the need and desire to physically travel decreases, particularly for work. The germ of such a scenario can be seen in the persistence of remote work situations throughout the COVID-19 pandemic. By some measures, more than half of employees would like to retain jobs that are either fully remote (11%) or sometimes remote (52%; Alexander et al., 2021). We hypothesize that such a scenario could become ever more extreme, resulting in what we call the locophilous scenario in which humans eat local food, live where they were born, and no longer travel beyond their home city (all travel occurs via virtual reality), or perhaps travel only for pleasure but not for work. Such a scenario would lead urban species to become very isolated. This scenario maximizes the rate of divergence among cities. In the locophilous scenario, each of the urban hubs in Figure 2 (as well as those elsewhere around the world) is likely to be like an island for most taxa, the continent of North America a series of urban archipelagos. The species most likely to diverge would be those least able to disperse on their own, likely the same species most likely to diverge on islands (e.g., Kisel and Barraclough, 2010). In this scenario, isolation combined with the differences among cities would be very likely to lead to adaptive evolution in each city and, as a result, the origin of species whose traits match the conditions of the city in which they live. We can expect big species of mammals, such as rats, in cold cities such as Anchorage and small species in hot cities such as Atlanta, Georgia. There might be resistant organisms in cities that overuse biocides but susceptible (and more easily managed) species in those that don't. As this occurs, we will face new questions, such as whether new species that evolve in isolated cities require conservation if they become rare. Will the new, dwarf rat of Rio deserve protection? Do these local species become like mascots for particular populations? Will they be embraced or will they be attacked? If the answers to these questions differ place to place, the resulting differences in selection pressures will likely lead to even more divergence.
At the opposite extreme, we might imagine a mobile future scenario in which movement of individuals around the world increases. Futurists have imagined scenarios in which hyperloops, flying cars, driverless vehicles and hypersonic aircraft actually allow increased travel per capita within and among cities (e.g., Muoio, 2015; See also… Mire, 2019). Increased per capita travel would be augmented further by increases in population sizes. Such movement could be fueled either on the basis of the continuing use of fossil fuel until the last dregs are gone (a kind of business as usual scenario with regard to energy sources; eg. Leggett et al., 1992) or while relying on new technologies. In either case, divergence of species among cities will occur primarily in the subset of urban-thriving species that are unable to move via the transport systems of the future. The more like plane-travel future transport is, the more likely it is that the species that spread (and hence fail to diverge) will be small. One imagines it will be relatively hard for a rat, much less a fox, to sneak onto a hypersonic plane or a hyperloop pod. However, smaller species are likely to have no trouble. On the one hand, travel restrictions have proven to slow the spread of coronaviruses. On the other hand, the Omicron variant of the virus that causes COVID-19 was able to reach virtually every country in the world in weeks or months (Grépin et al., 2021). Similarly, despite many attempts to control the arrival of microbes on space stations, an extraordinary diversity of bacteria, fungi and even arthropods that have traveled in space shuttles to the International Space Station (ISS; Checinska Sielaff et al., 2019) or, previously, the MIR (Ott et al., 2004). The more like big ship travel future transport is, the more likely it is that some big species will maintain gene flow among cities and fail to diverge (rats travel on ships but not often on planes). The more like space travel future transport is, the more likely it is that many species become isolated and diverge.
It is an open question what an ideal transportation scenario looks like with regard to the knock on effects of diversification of wild species on humans. What kind of diversification should we try to favor? For parasites of humans, ideally we want to reduce the probability that they evolve in the first place, but we also want to be able to reduce their spread once they evolve (as we have seen with novel variants of the virus that causes COVID-19), as well as the potential that they evolve increased virulence or increased abilities to spread.
In any scenario in which global travel occurs with the frequency that it occurs today, such an ability will require the ability to detect new species, perhaps stop travel to particular regions when necessary (although the COVID-19 pandemic has shown just how difficult this can be), perhaps the ability to rapidly detect the presence of particular pathogens. It seems as though the goal for dangerous pest species, such as mosquito vectors of pathogens, should be similar. What should be the goal with regard to other urban species? Should we try to engender a greater diversity, for example, of indoor spiders? Should we restrict the movement of urban ornamentals and hence their potential for rapid evolution in situ, perhaps driven by natural selection and cultural selection? The answers are not clear-cut, in no small part because these questions have barely been posed.
A second set of scenarios relate to biomass and the relative dominance of different parts of the urban food web and food landscape as well as to where and how waste is processed. At one extreme is the gray world vision of the future in which urban biomass is nearly entirely composed of anthromass, petmass and stored food and waste and in which that collective biomass is subsidized by trophic subsidies from outside of urban ecosystems. This vision is embodied in the example of the part of Manhattan around Times Square in Figure 1. One can imagine low human population density gray world futures, in which low to medium density cities–geographically dominated by suburbs–cover enormous areas, as is currently the case for parts of the Northeastern megacity in the United States (Figure 2) and is predicted to be the case in the future for the southeastern megacity of Charlanta (Terando et al., 2014). Alternatively, future cities might be both gray and extraordinarily high density. In the former scenario, low density megacities may act like giant islands. In the latter, geographically smaller, more concentrated cities may act like smaller islands with more independent fates. A consideration of these two extremes, which we might call several large megacities or many small microcitie), connects the consideration of future cities with the large literature focused on ideal sizes of habitat patches for conservation (Diamond, 1975; Diamond and May, 1976; Simberloff and Abele, 1982).
At the other extreme relative to the gray-world model of the future, we might envision a green-world model of the futurein which plant biomass dominates. Imagine buildings covered with forests, built of living walls covered with cyanobacterial mats (Chew et al., 2021) and filled with vertical gardens (Despommier, 2010) sustained with nutrients from human waste streams. Such a city might feature native plants (and their dependent species) from natural habitats that are mimicked by urban habitats. Cliff-dwelling plants, even rare species, might be gardened on the sides of those buildings not covered in algae (Larson, 2004). Epiphytes might be gardened on buildings in tropical cities. In this scenario, we might imagine cities inspired by ecological insights, cities that rely on gardened urban ecosystems to carry out ecological processes that are now engineered or, as with waste treatment, displaced. In many ways, a green-world future seems very much preferable to a gray-world future; however, if a green world future includes not only high plant biomass but also a high biomass of wild birds and mammals, it might also favor the emergence of spillover species of parasites (Gibb et al., 2020).
In either a green- or gray-world future, cities might also differ with regard to their efficiency of resource use. At one extreme is probably the current reality, a high-waste future in which much of the food that comes into the city is wasted [Parfitt et al., 2010; Food and Agriculture Organization of the United Nations (FAO), 2011], ending up in open landfills or dumped into rivers, or possibly transported out of the city into waste treatment facilities. Were this to continue, we predict it will fuel the evolution of waste dependent species indoors and outdoors. At the opposite extreme, we might imagine a closed loop, low-waste future in which food waste is minimized and in which unavoidable food waste is composted or up-cycled into new products and human waste is used in energy production or for other uses. In this latter scenario, the reduction of food waste in urban systems would radically affect the outdoor gray and green habitats of cities. A temporary version of such a scenario was mimicked during the early days of the pandemic when major cities were quarantined, restaurants were closed down and patterns of food waste availability were dramatically altered. During this interval, rat populations appear to have needed to seek out alternate food sources and moved from commercial to residential areas (Parsons et al., 2021). In an urban future in which waste is greatly reduced, one wonders, would rats and pigeons still persist in cities?
