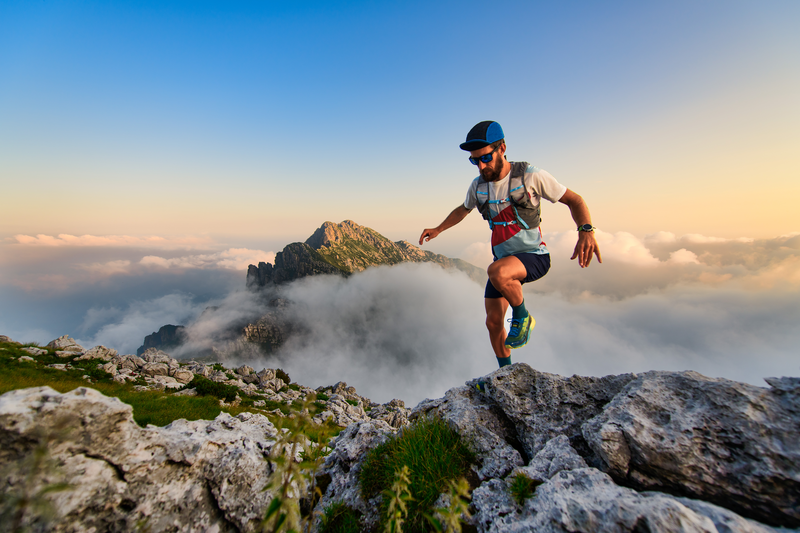
94% of researchers rate our articles as excellent or good
Learn more about the work of our research integrity team to safeguard the quality of each article we publish.
Find out more
ORIGINAL RESEARCH article
Front. Conserv. Sci. , 03 December 2021
Sec. Animal Conservation
Volume 2 - 2021 | https://doi.org/10.3389/fcosc.2021.718562
This article is part of the Research Topic Animal Behavior After Translocation into Novel Environments View all 11 articles
Conservation translocations, which involve the intentional movement and release of organisms for conservation benefit, are increasingly required to recover species of conservation concern. In order to maximize post-release survival, and to accomplish conservation translocation objectives, animals must exhibit behaviors that facilitate survival in the wild. The Vancouver Island marmot (Marmota vancouverensis) is a critically endangered endemic in Canada which has been captive-bred for 24 years for reintroductions and reinforcements that have increased the wild population from ~30 to more than 200 individuals. Despite this success many marmots are killed by predators after release and predation represents a major hurdle to full marmot recovery. To better understand if captive-bred marmots are prepared for the novel environment into which they will be released, and to determine whether such suitability changes over time, we presented taxidermy mounts of mammalian predators and non-predators to marmots that were wild-caught, and captive born for between one and five generations. We also examined mortality of offspring from marmots we tested that had been released to the wild. A minimum of 43% of offspring were killed by predators in the wild over 17 years, most by cougars. Marmots in captivity generally responded to taxidermy mounts by decreasing foraging and increasing vigilance, and overall responded more strongly to predators than non-predators, especially wolves. However, marmots in captivity for more than two generations lacked discrimination between cougars, non-predators, and controls, suggesting a rapid loss of predator recognition. This study was only possible because predator-recognition trials were initiated early in the conservation translocation program, and could then be repeated after a number of generations. The finding that changes occurred relatively rapidly (within five generations during which changes in genetic diversity were negligible) suggests that behavioral suitability may deteriorate more rapidly than genetics would suggest. Strategies addressing potential behavior loss should be considered, including sourcing additional wild individuals or pre-release training of captive-born individuals. Subsequently, post-release survival should be monitored to determine the efficacy of behavior-optimization strategies.
With one million species at risk of extinction (IPBES, 2019), conservation interventions are critical to retain biodiversity. Conservation translocations involve intentional movement of species from one location to another to restore populations and ecosystems (IUCN/SSC, 2013) and have improved the conservation status of many species (Hoffmann et al., 2010, 2015). There is a growing realization that knowledge of behavior is important for successful conservation science (Greggor et al., 2016) which is especially true for translocations (Berger-Tal et al., 2020). In North America alone, 58% of species in conservation translocation programs have captive breeding as a component of the program (Brichieri-Colombi et al., 2018). However, captive breeding for translocation creates significant challenges associated with managing the genetics, fertility, health, behavior and reproduction of extremely small populations.
Post-release behavior can affect the fate of individuals in many ways and thus can impact the ultimate success of a translocation project (Berger-Tal et al., 2020). Mortality in the wild due to predation post-release is a major challenge (Moseby et al., 2011; Brichieri-Colombi and Moehrenschlager, 2016), and especially plagues individuals released from captive populations (Griffin et al., 2000; Harrington et al., 2013). Wild animals entering a conservation breeding program are exposed to a novel environment, one where they are typically raised in a relaxed selective environment with abundant food, no mate competition, and no natural predators where they will likely become naïve to conditions in the wild (i.e., captive selection; Frankham et al., 1986). Further, the risk of captive selection increases with the number of generations a population has remained in captivity (Swaisgood, 2010). Once captive-born individuals are released into the wild they are exposed to a novel environment as well; one where they must learn to forage and evade predators.
Many animals make tradeoffs between remaining vigilant to avoid predators and focusing attention on other fundamental aspects of life, such as foraging (Houston et al., 1993). Life in captivity will affect these tradeoffs as the frequency with which animals encounter predators influences how strongly they will respond to predators at each encounter. Individuals that are infrequently at high risk of predation will respond more strongly on those rare occasions when predators are present (i.e., the Predation Risk Allocation hypothesis; Lima and Bednekoff, 1999). Therefore, one effect of a life in captivity without predators may be that individuals increase their responses to predators over time. While increasing vigilance can be beneficial to avoid predators, it can come at a cost, as animals may need to reduce other behaviors, like foraging (Fortin et al., 2004), in order to allocate more time to vigilance. This potential shift in behavior should be of concern for any captive breeding program that plans to reintroduce animals back to the wild. Conservation breeding and reintroductions are often long-term endeavors with many programs lasting for decades [e.g., the Arabian Oryx (Oryx leucoryx) 40 years, (Islam et al., 2013); whooping crane (Grus americana) 70 years, (Barrett and Stehn, 2010); and black-footed ferret (Mustela nigripes) more than 30 years, (Jachowski et al., 2011)]. Given the length of these programs and the possibility of captive selection it is prudent to monitor behavior within captive and wild populations of conservation translocation programs over time.
