- 1Behavioural Ecology Group, Wageningen University and Research, Wageningen, Netherlands
- 2Animal Ecology Group, Utrecht University, Utrecht, Netherlands
- 3Centre for Planetary Health and Food Security, Griffith University, Nathan, QLD, Australia
- 4Department of Biological Sciences, University of Alberta, Edmonton, AB, Canada
- 5Instituto de Investigación en Recursos Cinegéticos, CSIC-UCLM-JCCM, Ciudad Real, Spain
Modern wildlife management has dual mandates to reduce human-wildlife conflict (HWC) for burgeoning populations of people while supporting conservation of biodiversity and the ecosystem functions it affords. These opposing goals can sometimes be achieved with non-lethal intervention tools that promote coexistence between people and wildlife. One such tool is conditioned taste aversion (CTA), the application of an evolutionary relevant learning paradigm in which an animal associates a transitory illness to the taste, odor or other characteristic of a particular food item, resulting in a long-term change in its perception of palatability. Despite extensive support for the power of CTA in laboratory studies, field studies have exhibited mixed results, which erodes manager confidence in using this tool. Here we review the literature on CTA in the context of wildlife conservation and management and discuss how success could be increased with more use of learning theory related to CTA, particularly selective association, stimulus salience, stimulus generalization, and extinction of behavior. We apply learning theory to the chronological stages of CTA application in the field and illustrate them by synthesizing and reviewing past applications of CTA in HWC situations. Specifically, we discuss (1) when CTA is suitable, (2) how aversion can be most effectively (and safely) established, (3) how generalization of aversion from treated to untreated food can be stimulated and (4) how extinction of aversion can be avoided. For each question, we offer specific implementation suggestions and methods for achieving them, which we summarize in a decision-support table that might be used by managers to guide their use of CTA across a range of contexts. Additionally, we highlight promising ideas that may further improve the effectiveness of CTA field applications in the future. With this review, we aspire to demonstrate the diverse past applications of CTA as a non-lethal tool in wildlife management and conservation and facilitate greater application and efficacy in the future.
Introduction
The growing prevalence of human-wildlife conflicts is of increasing conservation concern and has invited comprehensive study of intervention methods (Conover, 2002; Eklund et al., 2017; van Eeden et al., 2018) with increasing emphasis on less invasive and non-lethal techniques (e.g., Smith et al., 2000; Shivik et al., 2003; McManus et al., 2015). One promising intervention type for mitigating conflict non-lethally is animal conditioning, which exploits the capacity for animals to learn via association. Associative learning is widespread in the animal kingdom because it enables animals to change their behavior within their lifetime, giving animals the potential to rapidly adapt to changes in the environment (Domjan, 2018).
Traditionally, learning theorists have distinguished between two main forms of associative learning; classical (or Pavlovian) and operant (or instrumental; Domjan, 2018) and both have been applied to wildlife management. In classical conditioning, animals learn via innate or primitive mechanisms to associate a biologically relevant stimulus (the unconditioned stimulus) with another stimulus (the conditioned stimulus) that consistently precedes or accompanies it. Under this paradigm, animals can change their behavior as a result of the presence of these stimuli, but this changed behavior does not in turn influence the stimuli they receive. In contrast, in operant conditioning, an animal's behavior does influence the presence of the unconditioned stimulus, i.e., animals have some voluntary control over their subsequent experience. In either type of conditioning, unconditioned stimuli can be presented (positive) or removed (negative) with conditions that animals naturally seek (attractive) or avoid (aversive) (Domjan, 2018). Applications of conditioning for the management of human-wildlife conflict are typically aversive, and involve one or more unconditioned stimuli that animals predictably avoid, such as pain, fear, or illness. As examples of management interventions based on associative learning, “chases” (rapid approaches) were used to invoke fear and teach elk (Cervus canadensis) greater wariness toward people (Kloppers et al., 2005) and rubber bullets were used to cause pain and fear in an attempt to teach black bears (Ursus americanus) to avoid human-use areas (Mazur, 2010). Associative learning has also been used when managers expose animals to a chemical added to a particular food or prey to induce nausea and so generate an association between the taste of the food, and an unconditioned stimulus (nausea) they would naturally avoid (Cowan et al., 2000). This particular form of classical conditioning is most commonly known as conditioned taste aversion (Garcia et al., 1974). An illustrative example of application of this technique in wildlife management was its use to teach black bears (Ursus americanus) not to eat pre-packaged military rations (Ternent and Garshelis, 1999).
Conditioned taste aversion (hereafter CTA) is achieved with associations that form in the brain stem, which makes it a particularly ancient and robust form of learning (Gaston, 1978). Observations that illustrate CTA were first described over a century ago (Schaeffer, 1911) and it became a field of study in the 1950's when CTA occurred inadvertently in the context of rodent control. Researchers discovered that rats (Rattus sp.) learned to avoid baits after ingesting a sub-lethal dose of the poison they contained for lethal population control (Elton, 1954), identifying the mechanism of a phenomenon that had already been described as “bait-shyness” (Rzóska, 1953). A contemporaneous laboratory study by Garcia et al. (1955) described similar aversion by Norway rats (Rattus norvegicus) to saccharin water after it was paired with exposure to nausea-causing radiation. The power of this learned aversion via classical conditioning was emphasized by the pre-conditioning preference Norway rats exhibited for saccharin water, relative to plain tap water (Garcia et al., 1955). Subsequent studies of CTA, or bait-shyness, revealed a widespread evolutionary predisposition of animals to learn quickly which food items in their environment make them sick and, thus, could be lethal with sufficient exposure (Rozin and Kalat, 1971; Garcia et al., 1974). The strong selection pressure to avoid fatal poisoning is the reason CTA can occur even when an animal is unconscious (Roll and Smith, 1972) and supports learning with only one or very few trials (Garcia et al., 1974). Conditioned taste aversion requires nausea, commonly followed by a gaping response, which makes it a distinct process from conditioned taste avoidance, which does not require nausea and gaping (Parker, 2003; Schier et al., 2019).
Intentional use of CTA to produce aversions in the context of wildlife management began in the 1970's (Figure 1), primarily by adding illness-inducing substances to conflict food. In a series of pioneering studies, Gustavson et al. (1974, 1976, 1982) used lithium chloride to induce nausea in coyotes (Canis latrans) and wolves (Canis lupus) after they had eaten from meat baits and animal carcasses in an attempt to reduce livestock depredation. Similarly, American crows (Corvus americanus) learned to avoid green-painted eggs after being subjected to some containing a non-lethal toxin called landrin (Nicolaus et al., 1983). Despite the promise of these early studies to use CTA in mitigation of predation, other studies failed to induce CTA and some workers identified flaws in methodology and data interpretation (Bekoff, 1975; Sterner and Shumake, 1978; Conover et al., 1979; Burns and Connolly, 1985; Wade, 1985). Animals sometimes failed to generalize among contexts or were able to detect and avoid only the treated baits. Moreover, carnivorous species might continue to kill domestic animals even if they did not consume them. These disparities initiated a decades-long debate about the efficacy of this technique and casual conversations by the authors of this review with wildlife managers suggested that these limitations contributed to a lasting culture of dismissing CTA as relevant tool. Despite these misgivings, researchers have continued to explore the use of CTA in a variety of contexts with a few studies published in most years (Figure 1), mostly on mammals and birds, but also on reptiles. More recent studies of CTA for wildlife management emphasize the contexts of successes (e.g., Baker et al., 2008; O'Donnell et al., 2010; Tobajas et al., 2020a; Ferguson et al., 2021) and the limitations that produce failures (e.g., Catry and Granadeiro, 2006; Neves et al., 2006). Lacking information about the correlates of success may unnecessarily remove a valuable non-lethal tool in the toolbox for promoting human-wildlife coexistence (Madden, 2004). There are no recent comprehensive reviews of the CTA literature to guide their use and facilitate more effective application in wildlife management.
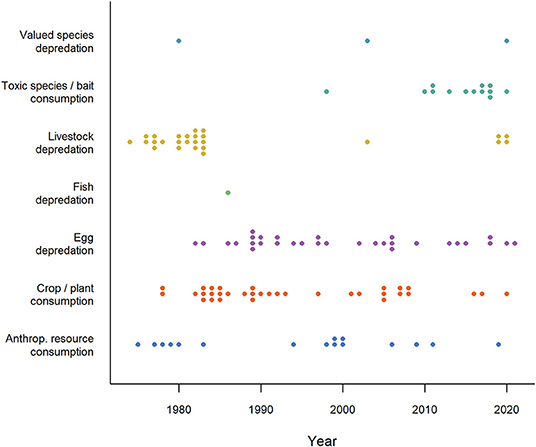
Figure 1. Timeline of publications (N = 124) investigating the application of conditioned taste aversion in seven human-wildlife contexts (1974-2021). The category “Valued species depredation” comprises depredation of species that do not fit in the other depredation categories (e.g., game, pets and protected species), “Anthropogenic resource consumption” comprises resources that do not fit the other HWC categories (e.g., beehives, landfills, food hand-outs). Numbers reported in this figure should be regarded as minimum estimates. Studies started in the 70's primarily focusing on livestock depredation, human resource use (e.g., beehives) and crop consumption, continued with egg depredation in the 80's and toxic species and bait consumption since the 90's. Note that time lags for search engines may have caused some recent studies (2020-2021) to be omitted. Studies were retrieved via systematic search and ad hoc retrievals (see Methodology).
The purpose of this paper is to gather and categorize the relevant English language literature to explore the distinct properties of CTA and the particular challenges that come with applying it to wild animals in the field, consequently facilitating a greater application and efficacy of CTA in human-wildlife conflict (hereafter HWC) mitigation. We were especially interested in aspects of learning theory underlying CTA, such as selective association, stimulus salience, stimulus generalization and extinction of behavior, that are likely to increase chances of success in applying CTA to HWC situations. These principles have not been systematically evaluated or reviewed in this context recently, although Greggor et al. (2020) mention these topics in their broader review of the role of cognition in explaining conservation successes and failures. In the following sections, we highlight the most relevant learning theory along a chronological description of the typical stages of CTA application in HWC (Figure 2). For each stage, we describe associated concepts, identify essential procedural aspects (e.g., safety), illustrate principles with empirical examples, and quantify relevant CTA studies conducted in the context of HWC. We start with describing how learning theory can be used to predict whether CTA is likely to be effective in a given situation (Stage 1: In which situations is CTA suitable?). Assuming CTA is suitable to the situation, we follow by describing how learning theory can guide the application of CTA (Stage 2: How should CTA be applied?), relating specifically to substance use and presentation. Because successful CTA in HWC requires that aversion to treated food is also generalized to untreated “conflict” resources, we devote the next section to the circumstances that stimulate or hamper such generalization (Stage 3: How can generalization of CTA to untreated resources be encouraged?). Lastly and importantly, we discuss the factors that affect if and how quickly a learned and generalized aversion is likely to go extinct (Stage 4: How can extinction of the aversion be delayed?). Before describing the four typical stages in CTA, we give a brief overview of the current literature base on CTA application in HWC. We categorize that literature and draw extensively from it to illustrate the stages of CTA. We give some indications of success, as reported by the respective authors of the included publications, but these should be interpreted cautiously as generalization, given the likely publication bias (most publications report at least partial success), frequent small sample sizes, potential confounding variables and the various definitions of success used by the authors. By presenting the literature base and illustrating the principles that are likely to contribute to success, we hope to position others to explore and examine these patterns of success and failure more extensively and objectively, particularly with the contexts, emetic compounds, and species that have attracted substantial past study.

Figure 2. Review outline. This review addresses, in chronological order, the key questions that arise when considering and applying conditioned taste aversion (CTA) to human-wildlife conflict (HWC) situations and highlights which aspects of learning theory and animal safety are especially relevant.
Methodology: How We Compiled the Relevant Literature
We collected published studies on CTA application in HWC by conducting a systematic search, using Web of Science and Scopus (September 2020), and supplemented this with literature retrieved “ad hoc” through Google Scholar, reference lists in other papers, and expert suggestions. Systematic search articles were retrieved from Web of Science (all databases) and Scopus using specified English-language search strings (Supplementary Tables 1, 2). These search strings led to 154 and 73 search hits in Web of Science and Scopus, respectively, and we collected a total of 53 publications ad hoc. We evaluated titles, abstracts, and when those were inconclusive, full texts for article relevance. We retained publications when they adhered to the following criteria: (1) the study aimed to induce CTA in non-human animals using an illness-inducing substance; (2) the study specifically mentioned potential to inform the use of CTA as a non-lethal tool in a HWC or other wildlife (conservation) management context (including humane rodent control); and (3) the study included a form of post-treatment monitoring to ensure the testing of conditioning rather than repellency alone. This final criterion required that authors measured the response of treated individuals exposed to the conflict resource when the illness-inducing substance was not present. We defined HWC broadly as the contexts in which wildlife threatens the security, livelihood, or interests of people, which can include the protection of endangered species. Studies conducted in the field as well as in captivity were included because both can provide valuable insights into future application in the field. These inclusion criteria, generated a total of 124 unique publications (Figure 1), with 82 from Web of Science and Scopus and 42 from ad hoc sources (104 journal articles, seven conference papers, four MSc theses, one PhD thesis and eight “other” publications such as reports).
