- 1Department of Biological Sciences, Macquarie University, Sydney, NSW, Australia
- 2NSW Department of Primary Industries, Fisheries, Sydney Institute of Marine Science, Sydney, NSW, Australia
Conservation measures often result in a “wicked problem,” i.e., a complex problem with conflicting aims and no clear or straightforward resolution without severe adverse effects on one or more parties. Here we discuss a novel approach to an ongoing problem, in which actions to reduce risk to humans, involve lethal control of otherwise protected species. To protect water users, nets are often used to catch potentially dangerous sharks at popular bathing beaches, yet in Australian waters one of the targeted species, the white shark (Carcharodon carcharias) is listed as Vulnerable, while bycatch includes the Critically Endangered grey nurse shark (Carcharias taurus). Recent, highly publicised, shark attacks have triggered demands for improved bather protection, whilst welfare and conservation organisations have called for removal of lethal measures. This leaves management and policy makers with a wicked problem: removing nets to reduce impacts on threatened species may increase risk to humans; or leaving the program as it is on the premise that the benefits provided by bather protection are greater than the impact on threatened and protected species. We used multivariate analysis and generalised additive models to investigate the biological, spatial-temporal, and environmental patterns influencing catch rates of threatened and of potentially dangerous shark species in the New South Wales shark nets over two decades to April 2019. Factors influencing catches were used to develop a matrix of potential changes to reduce catch of threatened species. Our proposed solutions include replacing existing nets with alternative mitigation strategies at key beaches where catch rate of threatened species is high. This approach provides stakeholders with a hierarchy of scenarios that address both social demands and threatened species conservation and is broadly applicable to human-wildlife conflict scenarios elsewhere.
Introduction
A human-wildlife conflict is characterised by animals posing a direct and recurrent hazard to the livelihood or safety of people, which in turn leads to the persecution of the species involved (IUCN SSC, 2021). These scenarios are frequently composed of a complex interplay of ecological, social, and climate factors, involved in forcing wildlife and people to co-occur and share limited resources (Abrahms, 2021). Human-wildlife conflict is a recurrent topic in conservation biology as management actions have implications for both the people and the animals involved (Dickman, 2010). While most research has generally approached the ecological and human dimensions of human-wildlife conflicts separately, identifying the linkages between both processes has been identified as a powerful strategy toward mitigation of these clashes (Teixeira et al., 2021). Decisions tend to be anthropocentric (i.e., favouring humans over animals), and mostly focused at current interests rather than considering its future significance for the people involved (Treves and Santiago-Ávila, 2020). While humans frequently underestimate or neglect risk from wild animals, we also show very little to no tolerance for it (Treves and Bruskotter, 2014). A risk-based approach to tackle human-wildlife conflicts means that decisions should be made based on the likelihood and consequence of these events, instead of focused on its probability of occurrence (Hudenko, 2012; Lischka et al., 2020).
Shark attacks on humans are the most global of all human-wildlife conflicts since they occur in all ocean basins and around all continents of the planet, bar Antarctica (Hardiman et al., 2019). They have received increasing attention worldwide following an apparent rise in the rate of incidents over the last three decades (Midway et al., 2019; Ryan et al., 2019). While shark attacks remain rare (~100 per year globally), they evoke powerful responses from both the public and government agencies as they often result in severe injuries or death (Sabatier and Huveneers, 2018). Approaches to mitigating shark-human interactions have historically relied on killing sharks in the area. Since 1937 this approach has used nets deployed off beaches to reduce local and/or transient populations of potentially dangerous sharks and thereby minimise the likelihood of interactions with people (Reid and Krogh, 1992; Dudley and Cliff, 2010; McPhee et al., 2021). However, a variety of non-targeted species are also bycaught in these programs (Krogh and Reid, 1996; Cliff and Dudley, 2011), raising community concerns about possible unintended broader ecosystem impacts.
Inevitably, management conundrums arise from addressing human-wildlife conflicts, which usually encompass ecological, economic, and socio-political groups with differing levels of decision-making power and values. As a result, proposed measures to address the conflict lead to resolutions satisfactory for some stakeholders yet to the detriment of others (Redpath et al., 2013; Bunnefeld et al., 2017). These are known as wicked problems because the complexity of these conflicts makes reaching ecologically desirable conservation outcomes challenging and precludes simple and well-defined solutions (Rittel and Webber, 1973). In a wicked problem, any proposed solution for the issue will generate a new, often different, problem, thus influencing any resolution of the solution and creating new difficulties (Game et al., 2014). Management needs to mitigate shark attacks are in many ways more driven by community fear of an incident than the actual risk, and provides management with a classic wicked problem, as nets deployed to protect bathers pose a threat to non-dangerous shark species and other marine taxa and their removal evokes strong, divergent public opinions.
The Shark Meshing Program (SMP) in New South Wales (NSW), Australia, is the longest-running initiative to reduce shark-human interactions in the world (Reid and Krogh, 1992). Claimed to be an affordable and effective practise to minimise shark peril (NSW Shark Menace Committee, 1929), it has run since 1937 and specifically targets three potentially dangerous sharks, white (Carcharodon carcharias), tiger (Galeocerdo cuvier), and bull (Carcharhinus leucas) sharks (Lee et al., 2018). It currently comprises 51 beaches and in a typical year, e.g., 2016–2017, captured 373 animals of which only 7.5% comprised target species (22 white, 3 tiger and 3 bull sharks) (Lee et al., 2018). The capture of species that are listed as threatened under NSW legislation, i.e., Fisheries Management Act 1994 (FM Act) and Biodiversity Conservation Act 2016 (BC Act); leads to a complex situation, with the program listed as a Key Threatening Process under both the FM Act and the BC Act because it threatens the survival of numerous threatened species, including white and grey nurse sharks (Carcharias taurus). Grey nurse sharks use predictable and well-documented aggregation sites, many of which have been given regulatory protection with site-specific fishing rules (Lynch et al., 2013). Even though the species has been protected since 1984 (Pollard et al., 1996), mortality arising from interactions with fishing gear appears to still be inhibiting their recovery (Robbins et al., 2013).
Mitigation of this Key Threatening Process could most readily be achieved by complete removal of nets, however, this may have perverse consequences. Beach goers and lifesavers are the people in the water exposed to potentially dangerous sharks, so they will be directly affected by any changes made to the program by the NSW state government and are therefore key stakeholders in any decision-making process. Beach authorities such as life savers are inevitably the first responders at shark attacks and, in fact, were the primary motivators for shark nets to be deployed in NSW following spates of shark-related severe injuries and fatalities (Conrick, 1989). Similarly, local councils who administer the facilities and beaches where nets are placed, receive substantial income from visitors using these amenities and are therefore important stakeholders. Although shark nets have reduced shark attacks by 88–91% in regions that they have been deployed (Dudley, 1997), there is substantial bycatch of harmless marine animals (Krogh and Reid, 1996; Cliff and Dudley, 2011; Dalton and Peddemors, 2018). Environmentally conscious public and organisations are therefore also important stakeholders in determining the future of shark nets. However, if nets were removed wholesale, even a single human fatality at a previously meshed beach, could trigger a negative public and political reaction, which may lead to calls to immediately reinstate the program and/or for renewed culling (Pawle, 2017) with possible adverse consequences for threatened species. This leads to the SMP falling into the category of a wicked problem, as the primary target species of the program, white sharks, are themselves a threatened species. While government and legislators are tasked with protecting this species to ensure their survival, they are simultaneously required to protect people from this protected species. Protection of human life is the highest priority for government, mandated by multiple regulations and legislation, yet retention of the status quo is inconsistent with conservation legislation that aims to abate, ameliorate, or eliminate the adverse effects of Key Threatening Processes.
Resolving wicked problems requires ingenuity combined with clear evidence of drivers of conflict (Guerrero et al., 2017; Mason et al., 2018), and an educated and supportive public and political community. Currently there are several alternatives to shark nets (McPhee et al., 2021), however, these might be more expensive and/or labour intensive and are not necessarily supported by the general public (Simmons and Mehmet, 2018). Replacing nets by more selective fishing gear such as a series of anchored buoys with baited hooks, known as “drumlines,” on which the hooked shark fights to fatal exhaustion, has been previously found to be an effective strategy to reduce the impacts of mitigation programs while still promoting beach safety (Cliff and Dudley, 2011). However, none of the available mitigation strategies can completely stop sharks from using a particular area (Guyomard et al., 2020) and the presence of potentially dangerous sharks might not necessarily equate to risk of shark attack. Here we use 22 years of empirical data on catch rates of sharks in the SMP to identify trends in capture of non-targeted threatened (i.e., grey nurse sharks) and targeted (i.e., white, tiger, and bull sharks) species and localised drivers of shark presence. We use the model outcomes to predict how possible changes in the operation of the SMP may affect both shark conservation and human safety. Our main objective is to reduce captures of grey nurse and white sharks for conservation purposes while maintaining low risk of shark interactions to humans. However, this is complicated by white sharks being one of the potentially dangerous species, wherein lower captures of this species could potentially lead to a higher risk of shark bites. By proposing a hierarchy of alternatives to shark nets, focused on the locations with higher captures of threatened species, we provide stakeholders with information by which to choose relative changes for threatened species conservation vs. perceived risk of shark bite. The methods adopted in this study may be applicable for addressing other human-wildlife conflict scenarios elsewhere.
Materials and Methods
Shark Meshing Program and Catch Data
The study area comprises ~190 km of the 2,137 km NSW coastline (Figure 1) that encompass three major coastal cities and a total of 51 beaches with a single shark net set off each beach. Shark nets are 150 m long and 6 m in height and set in the bottom half of the water column on the sandy seabed approximately 500 m offshore in 10–12 m depth water (Krogh and Reid, 1996). Catch data used for analyses was provided directly by the NSW government to the research team and spanned 22 years from 1998 to 2019. Throughout this time, nets were only in the water for 8 months of the year (01 September to 30 April) as they are removed over winter to reduce potential whale entanglements during historically low months of beach use by swimmers, albeit wetsuits enable surfers to surf year-round. During the first 11 years of sampling, i.e., 1998–2008 (hereafter Period 1), nets were deployed for a minimum of 9 weekdays and every weekend per month. During this period, the maximum time that nets could fish without being checked was 4 days (96 h). From January 2009 onwards (hereafter referred as Period 2) nets were continuously in the water, also from September to April, but checked every 72 h as an effort to reduce mortality of captured individuals (Reid et al., 2011). Detailed descriptions of the SMP methodology can be found in Reid et al. (2011). Live sharks were sexed, measured to fork length and released. Maturity of sharks was determined using published sex-specific maturation sizes (Kohler et al., 1996; Lucifora et al., 2002; Cruz-Martínez et al., 2005; Holmes et al., 2015).
