- 1Conservation Science and Wildlife Health, San Diego Zoo Wildlife Alliance, Escondido, CA, United States
- 2Pacific Internship Programs for Exploring Science, University of Hawai‘i at Hilo, Hilo, HI, United States
- 3Department of Biology, University of Hawai‘i at Hilo, Hilo, HI, United States
Although supplemental feeding is commonly used as a conservation strategy during animal translocations, it comes with a number of pros and cons which can be hard to quantify. Providing additional food resources may lead to improved physical health, survivorship, and reproduction. However, offering predictable food sources could make individuals more conspicuous to predators and less aware of their surroundings, disrupting their natural predator-prey dynamic. Decisions such as release cohort size and supplemental feeder design could influence the balance of these costs and benefits, depending on how animals behave in the face of predation risk and static food sources. Additionally, animals released to the wild from long term human care must balance foraging and predation risk while adjusting to a novel environment. To help conservation managers make informed decisions in light of these potential costs, we studied the behavior of a cohort of 11 conservation-bred ‘alalā (Corvus hawaiiensis) at supplemental feeding stations after release into the wild. Vigilance, foraging behavior and social group size was quantified via 1,320 trail camera videos of ‘alalā over the span of 12 months. We found that vigilance increased over time since release, suggesting that ‘alalā learn and adjust to their novel surroundings. Both vigilance and eating decreased with group size, indicating that although conspecifics may share the burden of scanning for threats, they also increase competition for food. We also found that the design of the feeder may have limited birds' abilities to express anti-predator behavior since less vigilance was observed in individuals that manipulated the feeder. Yet, birds may have been able to offset these costs since they increasingly scrounged for food scraps next to the feeder as time progressed. We discuss how changes to behavior over time, social interactions, and feeder design should all be considered when planning supplemental feeding as part of wildlife translocations.
Introduction
Humans often disrupt the natural balance between predators and their prey (Carthey and Blumstein, 2018). By inadvertently introducing non-native predators into delicate ecosystems, or subsidizing natural predators by introducing abundant non-native prey, we increase the vulnerability of native prey to predation. Furthermore, bringing animals into human care as part of conservation efforts can disrupt and erode natural anti-predator responses (McPhee and Carlstead, 2010; Crane et al., 2015; Shier, 2016). Accordingly, an unstable predator-prey relationship can be a major factor leading to species decline or can impede recovery efforts. In the case of conservation translocations, animals are released into novel environments and are highly vulnerable to predation due to a lack of specific knowledge about predators, and the locations of cover, refuge and other habitat features that help prey avoid detection or evade threats. Predation can have direct negative effects on translocation outcomes since naïve animals easily succumb to predation (Berger-Tal et al., 2020) or indirect effects, since time spent engaged in anti-predator behavior, such as vigilance, may prevent animals from gaining fitness benefits from foraging or breeding (Lima and Dill, 1990; Brown et al., 1999). These costs of translocation are likely to be more severe in the initial period following release, before animals have fully adjusted to the novel environment and become familiar with predators, varying predation risks on the landscape, and effective anti-predator behaviors.
One translocation tool that has the potential to influence predator/prey dynamics is supplemental feeding. Supplemental feeding is commonly used at release sites as a strategy to increase food resource availability for species that are resource-limited due to anthropogenic habitat change or for naïve reintroduced animals that have not yet learned to exploit wild food (Boutin, 1990; Ruiz-Miranda and Swaisgood, 2019). Thus, supplemental feeding is often prioritized in the initial period following release to counter the challenges of learning to forage in a novel environment. If done well, supplemental feeding can also help anchor animals to the release site, which can increase survival (Lockwood et al., 2005; Liu et al., 2016) and reproduction (Rodriguez-Hidalgo et al., 2010), facilitate monitoring and help animals take advantage of other management actions that may occur there, such as invasive predator control, or habitat restoration. However, provisioning food to animals also comes at a cost. Supplemental feeding can influence space use (Mertes et al., 2019), spread disease (Sorensen et al., 2014), and potentially increase predation risk if predators learn prey are concentrated at a resource (although evidence for the latter is slim; see Robb et al., 2008). These costs are not always straightforward to predict, however, because supplemental feeding could also decrease predation if food resources decrease time spent in dangerous foraging activities or good nutritional condition renders evasive actions more effective. As a consequence, supplemental feeding should be discontinued once animals have learned to forage and exploit the release environment for food resources sufficiently for survival and reproduction.
Prey species can also adopt behavioral strategies for avoiding predation. Vigilance is a behavioral adaptation in which animals monitor their surroundings for potential threats (Quenette, 1990; Treves, 2000; Beauchamp, 2015). While engaging in vigilance may reduce vulnerability to predators and competitors, excessive vigilance takes time away from foraging, social behavior, and other survival-relevant activities (Lima, 1987; Lima and Dill, 1990). Documenting how animals balance vigilance and eating at supplemental feeders can offer a window into the risk assessment of released animals, whereby an increased level of vigilance behavior through time may indicate learning about predation. Therefore, assessing vigilance at feeders can help evaluate the behavioral competency of reintroduced animals and how elements of the release strategy may interact with a species' basic behavioral biology. For instance, decisions made about release cohort size may influence the likelihood of animals exhibiting a beneficial balance of vigilance and feeding, since having larger groups of animals influences vigilance levels in many species (Colagross and Cockburn, 1993; Roberts, 1996). This group size effect predicts that individuals in larger groups have less need for vigilance since larger groups have more eyes to look out for threats and offer a reduced individual likelihood of predation (Saino, 1994; Ward and Low, 1997). However, larger groups also deplete resources more quickly, can be more conspicuous, and have other associated costs, such as conspecific aggression (Robinette and Ha, 2001).