We divided these 124 publications among experiments as described by the authors of the original publications or in relation to separate “experiments” we identified via the criteria pertinent to our review. These criteria included multiple unique subject species, food resources (including different tastes but no other stimuli), unique illness-inducing substances, substance application methods (resource or subject), and/or food resource novelty. Application of author or our own criteria resulted in a total of 256 experiments from which we extracted meta-data including both publication and experiment characteristics (Supplementary Data 1). Relevant meta-data included: DOI, source (systematic search or ad hoc retrieval), country of study, species, HWC category (e.g., livestock predation), lab or field experiment, number of individuals, study design, the (food) resource used for conditioning, the illness-inducing substance, substance application method, novelty of the (food) resource, and the outcome measure. We synthesized these meta-data in a spreadsheet that can be sorted and manipulated by others (Supplementary Data 1). For seven of the 124 relevant publications (comprising at least nine experiments), the full-text could not be retrieved and thus not all the meta-data could be extracted (e.g., Forthman et al., 2005). Figures were created using the “ggplot2” (Wickham, 2016) and “beeswarm” (Eklund, 2021) packages in R (R Core Team, 2020) unless noted otherwise. We do not claim our overview to be complete or entirely without bias, yet we do believe it represents the vast majority of HWC-related CTA studies conducted (and published in English) to date and that it will give us a valuable insight into how, when and where CTA has been applied to address HWC. It may also serve as a starting point for future meta-analyses on standardized subsets of the literature.
Overview of the Current Literature Base ON CTA Applications in HWC
In decreasing order of frequency, applications of CTA for HWC include the mitigation of crop/plant consumption (e.g., crop-raiding, grazing of lawns), egg depredation (including those of protected species), livestock depredation, anthropogenic resource consumption (e.g., beehives and hand-outs, excluding the previous categories), toxic species/bait consumption (e.g., cane toad consumption), valued species depredation (e.g., protected species, pets and game, excluding the previous categories) and, lastly, fish depredation with, notably, just one study (Supplementary Data 1). The majority of studies we found addressed HWC in carnivores (e.g., coyote, Canis latrans), followed by songbirds (e.g., red-winged blackbird, Agelaius phoeniceus) (Table 1). CTA in a HWC context has been studied in at least 11 different orders (Figure 3), comprising 26 families and 56 unique (sub)species (Table 1). Most studies we retrieved were conducted in USA, UK, Australia and Canada, but several recent studies occurred in Spain and Portugal, with a total of at least 14 countries having trialed the method (Table 1; Supplementary Figure 1). At least 26 different illness-inducing substances have been tested (Table 1; Supplementary Figure 2) and we go in more detail on this topic in Stage 2 of this review. However, sample sizes were frequently small (i.e., up to 10 individuals) or, particularly in field studies, were not precisely known (Table 1; Figure 4), with the exception of studies in the context of crop/plant consumption and toxic species/bait consumption (Table 1). A majority of the studies were conducted in captive settings (Figure 4), but with expected relevance to the field situations where HWC occurs.
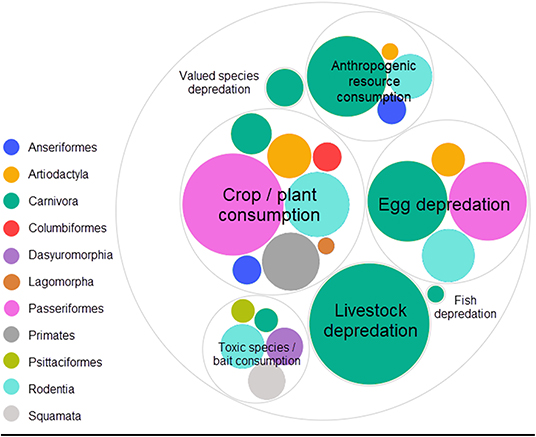
Figure 3. Relative number of experiments per taxonomic order categorized by human-wildlife conflict category. The eleven orders belonged to three classes (birds, mammals and reptiles). One experiment is defined as a unique species, food, novelty and/or substance (application) combination. One publication can thus comprise several experiments. Valued species depredation' comprises depredation of species that do not fit in the other depredation categories (e.g., game, pets and protected species), “Anthropogenic resource consumption” comprises resources that do not fit the other HWC categories (e.g., beehives, landfills, food hand-outs). Studies were retrieved via systematic search and ad hoc retrievals (see Methodology). Figure was created using RAW Graphs 2.0 beta (Mauri et al., 2017) and further edited in Snagit Editor Version 2020.1.3 (TechSmith Corp., Okemos, USA).
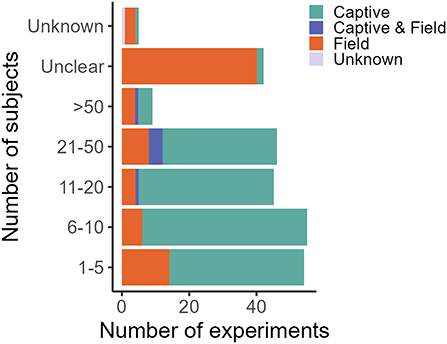
Figure 4. Number of subjects in captive and field experiments. One experiment is defined as a unique species, food, novelty and/or substance (application) combination. One publication can thus comprise several experiments. Sample size in field studies compared to captive studies is disproportionally often “unclear” due to lack of individual-based data. The classification of “unknown” was given to studies for which the information could not be accessed. Studies were retrieved via systematic search and ad hoc retrievals (see Methodology).
This overview reveals substantial biases in the HWC contexts, geographic locations, and study species that limit the generality of the principles we describe below. For example, application of CTA with crop species has been trialed frequently (Table 1; Figure 1), but may not generalize to plant species that are to be protected for their cultural value or because they are at risk of extinction. Also, note that searches were only conducted in English, creating a bias for English-speaking countries in our overview, yet substantial relevant work may exist in many other languages and regions, especially South America, eastern Europe, and Asia. Nevertheless, even among English-speaking countries there appears substantial variation in the number of HWC related CTA studies published that does not necessarily correlate with the frequency or severity of HWC or human population density. For example, the USA accounted for more than half of all studies published, despite a moderate human population density. Instead, the prevalence of studies in USA likely relates to research traditions, stemming from the emphasis there on operant conditioning (Domjan, 2018). All but one of the studies conducted between 1974 and 1983 occurred in North America (e.g., Gustavson et al., 1974; Figure 1) or by American researchers in Australia (Gustavson et al., 1983; Supplementary Data 1). The single study conducted seemingly independently occurred in Japan (Matsuzawa et al., 1983). Nevertheless, other influences, such as intercountry variation in academic resources and productivity cannot be ruled out. There is a similarly strong bias toward terrestrial species; studies almost exclusively focused on terrestrial species and contexts, with only one study in our literature overview focusing on a semiaquatic species, sea lions (Zalophus californianis), which was conducted in captivity (Kuljis, 1986). A majority were also generalist foragers, which may generally be more suited to CTA than specialist foragers. Revealing these biases in the literature base may assist other researchers to investigate species, contexts, and locations where CTA could be applicable, but has not yet been investigated. For example, using CTA to reduce conflicts with semi-aquatic species has been used only rarely, but may potentially have more widespread application.
Stage 1: in Which Situations is CTA (Most) Suitable?
Selective Association
Effective application of associative learning requires the unconditioned stimulus to be evolutionarily relevant and the combination with the conditioned stimulus to be biologically meaningful (Domjan, 2018). Unconditioned aversive stimuli, like illness, pain and fear are universal indicators of danger. The animals that are alive today are the descendants of the animals that effectively learned to identify and subsequently avoid those dangers. There has thus been strong selection pressure on animals' ability to remember the characteristics (conditioned stimuli, CS) of those things that generate illness, pain or fear (unconditioned stimuli, US). This principle is the reason that some CS-US combinations form associations more readily with particular sensory modalities than other combinations. For example, research on Norway rats decades ago demonstrated their inability to associate audio-visual stimuli with nausea, while they were able to rapidly associate taste stimuli with nausea (Garcia and Koelling, 1966). In contrast, the rats associated audio-visual stimuli, but not taste stimuli, with pain (Garcia and Koelling, 1966). This essential limitation of selective association is sometimes overlooked by wildlife managers who might attempt to use illness to teach animals to avoid a particular location or to punish mammals with pain for consumption of a particular food.
Stimulus Salience
Salience of the conditioned stimulus (i.e., overt food characteristic) plays a crucial role in effective conditioning. A stimulus can be referred to as salient when it is noticeable (e.g., prominent, conspicuous) in comparison to its surroundings. Conditioned stimuli that are paired with illness are most likely to be noticeable when they relate to the sensory modality that the species primarily uses to find food. In CTA, the unconditioned stimulus is always illness, but the most relevant conditioned stimulus for the animal may, thus, not always be taste. Foraging activates several sensory modalities in animals, including visual, gustatory, and olfactory systems (Gustavson and Nicolaus, 1987). For most mammals, taste and smell are, indeed, the primary characteristics that individuals use to identify a particular food. However, for birds, poorly developed olfactory lobes and excellent visual acuity makes vision the main modality for identifying food. This sensory capacity makes visual characteristics the most relevant conditioned stimuli for birds to associate food with subsequent nausea (Wilcoxon et al., 1971; Brett et al., 1976). Thus, in certain applications of CTA, the conflict food will need to have distinct visual characteristics, or supplementary odor or visual stimuli will need to be added, as so to create a conditioned “food” aversion (CFA), rather than conditioned “taste” aversion, and we return to this topic below (Stage 3).
Next to sensory capacity, stimulus salience can be influenced by the intensity of the conditioned stimulus (e.g., strong taste of the food), but also novelty (Foley et al., 2014). Novelty often draws the attention of the animal and can thereby affect whether a food characteristic will be linked to the illness. Generally, novel relationships between unfamiliar stimuli and their consequences are the most quickly learned (Rescorla and Wagner, 1972).
Exposure Experience
Establishing CTA is more challenging when animals have previously and repeatedly consumed a certain food without getting sick (Lubow, 1989; Delacasa and Lubow, 1995). This is because it takes longer for a familiar stimulus (e.g., a certain food characteristic) to acquire meaning (e.g., serve as a signal for illness) than a new stimulus, a concept that is referred to as latent inhibition (Lubow, 1973). Indeed, rats that were exposed to both a novel and a known food, followed by an induced illness, more readily associated the novel food to the experienced sickness (Revusky and Bedarf, 1967). As a consequence, animals with benign pre-exposure to foods, are likely to require more trials to effectively establish CTA (e.g., Maguire et al., 2009). Studies in which animals were purposely pre-exposed to the food to obtain a baseline level of consumption (e.g., Baker et al., 2008; Tobajas et al., 2020a) may thus only provide a conservative estimate of the effectiveness of CTA. In our review, 56% of experiments involved subjects that were (made) familiar with the food and only 23% involved a completely novel food resource (Table 1). Rates of novelty in the experimental food were much higher in experiments inducing CTA against toxic prey or baits (86%), most likely due to the lethal consequences of pre-exposure in this conflict category. When considering only studies that were entirely executed in the field, only one out of 79 experiments involved an (assumingly) completely novel food resource (Supplementary Data 1). Lack of novelty in the field was a consequence of pre-existing conflict, a before-after study design or both. Authors of field experiments that clearly involved pre-existing conflict reported (partial) success in establishing aversion in 58% of the cases, while field experiments that clearly did not involve pre-existing conflict (only ten), reported (partial) success in 90% of cases (Supplementary Table 2).
Pre-exposure to the food can sometimes be reduced to promote a stronger association with illness by, for example, targeting young animals, conditioning individuals before reintroduction of animals to a novel environment, planning conditioning just before the presence of seasonal foods, or by adding novel stimuli to the conditioned foods. In cases of crop-raiding or egg depredation, it could be beneficial to start experiments immediately before the onset of the growing or breeding season (Tobajas et al., 2020a). This timing could make the food relatively new to older generations, and entirely new to yearlings. At the same time, this would also prevent any potentially adverse effects of CTA on breeding animals caused by predators being attracted to the artificial increase in food resources [but see Ferguson et al. (2021) for an innovative way to reduce such attraction]. Only in 53% of 19 field experiments that clearly involved pre-existing seasonal conflict, CTA appeared to be (partially) conducted outside the conflict season. Of these studies, two-thirds reported a (partial) success, while field experiments conducted during the season of conflict most often reported failure (Supplementary Data 1). Another option is to artificially increase novelty by adding a new stimulus to a familiar, conditioned stimulus, such as a visual or odor stimulus (Baker et al., 2007, 2008; Tobajas et al., 2021a). For example, domestic chicks avoided a particular taste sooner when a novel color or odor stimulus was added to quinine-flavored water (Roper and Marples, 1997). Adding novel stimuli to enhance recognition of foods targeted with CTA training is only useful for management when the food items that the animal will subsequently encounter also contain this novel stimulus. This is typically easier to achieve when humans partially control the presentation of food items, such as in agriculture, certain protected breeding populations, or baiting projects. This may explain why, thus far, supporting stimuli have been added only in <25% of field experiments and mostly with the aim of facilitating generalization (see Stage 3) rather than increasing novelty (Supplementary Data 1).
Stage 2: How Should CTA be Applied?
Stimulus Intensity
Generally, a more powerful or intense unconditioned stimulus (e.g., illness) creates a stronger conditioned response (Annau and Kamin, 1961; Smith, 1968). CTA relies on causing gastrointestinal distress in the animal and most substances considered for use in CTA are selected based on their property to effectively cause nausea and vomiting (Massei and Cowan, 2002; Massei et al., 2003; Tobajas et al., 2019a). Of the over twenty substances applied, lithium chloride, methiocarb, thiabendazole, ethinyl estradiol, carbachol and levamisole have been used most often (Figure 5), with thiabendazole most frequently in the last 10 years (Table 1). The substances used in the last 10 years (since 2011) that showed promising results are listed in Table 2, along with the associated species, conflict context, food types, field or captive setting, and references. An important consideration in field applications is environmental stability of the substance. If the treated food is deployed too long before being consumed by target animals, the substance might break down and lose potency, consequently failing to induce CTA. Presenting the substance in gelatin capsules may help delay break down (e.g., Cornell and Cornely, 1979).