Conservation translocations should include behavioral monitoring (IUCN/SSC, 2013). In addition to long-term monitoring, experts have been calling for increased use of rigorous experimental design and evidence-based decisions within conservation translocation science (Seddon et al., 2007). Replicating studies of endangered or threatened species is not always possible for many reasons, in part due to resource limitations and small samples sizes (Shaw et al., 2021), making those few studies that are possible even more valuable. However, studies with small sample sizes must be interpreted with caution and statistical analyses must be applied appropriately (Bissonette, 1999).
Vancouver Island marmots (Marmota vancouverensis; hereafter, marmots) are social, ground dwelling, rodents endemic to Vancouver Island, Canada and are listed as Critically Endangered on the IUCN Red List (Roach, 2017). In 1997 a conservation breeding program was deemed necessary to help save the species (Casimir et al., 2007), which had declined to ~30 individuals in the wild. The wild population of marmots has increased to ~200 individuals through reintroductions from 2003 to 2020 (Marmot Recovery Foundation, 2020) with an additional ~100 currently in captivity. Recent studies have shown that predation is the leading cause of mortality for marmots in the wild and captive-born marmots have lower annual survival than wild-born marmots post release (Aaltonen et al., 2009; Jackson et al., 2016). Captive-born individuals may have reduced or altered predator recognition abilities or anti-predator behaviors as a result of their captive environment (McPhee, 2003; Blumstein et al., 2004). Indeed, Vancouver Island marmots remain vulnerable to predation by wolves, cougars, and golden eagles in the wild (Vancouver Island Marmot Recovery Team, 2017).
Between 2002 and 2004, Blumstein et al. (2006) tested captive marmots for their ability to discriminate between mammalian predators and non-predators. Behavioral observations indicated that wild-born and captive-born marmots were equally able to discriminate between predators and non-predators while in captivity (Blumstein et al., 2006). These findings were encouraging for the reintroduction program. However, the reduced survival of captive-born marmots relative to wild-born conspecifics (Lloyd et al., 2018) begs further research into their captive environment and behaviors. Even with higher post-release survival from a novel “stepping-stone” release approach, where individuals were released to a relatively safe and established site known for high survival, before being moved to a final release site, Lloyd et al. (2018) call for research as to how to best prepare marmots in captivity before reintroduction.
Here, we build on Blumstein et al. (2006) by repeating the previous study with new individuals including those that have been captive for additional generations. We seek to determine if there is a compounding effect on anti-predator behavior as marmots are in captivity for increasing generations. Additionally, we add a pilot study testing marmot discrimination between avian predators and non-predators. Given the marmots we test will be released to the wild or have offspring released, the way they respond to predators in captivity may, ultimately, influence species recovery. Being moved into a novel environment presents challenges for any animal, and this is particularly true when animals that have lived their whole lives in the relative safety of captivity are released into the wild. The objective of this study is to provide insight into the effect that multiple generations of captive living have on anti-predator behavior and the relationship between that behavior and potential mortality in the wild.
Marmots live in polygynous colonies with one or more family groups, are semi-fossorial, and hibernate in burrows for over 200 days each year. Female marmots can breed as early as 2 years of age, but typically first reproduce when 3 or 4 years old. Marmots in the wild have been recorded living 10 years (Bryant, 2005) and in captivity marmots live up to 14 years. Marmots are found in sub-alpine meadows at high elevations (COSEWIC, 2008).
Predator discrimination trials were conducted from July–August each year, from 2016 to 2018 following Blumstein et al. (2006). Marmots were tested at the Devonian Wildlife Conservation Center (DWCC), an off-site conservation breeding facility of the Calgary Zoo located ~30 km south of Calgary, Alberta, Canada. Marmots were housed in enclosures that had both indoor and outdoor sections. Marmots were constrained to outdoor sections of their enclosures and only one marmot was allowed into the outside section at a time so that marmots were not responding to their neighbors. The outdoor section was a yard (either 3.7 × 1.8 m or 3.6 × 3.4 m) giving the marmots a view of the surrounding area and adjacent enclosures. Each outdoor space had three hay bales; two bales on the ground with a gap between them, and a third bale on top. The gap between the two bales on the ground left a space large enough for the marmot to enter where it could hide. Barriers were placed between adjacent outdoor enclosures to ensure that each marmot was only exposed to experimental stimuli at the appropriate time. Video cameras (HikVision 3 Megapixel Ultra HD IP Camera; Bordo Security, Calgary) were installed in each outdoor enclosure to record the marmot's behavior. For smaller enclosures, one camera was mounted in the center of the roof, whereas, for larger enclosures two cameras were mounted at the top on opposite corners. All marmots that were available for testing from this captive population were included. To reduce stressors, we did not test animals that reproduced in a given year.
The majority of captive-born marmots are released onto mountains within the Nanaimo Lakes and Strathcona regions on Vancouver Island with some being released into extra-liminal colonies (Jackson et al., 2016). As part of the overall recovery program for marmots, post-release survival monitoring is conducted every year by the Marmot Recovery Foundation. Marmots are tracked post-release using VHF telemetry and whenever possible, remains are recovered to determine the cause of mortality (Jackson et al., 2016; Marmot Recovery Foundation, 2020).
Marmots were exposed to taxidermy mounts of predators and non-predators; see Blumstein et al. (2006) for photos of mounts. Each mount was placed on a wheeled cart and rolled along a track ~2 m from the marmots' enclosures. Blinds were placed at each end of the track which hid the taxidermy mount from the marmot's view before a trial was started and in which an observer could hide and pull the cart along the track via string and pulley reel. The track and blinds were set up at least 2 days before the trials were run to allow the marmots to acclimatize to the new equipment. Following Blumstein et al. (2006), each target marmot was exposed to four mammalian stimuli (marmot, goat, wolf, and cougar), and two procedural controls-the cart alone, and a blank where no stimulus was presented. These mammalian taxidermy mounts, cart, and tracks were the same as used in the previous study and each stimulus was presented to each marmot a single time. Starting in 2017, we also included four avian stimuli mounted in a perched position and tested the avian mounts on 10 marmots, see Supplementary Materials for details on avian trials.
