- 1Laboratory of Zoology, Research Institute for Biosciences, University of Mons, Mons, Belgium
- 2Smithsonian Tropical Research Institute, Gamboa, Panama
The unsustainable use of ecosystems by human societies has put global biodiversity in peril. Bees are, in this context, a popular example of a highly diversified group of pollinators whose collapse is a major concern given the invaluable ecosystem services they provide. Amongst them, bumblebees (Bombus) have increasingly drawn the attention of scientists due to their dramatic population declines globally. This regression has converted them into popular conservation entities, making them the second most studied group of bees worldwide. However, in addition to have become relevant models in the fields of ecology, evolution and biogeography, bumblebees have also been used as models for studying wild bee decline and conservation worldwide. Integrating evidence from the comparative ecology and resilience of bumblebees and wild bees, I discuss the relevance of using Bombus as radars for wild bee decline worldwide. Responses of bumblebees to environmental changes are generally not comparable with those of wild bees because of their relatively long activity period, their inherent sensitivity to high temperatures, their relatively generalist diet breadth and many aspects arising from their eusocial behavior. Moreover, important differences in the available historical data between bumblebees and other bees make comparisons of conservation status even more arduous. Overall, these results reinforce the need for conservation actions that consider a higher level of understanding of ecological diversity in wild bees, highlight the need for an updated and more extensive sampling of these organisms, and emphasize that more caution is required when extrapolating trends from model species.
Introduction
The unsustainable use of ecosystems by human societies has made 75–95% of the terrestrial biosphere to be altered by anthropogenic stressors, with considerable impacts on its fauna and flora (Kennedy et al., 2019; Ellis et al., 2021; Wagner et al., 2021). Among the myriad of threatened organisms are bees (Hymenoptera: Anthophila), a highly diversified group of pollinators represented by more than 20.000 described species globally (Michener, 2007; Zattara and Aizen, 2021). Their collapse in both abundance and diversity is a critical issue for ecosystems and human societies given the invaluable ecosystem services they provide in the perpetuation of numerous wild plants and crops worldwide (Matias et al., 2017; Ollerton, 2017; Porto et al., 2020). As for most threatened insects (Wagner, 2020; Wagner et al., 2021), bee decline involves climate change, landscape destruction, the misuse/overuse of pesticides, the arrival of invasive species and the transmission of pathogens as predominant drivers (Cariveau and Winfree, 2015; Carvalheiro et al., 2020; Mathiasson and Rehan, 2020; LeBuhn and Vargas Luna, 2021).
Bumblebees (Bombus spp.), known by ~270 species at the global scale, are a clade of bees mostly widespread across America, Europe and Asia, with distributions encompassing all biomes except extremely xeric deserts (Williams, 1998; Williams and Jepsen, 2021). These pollinators are increasingly used as models in a large array of scientific fields including ecology (Phelan et al., 2021), evolution (Tian et al., 2019; Wood et al., 2021b) and biogeography (Ghisbain et al., 2020; Potapov et al., 2021). Most concerningly however, bumblebees have increasingly drawn the attention of scientists worldwide due to the dramatic regressions of their populations across all continents they occupy (Bartomeus et al., 2013; Kerr et al., 2015; Aizen et al., 2019; Rasmont et al., 2021). These repeated patterns of decline have converted them into popular conservation entities, making them the second most studied bees worldwide after the honeybee Apis mellifera (Cameron and Sadd, 2020).
Against this context of global bee decline, Wood et al. (2020) discussed the relevance of using the A. mellifera as a sensor for wild bee decline. The authors showed that despite some of the responses of honeybees to anthropic stressors being similar to those of wild bees, this managed species is not a suitable surrogate for detecting declines in wild bees. In this review, I raise a similar concern for bumblebees, discussing their relevance as models to global changes for other wild bees.
Bumblebees as Flagship Species for Bee Conservation
Bumblebees have always been immensely popular due to their great cultural value in north temperate regions (Rasmont et al., 2021). The overall colorful appearance, abundance and diversity of bumblebees in areas frequented by natural historians of the northern hemisphere have made them especially well-represented in museum collections (Kleijn and Raemakers, 2008; Cameron et al., 2011; Wood et al., 2019, 2021b). This has led scientists to rapidly acquire paramount quantities of data in comparison to other groups of wild bees (Nieto et al., 2014). As a direct benefit of having acquired so much biogeographic data on these culturally “cherished” animals is the speed at which their decline has been reported in Europe (Free and Butler, 1959; Williams, 1986; Rasmont and Mersch, 1988; Williams and Osborne, 2009). Among the array of insects that have shown dramatic declines since then, bumblebees showed an additional characteristic that would allow them to get even more attention: their ability to be domesticated (Velthuis and van Doorn, 2006). The immense economic importance of this management for global agriculture, and later the subsequent use of managed colonies for research purposes rapidly ranked bumblebees higher than ever in our conservation priorities (Cameron and Sadd, 2020; Rasmont et al., 2021). For all these reasons, bumblebees were intensively investigated compared to the rest of wild bees and are therefore usually used as main examples to illustrate the decline of other bee species. Research about their decline is now extensively cited in the scientific literature to illustrate the regression of other native/wild bees (e.g., literature cited in Carvalheiro et al., 2020; Reilly et al., 2020; Kammerer et al., 2021; Zattara and Aizen, 2021) but also of pollinators in general (e.g., in Potts et al., 2010; Sevenello et al., 2020; Dicks et al., 2021), and of even higher taxonomical levels such as insects and arthropods (e.g., in Harvey et al., 2020; Høye, 2020; Wagner, 2020; Halsch et al., 2021). It is not uncommon that works exclusively focusing on bumblebees are cited as references to support statements about threats to wild, native bees (e.g., in Winfree, 2010; Phelps et al., 2018; Vega-Hidalgo et al., 2020) or to pollinators in general (e.g., in Potts et al., 2016; Dicks et al., 2021). However, are bumblebees objectively that convincing as models for wild bee decline?