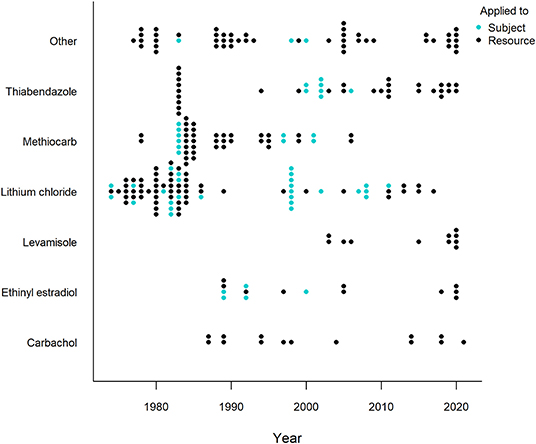
Figure 5. Timeline of the six most frequently applied illness-inducing substances in human-wildlife conflict context (1974-2021). All other substances are grouped in “Other” and represents the (at least) 20 other substances/substance combinations used. Each dot represents an experiment, with one experiment defined as a unique species, food, novelty and/or substance (application) combination. One publication can thus comprise several experiments. Different colors refer to how the substance was administered, either directly applied to the subject (e.g., injected; blue) or applied to the food resource (black). Studies were retrieved via systematic search and ad hoc retrievals (see Methodology).
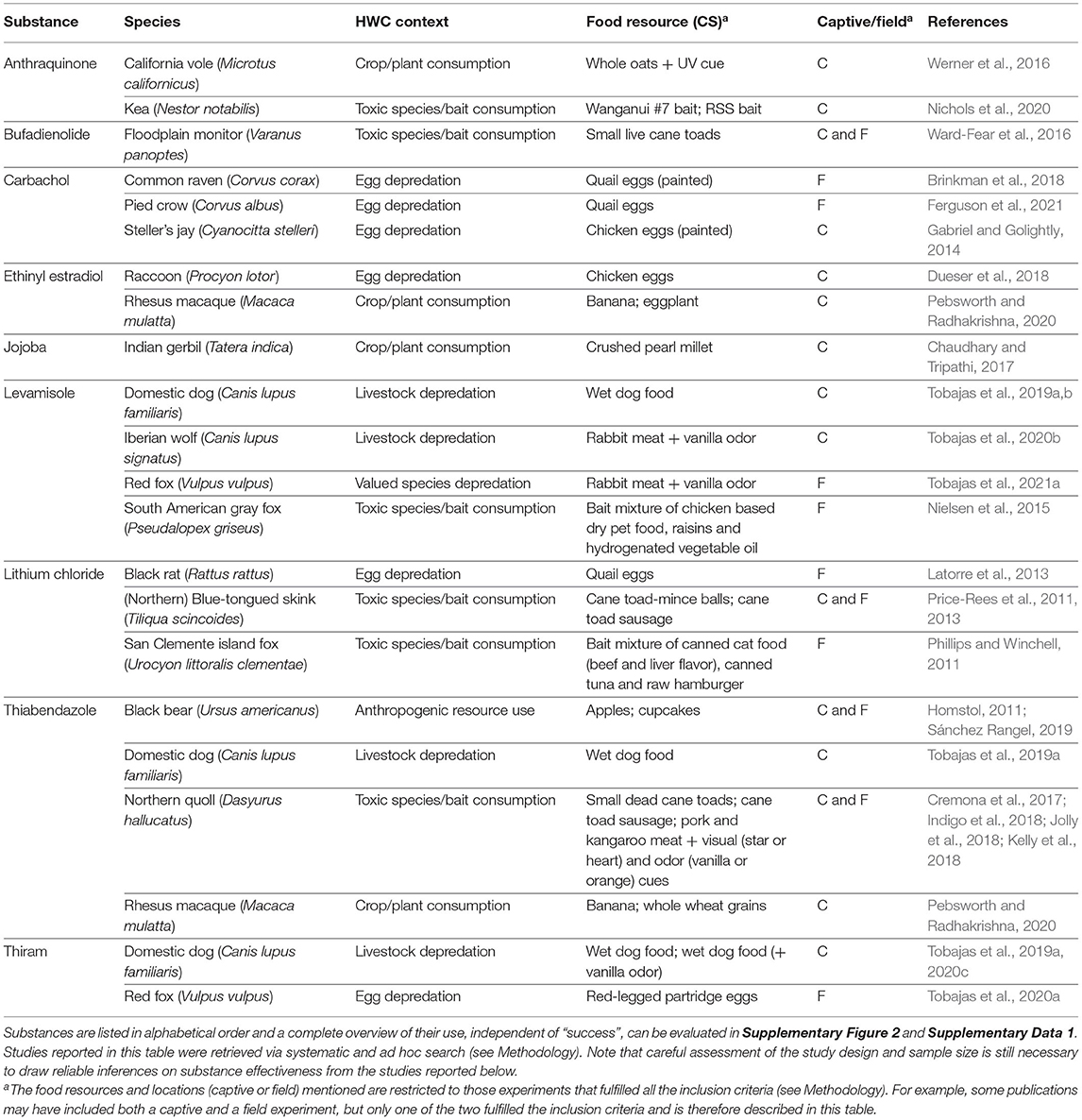
Table 2. Examples of emetics used in the last 10 years that were reported to, in some degree, successfully (as defined by the authors of the respective studies) induce CTA to a conditioned stimulus (CS) in a current or prospective future wildlife conservation or management context.
Next to the substance type, dosage typically determines stimulus intensity. From an evolutionary perspective, it is expected that effective substances can cause CTA at low dosages (Lin et al., 2017), because larger doses would be lethal. While low dosages of lithium chloride were effective at reducing subsequent food intake, the CTA was shown to be stronger when the substance dosage was higher (Nachman and Ashe, 1973; Garcia et al., 1974; du Toit et al., 1991). Although it may seem like a safe option to start with a low dose and increase it when the dose does not appear effective, increasing the intensity of an aversive stimulus during the experiment (e.g., Dorrance and Roy, 1978; Neves et al., 2006), generally does not work as well as starting out with a high intensity stimulus at the onset (Domjan, 2018). Working with large doses in the wild is challenging, however, because experimenters cannot necessarily control how much treated bait an individual ingests. The efficacy of CTA can be reduced when an individual ingests too much bait and becomes incapacitated or, if it vomits too quickly, preventing it from ingesting or digesting enough substance to generate an association with nausea (Conover et al., 1977). Higher doses also increase the detectability of the emetic substance in species with a keen sense of smell (e.g., Cagnacci et al., 2005). Preventing target animals from detecting the treatment compound is essential for generalization of a CTA to the food that is to be protected and we return to this point in Stage 3 below. Only 10% of field experiments explicitly reported on having observed signs of nausea (Supplementary Data 1). Without post-ingestion monitoring, it is almost impossible to rule out the use of a wrong dosage when there has been a failure to generate aversion to the conflict food even though other factors may have contributed more to that outcome.
Stimulus Interval
For the conditioned stimulus (food characteristic) and unconditioned stimulus (illness) to become associated, they have to be related via learning in both timing and information. Even though other conditioning methods require the time interval to be on the scale of seconds or minutes (Domjan, 2018), the time interval between the conditioned and unconditioned stimulus in CTA applications can be as long as tens of minutes to several hours (Garcia et al., 1966; Cowan et al., 2000; Domjan, 2018). CTA formation becomes significantly less robust at short intervals of several seconds compared to longer intervals of 15-30 minutes (Schafe et al., 1995) and an interval of 0 (i.e., simultaneous presentation) has proven ineffective in many cases (Domjan, 2018). From an evolutionary perspective, it is logical that CTA requires a standard passage of time for ingestion and digestion to induce nausea. Within this natural time-interval range, ideal timing might often be between 30 minutes and 2 hours (Tobajas et al., 2019a). Longer intervals can cause the retention of some of the specific characteristics of the food to be lost or interfere with other similar stimuli (Land et al., 1998). A fast working substance will, thus, stimulate retention of the correct conditioned stimulus by lowering the chance that an individual eats another food in the meantime. Moreover, a quick onset of nausea will minimize the chance of an individual ingesting dangerous amounts of the substance.
Stimulus Repetition
The reliability with which a conditioned stimulus predicts an unconditioned stimulus (i.e., the stimulus contingency) is an important predictor of effective conditioning of many sorts (Domjan, 2018). Although CTA is exceptional, in that it may be effective after only one trial (Garcia et al., 1974), additional trials, to increase the absolute and relative exposure to the illness in combination with the food, are likely to increase the strength of the CTA (Fenwick et al., 1975; Navarro et al., 2000; Tobajas et al., 2020c). Preparatory captive experiments can be used to estimate the minimum number of trials that are needed for the desired level of aversion to develop, especially when animals have been pre-exposed to the food. In field situations, it is more challenging to control the number of trials one individual is exposed to compared to captive settings. Indeed, in <15% of field studies (four experiments) that clearly involved pre-existing conflict, could the authors be sure that subjects were repeatedly exposed to the emetic and in only 16% of studies did authors explicitly state subjects were not repeatedly exposed (Supplementary Data 1). In some cases, the probability of exposure can be managed by using a suitable spatial and temporal distribution of treated food items, informed by the species movement behavior (Indigo et al., 2019; Tobajas et al., 2020a). Yet, in field experiments, exposure duration often varied from a few days up to several months, even within species (Supplementary Data 1), suggesting that not all studies use the same species-informed distribution of treated food items. In addition, presenting the treated items in a way that discourages systematic consumption by non-target species (Tobajas et al., 2020a), such as in rings of powdered insecticide (Indigo et al., 2018), will also improve exposure probability.
Animal Safety
Inflicting sickness is what gives CTA its evolutionary relevance and power to deter consumption in the future. Thus, it is not possible to create a CTA without a degree of suffering. Nonetheless, an ethical approach to CTA requires that practitioners attempt to limit the magnitude and frequency of illness to that required to make the technique effective for protecting the food source. In addition, lethal (side) effects on both target and non-target species should be actively prevented (Smith et al., 2021). The trade-off between guaranteeing ethical treatment of and safety for wildlife and the environment vs. achieving CTA is probably one of the biggest challenges of applying CTA in the field.
To avoid unnecessary suffering, incapacitation, or death of animals, it is important to use a chemical substance with a large acute margin of safety, meaning a high dose is required to reach severe toxicity while a low dose remains effective in inducing aversion (Tobajas et al., 2019a). The acute margin of safety is calculated as the ratio between LD50 (the dose that kills 50% of the animals) and the minimum potential dose to induce CTA in the target species. In addition, time until recovery should be short. Without this information, animals may suffer for an unnecessary length of time (Cornell and Cornely, 1979). Optimal dosages can be determined by either performing captive trials with the species of interest, or similar species, or by using existing literature on the substance (e.g., Table 2). The dosage should also always be matched to the body mass of the target species, to ensure that the dosage is not fatal to the smallest species and individuals involved (e.g., Nicolaus and Nellis, 1987). Additionally, it should be considered that animals might consume multiple food items in quick succession. Thus, the amount of substance per food item should include a high margin of safety to account for this (Tobajas et al., 2019a).
Potential detrimental side-effects of substances and vulnerability of particular classes of animals should always be considered a priori. Even though all of the substances in Table 2 were to some degree effective, they are not equally recommended for use. Specifically, the use of a hormone such as ethinyl estradiol, a synthetic estrogen, can have detrimental effects for certain individuals in the population. For example, in a study of raccoons (Procyon lotor), ethinyl estradiol was safely ingested by most raccoons, but it apparently caused the death of a pregnant female (Dueser et al., 2018) and the compound is known to have detrimental effects on unborn fetuses (Yasuda et al., 1981). Besides the negative effects on health, this hormone has different effects on CTA formation for males and females. CTA induction with ethinyl estradiol was less effective in female than male Norway rats (De Beun et al., 1991), although no such difference was observed in raccoons (Dueser et al., 2018). Potential adverse effects of chemical compounds, such as fluoxetine hydrochloride, on the central neural system should also be considered. Although this substance has produced long-lasting CTA in Norway rats (Massei and Cowan, 2002), it modifies the natural behavior of animals, exciting or depressing behavior, which could make animals more vulnerable to other risks for injury or death in the wild.
A particular safety concern of CTA in the field is consumption by non-target species. Studying the effects of the substance on the non-target species that are likely to be affected can help determine a safe dosage (Tobajas et al., 2019a). For example, a project to protect California condor (Gymnogyps californianus) eggs from ravens (Corvus corax) showed that the condor itself was most likely to be affected by the intervention, but could be safely protected with the compound landrin (Quick and Hill, 1988). Further, research should explore use of selective bait deployment methods to reduce ingestion by non-target animals and the smart use of lures or attractants to increase the food detectability specifically by the target species (Ferreras et al., 2018; Tobajas et al., 2021b). Another way to avoid bait consumption by non-target species is to directly inject the substance into the subject after it has eaten the relevant food. Although safer and more likely to be effective (the substance can by definition not be detected in the food), this is practically and logistically very challenging to manage in the field. Although this technique was used historically in over 20% of (almost exclusively captive) applications, it has rarely been used in the last 10 years (Figure 5).
