- 1Department of Primatology, Max Planck Institute for Evolutionary Anthropology, Leipzig, Germany
- 2Institute of Conservation Science and Learning, Bristol Zoological Society, Bristol, United Kingdom
- 3Dian Fossey Gorilla Fund, Atlanta, GA, United States
- 4Great Apes Survival Partnership, United Nations Environment Programme, Nairobi, Kenya
- 5Institute of Tropical Forest Conservation, Mbarara University of Science and Technology, Kabale, Uganda
- 6School of Human Sciences, The University of Western Australia, Perth, WA, Australia
- 7Iluka Resources, Stony Creek, VA, United States
Water plays a vital role in many aspects of sustaining life, including thermoregulation. Given that increasing temperatures and more extreme weather events due to climate change are predicted to influence water availability, understanding how species obtain and use water is critical. This is especially true for endangered species in small isolated populations which are vulnerable to drought and the risk of extinction. We examined the relationship between the frequency of water drinking and maximum temperature and rainfall in 21 groups of wild gorillas from the two mountain gorilla populations (Bwindi and Virunga), between 2010 and 2020. In both populations, we found that the frequency of water drinking significantly increased at higher maximum temperatures than cooler ones, but we found no consistent relationship between water drinking and rainfall. We also found that Virunga gorillas relied more on foods with higher water content than Bwindi gorillas, which in part likely explains why they drink water much less frequently. These findings highlight that even in rainforest mammals that gain most of their water requirements from food, access to free-standing water may be important because it likely facilitates evaporative cooling in response to thermoregulatory stress. These results have important implications for conservation and behavior of mountain gorillas in the face of continued increases in temperature and frequency of extreme weather events associated with climate change.
Introduction
Water has numerous essential roles for animals, such as being a medium for chemical reactions to occur, a transporter of metabolic and waste products, a lubricant and shock absorber (Robbins, 1983; Jéquier and Constant, 2010). In addition, water plays a vital role in thermoregulation (National Research Council, 2003; Mitchell et al., 2009; Jéquier and Constant, 2010; Withers et al., 2016). Maintaining water balance is a major homeostatic objective and critical for growth, reproduction and survival (Karasov and del Rio, 2020). Water requirements are influenced by several factors, such as climatic conditions, diet and metabolic rates (Robbins, 1983; Jéquier and Constant, 2010; Karasov and del Rio, 2020).
Terrestrial animals gain water from food (preformed water), metabolic water resulting from the oxidation of macronutrients and through drinking water (Robbins, 1983; Jéquier and Constant, 2010). Most animals rely on free-standing water sources, but some are able to obtain most of their water needs from metabolic and preformed water (Withers et al., 2016). Understanding how animals obtain and use water is particularly important in the face of climate change, as increasing global temperatures and more extreme weather events are predicted to influence water availability and can have a negative impact on animals' ability to maintain homeostasis (Hetem et al., 2014; Fuller et al., 2016; Zhang et al., 2019).
Increasing temperature leads to the increased need for evaporative cooling, particularly in endotherms, which requires water (National Research Council, 2003; Withers et al., 2016; Mitchell et al., 2018; Karasov and del Rio, 2020). To compensate for increased water loss when temperatures are high, animals often increase the amount of water consumption (Adams and Hayes, 2008; Dias et al., 2014; Harris et al., 2015; Mella et al., 2019; Chaves et al., 2021). Animals may also increase water drinking during dry periods, which coincide with the times of highest temperature in some areas (Harris et al., 2015; Mella et al., 2019). In addition, the water content of foods may be lower during times of low rainfall and so animals may need to supplement water intake with drinking (Fuller et al., 2016). Animals may also prioritize water rich foods to supplement preformed water intake during periods of low rainfall (Ciani et al., 2001; Sato et al., 2014).
Decreased water availability has been shown to drive increased mortality rates in several species (Cayton and Haddad, 2018; Riddell et al., 2019; Young et al., 2019; Campos et al., 2020). In contrast, rainforest dwelling species may obtain most or all of their water requirements from their diet (Karasov and del Rio, 2020). However, increasing temperatures may lead to increased water drinking as a means to thermoregulate and avoid dehydration (Dias et al., 2014; Chaves et al., 2021). Monitoring changes in water drinking behavior can serve as an early warning indicator of the impacts of climate change as the duration of dry spells and temperatures increase.
A considerable proportion of primate species (22%; 134 of 604) are predicted to be vulnerable to the impacts of drought (Zhang et al., 2019). Moreover, primate habitats are predicted to experience 10% more warming than the global mean increase in temperature, with 86% of primate species likely to experience increases of over 3°C in maximum temperatures by 2050 (Graham et al., 2016; Carvalho et al., 2019). Higher elevation regions are also experiencing faster increases in temperature than lower elevation ones (Wang et al., 2016). Given the vulnerability of primates to dry spells, it is vital to look for indicators of physiological stress in endangered species, such as changes in the occurrence of water drinking caused by climate change (Chapman et al., 2006; Bernard and Marshall, 2020).
Understanding how endangered mountain gorillas obtain and use water is particularly warranted as they are vulnerable to the risk of extinction for a number of reasons. Only around 1,000 individuals remain in two small isolated mountaintop islands of Uganda, Rwanda, and the Democratic Republic of the Congo over an area of approximately 784 km2 (Eckardt et al., 2019; Granjon et al., 2020). The slow life history of mountain gorillas limits their ability to evolve beneficial adaptions that are better suited to new climatic conditions sufficiently quickly to mitigate the detrimental effects of climate change (Robbins, 2011). Mountain gorillas are also geographically highly restricted due to intense human pressure surrounding their current habitat, such that they cannot disperse to other areas (Robbins, 2011).