Our predictions relative to scenarios of transportation, green/gray habitat distribution and waste use assume people continue to live in big, and perhaps even ever bigger cities. However, over the intermediate time scale of centuries and millennia urban populations might decline, whether because of declining global populations, urban disease outbreaks, the collapse of governance or some mix of these factors (Diamond, 2006). Periods in which cities decline in population can be found across cultures and time periods in the past and seem likely in the future, even if only in some regions (such as those with climates outside of the current human niche; Hsiang et al., 2013; Cookson et al., 2019). In this regard, we might think of evolution in cities as having an additional temporal dynamic, beyond that of the generations of populations of organisms, the dynamic of the rise and fall of cities. The rise and fall of cities is akin to the emergence of oceanic islands due to volcanism and their disappearance due to sea level rise. It may often occur over shorter time periods and yet nonetheless represents a key component of urban evolutionary dynamics. In this regard, it would be useful to study what happened to urban associated species in the past when cities collapsed, for example, following the fall of the Western Roman empire when the population of the city of Rome declined by as much as 90%. It is known that some urban species, such as a crab that dwells in the Cloaca Maxima of Rome (Scalici et al., 2008), were associated primarily or even exclusively with Roman cities. What happened to such species as the people of Rome died and dispersed? What happened to the species living in Angkor Wat (Carter et al., 2019)? Or in the homes associated with Mayan and Aztec urbanizations? We do not yet know, but we could know. Urban ecologists working with archaeologists could better understand the future of biodiversity by studying the rise and fall of ancient cities.
If we consider even longer time scales of millions, tens of millions or hundreds of millions of years, humans will go extinct. That all species go extinct has been called the first law of paleontology (Marshall, 2017). When humans do go extinct, many of the species that have evolved in response to urbanization are likely to go extinct as well, suffering from a great spasm of coextinction (Stork and Lyal, 1993). In this way, the tableau that results from the origin of urban species unfolds, is shaped and even exists only so long as we exist.
Data Availability Statement
Publicly available datasets were analyzed in this study. This data can be found here: Department of Information Technology Telecommunication (2018)−6 in Resolution are available at: https://data.cityofnewyork.us/Environment/Land-Cover-Raster-Data-2017-6in-Resolution/he6d-2qns, United States Commutes and Megaregions data for GIS are available at the following Figshare link: https://doi.org/10.15131/shef.data.4110156, and Plodia occurrence data available through GBIF at https://doi.org/10.15468/cade8j.
Author Contributions
All authors listed have made a substantial, direct, and intellectual contribution to the work and approved it for publication.
Conflict of Interest
The authors declare that the research was conducted in the absence of any commercial or financial relationships that could be construed as a potential conflict of interest.
The handling editor CS is currently organizing a Research Topic with one of the authors JB.
Publisher's Note
All claims expressed in this article are solely those of the authors and do not necessarily represent those of their affiliated organizations, or those of the publisher, the editors and the reviewers. Any product that may be evaluated in this article, or claim that may be made by its manufacturer, is not guaranteed or endorsed by the publisher.
Supplementary Material
The Supplementary Material for this article can be found online at: https://www.frontiersin.org/articles/10.3389/fcosc.2022.761449/full#supplementary-material
References
Aardema, M. L., vonHoldt, B. M., Fritz, M. L., and Davis, S. R. (2020). Global evaluation of taxonomic relationships and admixture within the Culex pipiens complex of mosquitoes. Parasit. Vectors 13, 8. doi: 10.1186/s13071-020-3879-8
Adhikari, B. K., Barrington, S., and Martinez, J. (2006). Predicted growth of world urban food waste and methane production. Waste Manag. Res. J. Int. Solid Wastes Public Clean. Assoc. ISWA 24, 421–433. doi: 10.1177/0734242X06067767
Alexander, A., De Smet, A., Langstaff, M., and Ravid, D. (2021). What Employees are Saying About the Future of Remote Work.
Allen, T., Murray, K. A., Zambrana-Torrelio, C., Morse, S. S., Rondinini, C., Di Marco, M., et al. (2017). Global hotspots and correlates of emerging zoonotic diseases. Nat. Commun. 8, 1124. doi: 10.1038/s41467-017-00923-8
Amanzougaghene, N., Fenollar, F., Raoult, D., and Mediannikov, O. (2020). Where are we with human lice? a review of the current state of knowledge. Front. Cell. Infect. Microbiol. 20, 474. doi: 10.3389/fcimb.2019.00474
Andrew, S. C., Taylor, M. P., Lundregan, S., Lien, S., Jensen, H., and Griffith, S. C. (2019). Signs of adaptation to trace metal contamination in a common urban bird. Sci. Total Environ. 650, 679–686. doi: 10.1016/j.scitotenv.2018.09.052
Arbogast, B. S., Drovetski, S. V., Curry, R. L., Boag, P. T., Seutin, G., Grant, P. R., et al. (2006). The origin and diversification of Galapagos mockingbirds. Evol. Int. J. Org. Evol. 60, 370–382. doi: 10.1111/j.0014-3820.2006.tb01113.x
Arnold, C., Wilson, E., Hurd, J., and Civco, D. (2020). 30 years of land cover change in connecticut, usa: a case study of long-term research, dissemination of results, and their use in land use planning and natural resource conservation. Land 9, 255. doi: 10.3390/land9080255
Balvín, O., Munclinger, P., Kratochvíl, L., and Vilímová, J. (2012). Mitochondrial DNA and morphology show independent evolutionary histories of bedbug Cimex lectularius (Heteroptera: Cimicidae) on bats and humans. Parasitol. Res. 111, 457–469. doi: 10.1007/s00436-012-2862-5
Barberán, A., Dunn, R. R., Reich, B. J., Pacifici, K., Laber, E. B., Menninger, H. L., et al. (2015). The ecology of microscopic life in household dust. Proc. R. Soc. B Biol. Sci. 282, 20151139. doi: 10.1098/rspb.2015.1139
Barnes, E. M., Kutos, S., Naghshineh, N., Mesko, M., You, Q., and Lewis, J. D. (2021). Assembly of the amphibian microbiome is influenced by the effects of land-use change on environmental reservoirs. Environ. Microbiol. 23, 4595–4611. doi: 10.1111/1462-2920.15653
Bar-On, Y. M., Phillips, R., and Milo, R. (2018). The biomass distribution on Earth. Proc. Natl. Acad. Sci. 115, 6506–6511. doi: 10.1073/pnas.1711842115
Bergmann, C. (1848). Über die Verhältnisse der Wärmeökonomie der Thiere zu ihrer Größe. Gött. Stud. 3, 595–708.