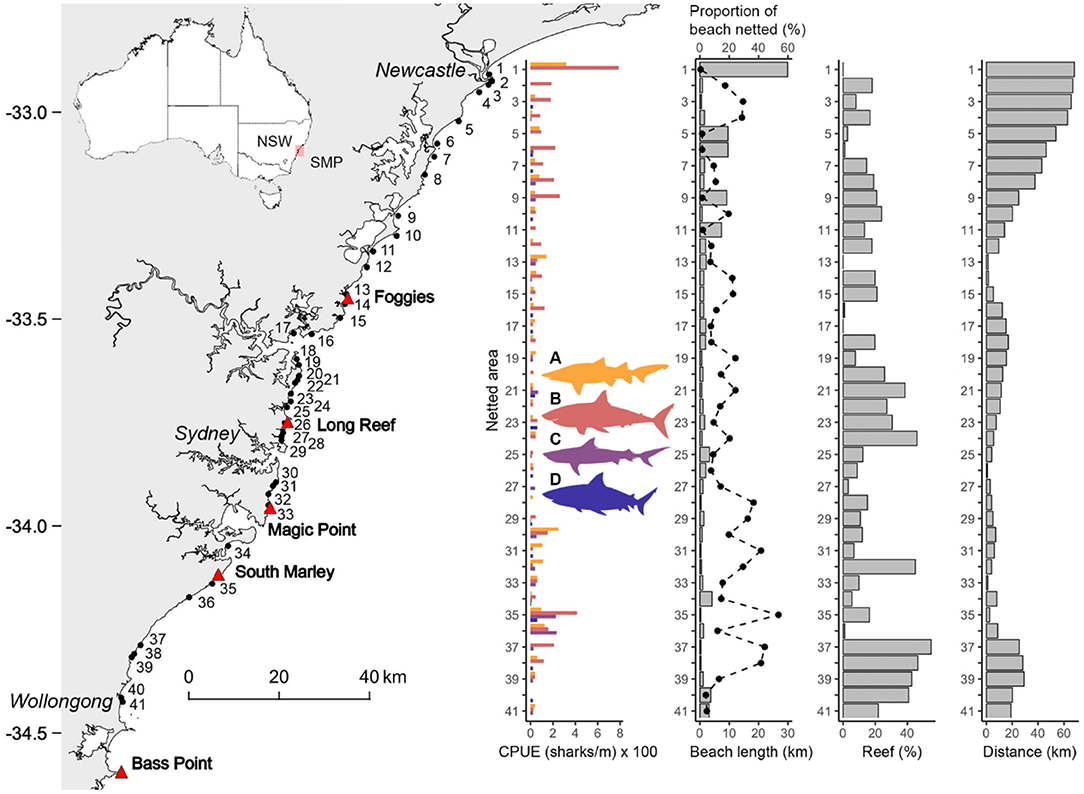
Figure 1. Map of the area covered by the Shark Meshing Program (SMP) showing the locations of the known aggregation sites of grey nurse sharks (triangles) and the 41 netted areas (circles), including total catch-per-unit-of-effort (CPUE) of (A) grey nurse, (B) white, (C) tiger, and (D) bull sharks between September 1998 and April 2019. The distributions of the physical parameters beach length, proportion of beach netted, percentage of reef area (Reef), and their corresponding distance from the nearest grey nurse shark aggregation site (Distance) are also represented.
Among the 51 beaches meshed within the SMP several are in close proximity. To avoid bias in catch data, we grouped nets that were within 1 km of each other into a single netted area, producing 41 independent netted areas for our analyses (Figure 1). This grouping resulted in uneven effort, e.g., areas with two or more nets vs. single net areas (Supplementary Table 1). Therefore, fishing effort was standardised to metres of net per fishing day (i.e., 4-day for Period 1 and 3-day for Period 2).
Total catch data comprised only the days in which a net was checked and at least one individual from any of the four shark species captured, so we conducted a second standardisation for analysing fine-scale species-specific catch rates similar to Lee et al. (2018). As nets were checked on Monday/Tuesday and Friday prior to 2009, fine-scale effort (i.e., actual days with fishing effort) could not be accurately determined for Period 1. From 2009, cheques occurred every Monday, Wednesday, and Friday, enabling subdivision of Period 2 into three weekly time intervals (Monday and Tuesday; Wednesday and Thursday; Friday to Sunday) of fishing effort. Catch data were matched using reported dates of capture. All catches of the four shark species are included in the analysis as mortality rates of target sharks in the nets are 95% for Period 1 and 86% for Period 2 and, although live sharks are tagged and released, post-release survivorship is unknown (Dalton and Peddemors, 2018). The impacts of the SMP were only assessed for the two threatened shark species captured, i.e., grey nurse and white sharks.
Physical and Environmental Variables
Seasonality in SMP catches was related to species-specific migratory patterns along the east coast of Australia using the 8 months of fishing effort. The influence of physical characteristics of netted areas influencing shark captures in the nets were assessed using: (i) proportion of each beach that is covered by a net (net:beach) (%); (ii) percentage of rocky reef cover within a 1 km2 quadrat centred on net location, downloaded from the NSW Government database (NSW Government, 2019); and (iii) distance to nearest known grey nurse shark aggregation site (Figure 1) to assess whether the species would be more vulnerable to capture in the nets deployed near to where these aggregations occur (Barker and Williamson, 2010). The environmental influence of sea surface temperature (SST; °C) was also tested (Wintner and Kerwath, 2018). Daily values of SST with 0.02° spatial resolution were downloaded from the Australian Ocean Data Network Portal (AODN, 2019) and used to calculate mean values per set during Period 2. The nearest quadrats of data available from the corresponding netted areas were used. To standardise habitat of netted areas with multiple net-sets, a 1 km2 quadrat was created around the nets for each area. The percentage of reef area covered in this polygon and distance from its midpoint to the nearest grey nurse shark aggregation site, were then included in the analysis. All physical variables were calculated using QGIS software (version 3.4.0).
Shark Occurrence Patterns
Data spanning the entire study period was investigated using a partial redundancy analysis (RDA). Each species was divided into sex-specific groups and maturation status prior to analysis. To account for the differences in net checking regimes between Period 1 and Period 2, a new categorical variable named SMP-period divided shark catches into before and after 2009. The RDA full model also included Area as a geographic component, given SMP distribution is effectively latitudinal (Figure 1), and the corresponding physical parameters of netted areas.
Zero-inflated generalised additive models (ZIGAM) were used to model shark fine-scale occurrence patterns. Models were calculated individually for each species, and the logarithm of fishing effort included as an offset covariate. Groups of continuous candidate predictor variables included: (i) spatial-temporal: netted area and month and (ii) environmental: SST, proportion of beach netted, proportion of reef area cover, and distance to nearest grey nurse shark aggregation site. Interactions between variables were tested to investigate whether complex relationships would better explain shark catch in the SMP. Multicollinearity was assessed with Pearson's correlations. Total number of sharks caught per net deployment were included as response variables using zero-inflated Poisson families of error distribution. Starting from the null models, new variables were gradually included to identify lower AIC values (Wagenmakers and Farrell, 2004). Final models were inspected for normal residual distribution.
Addressing the Wicked Problem
To determine whether alternatives to shark nets could assist in shark conservation whilst still affording protection from being bitten by a shark, differences in captures of each species were investigated considering potential scenarios of removing particular netted areas from the SMP. For this purpose, the significant variables found to influence catches of the threatened species (i.e., grey nurse and white sharks) or modifying the netted areas with the highest captures of grey nurse sharks, were used. The values of each covariate responsible for a higher likelihood of capture were used to estimate reductions in catch rates for each species. Each scenario is hereafter referred to as potential changes, and the amount of catch reduction per species compared using ANOVA with post-hoc Tukey tests.
The effects of each potential change upon vulnerability to capture for each shark species were compared based on productivity and susceptibility scores, which are continuous indices ranging from zero to three. This approach is similar to what is used in fisheries research to assess stock vulnerability to becoming overfished (Stobutzki et al., 2001; Patrick et al., 2009; Gallagher et al., 2014). The productivity score has a biological meaning and relates to the capacity of a shark species to produce maximum sustainable yields and to recover if its population is being depleted (Patrick et al., 2009), whereas here the susceptibility score relates to the potential of a species to be impacted by their captures in the SMP. Species-specific productivity scores were obtained from Patrick et al. (2009). Susceptibility scores were calculated based on the average of the productivity score and the following attributes: (i) seasonal migrations: number of months present within the SMP region in relation to total meshing period, i.e., months captured against months with SMP effort; (ii) spatial overlap: overall area used when present in relation to the total 41 meshed areas, i.e., overlap between northernmost and southernmost locations for each species from telemetry studies and the SMP net deployments; (iii) mortality: number of alive vs. dead sharks from each species in the SMP since 2009 (i.e., the last modification of the program); (iv) desirability: 0 = non-targeted and 3 = targeted; (v) management strategy: 0 = no recovery plan in place in Australian waters and 3 = recovery plan in place in Australian waters; (vi) SMP impact: 0 = SMP is not a Key Threatening Process and 3 = SMP is a Key Threatening Process; and (vii) population impact: maximum number of individuals caught per year in the SMP in relation to the East Australian population of the species (Supplementary Table 2). The attributes were first calculated as percentages from either the SMP or published data (Lincoln-Smith and Roberts, 2010; Otway and Ellis, 2011; Tillett et al., 2012; Holmes et al., 2017; Hillary et al., 2018; Bruce et al., 2019; Lee et al., 2019), and then standardised to the same 0–3 scale used in Patrick et al. (2009) for comparison purposes. The catch reduction rates for each species from potential changes to the SMP were similarly converted to this scale in order to be also included as attributes for the final vulnerability scores, calculated using the formula:
In which X is the susceptibility score for the species in the SMP, Y is the scaled catch reduction rate, and Z is the percentage fishing effort reduction (i.e., number of nets removed from the current total 51 nets in the SMP) for the corresponding potential change (Supplementary Table 2). The significance level of the vulnerability score variations as a function of the potential changes were assessed using ANOVA.