Additionally, animals' anti-predator responses are often not static. Many species respond flexibly to changes in predation risk, therefore the perceived costs of foraging in translocation contexts may change over time as animals gain more information about their novel environment. During initial release periods, animals may have to take riskier strategies, if they are unable to locate alternative food sources. Yet, as animals become more aware of the activity of predators, presence of conspecifics or foraging options, they may be able to more safely exploit resources (Berger-Tal and Saltz, 2014). Being able to learn post-release has been shown to help boost survival (Krochmal et al., 2018), but animals commonly fail to learn the behaviors they need (Berger-Tal et al., 2020). Therefore, identifying changes in how animals interact with or use supplemental feeders over time may serve as one marker of their adjustment to the novel dangers of the wild, and a sign of learning. Specifically, the temporal pattern of post-release changes in reliance on supplemental food and vigilance behavior provide insights into patterns of learning how to forage on natural foods and accumulation of knowledge about predation risk.
We illustrate the utility of investigating the complex interactions between social and antipredator behavior at supplemental feeders, with newly released ‘alalā (Corvus hawaiiensis). ‘Alalā, i.e., the Hawaiian crow, are the only extant endemic corvid species in the Hawaiian Islands. They are the sole seed disperser for many native plants (Culliney et al., 2012), and they have a complex social system, which varies with age. Younger birds are gregarious with a hierarchy of dominant and submissive birds. As they mature, they form pair bonds and establish territories which they defend from conspecifics (Banko et al., 2002). Their only native predator is the ‘io, the Hawaiian hawk (Buteo solitarius). ‘Alalā declined severely in the late twentieth century due to threats of predation by non-native mammals such as mongooses, rats, and feral cats, habitat degradation, disease and human conflict (U. S. Fish Wildlife Service, 2009). Despite conservation breeding and releases for the ‘alalā in the 1990s (Keuhler et al., 1995), ‘alalā were declared extinct in the wild in 2002 (U. S. Fish Wildlife Service, 2009). A new wave of reintroductions began in 2016 once the conservation breeding population had stabilized. Release strategies have thus far explicitly targeted the gregarious juvenile stage for release, in the hope that larger groups of birds would help each other in anti-predator defense, as has been documented in some, but not all corvid species (Dlaz and Asensio, 1991; Henderson and Hart, 1991; Ward and Low, 1997; Rolando et al., 2001). However, the extent to which corvid comparisons on the group size effect translate to ‘alalā may depend on the social system being compared, which varies greatly between corvid species. Also, it was anticipated that release birds' conservation breeding background might impact their anti-predator behavior since they did not develop with the same predation threats as their ancestors (McPhee and Carlstead, 2010; Crane et al., 2015; Shier, 2016). In attempts to combat their potential predator naivety, all birds were exposed to anti-predator training prior to release (Greggor et al., 2021).
As part of post-release monitoring efforts for ‘alalā, and to better assess the anti-predator costs of providing supplemental food, we measured the prevalence of vigilance and eating behavior within naturally forming social groups at feeding stations. We used camera trap videos taken during the 1st year post-release of the 11 birds released in 2017. By doing so, we hoped to determine whether the birds' vigilance for aerial threats changed over time and was influenced by the size of foraging groups. We hypothesized that the ‘alalā would either exhibit lower vigilance over time as they overcame any initial post-release stress in response to novelty, or would increase vigilance over time if the birds discovered elevated predation risk. Additionally, should the custom plastic food feeder impose costs in terms of anti-predator behavior, we would expect to see decreased vigilance when birds interact with it. However, having multiple group mates around could offset this cost, if ‘alalā are able to be less vigilant and eat more food when conspecifics are present. Such a group effect would support the management strategy of releasing larger, socialized, juvenile cohorts as a way of combatting predation risk.
Materials and Methods
Study Area
This study examines trends in vigilance in ‘alalā at supplemental feeding stations from October 2017 to September 2018 in the Pu'u Maka'ala Natural Area Reserve. The study covered 11 newly released ‘alalā: seven males and four females. They were hatched in 2016 from the Keauhou Bird Conservation Center on Hawai‘i island, and were reared either by hand with ‘alalā puppets or by adult ‘alalā in large outdoor aviaries. Each bird was identified with a unique combination of a metal and three colored plastic leg bands. The 11 birds were released in two separate groups, one late September 2017, the other in early October 2017. The habitat surrounding the release site is primarily mesic and wet forest. ‘Ohi‘a trees (Meterosideros polymorpha) dominate the forest canopy, with a variety of native fruiting trees and ferns composing the lower tiers. Historically, cattle grazed portions of the reserve, resulting in patches of grassland and shrubland. The reserve is also home to ‘io, and other potential predators include introduced mammals such as small Indian mongooses (Herpestes javanicus), feral cats (Felis catus), and rats (Rattus sp.).
Daily supplemental food was provided in the forest near the release site at three to four stations. The stations consisted of an elevated platform upon which sat a feeder (made by Tuft Plastic Molders) which birds were trained to open prior to release (see video in Supplementary Material). Due to the size, weight, and design of the hopper, ‘alalā were the only species in the area capable of manipulating the lid. Other species were only recorded at the station in rare instances, but their data was not used as part of this study. Throughout the day as ‘alalā foraged from the feeder, food scraps would accumulate on the feeding platform. The platforms were cleaned daily when the feeder was removed at dusk or in the late afternoon, leaving no food available until the following morning. Each station was periodically moved around the site as needed for management reasons.