Berlow, M., Phillips, J. N., and Derryberry, E. P. (2021). Effects of urbanization and landscape on gut microbiomes in white-crowned sparrows. Microb. Ecol. 81, 253–266. doi: 10.1007/s00248-020-01569-8
Bertone, M. A., Leong, M., Bayless, K. M., Malow, T. L. F., Dunn, R. R., and Trautwein, M. D. (2016). Arthropods of the great indoors: characterizing diversity inside urban and suburban homes. PeerJ. 4, e1582. doi: 10.7717/peerj.1582
Booth, W. (2018). Population genetics of bed bugs, in Advances in the Biology and Management of Modern Bed Bugs, eds. Doggett, S., Miller, D., and Lee, C.-Y., (Hoboken, NJ.: John Wiley and Sons), pp. 173–182.
Booth, W., Balvín, O., Vargo, E. L., Vilímov,á, J., and Schal, C. (2015). Host association drives genetic divergence in the bed bug, Cimex lectularius. Mol. Ecol. 24, 980–992. doi: 10.1111/mec.13086
Booth, W., Saenz, V. L., Santangelo, R. G., Wang, C., Schal, C., and Vargo, E. L. (2012). Molecular markers reveal infestation dynamics of the bed bug (Hemiptera: Cimicidae) within apartment buildings. J. Med. Entomol. 49, 535–546. doi: 10.1603/ME11256
Bosse, M., Spurgin, L. G., Laine, V. N., Cole, E. F., Firth, J. A., Gienapp, P., et al. (2017). Recent natural selection causes adaptive evolution of an avian polygenic trait. Science. 17:3298. doi: 10.1126/science.aal3298
Brans, K. I., Almeida, R. A., and Fajgenblat, M. (2021). Genetic differentiation in pesticide resistance between urban and rural populations of a nontarget freshwater keystone interactor, Daphnia magna. Evol. Appl. 14, 2541–2552. doi: 10.1111/eva.13293
Brans, K. I., Jansen, M., Vanoverbeke, J., Tüzün, N., Stoks, R., and De Meester, L. (2017). The heat is on: Genetic adaptation to urbanization mediated by thermal tolerance and body size. Glob. Change Biol. 23, 5218–5227. doi: 10.1111/gcb.13784
Brown, J., Gillooly, J., Allen, A., Savage, V., and m West, G. (2004). Toward a metabolic theory of ecology. Ecology 85, 1771–1789. doi: 10.1890/03-9000
Brown, J. H. (1948). Alberta: The only rat-free province in Canada. Can. J. Public Health Rev. Can. Santee Publique 39, 367–374.
Bryant, E. H. (1977). Morphometric adaptation of the housefly, musca domestica l., in the united states. Evol. Int. J. Org. Evol. 31, 580–596. doi: 10.1111/j.1558-5646.1977.tb01046.x
Burger, J., Anderson, R., Balk, M., and Fristoe, T. (2019). A Constraint-based model of Dynamic Island Biogeography: environmental history and species traits predict hysteresis in populations and communities. Front. Biogeogr. 3, 44383. doi: 10.21425/F5FBG44383
Burger, R. (2021). Universal rules of life: metabolic rates, biological times and the equal fitness paradigm. Ecol. Lett. 21:509. doi: 10.22541/au.161226628.83797509/v1
Burgess, I. F. (1995). Human Lice and their Management, in Advances in Parasitology, eds. Baker, J. R., Muller, R., and Rollinson, D., (Academic Press), pp. 271–342.
Burrows, B. C., Mayne, A. J., and Newbury, P. (1992). Into the Twenty-First Century: A Handbook for a Sustainable Future. Twickenham: New York Univ Pr.
Burt, S. A., Vos, C. J., Buijs, J. A., and Corbee, R. J. (2021). Nutritional implications of feeding free-living birds in public urban areas. J. Anim. Physiol. Anim. Nutr. 105, 385–393. doi: 10.1111/jpn.13441
Buxton, P. A. (1947). The Louse: An Account of the Lice Which Infest Man, Their Medical importance and Control. 2nd edition. Arnold, E.,
Byers, K. A., Booker, T. R., Combs, M., Himsworth, C. G., Munshi-South, J., Patrick, D. M., et al. (2021). Using genetic relatedness to understand heterogeneous distributions of urban rat-associated pathogens. Evol. Appl. 14, 198–209. doi: 10.1111/eva.13049
Byrne, K., and Nichols, R. A. (1999). Culex pipiens in London Underground tunnels: differentiation between surface and subterranean populations. Heredity 82, 7–15. doi: 10.1038/sj.hdy.6884120
Camarillo-Guerrero, L. F., Almeida, A., Rangel-Pineros, G., Finn, R. D., and Lawley, T. D. (2021). Massive expansion of human gut bacteriophage diversity. Cell 184, 1098–1109. doi: 10.1016/j.cell.2021.01.029
Campbell-Staton, S., Winchell, K., Rochette, N., Fredette, J., Maayan, I., Schweizer, R., et al. (2020). Parallel selection on thermal physiology facilitates repeated adaptation of city lizards to urban heat islands. Nat. Ecol. Evol. 4, 1–7. doi: 10.1038/s41559-020-1131-8
Carlen, E., and Munshi-South, J. (2021). Widespread genetic connectivity of feral pigeons across the Northeastern megacity. Evol. Appl. 14, 150–162. doi: 10.1111/eva.12972
Carter, A., Stark, M., Quintus, S., Zhuang, Y., Wang, H., Piphal, H., et al. (2019). Temple occupation and the tempo of collapse at Angkor Wat, Cambodia. Proc. Natl. Acad. Sci. U. S. A. 19, 116. doi: 10.1073/pnas.1821879116
Checinska Sielaff, A., Urbaniak, C., Mohan, G. B. M., Stepanov, V. G., Tran, Q., Wood, J. M., et al. (2019). Characterization of the total and viable bacterial and fungal communities associated with the International Space Station surfaces. Microbiome 7, 50. doi: 10.1186/s40168-019-0666-x
Cheptou, P.-O., Carrue, O., Rouifed, S., and Cantarel, A. (2008). Rapid evolution of seed dispersal in an urban environment in the weed Crepis sancta. Proc. Natl. Acad. Sci. 105, 3796–3799. doi: 10.1073/pnas.0708446105
Chew, K. W., Khoo, K. S., Foo, H. T., Chia, S. R., Walvekar, R., and Lim, S. S. (2021). Algae utilization and its role in the development of green cities. Chemosphere 268, 129322. doi: 10.1016/j.chemosphere.2020.129322
Combs, M., Byers, K. A., Ghersi, B. M., Blum, M. J., Caccone, A., Costa, F., et al. (2018a). Urban rat races: spatial population genomics of brown rats (Rattus norvegicus) compared across multiple cities. Proc. R. Soc. B Biol. Sci. 285, 20180245. doi: 10.1098/rspb.2018.0245
Combs, M., Puckett, E. E., Richardson, J., Mims, D., and Munshi-South, J. (2018b). Spatial population genomics of the brown rat (Rattus norvegicus) in New York City. Mol. Ecol. 27, 83–98. doi: 10.1111/mec.14437
Conrad, Z., Niles, M. T., Neher, D. A., Roy, E. D., Tichenor, N. E., and Jahns, L. (2018). Relationship between food waste, diet quality, and environmental sustainability. PloS One 13, e0195405. doi: 10.1371/journal.pone.0195405
Cookson, E., Hill, D. J., and Lawrence, D. (2019). Impacts of long term climate change during the collapse of the Akkadian Empire. J. Archaeol. Sci. 106, 1–9. doi: 10.1016/j.jas.2019.03.009
Cox, P. D. (1978). The importance of phycitines on imports to Britain in relation to their biology, in Proceedings of the Second International Working Conference on Stored-Product Entomology (Nigeria: Ibadan), 380–395.