Mountain gorillas generally obtain sufficient quantities of water from the vegetation they consume and they rarely drink water (Schaller, 1963). The water content of mountain gorilla foods is high, with most foods comprising between 60 and 90% water (Rothman et al., 2006; Grueter et al., 2016), with little seasonal variation (within-species) in the Virunga Volcanoes (Watts, 1998). Mountain gorilla habitat is characterized by bimodal rainfall distribution (heavier rains in March-May and lighter rains in September-November), with temperature mostly being a function of elevation and showing little seasonal variation (Polansky and Robbins, 2013; Seimon and Phillips, 2015; Diem et al., 2019; Eckardt et al., 2019). Both Uganda and Rwanda are already experiencing the impact of climate change with increasing temperatures and frequencies of extreme weather events (Safari, 2012; McGahey et al., 2013; Tenge et al., 2013; Nsubuga et al., 2014; Nsubuga and Rautenbach, 2018). Mean annual temperature increases of approximately 2.1°C have been recorded over the last 5 decades (McGahey et al., 2013; Nsubuga and Rautenbach, 2018). Future projections indicate that this trend is likely to continue, with increases of 1 to 2.5°C between 2000 and 2050 (Tenge et al., 2013; Nsubuga and Rautenbach, 2018). Furthermore, rainfall has become less seasonal, with both an increase in rainfall over time and increases in the duration of dry spells, trends that are likely to continue in the future (Kizza et al., 2009; McGahey et al., 2013; Diem et al., 2019; Salerno et al., 2019; Ojara et al., 2020).
To investigate if changes in climatic conditions could impact water drinking patterns of mountain gorillas, we examined water drinking behavior between 2010 and 2020 in the two remaining populations of mountain gorillas and correlated this to local maximum temperature and rainfall. We tested the prediction that mountain gorillas drink more often during hotter and drier periods. After observing notable differences in the frequency of water drinking between the two populations, we compared the water content of key foods between the two mountain gorilla populations to see if this could explain differences in their behavior.
Materials and Methods
Subjects and Observation Period
We recorded water drinking behavior in the two mountain gorilla populations in Bwindi Impenetrable National Park (BINP), Uganda and in Volcanoes National Park (VNP), Rwanda region of the Virunga Massif. Five groups were monitored in BINP between Jul 2010 and Oct 2019 (between 0°53′ and 1°08′N and 29°35′ and 29°50′E) at an altitude of 2,100–2,500 m (Supplementary Figure 1). Sixteen groups were monitored in VNP by Dian Fossey Gorilla Fund's Karisoke Research Center between Jan 2012 and Dec 2020 (between 1°21′ and 1°35′S and 29°22′ and 29°44′E) at an altitude of 2,500–4,000 m (unpublished data; Supplementary Figure 1). Water drinking events were recorded on an ad libitum basis and were defined by at least one individual in a group, consuming free-standing water, such as a stream, river, puddle or swamp. This was usually achieved by directly drinking water with the mouth or using cupped hands and dipping the arm in the water and sucking water from the hair. Groups were monitored for an average of 3.45 h per observation day (mean = 4.0 h and 2.9 h for Bwindi and Virunga, respectively) between approximately 09:00 and 13:00.
Climate Variables
In BINP, temperature and rainfall data were recorded at the Ruhija weather station (altitude: 2,301 m) operated by the Institute of Tropical Forest Conservation. The weather station is situated at the park border, close to where the study groups range (approx. <5 km away). In VNP, temperature and rainfall data were recorded at two weather stations outside the park operated by the Rwanda Meteorological Institute (Kinigi and Busogo; altitude: 2,200 and 2,100 m, respectively) approximately 5–10 km from the park boundary. For each gorilla group, we prioritized using the weather data from the closest weather station. At each weather station, temperature and rainfall readings were taken every 5 min. Maximum temperature and summed rainfall were computed per 24 h period. Due to equipment malfunction some gaps are present in the weather data (Supplementary Figures 2, 3).
Weather data were binned into 9-day intervals (see data point—statistical analysis) following recommendations from Seimon and Phillips (2015) that this timeframe provides greater temporal resolution than a month and better captures intra-seasonal variation in climate conditions in this region. Whilst intra-seasonal fluctuations were strongly evident in the 9-day intervals, they were masked by averaging in the monthly means (Seimon and Phillips, 2015). Only 9-day intervals which comprised at least 7 days of weather data (78%) were included in the analysis. In each 9-day interval we extracted the average maximum temperature and summed rainfall.
Statistical Analysis
To test the predictions that mountain gorillas drink more often during drier and hotter periods than wetter and cooler ones, we fitted a generalized linear mixed model (GLMM) with Poisson error structure and log link function, for each population, implemented with the function “glmer” of the “lme4” package (Bates et al., 2015) in R (R Core Team, 2020). The response variable was the summed water drinking events during each 9-day-group period. To control for variation in observation effort, we included the number of hours of observation time (per 9-day-group period) as an offset term (log-transformed). The data points consisted of each 9-day period during which at least one daily observation was made per group. The test predictors included the average maximum temperature and summed rainfall over each 9 day period. As control variables, we included the median Julian date of each data point (time) and a temporal autocorrelation term to account for the potential for residuals of data points close in time being more similar to each other than residuals of data points more distantly spaced, using a function developed in R by Roger Mundry. We included social group ID as a random effect. In addition, we included random slopes for the effects of the test predictors on the response to vary between social group ID as much as possible (random slopes were excluded in the Bwindi model due to high model complexity) (Schielzeth and Forstmeier, 2009; Barr et al., 2013).