Trials were conducted in the mornings from 07:00 h to noon, or until the temperature reached 25°C. For each trial the marmot was baited (with carrots, lettuce, or leaf-eater biscuit) to a central location in their enclosure on top of the stacked hay bales. Trials started once the marmot was calmly eating. Stimuli were pulled at a consistent rate until directly in front of the target marmot's enclosure, where they remained for 1 min. Subsequently, each stimulus was pulled into the researcher's blind and out of sight of the target marmot. The marmots were recorded on video for 1 min before each stimulus was presented, for 1 min with each stimulus in sight, and for 2 min after the stimulus was returned to a blind. The stimuli were presented to the marmots in a randomized order based on a Latin square design (Bradley, 1958). We waited at least 1 h between tests of marmots that formed a mating pair. Each marmot was exposed to a maximum of two stimuli per day, with the second stimulus being presented only after the marmot's behavior returned to a natural baseline and at least an hour had passed since the previous presentation. If the target marmot alarm called during the stimulus presentation, we waited at least 30 min before testing the next marmot to minimize possible carry-over effects. Because this study was conducted over 3 years (2016–2018) not all marmots were exposed to all stimuli in the same year.
Videos were scored by an observer that was unaware of which marmot they were watching and which stimulus was being presented. The videos were scored in JWatcher version 1.0 (Blumstein et al., 2020), and followed the same ethogram used by Blumstein et al. (2006). We focused on the proportion of time that marmots spent doing three sets of behaviors; (1) Foraging (head down ingesting food, or manipulating food with their paws); (2) Vigilant at the burrow or in the burrow (head raised, not manipulating food with their paws while standing near the burrow entrance or inside the pile of hay bales out of sight); and (3) High vigilance away from the burrow (standing on their hind legs and looking while more than one body length away from the burrow entrance).
We conducted statistical analyses in RStudio (RStudio Team, 2020) following Blumstein et al. (2006) and combined all marmots from Blumstein et al. (2006) and this study for analysis. We fitted mixed-effect models using the glmmTMB package (Brooks et al., 2017) assuming a beta distribution (logit link) for each of the three behaviors of interest (foraging, vigilance, and high vigilance). We divided marmots into three groups based on origin; wild caught, first and second generation captive born, and third, fourth, and fifth generation captive born. Furthermore, for analysis the 1 min focal period while the stimulus was present was divided into four 15 s time bins so that we could capture the immediate response and potentially track changes in response over time. We fitted models with stimulus order, generations in captivity, age, sex, stimulus type, and time bin as fixed effects, along with the interactions between origin, stimulus, age, sex, and time bin. We also included two random effects; a marmot ID which was unique to each individual and testing site which was one each of the three sites where testing was conducted. In 2002–2004 trials were conducted at all three sites, while those conducted from 2016–2018 were all conducted at the same site. We chose fixed effects, random effects, and interactions based on our knowledge of marmots and our study objective. We selected candidate models to include all fixed effects and interactions of interest. Due to our sample size we could not include a global model with all interactions and fixed effects together because such a model would not converge. Therefore, we instead selected our most highly parameterized model as the global model (Burnham and Anderson, 2002). We also fitted models with each of the fixed effects alone, and one with the intercept only. We calculated goodness of fit statistics based on our global models using the r2_nakagawa function from the Performance package (Lüdecke et al., 2021), calculated AICc values and AICc weights using the MuMIn package (Bartoń, 2020) and ranked all models by AICc values (Burnham and Anderson, 2002). We conducted model averaging using the model.avg function from the MuMIn package (Bartoń, 2020). Avian stimuli were also analyzed following the above methods (Supplementary Materials).
Marmots allocated no time to high vigilance away from the burrow in >90% of trials. With such high zero-inflation, models did not converge and did not produce interpretable results, therefore we did not include this behavior in further analyses.
Of the 34 marmots tested (21 from 2002–2004 and 13 from 2016–2018), 17 were wild-caught (male n = 13; female n = 4), and 17 were captive born. Eleven (male n = 7; female n = 4) of 17 captive-born marmots were first or second generation in captivity, and six marmots (male n = 4; female n = 2) were third, fourth, or fifth generation in captivity. The marmots ranged in age from one to 10 years (mean 3.0 ± 2.5 SD), 10 marmots were female, and 24 were male (Supplementary Table 1). Ten of 34 marmots presented with the mammal taxidermy mounts were also exposed to the avian taxidermy mounts, and the cart with pedestal (see Supplementary Materials for avian results). The 60 s period before stimuli were presented was omitted from analysis after running an ANOVA for each response variable and finding no relationship between any behavior and the stimulus each marmot was later presented with (Foraging; DFn = 5, DFd = 165, F = 1.059, p = 0.385; Vigilance; DFn = 5, DFd = 165, F = 1.774, p = 0.121). We also omitted the second minute post stimulus presentation because we removed the mount at 60 s and this confounded interpretation.
Between 2003 and 2020, 96 marmots that had at least one parent tested for predator discrimination were released and monitored in the wild using VHF telemetry. Of the 96 offspring released over 17 years, 53 marmots were confirmed dead (unpublished data, Marmot Recovery Foundation). Of these 53 marmots, 23 (43%) were killed by predators, (14 killed by cougars, five killed by eagles, and six marmots killed by an unknown predator); 14 (26%) died during hibernation, and 16 (30%) died from unknown or other causes. In addition to these 53 marmots, the fate of 40 marmots is unknown and three marmots released in 2020 are likely still alive.
Nine marmots tested between 2002 and 2004 were released to the wild, and none of the marmots tested between 2016 and 2018 have been released. Of these nine marmots, three were killed by predators (two by cougars; one by an eagle) and four died of other causes, during hibernation, or for unknown reasons. Eight of nine marmots died in the year they were released, and one survived in the wild for 8 years.