Crissman, J. R., Booth, W., Santangelo, R. G., Mukha, D. V., Vargo, E. L., and Schal, C. (2010). Population genetic structure of the German cockroach (Blattodea: Blattellidae) in apartment buildings. J. Med. Entomol. 47, 553–564. doi: 10.1093/jmedent/47.4.553
Cucchi, T., Auffray, J.-C., and Vigne, J.-D. (2012). “On the origin of the house mouse synanthropy and dispersal in the Near East and Europe: zooarchaeological review and perspectives,” in Evolution of the House Mouse, eds. Macholán, M., Baird, S. J. E., Munclinger, P, and Piálek, J., (Cambridge: Cambridge University Press).
Darwin, C. (1868). The Variation of Animals and Plants under Domestication (Volume 1): Darwin, Charles: 9781406842494: Amazon.com: Books. London: John Murray Available online: https://www.amazon.com/Variation-Animals-Plants-under-Domestication/dp/1406842494 (accessed August 7, 2021).
Davies, T. G. E., Field, L. M., and Williamson, M. S. (2012). The re-emergence of the bed bug as a nuisance pest: implications of resistance to the pyrethroid insecticides. Med. Vet. Entomol. 26, 241–254. doi: 10.1111/j.1365-2915.2011.01006.x
de Sola Pool, I. (1977). The communications/transportation tradeoff. Policy Stud. J. 6, 74. doi: 10.1111/j.1541-0072.1977.tb01167.x
Department of Information Technology Telecommunication (2018). Land Cover Raster Data (2017) – 6in Resolution. Available online: https://opendata.cityofnewyork.us/.
Derryberry, E. P., and Luther, D. (2021). What is Known—and not Known—About Acoustic Communication in an Urban Soundscape. Integr. Comp. Biol. 61, 1783–1794. doi: 10.1093/icb/icab131
Des Roches, S., Brans, K. I., Lambert, M. R., Rivkin, L. R., Savage, A. M., Schell, C. J., et al. (2021). Socio-eco-evolutionary dynamics in cities. Evol. Appl. 14, 248–267. doi: 10.1111/eva.13065
Des Roches, S., Post, D. M., Turley, N. E., Bailey, J. K., Hendry, A. P., Kinnison, M. T., et al. (2018). The ecological importance of intraspecific variation. Nat. Ecol. Evol. 2, 57–64. doi: 10.1038/s41559-017-0402-5
Despommier, D. D. (2010). The vertical farm: feeding the world in the 21st century. 1st ed. New York, NY: Thomas Dunne Books/St. Martin's Press.
Diamond, J. (1975). The Island Dilemma: lessons of modern biogeographic studies for the design of natural reserves. Biol. Conserv. 7, 129–146. doi: 10.1016/0006-3207(75)90052-X
Diamond, J. M. (2006). Collapse: how societies choose to fail or succeed. Harmondsworth: Penguin Books.
Diamond, J. M., and May, R. M. (1976). Island biogeography and the design of natural reserves, in Theoretical ecology, ed. May, R. M., (Philadelphia: Saunders), 163–186.
Diamond, S. E., and Martin, R. A. (2020). Evolutionary consequences of the urban heat island, in Urban Evolutionary Biology (Oxford, New York: Oxford University Press), 91–110.
Diamond, S. E., and Martin, R. A. (2021). Evolution in Cities. Annu. Rev. Ecol. Evol. Syst. 52, 519–540. doi: 10.1146/annurev-ecolsys-012021-021402
DiBlasi, E., Johnson, K. P., Stringham, S. A., Hansen, A. N., Beach, A. B., Clayton, D. H., et al. (2018). Phoretic dispersal influences parasite population genetic structure. Mol. Ecol. 27, 2770–2779. doi: 10.1111/mec.14719
Dunn, R. R. (2010). Global Mapping of Ecosystem Disservices: The Unspoken Reality that Nature Sometimes Kills us. Biotropica 42, 555–557. doi: 10.1111/j.1744-7429.2010.00698.x
Dunn, R. R., Davies, J., Harris, N. C., and Gavin, M. C. (2010). Global drivers of human pathogen richness and prevalence. Proc. R. Soc. B Biol. Sci. 277, 2587–2595. doi: 10.1098/rspb.2010.0340
Dunn, R. R., Fierer, N., Henley, J. B., Leff, J. W., and Menninger, H. L. (2013). Home life: factors structuring the bacterial diversity found within and between homes. PloS One 8, e64133. doi: 10.1371/journal.pone.0064133
Ervynck, A. (2002). Sedentism of Urbanism? On the Origin of the Commensal Black Rat (Rattus rattus), in Bones and the Man: Studies in Honour of Don Brothwell, eds. Dobney, K., and O'Conner, T., (Oxford England : Oakville, CT: Oxbow Books).
Fahey, T. J., Siccama, T. G., Driscoll, C. T., Likens, G. E., Campbell, J., Johnson, C. E., et al. (2005). The biogeochemistry of carbon at hubbard brook. Biogeochemistry 75, 109–176. doi: 10.1007/s10533-004-6321-y
Fardisi, M., Gondhalekar, A. D., Ashbrook, A. R., and Scharf, M. E. (2019). Rapid evolutionary responses to insecticide resistance management interventions by the German cockroach (Blattella germanica L.). Sci. Rep. 9, 8292. doi: 10.1038/s41598-019-44296-y
Fattorini, S., Mantoni, C., Simoni, L., and Galassi, D. (2017). Island biogeography of insect conservation in urban green spaces. Environ. Conserv. 45, 1–10. doi: 10.1017/S0376892917000121
Ferris, K. G., Chavez, A. S., Suzuki, T. A., Beckman, E. J., Phifer-Rixey, M., Bi, K., et al. (2021). The genomics of rapid climatic adaptation and parallel evolution in North American house mice. PLOS Genet. 17, e1009495. doi: 10.1371/journal.pgen.1009495
Food and Agriculture Organization of the United Nations (FAO) (2011). Global food losses and food waste: extent, causes and prevention. Rome, Italy: Food and Agriculture Organization of the United Nations (FAO).