Data Collection
On any given day, up to three of the supplemental feeding stations were equipped with a Bushnell Trophy Trail Camera. The cameras collected 10 s of video footage when they detected motion at stations, with 2 s gaps between consecutive videos. Collecting data via non-obtrusive trail cameras increased the likelihood that we would obtain a representative sample of feeding events, and that vigilance behaviors would not be biased by human observers. Each day field monitoring staff reviewed the footage, saved clips with identifiable ‘alalā and named the videos with the date and birds' band combinations. This study focuses on a subset of the tens of thousands of videos collected during the first full year post-release. Ten videos per bird per month were randomly selected in R (R Core Team, 2019), for a total of 120 videos per bird. In videos with multiple ‘alalā present, the focal bird was labeled as the first in view of that video sequence or the closest to the camera. Videos in which the focal bird's behaviors were obscured were replaced with a new video chosen at random for the same bird within the same month.
A group of three researchers coded the behaviors in each video according to an ethogram (Table 1), noting the total number of ‘alalā present, and behaviors such as: aerial vigilance level (Figure 1), and whether the focal bird ate, opened the feeder, scrounged food removed from the feeder by others, flew away, or engaged in social behaviors. Vigilance was coded as an ordinal variable, to help capture an increasing expression of wariness, based on head angle, and with or without the presence of head movements (scanning), which would afford a wider field of view. We did not record duration or frequency for any of the behavioral variables because although the trail camera videos were a standard duration (10 s), the random subset we selected varied in whether they caught birds at the beginning, middle or end of a given behavior, which would mean that duration and frequency would often fail to accurately capture the full behavioral bout. When we recoded a subset of videos (16%), we found that the total number of birds and behaviors were consistently recorded (no. of birds, 97% concurrence between coders; vigilance, 89%; eats, 93%; opened feeder, 93%).
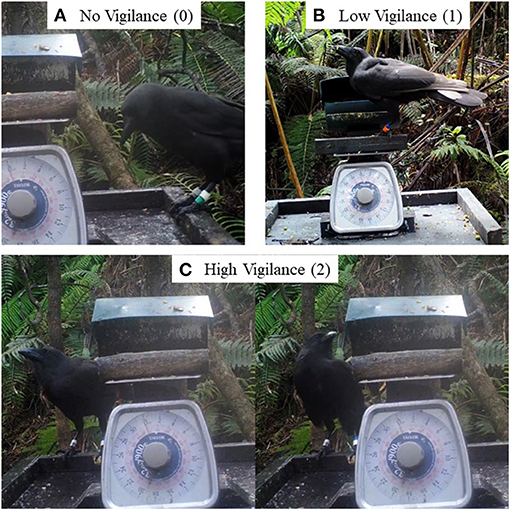
Figure 1. Photo depictions of vigilance levels. Each pane shows a different level of vigilance. Birds either (A) spent the entire video with their head oriented downwards, (B) glanced upwards in one direction at some point during the video, or (C) oriented their head in multiple directions during the video.
Analysis
The behavioral data were used to determine if vigilance, eating, and the effect of conspecifics on behavior changed over time. Specifically, we aimed to answer three main questions: (1) what predicted the occurrence of vigilance for aerial threats at the feeding stations, (2) what conditions predicted birds eating at the feeding stations, and (3) did birds adopt different feeding strategies depending on the number of conspecifics present? A separate model was built for each question and all were investigated with regards to time since release. Social behaviors, such as aggression or submission, occurred too infrequently for robust statistical analysis (7.6% of videos).
Vigilance behavior was separated into three categories, with birds showing no (0), low (1), or high levels of vigilance (2), and was analyzed using a Cumulative Link Mixed Model (CLMM) with a logit link function, using the “ordinal” package (Christensen, 2019). The total number of birds in the video, sex of the focal bird, time in months since release, the type of contact with the feeder, whether or not the bird ate, and whether they took flight during the video were all included as fixed, explanatory variables. Bird identity was used as a random effect to account for repeated measures of individuals over time. Controlling for individual variation is necessary since vigilance levels can vary at the individual level, even when group size effects are present (Carter et al., 2009).
The factors that influenced whether or not a bird ate (Y/N) were analyzed with a Generalized Linear Mixed Model (GLMM) with a binomial error structure and a logit link function. The total number of birds present in the videos, sex of the focal bird, time in months since release, and whether or not the bird opened the feeder were all considered as explanatory variables. Similar to the vigilance model, individual ID was used as a random effect.
Finally, using just the observations where birds were observed eating at the feeding stations, we investigated whether ‘alalā were more likely to scrounge from the platform than eat from the plastic feeder (scrounge Y/N), based on the number of birds present. Scrounging behavior, i.e., taking advantage of a resource provided by a “producer” (Barnard and Sibly, 1981), could be advantageous for ‘alalā if birds are able to consume scraps left by others without having to interact with the feeder itself. Since active scrounging via social interaction with a conspecific occurred exceedingly rarely (2.8%), only passive scrounging was considered. We used a GLMM with a binomial error structure and a logic link function. We included the additional covariates of sex, and time in months since release. Individual ID was used as a random effect.
All models were simplified using backwards stepwise elimination, based on changes to AIC values (Burnham and Anderson, 2002). If dropping a given term did not increase the AIC by more than 2 points, then the term was excluded from the final model. Model selection was then confirmed by comparing Akaike weights between models with the MuMIn package (Barton, 2020).
Results
What Predicts the Occurrence of Vigilance?
‘Alalā displayed some level of vigilance toward aerial threats in 87.6% of observations, but were not equally likely to be vigilant in all situations. Individual ‘alalā were less likely to be vigilant as group size increased at the food platforms (CLMM, N = 1,320, coef = −0.32 ± 0.1, z = −3.16; Figure 2). Vigilance slowly and slightly increased the longer the birds spent in the wild (coef = 0.06 ± 0.02, z = 3.08; Figure 3). Also, regardless of whether they used their beak or foot to open the feeder, ‘alalā were less likely to be vigilant when interacting with the feeder (beak, coef = −0.59 ± 0.17, z = −3.42; foot, coef = −0.83 ± 0.23, z = −3.64), but there was no relationship between whether or not they were seen eating and their vigilance levels. Birds who took flight during videos were less likely to be vigilant (coef = −0.60 ± 0.23, z = −2.57), meaning that birds were less likely to be vigilant immediately before leaving the feeder. Finally, sex had no effect on vigilance (Table 2).
