- 1Environmental Science and Engineering, University of Texas, El Paso, TX, United States
- 2National Oceanic and Atmospheric Administration, Southwest Fisheries Science Center, La Jolla, CA, United States
- 3Asociación ProCosta, San Salvador, El Salvador
- 4Fauna and Flora International, Managua, Nicaragua
- 5Ocean and Earth Science, University of Southampton, Southampton, United Kingdom
- 6Biology Department, University of New Mexico, Albuquerque, NM, United States
Successful conservation of endangered, migratory species requires an understanding of habitat use throughout life stages. When dedicated scientific studies are difficult to conduct, local expert knowledge can provide crucial baseline data to guide study design and aid data interpretation. In 2008, fishers in El Salvador demonstrated that eastern Pacific hawksbill sea turtles (Eretmochelys imbricata)—a population conservation biologists considered virtually extirpated—use mangrove estuaries as nesting habitat rather than open-coast beaches used by hawksbills in other regions. We confirmed and amplified this observation by using fishers' ecological knowledge to guide biological sampling for stable isotope analysis to assess if eastern Pacific hawskbills use mangrove-dominated estuaries as developmental habitats. We found that immature hawksbills experience a pelagic stage and then recruit to estuaries at ~37 cm curved carapace length, where they increase reliance on estuarine resources until they approach adult sizes. This life history strategy makes them especially vulnerable to in-water nearshore threats, and necessitates targeted expansion of conservation efforts throughout the eastern Pacific. Our analysis also provides a model for integrating traditional scientific approaches with local knowledge—a model that could yield crucial advances in other understudied regions.
Introduction
Endangered species conservation efforts often focus on identifying key habitats or regions where protection may achieve tangible and enduring benefits. It can take decades, however, to obtain detailed scientific information on a population's habitat and resource use, especially for rare and critically endangered species most needing protection. For this reason, local ecological knowledge—learned from experience or cultural transmission —can be especially valuable in conservation science and management (Johannes et al., 2000; Drew, 2005; Haggan et al., 2007; Ostrom, 2009; Virapongse et al., 2016; Wedemeyer-Strombel et al., 2019; Kiszka et al., 2021).
Here, we use a combination of fishers' ecological knowledge (FEK) and stable isotope analysis to study the habitat use of eastern Pacific hawksbill sea turtles (Eretmochelys imbricata). Sea turtles–including hawksbill turtles elsewhere in the world–typically nest on sandy beaches along open coastlines. Hawksbill turtles are pantropical and critically endangered throughout their range (IUCN, 2018). Based on a lack of observations of nesting on beaches, the eastern Pacific hawksbill population that ranges from Mexico to Peru was thought to be on the verge of extirpation as recently as 2008 (Gaos and Yañez, 2012). However, consideration of FEK led to the scientific “re-discovery” of this population in 2008 and the recognition that the population nests within mangrove estuary habitats (Vásquez and Liles, 2008; Gaos et al., 2010; Guzmán Hernández et al., 2010), a behavior previously unheard of in sea turtles.
Since this discovery, multiple studies have suggested that these mangrove estuaries are important habitats for hawksbill adults for both nesting and foraging (Gaos et al., 2010, 2018; Liles et al., 2015b). More than 70% of the ~700 nesting females in the eastern Pacific population nest on shores within two mangrove estuaries: Bahía de Jiquilisco, El Salvador and Estero Padre Ramos, Nicaragua (Gaos et al., 2017). Although it is likely that mangrove estuaries also provide important habitat for other stages in these hawksbills' life cycle, the importance of these habitats throughout the life cycle is poorly understood (Liles et al., 2017; Gaos et al., 2018; Wildermann et al., 2018).
In this study, we employ a social–ecological systems approach to address this question. We captured FEK through interviews to initially identify the likelihood of extensive hawksbill dependence on estuaries and secondarily to guide sampling of tissues for stable isotope analysis. By combining these sources of information, we were able to quantify the use of estuarine resources in hawksbills as a function of body size (proxy for age), revealing an estuarine developmental phase for this critically endangered population. Additionally, our findings emphasize the accuracy of FEK, and the importance of collaborating with local experts to improve conservation output and efficiency, especially in areas where humans and endangered species share critical resources. Although the theoretical framing and methodological structure used to obtain FEK data were published in Wedemeyer-Strombel et al. (2019), the data themselves are analyzed for the first time in the current study. When combined, FEK and stable isotope data created a robust understanding of hawksbill habitat use throughout these mangrove estuaries.
Stable isotope analysis has become a commonly used tool in studies to identify habitat use by sea turtles (Hobson, 2008; Jones and Seminoff, 2013; Haywood et al., 2019), and other cryptic marine species (Kiszka et al., 2021; Smith et al., 2021). The basic premise of this approach is that the isotopic composition of animal tissues mirrors that of their food and water resources (Hobson, 2008), which can vary among habitats. For example, mangroves have distinctly lower carbon isotope (δ13C) values than phytoplankton because of the CO2 limitation in aquatic environments (Hunt, 1970; Herzka, 2005). In addition, bacterial methanogenesis in anoxic sediments in mangrove habitats strongly fractionates carbon resulting in low methane δ13C, and by extension uptake of methane-derived dissolved inorganic carbon by mangrove roots may also contribute to relatively low δ13C values observed in mangrove tissues (Hunt, 1970; Machiwa, 2000). Foraging in ecosystems where mangroves contribute significantly to primary production is therefore likely to result in a significant decrease in consumer tissue δ13C values compared to consumers foraging in open marine phytoplankton-fueled habitats. We exploit these differences to infer to what extent eastern Pacific hawksbills of each life stage use estuarine mangrove habitats compared to oceanic habitats.
Methods
Study Sites
This study was conducted at the two primary nesting localities for hawksbills in the eastern Pacific (Gaos et al., 2010): Bahía de Jiquilisco, El Salvador (13°13′N, 88°32′W) and Estero Padre Ramos, Nicaragua (12°48′N, 87°28′W; Figure 1). Bahía de Jiquilisco (635 km2) is a UNESCO Biosphere Reserve and the largest mangrove estuary in El Salvador (MARN, 2014). Estero Padre Ramos is much smaller (88 km2) and was declared a Nature Reserve in 1983 (MARENA, 2003). Both sites are dominated by red mangrove (Rhizophora mangle) that support artisanal fishing communities and are threatened by the conversion of mangrove forests to shrimp farms.