Fountain, T., Duvaux, L., Horsburgh, G., Reinhardt, K., and Butlin, R. K. (2014). Human-facilitated metapopulation dynamics in an emerging pest species, Cimex lectularius. Mol. Ecol. 23, 1071–1084. doi: 10.1111/mec.12673
Freschi, L., Vargas, R., Husain, A., Kamal, S. M. M., Skrahina, A., Tahseen, S., et al. (2021). Population structure, biogeography and transmissibility of Mycobacterium tuberculosis. Nat. Commun. 12, 6099. doi: 10.1038/s41467-021-26248-1
Gibb, R., Redding, D. W., Chin, K. Q., Donnelly, C. A., Blackburn, T. M., Newbold, T., et al. (2020). Zoonotic host diversity increases in human-dominated ecosystems. Nature 584, 398–402. doi: 10.1038/s41586-020-2562-8
Gilbert, C., Chateigner, A., Ernenwein, L., Barbe, V., Bézier, A., Herniou, E. A., et al. (2014). Population genomics supports baculoviruses as vectors of horizontal transfer of insect transposons. Nat. Commun. 5, 3348. doi: 10.1038/ncomms4348
Golem, D. L., and Byrd-Bredbenner, C. (2015). Emergency Food Supplies in Food Secure Households. Prehospital Disaster Med. 30, 359–364. doi: 10.1017/S1049023X15004884
Grépin, K. A., Ho, T.-L., Liu, Z., Marion, S., Piper, J., Worsnop, C. Z., et al. (2021). Evidence of the effectiveness of travel-related measures during the early phase of the COVID-19 pandemic: a rapid systematic review. BMJ Glob. Health 6, e004537. doi: 10.1136/bmjgh-2020-004537
Guiry, E., and Buckley, M. (2018). Urban rats have less variable, higher protein diets. Proc. R. Soc. B Biol. Sci. 285, 20181441. doi: 10.1098/rspb.2018.1441
Henk, D. A., Eagle, C., Brown, K., Berg, M. A., Dyer, P., Peterson, S., et al. (2011). Speciation despite globally overlapping distributions in Penicillium chrysogenum: The population genetics of Alexander Fleming's lucky fungus. Mol. Ecol. 20, 5244. doi: 10.1111/j.1365-294X.2011.05244.x
Hopkins, G. R., Gaston, K. J., Visser, M. E., Elgar, M. A., and Jones, T. M. (2018). Artificial light at night as a driver of evolution across urban–rural landscapes. Front. Ecol. Environ. 16, 472–479. doi: 10.1002/fee.1828
Hsiang, S. M., Burke, M., and Miguel, E. (2013). Quantifying the influence of climate on human conflict. Science. 13, 537. doi: 10.1126/science.1235367
Huang, S., Hamer, G. L., Molaei, G., Walker, E. D., Goldberg, T. L., Kitron, U. D., et al. (2009). Genetic variation associated with mammalian feeding in Culex pipiens from a West Nile virus epidemic region in Chicago, Illinois. Vector Borne Zoonotic Dis. Larchmt. N 9, 637–642. doi: 10.1089/vbz.2008.0146
Huseyin, C. E., O'Toole, P. W., Cotter, P. D., and Scanlan, P. D. (2017). Forgotten fungi-the gut mycobiome in human health and disease. FEMS Microbiol. Rev. 41, 479–511. doi: 10.1093/femsre/fuw047
Igea, J., and Tanentzap, A. J. (2020). Angiosperm speciation cools down in the tropics. Ecol. Lett. 23, 692–700. doi: 10.1111/ele.13476
Jacob, G., Prévot-Julliard, A.-C., and Baudry, E. (2015). The geographic scale of genetic differentiation in the feral pigeon (Columba livia): implications for management. Biol. Invas. 17, 23–29. doi: 10.1007/s10530-014-0713-2
Jacquier, L., Doums, C., Four-Chaboussant, A., Peronnet, R., Tirard, C., and Molet, M. (2021). Urban colonies are more resistant to a trace metal than their forest counterparts in the ant Temnothorax nylanderi. Urban Ecosyst. 24, 561–570. doi: 10.1007/s11252-020-01060-9
Jezkova, T., and Wiens, J. J. (2018). Testing the role of climate in speciation: New methods and applications to squamate reptiles (lizards and snakes). Mol. Ecol. 27, 2754–2769. doi: 10.1111/mec.14717
Johnson, M. T. J., and Munshi-South, J. (2017). Evolution of life in urban environments. Science 358, eaam8327. doi: 10.1126/science.aam8327
Johnson, M. T. J., Thompson, K. A., and Saini, H. S. (2015). Plant evolution in the urban jungle. Am. J. Bot. 102, 1951–1953. doi: 10.3732/ajb.1500386
Jones, E. P., Eager, H. M., Gabriel, S. I., Jóhannesdóttir, F., and Searle, J. B. (2013). Genetic tracking of mice and other bioproxies to infer human history. Trends Genet. 29, 298–308. doi: 10.1016/j.tig.2012.11.011
Jones, K. E., Patel, N. G., Levy, M. A., Storeygard, A., Balk, D., Gittleman, J. L., et al. (2008). Global trends in emerging infectious diseases. Nature 451, 990–993. doi: 10.1038/nature06536
Kaza, S., Yao, L. C., Bhada-Tata, P., and Van Woerden, F. (2018). What a Waste 2.0: A Global Snapshot of Solid Waste Management to 2050. Washington, DC: World Bank.
Kisel, Y., and Barraclough, T. G. (2010). Speciation has a spatial scale that depends on levels of gene flow. Am. Nat. 175, 316–334. doi: 10.1086/650369
Lachance, M.-A., and Fedor, A. N. (2014). Catching speciation in the act: Metschnikowia bowlesiae sp. nov., a yeast species found in nitidulid beetles of Hawaii and Belize. Antonie Van Leeuwenhoek 105, 541–550. doi: 10.1007/s10482-013-0106-z
Lack, J. B., Hamilton, M. J., Braun, J. K., Mares, M. A., and Van Den Bussche, R. A. (2013). Comparative phylogeography of invasive Rattus rattus and Rattus norvegicus in the U.S. reveals distinct colonization histories and dispersal. Biol. Invasions 15, 1067–1087. doi: 10.1007/s10530-012-0351-5
Larson, D. (2004). The Urban Cliff Revolution: New Findings on the Origins and Evolution of Human Habitats. 1st edition. Markham: Fitzhenry and Whiteside.
Leggett, J., Pepper, W., and Swart, R. (1992). Emissions scenarios for IPCC: An update. Camb. Univ. Press Camb. UK WMO/UNEP Intergovernment Panel Clim Change 11, 69–95.
Legner, E. F., and McCoy, C. W. (1966). The housefly, musca domestica linnaeus, as an exotic species in the western hemisphere incites biological control studies1. Can. Entomol. 98, 243–248. doi: 10.4039/Ent98243-3
Lewis, E., Phoenix, G. K., Alexander, P., David, J., and Cameron, R. W. F. (2019). Rewilding in the Garden: are garden hybrid plants (cultivars) less resilient to the effects of hydrological extremes than their parent species? a case study with primula. Urban Ecosyst. 22, 841–854. doi: 10.1007/s11252-019-00865-7
Li, B., Deng, A., Li, K., Hu, Y., Li, Z., Xiong, Q., et al. (2021). Viral infection and transmission in a large, well-traced outbreak caused by the SARS-CoV-2 Delta variant. medRxiv 2021.07.07.21260122.