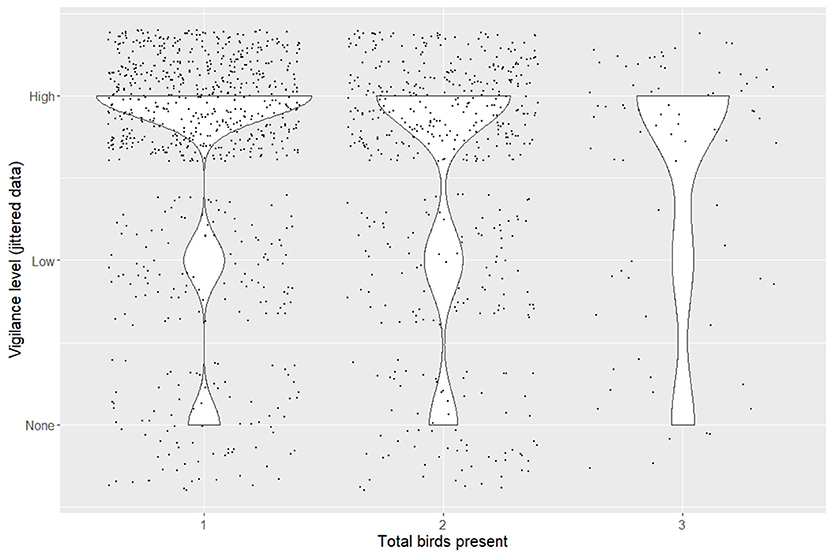
Figure 2. Ordinal levels of vigilance depending upon the number of birds present. Violin plots and raw data, jittered around their category, using the geom_jitter function from the ggplot2 package, are included to illustrate the distribution of the data between vigilance categories. The total number of birds present represent 1, 2, and 3 or more individuals. In videos with more birds present, vigilance levels were less skewed toward the highest category of vigilance (2).
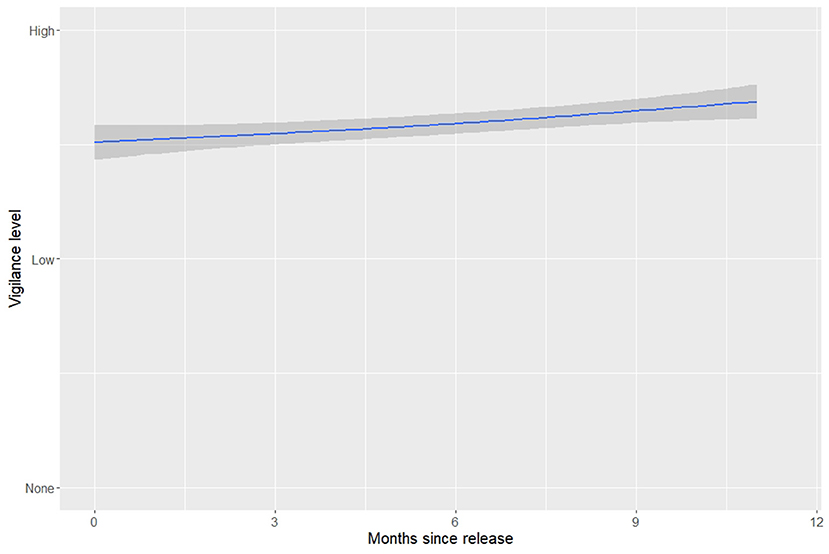
Figure 3. Likelihood of vigilance based on months elapsed since release. Vigilance was a ordinal variable with a range of 0–2. The shaded region shows the standard error around the raw data mean for each month since release.
What Predicts Eating?
The more birds present on the food platforms, the less likely any individual ate (GLMM, n = 1,320, B = −0.49 ± 0.10, z = −4.73). Also, focal individuals were less likely to be observed eating if they were seen taking flight during the video (B = −1.09 ± 0.22, z = −5.03). ‘Alalā were more likely to be seen eating if they were interacting with the feeder (B = 0.37 ± 0.18, z = 2.06). Sex, and time since release had no effect on the likelihood that birds ate during the videos (Table 3).
What Influences Scrounging?
The number of birds present on the food platform did not influence the likelihood that birds would scrounge from the platform rather than eat from the feeder (Table 4). However, birds became increasingly likely to scrounge as the months passed since release (GLMM, n = 982, B = 0.15 ± 0.02, z = 6.76). Sex also had no effect on the likelihood that birds would scrounge.
Discussion
Supplemental feeding can provide translocated individuals with additional food resources in a novel environment, but it may come at the cost of an increased risk of predation. We studied ‘alalā antipredator vigilance, eating behavior, and group size over time to assess the potential benefits and risks of supplemental feeding. Overall, the ‘alalā were often vigilant at the feeding stations, with 87.6% of observations showing some form of scanning behavior. However, the birds' vigilance levels depended on a number of factors. Individual likelihood of vigilance decreased with group size, but increased over time since release. The supplemental feeding setup also added potential costs as birds showed decreased vigilance while using the feeder, but ‘alalā appear to have several feeding strategies which can offset these costs, such as scrounging. We discuss the relative costs and benefits of supplemental feeding that we documented, in the context of potential release strategies, as well as changes through time that may inform when to adjust supplemental feeding.