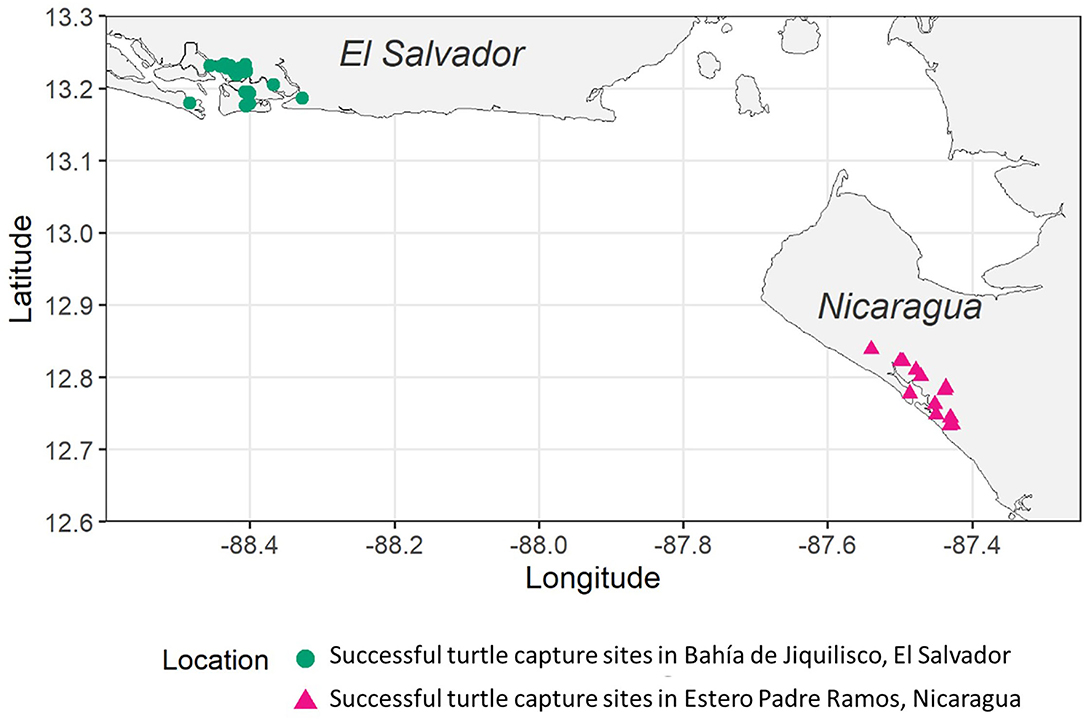
Figure 1. Map of the study site locations: green circles represent successful turtle capture locations in Bahia de Jiquilisco, El Salvador, and pink triangles represent successful turtle capture locations in Estero Padre Ramos, Nicaragua.
Informant Directed Semi-structured Interviews
We conducted interviews in May and June 2016 in fishing communities surrounding Bahía de Jiquilisco and Estero Padre Ramos. These communities were chosen because they (1) have been crucial in the scientific rediscovery of this population (Vásquez and Liles, 2008; Gaos and Yañez, 2012), (2) have implemented sea turtle and habitat conservation initiatives for at least a decade (Gaos et al., 2010; Liles et al., 2015a), and (3) have strong cultural connections to hawksbills (Liles et al., 2015a). The fishers work primarily within the estuaries, and can provide important insights about within-estuary habitat use by hawksbills based on personal experience. We asked 69 people for an interview, and 68 total interviews were conducted (38 in Bahía de Jiquilisco, 30 in Estero Padre Ramos). All informants gave verbal consent prior to being interviewed, 62 informants gave additional verbal consent to be recorded (34 in Bahía de Jiquilisco, 28 in Estero Padre Ramos). The University of Texas at El Paso Institutional Review Board approved all interview practices (IRBNET ID 896427-1). For details on interview methodology, please see Wedemeyer-Strombel et al. (2019) and the Supplementary Materials.
Partially-Emergent Thematic-Analysis
To elucidate the themes throughout the FEK, the 62 recorded interviews were translated, transcribed, and analyzed using a partially-emergent-thematic analysis (Kincheloe and McLaren, 2005; Wedemeyer-Strombel, 2019). The appropriate conceptual framework for analyzing interview transcripts emerged from a synthesis of state-of-the art research on the involvement of lay persons in conservation, rather than being determined a priori. This emergent approach has been successfully used by social scientists, especially when questions of sociological context were central to an inquiry (Kincheloe and McLaren, 2005). During initial interview processing, interviews were translated into single units of meaning labeled as “utterances.”
Once the data were organized into utterances, a codebook was developed to define four main knowledge categories and used to train coders and assess intercoder reliability (Krippendorff, 2013). Coding was done using NVivo 10.0 qualitative software (QSR International, Doncaster, Victoria, Australia). Depending on fit, individual utterances were coded into zero, one, two, three, and/or four categories (Supplementary Table 1). To ensure coder objectivity, coders independently coded ~20% of utterances, and calculated intercoder reliability across all categories with weighted Cohen's kappa (0.98; Cohen, 1968). This coding approach yields distinct themes (Table 1) that can provide a more in-depth understanding and support ranking of the FEK, analogous to a principle-component analysis in quantitative studies. The knowledge themes that emerged through this process (Peterson et al., 1994), were then organized into discrete categories (Wedemeyer-Strombel, 2019) per Corbin and Strauss (1990) and Kincheloe and McLaren (2005).
Turtle Capture
We used maps of hawksbill habitat use created exclusively from FEK (Figures 1, 3 in Wedemeyer-Strombel et al., 2019) to target locations for in-water capture of turtles for tissue collection. We identified recommended locality points and created a 500-m-radius buffer around them (Bolstad, 2012), as known hawksbill displacement at these sites is generally <500 m (Torres Gago et al., 2016). We conducted sampling surveys both within and outside of the buffer areas to assess the effectiveness of FEK in identifying critical foraging areas, and to reduce survey sampling bias.
We identified turtles as immature when their curved carapace length was between 30 and 65 cm following Liles et al. (2015b) and Wildermann et al. (2018). Hawksbills were captured during in-water surveys in Bahía de Jiquilisco (n = 99) and Estero Padre Ramos (n = 74) from April 2016 to January 2017, and December 2015 to December 2016, respectively (Figure 1). We also sampled an additional 12 immature hawksbills that were bycaught in nearshore fisheries within Bahía de Jiquilisco. For all turtles, we collected skin and carapace tissue samples for stable isotope analysis after measurements and tagging were completed. Skin of nesting females (n = 207) were sampled between 2008 and 2014 during monitoring patrols at both sites (Gaos et al., 2016).
Skin and Carapace Tissue Collection
Two ~6-mm-diameter skin samples were collected from the shoulder region of each turtle (Gaos et al., 2016). We removed underlying tissue from samples so that only the epidermis layer was retained, which was stored in a saturated NaCl solution until analysis.
Two 6-mm-diameter scute samples were collected from each of the second vertebral scutes of the carapace (Reich et al., 2007), which were stored in Whirl Pak™ bags with dry NaCl until analysis. We collected scute samples using a novel drilling tool derived from FEK (Wedemeyer-Strombel, 2019; Wedemeyer-Strombel et al., 2019). Turtle scutes grow incrementally, allowing assessment of change in isotopic composition over time within an individual turtle (Palaniappan, 2007; Reich et al., 2007; Jones and Seminoff, 2013). On the second lateral scute, oldest scutes were sampled from a posterior site and youngest from an anterior site (Reich et al., 2007). Specifically, we used this approach to assess developmental shifts from pelagic to mangrove habitats, which we hypothesized to be isotopically distinct between oldest and newest scute layers (Supplementary Figure 1).
Prey Collection
Putative prey items based on FEK were collected from within each estuary, including barnacles (Balanus sp.), red algae (Rhodophyta), sponges (Axinella sp., Aplysina sp., and Geodia sp.), as well as red mangrove roots, leaves, and seeds. These samples were dried and stored in plastic bags or preserved in 70% EtOH if too large for drying in the field.
We did not obtain isotope data of putative hawksbill prey sources from nearshore pelagic habitats in the eastern Pacific due to fieldwork and budget constraints. Rather, we rely on research summarized by Herzka (2005) and Bouillon et al. (2011), which indicates that pelagic- and mangrove-dominated estuarine ecosystems in the eastern Pacific have distinct isotope values. The mangrove-based putative prey that we sampled provided a reference for estuary habitat/diet, and previously published values of pelagically captured olive ridley turtles (Lepidochelys olivacea; Peavey et al., 2017) and particulate organic matter (POM; Trueman and St John Glew, 2019) were used as references for offshore-non-estuary habitat and diet.