We checked for under/overdispersion in the two models. The dispersion parameters were close to the ideal value of 1 (1.28 and 1.15, for the Bwindi and Virunga models, respectively). We verified that collinearity among predictor variables was not an issue, by examining variance inflation factors derived from a model without random effects or random slopes using the “vif” function from the “car” package (Fox and Weisberg, 2011); max vif = 1.1 and 1.0 for the Bwindi and Virunga models, respectively. We checked for model stability by rerunning the models after excluding one social group at a time and comparing the estimates with the original model. No stability issues were found. We z-transformed the predictor variables (to a mean of 0 and standard deviation of 1). We compared the fit of each full model against a corresponding null model with the test predictors excluded, using a likelihood ratio test (Forstmeier and Schielzeth, 2011). Individual p-values were derived by comparing the full model, with a reduced model not comprising each predictor (excluded one at a time) using the “drop1” function. We determined 95% confidence intervals using the function “bootMer” of the “lme4” package (Bates et al., 2015).
Water Content of Foods
To gain an indication of how much water each population obtained from the foods they consumed, we compared the water content of key mountain gorilla foods (Supplementary Table 1). The water content of foods was taken from Ganas et al. (2008), unpublished data, and Grueter et al. (2016). We accounted for differences in dietary importance in the two populations, by weighting each food based on the proportion of energy it contributed to the total amount consumed per population (Wright et al., 2015; Supplementary Table 1). Key foods were defined as those contributing to at least 1% of the total energy consumed per group. To test for a significant difference between the two populations, we used a Welch two-sample t-test.
Results
Water Drinking
Bwindi gorillas were observed drinking during 1.5% of observation hours (230 drinking events during 15,340 observation hours) or 6.0% of group observation days (230 out of 3,803; N = 586 data points–9 day periods). Virunga gorillas were observed drinking during 0.2% of observation hours (131 drinking events during 62,335 observation hours) or 0.6% of group observation days (131 out of 22,010; N = 2,690 data points–9 day periods).
Mountain gorillas in both populations drank water significantly more often at higher average maximum temperatures than cooler ones (Table 1; Figure 1). An increase in average maximum temperature by one standard deviation was associated with a 35 and 46% increase in water drinking events per hour in Bwindi and Virunga, respectively. We found no significant association between water drinking and rainfall in either population (Table 1). The frequency of water drinking in both populations seemed to have increased over time (with a weak trend in Bwindi; Table 1).
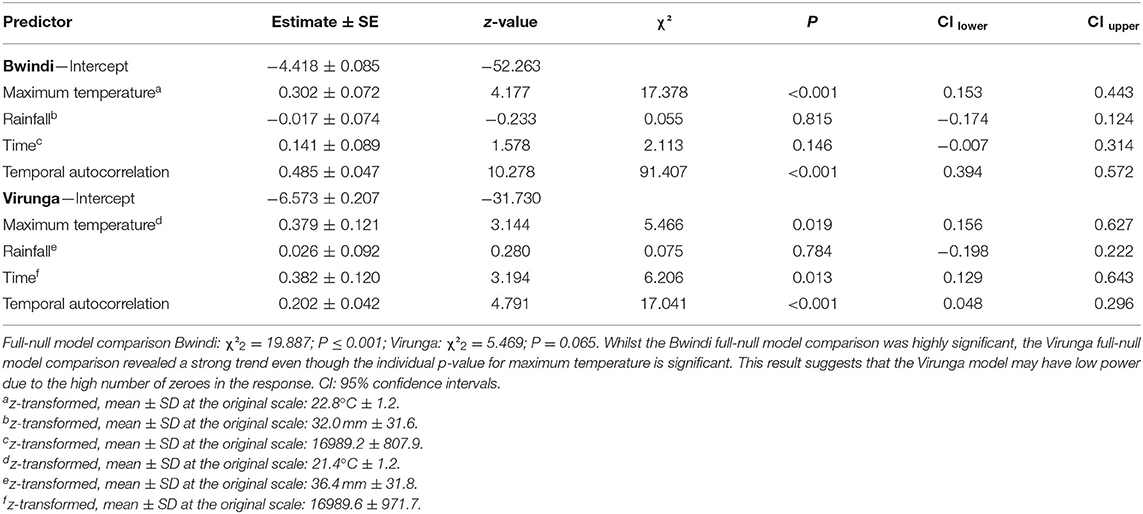
Table 1. Effects of average maximum temperature, rainfall and the control variables time and temporal autocorrelation on the frequency of water drinking in the two mountain gorilla populations.
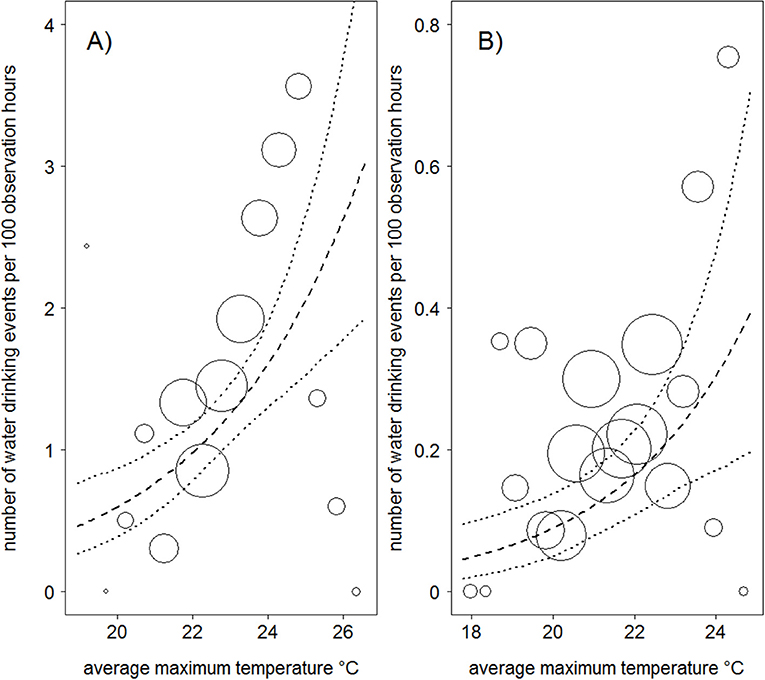
Figure 1. Relationship between average maximum temperature and the frequency of water drinking in Bwindi (A) and Virunga (B) mountain gorillas. The area of the circles represents the sample size (N = 586 − 9 day periods and N = 2,690 − 9 day periods, for the Bwindi and Virunga populations, respectively). The dashed line is the fitted model after controlling for the other variables in the model, and the dotted lines are its 95% confidence intervals.