Releasing larger groups of birds has the potential to offer anti-predator benefits if birds are more likely to share vigilance duties among a group and have greater opportunities to feed when together. In ‘alalā we found that group size was linked to both vigilance and eating, but not in a fully advantageous way. Individual ‘alalā were less vigilant as the group size increased, consistent with a large body of literature (Dlaz and Asensio, 1991; Henderson and Hart, 1991; Ward and Low, 1997; Rolando et al., 2001). However, ‘alalā also showed a decrease in the likelihood of eating as the group size increased, suggesting that while there may have been anti-predator benefits, foraging together also posed energy intake costs. This is a departure from the general assumption that individuals will spend more time feeding if they are spending less time scanning for threats (Henderson and Hart, 1991; Saino, 1994), and may be due to other factors such as competition (Saino, 1994). However, perhaps feeding more efficiently, and safely, over shorter time periods, could negate any energy intake lost due to conspecific distraction.
Alternatively, the feeder setup, not social distraction, limited birds' ability to eat in groups. Many factors must be considered when designing bird feeding stations, such as: limiting heterospecific foraging, making food accessible to the target species, and preventing food from spoiling. To reduce these potential side effects, ‘alalā were trained to open custom plastic feeders at the feeding stations. While this prevented other bird species from foraging on the food, it limited the number of ‘alalā that could access the feeder at once. When more dominant birds feed from the feeder, others would have to wait for access. Thus, there was little competitive advantage to eating efficiently for dominant birds in this scenario. The design of the supplemental feeding stations also likely influenced the ability or motivation of birds to engage in anti-predator behavior. Birds that were seen interacting with the feeder displayed less vigilance. Manipulating the lid required diverting attention away from potential threats and the lid obscured the surrounding view (see video in Supplementary Material). There is the potential that the feeder could serve as an ecological trap, if it increases their susceptibility to predation (Robb et al., 2008; Robertson et al., 2013). However, all 11 birds survived the 12-month study period, despite the persistent presence of ‘io in the surrounding forest (Greggor et al., 2021). Therefore, while the feeder setup did not appear detrimental during the initial year of release, future designs may want to consider ways of allowing vigilance while accessing food, and facilitating safe scrounging, to reduce the potential costs of the feeder.
Despite the relatively stable costs and benefits of using the feeders during the study period, the vigilance behavior of ‘alalā increased slowly over the 1st year post-release. Since the released ‘alalā had been raised in human care, their greater vigilance over time may signify an adjustment to the wild and its predators. Such behavioral acclimatization periods are common in translocations (Shier, 2016), and in other species, being able to learn post-release has been shown to help boost survival (Krochmal et al., 2018). The increase in vigilance we documented does not mirror a classic learning curve, which suggests that ‘alalā were not entirely naïve or defenseless upon release and may only have needed to fine-tune their anti-predator behavior over time. In tandem to increases in vigilance, we also saw a greater reliance on scrounging behavior over time. By foraging on food scraps discarded from the feeder, birds may have learned to avoid the vigilance costs imposed by sticking their head into the feeder. These two behaviors together suggest that the management strategy of releasing juveniles ahead of sexual maturity and territorial conflict may have allowed for a period of relative safety in numbers while birds learned new strategies, which helped the cohort survive and adjust. However, the relative costs and benefits we documented of supplemental feeding likely change as birds mature and become territorial, around 3–4 years of age (Banko et al., 2002). As conspecific aggression becomes more prevalent, group feeding may require additional vigilance directed toward conspecifics rather than predators, or it may not be tolerated by dominant individuals. Older birds would be expected to favor solitary or pair feeding to offset these social costs, which could make supplemental feeders more dangerous. Therefore, the feeding strategies that work early in a release process may not always be ideal in later stages.
A common criterion employed for withdrawing supplemental food is evidence for reduced reliance on this food resource as the reintroduced animals learn to exploit natural food resources. While we could not quantify the volume of food consumed in each foraging bout, our findings indicate that there was no change over time in the likelihood ‘alalā would be seen eating at the feeding platforms when caught on camera. Despite their static foraging likelihood, spatial analyses suggest they spent increasing time away from the feeders (Smetzer et al., 2021) and unpublished data have documented ‘alalā foraging on a diversity of native fruits and “bark flaking” to obtain insect prey (Masuda et al.). Therefore, it is yet unclear whether ‘alalā had preferences for supplemental food, or simply continued to exploit its ready availability. While the 1 year period of this study exceeds the supplemental feeding duration of many translocation programs, in some programs long-term food supplementation for >1 year has been shown to be beneficial (Chauvenet et al., 2012; White et al., 2012). Future research is required to determine whether birds' wild foraging ability was compromised, wild foods were insufficient, or some other factor was involved in the continued reliance on provisioned food for reintroduced ‘alalā. Comparisons of vigilance levels during wild-type foraging to those seen at the supplemental feedings will also be useful for determining whether the relative perceived risks differed between food types.
Supplemental feeding is used in many translocation projects (Boutin, 1990; Ruiz-Miranda and Swaisgood, 2019), but the costs of offering supplemental food are less often considered than the potential benefits (Ferrer et al., 2018). Our results highlight how evaluating anti-predator vigilance, foraging, and social environment can illuminate translocation outcomes in the supplemental feeding context. While we cannot ascertain from our data how supplemental feeding affects predation and survival, we can learn how recently released birds use their behavior to adapt to the potential risks of feeding at supplemental food stations, and how their behavioral choices may offset costs and change through time as they gain knowledge of relative risks of feeding at concentrated food sources.