Stable Isotope Analysis
We collected both skin and scute samples, which were stored in NaCl in the field for transport to the laboratory. In addition to skin, we collected the top (oldest tissue) and bottom (newest tissue) layers from the right marginal scute from a subset of turtles (Bahía de Jiquilisco, n = 10; Estero Padre Ramos, n = 7; Supplementary Figure 1). Successive sea turtle scute layers provide chronological ecological data (Reich et al., 2007; Vander Zanden et al., 2010). We cannot assume an exact timeframe represented by hawksbill scutes, therefore, we focused our analysis on the oldest and newest scute layers, which show the turtles' chronological foraging history, even though exact time (in years) is unknown.
Skin and scute samples were rinsed repeatedly over 2 days with deionized (DI) water to remove any residual NaCl from storage. Skin and scute samples were then lipid-extracted via three 24-h soaks in a 2:1 chloroform:methanol solvent solution, followed by thorough rinsing in DI water and dried via lyophilization for skin or air-dried under the hood for scute. Finally, we weighed ~0.5–0.6 mg of dried tissue (skin and scute) into tin capsules for δ13C and δ15N analysis.
Barnacles and sponges were rinsed but were not lipid extracted as weight percent [C]/[N] ratios confirmed that the organic (digestible) fraction of these potential prey is primarily composed of protein. Mean (±SD) [C]/[N] ratios of barnacles (3.7 ± 0.4) are within the theoretical range of pure protein (Table 2), while sponges have higher mean (±SD) [C]/[N] ratios owing to their high silica content and thus required larger samples to obtain δ13C and δ15N values (see below). Barnacles were demineralized in 0.5N HCl to remove inorganic carbon (carbonate). Samples were then rinsed in DI water, lyophilized, and weighed into tin capsules for δ13C and δ15N analysis. Barnacles, sponges, and mangroves were weighed to ~0.4–0.6 mg, 1.0–3.5 mg, and ~4–8 mg, respectively.
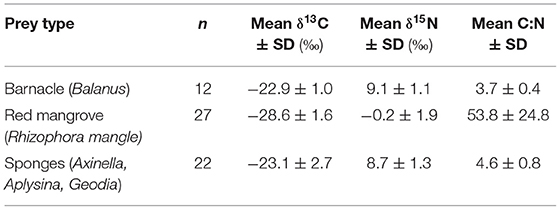
Table 2. Mean (±SD) δ13C, δ15N (‰), and C:N values of putative prey items collected from Bahía de Jiquilisco, El Salvador and Estero Padre Ramos, Nicaragua.
δ13C and δ15N values of turtle tissues and putative prey samples were measured with a Costech 4010 (Valencia, CA) elemental analyzer interfaced with a Thermo Scientific Delta V Plus isotope ratio mass spectrometer at the University of New Mexico Center for Stable Isotopes (Albuquerque, NM). Isotopic results are expressed as δ values, where δ13C or δ15N = (Rsample – Rstandard/Rstandard), and Rsample and Rstandard are the 13C/12C or 15N/14N of the sample and standard, respectively. The internationally accepted standards for δ13C and δ15N values are Vienna Pee Dee Belemnite (V-PDB) and atmospheric nitrogen, respectively, and units are parts per thousand, or per mille (‰). Precision for δ13C and δ15N values was estimated by analysis of internal protein standards; mean within run variation (SD) was ≤ 0.2‰ for both δ13C and δ15N values. We also analyzed the weight percent carbon and nitrogen concentrations, reported as [C]/[N], of each sample via comparison with protein standards with known [C] and [N].
Statistical Analysis
The following statistical analyses were performed in R (R Core Team, 2018). Linear regression models were run to predict δ13C values as a function of curved carapace length for immature turtles (37–60 cm curved carapace length) for each site separately, and for both localities together. Generalized additive models (GAMs) were fit to δ13C values as a function of curved carapace length across the whole curved carapace length range at both sites to account for the non-linear relationship; adding in location as a covariate did not significantly improve the GAM. The two most extreme turtle skin δ13C values that we measured were used to characterize tissue isotopic compositions associated with foraging in nearshore marine (−16‰) and estuarine (−23‰) habitats. These end member values are consistent with δ13C values of potential prey collected within estuaries (Table 2) and published isotope data for nearshore marine ecosystems (McMahon et al., 2011; Magozzi et al., 2017; Trueman and St John Glew, 2019).
To aid visualization in Figure 2, we applied an offset of +4 per mil to primary production δ13C values—measured mangrove (this study) and modeled phytoplankton (Magozzi et al., 2017)—estimating the combined effect of isotopic discrimination across trophic levels, and tissue-specific isotopic discrimination. We stress that isotopic discrimination between primary producers and turtle tissues is unknown, thus our ecological inferences are based on differences in the relative isotopic composition of turtle tissues, rather than attempting to quantify resource use via a mixing model.
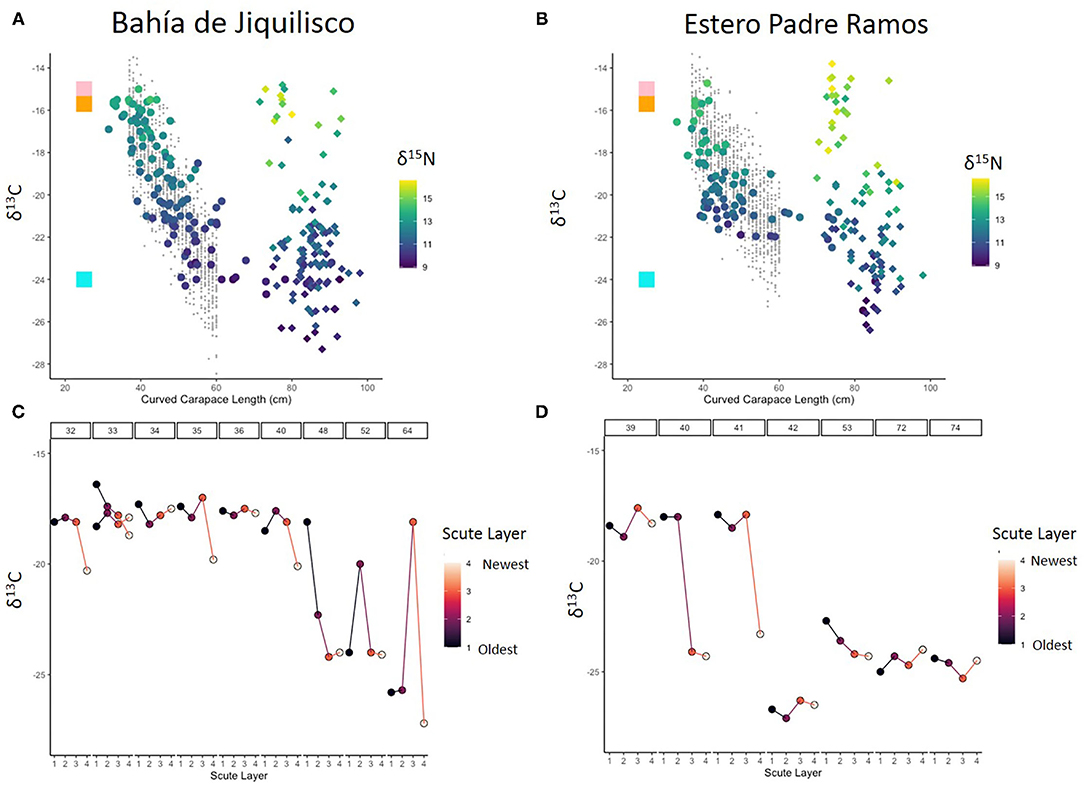
Figure 2. Bulk δ13C and δ15N isotope values (‰) for hawksbill skin (A,B) and scute (C,D). Curved carapace length vs. δ13C for in-water captured turtles (circles) and nesting turtles (diamonds) at (A) Bahía de Jiquilisco (n = 228), (B) Estero Padre Ramos (n = 164); viridis color palette denotes skin δ15N values and small gray circles represent modeled data. For reference, median δ13C values were included for mangrove (turquoise square), eastern Pacific pelagic olive Ridley turtles (orange square; Peavey et al., 2017), and modeled eastern Pacific phytoplankton (pink square; Magozzi et al., 2017). Reference values of primary producers (mangrove and phytoplankton) were offset by 4‰ to estimate correction for trophic discrimination. Scute layer vs. δ13C values at (C) Bahía de Jiquilisco (n = 10) and (D) Estero Padre Ramos (n = 12); Curved carapace length is denoted in boxes along the top of each panel. Scute layer numbers represent relative time prior to capture date, with lower numbers reflecting older time periods, and higher numbers reflecting more recent time periods.