Characteristics of unconditioned stimulus intensity and safety are often and appropriately incorporated in legislation about animal welfare, leading to restrictions in the substances that are allowed, as well as the dosages of application and density of treated of baits. For example, in order to allow for multiple baits and uptake by small individuals and non-target species, the Australian Pesticides and Veterinary Medicines Authority (APVMA) set the allowed dosage of thiabendazole for Northern quolls (Dasyurus hallucatus) at < a ninth of the LD50 (Indigo et al., 2018). The dosage was calculated for uptake by small females (200 g), but may have been insufficient to effectively induce CTA in large males, which can be more than four times as heavy (Indigo et al., 2018). The APVMA also limited the number of baits that could be deployed at the same time, resulting in many quolls encountering an empty baiting station (Indigo et al., 2018). Similarly, as a result of stringent environmental standards required by the U.S. Environmental Protection Agency, the number of commercially available bird repellents was reduced (Clark, 1998; Sayre and Clark, 2001) and concerns about suffering also led to the loss of effective bird CTA products containing methiocarb (Dolbeer et al., 1994). Finding the right balance between safety and effectiveness within the boundaries of the law is an important non-trivial challenge that requires serious attention when considering the use of CTA. More use of complementary wildlife technology may provide some solutions, for example via the development of bait stations that can control the entrance of animals via ID-coded tags or AI-aided individual recognition (Berger-Tal and Lahoz-Monfort, 2018).
Stage 3: How Can Generalization of CTA to Untreated Resources be Encouraged?
Stimulus Specificity
Animals have some ability to generalize food aversions from one food to another similar food (Nachman, 1963; Kelly et al., 2018), but there are substantial limits. For successful aversion of the target food to occur, the animal needs to generalize the CTA by linking the illness to those stimuli that are shared between the treated and untreated food resources. A significant hurdle in the application of CTA in the field is that the characteristics of the food used during a conditioning period often differ from the characteristics of the food targeted for protection that the animals will encounter in the wild. For example, predators might be trained to avoid the eggs of a species at risk via the use of eggs of domestic chickens or quail (Brinkman et al., 2018). The discrepancy between treated and untreated foods is primarily caused by ethical, legal and practical constraints, e.g., in the use of eggs from threatened species or live prey, but it may, thus, prevent animals from generalizing a learned aversion between food types.
It is challenging to predict which conditioned stimulus (i.e., food characteristic) an animal will use. When coyotes were trained not to consume hamburgers, they did not generalize their aversion to other meats such as hotdogs (Cornell and Cornely, 1979). Yet, when CTA was used to condition aversion in black bears against meals-ready-to-eat (MREs), the bears also rejected MRE types other than the ones they were trained on (Ternent and Garshelis, 1999). Possibly the bears conditioned to an (odor) characteristic that was shared among the different MREs, e.g., the scent of a particular cooking oil, preservative or seasoning, or possibly the packaging. In the following paragraphs, we go into more detail about the factors that may hamper or promote stimulus generalization. We use the term CTA throughout, even though some animals may have associated illness with a stimulus other than taste. In those cases, conditioned food aversion (CFA) would be the more appropriate term, but it is not always possible to distinguish these mechanisms, particularly in field studies. Moreover, “second-order conditioning,” with the animal associating taste to the illness and subsequently associating another stimulus, such as odor, to the taste, (Gustavson et al., 1974) or “taste potentiated odor aversion” (Lett, 1984) may be at play (see “Supporting stimuli”), allowing the animal to avoid the food resource before having to taste it again.
Stimulus Detectability in Treated Food
For CTA to be learned as an association to a food, the emetic used to generate the illness must not be detectable by target animals via a novel smell, taste or color. When this occurs, animals simply avoid the treated food and continue to consume untreated variants. Many studies have demonstrated that some CTA emetics can be detected by animals (Burns, 1980; Ellins and Martin, 1981; Massei et al., 2003; Catry and Granadeiro, 2006), which can severely hamper the intervention because the animals associate the more salient stimulus (i.e., the emetic and not the food) with the illness (Conover, 1984; Cagnacci et al., 2005). One potential way to overcome this problem of detectability is to present treated and control foods at the same time and place, which could make it harder for animals to link substance characteristics to the illness, a procedure that showed promise in American black bears (Homstol, 2011). Similar confusion may also be a reason that CTA efficacy was maximized in crows when only 50% of the baits were treated (Dimmick and Nicolaus, 1990). Another option is to mask the taste, smell or color of the illness-inducing substance. After several reports of failed CTA generalization with levamisole (Massei et al., 2003; Gentle et al., 2004; Cagnacci et al., 2005), Cotterill et al. (2006) attempted to mask the bitter taste of levamisole by forming an ion-exchange resin. After the levamisole was altered to be tasteless, Norway rats formed a CTA against food containing the levamisole-resinate and generalized this to untreated food (Cotterill et al., 2006). Unfortunately, using the resinate creates the need to use a bigger volume of substance for the same effective dose. When (Nielsen et al., 2015) applied the levamisole-resinate to small baits for foxes, the normal levamisole actually appeared more effective than the resinate, likely because the foxes were able to detect the relatively high proportion of levamisole-resinate (Nielsen et al., 2015). Levamisole-resinate might thus only be effective when it is applied to food items which allow for a low proportion of levamisole-resinate relative to food content, masking the increased volume. Another attempt to hide levamisole's taste was made by micro-encapsulating it. However, dogs (Canis lupus familiarus) were still able to detect the levamisole and the encapsulation might have even weakened the CTA by delaying the release of levamisole (Tobajas et al., 2019b). A recent study with captive wolves showed more promising results with new kind of micro-granulated levamisole (Tobajas et al., 2020b), but here, too, the increased volume needs to be masked by a relatively big food item. Whenever possible, researchers should use an undetectable substance (Tobajas et al., 2019a; Pebsworth and Radhakrishna, 2020), such as the fungicide thiram (Table 2; Supplementary Figure 2) that has been shown to be undetectable by domestic dogs and wild foxes (Tobajas et al., 2020a,c). Nevertheless, <5% of field experiments explicitly reports using either a resinate, micro-encapsulation or thiram (one experiment for each; Supplementary Data 1).
In addition to the detectability of emetics, animals might perceive other differences between treated and untreated foods, such as differences in palatability, texture, shape, color or smell. In order for CTA to be effective, the food item should be fresh and palatable. A piece of spoiled food with a potent substance will be theoretically effective at inducing CTA, but could be less likely to be consumed by the target animal or the animal might develop an aversion specific to spoiled foods (Nicolaus and Nellis, 1987). Similarly, when artificial foods are used for training instead of natural foods and these natural foods are prone to spoiling (e.g., eggs), the difference might help predators discriminate between treated (artificial) food items and untreated (natural) food items (Brinkman et al., 2018). It is thus important to implement methods to guarantee long-term or equal palatability. Many CTA interventions do this by regularly replenishing baits or eggs (e.g., Dimmick and Nicolaus, 1990; Indigo et al., 2018; Tobajas et al., 2020a, 2021a).
The ability to distinguish between treated and untreated foods varies among species due to differences in sensory capabilities as well as the primary sensory modalities used for detecting food. Birds can see in the ultraviolet spectrum and as a consequence treated eggs that are painted to resemble untreated natural eggs may look very similar to humans but very different to birds (Dimmick and Nicolaus, 1990). The ability, or choice, to distinguish may also vary among individuals owing to sensory sensitivity or prior experience with the food. For example, use of methiocarb to protect grapes from gray-backed white-eyes (Zosterops lateralis) was generalized to untreated grapes only by the birds that had not previously fed on grapes (Rooke, 1984).
Surrogate Stimuli
CTA has been used in attempts to deter predators from consuming livestock (Table 1; Figures 1, 3), most often by treating carcasses that are used as a surrogate for live prey. Indeed, 100% of all field experiments involving conflicts with live animal predation used carcasses or some kind of bait (Supplementary Data 1). However, dead animals or animal parts present as very different stimuli compared to live animals. Moreover, an animal may be sensitive to different kinds of stimuli depending on whether it is searching, attacking or consuming a prey (Langley and Knapp, 1982). Early studies on CTA interventions in predator conflicts used live prey (Horn, 1983), but such approaches are no longer ethically tenable and are often outlawed by legislation (Marshall et al., 2019). This circumstance promoted the use of baits and carcasses as proxies, but with mixed success. Although animals often develop an aversion to the baits/carcasses, this aversion is mentioned to rarely generalize to live prey (Conover et al., 1977; Burns and Connolly, 1980). Surprisingly, however, 78% of field experiments (seven out of nine) that used baits or carcasses in situations of live prey predation, and which mentioned a clear study outcome, reported some degree of success in reducing predation (Supplementary Data 1). Read et al. (2016) have promoted the use of “Trojan prey” wherein livestock could be fitted with harnesses or collars containing a noxious substance, making them more analogous to any other livestock animal. While such a method still uses live prey as a stimulus, an important difference to earlier, and arguably less ethically defensible, studies is that collared animals are not deliberately “fed” to predators. Instead, collared and non-collared animals mix in a herd, and therefore, have the same probability, in principle, of being attacked. Yet predators may learn to discriminate between collared and non-collared prey or learn to avoid the collar during the attack. An early study on non-lethal toxic collars with known livestock killing (i.e., pre-exposed) coyotes in captivity did not reveal promising results because the coyotes resumed killing an average of 40 days after treatment (Burns et al., 1984). Further rigorously designed studies are needed to determine the effectiveness of “Trojan prey” approaches.
The challenge of generalizing to live prey was also encountered in the effort to protect native predators from the invasive, toxic cane toad (Rhinella marina) in Australia. In order to condition animals in situ with safer and practical food stimulus researchers created “toad sausages” (Price-Rees et al., 2011). These sausages differed from live toads visually via both shape and (lack of) movement, but conditioning bluetongue lizards (Tiliqua scincoides) with cane toad sausages still increased lizard survival in the wild (Price-Rees et al., 2013). This result may have occurred because visual stimuli do not play a key role in lizard foraging behavior (Kelly et al., 2018), or because live cane toads do not move much when under attack. Captive monitor lizards (Varanus panoptes) (Ward-Fear et al., 2017) and Northern quolls (Indigo et al., 2018) showed a corresponding decreased interest in live or dead toads after conditioning with sausages. Unfortunately, the training of monitor lizards did not increase their survival after release into the wild, while in situ conditioning with live toads did (Ward-Fear et al., 2016).
Supporting Stimuli
One promising strategy to promote generalization from the conditioned stimulus used in training to the living “food resource” would be to add additional olfactory or visual stimuli or both, depending on the primary senses used by the species during foraging (Elmahdi et al., 1985; Baker et al., 2008; Tobajas et al., 2019b). Learned aversions are often stronger when both taste and odor stimuli are involved, a phenomenon called “taste potentiated odor aversion” (TPOA) (Rusiniak et al., 1979; Palmerino et al., 1980; Lett, 1984). The association between smell and taste is advantageous because aversion to smell allows animals to avoid the more damaging effects of ingestion. Moreover, odor aversion is very useful in HWC because it can prevent animals from tasting and thus harming a live animal or resource. The use of TPOA was well-illustrated with crop-raiding badgers (Meles meles) exposed to baits coated with the emetic ziram, some of which were also paired with clove oil as an odor stimulus (Baker et al., 2007). In the treatment where a combination of taste and odor (bait and clove oil) was used, the aversion was successfully generalized to untreated bait sprayed with clove oil, while in the ziram only treatment no generalization occurred. A subsequent field study yielded similar, promising results with badgers averting clove oil-sprayed maize (Baker et al., 2008). These studies suggest that in order to protect all crops, they only have to be sprayed with the relatively harmless clove oil after the initial conditioning phase. TPOA might also protect prey from predation during animal translocations. This strategy consists of using TPOA to condition predators, and applying the odor stimulus to a buffer zone to protect areas with translocated prey (e.g., rabbits, Oryctolagus cuniculus), to reduce predation short after release, a time during which translocated animals are more vulnerable (Tobajas et al., 2021a). Similarly, TPOA may be used to protect domestic livestock from large carnivore predation by creating an odor buffer zone in fenced areas that confine livestock (Tobajas et al., 2020b). Up to now, only very few field experiments (four), comprising three Carnivora families, used TPOA and all reported some degree of success (Supplementary Data 1).
Next to taste and odor, many species use visual and tactile stimuli to find food. Such stimuli could also be added to the food to stimulate generalized aversion. Tactile stimuli are more likely to be effective with species that handle their food before ingestion, such as rats, monkeys and raccoons. When the taste of two food items is identical, aversion can still be formed purely based on different tactile stimuli (Domjan and Hanlon, 1982; Domjan et al., 1982). Visual stimuli are often effective in stimulating generalization in birds. Elmahdi et al. (1985) applied a combination of the white substance calcium carbonate and the nauseating substance methiocarb to several crop types. The aversion to methiocarb treated crops was generalized to untreated crops with the same white (calcium carbonate) coating. Because species that primarily use visual stimuli can be very sensitive to different gradients in color, the visual stimulus should always be added together with the (to humans) likewise colored emetic during the conditioning phase, not only afterwards (e.g., Rooke, 1984), to maximize the chance of generalized aversion. Three field experiments, all restricted to birds (corvids), focused on using visual support stimuli in CTA applications and all claimed to be successful, while no such field studies were conducted with non-avian species (Supplementary Data 1). One of the few captive non-avian experiments on this topic studied which characteristics of a food item could be changed for quolls to generalize their learned aversion (Kelly et al., 2018). For these animals, natural taste turned out to be a more important stimulus than visual shape to generalize aversion. Studies in Norway rats also indicated little relevance for visual food stimuli (Garcia and Koelling, 1967; Miller and Domjan, 1981). Nevertheless, for non-avian species that rely heavily on vision for foraging, there may be merit in attempting to enhance associations through manipulating relevant, visual stimuli.