A wicked problem matrix was developed for our case study of the SMP, providing a series of hypothetical potential changes that could be implemented to the program. These ranged from leaving the status quo, through to selective removal of nets potentially combined with alternative mitigation options, to completely removing all shark nets without replacement with any other mitigative measures. Expected consequences of potential actions for some key stakeholder groups involved in the SMP context (Fraser-Baxter and Medvecky, 2018; Simmons and Mehmet, 2018; SharkSmart NSW, 2021) and for the shark species were graded from low to high resistance and risk.
Five main stakeholder groups were identified: (i) Non-Government Organisations (NGO): supporting conservation through non-lethal measures, (ii) beach goers: people directly affected by any changes to the SMP, (iii) lifesaving associations (volunteer and professional): tasked with protecting beach goers and inevitably, the first responders in the case of a shark attack, (iv) local councils: potential beneficiaries of shark bite mitigation measures, and (v) NSW government: through the Department of Primary Industries Fisheries, is the government agency that administers the SMP. Five groups of primary actions were identified: (i) status quo: leaving the program as is, (ii) selective removal: removing nets as identified in Table 1, (iii) selective replacement: replacing some nets with alternatives, (iv) total replacement: fully removing nets and replacing with alternatives, and (v) completely removing any shark bite mitigation measures (Table 2). Alternatives were identified through stakeholder questionnaires as being the most strongly supported alternative measures as described in the current literature (Crossley et al., 2014; Gray and Gray, 2017; Simmons and Mehmet, 2018). These include Shark Management Alert in Real-time (SMART) drumlines (Guyomard et al., 2019; Tate et al., 2019), traditional drumlines (Sumpton et al., 2011; McPhee et al., 2021), helicopters (Crossley et al., 2014), drones (Butcher et al., 2019), listening stations (VR4Gs) for detecting tagged sharks and informing the public through smartphone apps in real-time (Simmons and Mehmet, 2018), and different combinations of these methods (Table 2). The alternatives are not an exhaustive list but examples of approaches that could be adopted based on current use in Australia and elsewhere (McPhee et al., 2021).
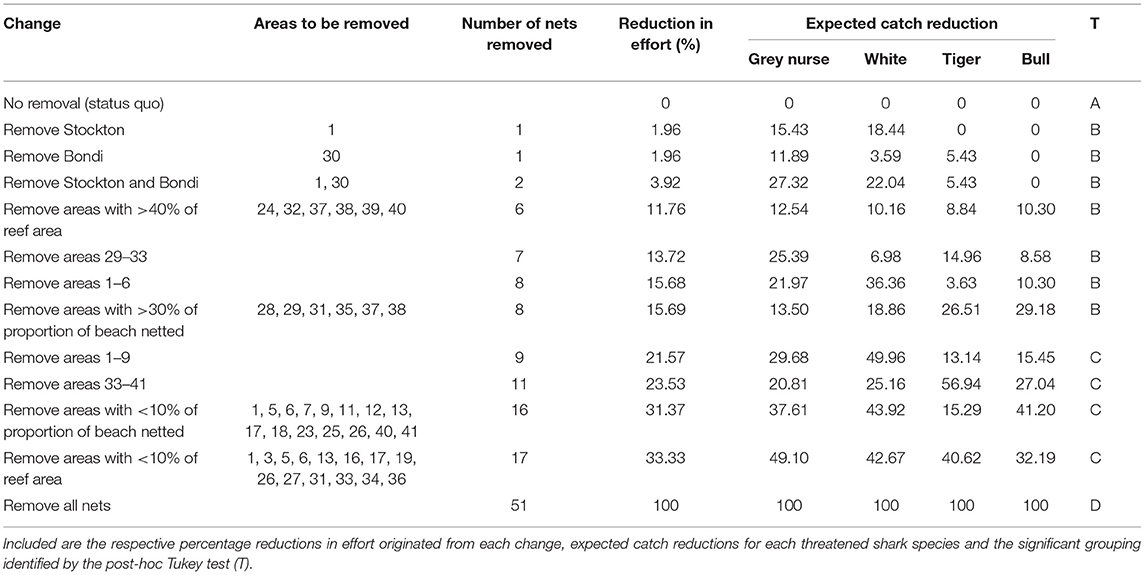
Table 1. Potential changes to the Shark Meshing Program fishing effort, based on the significant variables identified by the fine-scale modelling approach.
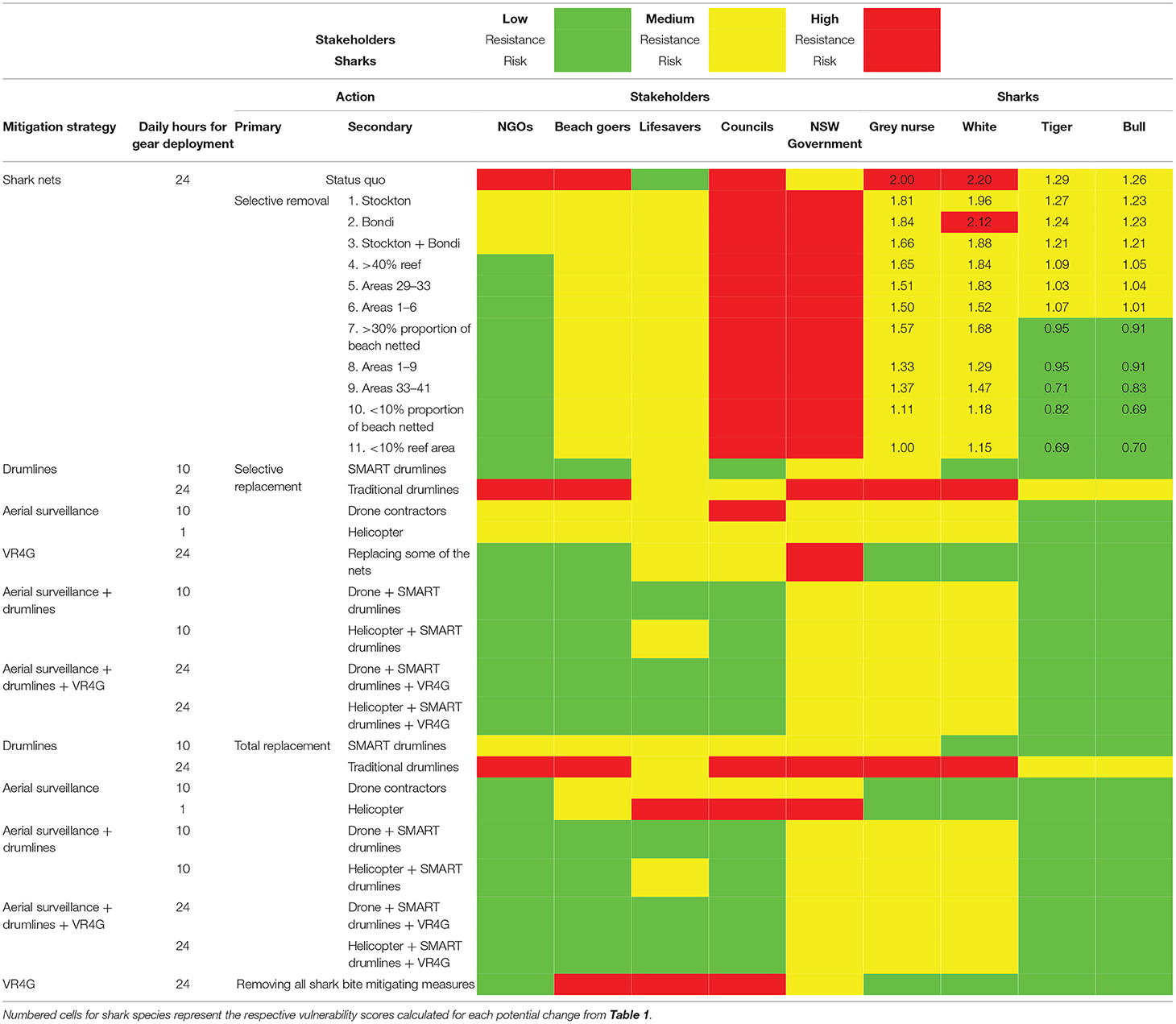
Table 2. Wicked problem matrix originated from the Shark Meshing Program, comprising a series of possible actions to the program and the expected outcomes for each stakeholder group and consequent risk for the shark species caught.
For the proposed changes, resistance levels for stakeholder groups were graded according to a traffic light scale based on results from open and targeted online questionnaires and forums, recently developed as part of the community engagement and social research by the NSW Department of Primary Industries, to better understand the opinions and attitudes of people toward coexisting with sharks (SharkSmart NSW, 2021; Simmons et al., 2021). Since the vulnerability scores calculated for each species are also returned using a traffic light scale, and directly included the corresponding scaled catch reduction rates of each proposed change, these were employed for the grading system of risk for sharks. Finally, the risk level of alternative mitigation strategies to each shark species were based on the literature as to whether they could lead to higher catch rates (i.e., high risk), lower catch rates (i.e., low risk). When information was not available and could not be fully determined it was scored as a medium risk. The significance levels were set at 0.05 and all statistical analyses were performed in R (version 4.0.5).
Results
Total Shark Catch
A total of 106 grey nurse, 217 white, 48 tiger, and 22 bull sharks were confirmed as caught during the study. Bull sharks were historically included in a “whaler shark” category and have only been identified to species level since 2010 (Lee et al., 2019), partly explaining the lower numbers caught in comparison to the other species, therefore bull shark species-specific data were only available for Period 2. More sharks were caught during Period 2 (72.5%). The total number of sharks found alive when nets were checked, and subsequently released, increased from 12.0% during Period 1–40.3% in Period 2. The total bull shark catch was low but equally distributed along the SMP region (Figure 1). Captures for the other species were widespread throughout the SMP region with localised peaks (Figure 1). Captures of grey nurse sharks were highest at Stockton Beach (Area-1; 0.7 sharks/year), followed by Bondi (Area-30; 0.5 sharks/year). Terrigal Beach (Area-13) was the only location near a known grey nurse shark aggregation site (0.7 km) with slightly higher captures of the species (Figure 1) but it still only had an average catch rate of 0.3 sharks/year. White shark catch was also highest at Stockton Beach (1.6 sharks/year), followed by Wattamolla (Area-35; 0.8 sharks/year) (Figure 1). Tiger sharks were mostly caught at Wattamolla (0.4 sharks/year) and Garie (Area-36; 0.5 sharks/year) beaches (Figure 1).