Li, Y., Fujiwara, K., Osada, N., Kawai, Y., Takada, T., Kryukov, A. P., et al. (2021). House mouse Mus musculus dispersal in East Eurasia inferred from 98 newly determined complete mitochondrial genome sequences. Heredity 126, 132–147. doi: 10.1038/s41437-020-00364-y
Liang, G., and Bushman, F. D. (2021). The human virome: assembly, composition and host interactions. Nat. Rev. Microbiol. 19, 514–527. doi: 10.1038/s41579-021-00536-5
Light, J. E., Toups, M. A., and Reed, D. L. (2008). What's in a name: The taxonomic status of human head and body lice. Mol. Phylogenet. Evol. 47, 1203–1216. doi: 10.1016/j.ympev.2008.03.014
Liu, G., Xu, K., Zhang, X., and Zhang, G. (2014). Factors influencing the service lifespan of buildings: An improved hedonic model. Habitat Int. 43, 274–282. doi: 10.1016/j.habitatint.2014.04.009
Losos, J. B., and Schluter, D. (2000). Analysis of an evolutionary species-area relationship. Nature 408, 847–850. doi: 10.1038/35048558
MacArthur, R. H., and Wilson, E. O. (1967). The Theory of Island Biogeography. Princeton: University Press.
Madden, A. A., Barberán, A., Bertone, M. A., Menninger, H. L., Dunn, R. R., and Fierer, N. (2016). The diversity of arthropods in homes across the United States as determined by environmental DNA analyses. Mol. Ecol. 25, 6214–6224. doi: 10.1111/mec.13900
Marshall, C. (2017). Five palaeobiological laws needed to understand the evolution of the living biota. Nat. Ecol. Evol. 1, 0165. doi: 10.1038/s41559-017-0165
Martin, M. (1934). Life History and Habits of the Pigeon Louse (Columbicola columbae (Linnaeus). Can. Entomol. 66, 1. doi: 10.4039/Ent666-1
Martin, R. A., Chick, L. D., Garvin, M. L., and Diamond, S. E. (2021). In a nutshell, a reciprocal transplant experiment reveals local adaptation and fitness trade-offs in response to urban evolution in an acorn-dwelling ant. Evolution 75, 876–887. doi: 10.1111/evo.14191
McDonald, D., Hyde, E., Debelius, J. W., Morton, J. T., Gonzalez, A., Ackermann, G., et al. (2018). American gut: an open platform for citizen science microbiome research. mSystems 3, e00031–e00018. doi: 10.1128/mSystems.00031-18
McKenzie, B., Koerber, W., Fields, A., Benetsky, M., and Rapino, M. (2010). Commuter-Adjusted Population Estimates: ACS 2006-10. Journey Work Migr. Stat. Branch US Census Bur., 20, 10.
Meadows, D. L. (1972). Toward a science of social forecasting. Proc. Natl. Acad. Sci. U. S. A. 69, 3828–3831. doi: 10.1073/pnas.69.12.3828
Michael, L. (1964). The Knowledge Explosion. BBC Horiz. Ser. 1. Available online at: https://www.youtube.com/watch?v=KT_8-pjuctM (accessed December 12, 2021).
Milbrath, L. W. (1989). Envisioning a Sustainable Society: Learning Our Way Out. SUNY Press. Available online at: https://www.amazon.com/Envisioning-Sustainable-Society-Learning-Environmental/dp/0791401634 (Accessed January 4, 2022).
Miles, L. S., Dyer, R. J., and Verrelli, B. C. (2018). Urban hubs of connectivity: contrasting patterns of gene flow within and among cities in the western black widow spider. Proc. Biol. Sci. 285, 20181224. doi: 10.1098/rspb.2018.1224
Mire, S. (2019). What's The Future Of Transportation?16 Experts Share Their Insights. Disruptor Dly. Available online at: https://www.disruptordaily.com/future-of-transportation/ (accessed January 5, 2022).
Muoio, D. (2015). 6 Predictions About the Future of Transportation. Bus. Insid. Available online at: https://www.businessinsider.com/6-predictions-about-the-future-of-transportation-2015-11 (Accessed January 5, 2022).
Murray-Stoker, D., and Johnson, M. T. J. (2021). Ecological consequences of urbanization on a legume-rhizobia mutualism. bioRxiv. doi: 10.1111/oik.08341
Nagle, R. (2014). Picking Up: On the Streets and Behind the Trucks with the Sanitation Workers of New York City. New York: Farrar, Straus and Giroux.
Natural Area Conservancy (2021). Nature Map of NYC. Available online at: https://naturalareasnyc.org/map (accessed August 8, 2021).
Neiderud, C.-J. (2015). How urbanization affects the epidemiology of emerging infectious diseases. Infect. Ecol. Epidemiol. 5, 10. doi: 10.3402/iee.v5.27060
Nelson, G. D., and Rae, A. (2016). An economic geography of the united states: from commutes to megaregions. PLOS ONE 11, e0166083. doi: 10.1371/journal.pone.0166083
NESCent Working Group on the Evolutionary Biology of the Built Environment Martin, L. J. Adams R. I. Bateman A. Bik H. M. Hawks J.. (2015). Evolution of the indoor biome. Trends Ecol. Evol. 30, 223–232. doi: 10.1016/j.tree.2015.02.001
Norton, B. A., Evans, K. L., and Warren, P. H. (2016). Urban Biodiversity and Landscape Ecology: Patterns, Processes and Planning. Curr. Landsc. Ecol. Rep. 1, 178–192. doi: 10.1007/s40823-016-0018-5
Oberndorfer, E., Lundholm, J., Bass, B., Coffman, R. R., Doshi, H., Dunnett, N., et al. (2007). Green roofs as urban ecosystems: ecological structures, functions, and services. BioScience 57, 823–833. doi: 10.1641/B571005
O'Neill, B. C., Kriegler, E., Riahi, K., Ebi, K. L., Hallegatte, S., Carter, T. R., et al. (2014). A new scenario framework for climate change research: the concept of shared socioeconomic pathways. Clim. Change 122, 387–400. doi: 10.1007/s10584-013-0905-2
Opatova, V., and Arnedo, M. A. (2014). Spiders on a hot volcanic roof: colonisation pathways and phylogeography of the canary islands endemic trap-door spider titanidiops canariensis (Araneae, Idiopidae). PloS One 9, e115078. doi: 10.1371/journal.pone.0115078
Ott, C. M., Bruce, R. J., and Pierson, D. L. (2004). Microbial characterization of free floating condensate aboard the Mir space station. Microb. Ecol. 47, 133–136. doi: 10.1007/s00248-003-1038-3
Palopoli, M., Fergus, D., Minot, S., Pei, D., Simison, W., Fernandez-Silva, I., et al. (2015). Global divergence of the human follicle mite Demodex folliculorum: Persistent associations between host ancestry and mite lineages. Proc. Natl. Acad. Sci. 9, 112. doi: 10.1073/pnas.1512609112
Panagiotakopulu, E., Buckland, P., and Kemp, B. (2010). Underneath Ranefer's floors – urban environments on the desert edge. J. Archaeol. Sci. - J ARCHAEOL SCI 37, 474–481. doi: 10.1016/j.jas.2009.09.048
Parfitt, J., Barthel, M., and Macnaughten, S. (2010). Food waste within food supply chains: quantification and potential for change to 2050 |. Philos. Trans. R. Soc. B Biol. Sci. 365, 3065–3081. doi: 10.1098/rstb.2010.0126
Parsons, M. H., Richardson, J. L., Kiyokawa, Y., Stryjek, R., Corrigan, R. M., Deutsch, M. A., et al. (2021). Rats and the COVID-19 pandemic: considering the influence of social distancing on a global commensal pest. J. Urban Ecol. 7, juab027. doi: 10.1093/jue/juab027
Perry, G. H. (2014). Parasites and human evolution. Evol. Anthropol. Issues News Rev. 23, 218–228. doi: 10.1002/evan.21427
Pierce, E. R. (1930). A Method of determining the Prevalence oî Rats in Ships. Med. Off. 43, 222–224.