The global model for vigilance included interactions between generations in captivity, age, stimulus, and time, and both order and sex without interactions (DF = 149, log likelihood = 3,988.4, AICc = −7,611.7, ΔAICc = 289.5, Conditional R2 = 0.476, Marginal R2 = 0.349) and had random effect variances of 5.026e-09 ± 7.089e-05 SD for Site, and 0.163 ± 0.403 SD for ID. The top model for vigilance included only generations in captivity, stimulus, and the interaction between these two variables and had an AICc weight of 0.274 (Table 1). When the interaction between generations in captivity and stimulus was removed (Model 14; Table 1) the model weight decreased to 0.003 suggesting the top model is 91.3 times more likely to be the best model (0.274/0.003; Zuur et al., 2009). The global model for foraging also included interactions between generations in captivity, age, stimulus, and time, and both order and sex without interactions (DF = 149, log likelihood = 2,991.4, AICc = −5,617.7, ΔAICc = 244.4, Conditional R2 = 0.704, Marginal R2 = 0.413) and had random effect variances of 0.103 ± 0.322 for Site and 0.447 ± 0.668 SD for ID. The top model for foraging had a weight of 0.242 and included order, age, stimulus, and time (Table 2). When generations in captivity or sex were added to this top model the weights dropped to 0.181 and 0.168 respectively (Table 2). The interaction between generations in captivity and stimulus is not retained in any of the top models for foraging (Table 2) and adding generations in captivity to the top model results in a decrease in model weight from 0.242 to 0.181 (Table 2). Models for both behaviors of interest which had AICc weights <0.001 and are presented in Supplementary Materials.
Table 1. Candidate models for the proportion of time marmots allocated to vigilance or time in the burrow ranked by AICc weight, with degrees of freedom (DF), log-likelihoods, AICc values, ΔAICc values, and AICc weights.
Table 2. Candidate models for the proportion of time marmots allocated to foraging ranked by AICc weight, with degrees of freedom (DF), log-likelihoods, AICc values, ΔAICc values, and AICc weights.
Marmots decreased foraging and increased vigilance at the burrow or time in the burrow in response to all avian stimuli relative to the blank (Supplementary Material).
Marmots responded to all mammal stimuli by decreasing foraging, and responded more strongly to the wolf than any other stimulus by decreasing foraging and increasing vigilance at the burrow or time in the burrow. The greatest decrease in foraging relative to the blank was in response to the wolf, followed by the cougar, then goat, marmot, and cart (Figure 1; Table 3).
Figure 1. Mean proportion of time and 95% CI per 15 s time bin that marmots (n = 34) spent foraging when presented with each of four mammal taxidermy mounts, a cart, and when a blank was scored. Baseline value represents proportion of 60 s prior to the stimulus being presented that marmots allocated to foraging.
Table 3. Model average mixed-effects models for the proportion of time Vancouver Island marmots (n = 34) allocated to behaviors of interest in response to taxidermy mounts of mammals, as well as an empty cart and a blank, where no stimulus was presented.
After decreasing foraging when each stimulus was presented, marmots gradually resumed foraging; relative to the first 15 s time bin of the presentation minute marmots allocated more time to foraging in the second, third, and fourth 15 s time bins (Figure 1; Table 3). Presenting stimuli to the marmots following a Latin squares design allowed us to control for the effect of presentation order and, not surprisingly, as trial number increased marmots allocated more time to foraging (Table 3).
Aside from decreasing foraging relative to the blank, marmots also allocated more time to vigilance at the burrow or time in the burrow (Figure 2). Our average mixed-effects model suggests marmots allocated significantly more time to vigilance at the burrow or time in the burrow (Figure 2) when presented with the wolf relative to the blank, however there were no significant differences relative to the blank for any other stimulus (Table 3).
Figure 2. Mean proportion of time and 95% CI per 15 s time bin that marmots (n = 34) spent vigilant at the burrow or in the burrow when presented with each of four mammal taxidermy mounts, a cart, and when a blank was scored. Baseline value represents proportion of 60 s prior to the stimulus being presented that marmots allocated to vigilance at the burrow or time in the burrow.
Wild-caught marmots increased vigilance and decreased foraging relative to the blank in response to all avian stimuli and the greatest responses exhibited were to the eagle and owl. However, third, fourth, and fifth generation captive-born marmots responded as strongly to the cart as they did to the predators and non-predators (Supplementary Material).
Wild caught, and captive-born marmots in all generations responded to mammal stimuli by increasing vigilance at the burrow or time in the burrow relative to the blank (Figure 3). However, wild-caught and first and second generation captive-born marmots generally did not differ in the amount of time allocated to vigilance at the burrow or in the burrow (Figures 3A,B; Table 3), while third, fourth, and fifth generation captive-born marmots allocated more time to vigilance at the burrow or in the burrow in response to all stimuli (Figure 3C; Table 3). Also, wild-caught and first and second generation captive born marmots had little difference between time allocated to vigilance or in the burrow for the blank and the cart (Figures 3A,B), however third, fourth, and fifth generation captive born marmots increased vigilance and time in the burrow in response to the cart, the predators, and non-predators together (Figure 3C). Despite increasing vigilance in response to all stimuli, the proportion of time allocated in response to the cougar was similar to the proportion allocated to vigilance in response to the marmot and cart (Figure 3C). This suggests these marmots are equally wary of this potential predator, a conspecific, and a control stimulus.
Figure 3. Mean proportion of time per 15 s time bin that Vancouver Island marmots (n = 34) allocated to vigilance at the burrow or time in the burrow in response to mammal stimuli and when a blank was scored. Panels from left to right; (A) wild-caught (n = 17), (B) captive-born 1st and 2nd generation (n = 11), and (C) captive-born 3rd, 4th, and 5th generation (n = 6).
Unlike for vigilance, models including the interaction between generations in captivity and stimulus for the proportion of time allocated to foraging carried no weight in our model set relative to the top model (Table 2). In addition, adding generations in captivity to the top model resulted in a decrease in model weight (Table 2) indicating this variable is of little importance for this response. Wild-caught marmots allocated less time to foraging in response to the predators (wolf and cougar) than to the non-predators (goat and marmot), and the cart (Figure 4A), and marmots that were first and second generation captive-born responded similarly to predators, non-predators, and the cart (Figure 4B). Marmots that were third, fourth and fifth generation captive-born also decreased foraging relative to the blank, however their responses to all stimuli but the wolf were similar (Figure 4C), suggesting these marmots may identify wolves as cause for alarm, but not cougars. However, that the interaction between generations in captivity and stimulus is not retained in any top models suggests that these differences should be interpreted with caution.
Figure 4. Mean proportion of time by 15 s time bin that Vancouver Island marmots (n = 34) allocated to foraging in response to various mammal stimuli and while a blank was scored. Panels from left to right; (A) Wild-caught (n = 17), (B) captive-born 1st and 2nd generation (n = 11), and (C) captive born 3rd, 4th, and 5th generation (n = 6).