Catches of grey nurse sharks were dominated by females (female:male = 5.93; Figure 2A). More even sex ratios were observed for white (female:male = 1.14; Figure 2B), tiger (female:male = 1.09; Figure 2C), and bull (female:male = 1.71, Figure 2D) sharks. While most grey nurse, tiger, and bull sharks caught were mature, white sharks were almost entirely juveniles with only 5 (2.3%) mature sharks caught during the entire study period (Figure 2). According to Dudley and Cliff (2010), bull sharks larger than 175.5 cm fork length are capable of severe tissue removal and should be considered as potentially hazardous to humans. Most white, tiger, and bull sharks caught in the SMP were indeed larger than 175.5 cm fork length (Figure 2).
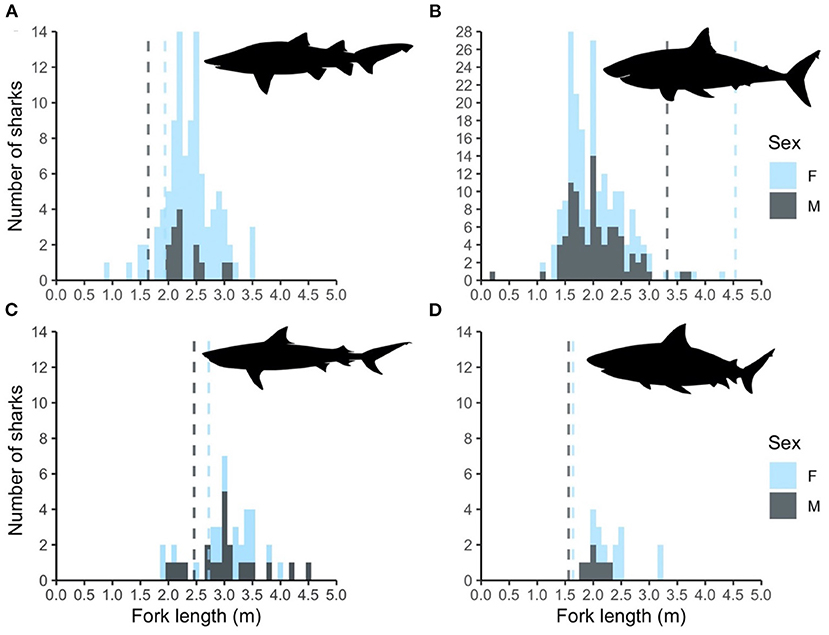
Figure 2. Histogram of fork length distribution of the (A) grey nurse, (B) white, (C) tiger, and (D) bull sharks caught in the Shark Meshing Program between 1998 and 2019 by sex. Dashed lines represent sex-specific maturation sizes for each of the species obtained from the literature.
Broad-Scale Occurrence Patterns
The final RDA model revealed that the variables Maturation Status (F = 272.8; p = 0.005), month (F = 13.9; p = 0.005), SMP-period (F = 9.7; p = 0.005) and Area (F = 2.5; p = 0.041) significantly influenced the total shark catches in the SMP (variance explained = 46.8%). While most groups showed similar trends, the patterns of female grey nurse, and male white and tiger sharks varied significantly (Figure 3). Female grey nurse sharks were predominantly adult and occurred more frequently during Period 2 (Figure 3). Similarly, male juvenile white sharks were more frequent during Period 2, but also during the first months of netting and in the northern area of the SMP (Figure 3). By contrast, male tiger sharks were caught toward the end of the netting season, especially during Period 1 (Figure 3).
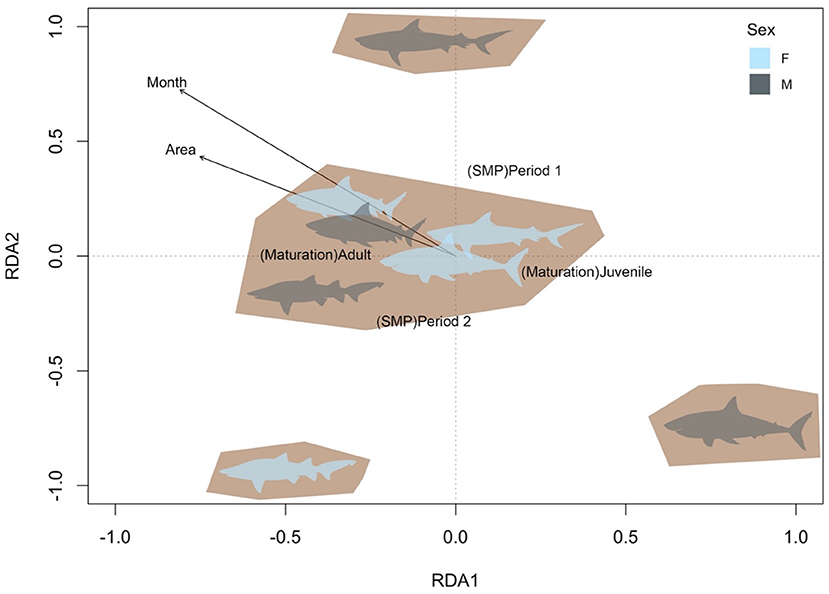
Figure 3. RDA triplots (first two axes from the redundancy analysis RDA1 and RDA2) of the total catch of female and male (colour legend) grey nurse, white, tiger and bull sharks in the Shark Meshing Program (SMP) between 1998 and 2019 according to the significant groupings identified (shaded areas) and constrained by the corresponding significant variables maturation status (Maturation), month, SMP period (SMP) and netted area (Area). Arrows represent the positive increments for the continuous variables (month and area).
Fine-Scale Occurrence Patterns
The capture of grey nurse sharks in the nets was influenced by interactions between netted area and month (deviance explained = 48.0%) and SST and proportion of reef substrate (84.6%) (Supplementary Tables 3, 4). Captures of grey nurse sharks had a northerly peak between September and January and a southerly peak in September (Figure 4A). The southern peak occurred between the aggregation sites of Long Reef and Magic Point, yet over 20 years only seven grey nurse sharks were caught at Area-13 (Terrigal Beach, 1.4 km from Foggies aggregation site) and three at Area-33 (Maroubra Beach, 1.3 km from Magic Point). Significantly lower grey nurse shark captures occurred between the aggregation sites of Foggies and Long Reef, particularly from January to April (Figure 4A). Overall, distance to nearest aggregation site did not significantly influence captures of grey nurse sharks in the SMP (Supplementary Tables 3, 4). However, captures of the species were related to cooler SSTs (<20°C) and exhibited a bi-modal topographic pattern, either more than 40% reef cover or when the seabed comprised <10% reef cover (i.e., sandy substrate) (Figure 4B).
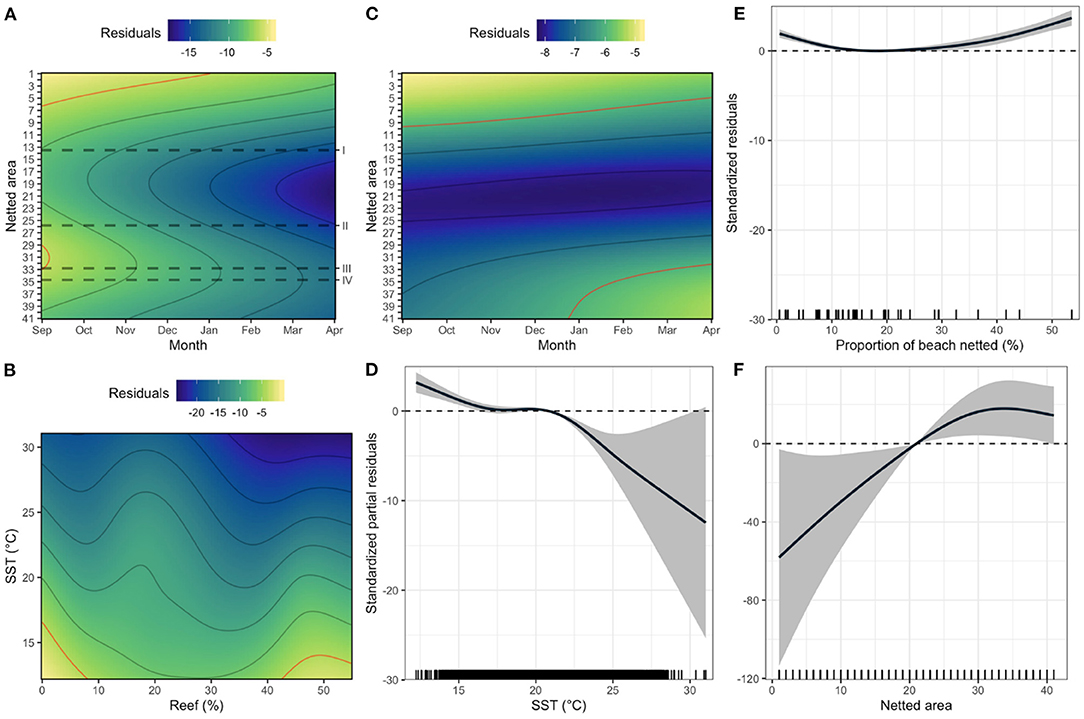
Figure 4. Fine-scale zero-inflated generalised additive models of (A,B) grey nurse, (C–E) white, and (F) tiger shark occurrence in the Shark Meshing Program catches. Spatial-temporal effects of netted area and month (A,C), and (B) sea surface temperature (SST) and percentage of reef area (Reef), and the individual effects of (D) SST, (E) proportion of beach netted, and (F) netted area. Colour scales, shaded areas and solid and dashed lines (D–F), respectively, represent the respective model residuals, the 95% confidence intervals, the raw data and the null effects. The red isolines (A–C) represent the highest residual contours for the corresponding models. Dashed lines (A) depict the grey nurse shark aggregation sites (I = Foggies, II = Long Reef, III = Magic Point, IV = South Marley).