Bumblebees are Aberrant Bees
Stronger Together?
Displaying a social behavior is more the exception than the rule in bees (around 1,000 social species over the 20,000 known bee species, cf. Michener, 2007; Wcislo and Fewell, 2017). Outside the fundamental aspect of this divergence in a life history trait, the fact that bumblebees fall into this relatively rare category of sociality makes their responses to global changes completely different to those of solitary bees (Schweiger et al., 2010).
Eusocial pollinators such as bumblebees mostly present both more flexible and longer activity periods, extending across several months from the time of nest foundation until sexuals are produced (Goulson, 2003). In contrast, wild solitary bees tend to have much narrower activity windows, with an increased likelihood to suffer from temporal mismatches (Gérard et al., 2020). In Bombus, this long activity period imposed by their sociality implies dietary generalism, a characteristic that allows the colony to benefit from resources all along its lifetime (Goulson, 2003). With few exceptions, bumblebees are not strictly restricted to the consumption of plants from a single species or even genus (Kleijn and Raemakers, 2008; Rasmont et al., 2021). Despite obvious dietary requirements for their survival (Wood et al., 2021b), bumblebees do not fall into the “oligolectic/monolectic” category encountered in many other bee genera (Rasmussen et al., 2020; Cane, 2021). This is a considerable advantage as specialized bees have shown greater range declines and higher sensibility to land use in comparison to more generalist bees (Biesmeijer et al., 2006; Palma et al., 2015). In addition to a certain degree of generalism, larger bees such as bumblebees are expected to cope well with patchy resources requiring longer flight ranges (cf. Greenleaf et al., 2007). This foraging success enables colony growth and enhances food storage, another invaluable buffer against the inclement weather or periods of sparse availability of adequate flowering plants (Wcislo and Fewell, 2017).
Another fundamental privilege given by sociality in a context of climate change is colony homeostasis. In contrast with solitary bees, worker bumblebees keep the ability to take shelter in a thermoregulated nest during unfavorable weather conditions. In a case of heat waves, groups of worker bumblebees use their wings to fan when temperature rises above optimal (Heinrich, 1985; Westhus et al., 2013; Wynants et al., 2021). Alternatively, the endothermic nature of bumblebees can also be helpful to warm up the nests in especially cold periods (Heinrich, 2004). Overall, temperatures in bumblebee nests are carefully regulated to setpoints conducive to optimize larval development, regardless of environmental challenges (Heinrich, 1985, 2004). In solitary bees however, homeostasis remains an individual matter.
Although many other “universal” advantages of sociality exist (e.g., increased defense against predators, brood care, etc), the so-called “superorganism resilience” of eusocial organisms is still not optimal in Bombus. For instance, while the effects of pesticides could be buffered by the high number of workers in social colonies compared to solitary bees, the solitary phase of the bumblebees' life cycle still makes them susceptible to face these chemicals (Straub et al., 2015).
Bear Wasps1 in a Warming World
Understanding the metabolism of bumblebees requires to understand their evolutionary history. It is most likely that bumblebees appeared about 25–40 million years ago in high-altitude regions of Northeast Asia (Hines, 2008). This period coincides with the sharp decline in temperature of the Eocene-Oligocene transition (about 34 million years ago), a period well-known to have caused a major extinction and turnover of organisms, replacing many tropical taxa organisms adapted to cooler climates. Except for a few species, bumblebees still constitute a mostly cold-adapted lineage to day. This trait leads to key difference in their distribution ranges compared to most other bees, as bumblebee diversity worldwide peaks in high altitudes, far from the actual hotspots of solitary bee diversity in Mediterranean areas (Michener, 2007; Orr et al., 2020; Boustani et al., 2021; Wood et al., 2021a) where Bombus are poorly represented (Rasmont et al., 2021). This contrast can be explained by the origin of bees themselves, likely to have radiated from the xeric regions of the highly seasonal interior of the old continent of Gondwanaland (Michener, 2007). Well-beyond being a simple evolutionary difference, the tendency of bumblebees to be cold-adapted now constitutes a special disadvantage in a context of global warming. While their especially hairy body displays interesting metabolic assets to overcome cool to freezing conditions, resisting to heat stress at the individual level is much more arduous. Several studies have shown the dramatic impact of heat stress in bumblebees across multiple subgenera, highlighting their global tendency to be sensitive to the heat (Pimsler et al., 2020; Zambra et al., 2020; Maebe et al., 2021a; Martinet et al., 2021a). Nevertheless, the temperatures tested by these studies are not especially uncommon in areas hosting a large diversity of solitary bees (Baldock et al., 2018; Lhomme et al., 2020). On the contrary, the remarkable resistance of bumblebees to cold climates, including areas where the ground is frozen for much of the year, allows them to take advantage of an ecological niche that is unoccupied by virtually all other Apoid Hymenoptera. Although a few species of solitary bees are also capable of endothermy (Stone, 1990), insects mostly remain classic ectotherms forced to strictly cope with the outside temperatures. This trait has eventually led bumblebees and most wild bees to occupy rather distinct climatic niches that do not respond similarly to the current climate change (IPCC, 2019) and that are not either expected to do so in long-term future (Spinoni et al., 2018), making comparisons of projected population trends under different climate change scenarios arduous.
The Bigger, the Better?
Because heat production is more efficient in larger bees (de Farias-Silva and Freitas, 2021), it is not surprising that cold-adapted animals like bumblebees display a comparatively big body size. In addition to thermoregulation, the fact that larger animals present lower rates of water loss might favor bumblebees against the issue of hydric stress (cf. Gérard et al., 2020; Maebe et al., 2021b). In addition, recent research found bumblebee body size to be correlated to habitat fragmentation, which suggests that larger bees such as bumblebees could be better selected in fragmented landscapes as they are able to reach adequate habitats at a longer distance (Theodorou et al., 2021). Finally, the comparatively large size of bumblebees could make them relatively more resilient to pesticides, as pesticide vulnerability could be negatively correlated with body size in bees (Arena and Sgolastra, 2014).