Increasing the generations in captivity resulted in loss of predator discrimination for Vancouver Island marmots which could have implications for their survival upon release to the wild. This loss of anti-predator behavior is unsurprising because captivity eliminates, or at least lowers, the threat of predation (Geffroy et al., 2020), as has been documented in a growing body of literature. For example, 16 species of birds reduced anti-predator behavior relative to wild-caught conspecifics in one captive generation (Carrete and Tella, 2015). Marmots in this study that have been captive for more than three generations have reduced ability to discriminate between one of their most significant predators (cougar) and non-predators, or controls (goat, marmot, and cart). Marmots exhibited a similar loss of predator recognition in response to avian stimuli as well, though our avian sample size was small. Interestingly, these marmots that have been captive for three to five generations had larger responses to experimental stimuli than marmots that were wild-caught or have been in captivity for one or two generations. While an increase of vigilance or time in the burrow in response to all stimuli may seem potentially beneficial, having that increase coupled with a loss of discrimination between predators and non-predators is concerning. For example, increasing vigilance in both elk (Cervus canadensis) and bison (Bison bison) reduced food consumption (Fortin et al., 2004). Due to the risk that predators present to marmots in the wild, vigilance and fleeing to a burrow can clearly be beneficial, however, this comes at a cost of reduced time foraging, and potentially lower body condition. Given that 26% of marmots die in hibernation each year, this tradeoff could be problematic. A comparison of the loss of anti-predator behavior in response to domestication, urbanization, and captivity found that loss due to captivity occurred more slowly over time (Geffroy et al., 2020). However, it's worth noting that domesticated herbivores lost anti-predator behaviors more rapidly than omnivores or carnivores and solitary animals lost these behaviors more quickly than social ones (Geffroy et al., 2020). If the same is true for captive animals, rather than domesticated, as social herbivores, marmots may lose anti-predator behaviors at a more moderate rate.
Predation accounted for 62% of mortality where the cause was confirmed and a previous study found 61% of marmots were killed by predators (Jackson et al., 2016), with the majority of marmots being killed by cougars and eagles, followed by wolves (Aaltonen et al., 2009; Jackson et al., 2016; MRF unpublished data). Captive-born marmots have higher mortality than wild-born marmots, and cougars depredate significantly more captive-born than wild-born marmots. However, there was no significant difference in wolf predation between captive and wild-born marmots (Aaltonen et al., 2009). Marmots responded more strongly to wolves than to cougars, regardless of the number of generations they have been in captivity, whereas the marmots' ability to discriminate between cougars and non-predators seems to diminish over time. Therefore, marmots retain anti-predator responses to wolves, but lose the ability to discriminate between cougars and non-predators, despite that cougars killed more marmots than do wolves. This could be either because marmots are better able to avoid predation by wolves, or because cougars are the greater threat.
One possible explanation for marmots' retention of a predator template for wolves is that perhaps wolves were historically a more significant predator than they have been for the last century. Wolves were hunted heavily on Vancouver Island for many years in an attempt to eradicate them, and were effectively extirpated from between 1950 and 1970 (Muñoz-Fuentes et al., 2009). In the greater Yellowstone ecosystem when wolves were reintroduced and wolf and cougar activity areas overlapped, cougars began making kills at higher elevations and in more rugged areas (Bartnick et al., 2013). Cougar populations on Vancouver Island have been gradually declining since the early to mid-1990s (Hatter, 2019), but it is unclear if there is a relationship between population trends in these two species. Steindler et al. (2018) found that greater bilbies (Macrotis lagotis) had greatest retention of anti-predator templates in response to a predator with which they had a longest history. Perhaps marmots' retention of responses to wolves results from a longer shared history. Any predator template marmots currently have for wolves was either retained while wolves were extirpated or has developed since wolves returned to Vancouver Island. If marmots retained the template while wolves were extirpated it is not surprising that they also do so over the timeframe of this study. However, if marmots developed the ability to identify wolves as a predator since the late 1970s, it is unclear why they retain the predator template for wolves in this study but not cougars. In addition, the extirpation and reestablishment of wolves is just one way in which it is possible that the predator community into which marmots have been reestablished over the last 20 years is novel relative to the community in which they had survived in the past. For example, forestry operations have created high-elevation cut blocks which are colonized by marmots, but also draw cougars and wolves, and the construction of resource roads has increased cougar and wolf mobility in marmot habitat (Vancouver Island Marmot Recovery Team, 2017). With consistent changes to the predators that these marmots face in the wild, captive-bred individuals must be able to retain the ability to respond to the predators they would have faced historically, and adapt to the novel community of predators into which they will be released.
While the marmots in this study have not received predator awareness training (e.g., Griffin et al., 2000), they had the opportunity to view potential predators at the facility where they were housed. For example, coyotes (Canis latrans) can be found in the area and could potentially be observed by captive marmots from a distance. Though coyotes are absent from Vancouver Island, they may serve as a proxy for wolves. However, while marmots in this study are potentially exposed to predators, encountering potential predators when those predators pose no risk will not necessarily maintain anti-predator behavior. Therefore, it is possible that beyond five generations in captivity, and without additional intervention, marmots will lose the ability to discriminate between wolves and non-predators as well.
In order to retain or renew anti-predator behaviors in captive populations many programs develop predator avoidance training, where predator cues are paired with a stimulus that elicits a negative response in target animals (Griffin et al., 2000; Shier, 2016). Predator avoidance training in vertebrates is reported as successfully modifying behavior in 80% of studies but unsuccessful attempts may be underrepresented due to the bias toward publishing positive results (Edwards et al., 2021). Also, few studies report on survival in the wild post-release, and of those studies that did, approximately half were unsuccessful due to high mortality (Edwards et al., 2021). In some instances, captive animals were trained to avoid historical predators, only to suffer high mortality from novel sources (such as humans and dogs; Vera Cortez et al., 2015). In addition, rather than simply training target animals to recognize predators, it is important that predator avoidance training evaluates whether animals have a relevant coping strategy to predators (e.g., freezing, or fleeing; Edwards et al., 2021).
If predator avoidance training is to be undertaken as part of marmot captive breeding it should be informed by causes of mortality in the wild. Therefore, marmots should be trained to identify and respond to cougars and eagles, while efforts should be taken to retain their responses to wolves. Our results suggest that this training should be initiated in the second or third generation in captivity, if not sooner.