White shark occurrence was influenced by the interaction between netted area and month (46.1%) and the environmental variables SST and proportion of beach netted (84.9%) (Supplementary Tables 3, 5). White shark catches exhibited a northerly peak between September and December and a southerly peak between January and April (Figure 4C). White sharks were more frequent when temperatures were cooler than 20°C (Figure 4D) particularly in areas with proportion of beach netted <10% and >30% (Figure 4E). Tiger shark catches were influenced by netted area (35.7%) (Supplementary Tables 3, 6), being mostly caught south of netted area 20 (Figure 4F).
Potential Changes to the SMP
We identified 12 scenarios where modifying the SMP could reduce captures of threatened sharks. These were divided into four significant groups according to the Tukey testing (Table 1). Removing all nets would reduce catches of grey nurse by 5.3 (±6.2) and white sharks by 9.8 (±7.3) sharks/year. It would, however, also reduce catches of tiger sharks by 2.5 (±1.3) and bull sharks by 2.4 (±1.8) sharks/year. On the other hand, removing nets from just Stockton, Bondi or both beaches (effort reductions between 2 and 4%; Tukey B) would lead to similar reductions to that achieved by reducing effort by 12–16% through removal of up to eight nets (Table 1). Stockton (3.5 ± 2.2 sharks/year) and Bondi (1.7 ± 0.8 sharks/year) beaches accounted for ~30% of all grey nurse shark catches. Removing nets solely from Stockton would result in higher reductions in grey nurse shark catch and also lower the capture of white sharks, while removing Bondi alone would have a much less pronounced effect on white sharks (Table 1). Removing more nets (22 to 33% effort reduction; Tukey C) could reduce grey nurse shark captures by a maximum ~50% (Table 1), but it would also greatly reduce captures of potentially dangerous sharks (Table 1), thereby feasibly increasing risk of shark bite if no alternative risk reduction measures are implemented.
The Wicked Problem Scenario
The potential changes would effectively reduce vulnerability scores for all species (F = 37.91; p < 0.001) (Figure 5). The status quo would maintain current impacts. At the other extreme, totally removing mitigation measures would completely alleviate impacts from the SMP (Table 2; Figure 5). However, this might increase bather vulnerability, inviting a backlash if serious injuries or fatalities followed. Intermediate options include switching from nets to using more selective, or less lethal, fishing methods, but how this might influence catch rates is unknown as comparable data is not available.
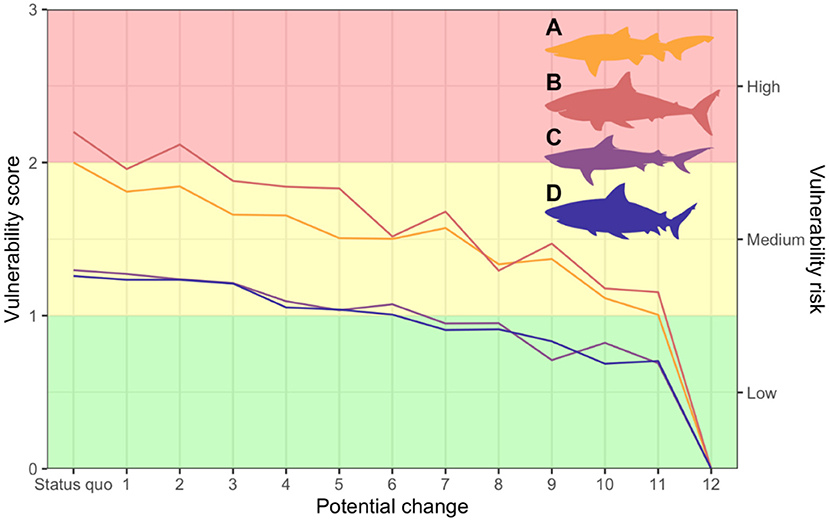
Figure 5. Variation in vulnerability scores for each shark species (A = grey nurse; B = white; C = tiger; D = bull) as a function of the potential changes identified (Table 2). Background colours separate the total vulnerability score range into three groups according to the same “traffic light” pattern for vulnerability risk (green = low; yellow = medium; red = high) used for the wicked matrix (Table 2).
Conventional drumlines that are operated in a manner that generally kills sharks are unpopular with the public (Table 2) (Gibbs and Warren, 2014). SMART drumlines significantly reduce mortality by providing a means of promptly responding to a catch (Guyomard et al., 2019). Selective replacement with SMART drumlines at specific beaches was the most acceptable modification to the SMP across all stakeholder groups (Table 2). However, many beaches are located further than the ~15 km required to reach SMART drumlines and successfully release animals alive (Tate et al., 2019). Removal and replacement of the northern-most beach, Stockton, provides the most conservation outcome for the least reduction in effort, but simultaneously reduces the catch of what is arguably the primary target species of the SMP (i.e., white sharks), thus emphasising the wicked nature of this problem (Table 1; Figure 4). Stockton is adjacent to a port and outside of flight restriction zones, hence alternative mitigation measures could include SMART drumlines and drones (Simmons and Mehmet, 2018). Similarly, replacement of the Bondi net alone would provide substantial benefits to grey nurse sharks (Table 1).
Discussion
We developed a hierarchical decision matrix of potential actions, based on catch trends, to reduce impact on threatened species whilst supporting bather safety in NSW (Tables 1, 2). The conflict-to-coexistence continuum proposes that strategies to address human-wildlife conflicts should consider all possible actions from negative (i.e., with higher impacts for the animals) to positive (i.e., excluding risk for animals), and the intermediate more practicable solutions focused on coexistence (Frank, 2016). Our analysis allows stakeholders to apply science-based decision-making processes to formulate strategies for this human-wildlife conflict of a wicked problem incorporating threatened and target species.
Shark Occurrence Patterns
Climate change is increasing the frequency and severity of human-wildlife conflicts by forcing animals and people to share increasingly scarce resources (Abrahms, 2021). Most of the shark catches in the SMP occurred in more recent years (i.e., Period 2). While this could be associated with a higher fishing effort (i.e., nets spending more time in the water during Period 2 compared to Period 1), it may also be related to changes in distributions of potentially dangerous sharks along the East coast of Australia in response to climate change (Niella et al., 2020).
The nearshore occurrence of both threatened shark species increases during periods of water temperatures below 20°C (Lee et al., 2018), particularly in rocky reef and sandy bottoms areas. Our findings corroborate catches of grey nurse sharks in the South African bather protection program (Wintner and Kerwath, 2018), with increased catch apparently driven by cold water upwellings (Roughan and Middleton, 2002; Oke and Griffin, 2011), particularly in the north of the SMP area (Everett et al., 2014). Juvenile white sharks occupy this region as part of their nursery (Bruce and Bradford, 2008, 2012) suggesting increased catch may be related to their exploration of their nursery area. Grey nurse sharks migrate southwards during the spring-summer period (Otway and Ellis, 2011).
White sharks were more frequent in meshed areas with higher proportions of beach covered by the SMP nets (proportion of beach netted > 40%). This, combined with higher occurrence during productive cold-water periods, suggests that when following their prey into the shallows these sharks become more vulnerable to capture. Even though proportion of beach netted was not a significant variable for grey nurse sharks, the southern increase in captures occurred at beaches with a high proportion of the beach covered by the net (Figure 1) and removing these predicted a reduction of catch of ~40% for the species (Table 1). Because SMP nets are set close to the beach, these sharks might have a higher likelihood of being caught at these shorter beaches as the reef is in proximity on either side of the net. Instead of being removed, these nets could be moved to deeper waters in an attempt to reduce captures of grey nurse sharks.
The southern region caught more tiger and white sharks, with white sharks mostly caught during the latter half of the season (January to April) coincident with known broadscale movements (Bruce et al., 2019), supporting South African research suggesting white shark catch was related to longshore movements (Cliff et al., 1989). The increased catch of both white sharks and grey nurse sharks in the nets on either extremity of the SMP region may therefore be due to the “boundary effect” first described by Cliff et al. (1989). The boundary effect proposes that sharks are more likely to be captured when they first encounter nets on the edge of these programs. Removing nets from extreme locations would not necessarily reduce shark catches but simply move the boundary. A more effective approach to reduce mortality of threatened species may rather be to change practises, for example deploying alternative mitigation strategies.
Addressing the Wicked Problem
Management of human-wildlife conflicts requires that both the human and ecological dimensions of the problem are properly identified and accounted for (Carter et al., 2014; Teixeira et al., 2021). Recognising local attitudes toward the animals involved is often among the main strategies to tackle these situations (Madden, 2008; Goodale et al., 2015; Dillon et al., 2016). This includes tolerance of wildlife presence (Bruskotter and Wilson, 2014; Slagle and Bruskotter, 2019), which in turn is affected by personal experiences such as being involved in direct contacts or attacks by these animals (Treves and Bruskotter, 2014; Dorresteijn et al., 2016).
At one end of our matrix, maintaining the status quo of the SMP would result in ongoing catches of threatened grey nurse and white sharks, and zero impact on reducing this Key Threatening Process to their population viability. In Australia, there are strong pressures toward adapting bather protection programs, particularly in Western Australia and New South Wales (Fraser-Baxter and Medvecky, 2018), suggesting that this option would not be supported by NGOs nor beach goers. Simultaneously, this option has limited value to the government organisation charged with enhancing the conservation status of these species. However, despite public and NGOs being supportive of taking nets out of the water, complete removal of the SMP may exacerbate the wicked problem due to the possibility of increased risks to human safety. Therefore, we focus on the evidence supporting the replacement of nets with alternatives.
It is unlikely that all nets could be replaced by new methods as logistically replacing nets with SMART drumlines off beaches further than 15 km from a port is unfeasible if sharks are to survive (Tate et al., 2019). However, traditional drumlines could be installed at remote beaches as they do not require immediate response following a capture and incur lower mortality than nets (Sumpton et al., 2011). Survivorship in traditional drumlines off Queensland varies considerably (grey nurse = 50%, white = 47.5%, tiger = 31%, bull = 25.9%; Sumpton et al., 2011). They may represent a compromise by reducing mortalities of threatened species while still providing some mitigation (Cliff and Dudley, 2011), however they are generally not supported by the majority of the community (Gibbs and Warren, 2014).