Despite these advantages, bigger animals are also expected to retain heat more due to their higher volume/surface ratio. As bumblebees become distressed when temperature reaches 35°C (Heinrich, 2004), one could consider their larger body as detrimental as it could cause overheating when foraging in warming environments. In contrast with other wild bees however, bumblebees are generally bigger under warmer climates (Gérard et al., 2018), although substantial intraspecific and species-specific variation in size has been recently demonstrated (Lozier et al., 2021). Importantly, evidence from an analysis involving >30,000 specimens from >430 species suggests that taxa with a larger body size (including bumblebees) have been more likely to decline over a 140-y period (Bartomeus et al., 2013). Overall, more work is needed to disentangle the impacts of body size in bumblebees (Oyen et al., 2021) to assess whether larger species might be favored or not in areas particularly affected by climate warming.
Domesticating an Outlier
The end of the 1980s was marked by an important event in the study of bumblebees: the commercial domestication of the buff-tailed bumblebee Bombus terrestris (Velthuis and van Doorn, 2006). Beyond the purely economic interest of this feat, the availability of colonies of this species on demand has allowed scientists in many countries to thoroughly study its ethology, metabolism and their resilience to different drivers of decline such as heat waves or pesticides. All in all, the domestication of B. terrestris has made it possible to test ex situ the impacts of the Anthropocene on a bee that is different from the honeybee, and with an unprecedented possibility of experimental replication. Recent studies have however highlighted the over-performance of B. terrestris against global stressors (e.g., heat stress) compared to other bumblebees (Zambra et al., 2020; Martinet et al., 2021a,b), making it an exception among bumblebees in the Anthropocene (Ghisbain et al., 2021).
The metabolic and physiological performances of B. terrestris have been mostly discussed in the current context of geographic expansion and invasions in the Anthropocene (Geslin et al., 2017; Aizen et al., 2020; Ghisbain et al., 2021). Very quickly after its domestication began, the buff-tailed bumblebee became invasive in very many areas of the globe where it was originally absent (Rendoll-Carcamo et al., 2017; Aizen et al., 2019; Chandler et al., 2019). This expansion syndrome is relatively uncommon among bumblebees and other bees in the context of global change, being again the exception rather than the rule (Rasmont et al., 2015, 2021; Ghisbain et al., 2021). This pattern of geographic expansion in the buff-tailed bumblebee may be explained by a series of traits whose combination in a single species is relatively exceptional. Bombus terrestris has a large and highly flexible diet (Rasmont et al., 2008), produces larger pupae than other bumblebees even on resources of lower quality (Romain Moerman et al., 2016), copes well with a variable climate and extreme weather events (Zambra et al., 2020), is an early-emerging bumblebee with the ability to be active in winter (Stelzer et al., 2010) and seems to have an inherently high dispersal ability (Rasmont et al., 2015). Instead, numerous other bumblebee species display a more restricted diet (Wood et al., 2019, 2021b), cannot stand pronounced heat waves (Martinet et al., 2021a), are univoltine and tend to decline or remain stable rather than expand (Cameron et al., 2011; Cameron and Sadd, 2020). Overall, this drastic difference between B. terrestris and all other bumblebees is an additional point questioning the relevance of using it as model for other wild, solitary species.
Similar Status for Dissimilar Bees?
The well-established and well-studied patterns of decline experienced by several bumblebees worldwide are very often used as example to illustrate the parallel non-Bombus bee decline, both at local (Drossart et al., 2019) and global scales (Potts et al., 2010, 2016). As a case study, I will focus here on the comparatively well-known European bee fauna, for which assessments of both bumblebee and wild bee population changes have been thoroughly reported (Nieto et al., 2014; Michez et al., 2019; Wood et al., 2020). In the latest European Red List of Bees, ~9% were assessed “threatened” against the IUCN criteria (Nieto et al., 2014). Importantly, more than a half of all European bees could not be assessed due to an insufficient amount of available data, making them fall in the category “Data Deficient.”
Figure 1 shows that regardless of the inclusion or not of the Data Deficient category, bumblebees appear to be especially threatened when separated from all other bees at the continental scale. Although results from Red Lists at national levels and following the same IUCN criteria also highlight the particular sensitivity of bumblebees against global changes (e.g., Drossart et al., 2019; Gogala, 2019; Quaranta et al., 2019), comparisons of that type remain nebulous. Indeed, the “Data Deficient” non-Bombus bees could include a substantial proportion of threatened species, making threatened non-Bombus bee ranging from ~4 to ~60%, respectively, if none or all data deficient were considered as threatened (Nieto et al., 2014). In addition, given that the Red Listing of Nieto et al. (2014) includes all historic data of European bees (including from areas where species have now vanished) and that bumblebee records have always been more represented in the last two centuries, both the EOO (Extent of Occurrence) and AOO (Area of Occupancy) calculations of the latter have benefitted from higher spatial resolution at the continental level. Overall, these fundamental sampling differences emphasize the difficulty to extrapolate trends from bumblebee to wild bee conservation, highlighting the need for an updated and more extensive sampling of the European bee fauna.
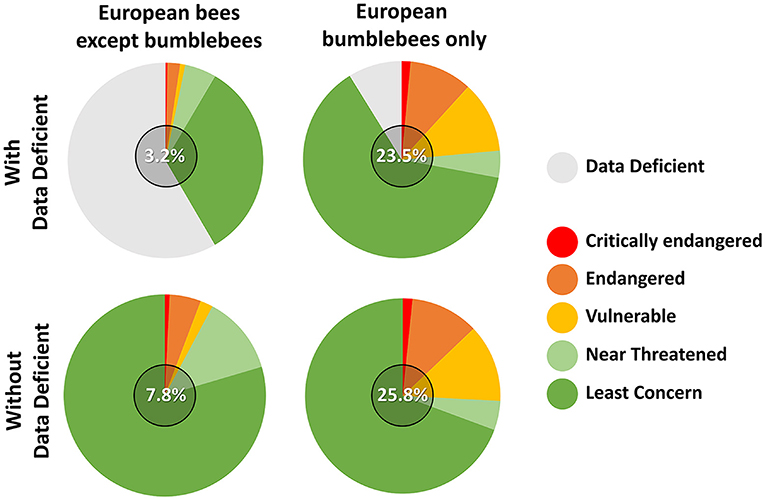
Figure 1. IUCN Red List status of the bees of Europe following Nieto et al. (2014) separating bumblebees from all other bees and including or not bees falling in the “Data Deficient” category. Numbers in the middle of the pie charts correspond to the percentage of threatened species (i.e., vulnerable, endangered and critically endangered).