The rate of change in proportional use of estuarine resources as a function of turtle carapace length during the transition between open coastal marine habitats and estuarine resources was then estimated from the slope of the best-fit linear model where y-axis units were scaled between 0 (no use of estuarine resources) to 1 (complete dependence on estuarine resources). The full range of measured data were simulated using Monte Carlo resampling of linear models including a 5% standard deviation around the predicted δ13C values.
We used ANOVA to compare mean δ13C and δ15N values of prey items collected within the estuaries (mangroves, barnacles, and sponges) using JMP 15 (SAS Institute Inc., 2019). Normality checks were carried out and the assumptions were met for the δ15N prey data, but not for the δ13C prey data. Thus, we ran an ANOVA and Tukey-Kramer post-hoc test to compare δ15N means, and for δ13C values we ran Kruskal-Wallis Tests and did a non-parametric comparison using the Wilcoxon Method.
Results
Fishers' Ecological Knowledge
Based entirely on FEK, we created the first-ever habitat use map for hawksbills of all sizes (hatchling – adult) within our study sites (Figures 1, 3 in Wedemeyer-Strombel et al., 2019), which was used exclusively to guide our in-water capture and sampling of turtles throughout both estuaries. In addition to locality-specific information, thematic analysis of interviews (see Methods) categorized FEK into four major themes (Table 1).
During interviews, fishers specifically mentioned seeing juvenile turtles in the estuaries throughout the year, which suggests that some immature hawksbills exhibit an estuarine developmental phase. FEK states that ecological mechanisms drive hawksbill estuarine associations, with food, safety (shelter), and environmental factors (e.g., rocky substrate, tides, water depth, and water temperature) emerging as the primary drivers. Fishers were often specific in naming the diet of eastern Pacific hawksbills, with barnacles (Balanus sp.) being the most common, followed by red mangroves (see Supplementary Materials for specific quotations). Further analysis of Theme 4 is beyond the scope of this study. Further analysis of the FEK data can be found in Wedemeyer-Strombel (2019), and the theoretical framework and advantages to our collaborative approach to working with the fishers can be found in Wedemeyer-Strombel et al. (2019).
Hawksbill Skin
We sampled a total of 392 hawksbills for δ13C and δ15N analysis (Table 3), including 228 from in or near Bahía de Jiquilisco and 164 from Estero Padre Ramos. Of these 392 turtles, 185 were captured in-water, of which 172 were immature turtles (30–65 cm curved carapace length, CCL) and 13 were adults (>65 cm CCL; Table 3). In Bahía de Jiquilisco, three of the 117 nesting adults were sampled twice on separate occasions. In Estero Padre Ramos one immature turtle was sampled twice and four nesting adults were each sampled twice on separate occasions (Supplementary Table 2). The relationship between skin δ13C value and curved carapace length in the transition period between 37 and 60 cm was best explained by a simple linear model describing two-component mixing between the two most extreme measured values taken as best estimates of end member values. δ13C and δ15N values for immature hawksbill skin (n = 172) decrease as CCL increases at both Bahía de Jiquilisco (R2 = 0.88, df = 116, p < 0.001) and Estero Padre Ramos (R2 = 0.86, df = 72, p < 0.001). Across all sampled non-nesting turtles collected from both study sites (immature n = 172, adult n = 13), both δ13C and δ15N values decrease non-linearly. General additive models (GAM) of sea turtle CCL predicting δ13C values as a function of CCL alone explained 72% of variance in δ13C values for Bahía de Jiquilisco turtles, and 55% of variance in δ13C values for Estero Padre Ramos turtles (Figures 2A,B).
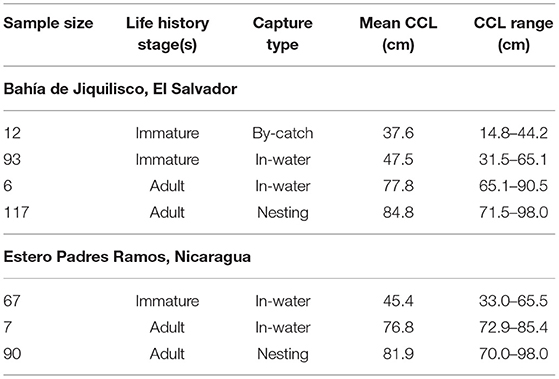
Table 3. Summary of stable isotope analysis sample sizes by life history stage, capture type, CCL mean, and range Total n = 392, Bahía de Jiquilisco (n = 228), and Estero Padre Ramos (n = 164).
A linear model predicting δ13C values in all immature turtles as a function of CCL (R2 = 0.48, df = 156, p < 0.001) suggests that immature turtles proportionally increase their reliance on mangrove-based estuarine resources by ~4% on average with each 1-cm increase in CCL (Figures 2A,B), or 40% for each 10-cm increase. This suggests that juvenile eastern Pacific hawksbills, with an average growth rate of 3.6 cm per year (Llamas et al., 2017), are increasing their reliance on mangrove-based estuarine resources by ~14.4% per year. Data from our resampled turtles (Supplementary Table 2) reflected the same increase in consumption of estuarine resources (indicated by lower δ13C values) with increased CCL.
Hawksbill Scute
We measured scute δ13C and δ15N values of 17 turtles (Figure 2; Supplementary Tables 3, 4) including 10 from Bahía de Jiquilisco and 7 from Estero Padre Ramos. For each of the 17 scutes we analyzed four scute layers to get a chronological history of turtle habitat use. Layers 1 and 4 represented the oldest and youngest tissue, respectively (Supplementary Figure 1; Supplementary Tables 3, 4).