Water Content of Foods
The water content of key Virunga foods, whilst accounting for differences in dietary importance, was significantly higher than key foods in Bwindi (Welch two-sample t-test t = −8.21, df = 171.43, p ≤ 0.001; Supplementary Table 1). The weighted mean water content of key Bwindi foods was 77.5%, whilst for key Virunga foods it was 87.2 %; Supplementary Table 1).
Discussion
In approximately 10 years of observations on 21 social groups in the only two mountain gorilla populations, we found that mountain gorillas in both populations drank water significantly more often at higher average maximum temperatures than cooler ones. Under these conditions, drinking water may be an effective way of reducing body temperature and reestablishing homeostasis. This result is similar to findings in several other species (Adams and Hayes, 2008; Dias et al., 2014; Harris et al., 2015; Mella et al., 2019; Chaves et al., 2021). However, it highlights a higher degree of reliance on free-standing water than expected in this rainforest dwelling ape species that consumes plants with high water content. Moreover, temperatures in this region are projected to continue increasing in the future by approximately 1 to 2.5°C between 2000 and 2050 (Tenge et al., 2013; Nsubuga and Rautenbach, 2018). Therefore, our results suggest that we should expect mountain gorillas to increasingly rely on free-standing water in the future to maintain water balance following lost water used in evaporative cooling.
We did not find support for the frequency of water drinking to be higher during periods of low rainfall, which may be due to similar temperatures in the dry and wet seasons (Polansky and Robbins, 2013; Seimon and Phillips, 2015; Eckardt et al., 2019). Furthermore, the intake of preformed water is likely to be similar in the wet and dry seasons in the Virungas because water content of important gorilla foods does not vary seasonally (Watts, 1998), but studies are needed to examine whether the water content of gorilla foods varies with altitude in this population and whether it fluctuates seasonally in Bwindi. Additionally, seasonal changes in diet are unlikely to lead to changes in preformed water intake because fruit and bamboo, the only seasonal foods in the Bwindi and Virunga populations, respectively (Vedder, 1984; Ganas et al., 2004; Wright et al., 2015), do not have appreciably lower or higher water content than other food items (Rothman et al., 2006; Grueter et al., 2016).
We found indications in both populations, but with a stronger effect in the Virungas, that the frequency of water drinking has increased over time. This may be due to increases in temperature linked to climate change over this period (McGahey et al., 2013; Nsubuga and Rautenbach, 2018), but due to the relatively short time frame (10 years) such a claim should be viewed with caution and other factors may be responsible.
Lastly, we confirmed previous reports that Virunga mountain gorillas drink water very infrequently (0.6% of observation days) (Schaller, 1963), whereas Bwindi mountain gorillas drink water more frequently (6% of observation days). Virungas gorillas likely obtained more water from food than Bwindi gorillas due to their reliance on plants with higher water content. Together with the higher maximum temperatures in Bwindi, these factors likely explain the population level differences in water drinking rates.
Overall, our results suggest that mountain gorillas may have to work harder to maintain water balance in the future, given that temperature and the duration of dry spells are expected to increase. Increasing water intake can be achieved by either obtaining more water from food or by drinking more water. It is unknown whether mountain gorillas seek out high water content foods, but we think it is unlikely that they are able to increase the amounts of food they eat due to high digestive constraints. A greater reliance on free-standing water has several important implications for mountain gorilla conservation and behavior. First, an increase in the frequency of water drinking may lead to an increase in the risk of parasite exposure compromising the health of the gorillas. Water sources in the mountain gorilla habitat are seasonally used by community members leading to habitat disturbance, increased risk of disease transmission and potentially interspecies competition over water (Bitariho et al., 2003; Akampurira et al., 2017; Gategeko et al., 2017). In addition, water sources inside gorilla protected areas have been shown to harbor parasites, such as Cryptosporidium spp. (Mugoya et al., 2019). Moreover, the risk of disease transmission in general is predicted to increase due to climate change, as pathogens are more likely to develop, survive, and spread under warmer and wetter conditions (Mills et al., 2010; Estrada-Peña et al., 2014).
Second, greater reliance on water sources is likely to have an impact on mountain gorilla ranging patterns. Small streams and swamps are not evenly distributed throughout BINP and VPN and at least some of these water sources seasonally dry up. Moreover, there are indications that some water levels have decreased over time (Akampurira et al., 2017; Hoek et al., 2019). A next step for understanding the impact of temperature on gorillas' water drinking patterns would be to create a detailed map of water availability, to better elucidate whether water is a limiting factor or not. Future studies which monitor water quantity and quality inside gorilla protected areas are also needed in this region. Mountain gorilla space use patterns on a daily, monthly and annual scale are influenced by food availability (Seiler et al., 2018), but it remains unknown if the need for free-standing water may alter such ranging patterns. Water availability influences ranging patterns in several other primates (Campos and Fedigan, 2009; Amoroso et al., 2020) and other forest vertebrates (Paredes et al., 2017).