The release of potentially dangerous sharks did not directly correlate with higher incidence of shark bites in South Africa (Cliff and Dudley, 2011), nor has it in NSW since it was formally implemented in 2009, supporting the use of non-lethal measures for mitigating shark hazard. Although approximately half of grey nurse sharks are released following capture in the SMP (Dalton and Peddemors, 2018), there is little information on post-release survivorship. Grey nurse sharks are prone to stress-induced lactic acid build-up and subsequent mortality following capture (Smale et al., 2012; Otway, 2015). Apparently positive post-release survival from the SMP (Dalton and Peddemors, 2018) and the two trials of shark nets on the NSW North Coast (NSW DPI, 2017, 2018) suggests that SMART drumlines will lead to even higher post-release survivorship for this species. SMART drumlines do not appear to attract potentially dangerous sharks into nearshore areas, however, neither are they or any other existing mitigation measure capable of completely stopping sharks from using a particular area (Guyomard et al., 2020). Combining SMART drumlines with aerial surveillance (Simmons and Mehmet, 2018) could provide a potential alternative to shark nets.
Drones are a cost-effective substitute for manned aircraft, however, their effectiveness still depends on the ability of the pilot to identify a shark in variable environmental conditions (Butcher et al., 2019). The development of artificial intelligence is likely to reduce reliance on pilots in future, but there will also still be issues related to proximity with airports and other critical infrastructure. Sole reliance on aerial surveillance may, therefore, not be practical in many coastal regions. Drones have been shown to be capable of acting as aerial support for shark detection in nearshore waters (Butcher et al., 2019; Gorkin et al., 2020), however, until flight automation becomes more advanced it is unlikely to be considered viable at understaffed beaches for beach authorities. They could, however, be applied in combination with SMART drumlines off more popular beaches, e.g., Bondi Beach where high grey nurse shark and low white shark captures, coupled with the popularity of this Australian beach, point to a high likelihood of success.
Recognising the ecological patterns underlying a conservation issue while including knowledge of human behaviour is an effective strategy for solving wicked problems, but the science must be evidence-based (Game et al., 2014; Mason et al., 2018). Grey nurse sharks aggregate at particular locations along the east coast of Australia (Bansemer and Bennett, 2008) leading to calls to remove nets near aggregation sites (Barker and Williamson, 2010). However, this and previous studies (Green et al., 2009) found no evidence that proximity to aggregation sites had any influence on capture probability (Supplementary Table 4), implying that removal of these nets would be an ineffective strategy to reduce the impacts of the SMP, possibly misdirecting efforts. This argues strongly for evidence-based conservation decision making, particularly when sensitive to public scrutiny. It is important to emphasise that the nets are not a barrier that prevents sharks from interacting with beach goers as they do not cover the entire beach, rather they are a fishing agent. Research trials of shark nets on the NSW north coast found that only 6 out of 145 animals (4%) were caught on the shoreward side of the nets (NSW DPI, 2018). By contrast in South Africa, 35% of the sharks caught were done so on the shoreward side of the nets, i.e., when moving out to sea (Dudley, 1997). While removing nets might be considered controversial for risk-averse government agencies, it is evident from our findings that management efforts focused at only two beaches (i.e., Stockton and Bondi) could substantially improve shark conservation with little projected risk for people in this region, provided alternative mitigation measures are implemented in their place.
In human-wildlife interactions people are not only part of the problem but the essential component to finding effective solutions toward coexistence (Frank and Glikman, 2019). Many human-wildlife conflicts involve humans venturing into wildlife habitat, or exploiting areas in new ways, whether becoming exposed to sharks in the sea or large dangerous wildlife on land. In the case of shark bite risk, while management agencies are tasked with developing public safety strategies the public can also contribute to lowering their own risk, for example, by wearing personal electronic deterrents (Bradshaw et al., 2021). Balancing human risk with increasingly disturbed environments and ever diminishing populations of wildlife (Bar-On et al., 2018) requires robust approaches. Effectively measuring stakeholder attitudes toward potential alternatives for human-wildlife conflict can be challenging, as they may have divergent perspectives toward wildlife (Ceauşu et al., 2019), and these might further vary with time depending on people's personal experiences, culture and changes in beliefs. It is also important to weight the impacts and trade-offs involved in the risk of conflicts during recreational activities (Kubo and Shoji, 2014), including their decision making process around the threat of shark bites. Under the context of the SMP, no single definite solution could be found to replace current methods as some stakeholders, including members of the public, were found to agree with the use of shark nets (Gray and Gray, 2017). Any changes to the program are likely to be location-specific, depending on feasibility of use for each of the alternatives proposed in this study, whilst simultaneously accounting for the attitudes of the people involved. While we acknowledge that our findings might not be definitive, we hope they will provide decision makers with sufficient understanding to move toward a more holistic approach to managing marine ecosystems in which humans and sharks can coexist. The framework adopted in our study incorporates (i) identification of species-specific occurrence patterns, (ii) determining potential actions toward reducing impacts for wildlife while keeping people safe, (iii) evaluation of stakeholder attitudes toward proposed alternatives. Our results indicate that integrating this framework into human-wildlife conflict resolution strategies is not only applicable to other programs of mitigating shark bite risk, but also for managing human-wildlife conflicts in other situations where empirical data on species occurrence and ecology are available.
Data Availability Statement
The datasets presented in this study can be found in online repositories. The names of the repository/repositories and accession number(s) can be found at: https://www.sharksmart.nsw.gov.au/__data/assets/pdf_file/0009/1246275/smp-2019-2020-annual-performance-report.pdf.
Ethics Statement
Ethical review and approval was not required for the animal study because data was obtained by a long-running (since 1937) government program.
Author Contributions
YN, VP, AS, and RH conceived the ideas and designed the methods. VP, AS, and MG collected the data. YN analysed the data and led the writing of the manuscript. All authors contributed to the article and approved the submitted version.
Conflict of Interest
The authors declare that the research was conducted in the absence of any commercial or financial relationships that could be construed as a potential conflict of interest.
Publisher's Note
All claims expressed in this article are solely those of the authors and do not necessarily represent those of their affiliated organizations, or those of the publisher, the editors and the reviewers. Any product that may be evaluated in this article, or claim that may be made by its manufacturer, is not guaranteed or endorsed by the publisher.
Acknowledgments
The NSW DPI staff and contractors associated with the Shark Meshing Program are thanked for their efforts to ensure accurate catch data, especially Scott Dalton and Cameron Doak. Financial support to YVN through an International Macquarie University Research Excellence Scholarship is acknowledged.
Supplementary Material
The Supplementary Material for this article can be found online at: https://www.frontiersin.org/articles/10.3389/fcosc.2021.720741/full#supplementary-material
References
Abrahms, B. (2021). Human-wildlife conflict under climate change. Science 373, 484–485. doi: 10.1126/science.abj4216
AODN (2019). Open Access Data to Ocean Data. Available online at: https://portal.aodn.org.au/ (accessed February 15, 2019).
Bansemer, C. S., and Bennett, M. B. (2008). Multi-year validation of photographic identification of grey nurse sharks, Carcharias taurus, and applications for non-invasive conservation research. Marine Freshwater Res. 59:322. doi: 10.1071/MF07184
Barker, S. M., and Williamson, J. E. (2010). Collaborative photo-identification and monitoring of grey nurse sharks (Carcharias taurus) at key aggregation sites along the eastern coast of Australia. Marine Freshwater Res. 61:971. doi: 10.1071/MF09215
Bar-On, Y. M., Phillips, R., and Milo, R. (2018). The biomass distribution on Earth. Proc. Natl. Acad. Sci. U.S.A. 115, 6506–6511. doi: 10.1073/pnas.1711842115
Bradshaw, C. J., Meagher, P., Thiele, M. J., Harcourt, R. G., and Huveneers, C. (2021). Predicting potential future reduction in shark bites on people. R. Soc. Open Sci. 8:201197. doi: 10.1098/rsos.201197
Bruce, B. D., and Bradford, R. W. (2008). Spatial Dynamics and Habitat Preferences of Juvenile White Sharks: Identifying Critical Habitat and Options for Monitoring Recruitment. Final Report to Department of the Environment, Water, Heritage and the Arts. Canberra, ACT.
Bruce, B. D., and Bradford, R. W. (2012). “Habitat use and spatial dynamics of juvenile white sharks, Carcharodon carcharias, in eastern Australia,” in Global Perspectives on the Biology and Life History of the White Shark, ed M. L. Domeier (Boca Raton, FL: CRC Press), 225–253.
Bruce, B. D., Harasti, D., Lee, K., Gallen, C., and Bradford, R. (2019). Broad-scale movements of juvenile white sharks Carcharodon carcharias in eastern Australia from acoustic and satellite telemetry. Mar. Ecol. Prog. Ser. 619, 1–15. doi: 10.3354/meps12969
Bruskotter, J. T., and Wilson, R. S. (2014). Determining where the wild things will be: using psychological theory to find tolerance for large carnivores. Conserv. Lett. 7, 158–165. doi: 10.1111/conl.12072
Bunnefeld, N., Nicholson, E., and Milner-Gulland, E. J. (2017). Decision- Making in Conservation and Natural Resource Management: Models for Interdisciplinary Approaches. Cambridge: Cambridge University Press. doi: 10.1017/9781316135938
Butcher, P., Piddocke, T., Colefax, A., Hoade, B., Peddemors, V., Borg, L., et al. (2019). Beach safety: can drones provide a platform for sighting sharks? Wildlife Res. 46, 701–712. doi: 10.1071/WR18119
Carter, N. H., Viña, A., Hull, V., McConnell, W. J., Axinn, W., Ghimire, D., et al. (2014). Coupled human and natural systems approach to wildlife research and conservation. Ecol. Soc. 19, 43–60. doi: 10.5751/ES-06881-190343
Ceauşu, S., Graves, R. A., Killion, A. K., Svenning, J. C., and Carter, N. H. (2019). Governing trade-offs in ecosystem services and disservices to achieve human-wildlife coexistence. Conserv. Biol. 33, 543–553. doi: 10.1111/cobi.13241
Cliff, G., and Dudley, S. F. J. (2011). Reducing the environmental impact of shark-control programs: a case study from KwaZulu-Natal, South Africa. Marine Freshwater Res. 62, 700–709. doi: 10.1071/MF10182
Cliff, G., Dudley, S. F. J., and Davis, B. (1989). Sharks caught in the protective gill nets off natal, South Africa. 2. the great white shark Carcharodon carcharias (Linnaeus). South African J. Marine Sci. 8, 131–144. doi: 10.2989/02577618909504556
Conrick, C. (1989). The Northern District Surf Life Saver: A History of Surf Life Saving in the Newcastle Region. Merewether, NSW: Newcastle Branch of the Surf Life Saving Association, 251.