Imperfect Models, Yet Better Than Nothing
The fact that bumblebees are imperfect models for understanding wild bee conservation does not make them completely irrelevant for the understanding of the basic ecological requirements of wild bees. Research on bumblebees has provided key insight into the importance of maintaining connected habitats with an adequate supply of high-quality resources for all bees (Potts et al., 2016; Drossart and Gérard, 2020). The lessons learned from bumblebees concerning the impacts of biological pollution caused by domesticated organisms must be a warning signal to avoid such repercussions on other bees (Aizen et al., 2019; Bartomeus et al., 2020). The pathogen spillover by domesticated species is, for instance, a significant cause of decline in native bumblebee fauna and another growing concern for other native wild bees (Graystock et al., 2016). Similarly, the impacts of chemical pollution caused by plant protection products, repeatedly tested in bumblebees, should also be a cause for alarm for other bees (Sgolastra et al., 2020). Last but not least, the overall impact of climate change on bumblebees as a driving force leading to species extirpation (Kerr et al., 2015; Rasmont et al., 2015) can be used as a preliminary understanding of how climatic variations could affect the population dynamics of bees.
Conclusion
Although species-specific understanding of population trajectories would be ideal for selecting the bee species that are at greater risk, the paramount diversity of ecological traits (e.g., activity period, specialization in nesting substrate, social level, diet breadth) of wild bees at the global scale forces conservation biologists to use model species as surrogates. However, as for honeybees (Wood et al., 2020), the responses of bumblebees and wild bees to similar threats are generally not comparable. Although many aspects resulting from bumblebee sociality such as colony homeostasis, optimized food intake and broader diet breadth could make them particularly resilient under specific stressors, their overall tendency to be cold-adapted makes them especially disadvantaged in a warming environment. Overall, these results reinforce the need for conservation actions that consider a broader array of ecological diversity in wild bees, which in turn requires additional research into their biological requirements.
Author Contributions
The author confirms being the sole contributor of this work and has approved it for publication.
Conflict of Interest
The author declares that the research was conducted in the absence of any commercial or financial relationships that could be construed as a potential conflict of interest.
Publisher's Note
All claims expressed in this article are solely those of the authors and do not necessarily represent those of their affiliated organizations, or those of the publisher, the editors and the reviewers. Any product that may be evaluated in this article, or claim that may be made by its manufacturer, is not guaranteed or endorsed by the publisher.
Acknowledgments
The author warmly thanks Elif Kardas, Maxence Gérard, Diego Cejas and three reviewers for their constructive comments on the manuscript. The author is a postdoctoral researcher supported by a grant from the F.R.S.-FNRS (Fonds National de la Recherche Scientifique, Belgium).
Footnotes
1. ^“bear wasp” is the literal translation of a widespread Chinese name for bumblebee.
References
Aizen, M.A., Arbetman, M.P., Chacoff, N.P., Chalcoff, V.R., Feinsinger, P., Garibaldi, L.A., et al. (2020). Invasive Bees and Their Impact on Agriculture, 1st Edn. Amsterdam: Elsevier Ltd. doi: 10.1016/bs.aecr.2020.08.001
Aizen, M. A., Smith-Ramírez, C., Morales, C. L., Vieli, L., Sáez, A., Barahona-Segovia, R. M., et al. (2019). Coordinated species importation policies are needed to reduce serious invasions globally: the case of alien bumblebees in South America. J. Appl. Ecol. 56, 100–106. doi: 10.1111/1365-2664.13121
Arena, M., and Sgolastra, F. (2014). A meta-analysis comparing the sensitivity of bees to pesticides. Ecotoxicology 23, 324–334. doi: 10.1007/s10646-014-1190-1
Baldock, D., Wood, T.J., Cross, I., and Smit, J. (2018). The Bees of Portugal. Entomofauna Supplement 221. Munich: Museum Witt
Bartomeus, I., Ascher, J. S., Gibbs, J., Danforth, B. N., Wagner, D. L., Hedtke, S. M., et al. (2013). Historical changes in northeastern US bee pollinators related to shared ecological traits. Proc. Natl. Acad. Sci. 110, 4656–4660. doi: 10.1073/pnas.1218503110
Bartomeus, I., Molina, F. P., Hidalgo-Galiana, A., and Ortego, J. (2020). Safeguarding the genetic integrity of native pollinators requires stronger regulations on commercial lines. Ecol. Solut Evid. 1:e12012. doi: 10.1002/2688-8319.12012
Biesmeijer, J. C., Roberts, S. P. M., Reemer, M., Ohlemüller, R., Edwards, M., Peeters, T., et al. (2006). Parallel declines in pollinators and insect-pollinated plants in Britain and the Netherlands. Science 313, 351–354. doi: 10.1126/science.1127863
Boustani, M., Rasmont, P., Dathe, H. H., Ghisbain, G., Kasparek, M., Michez, D., et al. (2021). The bees of Lebanon (Hymenoptera: Apoidea: Anthophila). Zootaxa 4976, 1–146. doi: 10.11646/zootaxa.4976.1.1