Third, Virunga mountain gorillas were found to have higher basal stress levels (assessed via fecal glucocorticoid metabolites) at higher average maximum temperatures and periods of high rainfall (Eckardt et al., 2019). This suggests that mountain gorillas may already be experiencing physiological signs of heat stress during periods of high temperature, but further investigation is necessary to elucidate this.
Future studies should examine to what extent mountain gorillas employ behavioral thermoregulation strategies to minimize heat exposure (and maximize heat loss through non-evaporative mechanisms), such as seeking cooler microclimates, restricting physical activity and modifying body posture orientation (Kosheleff and Anderson, 2009; Terrien et al., 2011; Fuller et al., 2016). Anecdotal observations for example indicate that mountain gorillas seek shade and maximize body contact with the ground when it is hot and are more likely to rest than feed during hot days (Eckardt et al., 2019; personal observation). However, behavioral thermoregulation strategies may be associated with fitness costs (Cunningham et al., 2021). Additional research into how much water intake from plants consumed changes as temperature changes would be a useful way to determine if the gorillas are able to achieve homeostasis through changes in their diet vs. increasing their consumption of free standing water.
Undoubtedly, the direct impact of climate change is likely to be a bigger problem for species living in more arid environments that face reduced access to sufficient quantities of either free-standing sources of water or preformed water (Fuller et al., 2016; Cayton and Haddad, 2018; Mitchell et al., 2018; Wessling et al., 2018; Riddell et al., 2019; Young et al., 2019; Campos et al., 2020). Many avenues of future research into this topic remain to better determine how much of a risk increased temperature poses for the mountain gorillas. However, this study emphasizes that climate change may have negative consequences even for rainforest dwelling mammals that routinely obtain nearly all of their water from dietary items. This may be especially true for endangered species in small isolated populations which are vulnerable to drought and the risk of extinction.
Data Availability Statement
The raw data supporting the conclusions of this article will be made available by the authors, without undue reservation.
Ethics Statement
Ethical review and approval was not required for the animal study because this observational study was conducted in accordance with guidelines of the Uganda Wildlife Authority, Rwanda Development Board, Dian Fossey Gorilla Fund and Max Planck Institute for Evolutionary Anthropology and adhered to all laws of Uganda and Rwanda.
Author Contributions
EW designed the study, collected and analyzed the data, and wrote the manuscript. WE designed the study, collected the data, and contributed to the writing of the manuscript. JR designed the study and edited the manuscript. RB contributed data and edited the manuscript. CG and JG-S contributed data and edited the manuscript. TS designed the study, contributed the data, and edited the manuscript. MR designed the study, collected the data, and helped to write the manuscript. All authors contributed to the article and approved the submitted version.
Funding
This project was funded by the Grand Duchy of Luxembourg under the United Nations Environment Programme Vanishing Treasures Programme, Max Planck Society and Dian Fossey Gorilla Fund.
Conflict of Interest
The authors declare that the research was conducted in the absence of any commercial or financial relationships that could be construed as a potential conflict of interest.
Publisher's Note
All claims expressed in this article are solely those of the authors and do not necessarily represent those of their affiliated organizations, or those of the publisher, the editors and the reviewers. Any product that may be evaluated in this article, or claim that may be made by its manufacturer, is not guaranteed or endorsed by the publisher.
Acknowledgments
We thank the Uganda Wildlife Authority, the Uganda National Council for Science and Technology and Rwanda Development Board for long-term support and permission to conduct research in BINP and VPN. We are indebted to all staff who have worked with the gorillas in BINP and VPN, including from the Max Planck Institute for Evolutionary Anthropology, the Institute of Tropical Forest Conservation and the Dian Fossey Gorilla Fund. Special thanks go to the Institute of Tropical Forest Conservation, the Tropical Ecology Assessment and Monitoring Network and the Rwanda Meteorological Institute for the climate data.
Supplementary Material
The Supplementary Material for this article can be found online at: https://www.frontiersin.org/articles/10.3389/fcosc.2022.738820/full#supplementary-material
References
Adams, R. A., and Hayes, M. A. (2008). Water availability and successful lactation by bats as related to climate change in arid regions of western North America. J. Anim. Ecol. 77, 1115–1121. doi: 10.1111/j.1365-2656.2008.01447.x
Akampurira, E., Bitariho, R., Babaasa, D., and Twinomuhangi, I. (2017). An Assessment of the Hydrological Systems in the Lake Edward Basin of Bwindi Impenetrable and Queen Elizabeth National Parks, S.W Uganda: Water Quality and Quantity Assessments. Institute of Tropical Forest Conservation.
Amoroso, C. R., Kappeler, P. M., Fichtel, C., and Nunn, C. L. (2020). Water availability impacts habitat use by Red-Fronted Lemurs (Eulemur rufifrons): an experimental and observational study. Int. J. Primatol. 41, 61–80. doi: 10.1007/s10764-020-00136-9
Barr, D. J., Levy, R., Scheepers, C., and Tily, H. J. (2013). Random effects structure for confirmatory hypothesis testing: keep it maximal. J. Mem. Lang. 68, 255–278. doi: 10.1016/j.jml.2012.11.001
Bates, D., Maechler, M., Bolker, B., and Walker, S. (2015). Fitting linear mixed-effects models using lme4. J. Stat. Softw. 67, 1–48. doi: 10.18637/jss.v067.i01
Bernard, A. B., and Marshall, A. J. (2020). Assessing the state of knowledge of contemporary climate change and primates. Evol. Anthropol Issues News Rev. 29, 317–331. doi: 10.1002/evan.21874
Bitariho, R., Babaasa, D., and Kasangaki, A. (2003). Impact of Water Harvesting on Kabiranyuma Swamp, Mgahinga Gorilla National Park, Southwest Uganda. Institute of Tropical Forest Conservation.