A powerful advantage of adding a visual stimulus is that it can be added to the environment of foraging animals, not only to the food item they consume. Mason and Reidinger (1983) tested this in captivity by attaching a colored rectangular card to the feeding bowl of red-winged blackbirds during the conditioning phase. In a subsequent preference test, the birds that received methiocarb showed a clear preference for the food bowl without the colored card, while birds that received a control substance showed no preference. Mason and Reidinger (1983) also showed that the aversion could be transferred from one food type to another, given that the other food contained the same color stimulus. This experiment has interesting implications for the use of visual stimuli in human-bird conflicts. While it might be undesirable to color all crop plants, it could be feasible to surround an entire field with distinctly colored flags that birds have trained to associate with the experience of illness.
Stage 4: How Can Extinction of the Aversion be Delayed?
Extinction Likelihood
Although CTA can be a robust and lasting form of associative learning, extinction can still occur. The reported minimum retention of CTA of animals in the field varies from days to over a year (Nicolaus and Nellis, 1987; Ternent and Garshelis, 1999; Tobajas et al., 2020a; Supplementary Data 1). In many studies, the duration is a conservative estimate, because the study ends before the aversion has extinguished (e.g., Gabriel and Golightly, 2014). Overall, there is little information on the expected retention of CTA, and it seems to vary widely between species, settings, and methodological approaches. Knowing the approximate extinction time is highly relevant because it informs managers when conditioning interventions should be repeated or when conditioning should be initiated, such as relative to when the arrival of a toxic prey species is expected (Indigo et al., 2018).
Extinction is not the reverse of aversion acquisition, but the learning of a new relationship, such as that a food characteristic does not predict illness or predicts reward (Revillo et al., 2014). The previously learned aversive relationship is not forgotten and can quickly resurface with reinforcement. However, the same principle holds for any previously learned rewarding relationships with the food. Multiple types of associations is the reason taste aversions are less successful when animals have had previously rewarding encounters with the food (Fenwick et al., 1975). Additionally, CTA extinction occurs sooner in individuals that have experience with a high variety of foods than in individuals raised on a more restricted diet, presumably because individuals with broader diets are less wary of novel foods and more likely to resample the conditioned food sooner (Gentle et al., 2006). However, there appears to be a trade-off when it comes to diet diversity, since aversions are also only likely to be persistent when sufficient alternative foods are available to the animal (Dethier and Yost, 1979; Ratcliffe et al., 2003), reducing the energetic and potential fitness cost of avoiding the treated food type (but see Potter and Held, 1999). Related to this, a learned aversion seems to be stronger in the presence of suitable alternative food (Dragoin et al., 1971).
Whether and how much “maintenance” exposure is necessary will thus depend on (novel) diet experience, but also on more general species-specific characteristics, such as lifespan and social system. Populations of short-lived, compared to long-lived, species will need more frequent retraining, since a proportion of naïve individuals will quickly recruit into such populations with each new generation. Amongst long-lived species, territorial species, such as ravens, may require much less maintenance than non-territorial species, since the trained territory holders will often keep (naïve) conspecifics at a distance (Avery et al., 1995). Lastly, the occurrence of cultural transmission of learned safety (or harm) in populations is expected to affect the food aversion extinction process and we discuss this in more detail below. Overall, it is helpful to keep in mind that not the entire population needs to be effectively (re)trained, but just an adequate proportion to reduce and keep consumption, or depredation, of the target food at acceptable levels.
Social Learning
CTA is more efficient as a management tool when individuals retain learned aversions for a long time and if it can transfer to the level of populations. Potential for population-level spread exists in social animals that obtain and use information about the palatability of food from conspecifics or social group members (Galef, 1987), which could both promote and reduce food aversions. Social extinction of CTA happens when a conditioned individual observes and (re)learns from naive (untrained) individuals. This phenomenon is known to occur in many social species, including Norway rats (Galef, 1986), cattle (Bos Taurus, Lane et al., 1990), sheep (Ovis aries, Thorhallsdottir et al., 1990), spotted hyenas (Crocuta crocuta, Yoerg, 1991) and tamarins (Saguinus fuscicollis and Saguinus labiatus, Prescott et al., 2005). In all cases, food aversion learned by individuals was later extinguished through social learning. In cattle, CTAs retained for as long as a year were lost after social contact with untrained conspecifics (Lane et al., 1990). A comparison between lambs and adult ewes suggested that young animals may be more prone to social extinction of CTA than adults (Thorhallsdottir et al., 1990). In addition to promoting extinction of aversion, social learning can also promote the establishment of a food aversion. Evidence for social transfer of CTA has been found in several bird species (Mason and Reidinger, 1982; Mason et al., 1984; Landová et al., 2017; Thorogood et al., 2018; Hämäläinen et al., 2020). In these studies, seeing a conspecific, or heterospecific (Mason et al., 1984; Hämäläinen et al., 2020), get sick from or reject a certain food type reduced the bird's preference for this food type. However, not all animals can learn food aversions from conspecifics and rats appear to be especially variable in their responses to conspecifics (Kuan and Colwill, 1997; Galef and Whiskin, 2000). Several studies show that Norway rats do not socially transfer food aversions (Galef et al., 1983, 1990; Galef, 1985) and may even target food that they observed another rat to consume, even if it later became ill (Galef et al., 1990).
In the context of human-wildlife conflict, little is known about the social transfer of food aversion. Only five experiments conducted completely in the field explicitly reported (anecdotal) evidence on the absence or presence of social learning effects (Supplementary Data 1). In addition, Cremona et al. (2017) specifically studied whether CTA transferred over generations after conditioning captive quolls to avert toxic cane toads and reintroducing them into the wild. They found that the offspring of trained female quolls exhibited higher rates of survival, but they could not determine whether this occurred because the young quolls had learned from their mothers not to consume toads or whether they benefited from a rapid genetic adaptation of avoidance acquired from their untrained fathers (Kelly and Phillips, 2019). In some wildlife conflict situations, social transfer of CTA was explicitly not observed. While foxes readily learned to avoid eggs in the study of Tobajas et al. (2020a), their offspring were observed to predate on the same kind of eggs. For most species, there is insufficient knowledge to predict whether social transfer, social extinction or both will occur during application of CTA in the wild.
Synthesis
In this review, we have provided guidance for CTA application in HWC contexts and synthesize these points in a decision table that might be used by wildlife managers (Table 3). We emphasize the need to identify a specific food type as the source of conflict, the importance of considering characteristics of both the treated species and the emetic to be used to create an aversion, the need to use pilot work or the literature to identify suitable dosages, and give specific additional guidance for several common types of conflict. Whilst our review of relevant topics was not exhaustive, our aim was to highlight a number of frequently occurring determinants of success to help managers decide whether CTA is appropriate to a given conflict situation and then to navigate a dispersed literature quickly to achieve effective application of CTA in a specific HWC context. We offer our review in the style of a systematic map (Berger-Tal et al., 2019), showing what literature exists and its characteristics. We share some very preliminary estimates of success based on the conclusions made by the respective authors as a guidance for readers, but stress that these assessments by authors are not comparable to a quantitative systematic review. Instead, we present our overview as a starting point for future critical appraisal and meta-analysis of particular combinations of emetics, application contexts, and species.
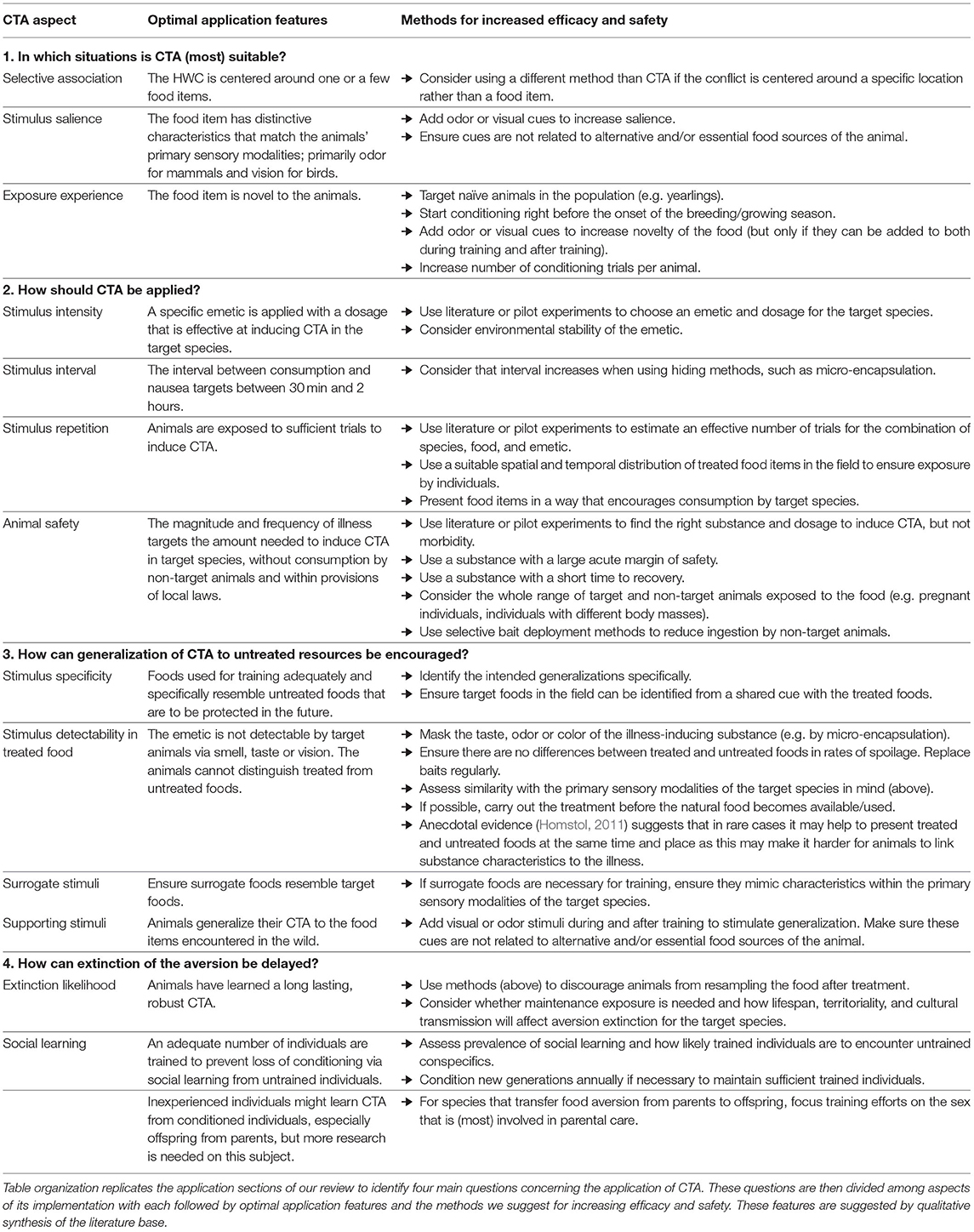
Table 3. Summary of decision points for the application of CTA in HWC contexts that could be used by wildlife managers.
In our review, we guided the reader chronologically from a brief history of the discovery and applications of CTA in wildlife management to the several steps involved in implementing it (Figure 2). We showed that CTA has already been applied to diverse contexts related to human wildlife conflict, albeit often with small sample sizes (Figure 4), and acknowledged that it was not always successful. We synthesized this information to suggest when CTA appears to be most suitable as an intervention, went on to describe how it can be applied, and reviewed the effects of stimulus intensity, timing, frequency, and safety. Then we described how to generalize aversive responses among contexts, avoid detection of emetic substances and use surrogates and supportive stimuli. We finished with a section on avoiding extinction of learned responses, and addressed the potential for conspecifics to both reinforce and reverse learned aversions. Future work might quantitatively evaluate the surprising number of factors our review revealed as contributing to the success of CTA in the field, particularly the learning principles underlying CTA.
Due to rapid learning, sometimes from a single trial, and long-term retention, CTA can be a valuable addition to the toolbox for human-wildlife coexistence. However, application of CTA, especially in the field, presents multiple challenges and considerable care must be taken to support ethical treatment of animals. Throughout our review, we identified some areas that first require further research before concrete recommendations can be offered. For example, livestock protection started out as the focus of many CTA studies in the 1970s and 1980s, but contradictory studies limited further research for several decades (reviewed in Tobajas et al., 2020b; Figure 1). A promising improvement of CTA application in this context appears to be the use of “undetectable” substances such as thiram (Tobajas et al., 2020a) and the addition of visual or odor stimuli to both the food used in training and to living livestock to increase the likelihood of generalization (Tobajas et al., 2019b). Also, the social extinction of food conditioning appears very relevant to the feasibility of CTA in the field, yet remains relatively understudied. Despite the limitations of past studies and the biases in in the literature base toward particular contexts, locations, and species, they sum to provide a valuable foundation for understanding whether and why CTA may be an effective management strategy in particular contexts. Practitioners and researchers conducting future interventions could maximize their contributions to a general understanding of CTA by monitoring target food consumption before and after an intervention on randomly assigned treated vs. untreated populations (i.e., a BACI design), using a meaningful sample size (Figure 4) and, where possible, with identifiable individuals. We thus hope that the information in our review will help wildlife managers increase their use and efficacy of this promising non-lethal tool to reduce many kinds of HWC in the future.
Author Contributions
LS and NT performed the literature search and categorization. NT wrote the first draft of the manuscript. LS wrote a revised version and created the figures with input from all authors. All authors commented on previous versions of the manuscript, read, approved the final manuscript, and jointly developed the concept for the article.
Funding
LS was funded by a NWO-Veni fellowship (VI.Veni.192.018). JT benefitted from a FPI Ph.D. scholarship (BES-2014-068987) funded by the Spanish Ministry of Economy and Competitiveness. CS was funded by an NSERC Discovery Grant (Canada; RGPIN-2017-05915).