Across both sites, scute results corresponded with skin results: δ13C values decreased with increasing CCL, indicating an increased reliance on estuarine food as turtles grew. These results were further supported by scute tissue δ13C values, which decreased with turtle size (Figures 2C,D), consistent with gradual increase in the use of mangrove estuary habitats as turtles developed. The smallest turtles (<40cm CCL) had δ13C values indicative of pelagic resources across all four scute layers, with their newest tissue generally having lower values than their oldest tissue—suggesting the initial transition in the use of a pelagic- to an estuarine-based foodweb. Turtles 40–50 cm CCL showed further use of the estuarine food web, with values of < -20‰ for most recent scute tissue. The largest turtles (50–80 cm CCL) had the lowest δ13C values, which remained consistent across scute layers for most individuals, indicative of heavy reliance on estuarine resources. Lastly, the scutes of turtles >65 cm CCL showed a high degree of variation in δ13C (Figure 2), consistent with adults moving between estuarine and phytoplankton fueled habitats (Table 1, Theme 2; Guzmán Hernández et al., 2010; Gaos et al., 2012a,b,c).
Putative Prey
Mean (±SD) δ13C and δ15N values for putative estuarine prey items are reported in Table 2. Mean δ13C values for all prey types were lower than −20‰, which is consistent with mangrove-based food webs (Fry and Ewel, 2003; McMahon et al., 2011). Mean δ13C values for prey bulk tissues (mangrove, barnacles, sponges) were significantly different (Chi square = 44.0571, p <0.0001, df = 2). Mangrove had significantly lower δ13C values than barnacles (p <0.0001) and sponges (p <0.0001), although δ13C values for the latter two prey types were not significantly different (p = 0.42). Mean δ15N values for prey types were significantly different [F(2, 61) = 250.465, p <00001]. Mangrove δ15N values were significantly lower than those for barnacles (p <0.0001) and sponges (p <0.0001); δ15N for the latter two prey types were not significantly different (p = 0.81).
The δ13C and δ15N values of potential hawksbill prey we collected from estuaries were ~8–10‰ and 10‰ lower, respectively, than those estimated for particulate organic matter (POM) in the eastern Pacific (Trueman and St John Glew, 2019). Further, immature hawksbills with the highest δ13C values, which are assumed to primarily forage in pelagic phytoplankton-fueled food webs (Figures 2A,B) had tissue δ13C values similar to those of olive ridley turtles captured off the coasts of El Salvador and Nicaragua (Peavey et al., 2017). Together, these patterns demonstrate that δ13C and δ15N values were reliable tracers to examine the use of mangrove vs. nearshore pelagic habitats in the eastern Pacific Ocean.
Discussion
Our results demonstrated how local ecological knowledge and quantitative approaches such as stable isotope analysis can be combined to provide multiple lines of evidence on important conservation questions. In Wedemeyer-Strombel et al. (2019) we detailed the theoretical framework and approach to collaborating with local fishers. In the current study, we analyzed the qualitative data collected through the collaborative interviews, and integrated it with stable isotope analysis to explore how and to what extent critically endangered hawksbill sea turtles used mangrove habitats throughout their life cycle in the eastern Pacific Ocean. While local fishers have known for generations that hawksbills use these estuaries throughout their life cycles, stable isotope analysis numerically supports this knowledge, helping bring it to the light of biological conservation. Both the FEK and tissue isotope data suggested that eastern Pacific hawksbills utilizing mangrove estuaries experienced an early pelagic stage and then recruited into mangrove habitats for an estuarine developmental stage, before switching to a mixed (estuarine and pelagic) strategy as adults (Table 1, Themes 1–3; Figures 2A–D). Once immature turtles recruited into the estuaries at ~37 cm CCL, they transitioned from a pelagic phytoplankton-based diet to an estuarine mangrove-based diet, increasing their reliance on estuarine resources as they increased in size (Table 1, Themes 1–3; Figures 2A–D). Once they reached adulthood (>65 cm CCL; Liles et al., 2015b; Wildermann et al., 2018), some individuals continued relying on resources in mangrove estuaries, while others foraged in pelagic nearshore food webs or used a mixture of these habitats (Table 1, Themes 2–3; Figures 2A–D).
Although this pattern may not apply for the entire eastern Pacific population (Llamas et al., 2017), the agreement in our study between FEK and isotope results across the two primary rookeries for this population suggest that this could be a prominent regional trend, especially for hawksbills using mangrove estuarine habitats. As such, we encourage the use of similar approaches to study other known estuarine rookeries for the eastern Pacific population (Gaos et al., 2017, 2018), and for hawksbills found in non-estuary habitats.
The importance of mangrove estuaries as developmental habitat for this imperiled sea turtle species could galvanize public and stakeholder support for the conservation of these habitats throughout the eastern Pacific region (Gaos et al., 2018). When Gaos et al. (2018) documented that turtles transition from a pelagic to a nearshore existence (termed natal foraging philopatry), they questioned what mechanisms may be driving this transition. FEK and isotopic data reported here showed a transition to, and dependency on, estuarine food webs through immature life stages: supporting resource availability as a potential mechanism driving natal foraging philopatry (Table 1, Themes 1–3; Figures 2A–D). Although without direct observation, we cannot exclude the possibility that some turtles foraged outside of the estuary, the FEK results and lack of stable isotope values reflecting coastal prey species suggests that this was an infrequent occurrence, if at all. Hawksbills are thought to primarily forage and associate with coral reefs (Wyneken et al., 2013); however, these habitats in the eastern Pacific are small with a patchy distribution, low species diversity, and are prone to disturbances (Cortés, 1997). In contrast, mangrove estuaries have an abundance of fish and invertebrate species (Rönnbäck, 1999) and are found throughout the population range for eastern Pacific hawksbills. Similarly, resource availability is thought to drive individuals of multiple sea turtle species and populations [e.g., flatbacks (Natator depressus), leatherbacks (Dermochelys coriacea), and eastern Pacific olive ridleys (Lepidochelys olivacea)] to remain in particular habitats throughout their lives (Bolten, 2003).
Hawksbill size at recruitment reported in our study is of particular importance, as the sizes of turtles first recruiting into estuary habitats is consistent with turtles found bycaught in artisanal fisheries off the coasts of El Salvador and Nicaragua (Liles et al., 2017). Unintended capture of hawksbills in nearshore eastern Pacific artisanal fisheries is believed to be the greatest source of mortality for the population, with an estimated minimum mortality of 227 hawksbills over a 3 year study (Liles et al., 2017). A better understanding of the use of pelagic nearshore habitats by small (<37 cm) hawksbills could help to ensure that the population is not experiencing high mortality within coastal fisheries. High mortality rates within these habitats could reverse the positive impacts of extensive conservation efforts currently underway to protect juvenile and nesting turtles in mangrove estuary ecosystems.
The baseline data used to conduct this study were generated through collaborations with local fishers in Bahía de Jiquilisco and Estero Padre Ramos who have long recognized that immature hawksbills utilize their mangrove estuary rookeries for foraging (Wedemeyer-Strombel, 2019), a phenomenon that has only recently been recognized in the scientific literature (Torres Gago et al., 2016; Gaos et al., 2018; Méndez-Salgado et al., 2020). In our study, FEK data not only identified specific foraging locations (Wedemeyer-Strombel, 2019; Wedemeyer-Strombel et al., 2019), but also demonstrated why hawksbills were foraging in these habitats. Isotope data corroborated the FEK results, highlighting the value, and importance of incorporating this under-utilized source of information early and often in conservation efforts. Although we recognize that FEK or its equivalent may not be available to all studies, neither is it limited to this case or this species. The agreement between our stable isotope results and the FEK elicited from our interviews indicates that local knowledge was highly reliable and suggests that it could provide previously unavailable baseline data to jumpstart, and even guide, future conservation research. Strategic integration of local knowledge with traditional scientific methods has strong potential to simultaneously enhance conservation research and strengthen public support for species management in any situation where humans and wildlife rely on shared resources.