Campos, F. A., and Fedigan, L. M. (2009). Behavioral adaptations to heat stress and water scarcity in white-faced capuchins (Cebus capucinus) in Santa Rosa National Park, Costa Rica. Am. J. Phys. Anthropol. 138, 101–111. doi: 10.1002/ajpa.20908
Campos, F. A., Kalbitzer, U., Melin, A. D., Hogan, J. D., Cheves, S. E., Murillo-Chacon, E., et al. (2020). Differential impact of severe drought on infant mortality in two sympatric neotropical primates. R. Soc. open sci. 7, 200302. doi: 10.1098/rsos.200302
Carvalho, J. S., Graham, B., Rebelo, H., Bocksberger, G., Meyer, C. F. J., Wich, S., et al. (2019). A global risk assessment of primates under climate and land use/cover scenarios. Glob. Change Biol. 25, 3163–3178. doi: 10.1111/gcb.14671
Cayton, H., and Haddad, N. (2018). Water availability coincides with population declines for an endangered butterfly. Diversity 10, 94. doi: 10.3390/d10030094
Chapman, C. A., Lawes, M. J., and Eeley, H. A. C. (2006). What hope for African primate diversity? Afr. J. Ecol. 44, 116–133. doi: 10.1111/j.1365-2028.2006.00636.x
Chaves, Ó. M., Fortes, V. B., Hass, G. P., Azevedo, R. B., Stoner, K. E., and Bicca-Marques, J. C. (2021). Flower consumption, ambient temperature and rainfall modulate drinking behavior in a folivorous-frugivorous arboreal mammal. PLoS ONE 16, e0236974. doi: 10.1371/journal.pone.0236974
Ciani, A. C., Martinoli, L., Capiluppi, C., Arahou, M., and Mouna, M. (2001). Effects of water availability and habitat quality on bark-stripping behavior in barbary macaques. Conserv. Biol. 15, 259–265. doi: 10.1111/j.1523-1739.2001.99019.x
Cunningham, S. J., Gardner, J. L., and Martin, R. O. (2021). Opportunity costs and the response of birds and mammals to climate warming. Front. Ecol. Environ. 19, 300–307. doi: 10.1002/fee.2324
Dias, P. A. D., Rangel-Negrín, A., Coyohua-Fuentes, A., and Canales-Espinosa, D. (2014). Factors affecting the drinking behavior of black howler monkeys (Alouatta pigra). Primates 55, 1–5. doi: 10.1007/s10329-013-0383-1
Diem, J. E., Konecky, B. L., Salerno, J., and Hartter, J. (2019). Is equatorial Africa getting wetter or drier? Insights from an evaluation of long-term, satellite-based rainfall estimates for western Uganda. Int. J. Climatol. 39, 3334–3347. doi: 10.1002/joc.6023
Eckardt, W., Stoinski, T. S., Rosenbaum, S., and Santymire, R. (2019). Social and ecological factors alter stress physiology of Virunga mountain gorillas (Gorilla beringei beringei). Ecol. Evol. 9, 5248–5259. doi: 10.1002/ece3.5115
Estrada-Peña, A., Ostfeld, R. S., Peterson, A. T., Poulin, R., and de la Fuente, J. (2014). Effects of environmental change on zoonotic disease risk: an ecological primer. Trends Parasitol. 30, 205–214. doi: 10.1016/j.pt.2014.02.003
Forstmeier, W., and Schielzeth, H. (2011). Cryptic multiple hypotheses testing in linear models: overestimated effect sizes and the winner's curse. Behav. Ecol. Sociobiol. 65, 47–55. doi: 10.1007/s00265-010-1038-5
Fuller, A., Mitchell, D., Maloney, S. K., and Hetem, R. S. (2016). Towards a mechanistic understanding of the responses of large terrestrial mammals to heat and aridity associated with climate change. Clim. Change Responses 3, 10. doi: 10.1186/s40665-016-0024-1
Ganas, J., Ortmann, S., and Robbins, M. M. (2008). Food preferences of wild mountain gorillas. Am. J. Primatol. 70, 927–938. doi: 10.1002/ajp.20584
Ganas, J., Robbins, M. M., Nkurunungi, J., Kaplin, B. A., and McNeilage, A. (2004). Dietary variability of mountain gorillas in Bwindi impenetrable National Park, Uganda. Int. J. Primatol. 25, 1043–1072. doi: 10.1023/B:IJOP.0000043351.20129.44
Gategeko, A., Munyarugendo, I., and Eckardt, W. (2017). “Conservation impacts in the Volcanoes National Park due to the water shortage in the Mudakama area,” in Poster Session Presented at the Conversation on Conservation (Kigali).