Polis, G. A., Anderson, W. B., and Holt, R. D. (1997). Toward an Integration of Landscape and Food Web Ecology: The Dynamics of Spatially Subsidized Food Webs. Annu. Rev. Ecol. Syst. 28, 289–316. doi: 10.1146/annurev.ecolsys.28.1.289
Pontes, A., Hutzler, M., Brito, P. H., and Sampaio, J. P. (2020). Revisiting the taxonomic synonyms and populations of saccharomyces cerevisiae—phylogeny, phenotypes, ecology and domestication. Microorganisms 8, 903. doi: 10.3390/microorganisms8060903
Puckett, E. E., Orton, D., and Munshi-South, J. (2020). Commensal rats and humans: integrating rodent phylogeography and zooarchaeology to highlight connections between human societies. Bioessays 42:1900160. doi: 10.1002/bies.201900160
Puckett, E. E., Park, J., Combs, M., Blum, M. J., Bryant, J. E., Caccone, A., et al. (2016). Global population divergence and admixture of the brown rat (Rattus norvegicus). Proc. R. Soc. B Biol. Sci. 283, 20161762. doi: 10.1098/rspb.2016.1762
Raab, R. W., Moore, J. E., Vargo, E. L., Rose, L., Raab, J., Culbreth, M., et al. (2016). New Introductions, Spread of Existing Matrilines, and High Rates of Pyrethroid Resistance Result in Chronic Infestations of Bed Bugs (Cimex lectularius L.) in Lower-Income Housing. PloS One 11, e0117805. doi: 10.1371/journal.pone.0117805
Rabosky, D. L., Title, P. O., and Huang, H. (2015). Minimal effects of latitude on present-day speciation rates in New World birds. Proc. R. Soc. B Biol. Sci. 282, 20142889. doi: 10.1098/rspb.2014.2889
Raskin, P., Monks, F., Ribeiro, T., van Vuuren, D., and Zurek, M. (2005). Global Scenarios in Historical Perspective, in Ecosystems and human well-being, pp. 35.
Raymond, B., and Erdos, Z. (2022). Passage and the evolution of virulence in invertebrate pathogens: Fundamental and applied perspectives. J. Invertebr. Pathol. 187, 107692. doi: 10.1016/j.jip.2021.107692
Reed, D. L., Smith, V. S., Hammond, S. L., Rogers, A. R., and Clayton, D. H. (2004). Genetic analysis of lice supports direct contact between modern and archaic humans. PLoS Biol. 2, e340. doi: 10.1371/journal.pbio.0020340
Reese, A. T., Savage, A., Youngsteadt, E., McGuire, K. L., Koling, A., Watkins, O., et al. (2016). Urban stress is associated with variation in microbial species composition-but not richness-in Manhattan. ISME J. 10, 751–760. doi: 10.1038/ismej.2015.152
Rivkin, L. R., Santangelo, J. S., Alberti, M., Aronson, M. F. J., de Keyzer, C. W., Diamond, S. E., et al. (2019). A roadmap for urban evolutionary ecology. Evol. Appl. 12, 384–398. doi: 10.1111/eva.12734
Rolland, J., Condamine, F. L., Jiguet, F., and Morlon, H. (2014). Faster speciation and reduced extinction in the tropics contribute to the mammalian latitudinal diversity gradient. PLoS Biol. 12, e1001775. doi: 10.1371/journal.pbio.1001775
Rosenfeld, J. A., Reeves, D., Brugler, M. R., Narechania, A., Simon, S., Durrett, R., et al. (2016). Genome assembly and geospatial phylogenomics of the bed bug Cimex lectularius. Nat. Commun. 7, 10164. doi: 10.1038/ncomms10164
Roth, S., Balvín, O., Siva-Jothy, M. T., Di Iorio, O., Benda, P., Calva, O., et al. (2019). Bedbugs Evolved before Their Bat Hosts and Did Not Co-speciate with Ancient Humans. Curr. Biol. CB 29, 1847–1853. doi: 10.1016/j.cub.2019.04.048
Rubel, D., and Wisnivesky, C. (2005). Magnitude and distribution of canine fecal contamination and helminth eggs in two areas of different urban structure, Greater Buenos Aires, Argentina. Vet. Parasitol. 133, 339–347. doi: 10.1016/j.vetpar.2005.06.002
Russell, J. C., Clout, M. N., and Towns, D. R. (2007). EARLY DETECTION AND ERADICATION OF INVADING RATS. Manag. Vertebr. Invasive Species. Available online: https://digitalcommons.unl.edu/nwrcinvasive/43 (accessed October 7, 2021).
Saenz, V. L., Booth, W., Schal, C., and Vargo, E. L. (2012). Genetic analysis of bed bug populations reveals small propagule size within individual infestations but high genetic diversity across infestations from the eastern United States. J. Med. Entomol. 49, 865–875. doi: 10.1603/ME11202
Scalici, M., Macale, D., Schiavone, F., Gherardi, F., and Gibertini, G. (2008). Effect of urban isolation on the dynamics of river crabs. Fundam. Appl. Limnol. Arch. Für Hydrobiol. 172, 167–174. doi: 10.1127/1863-9135/2008/0172-0167
Schulten, G. G. M., and Roorda, F. A. (1984). Storage insects in imported products mainly of tropical origin. Entomol. Ber. 44, 65–69.
Shao, Y., Tian, H.-Y., Zhang, J.-J., Kharrati-Koopaee, H., Guo, X., Zhuang, X.-L., et al. (2020). Genomic and phenotypic analyses reveal mechanisms underlying homing ability in Pigeon. Mol. Biol. Evol. 37, 134–148. doi: 10.1093/molbev/msz208
Sibly, R. M., Brown, J. H., and Kodric-Brown, A. (2012). Metabolic Ecology: A Scaling Approach. West Sussex, UK: John Wiley and Sons.
Simberloff, D., and Abele, L. G. (1982). Refuge design and island biogeographic theory: effects of fragmentation. Am. Nat. 120, 41–50. doi: 10.1086/283968
Sjodin, B. M. F., Puckett, E. E., Irvine, R. L., Munshi-South, J., and Russello, M. A. (2021). Global origins of invasive brown rats (Rattus norvegicus) in the Haida Gwaii archipelago. Biol. Invasions 23, 611–623. doi: 10.1007/s10530-020-02390-7
Smith, F. A., Betancourt, J. L., and Brown, J. H. (1995). Evolution of body size in the woodrat over the past 25,000 years of climate change. Science 270, 2012–2014. doi: 10.1126/science.270.5244.2012
Start, D., Bonner, C., Weis, A. E., and Gilbert, B. (2018). Consumer-resource interactions along urbanization gradients drive natural selection. Evol. Int. J. Org. Evol. 72, 1863–1873. doi: 10.1111/evo.13544
Stier, A., Berman, M. G., and Bettencourt, L. (2020). COVID-19 Attack Rate Increases with City Size. Rochester, NY: Social Science Research Network Available online: https://papers.ssrn.com/abstract=3564464 (Accessed January 4, 2022).