Cameron, S. A., Lozier, J. D., Strange, J. P., Koch, J. B., Cordes, N., Solter, L. F., et al. (2011). Patterns of widespread decline in North American bumble bees. Proc. Natl. Acad. Sci. USA 108, 662–667. doi: 10.1073/pnas.1014743108
Cameron, S. A., and Sadd, B. M. (2020). Global trends in bumble bee health. Annu. Rev. Entomol. 65, 209–232. doi: 10.1146/annurev-ento-011118-111847
Cane, J. H. (2021). A brief review of monolecty in bees and benefits of a broadened definition. Apidologie 52, 17–22. doi: 10.1007/s13592-020-00785-y
Cariveau, D. P., and Winfree, R. (2015). Causes of variation in wild bee responses to anthropogenic drivers. Curr. Opin. Insect Sci. 10, 104–109. doi: 10.1016/j.cois.2015.05.004
Carvalheiro, L. G., Biesmeijer, J. C., Franzén, M., Aguirre-Gutiérrez, J., Garibaldi, L. A., Helm, A., et al. (2020). Soil eutrophication shaped the composition of pollinator assemblages during the past century. Ecography 43, 209–221. doi: 10.1111/ecog.04656
Chandler, D., Cooper, E., and Prince, G. (2019). Are there risks to wild European bumble bees from using commercial stocks of domesticated Bombus terrestris for crop pollination? J. Apic. Res. 58, 665–681. doi: 10.1080/00218839.2019.1637238
de Farias-Silva, F. J., and Freitas, B. M. (2021). Thermoregulation in the large carpenter bee Xylocopa frontalis in the face of climate change in the Neotropics. Apidologie 52, 341–357. doi: 10.1007/s13592-020-00824-8
Dicks, L., Breeze, T., Ngo, H., Senapathi, D., An, J., Aizen, M., et al. (2021). A global assessment of drivers and risks associated with pollinator decline. Nat. Ecol. Evol. 5, 1453–1461. doi: 10.1038/s41559-021-01534-9
Drossart, M., and Gérard, M. (2020). Beyond the decline of wild bees: optimizing conservation measures and bringing together the actors. Insects 11:649. doi: 10.3390/insects11090649
Drossart, M., Rasmont, P., Vanormelingen, P., Dufrêne, M., Folschweiller, M., Pauly, A., et al. (2019). Belgian Red List of Bees. Mons: Presse universitaire de l'Université de Mons.
Ellis, E. C., Gauthier, N., Klein Goldewijk, K., Bliege Bird, R., Boivin, N., Díaz, S., et al. (2021). People have shaped most of terrestrial nature for at least 12,000 years. Proc Natl Acad Sci USA 118:e2023483118. doi: 10.1073/pnas.2023483118
Gérard, M., Vanderplanck, M., Franzen, M., Kuhlmann, M., Potts, S. G., Rasmont, P., et al. (2018). Patterns of size variation in bees at a continental scale: does Bergmann's rule apply? Oikos 127, 1095–1103. doi: 10.1111/oik.05260
Gérard, M., Vanderplanck, M., Wood, T., and Michez, D. (2020). Global warming and plant–pollinator mismatches. Emerg Top. Life Sci. 4, 77-86. doi: 10.1042/ETLS20190139
Geslin, B., Gauzens, B., Baude, M., Dajoz, I., Fontaine, C., Henry, M., et al. (2017). Massively introduced managed species and their consequences for plant–pollinator interactions. Adv. Ecol. Res. 57, 147–199. doi: 10.1016/bs.aecr.2016.10.007
Ghisbain, G., Gérard, M., Wood, T. J., Hines, H. M., and Michez, D. (2021). Expanding insect pollinators in the Anthropocene. Biol Rev. 96, 2755–2770. doi: 10.1111/brv.12777
Ghisbain, G., Michez, D., Marshall, L., Rasmont, P., and Dellicour, S. (2020). Wildlife conservation strategies should incorporate both taxon identity and geographical context - further evidence with bumblebees. Divers. Distrib. 26, 1741–1751. doi: 10.1111/ddi.13155
Gogala, A. (2019). Threatened bee species of Europe in Slovenia/OgroŽene čebele Evrope v Sloveniji. Folia biologica et geologica 59, 21–43. doi: 10.3986/fbg0042
Graystock, P., Blane, E. J., McFrederick, Q. S., Goulson, D., and Hughes, W. O. H. (2016). Do managed bees drive parasite spread and emergence in wild bees? Int. J. Parasitol. Parasites Wildl. 5, 64–75. doi: 10.1016/j.ijppaw.2015.10.001
Greenleaf, S. S., Williams, N. M., Winfree, R., and Kremen, C. (2007). Bee foraging ranges and their relationship to body size. Oecologia 153, 589–596. doi: 10.1007/s00442-007-0752-9
Halsch, C.A., Shapiro, A.M., Foordyce, J.A., Nice, C.C., Thorne, J.H., Waetjen, D.P., et al. (2021). Insects and recent climate change. Proc. Nat. Acad. Sci. 118:e2002543117. doi: 10.1073/pnas.2002543117
Harvey, J.A., Heinen, R., Gols, R., and Thakur, M.P. (2020). Climate change-mediated temperature extremes and insects: from outbreaks to breakdowns. Glob Chang Biol 26, 6685–6701. doi: 10.1111/gcb.15377
Heinrich, B. (1985). “The social physiology of temperature regulation in honeybees” in Experimental Behavioral Ecology and Sociobiology, eds B. Hölldobler and M. Lindauer (Sunderland, MA: Sinauer), 393–406.
Hines, H. M. (2008). Historical biogeography, divergence times, and diversification patterns of bumble bees (Hymenoptera: Apidae: Bombus). Syst. Biol. 57, 58–75. doi: 10.1080/10635150801898912
Høye, T.T. (2020). Arthropods and climate change – arctic challenges and opportunities. Curr. Opin. Insect. Sci. 41, 40–45. doi: 10.1016/j.cois.2020.06.002
IPCC (2019). Climate Change and Land: An IPCC Special Report on Climate Change, Desertification, Land Degradation, Sustainable Land Management, Food Security, and Greenhouse Gas Fluxes in Terrestrial Ecosystems – Summary for Policy Makers. Geneva: IPCC. Available online at: https://bit.ly/2U1gzza (accessed August 10, 2021).