Studying endangered species often means having limited control over sample size and aspects of experimental design. This is of particular importance when our sample is subdivided into groups by generations in captivity. Though our sample of marmots that were 3rd, 4th, and 5th generation in captivity was particularly small (n = 6), the potential implications of the relationships we have observed should not be overlooked. With ca. 100 adult marmots in captivity and ca. 200 in the wild, our sample of 34 marmots represents ca. 11% of the global population. As such, the inferences we draw about the population may, ironically, be robust compared to a similar study of a non-endangered species where only a small fraction of the individuals are sampled. Because marmots that have been captive for more than three generations have only been available for study in the last few years, it is imperative to understand the impact captivity is having on behavior. In addition, our sample suffered from an imbalance of males and females. While our models did not suggest sex differences in marmot responses, it is unclear if this is due to an actual lack of differences or an imbalance in sampling. Future analyses may permit a more balanced sex ratio.
Much effort is placed in maintaining genetic diversity in captive populations (Willoughby et al., 2017) and while this is clearly important, this study suggests it is also important to include the maintenance of behavior as well. This captive population of marmots has retained genetic diversity relative to the wild population over the last three generations (Barrett et al., 2021), despite the loss of anti-predator behavior described here. Therefore, this study highlights the importance of evaluating whether individuals in captive populations change behaviors that may affect post-release fitness. Taken alone, results from Blumstein et al. (2006) suggested there was no effect of captivity on anti-predator behavior. However, including marmots born in captivity for several more generations suggests otherwise. Even for programs that include periodic training to maintain anti-predator behavior it would be beneficial to continue to monitor the effectiveness of their training.
The captive environment is novel for the wild-caught animals that establish captive breeding populations, and life in the wild is novel for captive-born individuals upon release. In addition to studying anti-predator behaviors of animals in captivity before their release to the wild, it is essential to continue studying those individuals after release as well. Given the large proportion of mortality attributed to predation in reintroduction programs (Berger-Tal et al., 2020) understanding the relationship between anti-predator behavior in captivity and mortality from predation in the wild is crucial for the success of captive breeding and reintroduction programs. We recommend that conservation translocation breeding programs conduct behavior assessments early, and continue to re-assess captive individual's behavior at regular intervals throughout a program. It is also essential that there is regular collaboration between those managing the captive and wild populations to inform how both populations are managed. Captive breeding can be a valuable tool to reestablish wildlife populations, and ensure those populations persist, however one cannot assume that an individual in captivity is prepared for life in the wild, and behavioral studies such as this are one of many pieces needed for success.
The raw data supporting the conclusions of this article will be made available by the authors, without undue reservation.
The animal study was reviewed and approved by the Calgary Zoo Welfare and Ethics in Research Committee, the Marmot Recovery Foundation, and the UCLA Animal Research Committee.
GD-M scored videos of marmots, drafted the manuscript, and assisted in planning and conducting analysis. JR conducted statistical analyses and edited the manuscript. NL developed the project, planned the experimental trials, edited the manuscript and assisted in planning, and interpreting analysis. DB conducted the study on which this one is based, assisted in repeating the trials, planning and interpreting statistical analyses, and editing the manuscript. AM developed the project, contributed in project planning, assisted in planning and interpreting statistical analyses, and edited the manuscript. All authors contributed to the article and approved the submitted version.
The authors declare that the research was conducted in the absence of any commercial or financial relationships that could be construed as a potential conflict of interest.
All claims expressed in this article are solely those of the authors and do not necessarily represent those of their affiliated organizations, or those of the publisher, the editors and the reviewers. Any product that may be evaluated in this article, or claim that may be made by its manufacturer, is not guaranteed or endorsed by the publisher.
We kindly thank B.D. Holland for advice and transfer of original materials. Thank you also to all who assisted with this study: C. Baird, V. Edwards, H. Elliott, J. Gellatly, L. Haines, L. Hébert, R. Kelly, A. Langill, F. LeTaro, M. Miskolczi, L. Payette-Brisson, L. Riches, C. Slade, K. Swan, K. Twamley, R. Wenman, and D. Whiteside. Thank you to the Marmot Recovery Foundation for kindly providing the post-release survival data. C. Jackson and A. Taylor for reviewing a draft of the manuscript. We also thank the reviewers, who made valuable contributions to the manuscript.
The Supplementary Material for this article can be found online at: https://www.frontiersin.org/articles/10.3389/fcosc.2021.718562/full#supplementary-material
Aaltonen, K., Bryant, A. A., Hostetler, J. A., and Oli, M. K. (2009). Reintroducing endangered Vancouver Island marmots: survival and cause-specific mortality rates of captive-born vs. wild-born individuals. Biol. Conserv. 142, 2181-2190. doi: 10.1016/j.biocon.2009.04.019
Barrett, C., and Stehn, T. V. (2010). A retrospective of whooping cranes in captivity,” in Proceedings of the North American Crane Workshop (Wisconsin Dells, WI).
Barrett, K. G., Amaral, G., Elphinstone, M., Mcadie, M. L., Davis, C. S., Janes, J. K., et al. (2021). Genetic Management on the Brink of Extinction: Sequencing Microsatellites Does Not Improve Estimates of Inbreeding in Wild and Captive Vancouver Island Marmots (Marmota vancouverensis) Conservation Genetics.
Bartnick, T. D., Van Deelen, T. R., Quigley, H. B., and Craighead, D. (2013). Variation in cougar (Puma concolor) predation habits during wolf (Canis lupus) recovery in the southern greater yellowstone ecosystem. Can. J. Zool. 91, 82-93. doi: 10.1139/cjz-2012-0147
Bartoń, K.. (2020). MuMIn: Multi-Model Inference. 1.43.17. Available online at: https://CRAN.R-project.org/package=MuMIn (accessed June 28, 2021).
Berger-Tal, O., Blumstein, D. T., and Swaisgood, R. R. (2020). Conservation translocations: a review of common difficulties and promising directions. Anim. Conserv. 23:10. doi: 10.1111/acv.12534
Bissonette, J. A.. (1999). Small sample size problems in wildlife ecology: a contingent analytical approach. Wildl. Biol. 5, 65–71. doi: 10.2981/wlb.1999.010
Blumstein, D. T., Daniel, J. C., and Evans, C. S. (2020). JWatcher 1.0. Available online at: jwatcher.ucla.edu (accessed February 1, 2021).