Stork, N. E., and Lyal, C. H. C. (1993). Extinction or “co-extinction” rates? Nature 366, 307–307. doi: 10.1038/366307a0
Tang, Q., Bourguignon, T., Willenmse, L., De Coninck, E., and Evans, T. (2019). Global spread of the German cockroach, Blattella germanica. Biol. Invasions 21, 693–707. doi: 10.1007/s10530-018-1865-2
Tang, Q., Low, G. W., Lim, J. Y., Gwee, C. Y., and Rheindt, F. E. (2018). Human activities and landscape features interact to closely define the distribution and dispersal of an urban commensal. Evol. Appl. 11, 1598–1608. doi: 10.1111/eva.12650
Taylor, J. W., Turner, E., Pringle, A., Dettman, J., and Johannesson, H. (2007). Fungal species: thoughts on their recognition, maintenance and selection, in Fungi in the Environment British Mycological Society Symposia., eds. Gadd, G., Dyer, P. S., and Watkinson, S. C., (Cambridge: Cambridge University Press), 313–339.
Terando, A. J., Costanza, J., Belyea, C., Dunn, R. R., McKerrow, A., and Collazo, J. A. (2014). The Southern Megalopolis: using the past to predict the future of urban sprawl in the Southeast U.S. PLoS ONE 9, e102261. doi: 10.1371/journal.pone.0102261
Thi, N. B. D., Kumar, G., and Lin, C.-Y. (2015). An overview of food waste management in developing countries: Current status and future perspective. J. Environ. Manage. 157, 220–229. doi: 10.1016/j.jenvman.2015.04.022
Thomson, V., Aplin, K. P., Cooper, A., Hisheh, S., Suzuki, H., Maryanto, I., et al. (2014). Molecular genetic evidence for the place of origin of the pacific rat, rattus exulans. PLOS ONE 9, e91356. doi: 10.1371/journal.pone.0091356
Toft, C. A., and Schoener, T. W. (1983). Abundance and diversity of orb spiders on 106 bahamian islands: biogeography at an intermediate trophic level. Oikos 41, 411–426. doi: 10.2307/3544101
U.S. Department of Agriculture Economic Research Service (2021). National Household Food Acquisition and Purchase Survey (FoodAPS). Available online at: https://www.ers.usda.gov/data-products/foodaps-national-household-food-acquisition-and-purchase-survey/data-access/ (accessed August 9, 2021).
U.S. Census Bureau (2019). Population Estimates Program (PEP). Available online: Retrieved from https://www.census.gov/quickfacts/ (accessed October 8, 2021).
United Nations Statistics Division (2017). Population density and urbanization. United Nations. Available online at: https://unstats.un.org/UNSD/Demographic/sconcerns/densurb/densurbmethods.htm.
Usinger, R. L. (1966). Monograph of Cimicidae (Hemiptera-Heteroptera). College Park, MD: Entomological Society of America. Available online at: https://www.nhbs.com/monograph-of-cimicidae-hemiptera-heteroptera-book (Accessed August 7, 2021).
van Vuuren, D. P., Edmonds, J., Kainuma, M., Riahi, K., Thomson, A., Hibbard, K., et al. (2011). The representative concentration pathways: an overview. Clim. Change 109, 5. doi: 10.1007/s10584-011-0148-z
Vargo, E. L., Crissman, J. R., Booth, W., Santangelo, R. G., Mukha, D. V., and Schal, C. (2014). Hierarchical genetic analysis of German cockroach (Blattella germanica) populations from within buildings to across continents. PloS One 9, e102321. doi: 10.1371/journal.pone.0102321
von Döhren, P., and Haase, D. (2015). Ecosystem disservices research: A review of the state of the art with a focus on cities. Ecol. Indic. 52, 490–497. doi: 10.1016/j.ecolind.2014.12.027
Walpole, S. C., Prieto-Merino, D., Edwards, P., Cleland, J., Stevens, G., and Roberts, I. (2012). The weight of nations: an estimation of adult human biomass. BMC Public Health 12, 439. doi: 10.1186/1471-2458-12-439
Warren, B. H., Simberloff, D., Ricklefs, R. E., Aguilée, R., Condamine, F. L., Gravel, D., et al. (2015). Islands as model systems in ecology and evolution: prospects fifty years after MacArthur-Wilson. Ecol. Lett. 18, 200–217. doi: 10.1111/ele.12398
Weissbrod, L., Marshall, F. B., Valla, F. R., Khalaily, H., Bar-Oz, G., Auffray, J.-C., et al. (2017). Origins of house mice in ecological niches created by settled hunter-gatherers in the Levant 15,000 y ago. Proc. Natl. Acad. Sci. 114, 4099–4104. doi: 10.1073/pnas.1619137114
Wolfe, N. D., Dunavan, C. P., and Diamond, J. (2007). Origins of major human infectious diseases. Nature 447, 279–283. doi: 10.1038/nature05775
Yakub, M., and Tiffin, P. (2017). Living in the city: urban environments shape the evolution of a native annual plant. Glob. Change Biol. 23, 2082–2089. doi: 10.1111/gcb.13528
Yu, H., Jamieson, A., Hulme-Beaman, A., Conroy, C. J., Knight, B., Speller, C., et al. (2021). Palaeogenomic analysis of black rat (Rattus rattus) reveals multiple European introductions associated with human economic history.
Keywords: urban, future, evolution, island biogeography, scenarios
Citation: Dunn RR, Burger JR, Carlen EJ, Koltz AM, Light JE, Martin RA, Munshi-South J, Nichols LM, Vargo EL, Yitbarek S, Zhao Y and Cibrián-Jaramillo A (2022) A Theory of City Biogeography and the Origin of Urban Species. Front. Conserv. Sci. 3:761449. doi: 10.3389/fcosc.2022.761449
Received: 19 August 2021; Accepted: 14 February 2022;
Published: 11 March 2022.
Edited by:
Christopher John Schell, University of Washington Tacoma, United StatesReviewed by:
Shelly L. Volsche, Boise State University, United StatesNishant Kumar, Wildlife Institute of India, India
Copyright © 2022 Dunn, Burger, Carlen, Koltz, Light, Martin, Munshi-South, Nichols, Vargo, Yitbarek, Zhao and Cibrián-Jaramillo. This is an open-access article distributed under the terms of the Creative Commons Attribution License (CC BY). The use, distribution or reproduction in other forums is permitted, provided the original author(s) and the copyright owner(s) are credited and that the original publication in this journal is cited, in accordance with accepted academic practice. No use, distribution or reproduction is permitted which does not comply with these terms.
*Correspondence: Robert R. Dunn, rrdunn@ncsu.edu