Conflict of Interest
The authors declare that the research was conducted in the absence of any commercial or financial relationships that could be construed as a potential conflict of interest.
Publisher's Note
All claims expressed in this article are solely those of the authors and do not necessarily represent those of their affiliated organizations, or those of the publisher, the editors and the reviewers. Any product that may be evaluated in this article, or claim that may be made by its manufacturer, is not guaranteed or endorsed by the publisher.
Supplementary Material
The Supplementary Material for this article can be found online at: https://www.frontiersin.org/articles/10.3389/fcosc.2021.744704/full#supplementary-material
Supplementary Figure 1. Geographic distribution of retrieved CTA studies. Searches were only conducted in English, so there is a bias for English-speaking countries in the number of HWC related CTA studies. Yet, substantial variation is apparent even among English-speaking countries. Studies were retrieved via systematic search and ad hoc retrievals (see Methodology).
Supplementary Figure 2. Relative use of illness-inducing subtances across 256 CTA experiments. Substances were either applied to the food resource or directly to the subject following consumption of the food resource. ANTU, Alpha-naphtyl-thiourea; APT, Antimony potassium tartrate; Bufa, Bufadienolide (natural cane toad toxin); Carba, Carbachol; Cinna, Cinnamamide; CupSu, Cupric sulfate; CyPP, Cyclophosphamide; DiAnt, Dimethyl anthranilate; E and L, Emetine + Lithium chloride; EE, Ethinyl estradiol; Emet, Emetine; FBZ, Fenbendazole; FCZ, Fluconazole; FLR, Fluralaner; HyCy, Hyoscyamine; JJB, Jojoba; Leva, Levamisole; LiCl, Lithium chloride; Lnd, Landrin; Met, Methiocarb; Parac, Paracetamol; SodCa, Sodium carbonate; SodMo, Sodium monofluoroacetate; TandM, Thiram + Methiocarb; TBZ, Thiabendazole; Thir, Thiram; Ziram, Ziram; ?, unknown substance. Studies were retrieved via systematic search and ad hoc retrievals (see Methodology).
Supplementary Table 1. Web of Science search string.
Supplementary Table 2. Scopus search string.
Supplementary Data 1. Conditioned taste aversion in conservation literature data sheets.
References
Annau, Z., and Kamin, L. J. (1961). The conditioned emotional response as a function of intensity of the US. J. Comp. Physiol. Psychol. 54, 428–432. doi: 10.1037/h0042199
Avery, M. L., Pavelka, M. A., Bergman, D. L., Decker, D. G., Knittle, C. E., and Linz, G. M. (1995). Aversive conditioning to reduce raven predation on California least tern eggs. Colon. Waterbirds 18, 131–138. doi: 10.2307/1521474
Baker, S. E., Ellwood, S. A., Slater, D., Watkins, R. W., and Macdonald, D. W. (2008). Food aversion plus odor cue protects crop from wild mammals. J. Wildl. Manag. 72, 785–791. doi: 10.2193/2005-389
Baker, S. E., Johnson, P. J., Slater, D., Watkins, R. W., and Macdonald, D. W. (2007). Learned food aversion with and without an odour cue for protecting untreated baits from wild mammal foraging. Appl. Anim. Behav. Sci. 102, 410–428. doi: 10.1016/j.applanim.2006.05.039
Bekoff, M. (1975). Predation and aversive conditioning in coyotes. Science 187, 1096–1096. doi: 10.1126/science.187.4181.1096
Berger-Tal, O., Greggor, A. L., Macura, B., Adams, C. A., Blumenthal, A., Bouskila, A., et al. (2019). Systematic reviews and maps as tools for applying behavioral ecology to management and policy. Behav. Ecol. 30, 1–8. doi: 10.1093/beheco/ary130
Berger-Tal, O., and Lahoz-Monfort, J. J. (2018). Conservation technology: the next generation. Conserv. Lett. 11:e12458. doi: 10.1111/conl.12458
Brett, L. P., Hankins, W. G., and Garcia, J. (1976). Prey-lithium aversions. III: buteo hawks. Behav. Ecol. 17, 87–98. doi: 10.1016/S0091-6773(76)90302-3
Brinkman, M. P., Garcelon, D. K., and Colwell, M. A. (2018). Evaluating the efficacy of carbachol at reducing corvid predation on artificial nests. Wildl. Soc. Bull. 42, 84–93. doi: 10.1002/wsb.852
Burns, R. J., and Connolly, G. E. (1980). Lithium chloride bait aversion did not influence prey killing by coyotes. Proc. Vertebrate Pest Conf. 9, 200–204. Available online at: https://escholarship.org/uc/item/4q50k26f (accessed March 19, 2021).
Burns, R. J., Connolly, G. E., and Griffiths, R. E. Jr. (1984). Repellent or aversive chemicals in sheep neck collars did not deter coyote attacks. Proc. Vertebrate Pest Conf. 11, 146–153. Available online at: https://escholarship.org/uc/item/1j88v715 (accessed June 28, 2021).
Burns, R. J. (1980). Evaluation of conditioned predation aversion for controlling coyote predation. J. Wildl. Manage. 44, 938–942. doi: 10.2307/3808330
Burns, R. J., and Connolly, G. E. (1985). A comment on “coyote control and taste aversion.” Appetite 6, 276–281. doi: 10.1016/S0195-6663(85)80018-0
Cagnacci, F., Massei, G., Cowan, D. P., and Delahay, R. J. (2005). Can learned aversion be used to control bait uptake by Eurasian badgers? Appl. Anim. Behav. Sci. 92, 159–168. doi: 10.1016/j.applanim.2004.11.003
Catry, T., and Granadeiro, J. P. (2006). Failure of methiocarb to produce conditioned taste aversion in carrion crows consuming little tern eggs. Waterbirds 29, 211–214. doi: 10.1675/1524-4695(2006)29[211:FOMTPC]2.0.CO;2
Chaudhary, V., and Tripathi, R. S. (2017). Feeding deterrence effects of defatted jojoba (Simmondisa chinensis) meal against Indian gerbil, Tatera indica (Hardwicke). Proc. Natl. Acad. Sci. India Sect. B Biol. Sci. 87, 663–670. doi: 10.1007/s40011-015-0633-7
Clark, L. (1998). Review of bird repellents. Proc. Vertebrate Pest Conf. 18, 330–337. doi: 10.5070/V418110214
Conover, M. R. (1984). Response of birds to different types of food repellents. J. Appl. Ecol. 21, 437–443. doi: 10.2307/2403420
Conover, M. R. (2002). Resolving Human-Wildlife Conflicts: The Science of Wildlife Damage Management, 1st Edn. Boca Raton: Lewis Publishers. doi: 10.1201/9781420032581.ch1
Conover, M. R., Francik, J. G., and Miller, D. E. (1977). An experimental evaluation of aversive conditioning for controlling coyote predation. J. Wildl. Manage. 41, 775–779. doi: 10.2307/3800006
Conover, M. R., Francik, J. G., and Miller, D. E. (1979). Aversive conditioning in coyotes: a reply. J. Wildl. Manage. 43, 209–211. doi: 10.2307/3800654
Cornell, D., and Cornely, J. E. (1979). Aversive conditioning of campground coyotes in Joshua tree national monument. Wildl. Soc. Bull. 7, 129–131. Available online at: https://www.jstor.org/stable/3781551 (accessed August 30, 2021).
Cotterill, J. V., Massei, G., and Cowan, D. P. (2006). Masking the taste of the conditioned taste aversion agent levamisole using an ion-exchange resin, for practical application in wildlife management. Pest Manag. Sci. 62, 120–125. doi: 10.1002/ps.1129
Cowan, D. P., Reynolds, J. C., and Gill, E. L. (2000). “Reducing predation through conditioned taste aversion,” in Behaviour and Conservation, Vol. 2, eds L. M. Gosling and W. J. Sutherland (Cambridge: Cambridge University Press), 281–299.
Cremona, T., Spencer, P., Shine, R., and Webb, J. K. (2017). Avoiding the last supper: parentage analysis indicates multi-generational survival of re-introduced “toad-smart” lineage. Conserv. Genet. 18, 1475–1480. doi: 10.1007/s10592-017-0973-3
De Beun, R., Jansen, E., Smeets, M. A. M., Niesing, J., Slangen, J. L., and van de Poll, N. E. (1991). Estradiol-induced conditioned taste aversion and place aversion in rats: sex-and dose-dependent effects. Physiol. Behav. 50, 995–1000. doi: 10.1016/0031-9384(91)90427-P
Delacasa, G., and Lubow, R. E. (1995). Latent inhibition in conditioned taste aversion: the roles of stimulus frequency and duration and the amount of fluid ingested during preexposure. Neurobiol. Learn. Mem. 64, 125–132. doi: 10.1006/nlme.1995.1051
Dethier, V. G., and Yost, M. T. (1979). Oligophagy and absence of food-aversion learning in tobacco hornworms, Manduca sexta. Physiol. Entomol. 4, 125–130. doi: 10.1111/j.1365-3032.1979.tb00187.x
Dimmick, C. R., and Nicolaus, L. K. (1990). Efficiency of conditioned aversion in reducing depredation by crows. J. Appl. Ecol. 27, 200–209. doi: 10.2307/2403578
Dolbeer, R. A., Avery, M. L., and Tobin, M. E. (1994). Assessment of field hazards to birds from methiocarb applications to fruit crops. Pestic. Sci. 40, 147–161. doi: 10.1002/ps.2780400210
Domjan, M. (2018). The Essentials of Conditioning and Learning, 4th Edn. Washington, DC: American Psychological Association. doi: 10.1037/0000057-000
Domjan, M., and Hanlon, M. J. (1982). Poison-avoidance learning to food-related tactile stimuli: avoidance of texture cues by rats. Anim. Learn. Behav. 10, 293–300. doi: 10.3758/BF03213713
Domjan, M., Miller, V., and Gemberling, G. A. (1982). Note on aversion learning to the shape of food by monkeys. J. Exp. Anal. Behav. 38, 87–91. doi: 10.1901/jeab.1982.38-87
Dorrance, M. J., and Roy, L. D. (1978). “Aversive conditioning tests of black bears in beeyards failed,” in Proceedings of the 8th Vertebrate Pest Conference (Sacramento, CA). Available online at: https://digitalcommons.unl.edu/vpc8/15 (accessed March 1, 1978).
Dragoin, W., Mccleary, G. E., and McCleary, P. (1971). A comparison of two methods of measuring conditioned taste aversions. Behav. Res. Methods Instrum. 3, 309–310. doi: 10.3758/BF03209954
du Toit, J. T., Provenza, F. D., and Nastis, A. (1991). Conditioned taste aversions: how sick must a ruminant get before it learns about toxicity in foods? Appl. Anim. Behav. Sci. 30, 35–46. doi: 10.1016/0168-1591(91)90083-A
Dueser, R. D., Martin, J. D., and Moncrief, N. D. (2018). Pen trial of estrogen-induced conditioned food aversion to eggs in raccoons (Procyon lotor). Appl. Anim. Behav. Sci. 201, 93–101. doi: 10.1016/j.applanim.2018.01.001
Eklund, A. (2021). Beeswarm: The Bee Swarm Plot, an Alternative to Stripchart (0.3.1.). Available online at: https://github.com/aroneklund/beeswarm (accessed September 03, 2021).
Eklund, A., López-Bao, J. V., Tourani, M., Chapron, G., and Frank, J. (2017). Limited evidence on the effectiveness of interventions to reduce livestock predation by large carnivores. Sci. Rep. 7, 1–9. doi: 10.1038/s41598-017-02323-w
Ellins, S. R., and Martin, G. C. (1981). Olfactory discrimination of lithium chloride by the coyote (Canis latrans). Behav. Neural Biol. 31, 214–224. doi: 10.1016/S0163-1047(81)91231-0
Elmahdi, E. M., Bullard, R. W., and Jackson, W. B. (1985). Calcium carbonate enhancement of methiocarb repellency for quelea. Trop. Pest Manag. 31, 67–72. doi: 10.1080/09670878509370949
Elton, C. (1954). “Research on rodent control by the bureau of animal population, September 1939 to July 1947,” in Control of Rats and Mice, ed D. Chitty (Oxford: Clarendon Press), 1–23.
Fenwick, S., Mikulka, P. J., and Klein, S. B. (1975). The effect of different levels of pre-exposure to sucrose on the acquisition and extinction of a conditioned aversion. Behav. Biol. 14, 231–235. doi: 10.1016/S0091-6773(75)90239-4
Ferguson, A. J., Thomson, R. L., Nelson-Flower, M. J., and Flower, T. P. (2021). Conditioned food aversion reduces crow nest predation: an improved framework for CFA trials. J. Nat. Conserv. 60:125970. doi: 10.1016/j.jnc.2021.125970
Ferreras, P., Díaz-Ruiz, F., and Monterroso, P. (2018). Improving mesocarnivore detectability with lures in camera-trapping studies. Wildl. Res. 45, 505–517. doi: 10.1071/WR18037
Foley, N. C., Jangraw, D. C., Peck, C., and Gottlieb, J. (2014). Novelty enhances visual salience independently of reward in the parietal lobe. J. Neurosci. 34, 7947–7957. doi: 10.1523/JNEUROSCI.4171-13.2014
Forthman, D. L., Strum, S. C., and Muchemi, G. (2005). “Applied conditioned taste aversion and the management and conservation of crop-raiding primates,” in Commensalism and Conflict: The Human-Primate Interface, Vol. 4, eds J. D. Paterson and J. Wallis (Norman, OK: American Society of Primatologists), 420–443.