In applying these results to other species or systems, there are several factors that need to be considered which may influence responses to supplemental food. Specifically, social dynamics and conspecific tolerance will determine whether and when larger group size is a positive or negative influence. In the early stages of a translocation program before sufficient data on predation and mortality are available, animals' choice of when to remain vigilant vs. when to feed can be informative, and perhaps predictive. ‘Alalā can remain vigilant, interrupting feeding to scan for potential predators, and adjust their level of vigilance adaptively in a manner suggesting they rely on conspecifics to detect predators while feeding. These are promising signs indicative of threat-sensitive anti-predator behavior. If such vigilance and adjustments to situational changes in risk are not observed in translocated animals, this may be an early warning sign that their behavior is maladaptive. Further, animals' behavior should change over time following release as they learn and adapt to novel circumstances (Berger-Tal and Saltz, 2014); if these temporal changes in behavior patterns are not observed, this may indicate that the translocated animal's ability to learn is deficient. Because predation—and anti-predator behavior—varies as a function of habitat features that influence detection by predators or escape options (Lima and Bednekoff, 1999; Valeix et al., 2009; Cooper and Blumstein, 2015), microhabitat should be carefully considered when locating supplemental feeders. Nearby cover may make escape options available or provide hiding places for predators, depending on the nature of the predator-prey relationship. If feeders are placed in areas that are too risky, released animals may become prey or may become so vigilant that they are not able to effectively utilize the feeder. An animal's vigilance behavior may be a sensitive assay of the risk associated with varying habitat features, increasing in riskier environment. Thus, monitoring how anti-predator vigilance varies with habitat type could help inform site selection for supplemental feeders. Due to its sensitivity and close relationship with potential fitness impacts, anti-predator vigilance is a particularly promising behavior to use as an indicator for behavior-based management (Berger-Tal et al., 2011). This case study with ‘alalā underscores the value of measuring behavior as part of post-release monitoring to inform evidence-based conservation management and improve translocation outcomes.
Data Availability Statement
The data and R code used for the original contributions presented in the study are included in the article/Supplementary Material, further inquiries can be directed to the corresponding author.
Ethics Statement
The animal study was reviewed and approved by San Diego Zoo Wildlife Alliance's IACUC (NIH A3675-01, USDA 93-R-0151, project 16-009). Permits for the release and subsequent monitoring of ‘alalā include a Threatened and Endangered Species Recovery permit (#TE060179-6) an MBTA permit (#MB09204C-1), State of Hawaii Natural Area Reserves Special Use Permit (#I1296), and a BBL permit (#24020) for capture, handling, holding, banding, and auxiliary marking of ‘alalā.
Author Contributions
AG, BM, and HL designed the study. HL collected video behavioral data. AG conducted the statistical analysis. HL and AG wrote the manuscript, with input from all authors. BM and RS secured funding. All authors contributed to the article and approved the submitted version.
Funding
This research was supported by the Pacific Internship Programs for Exploring Science (PIPES) through funding from the National Science Foundation Research Experiences for Undergraduates (DEB 1757875). Funding for the ‘alalā release and monitoring during data collection for this study was provided by the National Fish and Wildlife Foundation, Moore Family Foundation, Max and Yetta Karasik Foundation, anonymous donors, and San Diego Zoo Wildlife Alliance. Funding for ‘alalā conservation breeding efforts was provided by the U.S. Fish and Wildlife Service, Hawaii Division of Forestry and Wildlife, anonymous donors, and San Diego Zoo Wildlife Alliance.
Conflict of Interest
The authors declare that the research was conducted in the absence of any commercial or financial relationships that could be construed as a potential conflict of interest.
Publisher's Note
All claims expressed in this article are solely those of the authors and do not necessarily represent those of their affiliated organizations, or those of the publisher, the editors and the reviewers. Any product that may be evaluated in this article, or claim that may be made by its manufacturer, is not guaranteed or endorsed by the publisher.
Acknowledgments
We would like to thank Amy Durham and Kawai Navares for help with video coding and discussing ethogram development. We also thank the dedicated ‘alalā field team for swapping, maintaining and archiving trail camera data, alongside general bird care. Finally, we thank the many members of the ‘alalā working group, without whom, the ‘alalā would not be alive today.
Supplementary Material
The Supplementary Material for this article can be found online at: https://www.frontiersin.org/articles/10.3389/fcosc.2021.701490/full#supplementary-material
Table S1. All R code used for analysis and plot creation in Lee et al., 2021.
Video S1. Video illustrating the field of view obstructed by feeder usage. Camera angles used for video analysis are reflected in Figure 1.
Datasheet 1. All data used for analysis in Lee et al., 2021.
References
Banko, P. C., Ball, D. L., and Banko, W. E. (2002). “Hawaiian Crow (Corvus hawaiiensis),” in Birds of North America, eds A. Poole and F. Gill (Philedelphia, PA: The Birds of North America, Inc.), 1–28.
Barnard, C. J., and Sibly, R. M. (1981). Producers and scroungers: a general model and its application to captive flocks of house sparrows. Anim. Behav. 29, 543–550. doi: 10.1016/S0003-3472(81)80117-0
Barton, K. (2020). MuMIn: Multi-Model Inference. R package version 1.43.17. Available online at: https://CRAN.R-project.org/package=MuMIn
Beauchamp, G. (2015). Animal Vigilance: Monitoring Predators and Competitiors. 1st ed. Amsterdam: Academic Press.