Graham, T. L., Matthews, H. D., and Turner, S. E. (2016). A global-scale evaluation of primate exposure and vulnerability to climate change. Int. J. Primatol. 37, 158–174. doi: 10.1007/s10764-016-9890-4
Granjon, A.-C., Robbins, M. M., Arinaitwe, J., Cranfield, M. R., Eckardt, W., Mburanumwe, I., et al. (2020). Estimating abundance and growth rates in a wild mountain gorilla population. Anim. Conserv. 23, 455–465. doi: 10.1111/acv.12559
Grueter, C. C., Robbins, A. M., Abavandimwe, D., Vecellio, V., Ndagijimana, F., Ortmann, S., et al. (2016). Causes, mechanisms, and consequences of contest competition among female mountain gorillas in Rwanda. Behav. Ecol. 27, 766–776. doi: 10.1093/beheco/arv212
Harris, G., Sanderson, J. G., Erz, J., Lehnen, S. E., and Butler, M. J. (2015). Weather and prey predict mammals' visitation to water. PLoS ONE 10, e0141355. doi: 10.1371/journal.pone.0141355
Hetem, R. S., Fuller, A., Maloney, S. K., and Mitchell, D. (2014). Responses of large mammals to climate change. Temperature 1, 115–127. doi: 10.4161/temp.29651
Hoek, Y., van der Tuyisingize, D., Eckardt, W., Garriga, N., and Derhé, M. A. (2019). Spatial variation in anuran richness, diversity, and abundance across montane wetland habitat in Volcanoes National Park, Rwanda. Ecol. Evol. 9, 4220–4230. doi: 10.1002/ece3.5054
Jéquier, E., and Constant, F. (2010). Water as an essential nutrient: the physiological basis of hydration. Eur. J. Clin. Nutr. 64, 115–123. doi: 10.1038/ejcn.2009.111
Karasov, W. H., and del Rio, C. M. (2020). “Water requirements and water flux,” in Physiological Ecology: How Animals Process Energy, Nutrients, and Toxins (Princeton University Press), 608–644. doi: 10.2307/j.ctvzsmfh4
Kizza, M., Rodhe, A., Xu, C.-Y., Ntale, H. K., and Halldin, S. (2009). Temporal rainfall variability in the Lake Victoria Basin in East Africa during the twentieth century. Theor. Appl. Climatol. 98, 119–135. doi: 10.1007/s00704-008-0093-6
Kosheleff, V. P., and Anderson, C. N. K. (2009). Temperature's influence on the activity budget, terrestriality, and sun exposure of chimpanzees in the Budongo Forest, Uganda. Am. J. Phys. Anthropol. 139, 172–181. doi: 10.1002/ajpa.20970
McGahey, D. J., Williams, D. G., Muruth, P., and Loubser, D. I. (2013). Investigating climate change vulnerability and planning for adaptation: learning from a study of climate change impacts on the Mountain Gorilla in the Albertine Rift. Nat. Sci. 05, 10–17. doi: 10.4236/ns.2013.55A002
Mella, V. S. A., McArthur, C., Krockenberger, M. B., Frend, R., and Crowther, M. S. (2019). Needing a drink: rainfall and temperature drive the use of free water by a threatened arboreal folivore. PLoS ONE 14, e0216964. doi: 10.1371/journal.pone.0216964
Mills, J. N., Gage, K. L., and Khan, A. S. (2010). Potential influence of climate change on vector-borne and zoonotic diseases: a review and proposed research plan. Environ. Health Perspect. 118, 1507–1514. doi: 10.1289/ehp.0901389
Mitchell, D., Fuller, A., and Maloney, S. K. (2009). Homeothermy and primate bipedalism: is water shortage or solar radiation the main threat to baboon (Papio hamadryas) homeothermy? J. Hum. Evol. 56, 439–446. doi: 10.1016/j.jhevol.2009.03.003
Mitchell, D., Snelling, E. P., Hetem, R. S., Maloney, S. K., Strauss, W. M., and Fuller, A. (2018). Revisiting concepts of thermal physiology: predicting responses of mammals to climate change. J. Anim. Ecol. 87, 956–973. doi: 10.1111/1365-2656.12818
Mugoya, G. J., Sente, C., Cumber, S. N., Taseera, K., Nkfusai, C. N., and Atuhaire, C. (2019). Cryptosporidium and giardia species in newly and previously habituated gorillas and nearby water sources in Bwi/ndi Impenetrable National Park, Uganda. Pan Afr. Med. J. 34, 112. doi: 10.11604/pamj.2019.34.112.19288
National Research Council (2003). “Water,” in Nutrient Requirements of Nonhuman Primates: Second Revised Edition (Washington, DC: The National Academies Press), 150–158. Available online at: https://www.nap.edu/catalog/9826/nutrient-requirements-of-nonhuman-primates-second-revised-edition
Nsubuga, F. W., Olwoch, J. M., and Rautenbach, H. (2014). Variability properties of daily and monthly observed near-surface temperatures in Uganda: 1960-2008: variability of daily and monthly temperatures in Uganda. Int. J. Climatol. 34, 303–314. doi: 10.1002/joc.3686
Nsubuga, F. W., and Rautenbach, H. (2018). Climate change and variability: a review of what is known and ought to be known for Uganda. IJCCSM 10, 752–771. doi: 10.1108/IJCCSM-04-2017-0090
Ojara, M. A., Lou, Y., Aribo, L., Namumbya, S., and Uddin, M. d. J. (2020). Dry spells and probability of rainfall occurrence for Lake Kyoga Basin in Uganda, East Africa. Nat. Hazards 100, 493–514. doi: 10.1007/s11069-019-03822-x
Paredes, O. S. L., Norris, D., Oliveira, T. G., and de Michalski, F. (2017). Water availability not fruitfall modulates the dry season distribution of frugivorous terrestrial vertebrates in a lowland Amazon forest. PLoS ONE 12, e0174049. doi: 10.1371/journal.pone.0174049
Polansky, L., and Robbins, M. M. (2013). Generalized additive mixed models for disentangling long-term trends, local anomalies, and seasonality in fruit tree phenology. Ecol. Evol. 3, 3141–3151. doi: 10.1002/ece.3.707
R Core Team (2020). A Laguage and Environment for Statistical Computing. Vienna,: R Foundation for Statistical Computing.
Riddell, E. A., Iknayan, K. J., Wolf, B. O., Sinervo, B., and Beissinger, S. R. (2019). Cooling requirements fueled the collapse of a desert bird community from climate change. Proc. Natl. Acad. Sci. U.S.A. 116, 21609–21615. doi: 10.1073/pnas.1908791116
Robbins, C. T. (1983). “Water,” in Wildlife Feeding and Nutrition, ed C. T. Robbins (New York, NY: Academic Press), 17–29. doi: 10.1016/B978-0-12-589382-4.50011-2
Robbins, M. M. (2011). “Gorillas: diversity in ecology and behavior,” in Primates in Perspective, eds C. J. Campbell, A. Fuentes, K. MacKinnon, S. Bearder, and R. Stumpf (New York, NY: Oxford University Press), 326–339.