Kammerer, M., Goslee, S.C., Douglas, M.R., Tooker, J.F., and Grozinger, C.M. (2021). Wild bees as winners and losers: relative impacts of landscape composition, quality, and climate. Glob. Change Biol. 27, 1250–1265. doi: 10.1111/gcb.15485
Kennedy, C. M., Oakleaf, J. R., Theobald, D. M., Baruch-Mordo, S., and Kiesecker, J. (2019). Managing the middle: A shift in conservation priorities based on the global human modification gradient. Glob. Change Biol. 25, 811–826. doi: 10.1111/gcb.14549
Kerr, J. T., Pindar, A., Galpern, P., Packer, L., Potts, S. G., Roberts, S. M., et al. (2015). Climate change impacts on bumblebees converge across continents. Science 349, 177–180. doi: 10.1126/science.aaa7031
Kleijn, D., and Raemakers, I. (2008). A retrospective analysis of pollen host plant use by stable and declining bumble bee species. Ecology 89, 1811–1823. doi: 10.1890/07-1275.1
LeBuhn, G., and Vargas Luna, J. (2021). Pollinator decline: what do we know about the drivers of solitary bee declines? Curr. Opin. Insect Sci. 46, 106–111. doi: 10.1016/j.cois.2021.05.004
Lhomme, P., Michez, D., Christmann, S., Scheuchl, E., Abdouni, I. E., Hamroud, L., et al. (2020). The wild bees (Hymenoptera: Apoidea) of Morocco. Zootaxa 4892, 1–159. doi: 10.11646/zootaxa.4892.1.1
Lozier, J. D., Parsons, Z. M., Rachoki, L., Jackson, J. M., Pimsler, M. L., Oyen, K. J., et al. (2021). Divergence in body mass, wing loading, and population structure reveals species-specific and potentially adaptive trait variation across elevations in montane bumble bees. Insect Syst. Divers. 5:3. doi: 10.1093/isd/ixab012
Maebe, K., De Baets, A., Vandamme, P., Vereecken, N. J., Michez, D., and Smagghe, G. (2021a). Impact of intraspecific variation on measurements of thermal tolerance in bumble bees. J. Therm. Biol. 99:103002. doi: 10.1016/j.jtherbio.2021.103002
Maebe, K., Hart, A. F., Marshall, L., Vandamme, P., Vereecken, N. J., Michez, D., et al. (2021b). Bumblebee resilience to climate change, through plastic and adaptive responses. Glob. Change Biol. 27, 4223-4237. doi: 10.1111/gcb.15751
Martinet, B., Dellicour, S., Ghisbain, G., Przybyla, K., Zambra, E., Lecocq, T., et al. (2021a). Global effects of extreme temperatures on wild bumblebees. Conserv. Biol. 35, 1507-1518. doi: 10.1111/cobi.13685
Martinet, B., Zambra, E., Przybyla, K., Lecocq, T., Anselmo, A., Nonclercq, D., et al. (2021b). Mating under climate change: impact of simulated heatwaves on the reproduction of model pollinators. Funct. Ecol. 35, 739–752. doi: 10.1111/1365-2435.13738
Mathiasson, M. E., and Rehan, S. M. (2020). Wild bee declines linked to plant-pollinator network changes and plant species introductions. Insect Conserv. Divers. 13, 595–605. doi: 10.1111/icad.12429
Matias, D. M. S., Leventon, J., Rau, A.-L., Borgemeister, C., and von Wehrden, H. (2017). A review of ecosystem service benefits from wild bees across social contexts. Ambio 46, 456–467. doi: 10.1007/s13280-016-0844-z
Michez, D., Rasmont, P., Terzo, M., and Vereecken, N. (2019). Bees of Europe. Verriéres le Buisson: NAP Editions.
Nieto, A., Roberts, S. P. M., Kemp, J., Rasmont, P., Kuhlmann, M., García Criado, M., et al. (2014). European Red List of Bees. Luxembourg: Publications Office of EU.