The use of participatory approaches to research that actively involve local fishers who live with hawksbills has important implications for conservation. Because natural resource management and environmental law enforcement are typically weaker in low-income regions, primary resource users often self-govern resource use in their local environment (Dietz et al., 2003), such as local fishers in El Salvador and Nicaragua. This local reality highlights the power and control local fishers wield in determining the success or failure of hawksbill conservation initiatives (Liles et al., 2015a) and underscores the importance of partnering with local residents to reduce threats to hawksbills in mangrove habitats, including boat collisions, destructive fishing practices, plastic pollution, and deforestation. The spatially restricted movement and foraging behavior of immature hawksbills within mangrove estuaries offers a unique and valuable conservation opportunity to focus protection efforts on high-use areas (Gaos et al., 2012a). Livelihood incentive programs that offer direct payments to local resource users for conservation outcomes have been successful in El Salvador and Nicaragua for protecting hawksbill nesting beaches (Liles et al., 2019) and monitoring hawksbill bycatch in lobster gillnet fisheries (Liles et al., 2017). These programs could be expanded to incentivize hawksbill protection at key developmental areas in Bahía de Jiquilisco and Estero Padre Ramos.
Our results provide a crucial link to guide future conservation in the region: mangrove estuaries serve as both a critical rookery and foraging ground for eastern Pacific hawksbills throughout their development. We also document that some young eastern Pacific hawksbills experience a pelagic stage and then recruit into mangrove estuaries, and forage extensively within estuarine food webs for several years as they transition to larger juvenile life stages, finally adopting a mixed pelagic-estuarine foraging strategy as adults (Table 1, Themes 1–3; Figures 2A–D). More broadly, our results show the impact and validity of integrating local ecological knowledge and quantitative scientific data. This integrated approach potentially has broad applicability to the many pressing conservation challenges in understudied regions throughout the world.
Data Availability Statement
The datasets presented in this study can be found in online repositories. The names of the repository/repositories and accession number(s) can be found at: dx.doi.org/10.6084/m9.figshare.13370165 and dx.doi.org/10.6084/m9.figshare.13370171.
Ethics Statement
The studies involving human participants were reviewed and approved by University of Texas at El Paso Institutional Review Board (896427-1). Written informed consent for participation was not required for this study in accordance with the national legislation and the institutional requirements. The animal study was reviewed and approved by CITES (15US844694/9), University of Texas at El Paso Animal Use Protocol (A-201608-1), El Salvador Ministry of the Environment and Natural Resources (MARN: MARN-DEV-GVS-007-2016; MARN-DEV-GVS-008-2016), and Nicaragua Ministry of the Environment and Natural Resources (MARENA: DGPN/DB-IC-014-2016).
Author Contributions
KW-S, ML, MP, TP, and JS conceived and designed the study. KW-S, ML, RS, SC, MV, and EA collected data. KW-S, ML, RS, SC, MV, EA, and VG coordinated fieldwork. KW-S, TP, and NH carried out qualitative analysis. KW-S, SN, and KS performed isotope lab work. KW-S, SN, JS, and CT analyzed isotope data. CT ran statistical analyses. KW-S led the writing. SN, JS, CT, MP, ML, TP, and KS provided important comments, suggestions during data analysis, and manuscript preparations. All authors contributed to the article and approved the submitted version.
Funding
We thank the University of Texas, El Paso Graduate School Dodson Research Grant and the PADI Foundation for financial support for this research. MP purchased some of our field equipment. Stable isotope analysis was supported by NSF award #1137336, Inter-university Training in Continental-scale Ecology, and by the National Oceanic and Atmospheric Administration, Southwest Fisheries Science Center. This material was based upon work supported by the National Science Foundation Graduate Research Fellowship under Grant No. DGE-1252521.
Author Disclaimer
Any opinion, findings, and conclusions or recommendations expressed in this material are those of the authors and do not necessarily reflect the views of the National Science Foundation.
Conflict of Interest
The authors declare that the research was conducted in the absence of any commercial or financial relationships that could be construed as a potential conflict of interest.
Publisher's Note
All claims expressed in this article are solely those of the authors and do not necessarily represent those of their affiliated organizations, or those of the publisher, the editors and the reviewers. Any product that may be evaluated in this article, or claim that may be made by its manufacturer, is not guaranteed or endorsed by the publisher.
Acknowledgments
We thank the communities of Bahía de Jiquilisco, El Salvador and Estero Padre Ramos, Nicaragua, and all the fishers who collaborated with us for sharing their time and knowledge. This work was made possible by collaborations with Asociación ProCosta, ICAPO, Flora and Fauna International, and the field support of David Melero. Thanks to NOAA Southwest Fisheries Science Center and Joel Schumacher, and the University of New Mexico's Center for Stable Isotopes, specifically John Whiteman, Sarah Foster, Deborah Boro, Laura Pages Barcelo, Laura Burkemper, and Nicu-Viorel Atudorei for their support. Special thank you to Matthew Burgess and Cali Turner Tomaszewicz for constructive comments on this manuscript.
Supplementary Material
The Supplementary Material for this article can be found online at: https://www.frontiersin.org/articles/10.3389/fcosc.2021.796868/full#supplementary-material
References
Bolstad, P. (2012). GIS Fundamentals: A First Text on Geographic Information Systems, 4th Edn. White Bear Lake, MN: Eider Press.