Gabriel, P. O., and Golightly, R. T. (2014). Aversive conditioning of Steller's Jays to improve marbled murrelet nest survival. J. Wildl. Manage. 78, 894–903. doi: 10.1002/jwmg.725
Galef, B. G. (1985). Direct and indirect behavioral pathways to the social transmission of food avoidance. Ann. N. Y. Acad. Sci. 443, 203–215. doi: 10.1111/j.1749-6632.1985.tb27074.x
Galef, B. G. (1986). Social interaction modifies learned aversions, sodium appetite, and both palatability and handling-time induced dietary preference in rats (Rattus norvegicus). J. Comp. Psychol. 100, 432–439. doi: 10.1037/0735-7036.100.4.432
Galef, B. G. (1987). Social influences on the identification of toxic foods by Norway rats. Anim. Learn. Behav. 15, 327–332. doi: 10.3758/BF03205027
Galef, B. G., McQuoid, L. M., and Whiskin, E. E. (1990). Further evidence that Norway rats do not socially transmit learned aversions to toxic baits. Anim. Learn. Behav. 18, 199–205. doi: 10.3758/BF03205259
Galef, B. G., and Whiskin, E. E. (2000). Demonstration of a socially transmitted flavor aversion in rats? Kuan and Colwill (1997) revisited. Psychon. Bull. Rev. 7, 631–635. doi: 10.3758/BF03213000
Galef, B. G., Wigmore, S. W., and Kennett, D. J. (1983). A failure to find socially mediated taste aversion learning in Norway rats (R. norvegicus). J. Comp. Psychol. 97, 358–363. doi: 10.1037/0735-7036.97.4.358
Garcia, J., Ervin, F. R., and Koelling, R. A. (1966). Learning with prolonged delay of reinforcement. Psychon. Sci. 5, 121–122. doi: 10.3758/BF03328311
Garcia, J., Hankins, W. G., and Rusiniak, K. W. (1974). Behavioral regulation of the milieu interne in man and rat. Science 185, 824–831. doi: 10.1126/science.185.4154.824
Garcia, J., Kimeldorf, D. J., and Koelling, R. A. (1955). Conditioned aversion to saccharin resulting from exposure to gamma radiation. Science 122, 157–158.
Garcia, J., and Koelling, R. A. (1966). Relation of cue to consequence avoidance learning. Psychon. Sci. 4, 123–124. doi: 10.3758/BF03342209
Garcia, J., and Koelling, R. A. (1967). A comparison of aversions induced by x-rays, toxins, and drugs in the rat. Radiat. Res. Suppl. 7, 439–450. doi: 10.2307/3583736
Gaston, K. E. (1978). Brain mechanisms of conditioned taste aversion learning: A review of the literature. Physiol. Psychol. 6, 340–353. doi: 10.3758/BF03326736
Gentle, M., Massei, G., and Quy, R. (2006). Diversity of diet influences the persistence of conditioned taste aversion in rats. Appl. Anim. Behav. Sci. 97, 303–311. doi: 10.1016/j.applanim.2005.08.005
Gentle, M., Massei, G., and Saunders, G. (2004). Levamisole can reduce bait monopolization in wild red foxes Vulpes vulpes. Mamm. Rev. 34, 325–330. doi: 10.1111/j.1365-2907.2004.00049.x
Greggor, A. L., Berger-Tal, O., and Blumstein, D. T. (2020). The rules of attraction: the necessary role of animal cognition in explaining conservation failures and successes. Annu. Rev. Ecol. Evol. Syst. 51, 483–503. doi: 10.1146/annurev-ecolsys-011720-103212
Gustavson, C. R., Garcia, J., Hankins, W. G., and Rusiniak, K. W. (1974). Coyote predation control by aversive conditioning. Science 184, 581–583. doi: 10.1126/science.184.4136.581
Gustavson, C. R., Gustavson, J. C., and Holzer, G. A. (1983). Thiabendazole-based taste aversions in dingoes (Canis familiaris dingo) and New Guinea wild dogs (Canis familiaris hallstromi). Appl. Anim. Ethol. 10, 385–388. doi: 10.1016/0304-3762(83)90187-6
Gustavson, C. R., Jowsey, J. R., and Milligan, D. N. (1982). A 3-year evaluation of taste aversion coyote control in Saskatchewan. J. Range Manag. 35, 57–59. doi: 10.2307/3898519
Gustavson, C. R., Kelly, D. J., Sweeney, M., and Garcia, J. (1976). Prey-lithium aversions. I: coyotes and wolves. Behav. Biol. 17, 61–72. doi: 10.1016/S0091-6773(76)90272-8
Gustavson, C. R., and Nicolaus, L. K. (1987). “Taste aversion conditioning in wolves, coyotes, and other canids: retrospect and prospect,” in Man and Wolf: Advances, Issues, and Problems in Captive Wolf Research, ed H. Frank (Berlin: Springer Science and Business Media), 169–203.
Hämäläinen, L., Mappes, J., Rowland, H. M., Teichmann, M., and Thorogood, R. (2020). Social learning within and across predator species reduces attacks on novel aposematic prey. J. Anim. Ecol. 89, 1153–1164. doi: 10.1111/1365-2656.13180
Homstol, L. (2011). Applications of learning theory to human-bear conflict: The efficacy of aversive conditioning and conditioned taste aversion (MSc. thesis), University of Alberta, Edmonton, Canada.
Horn, S. W. (1983). An evaluation of predatory suppression in coyotes using lithium chloride-induced illness. J. Wildl. Manage. 47, 999–1009. doi: 10.2307/3808158
Indigo, N., Smith, J., Webb, J. K., and Phillips, B. (2018). Not such silly sausages: evidence suggests northern quolls exhibit aversion to toads after training with toad sausages. Austral Ecol. 43, 592–601. doi: 10.1111/aec.12595
Indigo, N., Smith, J., Webb, J. K., and Phillips, B. L. (2019). Bangers and cash: baiting efficiency in a heterogeneous population. Wildl. Soc. Bull. 43, 669–677. doi: 10.1002/wsb.1012
Jolly, C. J., Kelly, E., Gillespie, G. R., Phillips, B., and Webb, J. K. (2018). Out of the frying pan: reintroduction of toad-smart northern quolls to southern Kakadu National Park. Austral Ecol. 43, 139–149. doi: 10.1111/aec.12551
Kelly, E., and Phillips, B. L. (2019). Targeted gene flow and rapid adaptation in an endangered marsupial. Conserv. Biol. 33, 112–121. doi: 10.1111/cobi.13149
Kelly, E., Phillips, B. L., and Webb, J. K. (2018). Taste overshadows less salient cues to elicit food aversion in endangered marsupial. Appl. Anim. Behav. Sci. 209, 83–87. doi: 10.1016/j.applanim.2018.09.003
Kloppers, E. L., Cassady, C., Clair, S., and Hurd, T. E. (2005). Predator-resembling aversive conditioning for managing habituated wildlife. Ecol. Soc. 10, 31–49. doi: 10.5751/ES-01293-100131
Kuan, L. A., and Colwill, R. M. (1997). Demonstration of a socially transmitted taste aversion in the rat. Psychon. Bull. Rev. 4, 374–377. doi: 10.3758/BF03210795
Kuljis, B. A. (1986). Report on Food Aversion Conditioning in Sea Lions (Zalophus californianis). National Marine Fisheries Service, Southwest Fisheries Center. Available online at: https://swfsc-publications.fisheries.noaa.gov/publications/CR/1986/1986Kuljis.pdf (accessed March 19, 2021).
Land, C., Harrod, S. B., and Riccio, D. C. (1998). The interval between the CS and the UCS as a determiner of generalization performance. Psychon. Bull. Rev. 5, 690–693. doi: 10.3758/BF03208846
Landová, E., Hotová Svádová, K., Fuchs, R., Štys, P., and Exnerová, A. (2017). The effect of social learning on avoidance of aposematic prey in juvenile great tits (Parus major). Anim. Cogn. 20, 855–866. doi: 10.1007/s10071-017-1106-6
Lane, M. A., Ralphs, M. H., Olsen, J. D., Provenza, F. D., and Pfister, J. A. (1990). Conditioned taste aversion: potential for reducing cattle loss to larkspur. J. Range Manag. 43, 127–131. doi: 10.2307/3899029
Langley, W. M., and Knapp, K. (1982). Importance of olfaction to suppression of attack response through conditioned taste aversion in the grasshopper mouse. Behav. Neural Biol. 36, 368–378. doi: 10.1016/S0163-1047(82)90772-5
Latorre, L., Larrinaga, A. R., and Santamaria, L. (2013). Rats and seabirds: effects of egg size on predation risk and the potential of conditioned taste aversion as a mitigation method. PLoS ONE 8:e76138. doi: 10.1371/journal.pone.0076138
Lett, B. T. (1984). Extinction of taste aversion does not eliminate taste potentiation of odor aversion in rats or color aversion in pigeons. Anim. Learn. Behav. 12, 414–420. doi: 10.3758/BF03199988
Lin, J. Y., Arthurs, J., and Reilly, S. (2017). Conditioned taste aversions: from poisons to pain to drugs of abuse. Psychon. Bull. Rev. 24, 335–351. doi: 10.3758/s13423-016-1092-8
Lubow, R. E. (1989). Latent Inhibition and Conditioned Attention Theory. Cambridge: Cambridge University Press. doi: 10.1017/CBO9780511529849
Madden, F. (2004). Creating coexistence between humans and wildlife: Global perspectives on local efforts to address human-wildlife conflict. Hum. Dimens. Wildl. 9, 247–257. doi: 10.1080/10871200490505675
Maguire, G. S., Stojanovic, D., and Weston, M. A. (2009). Conditioned taste aversion reduces fox depredation on model eggs on beaches. Wildl. Res. 36, 702–708. doi: 10.1071/WR09123
Marshall, L., McCormick, W. D., and Cooke, G. M. (2019). Perception of the ethical acceptability of live prey feeding to aquatic species kept in captivity. PLoS ONE 14:e0216777. doi: 10.1371/journal.pone.0216777
Mason, J. R., Arzt, A. H., and Reidinger, R. F. (1984). Comparative assessment of food preferences and aversions acquired by blackbirds via observational learning. Auk 101, 796–803. doi: 10.2307/4086906
Mason, J. R., and Reidinger, R. F. (1982). Observational learning of food aversions in red-winged blackbirds (Agelaius phoeniceus). Auk 99, 548–554.
Mason, J. R., and Reidinger, R. F. (1983). Importance of color for methiocarb-induced food aversions in red-winged blackbirds. J. Wildl. Manag. 47, 383–393. doi: 10.2307/3808511
Massei, G., and Cowan, D. P. (2002). Strength and persistence of conditioned taste aversion in rats: evaluation of 11 potential compounds. Appl. Anim. Behav. Sci. 75, 249–260. doi: 10.1016/S0168-1591(01)00190-3
Massei, G., Lyon, A., and Cowan, D. P. (2003). Potential compounds for inducing conditioned taste aversion in ferrets. NZ. J. Zool. 30, 95–100. doi: 10.1080/03014223.2003.9518328
Matsuzawa, T., Hasegawa, Y., Gotoh, S., and Wada, K. (1983). One-trial long-lasting food-aversion learning in wild Japanese monkeys (Macaca-fuscata). Behav. Neural Biol. 39, 155–159. doi: 10.1016/S0163-1047(83)90791-4
Mauri, M., Elli, T., Caviglia, G., Uboldi, G., and Azzi, M. (2017). “RAWGraphs: a visualisation platform to create open outputs,” in Proceedings of the 12th Biannual Conference on Italian SIGCHI Chapter (Cagliari). doi: 10.1145/3125571.3125585
Mazur, R. L. (2010). Does aversive conditioning reduce human–black bear conflict? J. Wildl. Manag. 74, 48–54. doi: 10.2193/2008-163
McManus, J. S., Dickman, A. J., Gaynor, D., Smuts, B. H., and Macdonald, D. W. (2015). Dead or alive? Comparing costs and benefits of lethal and non-lethal human-wildlife conflict mitigation on livestock farms. Oryx 49, 687–695. doi: 10.1017/S0030605313001610
Miller, V., and Domjan, M. (1981). Specificity of cue to consequence in aversion learning in the rat: control for US-induced differential orientations. Anim. Learn. Behav. 9, 339–345. doi: 10.3758/BF03197841
Nachman, M. (1963). Learned aversion to the taste of lithium chloride and generalization to other salts. J. Comp. Physiol. Psychol. 56, 343–349. doi: 10.1037/h0046484
Nachman, M., and Ashe, J. H. (1973). Learned taste aversions in rats as a function of dosage, concentration, and route of administration of LiCl. Physiol. Behav. 10, 73–78. doi: 10.1016/0031-9384(73)90089-9
Navarro, M., Spray, K. J., Cubero, I., Thiele, T. E., and Bernstein, I. L. (2000). cFos induction during conditioned taste aversion expression varies with aversion strength. Brain Res. 887, 450–453. doi: 10.1016/S0006-8993(00)03032-8
Neves, V. C., Panagiotakopoulos, S., and Furness, R. W. (2006). A control taste aversion experiment on predators of roseate tern (Sterna dougallii) eggs. Eur. J. Wildl. Res. 52, 259–264. doi: 10.1007/s10344-006-0044-4
Nichols, M., Bell, P., Mulgan, N., and Taylor, A. (2020). Conditioned aversion in kea to cereal bait: a captive study using anthraquinone. Appl. Anim. Behav. Sci. 230:105077. doi: 10.1016/j.applanim.2020.105077
Nicolaus, L. K., Cassel, J. F., Carlson, R. B., and Gustavson, C. R. (1983). Taste-aversion conditioning of crows to control predation on eggs. Science 220, 212–214. doi: 10.1126/science.220.4593.212
Nicolaus, L. K., and Nellis, D. W. (1987). The first evaluation of the use of conditioned taste aversion to control predation by mongooses upon eggs. Appl. Anim. Behav. Sci. 17, 329–346. doi: 10.1016/0168-1591(87)90156-0
Nielsen, S., Travaini, A., Vassallo, A. I., Procopio, D., and Zapata, S. C. (2015). Conditioned taste aversion in the grey fox (Pseudalopex griseus), in Southern Argentine Patagonia. Appl. Anim. Behav. Sci. 163, 167–174. doi: 10.1016/j.applanim.2014.12.006
O'Donnell, S., Webb, J. K., and Shine, R. (2010). Conditioned taste aversion enhances the survival of an endangered predator imperilled by a toxic invader. J. Appl. Ecol. 47, 558–565. doi: 10.1111/j.1365-2664.2010.01802.x
Palmerino, C., Rusiniak, K., and Garcia, J. (1980). Flavor-illness aversions: the peculiar roles of odor and taste in memory for poison. Science 208, 753–755. doi: 10.1126/science.7367891
Parker, L. A. (2003). Taste avoidance and taste aversion: evidence for two different processes. Anim. Learn. Behav. 31, 165–172. doi: 10.3758/BF03195979
Pebsworth, P., and Radhakrishna, S. (2020). Using conditioned taste aversion to reduce human-non-human primate conflict: a comparison of four potentially illness-inducing drugs. Appl. Anim. Behav. Sci. 225:104948. doi: 10.1016/j.applanim.2020.104948
Phillips, R. B., and Winchell, C. S. (2011). Reducing non-target recaptures of an endangered predator using conditioned aversion and reward removal. J. Appl. Ecol. 48, 1501–1507. doi: 10.1111/j.1365-2664.2011.02044.x
Potter, D. A., and Held, D. W. (1999). “Absence of food-aversion learning by a polyphagous scarab, Popillia japonica, following intoxication by geranium, Pelargonium × hortorum,” in Proceedings of the 10th International Symposium on Insect-Plant Relationships (Dordrecht: Springer), 83–88. doi: 10.1007/978-94-017-1890-5_9
Prescott, M. J., Buchanan-Smith, H. M., and Smith, A. C. (2005). Social interaction with non-averse group-mates modifies a learned food aversion in single-and mixed-species groups of tamarins (Saguinus fuscicollis and S. labiatus). Am. J. Primatol. 65, 313–326. doi: 10.1002/ajp.20118
Price-Rees, S. J., Webb, J. K., and Shine, R. (2011). School for skinks: can conditioned taste aversion enable bluetongue lizards (Tiliqua scincoides) to avoid toxic cane toads (Rhinella marina) as prey? Ethology 117, 749–757. doi: 10.1111/j.1439-0310.2011.01935.x
Price-Rees, S. J., Webb, J. K., and Shine, R. (2013). Reducing the impact of a toxic invader by inducing taste aversion in an imperilled native reptile predator. Anim. Conserv. 16, 386–394. doi: 10.1111/acv.12004
Quick, D. L. F., and Hill, E. F. (1988). Sublethal landrin toxicity: behavioral and physiological effects on captive vultures. J. Wildl. Manag. 52, 233–237. doi: 10.2307/3801227
R Core Team (2020). R: A Language and Environment for Statistical Computing. Vienna: R Foundation for Statistical Computing.