Ollerton, J. (2017). Pollinator diversity: distribution, ecological function, and conservation. Annu. Rev. Ecol. Evol. Syst. 48, 353–376. doi: 10.1146/annurev-ecolsys-110316-022919
Orr, M. C., Hughes, A. C., Chesters, D., Pickering, J., Zhu, C.-D., and Ascher, J. S. (2020). Global patterns and drivers of bee distribution. Curr. Biol. 31, 451–458.e4. doi: 10.1016/j.cub.2020.10.053
Oyen, K. J., Jardine, L. E., Parsons, Z. M., Herndon, J. D., Strange, J. P., Lozier, J. D., et al. (2021). Body mass and sex, not local climate, drive differences in chill coma recovery times in common garden reared bumble bees. J. Comp. Physiol. B. 191, 843–854. doi: 10.1007/s00360-021-01385-7
Palma, A. D., Kuhlmann, M., Roberts, S. P. M., Potts, S. G., Börger, L., Hudson, L. N., et al. (2015). Ecological traits affect the sensitivity of bees to land-use pressures in European agricultural landscapes. J. Appl. Ecol. 52, 1567–1577. doi: 10.1111/1365-2664.12524
Phelan, N., Suddaby, D., and Stanley, D. A. (2021). Investigating the ecology of the Great Yellow Bumblebee (Bombus distinguendus) within the wider bumblebee community in North-West Ireland. J. Insect Conserv. 25, 297–310. doi: 10.1007/s10841-021-00299-7
Phelps, J.D., Strang, C.G., Gbylik-Sikorska, M., Sniegocki, T., Posyniak, A., and Sherry, D.F. (2018). Imidacloprid slows the development of preference for rewarding food sources in bumblebees (Bombus impatiens). Ecotoxicology 27, 175–187. doi: 10.1007/s10646-017-1883-3
Pimsler, M. L., Oyen, K. J., Herndon, J. D., Jackson, J. M., Strange, J. P., Dillon, M. E., et al. (2020). Biogeographic parallels in thermal tolerance and gene expression variation under temperature stress in a widespread bumble bee. Sci. Rep. 10:17063. doi: 10.1038/s41598-020-73391-8
Porto, R. G., de Almeida, R. F., Cruz-Neto, O., Tabarelli, M., Viana, B. F., Peres, C. A., et al. (2020). Pollination ecosystem services: a comprehensive review of economic values, research funding and policy actions. Food Sec. 12, 1425–1442. doi: 10.1007/s12571-020-01043-w
Potapov, G. S., Berezin, M. V., Kolosova, Y. S., Kondakov, A. V., Tomilova, A. A., Spitsyn, V. M., et al. (2021). The last refugia for a polar relict pollinator: isolates of Bombus glacialis on Novaya Zemlya and Wrangel Island indicate its broader former range in the Pleistocene. Polar Biol. 44, 1691–1709. doi: 10.1007/s00300-021-02912-6
Potts, S.G., Biesmeijer, J.C., Kremen, C., Neumann, P, Schweiger, O., and Kunin, W. E. (2010). Global pollinator declines: trends, impacts and drivers. Trends Ecol. Evol. 25, 345–353. doi: 10.1016/j.tree.2010.01.007
Potts, S. G., Imperatriz-Fonseca, V., Ngo, H. T., Aizen, M. A., Biesmeijer, J. C., Breeze, T. D., et al. (2016). Safeguarding pollinators and their values to human well-being. Nature 540, 220–229. doi: 10.1038/nature20588
Quaranta, M., Cornalba, M., Biella, P., Comba, M., Battistoni, A., Rondinini, C., et al. (2019). Lista rossa IUCN delle api italiane minacciate (IUCN Red List of Italian threatened bees). Rome: Comitato Italiano IUCN e Ministero dell'Ambiente e della Tutela del Territorio e del Mare.
Rasmont, P., Coppée, A., Michez, D., and De Meulemeester, T. (2008). An overview of the Bombus terrestris (L. 1758) subspecies (Hymenoptera: Apidae). Ann. Soc. Entomol. Fr. (n.s.) 44, 243–250. doi: 10.1080/00379271.2008.10697559
Rasmont, P., Franzen, M., Lecocq, T., Harpke, A., Roberts, S., Biesmeijer, K., et al. (2015). Climatic risk and distribution atlas of European bumblebees. BioRisk 10, 1–236. doi: 10.3897/biorisk.10.4749
Rasmont, P., Ghisbain, G., and Terzo, M. (2021). Bumblebees of Europe and Neighbouring Regions. Verriéres le Buisson: NAP Editions.
Rasmont, P., and Mersch, P. (1988). Première estimation de la dérive faunique chez les bourdons de la Belgique (Hymenoptera: Apidae). Ann. Soc. R. Zool. Belg. 118, 141–147.
Rasmussen, C., Engel, M. S., and Vereecken, N. J. (2020). A primer of host-plant specialization in bees. Emerg. Top. Life Sci. 4, 7–17. doi: 10.1042/ETLS20190118
Reilly, J. R., Artz, D. R., Biddinger, D., Bobiwash, K., Boyle, N. K., Brittain, C., et al. (2020). Crop production in the USA is frequently limited by a lack of pollinators. Proc. R. Soc. B. Biol. Sci. 287:20200922. doi: 10.1098/rspb.2020.0922
Rendoll-Carcamo, J. A., Contador, T. A., Saavedra, L., and Montalva, J. (2017). First record of the invasive bumblebee Bombus terrestris (Hymenoptera: Apidae) on Navarino Island, southern Chile (55°S). J. Melittol. 71, 1–5. doi: 10.17161/jom.v0i71.6520
Romain Moerman, R., Roger, N., De Jonghe, R., Michez, D., and Vanderplanck, M. (2016). Interspecific variation in bumblebee performance on pollen diet: new insights for mitigation strategies. PLoS ONE 11:e0168462. doi: 10.1371/journal.pone.0168462
Schweiger, O., Biesmeijer, J. C., Bommarco, R., Hickler, T., Hulme, P. E., Klotz, S., et al. (2010). Multiple stressors on biotic interactions: how climate change and alien species interact to affect pollination. Biol. Rev. 85, 777–795. doi: 10.1111/j.1469-185X.2010.00125.x
Sevenello, M., Sargent, R.D., and Forrest, J.R.K (2020). Spring wildflower phenology and pollinator activity respond similarly to climatic variation in an eastern hardwood forest. Oecologia 193, 475–488. doi: 10.1007/s00442-020-04670-4
Sgolastra, F., Medrzycki, P., Bortolotti, L., Maini, S., Porrini, C., Simon-Delso, N., et al. (2020). Bees and pesticide regulation: lessons from the neonicotinoid experience. Biol. Conserv. 241:108356. doi: 10.1016/j.biocon.2019.108356
Spinoni, J., Vogt, J. V., Barbosa, P., Dosio, A., McCormick, N., Bigano, A., et al. (2018). Changes of heating and cooling degree-days in Europe from 1981 to 2100. Int. J. Climatol 38, 191–208. doi: 10.1002/joc.5362
Stelzer, R. J., Chittka, L., Carlton, M., and Ings, T. C. (2010). Winter active bumblebees (Bombus terrestris) achieve high foraging rates in urban Britain. PLoS ONE 5:e9559. doi: 10.1371/journal.pone.0009559
Stone, G. N. (1990). Endothermy and thermoregulation in solitary bees (PhD thesis). University of Oxford, Oxford, United Kingdom.