Blumstein, D. T., Daniel, J. C., and Springett, B. P. (2004). A test of the multi-predator hypothesis: rapid loss of antipredator behavior after 130°years of isolation. Ethology 110, 919–934. doi: 10.1111/j.1439-0310.2004.01033.x
Blumstein, D. T., Holland, B. D., and Daniel, J. C. (2006). Predator discrimination and “personality” in captive Vancouver Island marmots (Marmota vancouverensis). Anim. Conserv. 9, 274-282. doi: 10.1111/j.1469-1795.2006.00033.x
Bradley, J. V.. (1958). Complete counterbalancing of immediate sequential effects in a latin square design. J. Am. Stat. Assoc. 53:4. doi: 10.2307/2281966
Brichieri-Colombi, T. A., Lloyd, N. A., Mcpherson, J. M., and Moehrenschlager, A. (2018). Limited contributions of released animals from zoos to North American conservation translocations. Conserv. Biol. 33, 33-39. doi: 10.1111/cobi.13160
Brichieri-Colombi, T. A., and Moehrenschlager, A. (2016). Alignment of threat, effort, and perceived success in North American conservation translocations. Conserv. Biol. 30, 1159-1172. doi: 10.1111/cobi.12743
Brooks, M. E., Kristensen, K., Van Benthem, K. J., Magnusson, A., Berg, C. W., Nielsen, A., et al. (2017). glmmTMB balances speed and flexibility among packages for zero-inflated generalized linear mixed modeling. R J. 9, 378–400. doi: 10.32614/RJ-2017-066
Bryant, A. A.. (2005). Reproductive rates of wild and captive Vancouver Island marmots (Marmota vancouverensis). Can. J. Zool. 83, 664-673. doi: 10.1139/z05-056
Burnham, K. P., and Anderson, D. R. (2002). Model Selection and Multimodel Inference: A Practical Information-Theoretic Approach. New York, NY: Springer-Verlag.
Carrete, M., and Tella, J. L. (2015). Rapid loss of antipredatory behaviour in captive-bred birds is linked to current avian invasions. Sci. Rep. 5:18274. doi: 10.1038/srep18274
Casimir, D. L., Moehrenschlager, A., and Barclay, R. M. R. (2007). Factors influencing reproduction in captive vancouver island marmots: implications for captive breeding and reintroduction programs. J. Mammal. 88, 1412-1419. doi: 10.1644/06-MAMM-A-264R1.1
COSEWIC (2008). “COSEWIC assessment and update status report on the Vancouver Island marmot Marmota vancouverensis in Canada,” in Committee on the Status of Endangered Wildlife in Canada, ed E. Canada (Ottawa).
Edwards, M. C., Ford, C., Hoy, J. M., Fitzgibbon, S., and Murray, P. J. (2021). How to train your wildlife: a review of predator avoidance training. Appl. Anim. Behav. Sci. 234:105170. doi: 10.1016/j.applanim.2020.105170
Fortin, D., Boyce, M., Merrill, E. H., and Fryxell, J. M. (2004). Foraging costs of vigilance in large mammalian herbivores. Oikos 107, 172–180. doi: 10.1111/j.0030-1299.2004.12976.x
Frankham, R., Hemmer, H., Ryder, O. A., Cothran, E. G., Soulé, M. E., Murray, N. D., et al. (1986). Selection in captive populations. Zoo Biol. 5, 127-138. doi: 10.1002/zoo.1430050207
Geffroy, B., Sadoul, B., Putman, B. J., Berger-Tal, O., Garamszegi, L. Z., Moller, A. P., et al. (2020). Evolutionary dynamics in the Anthropocene: life history and intensity of human contact shape antipredator responses. PLoS Biol 18:e3000818. doi: 10.1371/journal.pbio.3000818
Greggor, A. L., Berger-Tal, O., Blumstein, D. T., Angeloni, L., Bessa-Gomes, C., Blackwell, B. F., et al. (2016). Research priorities from animal behaviour for maximising conservation progress. Trends Ecol. Evol. 31, 953-964. doi: 10.1016/j.tree.2016.09.001
Griffin, A. S., Blumstein, D. T., and Evans, C. S. (2000). Training captive-bred or translocated animals to avoid predators. Conserv. Biol. 14, 1317–1326. doi: 10.1046/j.1523-1739.2000.99326.x
Harrington, L. A., Moehrenschlager, A., Gelling, M., Atkinson, R. P., Hughes, J., and Macdonald, D. W. (2013). Conflicting and complementary ethics of animal welfare considerations in reintroductions. Conserv. Biol. 27, 486-500. doi: 10.1111/cobi.12021
Hatter, I. W.. (2019). Statistical Population Reconstruction of Cougars in British Columbia. Nelson, BC: Nature Wise Consulting.
Hoffmann, M., Duckworth, J. W., Holmes, K., Mallon, D. P., Rodrigues, A. S., and Stuart, S. N. (2015). The difference conservation makes to extinction risk of the world's ungulates. Conserv. Biol. 29, 1303-1313. doi: 10.1111/cobi.12519
Hoffmann, M., Hilton-Taylor, C., Angulo, A., Böhm, M., Brooks, T. M., Butchart, S. H. M., et al. (2010). The impact of conservation on the status of the world's vertebrates. Science 330, 1503-1509. doi: 10.1126/science.1194442
Houston, A. I., Mcnamara, J. M., and Hutchinson, J. M. C. (1993). General results concerning the trade-off between gaining energy and avoiding predation. Philos. Trans. R. Soc. Lond., B, Biol. Sci. 341, 375-397. doi: 10.1098/rstb.1993.0123
IPBES (2019). Summary for Policymakers of the Global Assessment Report on Biodiversity and Ecosystem Services of the Intergovernmental Science-Policy Platform on Biodiversity and Ecosystem Services. IPBES Secretariat.
Islam, M. Z.-U., Ismail, K., and Boug, A. (2013). Restoration of the endangered Arabian Oryx Oryx leucoryx, Pallas 1766 in Saudi Arabia lessons learnt from the twenty years of re-introduction in arid fenced and unfenced protected areas. Zool. Middle East 54, 125-140. doi: 10.1080/09397140.2011.10648904
IUCN/SSC (2013). Guidelines for Reintroductions and Other Conservation Translocations. Version 1.0.IUCN Species Survival Commission.