Rothman, J. M., Dierenfeld, E. S., Molina, D. O., Shaw, A. V., Hintz, H. F., and Pell, A. N. (2006). Nutritional chemistry of foods eaten by gorillas in Bwindi Impenetrable National Park, Uganda. Am. J. Primatol. 68, 675–691. doi: 10.1002/ajp.20243
Safari, B. (2012). Trend analysis of the mean annual temperature in Rwanda during the last fifty two years. J. Environ. Protect. 2012, 36065. doi: 10.4236/jep.2012.36065
Salerno, J., Diem, J. E., Konecky, B. L., and Hartter, J. (2019). Recent intensification of the seasonal rainfall cycle in equatorial Africa revealed by farmer perceptions, satellite-based estimates, and ground-based station measurements. Clim. Change 153, 123–139. doi: 10.1007/s10584-019-02370-4
Sato, H., Ichino, S., and Hanya, G. (2014). Dietary modification by common brown lemurs (Eulemur fulvus) during seasonal drought conditions in western Madagascar. Primates 55, 219–230. doi: 10.1007/s10329-013-0392-0
Schaller, G. B. (1963). The Mountain Gorilla - Ecology and Behavior. Chicago, IL: University of Chicago Press.
Schielzeth, H., and Forstmeier, W. (2009). Conclusions beyond support: overconfident estimates in mixed models. Behav. Ecol. 20, 416–420. doi: 10.1093/beheco/arn145
Seiler, N., Boesch, C., Stephens, C., Ortmann, S., Mundry, R., and Robbins, M. M. (2018). Social and ecological correlates of space use patterns in Bwindi mountain gorillas. Am. J. Primatol. 80, e22754. doi: 10.1002/ajp.22754
Seimon, A., and Phillips, G. P. (2015). “Projected climatological and ecological changes in the 21st century across the mountain gorilla's range,” in The Implications of Global Climate Change for Mountain Gorilla Conservation in the Albertine Rift, 10–21.
Tenge, N. G., Alphonse, M., and Thomas, T. S. (2013). “Chapter 9: Rwanda,” in East African Agriculture and Climate Change: A Comprehensive Analysis, eds M. Waithaka, G. C. Nelson, T. S. Thomas, and M. Kyotalimye (Washington, DC: International Food Policy Research Institute), 247–277.
Terrien, J., Perret, M., and Aujard, F. (2011). Behavioral thermoregulation in mammals: a review. Front. Biosci. 16, 1428–1444. doi: 10.2741/3797
Vedder, A. L. (1984). Movement patterns of a group of free-ranging mountain gorillas (Gorilla gorilla beringei) and their relation to food availability. Am. J. Primatol. 7, 73–88. doi: 10.1002/ajp.1350070202
Wang, Q., Fan, X., and Wang, M. (2016). Evidence of high-elevation amplification versus Arctic amplification. Sci. Rep. 6, 1–8. doi: 10.1038/srep19219
Watts, D. P. (1998). Seasonality in the ecology and life histories of mountain gorillas (Gorilla gorilla beringei). Int. J. Primatol. 19, 929–948. doi: 10.1023/A:1020366018187
Wessling, E. G., Kühl, H. S., Mundry, R., Deschner, T., and Pruetz, J. D. (2018). The costs of living at the edge: Seasonal stress in wild savanna-dwelling chimpanzees. J. Hum. Evol. 121, 1–11. doi: 10.1016/j.jhevol.2018.03.001
Withers, P. C., Cooper, C. E., Maloney, S. K., Bozinovic, F., and Neto, A. P. C. (2016). Ecological and Environmental Physiology of Mammals. Oxford: OUP.
Wright, E., Grueter, C. C., Seiler, N., Abavandimwe, D., Stoinski, T. S., Ortmann, S., et al. (2015). Energetic responses to variation in food availability in the two mountain gorilla populations (Gorilla beringei beringei). Am. J. Phys. Anthropol. 158, 487–500. doi: 10.1002/ajpa.22808
Young, C., Bonnell, T. R., Brown, L. R., Dostie, M. J., Ganswindt, A., Kienzle, S., et al. (2019). Climate induced stress and mortality in vervet monkeys. R. Soc. Open Sci. 6, 191078. doi: 10.1098/rsos.191078
Keywords: mountain gorillas, climate change, temperature, heat stress, water drinking
Citation: Wright E, Eckardt W, Refisch J, Bitariho R, Grueter CC, Ganas-Swaray J, Stoinski TS and Robbins MM (2022) Higher Maximum Temperature Increases the Frequency of Water Drinking in Mountain Gorillas (Gorilla beringei beringei). Front. Conserv. Sci. 3:738820. doi: 10.3389/fcosc.2022.738820
Received: 03 August 2021; Accepted: 15 February 2022;
Published: 10 March 2022.
Edited by:
Mauricio Talebi, Federal University of São Paulo, BrazilReviewed by:
Qiang Dai, Chengdu Institute of Biology (CAS), ChinaWei Wei, China West Normal University, China
Copyright © 2022 Wright, Eckardt, Refisch, Bitariho, Grueter, Ganas-Swaray, Stoinski and Robbins. This is an open-access article distributed under the terms of the Creative Commons Attribution License (CC BY). The use, distribution or reproduction in other forums is permitted, provided the original author(s) and the copyright owner(s) are credited and that the original publication in this journal is cited, in accordance with accepted academic practice. No use, distribution or reproduction is permitted which does not comply with these terms.
*Correspondence: Edward Wright, ZWR3YXJkX3dyaWdodEBldmEubXBnLmRl