Bolten, A. B. (2003). “Variation in sea turtle life history patterns: neritic vs. oceanic developmental stages,” in The Biology of Sea Turtles, Vol. 2, eds P. L. Lutz, J. Musick, and J. Wyneken (Boca Raton, FL: CRC Press), 243–257. doi: 10.1201/9781420040807.ch9
Bouillon, S., Connolly, R., and Gillikin, D. (2011). “Use of stable isotopes to understand food webs and ecosystem functioning in estuaries,” in Treatise on Estuarine and Coastal Science, eds E. M. Wolanski and D. S. McLusky (Waltham, MA: Academic Press), 143–173. doi: 10.1016/B978-0-12-374711-2.00711-7
Cohen, J. (1968). Weighted kappa: nominal scale agreement with provision for scaled disagreement or partial credit. Psychol. Bull. 70, 213–220. doi: 10.1037/h0026256
Corbin, J., and Strauss, A. (1990). Grounded theory research: procedures, canons, and evaluative criteria. Qual. Sociol. 13, 3–21. doi: 10.1007/BF00988593
Cortés, J. (1997). Biology and geology of eastern Pacific coral reefs. Coral Reefs 16, S39–S46. doi: 10.1007/s003380050240
Dietz, T., Ostrom, E., and Stern, P. C. (2003). The struggle to govern the commons. Science 302, 1907–1912. doi: 10.1126/science.1091015
Drew, J. A. (2005). Use of traditional ecological knowledge in marine conservation. Conserv. Biol. 19, 1286–1293. doi: 10.1111/j.1523-1739.2005.00158.x
Fry, B., and Ewel, K. C. (2003). Using stable isotopes in mangrove fisheries research - a review and outlook. Isotopes Environ. Health Stud. 39, 191–196. doi: 10.1080/10256010310001601067
Gaos, A. R., Abreu-Grobois, F. A., Alfaro-Shigueto, J., Amorocho, D., Arauz, R., Baquero, A., et al. (2010). Signs of hope in the eastern Pacific: international collaboration reveals encouraging status for a severely depleted population of hawksbill turtles Eretmochelys imbricata. Oryx 44, 595–601. doi: 10.1017/S0030605310000773
Gaos, A. R., Lewison, R. L., Jensen, M. P., Liles, M. J., Henriquez, A., Chavarria, S., et al. (2018). Rookery contributions, movements and conservation needs of hawksbill turtles at foraging grounds in the eastern Pacific Ocean. Mar. Ecol. Progr. Ser. 586, 203–216. doi: 10.3354/meps12391
Gaos, A. R., Lewison, R. L., Liles, M. J., Gadea, V., Altamirano, E., Henriquez, A. V., et al. (2016). Hawksbill turtle terra incognita: conservation genetics of eastern Pacific rookeries. Ecol. Evol. 6, 1251–1264. doi: 10.1002/ece3.1897
Gaos, A. R., Lewison, R. L., Wallace, B. P., Yañez, I. L., Liles, M. J., Nichols, W. J., et al. (2012a). Spatial ecology of critically endangered hawksbill turtles Eretmochelys imbricata: implications for management and conservation. Mar. Ecol. Progr. Ser. 450, 181–194. doi: 10.3354/meps09591
Gaos, A. R., Lewison, R. L., Yañez, I. L., Wallace, B. P., Liles, M. J., Nichols, W. J., et al. (2012b). Shifting the life-history paradigm: discovery of novel habitat use by hawksbill turtles. Biol. Lett. 8, 54–56. doi: 10.1098/rsbl.2011.0603
Gaos, A. R., Lewison, R. R., Wallace, B. P., Yañez, I. L., Liles, M. J., Baquero, A., et al. (2012c). Dive behaviour of adult hawksbills (Eretmochelys imbricata, Linnaeus 1766) in the eastern Pacific Ocean highlights shallow depth use by the species. J. Exp. Mar. Biol. Ecol. 432–433, 171–178. doi: 10.1016/j.jembe.2012.07.006
Gaos, A. R., Liles, M. J., Gadea, V., Pena, A., Vallejo, F., Miranda, C., et al. (2017). Living on the edge: hawksbill turtle nesting and conservation along the eastern Pacific Rim. Latin Am. J. Aquat. Res. 45, 572–584. doi: 10.3856/vol45-issue3-fulltext-7
Gaos, A. R., and Yañez, I. L. (2012). “Saving the eastern Pacific hawksbill from extinction: Last chance or chance lost?,” in Sea Turtles of the Eastern Pacific: Advances in Research and Conservation, eds J. A. Seminoff and B. P. Wallace (Tucson: University of Arizona Press), 244–262. doi: 10.2307/j.ctv21hrddc.14
Guzmán Hernández, V. G. A., Pedro, A., and Huerta Rodríguez, P. (2010). Anidaciones de tortuga carey (E. imbricata) dentro de una laguna costera en el sureste de México. JAINA Boletín Informativo 21.
Haggan, N., Neis, B., and Baird, I. G., (eds.). (2007). Fishers' Knowledge in Fisheries Science and Management. Paris: UNESCO Publishing.
Haywood, J. C., Fuller, W. J., Godley, B. J., Shutler, J. D., Widdicombe, S., and Broderick, A. C. (2019). Global review and inventory: how stable isotopes are helping us understand ecology and inform conservation of marine turtles. Mar. Ecol. Progr. Ser. 613, 217–245. doi: 10.3354/meps12889
Herzka, S. Z. (2005). Assessing connectivity of estuarine fishes based on stable isotope ratio analysis. Estuar. Coast. Shelf Sci. 64, 58–69. doi: 10.1016/j.ecss.2005.02.006
Hobson, K. A. (2008). Applying isotopic methods to tracking animal movements. Terrestr. Ecol. 2, 45–78. doi: 10.1016/S1936-7961(07)00003-6
Hunt, J. (1970). “The significange of carbon isotope variations in marine sediments,” in Advances in Organic Geochemistry, eds G. S. Hobson and G. C. Speers (Oxford: Pergamon Press), 27–35. doi: 10.1016/B978-0-08-012758-3.50005-0
IUCN (2018). 2018 IUCN Red List of Threatened Species. IUCN-SSC. Available online at: http://www.iucnredlist.org (accessed October 1, 2021).
Johannes, R., Freeman, M., and Hamilton, R. J. (2000). Ignore fishers' knowledge and miss the boat. Fish Fish. 1, 257–271. doi: 10.1046/j.1467-2979.2000.00019.x
Jones, T. T., and Seminoff, J. A. (2013). “Feeding biology, advances from field-based observations, physiological studies, and molecular techniques,” in The Biology of Sea Turtles Vol. 3, eds J. Wyneken, K. J. Lohmann, and J. A. Musick (Boca Raton, FL: CRC Press), 211–248.
Kincheloe, J. L., and McLaren, P. (2005). “Rethinking critical theory and qualitative research,” in The Sage Handbook of Qualitative Research, eds N. K. Denzin and Y. S. Lincoln (Los Angeles, CA: Sage Publications, Inc.), 303–342.
Kiszka, J. J., Caputo, M., Méndez-Fernandez, P., and Fielding, R. (2021). Feeding ecology of elusive Caribbean Killer whales inferred from bayesian stable isotope mixing models and whalers' ecological knowledge. Front. Mar. Sci. 8:648421. doi: 10.3389/fmars.2021.648421
Krippendorff, K. (2013). Content Analysis: An Introduction to Its Methodology. Thousand Oaks, CA: Sage Publications.
Liles, M. J., Gaos, A. R., Bolanos, A. D., Lopez, W. A., Arauz, R., Gadea, V., et al. (2017). Survival on the rocks: high bycatch in lobster gillnet fisheries threatens hawksbill turtles on rocky reefs along the Eastern Pacific coast of Central America. Latin Am. J. Aquat. Res. 45, 521–539. doi: 10.3856/vol45-issue3-fulltext-3
Liles, M. J., Peterson, M. J., Lincoln, Y. S., Seminoff, J. A., Gaos, A. R., and Peterson, T. R. (2015a). Connecting international priorities with human wellbeing in low-income regions: lessons from hawksbill turtle conservation in El Salvador. Local Environ. 20, 1383–1404. doi: 10.1080/13549839.2014.905516
Liles, M. J., Peterson, M. J., Seminoff, J. A., Altamirano, E., Henríquez, A. V., Gaos, A. R., et al. (2015b). One size does not fit all: importance of adjusting conservation practices for endangered hawksbill turtles to address local nesting habitat needs in the eastern Pacific Ocean. Biol. Conservat. 184, 405–413. doi: 10.1016/j.biocon.2015.02.017
Liles, M. J., Peterson, T. R., Seminoff, J. A., Gaos, A. R., Altamirano, E., Henríquez, A. V., et al. (2019). Potential limitations of behavioral plasticity and the role of egg relocation in climate change mitigation for a thermally sensitive endangered species. Ecol. Evol. 9, 1603–1622. doi: 10.1002/ece3.4774
Llamas, I., Flores, E., Abrego, M., Seminoff, J., Hart, C., Pena, B., et al. (2017). Distribution, size range and growth rates of hawksbill turtles at a major foraging ground in the eastern Pacific Ocean. Latin Am. J. Aquat. Res. 45, 597–605. doi: 10.3856/vol45-issue3-fulltext-9
Machiwa, J. (2000). δ13C signatures of flora, macrofauna and sediment of a mangrove forest partly affected by sewage wastes. Tanzania J. Sci. 26, 15–28. doi: 10.4314/tjs.v26i1.18325
Magozzi, S., Yool, A., Vander Zanden, H. B., Wunder, M. B., and Trueman, C. N. (2017). Using ocean models to predict spatial and temporal variation in marine carbon isotopes. Ecosphere 8:e01763. doi: 10.1002/ecs2.1763
McMahon, K. W., Berumen, M. L., Mateo, I., Elsdon, T. S., and Thorrold, S. R. (2011). Carbon isotopes in otolith amino acids identify residency of juvenile snapper (Family: Lutjanidae) in coastal nurseries. Coral Reefs 30, 1135–1145. doi: 10.1007/s00338-011-0816-5
Méndez-Salgado, E., Chacón-Chaverri, D., Fonseca, L., Rojas-Morales, D., and Seminoff, J. (2020). Trophic ecology of hawksbill turtles (Eretmochelys imbricata) in Golfo Dulce, Costa Rica: integrating oesophageal lavage and stable isotope (δ13C, δ15N) analysis. Latin Am. J. Aquat. Res. 48, 114–130. doi: 10.3856/vol48-issue1-fulltext-2230
Ostrom, E. (2009). A general framework for analayzing sustainability of social-ecological systems. Science 325, 419–422. doi: 10.1126/science.1172133
Palaniappan, P. M. (2007). The carapace scutes of hawskbill turtles (Eretmocheyls imbricata): Development, growth dynamics and utility as an age indicator (Ph.D.), Charles Darwin University, Casuarina, NT, Australia.