Jachowski, D. S., Gitzen, R. A., Grenier, M. B., Holmes, B., and Millspaugh, J. J. (2011). The importance of thinking big: large-scale prey conservation drives black-footed ferret reintroduction success. Biol. Conserv. 144, 1560-1566. doi: 10.1016/j.biocon.2011.01.025
Jackson, C. L., Schuster, R., and Arcese, P. (2016). Release date influences first-year site fidelity and survival in captive-bred Vancouver Island marmots. Ecosphere 7:e01314. doi: 10.1002/ecs2.1314
Lima, S. L., and Bednekoff, P. A. (1999). Temporal variation in danger drives antipredator behavior: the predation risk allocation hypothesis. Am. Nat. 153:10. doi: 10.1086/303202
Lloyd, N. A., Hostetter, N. J., Jackson, C. L., Converse, S. J., and Moehrenschlager, A. (2018). Optimizing release strategies: a stepping-stone approach to reintroduction. Anim. Conserv. 22, 105-115. doi: 10.1111/acv.12448
Lüdecke, D., Ben-Shachar, M. S., Patil, I., Waggoner, P., and Makowski, D. (2021). Performance: an R package for assessment, comparison and testing of statistical models. J. Open Source Softw. 6:3139. doi: 10.21105/joss.03139
McPhee, M. E.. (2003). Generations in captivity increases behavioral variance: considerations for captive breeding and reintroduction programs. Biol. Conserv. 115, 71–77. doi: 10.1016/S0006-3207(03)00095-8
Moseby, K. E., Read, J. L., Paton, D. C., Copley, P., Hill, B. M., and Crisp, H. A. (2011). Predation determines the outcome of 10 reintroduction attempts in arid South Australia. Biol. Conserv. 144, 2863-2872. doi: 10.1016/j.biocon.2011.08.003
Muñoz-Fuentes, V., Darimont, C. T., Paquet, P. C., and Leonard, J. A. (2009). The genetic legacy of extirpation and re-colonization in Vancouver Island wolves. Conserv. Genet. 11, 547-556. doi: 10.1007/s10592-009-9974-1
Roach, N.. (2017). “Marmota vancouverensis, Vancouver Island Marmot” in The IUCN Red List of Threatended Species.. Available online at: https://doi.org/10.2305/IUCN.UK.2017-2.RLTS.T12828A22259184.en (accessed October 5, 2020).
RStudio Team (2020). RStudio: Integrated Development for R. Available online at: http://www.rstudio.com/ (accessed October 5, 2020).
Seddon, P. J., Armstrong, D. P., and Maloney, R. F. (2007). Developing the science of reintroduction biology. Conserv. Biol. 21, 303-312. doi: 10.1111/j.1523-1739.2006.00627.x
Shaw, R. C., Greggor, A. L., and Plotnik, J. M. (2021). The challenges of replicating research on endangered species. Anim. Behav. Cogn. 8, 240-246. doi: 10.26451/abc.08.02.10.2021
Shier, D.. (2016). “Manipulating animal behavior to ensure reintroduction success,” in Conservation Behavior: Applying Behavioral Ecology to Wildlife Conservation and Management, eds D. Saltz, and O. Berger-Tal (Cambridge: Cambridge University Press), 275–304. doi: 10.1017/CBO9781139627078.014
Steindler, L. A., Blumstein, D. T., West, R., Moseby, K. E., and Letnic, M. (2018). Discrimination of introduced predators by ontogenetically naïve prey scales with duration of shared evolutionary history. Anim. Behav. 137, 133-139. doi: 10.1016/j.anbehav.2018.01.013
Swaisgood, R.. (2010). The conservation-welfare nexus in reintroduction programmes: a role for sensory ecology. Anim. Welf. 19, 125–137.
Vancouver Island Marmot Recovery Team (2017). Recovery Plan for the Vancouver Island Marmot (Marmota vancouverensis) in British Columbia. Vancouver Island Marmot Recovery Team.
Vera Cortez, M., Valdez, D. J., Navarro, J. L., and Martella, M. B. (2015). Efficiency of antipredator training in captive-bred greater rheas reintroduced into the wild. Acta Ethol. 18, 187-195. doi: 10.1007/s10211-014-0206-4
Willoughby, J. R., Ivy, J. A., Lacy, R. C., Doyle, J. M., and Dewoody, J. A. (2017). Inbreeding and selection shape genomic diversity in captive populations: Implications for the conservation of endangered species. PLoS ONE 12:e0175996. doi: 10.1371/journal.pone.0175996
Keywords: conservation translocation, reintroduction, anti-predator, behavior, vigilance, captive breeding
Citation: Dixon-MacCallum GP, Rich JL, Lloyd N, Blumstein DT and Moehrenschlager A (2021) Loss of Predator Discrimination by Critically Endangered Vancouver Island Marmots Within Five Generations of Breeding for Release. Front. Conserv. Sci. 2:718562. doi: 10.3389/fcosc.2021.718562
Received: 01 June 2021; Accepted: 29 October 2021;
Published: 03 December 2021.
Edited by:
Katherine Moseby, University of New South Wales, AustraliaReviewed by:
David Saltz, Ben-Gurion University of the Negev, IsraelCopyright © 2021 Dixon-MacCallum, Rich, Lloyd, Blumstein and Moehrenschlager. This is an open-access article distributed under the terms of the Creative Commons Attribution License (CC BY). The use, distribution or reproduction in other forums is permitted, provided the original author(s) and the copyright owner(s) are credited and that the original publication in this journal is cited, in accordance with accepted academic practice. No use, distribution or reproduction is permitted which does not comply with these terms.
*Correspondence: Graham P. Dixon-MacCallum, Z3JhaGFtZG1AY2FsZ2FyeXpvby5jb20=
Disclaimer: All claims expressed in this article are solely those of the authors and do not necessarily represent those of their affiliated organizations, or those of the publisher, the editors and the reviewers. Any product that may be evaluated in this article or claim that may be made by its manufacturer is not guaranteed or endorsed by the publisher.
Research integrity at Frontiers
Learn more about the work of our research integrity team to safeguard the quality of each article we publish.