Straub, L., Williams, G. R., Pettis, J., Fries, I., and Neumann, P. (2015). Superorganism resilience: eusociality and susceptibility of ecosystem service providing insects to stressors. Curr. Opin. Insect Sci. 12, 109–112. doi: 10.1016/j.cois.2015.10.010
Theodorou, P., Baltz, L. M., Paxton, R. J., and Soro, A. (2021). Urbanization is associated with shifts in bumblebee body size, with cascading effects on pollination. Evol. Appl. 14, 53–68. doi: 10.1111/eva.13087
Tian, L., Rahman, S. R., Ezray, B. D., Franzini, L., Strange, J. P., Lhomme, P., et al. (2019). A homeotic shift late in development drives mimetic color variation in a bumble bee. Proc. Natl. Acad. Sci. USA 116, 11857–11865. doi: 10.1073/pnas.1900365116
Vega-Hidalgo, A., Añino, Y., krichilsky, E., Smith, A.R., Santos-Murgas, A., and Gálvez, D. (2020). Decline of native bees (Apidae: Euglossa) in a tropical forest of Panama. Apidologie 51, 1038–1050. doi: 10.1007/s13592-020-00781-2
Velthuis, H. H. W., and van Doorn, A. (2006). A century of advances in bumblebee domestication and the economic and environmental aspects of its commercialization for pollination. Apidologie 37, 421–451. doi: 10.1051/apido:2006019
Wagner, D. L. (2020). Insect declines in the Anthropocene. Annu. Rev. Entomol. 65, 457–480. doi: 10.1146/annurev-ento-011019-025151
Wagner, D. L., Grames, E. M., Forister, M. L., Berenbaum, M. R., and Stopak, D. (2021). Insect decline in the Anthropocene: death by a thousand cuts. Proc. Natl. Acad. Sci. USA 118:e2023989118. doi: 10.1073/pnas.2023989118
Wcislo, W., and Fewell, J. H. (2017). “Sociality in Bees” in Comparative Social Evolution, eds D. R. Rubenstein and P. Abbot (Cambridge: Cambridge University Press), 50–83. doi: 10.1017/9781107338319.004
Westhus, C., Kleineidam, C. J., Roces, F., and Weidenmüller, A. (2013). Behavioural plasticity in the fanning response of bumblebee workers: impact of experience and rate of temperature change. Anim. Behav. 85, 27–34. doi: 10.1016/j.anbehav.2012.10.003
Williams, P.H. (1986). Environmental change and the distributions of British bumble bees (Bombus Latr.). Bee World 67, 50–61. doi: 10.1080/0005772X.1986.11098871
Williams, P.H. (1998). An annotated checklist of bumble bees with an analysis of patterns of description (Hymenoptera: Apidae, Bombini). Bull. Nat. Hist. Museum London (Entomology) 67, 79–152.
Williams, P.H., and Jepsen, S. (2021). IUCN Bumblebee Specialist Group Annual Report 2020. London: National History Museum
Williams, P. H., and Osborne, J. L. (2009). Bumblebee vulnerability and conservation world-wide. Apidologie 40, 367–387. doi: 10.1051/apido/2009025
Winfree, R. (2010). The conservation and restoration of wild bees. Ann. N.Y. Acad. Sci. 1195, 169–197. doi: 10.1111/j.1749-6632.2010.05449.x
Wood, T. J., Ghisbain, G., Michez, D., and Praz, C. J. (2021a). Revisions to the faunas of Andrena of the Iberian Peninsula and Morocco with the descriptions of four new species (Hymenoptera: Andrenidae). EJT 758, 147–193. doi: 10.5852/ejt.2021.758.1431
Wood, T. J., Ghisbain, G., Rasmont, P., Kleijn, D., Raemakers, I., Praz, C., et al. (2021b). Global patterns in bumble bee pollen collection show phylogenetic conservation of diet. J. Anim. Ecol. 90, 2421-2430. doi: 10.1111/1365-2656.13553
Wood, T. J., Gibbs, J., Graham, K. K., and Isaacs, R. (2019). Narrow pollen diets are associated with declining Midwestern bumble bee species. Ecology 100:e02697. doi: 10.1002/ecy.2697
Wood, T. J., Michez, D., Paxton, R. J., Drossart, M., Neumann, P., Gérard, M., et al. (2020). Managed honey bees as a radar for wild bee decline? Apidologie 51, 1100–1116. doi: 10.1007/s13592-020-00788-9
Wynants, E., Lenaerts, N., Wäckers, F., and Van Oystaeyen, A. (2021). Thermoregulation dynamics in commercially reared colonies of the bumblebee Bombus terrestris. Physiol. Entomol. 46, 110–118. doi: 10.1111/phen.12350
Zambra, E., Martinet, B., Brasero, N., Michez, D., and Rasmont, P. (2020). Hyperthermic stress resistance of bumblebee males: test case of Belgian species. Apidologie 51, 911-920. doi: 10.1007/s13592-020-00771-4
Keywords: bees, bumblebees, Bombus, conservation, decline, global change, resilience
Citation: Ghisbain G (2021) Are Bumblebees Relevant Models for Understanding Wild Bee Decline? Front. Conserv. Sci. 2:752213. doi: 10.3389/fcosc.2021.752213
Received: 02 August 2021; Accepted: 03 November 2021;
Published: 07 December 2021.
Edited by:
Oded Berger-Tal, Ben-Gurion University of the Negev, IsraelReviewed by:
VP Uniyal, Wildlife Institute of India, IndiaMarina Paula Arbetman, National University of Comahue, Argentina
Jonathan Berenguer Koch, University of Hawaii at Hilo, United States
Copyright © 2021 Ghisbain. This is an open-access article distributed under the terms of the Creative Commons Attribution License (CC BY). The use, distribution or reproduction in other forums is permitted, provided the original author(s) and the copyright owner(s) are credited and that the original publication in this journal is cited, in accordance with accepted academic practice. No use, distribution or reproduction is permitted which does not comply with these terms.
*Correspondence: Guillaume Ghisbain, Z3VpbGxhdW1lLmdoaXNiYWluJiN4MDAwNDA7dW1vbnMuYWMuYmU=