Ratcliffe, J. M., Fenton, M. B., and Galef, B. G. (2003). An exception to the rule: common vampire bats do not learn taste aversions. Anim. Behav. 65, 385–389. doi: 10.1006/anbe.2003.2059
Read, J. L., Peacock, D., Wayne, A. F., and Moseby, K. E. (2016). Toxic trojans: can feral cat predation be mitigated by making their prey poisonous? Wildl. Res. 42, 689–696. doi: 10.1071/WR15125
Rescorla, R. A., and Wagner, A. R. (1972). “A theory of Pavlovian conditioning: variations in the effectiveness of reinforcement and non-reinforcement,” in Classical Conditioning II: Current Research and Theory (New York, NY: Appleton-Century-Crofts), 64–99.
Revillo, D. A., Castello, S., Paglini, G., and Arias, C. (2014). Reacquisition, reinstatement, and renewal of a conditioned taste aversion in preweanling rats. Dev. Psychobiol. 56, 713–725. doi: 10.1002/dev.21140
Revusky, S. H., and Bedarf, E. W. (1967). Association of illness with prior ingestion of novel foods. Science 155, 219–220. doi: 10.1126/science.155.3759.219
Roll, D. L., and Smith, J. C. (1972). “Conditioned taste aversion in anasthetized rats,” in Biological Boundaries of Learning, eds M. E. P. Sehgman, and J. L. Hager (New York, NY: Appleton-Century-Crofts), 98–102.
Rooke, I. J. (1984). Methiocarb-induced aversion to grapes by gray-backed white-eyes. J. Wildl. Manage. 48, 444–449. doi: 10.2307/3801176
Roper, T. J., and Marples, N. M. (1997). Odour and colour as cues for taste-avoidance learning in domestic chicks. Anim. Behav. 53, 1241–1250. doi: 10.1006/anbe.1996.0384
Rozin, P., and Kalat, J. W. (1971). Specific hungers and poison avoidance as adaptive specializations of learning. Psychol. Rev. 78, 459–486. doi: 10.1037/h0031878
Rusiniak, K. W., Hankins, W. G., Garcia, J., and Brett, L. P. (1979). Flavor-illness aversions: potentiation of odor by taste in rats. Behav. Neural Biol. 25, 1–17. doi: 10.1016/S0163-1047(79)90688-5
Rzóska, J. (1953). Bait shyness, a study in rat behaviour. Br. J. Anim. Behav. 1, 128–135. doi: 10.1016/S0950-5601(53)80011-0
Sánchez Rangel, L. (2019). Condicionamiento aversivo al sabor para manejo de conflictos con oso negro (Ursus Americanus Pallas, 1780) (MSc. thesis). Universidad Autónoma Nuevo Leon, San Nicolás de los Garza, Mexico. Available online at: http://eprints.uanl.mx/17647/ (accessed March 19, 2021).
Sayre, R. W., and Clark, L. (2001). Effect of primary and secondary repellents on European starlings: an initial assessment. J. Wildl. Manage. 65, 461–469. doi: 10.2307/3803098
Schaeffer, A. A. (1911). Habit formation in frogs. J. Anim. Behav. 1, 309–335. doi: 10.1037/h0070752
Schafe, G. E., Sollars, S. I., and Bernstein, I. L. (1995). The CS-US interval and taste aversion learning: a brief look. Behav. Neurosci. 109, 799–802. doi: 10.1037/0735-7044.109.4.799
Schier, L. A., Hyde, K. M., and Spector, A. C. (2019). Conditioned taste aversion vs. avoidance: a re-examination of the separate processes hypothesis. PLoS ONE 14:e0217458. doi: 10.1371/journal.pone.0217458
Shivik, J. A., Treves, A., and Callahan, P. (2003). Nonlethal techniques for managing predation: primary and secondary repellents. Conserv. Biol. 17, 1531–1537. doi: 10.1111/j.1523-1739.2003.00062.x
Smith, B. P., Snijders, L., Tobajas, J., Whitehouse-Teed, K., van Bommel, L., Pitcher, B., et al. (2021). “11: deterring and repelling wildlife,” in Ethical Wildlife Research in Australia, eds B. Smith, H. Waudby, and C. Alberthsen (Clayton: Csiro Publishing) (in press).
Smith, M. C. (1968). CS-US interval and US intensity in classical conditioning of the rabbit's nictitating membrane response. J. Comp. Physiol. Psychol. 66, 679–687. doi: 10.1037/h0026550
Smith, M. E., Linnell, J. D. C., Odden, J., and Swenson, J. E. (2000). Review of methods to reduce livestock depredation II. Aversive conditioning, deterrents and repellents. Acta Agric. Scand. A Anim. 50, 304–315. doi: 10.1080/090647000750069502
Sterner, R. T., and Shumake, S. A. (1978). Bait-induced prey aversions in predators: some methodological issues. Behav. Biol. 22, 565–566. doi: 10.1016/S0091-6773(78)92799-2
Ternent, M. A., and Garshelis, D. L. (1999). Taste-aversion conditioning to reduce nuisance activity by black bears in a Minnesota military reservation. Wildl. Soc. Bull. 27, 720–728.
Thorhallsdottir, A. G., Provenza, F. D., and Balph, D. F. (1990). Social influences on conditioned food aversions in sheep. Appl. Anim. Behav. Sci. 25, 45–50. doi: 10.1016/0168-1591(90)90068-O
Thorogood, R., Kokko, H., and Mappes, J. (2018). Social transmission of avoidance among predators facilitates the spread of novel prey. Nat. Ecol. Evol. 2, 254–261. doi: 10.1038/s41559-017-0418-x
Tobajas, J., Descalzo, E., Mateo, R., and Ferreras, P. (2020a). Reducing nest predation of ground-nesting birds through conditioned food aversion. Biol. Conserv. 242:108405. doi: 10.1016/j.biocon.2020.108405
Tobajas, J., Descalzo, E., Villafuerte, R., Jimenez, J., Mateo, R., and Ferreras, P. (2021a). Conditioned odor aversion as a tool for reducing post-release predation during animal translocations. Anim. Conserv. 24, 373–385. doi: 10.1111/acv.12643
Tobajas, J., Gómez-Ramírez, P., Ferreras, P., García-Fernández, A. J., and Mateo, R. (2020c). Conditioned food aversion in domestic dogs induced by thiram. Pest Manag. Sci. 76, 568–574. doi: 10.1002/ps.5548
Tobajas, J., Gómez-Ramírez, P., María-Mojica, P., Navas, I., García-Fernández, A. J., Ferreras, P., et al. (2019a). Selection of new chemicals to be used in conditioned aversion for non-lethal predation control. Behav. Process. 166:103905. doi: 10.1016/j.beproc.2019.103905
Tobajas, J., Gómez-Ramírez, P., María-Mojica, P., Navas, I., García-Fernández, A. J., Ferreras, P., et al. (2019b). Conditioned food aversion mediated by odour cue and microencapsulated levamisole to avoid predation by canids. Eur. J. Wildl. Res. 65:32. doi: 10.1007/s10344-019-1271-9
Tobajas, J., Ruiz-Aguilera, M. J., López-Bao, J. V., Ferreras, P., and Mateo, R. (2020b). The effectiveness of conditioned aversion in wolves: insights from experimental tests. Behav. Process. 181:104259. doi: 10.1016/j.beproc.2020.104259
Tobajas, J., Descalzo, E., Mateo, R., and Ferreras, P. (2021b). Using lures for improving selectivity of bait intake by red foxes. Wildl. Res. (in press). doi: 10.1071/WR21002
van Eeden, L. M., Crowther, M. S., Dickman, C. R., Macdonald, D. W., Ripple, W. J., Ritchie, E. G., et al. (2018). Managing conflict between large carnivores and livestock. Conserv. Biol. 32, 26–34. doi: 10.1111/cobi.12959
Wade, D. A. (1985). Brief comments on “coyote control and taste aversion.” Appetite 6, 268–271. doi: 10.1016/S0195-6663(85)80016-7
Ward-Fear, G., Pearson, D. J., Brown, G. P., Rangers, B., and Shine, R. (2016). Ecological immunization: in situ training of free-ranging predatory lizards reduces their vulnerability to invasive toxic prey. Biol. Lett. 12:20150863. doi: 10.1098/rsbl.2015.0863
Ward-Fear, G., Thomas, J., Webb, J. K., Pearson, D. J., and Shine, R. (2017). Eliciting conditioned taste aversion in lizards: live toxic prey are more effective than scent and taste cues alone. Integr. Zool. 12, 112–120. doi: 10.1111/1749-4877.12226
Werner, S. J., DeLiberto, S. T., Baldwin, R. A., and Witmer, G. W. (2016). Repellent application strategy for wild rodents and cottontail rabbits. Appl. Anim. Behav. Sci. 185, 95–102. doi: 10.1016/j.applanim.2016.10.008
Wilcoxon, H. C., Dragoin, W. B., and Kral, P. A. (1971). Illness-induced aversions in rat and quail: relative salience of visual and gustatory cues. Science 171, 826–828. doi: 10.1126/science.171.3973.826
Yasuda, Y., Kihara, T., and Nishimura, H. (1981). Effect of ethinyl estradiol on development of mouse fetuses. Teratology 23, 233–239. doi: 10.1002/tera.1420230208
Keywords: conditioned food aversion, conservation behavior, HWC, learned aversion, non-lethal management, predator control, wildlife management
Citation: Snijders L, Thierij NM, Appleby R, St. Clair CC and Tobajas J (2021) Conditioned Taste Aversion as a Tool for Mitigating Human-Wildlife Conflicts. Front. Conserv. Sci. 2:744704. doi: 10.3389/fcosc.2021.744704
Received: 20 July 2021; Accepted: 13 September 2021;
Published: 13 October 2021.
Edited by:
Joshua Plotnik, Hunter College (CUNY), United StatesReviewed by:
Cécile Sarabian, Kyoto University, JapanOded Berger-Tal, Ben-Gurion University of the Negev, Israel
Copyright © 2021 Snijders, Thierij, Appleby, St. Clair and Tobajas. This is an open-access article distributed under the terms of the Creative Commons Attribution License (CC BY). The use, distribution or reproduction in other forums is permitted, provided the original author(s) and the copyright owner(s) are credited and that the original publication in this journal is cited, in accordance with accepted academic practice. No use, distribution or reproduction is permitted which does not comply with these terms.
*Correspondence: Lysanne Snijders, lysanne.snijders@wur.nl