Crossley, R., Collins, C. M., Sutton, S. G., and Huveneers, C. (2014). Public perception and understanding of shark attack mitigation measures in Australia. Human Dimensions Wildlife 19, 154–165. doi: 10.1080/10871209.2014.844289
Cruz-Martínez, A., Chiappa-Carrara, X., and Arenas-Fuentes, V. (2005). Age and growth of the bull shark, Carcharhinus leucas, from southern Gulf of Mexico. J. Northwest Atlantic Fishery Sci. 35, 367–374. doi: 10.2960/J.v35.m481
Dalton, S., and Peddemors, V. (2018). Shark Meshing (Bather Protection) Program 2017/18 Annual Performance Report. Sydney, NSW.
Dickman, A. J. (2010). Complexities of conflict: the importance of considering social factors for effectively resolving human-wildlife conflict. Anim. Conserv. 13, 458–466. doi: 10.1111/j.1469-1795.2010.00368.x
Dillon, J., Stevenson, R. B., and Wals, A. E. J. (2016). Moving from citizen to civic science to address wicked conservation problems. Conserv. Biol. 30, 450–455. doi: 10.1111/cobi.12689
Dorresteijn, I., Milcu, A. I., Leventon, J., Hanspach, J., and Fischer, J. (2016). Social factors mediating human-carnivore coexistence: understanding thematic strands influencing coexistence in Central Romania. Ambio 45, 490–500. doi: 10.1007/s13280-015-0760-7
Dudley, S. F. J. (1997). A comparison of the shark control programs of New South Wales and Queensland (Australia) and KwaZulu-Natal (South Africa). Ocean Coast. Manag. 34, 1–27. doi: 10.1016/S0964-5691(96)00061-0
Dudley, S. F. J., and Cliff, G. (2010). “Shark-control: methods, efficacy, and ecological impact,” in Sharks and Their Relatives II: Biodiversity, Adaptive Physiology and Conservation, eds J. C. Carrier, J. A. Musick, and M. R. Heithaus (Boca Raton: CRC Press), 567–592. doi: 10.1201/9781420080483-c14
Everett, J. D., Baird, M. E., Roughan, M., Suthers, I. M., and Doblin, M. A. (2014). Relative impact of seasonal and oceanographic drivers on surface chlorophyll a along a Western Boundary Current. Prog. Oceanogr. 120, 340–351. doi: 10.1016/j.pocean.2013.10.016
Frank, B. (2016). Human–wildlife conflicts and the need to include tolerance and coexistence: an introductory comment. Soc. Natural Resour. 29, 738–743. doi: 10.1080/08941920.2015.1103388
Frank, B., and Glikman, J. A. (2019). “Human-wildlife conflicts and the need to include coexistence,” in Human-Wildlife Interactions: Turning Conflict Into Coexistence, eds B. Frank, J. Glikman, and S. Marchini S (Cambridge: Cambridge University Press), 1–14. doi: 10.1017/9781108235730.004
Fraser-Baxter, S., and Medvecky, F. (2018). Evaluating the media's reporting of public and political responses to human-shark interactions in NSW, Australia. Marine Policy 97, 109–118. doi: 10.1016/j.marpol.2018.08.020
Gallagher, A. J., Orbesen, E. S., Hammerschlag, N., and Serafy, J. E. (2014). Vulnerability of oceanic sharks as pelagic longline bycatch. Glob. Ecol. Conserv. 1, 50–59. doi: 10.1016/j.gecco.2014.06.003
Game, E. T., Meijaard, E., Sheil, D., and Mcdonald-Madden, E. (2014). Conservation in a wicked complex world; challenges and solutions. Conserv. Lett. 7, 271–277. doi: 10.1111/conl.12050
Gibbs, L., and Warren, A. (2014). Killing Sharks: cultures and politics of encounter and the sea. Austral. Geographer 45, 101–107. doi: 10.1080/00049182.2014.899023
Goodale, K., Parsons, G. J., and Sherren, K. (2015). The nature of the nuisance-damage or threat-determines how perceived monetary costs and cultural benefits influence farmer tolerance of wildlife. Diversity 7, 318–341. doi: 10.3390/d7030318
Gorkin, R. I., Adams, K., Berryman, M. J., Aubin, S., Li, W., Davis, A. R., et al. (2020). Sharkeye: real-time autonomous personal shark alerting via aerial surveillance. Drones 4:18. doi: 10.3390/drones4020018
Gray, G. M. E., and Gray, C. A. (2017). Beach-user attitudes to shark bite mitigation strategies on coastal beaches; Sydney, Australia. Human Dimensions Wildlife 22, 282–290. doi: 10.1080/10871209.2017.1295491
Green, M., Ganassin, C., and Reid, D. D. (2009). Report into the NSW Shark Meshing (bather protection) Program. Sydney, NSW.
Guerrero, A. M., Shoo, L., Iacona, G., Standish, R. J., Catterall, C. P., Rumpff, L., et al. (2017). Using structured decision-making to set restoration objectives when multiple values and preferences exist. Restorat. Ecol. 25, 858–865. doi: 10.1111/rec.12591
Guyomard, D., Lee, K. A., Perry, C., Jaquemet, S., and Cliff, G. (2020). SMART drumlines at Réunion Island do not attract bull sharks Carcharhinus leucas into nearshore waters: evidence from acoustic monitoring. Fish. Res. 225:105480. doi: 10.1016/j.fishres.2019.105480
Guyomard, D., Perry, C., Tournoux, P. U., Cliff, G., Peddemors, V., and Jaquemet, S. (2019). An innovative fishing gear to enhance the release of non-target species in coastal shark-control programs: The SMART (shark management alert in real-time) drumline. Fish. Res. 216, 6–17. doi: 10.1016/j.fishres.2019.03.011
Hardiman, N., Burgin, S., and Shao, J. (2019). News media portrayal of attributed stakeholder attitudes to shark management in Australia. Human Dimensions Wildlife 24, 548–563. doi: 10.1080/10871209.2019.1663455
Hillary, R. M., Bravington, M. V., Patterson, T. A., Grewe, P., Bradford, R., Feutry, P., et al. (2018). Genetic relatedness reveals total population size of white sharks in eastern Australia and New Zealand. Sci. Rep. 8:2661. doi: 10.1038/s41598-018-20593-w
Holmes, B. J., Peddemors, V. M., Gutteridge, A. N., Geraghty, P. T., Chan, R. W. K., Tibbetts, I. R., et al. (2015). Age and growth of the tiger shark Galeocerdo cuvier off the east coast of Australia. J. Fish Biol. 87, 422–448. doi: 10.1111/jfb.12732
Holmes, B. J., Williams, S. M., Otway, N. M., Nielsen, E. E., Maher, S. L., Bennett, M. B., et al. (2017). Population structure and connectivity of tiger sharks (Galeocerdo cuvier) across the Indo-Pacific Ocean basin. R. Soc. Open Sci. 4:170309. doi: 10.1098/rsos.170309
Hudenko, H. W. (2012). Exploring the influence of emotion on human decision making in human-wildlife conflict. Human Dimensions Wildlife 17, 16–28. doi: 10.1080/10871209.2012.623262
IUCN SSC (2021). Human-Wildlife Conflict Task Force. Available online at: https://www.hwctf.org/about (accessed July 13, 2021).
Kohler, N. E., Casey, J. G., and Turner, P. A. (1996). Length-Length and Length-Weight Relationships for 13 Shark Species From the Western North Atlantic. Narragansett.
Krogh, M., and Reid, D. (1996). Bycatch in the protective shark meshing programme off south-eastern New South Wales, Australia. Biol. Conserv. 77, 219–226. doi: 10.1016/0006-3207(95)00141-7
Kubo, T., and Shoji, Y. (2014). Trade-off between human-wildlife conflict risk and recreation conditions. Eur. J. Wildl. Res. 60, 501–510. doi: 10.1007/s10344-014-0812-5
Lee, K. A., Roughan, M., Harcourt, R. G., and Peddemors, V. M. (2018). Environmental correlates of relative abundance of potentially dangerous sharks in nearshore areas, southeastern Australia. Mar. Ecol. Prog. Ser. 599:157–179. doi: 10.3354/meps12611
Lee, K. A., Smoothey, A. F., Harcourt, R. G., Roughan, M., Butcher, P. A., and Peddemors, V. M. (2019). Environmental drivers of abundance and residency of a large migratory shark, Carcharhinus leucas, inshore of a dynamic western boundary current. Mar. Ecol. Prog. Ser. 622, 121–137. doi: 10.3354/meps13052
Lincoln-Smith, M., and Roberts, C. (2010). Development and Implementation of a Population Estimation Protocol to Provide an Estimate of East Coast Population Numbers for Grey Nurse Sharks (Carcharias taurus). Canberra, ACT: Cardno Ecology Lab.