Peavey, L. E., Popp, B. N., Pitman, R. L., Gaines, S. D., Arthur, K. E., Kelez, S., et al. (2017). Opportunism on the high seas: foraging ecology of olive ridley turtles in the eastern Pacific Ocean. Front. Mar. Sci. 4:348. doi: 10.3389/fmars.2017.00348
Peterson, T. R., Witte, K., Enkerlin-Hoeflich, E., Espericueta, L., Flora, J. T., Florey, N., et al. (1994). Using informant directed interviews to discover risk orientation: how formative evaluations based in interpretive analysis can improve persuasive safety campaigns. J. Appl. Commun. Res. 22, 199–215. doi: 10.1080/00909889409365398
R Core Team (2018). A Language and Environment for Statistical Computing. Vienna: R Foundation for Statistical Computing.
Reich, K. J., Bjorndal, K. A., and Bolten, A. B. (2007). The 'lost years' of green turtles: using stable isotopes to study cryptic lifestages. Biol. Lett. 3, 712–714. doi: 10.1098/rsbl.2007.0394
Rönnbäck, P. (1999). The ecological basis for economic value of seafood production supported by mangrove ecosystems. Ecol. Econ. 29, 235–252. doi: 10.1016/S0921-8009(99)00016-6
Smith, K. J., Trueman, C. N., France, C. A. M., Sparks, J. P., Brownlow, A. C., Dähne, M., et al. (2021). Stable isotope analysis of specimens of opportunity reveals ocean-scale site fidelity in an elusive whale species. Front. Conserv. Sci. 2:653766. doi: 10.3389/fcosc.2021.653766
Torres Gago, P., Abreu-Grobois, A., Gaos, A., Mairena, D., Rivas, S., Altamirano, E., et al. (2016). “Movement patterns of juvenile and sub-adult hawksbill turtles (Eretmochelys imbricata) in mangrove estuaries along the pacific coast of El Salvador and Nicaragua,” in 36th Annual Symposium on Sea Turtle Biology and Conservation (Lima).
Trueman, C. N., and St John Glew, K. (2019). “Isotopic tracking of marine animal movement,” in Tracking Animal Migration with Stable Isotopes, 2 Edn, eds K. A. Hobson and L. I. Wassenaar (London: Elsevier), 137–172. doi: 10.1016/B978-0-12-814723-8.00006-4
Vander Zanden, H. B., Bjorndal, K. A., Reich, K. J., and Bolten, A. B. (2010). Individual specialists in a generalist population: results from a long-term stable isotope series. Biol. Lett. 6, 711–714. doi: 10.1098/rsbl.2010.0124
Vásquez, M., and Liles, M. J. (2008). “Estado actual de las tortugas marinas en El Salvador, con énfasis en la tortuga carey,” in XII Congreso de la Sociedad Mesoamericana para la Biología y la Conservación (San Salvador).
Virapongse, A., Brooks, S., Metcalf, E. C., Zedalis, M., Gosz, J., Kliskey, A., et al. (2016). A social-ecological systems approach for environmental management. J. Environ. Manag. 178, 83–91. doi: 10.1016/j.jenvman.2016.02.028
Wedemeyer-Strombel, K. R. (2019). Fishers' ecological knowledge and stable isotope analysis: A social-ecological systems approach to endangered species conservation (Ph.D). University of Texas at El Paso, El Paso, TX, United States.
Wedemeyer-Strombel, K. R., Peterson, M. J., Sanchez, R. N., Chavarría, S., Valle, M., Altamirano, E., et al. (2019). Engaging fishers' ecological knowledge for endangered species conservation: four advantages to emphasizing voice in participatory action research. Front. Commun. 4:30. doi: 10.3389/fcomm.2019.00030
Wildermann, N. E., Gredzens, C., Avens, L., Barrios-Garrido, H. A., Bell, I., Blumenthal, J., et al. (2018). Informing research priorities for immature sea turtles through expert elicitation. Endang. Spec. Res. 37, 55–76. doi: 10.3354/esr00916
Keywords: Eretmochelys imbricata, habitat use, local knowledge, foraging ecology, fishers' ecological knowledge, mangrove estuary, stable isotope analysis
Citation: Wedemeyer-Strombel KR, Seminoff JA, Liles MJ, Sánchez RN, Chavarría S, Valle M, Altamirano E, Gadea V, Hernandez N, Peterson MJ, Smith KJ, Trueman CN, Peterson TR and Newsome SD (2021) Fishers' Ecological Knowledge and Stable Isotope Analysis Reveal Mangrove Estuaries as Key Developmental Habitats for Critically Endangered Sea Turtles. Front. Conserv. Sci. 2:796868. doi: 10.3389/fcosc.2021.796868
Received: 17 October 2021; Accepted: 24 November 2021;
Published: 23 December 2021.
Edited by:
Oded Berger-Tal, Ben-Gurion University of the Negev, IsraelReviewed by:
Gilberto Corso, Federal University of Rio Grande do Norte, BrazilEduardo Cuevas, Laboratorio de Ecología Espacial y del Movimiento, Mexico
Chelsea E. Clyde-Brockway, The Leatherback Trust, Costa Rica
Copyright © 2021 Wedemeyer-Strombel, Seminoff, Liles, Sánchez, Chavarría, Valle, Altamirano, Gadea, Hernandez, Peterson, Smith, Trueman, Peterson and Newsome. This is an open-access article distributed under the terms of the Creative Commons Attribution License (CC BY). The use, distribution or reproduction in other forums is permitted, provided the original author(s) and the copyright owner(s) are credited and that the original publication in this journal is cited, in accordance with accepted academic practice. No use, distribution or reproduction is permitted which does not comply with these terms.
*Correspondence: Kathryn R. Wedemeyer-Strombel, krwedemeyer@gmail.com