Berger-Tal, O., Blumstein, D. T., and Swaisgood, R. R. (2020). Conservation translocations: a review of common difficulties and promising directions. Anim. Conserv. 23, 121–131. doi: 10.1111/acv.12534
Berger-Tal, O., Polak, T., Oron, A., Lubin, Y., Kotler, B. P., and Saltz, D. (2011). Integrating animal behavior and conservation biology: a conceptual framework. Behav. Ecol. 22, 236–239. doi: 10.1093/beheco/arq224
Berger-Tal, O., and Saltz, D. (2014). Using the movement patterns of reintroduced animals to improve reintroduction success. Curr. Zool. 60, 515–526. doi: 10.1093/czoolo/60.4.515
Boutin, S. (1990). Food supplementation experiments with terrestrial vertebrates: patterns, problems, and the future. Can. J. Zool. 68, 203–220. doi: 10.1139/z90-031
Brown, J. S., Laundr,é, J. W., and Gurung, M. (1999). The ecology of fear: optimal foraging, game theory, and trophic interactions. J. Mammal. 80, 385–399. doi: 10.2307/1383287
Burnham, K. P., and Anderson, D. R. (2002). Model Selection and Multimodal Inference: A Practical Information-Theoretic Approach. New York, NY: Springer-Verlag.
Carter, A. J., Pays, O., and Goldizen, A. W. (2009). Individual variation in the relationship between vigilance and group size in eastern grey kangaroos. Behav. Ecol. Sociobiol. 64, 237–245. doi: 10.1007/s00265-009-0840-4
Carthey, A. J. R., and Blumstein, D. T. (2018). Predicting predator recognition in a changing world. Trends Ecol. Evol. 33, 106–115. doi: 10.1016/j.tree.2017.10.009
Chauvenet, A. L. M., Ewen, J. G., Armstrong, D. P., Coulson, T., Blackburn, T. M., Adams, L., et al. (2012). Does supplemental feeding affect the viability of translocated populations? The example of the hihi. Anim. Conserv. 15, 337–350. doi: 10.1111/j.1469-1795.2012.00522.x
Christensen, R. H. B. (2019). Ordinal: Regression Models for Ordinal Data. Available online at: https://cran.r-project.org/package=ordinal
Colagross, A. M. L., and Cockburn, A. (1993). Vigilance and grouping in the eastern grey kangaroo, macropus giganteus. Aust. J. Zool. 41, 325–334. doi: 10.1071/ZO9930325
Cooper, W. E. J., and Blumstein, D. T. (2015). Escaping From Predators: An Intergrated View of Escape Decisions. Cambridge: Cambridge University Press.
Crane, A. L., Lampe, M. J., and Mathis, A. (2015). Maladaptive behavioural phenotypes in captive-reared darters (Etheostoma caeruleum, Storer 1845). J. Appl. Ichthyol. 31, 787–792. doi: 10.1111/jai.12769
Culliney, S., Pejchar, L., Switzer, R., and Ruiz-Gutierrez, V. (2012). Seed dispersal by a captive corvid: the role of the ‘Alala (Corvus hawaiiensis) in shaping Hawai'i's plant communities. Ecol. Appl. 22, 1718–1732. doi: 10.1890/11-1613.1
Dlaz, J. A., and Asensio, B. (1991). Effects of group size and distance to protective cover on the vigilance behaviour of black-billed magpies pica pica. Bird Study 38, 38–41. doi: 10.1080/00063659109477064
Ferrer, M., Morandini, V., Baguena, G., and Newton, I. (2018). Reintroducing endangered raptors: a case study of supplementary feeding and removal of nestlings from wild populations. J. Appl. Ecol. 55, 1360–1367. doi: 10.1111/1365-2664.13014
Greggor, A. L., Masuda, B., Gaudioso-Levita, J. M., Nelson, J. T., White, T. H., Shier, D. M., et al. (2021). Pre-release training, predator interactions and evidence for persistence of anti-predator behavior in reintroduced ‘alalā, Hawaiian crow. Glob. Ecol. Conserv. 28:e01658. doi: 10.1016/j.gecco.2021.e01658
Henderson, I. G., and Hart, P. J. B. (1991). Age-specific differences in the winter foraging strategies of rooks Corvus frugilegus. Oecologia 85, 492–497. doi: 10.1007/BF00323760
Keuhler, C., Harrity, P., Lieberman, A., and Kuhn, M. (1995). Reintroduction of hand-reared alala corvus hawaiiensis in hawaii. Oryx 29, 261–266. doi: 10.1017/S0030605300021256
Krochmal, A. R., Roth, T. C., and O'Malley, H. (2018). An empirical test of the role of learning in translocation. Anim. Conserv. 21, 36–44. doi: 10.1111/acv.12357
Lima, S. L. (1987). Vigilance while feeding and its relation to the risk of predation. J. Theor. Biol. 124, 303–316. doi: 10.1016/S0022-5193(87)80118-2
Lima, S. L., and Bednekoff, P. A. (1999). Temporal variation in danger drives antipredator behavior: the predation risk allocation hypothesis. Am. Nat. 153, 649–659. doi: 10.1086/303202
Lima, S. L., and Dill, L. M. (1990). Behavioral decisions made under the risk of predation: a review and prospectus. Can. J. Zool. 68, 619–640. doi: 10.1139/z90-092
Liu, B., Li, L., Lloyd, H., Xia, C., Zhang, Y., and Zheng, G. (2016). Comparing post-release survival and habitat use by captive-bred Cabot's Tragopan (Tragopan caboti) in an experimental test of soft-release reintroduction strategies. Avian Res. 7, 1–9. doi: 10.1186/s40657-016-0053-2
Lockwood, M. A., Griffin, C. P., Morrow, M. E., Randel, C. J., and Silvy, N. J. (2005). Survival, movements, and reproduction of released captive-reared Attwater's prairie-chicken. J. Wildl. Manage. 69, 1251–1258. doi: 10.2193/0022-541X(2005)069[1251:SMAROR.0.CO;2]10.2193/0022-541X(2005)069[1251:SMAROR]2.0.CO;2
McPhee, M. E., and Carlstead, K. (2010). “The importance of maintaining natural behaviors in captive mammals,” in Wild Mammals in Captivity: Principles and Techniques for Zoo Management, eds D. G. Klieman and K. V. Thompson (Chicago: University of Chicago Press), 303–313.