Lischka, S. A., Teel, T. L., Johnson, H. E., Larson, C., Breck, S., and Crooks, K. (2020). Psychological drivers of risk-reducing behaviors to limit human-wildlife conflict. Conserv. Biol. 34, 1383–1392. doi: 10.1111/cobi.13626
Lucifora, L. O., Menni, R. C., and Escalante, A. H. (2002). Reproductive ecology and abundance of the sand tiger shark, Carcharias taurus, from the southwestern Atlantic. ICES J. Marine Sci. 59, 553–561. doi: 10.1006/jmsc.2002.1183
Lynch, T. P., Harcourt, R., Edgar, G., and Barrett, N. (2013). Conservation of the critically endangered Eastern Australian population of the grey nurse shark (Carcharias taurus) through cross-jurisdictional management of a network of marine-protected areas. Environ. Manage. 52, 1341–1354. doi: 10.1007/s00267-013-0174-x
Madden, F. M. (2008). The growing conflict between humans and wildlife: law and policy as contributing and mitigating factors. J. Int. Wildlife Law Policy 11, 189–206. doi: 10.1080/13880290802470281
Mason, T. H. E., Pollard, C. R. J., Chimalakonda, D., Guerrero, A. M., Kerr-Smith, C., Milheiras, S. A. G., et al. (2018). Wicked conflict: using wicked problem thinking for holistic management of conservation conflict. Conserv. Lett. 11, 1–9. doi: 10.1111/conl.12460
McPhee, D. P., Blount, C., Lincoln Smith, M. P., and Peddemors, V. M. (2021). A comparison of alternative systems to catch and kill for mitigating unprovoked shark bite on bathers or surfers at ocean beaches. Ocean Coastal Manage. 201:105492. doi: 10.1016/j.ocecoaman.2020.105492
Midway, S. R., Wagner, T., and Burgess, G. H. (2019). Trends in global shark attacks. PLoS ONE 14:e0211049. doi: 10.1371/journal.pone.0211049
Niella, Y., Smoothey, A. F., Peddemors, V., and Harcourt, R. (2020). Predicting changes in distribution of a large coastal shark in the face of the strengthening East Australian Current. Mar. Ecol. Prog. Ser. 642, 163–177. doi: 10.3354/meps13322
NSW DPI (2018). Second NSW North Coast shark-meshing trial. NSW DPI – Fisheries Final Report Series No 157. Port Stephens, NSW.
NSW Government (2019). Nearshore Features Shapefile. Available online at: https://data.nsw.gov.au/data/dataset/nsw-nearshore-coastal-reef-extent-2017/resource/b41314d8-38c0-4ecb-8e5c-ee2c2a282131 (accessed February 15, 2019).
NSW Shark Menace Committee (1929). Summary of the New South Wales Shark Menace Committee's Report. Sydney, NSW: NSW Government Printer.
Oke, P. R., and Griffin, D. A. (2011). The cold-core eddy and strong upwelling off the coast of New South Wales in early 2007. Deep-Sea Res. II 58, 574–591. doi: 10.1016/j.dsr2.2010.06.006
Otway, N. M. (2015). Serum biochemical reference intervals for free-living sand tiger sharks (Carcharias taurus) from east Australian waters. Vet. Clin. Pathol. 44, 262–274. doi: 10.1111/vcp.12254
Otway, N. M., and Ellis, M. T. (2011). Pop-up archival satellite tagging of Carcharias taurus: movements and depth/temperature-related use of south-eastern Australian waters. Marine Freshwater Res. 62:607. doi: 10.1071/MF10139
Patrick, W. S., Spencer, P., Ormseth, O., Cope, J., Field, J., and Kobayashi, D. (2009). Use of Productivity and Susceptibility Indices to Determine Stock Vulnerability, With Example Applications to Six U.S. Fisheries. U.S. Dep. Commer., NOAA Tech. Memo. Seattle, WA: NMFS-F/SPO-101, 90.
Pawle, F. (2017). Submission to the Senate Inquiry Into Shark Mitigation Strategies. Shark Mitigation and Deterrent Measures. Available online at: https://www.aph.gov.au/DocumentStore.ashx?id=c319b6c2-f1f3-461b-88b8-1a875d7e0569&subId=464435 (accessed July 13, 2021).
Pollard, D. A., Lincoln-Smith, M. P., and Smith, A. K. (1996). The biology and conservation status of the grey nurse shark (Carcharias taurus Rafinesque 1810) in New South Wales, Australia. Aquatic Conserv. 6, 1–20. doi: 10.1002/(SICI)1099-0755(199603)6:1<1::AID-AQC177>3.0.CO;2-#
Redpath, S. M., Young, J., Evely, A., Adams, W. M., Sutherland, W. J., Whitehouse, A., et al. (2013). Understanding and managing conservation conflicts. Trends Ecol. Evol. 28, 100–109. doi: 10.1016/j.tree.2012.08.021
Reid, D. D., and Krogh, M. (1992). Assessment of catches from protective shark meshing off New South Wales beaches between 1950 and 1990. Marine Freshwater Res. 43, 283–296. doi: 10.1071/MF9920283
Reid, D. D., Robbins, W. D., and Peddemors, V. M. (2011). Decadal trends in shark catches and effort from the New South Wales, Australia, Shark Meshing Program 1950–2010. Marine and Freshwater Research, 62, 676–693.
Rittel, H. W., and Webber, M. M. (1973). Dilemmas in a general theory of planning. Policy Sci. 4, 155–169. doi: 10.1007/BF01405730
Robbins, W. D., Peddemors, V. M., Broadhurst, M. K., and Gray, C. A. (2013). Hooked on fishing? Recreational angling interactions with the critically endangered grey nurse shark Carcharias taurus in eastern Australia. Endangered Species Res. 21, 161–170. doi: 10.3354/esr00520
Roughan, M., and Middleton, J. H. (2002). A comparison of observed upwelling mechanisms off the east coast of Australia. Cont. Shelf Res. 22, 2551–2572. doi: 10.1016/S0278-4343(02)00101-2
Ryan, L. A., Lynch, S. K., Harcourt, R., Slip, D. J., Peddemors, V., Everett, J. D., et al. (2019). Environmental predictive models for shark attacks in Australian waters. Mar. Ecol. Prog. Ser. 631, 165–179. doi: 10.3354/meps13138
Sabatier, E., and Huveneers, C. (2018). Changes in media portrayal of human-wildlife conflict during successive fatal shark bites. Conserv. Soc. 16, 338–350. doi: 10.4103/cs.cs_18_5
SharkSmart NSW (2021). NSW Department of Primary Industries. Available online at: https://www.sharksmart.nsw.gov.au/technology-trials-and-research/social-research (accessed May 11, 2021).
Simmons, P., Mehmet, M., Curley, B., Ivory, N., Callaghan, K., Wolfenden, K., et al. (2021). A scenario study of the acceptability to ocean users of more and less invasive management after shark-human interactions. Marine Policy 129:104558. doi: 10.1016/j.marpol.2021.104558
Simmons, P., and Mehmet, M. I. (2018). Shark management strategy policy considerations: community preferences, reasoning and speculations. Marine Policy 96, 111–119. doi: 10.1016/j.marpol.2018.08.010
Slagle, K., and Bruskotter, J. T. (2019). “Tolerance for wildlife: a psychological perspective,” in Human-Wildlife Interactions: Turning Conflict Into Coexistence, eds B. Frank, J. A. Glickman, and S. Marchini (Cambridge: Cambridge University Press). 85–106. doi: 10.1017/9781108235730.008
Smale, M. J., Booth, A. J., Farquhar, M. R., Meýer, M. R., and Rochat, L. (2012). Migration and habitat use of formerly captive and wild raggedtooth sharks (Carcharias taurus) on the southeast coast of South Africa. Marine Biol. Res. 8, 115–128. doi: 10.1080/17451000.2011.617756
Stobutzki, I., Miller, M., and Brewer, D. (2001). Sustainability of fishery bycatch: a process for assessing highly diverse and numerous bycatch. Environ. Conserv. 28, 167–181. doi: 10.1017/S0376892901000170
Sumpton, W. D., Taylor, S. M., Gribble, N. A., McPherson, G., and Ham, T. (2011). Gear selectivity of large-mesh nets and drumlines used to catch sharks in the Queensland Shark Control Program. Afr. J. Mar. Sci. 33, 37–43. doi: 10.2989/1814232X.2011.572335
Tate, R. D., Cullis, B. R., Smith, S. D. A., Kelaher, B. P., Brand, C. P., Gallen, C. R., et al. (2019). The acute physiological status of white sharks (Carcharodon carcharias) exhibits minimal variation after capture on SMART drumlines. Conserv. Physiol. 7, 1–9. doi: 10.1093/conphys/coz090
Teixeira, L., Tisovec-Dufner, K. C., Marin, G. D. L., Marchini, S., Dorresteijn, I., and Pardini, R. (2021). Linking human and ecological components to understand human-wildlife conflicts across landscapes and species. Conserv. Biol. 35, 285–296. doi: 10.1111/cobi.13537
Tillett, B. J., Meekan, M. G., Field, I. C., Thorburn, D. C., and Ovenden, J. R. (2012). Evidence for reproductive philopatry in the bull shark Carcharhinus leucas. J. Fish Biol. 80, 2140–2158. doi: 10.1111/j.1095-8649.2012.03228.x
Treves, A., and Bruskotter, J. T. (2014). Tolerance for predatory wildlife. Science 344, 476–477. doi: 10.1126/science.1252690
Treves, A., and Santiago-Ávila, F. J. (2020). Myths and assumptions about human-wildlife conflict and coexistence. Conserv. Biol. 34, 811–818. doi: 10.1111/cobi.13472
Wagenmakers, E. J., and Farrell, S. (2004). AIC model selection using Akaike weights. Psychon. Bull. Rev. 11, 192–196. doi: 10.3758/BF03206482
Keywords: human-wildlife conflict, shark, wicked problem, conservation, threatened species
Citation: Niella Y, Peddemors VM, Green M, Smoothey AF and Harcourt R (2021) A “Wicked Problem” Reconciling Human-Shark Conflict, Shark Bite Mitigation, and Threatened Species. Front. Conserv. Sci. 2:720741. doi: 10.3389/fcosc.2021.720741
Received: 04 June 2021; Accepted: 22 September 2021;
Published: 20 October 2021.
Edited by:
Alexandra Zimmermann, University of Oxford, United KingdomReviewed by:
Lily M. van Eeden, Arthur Rylah Institute for Environmental Research (ARI), AustraliaEmily Pomeranz, State of Michigan, United States
Copyright © 2021 Niella, Peddemors, Green, Smoothey and Harcourt. This is an open-access article distributed under the terms of the Creative Commons Attribution License (CC BY). The use, distribution or reproduction in other forums is permitted, provided the original author(s) and the copyright owner(s) are credited and that the original publication in this journal is cited, in accordance with accepted academic practice. No use, distribution or reproduction is permitted which does not comply with these terms.
*Correspondence: Yuri Niella, eXVyaS5uaWVsbGFAZ21haWwuY29t