Mertes, K., Stabach, J. A., Songer, M., Wacher, T., Newby, J., Chuven, J., et al. (2019). Management background and release conditions structure post-release movements in reintroduced ungulates. Front. Ecol. Evol. 7:470. doi: 10.3389/fevo.2019.00470
Quenette, P.-Y. (1990). Functions of vigilance behaviour in mammals: a review. Acta Oecologica 11, 801–818.
R Core Team (2019). R: A Language and Environment for Statistical Computing. Vienna: R Foundation for Statistical Computing.
Robb, G. N., McDonald, R. A., Chamberlain, D. E., and Bearhop, S. (2008). Food for thought: supplementary feeding as a driver of ecological change in avian populations. Front. Ecol. Environ. 6:60152. doi: 10.1890/060152
Roberts, G. (1996). Why individual vigilance declines as group size increases. Anim. Behav. 51, 1077–1086. doi: 10.1006/anbe.1996.0109
Robertson, B. A., Rehage, J. S., and Sih, A. (2013). Ecological novelty and the emergence of evolutionary traps. Trends Ecol. Evol. 28, 552–560. doi: 10.1016/j.tree.2013.04.004
Robinette, R. L., and Ha, J. C. (2001). Social and ecological factors influencing vigilance by northwestern crows, corvus caurinus. Anim. Behav. 62, 447–452. doi: 10.1006/anbe.2001.1772
Rodriguez-Hidalgo, P., Gortazar, C., Tortosa, F. S., Rodriguez-Vigal, C., Fierro, Y., and Vicente, J. (2010). Effects of density, climate, and supplementary forage on body mass and pregnancy rates of female red deer in Spain. Oecologia 164, 389–398. doi: 10.1007/s00442-010-1663-8
Rolando, A., Caldoni, R., De Sanctis, A., and Laiolo, P. (2001). Vigilance and neighbour distance in foraging flocks of red-billed choughs, Pyrrhocorax pyrrhocorax. J. Zool. 253, 225–232. doi: 10.1017/S095283690100019X
Ruiz-Miranda, C., and Swaisgood, R. R. (2019). “Moving animals in the right direction: making conservation translocation an effective tool,” in International Wildlife: Contemporary Challenges in a Changing World, eds J. L. Koprowski and P. R. Krausman (Baltimore, MD: John Hopkins University Press), 141–156.
Saino, N. (1994). Time budget variation in relation to flock size in carrion crows, Corvus corone corone. Anim. Behav. 47, 1189–1196. doi: 10.1006/anbe.1994.1157
Shier, D. (2016). “Manipulating animal behavior to ensure reintroduction success,” in Conservation behavior: applying behavioral ecology to wildlife conservation and management, eds O. Berger-Tal and D. Saltz (Cambridge: Cambridge University Press), 275–304. doi: 10.1017/CBO9781139627078.014
Smetzer, J.R., Greggor, A.L., Paxton, K.L., Masuda, B., and Paxton, E.P. (2021). Automated telemetry reveals post-reintroduction exploratory behavior and movement patterns of an endangered corvid, ‘Alalā (Corvus hawaiiensis) in Hawai‘i, USA. Global Ecol. Conserv. 26:e01522. doi: 10.1016/j.gecco.2021.e01522
Sorensen, A., van Beest, F. M., and Brook, R. K. (2014). Impacts of wildlife baiting and supplemental feeding on infectious disease transmission risk: a synthesis of knowledge. Prev. Vet. Med. 113, 356–363. doi: 10.1016/j.prevetmed.2013.11.010
Treves, A. (2000). Theory and method in studies of vigilance and aggregation. Anim. Behav. 60, 711–722. doi: 10.1006/anbe.2000.1528
U. S Fish Wildlife Service (2009). Revised Recovery Plan for the ‘Alala (Corvus hawaiiensis). Portland: Oregon.
Valeix, M., Loveridge, A. J., Chamaillé-Jammes, S., Davidson, Z., Murindagomo, F., Fritz, H., et al. (2009). Behavioral adjustments of African herbivores to predation risk by lions: spatiotemporal variations influence habitat use. Ecology 90, 23–30. doi: 10.1890/08-0606.1
Ward, C., and Low, B. S. (1997). Predictors of vigilance for American Crows foraging in an urban environment. Wilson Bull. 109, 481–489.
Keywords: corvid, conservation behavior, group size effect, post-release monitoring, translocation
Citation: Lee HN, Greggor AL, Masuda B and Swaisgood RR (2021) Anti-Predator Vigilance as an Indicator of the Costs and Benefits of Supplemental Feeding in Newly Released ‘Alalā (Corvus hawaiiensis). Front. Conserv. Sci. 2:701490. doi: 10.3389/fcosc.2021.701490
Received: 28 April 2021; Accepted: 06 July 2021;
Published: 05 August 2021.
Edited by:
Philip J. Seddon, University of Otago, New ZealandReviewed by:
Christine Steiner Sao Bernardo, Instituto Ecótono (IEco), BrazilHuw Lloyd, Manchester Metropolitan University, United Kingdom
Copyright © 2021 Lee, Greggor, Masuda and Swaisgood. This is an open-access article distributed under the terms of the Creative Commons Attribution License (CC BY). The use, distribution or reproduction in other forums is permitted, provided the original author(s) and the copyright owner(s) are credited and that the original publication in this journal is cited, in accordance with accepted academic practice. No use, distribution or reproduction is permitted which does not comply with these terms.
*Correspondence: Alison L. Greggor, YWdyZWdnb3JAc2R6d2Eub3Jn